- 1Key Laboratory of Engineering Oceanography, Second Institute of Oceanography, Ministry of Natural Resources, Hangzhou, China
- 2Key Laboratory of Nearshore Engineering Environment and Ecological Security of Zhejiang Province, Second Institute of Oceanography, Ministry of Natural Resources, Hangzhou, China
- 3School of Life Sciences, Fudan University, Shanghai, China
- 4Department of Biogeochemical Processes, Max Planck Institute for Biogeochemistry, Jena, Germany
- 5Wetland Ecosystem Research Station of Hangzhou Bay, Research Institute of Subtropical Forestry, Chinese Academy of Forestry, Hangzhou, China
- 6State Key Laboratory of Herbage Improvement and Grassland Agro-ecosystems, College of Pastoral Agriculture Science and Technology, Lanzhou University, Lanzhou, China
The spatial pattern of leaf stoichiometry is critical in predicting plant palatability and ecosystem productivity and nutrient cycling rates and thus is a major focus of community ecological research. Coastal wetlands as vital blue carbon ecosystems, with high possibility to be vulnerable to plant invasion, studies focused on stoichiometry and its pattern are important to unveil the elements cycling process. However, previous studies have mainly focused on stoichiometry in terrestrial ecosystems, there are few studies conducted on coastal wetland ecosystems, especially the studies that compare leaf stoichiometry between native and non-native plants in coastal wetlands. In this study, we compared the latitudinal patterns of leaf nutrient contents and their stoichiometric ratios between native and non-native plant species across coastal wetland ecosystems and investigated whether leaf stoichiometric patterns were driven by climatic factors. We used a compiled global data set of 954 records to conduct a systematic meta-analysis. The results showed that there were significant differences in latitudinal patterns of leaf carbon (C) and nitrogen (N) contents and C:N ratio between native and non-native species, as well as significant differences in leaf C, N, and phosphorus (P) contents. For native species, we found significant latitudinal patterns in leaf C, N, and P contents and C:N and C:P ratios, whereas for non-native species, we found significant latitudinal patterns in leaf N content and C:P and N:P ratios. Mean annual temperature of the data collection site was a significant predictor of leaf stoichiometry of native plants but only of leaf N content and C:P ratio of non-native plants. Thus, we demonstrated spatial heterogeneity in leaf stoichiometries between native and non-native plants in coastal wetlands, indicating that such differences should be emphasized in future biogeochemical models and plant-herbivore interaction studies owing to the important role of wetland plants in global C, N, and P cycles. Our findings increase understanding of plant-related nutrient and elements cycling in coastal wetlands, as well as improve predictions of plant growth rates and vegetation productivity across large scales under plant invasion scenarios.
Introduction
Latitudinal gradients in biotic interactions are a classic paradigm in ecology (Kinlock et al., 2018; Zvereva and Kozlov, 2022). The spatial heterogeneity of both biotic and abiotic factors is largely responsible for latitudinal gradients in plant traits (Abdala-Roberts et al., 2016; Reese et al., 2016; Moreira et al., 2018). For example, plant defensive traits are greater at low latitudes than at high latitudes, mainly due to stronger biotic interactions at low latitudes (Anstett et al., 2014). However, the cost of resource acquisition may also be a key driver of latitudinal variation in plant defenses (Kooyers et al., 2017). Leaf stoichiometry (i.e., leaf carbon (C), nitrogen (N), and phosphorus (P) contents) is also one of the most important plant traits and is an effective predictor of plant palatability, ecosystem function and nutrient cycling rates, and role of plants in global biogeochemical models (Kattge et al., 2009; Chen et al., 2013; Koerselman and Meuleman, 1996; Elser et al., 2003; Güsewell, 2004; Joshi and Vrieling, 2005; Kerkhoff et al., 2006; Leishman et al., 2007; Kattge et al., 2009; Li et al., 2009; Ehrenfeld, 2010; Huang et al., 2010; Kurokawa et al., 2010; Kitajima et al., 2012; Funk, 2013; Funk and Cornwell, 2013; Heberling and Fridley, 2013; Cronin et al., 2015; Funk et al., 2017; Ju et al., 2017; Kooyers et al., 2017; Kinlock et al., 2018; Fox and Weisberg, 2019; Ju et al., 2019; Guo et al., 2020; Harvey and Leffler, 2020; Liu et al., 2020; Hu et al., 2021; Guo et al., 2023; Guo et al., 2024; Liu et al., 2021; Zhang et al., 2021). However, we lack a sufficiently comprehensive research on latitudinal patterns of leaf stoichiometry.
Leaf C is a major component of carbohydrates, representing starch reserves and CO2 fixation capacity (Rogers et al., 2004), and it is also strongly correlated with cellulose and hemicellulose contents (Kitajima et al., 2012). Leaf N is a major component of amino acids and proteins, and thus, high leaf N indicates high nutrient levels, which lead to high decomposition rates and palatability to herbivores (Schädler et al., 2003; Kurokawa et al., 2010). In addition, many secondary metabolites are also nitrogenous compounds, which can have major effects on plant–insect interactions (Waller, 2012). Leaf P is an important component of nucleotides in genetic materials (e.g., DNA) and energy-transfer compounds (e.g., ATP) (Ågren, 2008; Guo et al., 2020). The leaf stoichiometric ratios of these critical nutrients (i.e., C:N, C:P, and N:P) have been used to indicate ecosystem nutrient limitation (Koerselman and Meuleman, 1996; Güsewell, 2004). Thus, studies of latitudinal patterns in leaf nutrient contents and stoichiometric ratios can significantly improve our understanding of ecosystem processes, including productivity, detritivory and decomposition, herbivory, and energy flow (Güsewell, 2004; Banks and Frost, 2017; Zhang et al., 2021; Guo et al., 2023).
Native and non-native plants have different resource allocation strategies; for example, non-native plants can grow faster and use resources more efficiently than native plants (Huang et al., 2010; Heberling and Fridley, 2013). Because of the differences in strategies, there may be large differences in leaf stoichiometry between non-native and native plants, which can lead to greater competitive capacity of non-natives (Harvey and Leffler, 2020; Zhu et al., 2020). Plant invasions by non-natives are an important component of anthropogenic global climate change and are considered one of the most serious environmental problems (Sala et al., 2000; Vilà et al., 2007; Bradley et al., 2010). At the scale of communities and ecosystems, plant invasions can threaten native community species richness and diversity and alter ecosystem C and N cycling and affect ecosystem structure and function (Ehrenfeld, 2010; Vilà et al., 2011; Pyšek et al., 2012). Therefore, we consider plant stoichiometry to be a useful functional trait for explaining and predicting the status of plant invasions at large scales. However, the differences in biogeographic patterns of leaf stoichiometry between native and non-native plants have not been systematically studied.
Coastal wetland ecosystems are currently one of the most fragile and sensitive ecosystems worldwide and are severely threatened and damaged by invasive plants (Li et al., 2009). In China, for example, coastal salt marshes have suffered from serious plant invasions, and non-native Spartina alterniflora has taken over all coastal wetlands in the country (Ju et al., 2017; Xu et al., 2022; Ning et al., 2024). Similarly, over the past century, the invasive plant Phragmites australis has steadily invaded the coastal salt marshes of North America, causing severe damage to native plant communities (Silliman and Bertness, 2004; Minchinton et al., 2006; Ning et al., 2021). The few empirical studies that investigated the leaf stoichiometry of salt marsh plants found significant latitudinal differences in leaf stoichiometry patterns between exotic and native plants (Cronin et al., 2015; Zhang et al., 2021). However, previous studies are all limited to a few model species, and the results may not be generalizable across species. Therefore, a systematic cross-species comparative study is needed on the spatial patterns of leaf nutrient contents and stoichiometric ratios of native and non-native plants across global coastal wetland ecosystems.
Here, we report a meta-analysis of the spatial patterns of leaf stoichiometry between native and non-native plants in coastal wetlands. Plants of the same identity in the same latitude face similar environmental factors, which may result in co-occurring plants with similar ecological strategies (Hu et al., 2021; Guo et al., 2024). This provides an opportunity to conduct cross-species spatial analyses of leaf stoichiometry. In this study, we integrated a data set of leaf stoichiometry from global coastal wetland ecosystems for cross-species ecological modelling. The effect of temperature on leaf stoichiometry was analyzed using linear regression. We hypothesized the following: (1) there are significant differences in leaf stoichiometry between native and non-native plants in coastal wetlands; (2) there are significant differences in spatial patterns of leaf stoichiometry between native and non-native plants in coastal wetlands; and (3) any differences between native and non-native plants are primarily related to differential responses to climatic factors (mainly temperature). We aim to increase understanding of patterns in two kinds of plant leaf stoichiometries and nutrient cycling in coastal wetlands, as well as improve predictions of biogeochemical models across large scales.
Materials and methods
Data collection
We first used a comprehensive dataset of leaf stoichiometry for coastal wetland plants containing six leaf stoichiometric traits (C, N, P, C:N, C:P and N:P) with 698 data points from 205 sites (Hu et al., 2021). This dataset was primarily collected from typical coastal wetlands (including tidal salt marshes and mangroves), and excluded studies of seedlings. This dataset also excluded data from significant disturbances and experimental treatments (e.g., grazing and reclamation), as well as data from greenhouses and common gardens (Hu et al., 2021). We then added some additional studies from recent years with data based on salt marshes on the east coast of China (Xu et al., 2020; Liu et al., 2020). In total, the data set contained 954 records. For all data, we also recorded the name of the plant species and the original location of the data. We used temperature as the climatic factor in the study and extracted mean annual temperature using recorded site information at worldclim.org. We did not include precipitation as a climatic factor in this study, because the focus was on salt marsh ecosystems, which have almost regular tides and are mostly less water-limited. We also determined plant identity (native or non-native) for all plant species in the data set using Plants of the World Online (powo.science.kew.org). In the end, our dataset contained 740 data points for native plants and 214 for non-native plants (Figure 1).
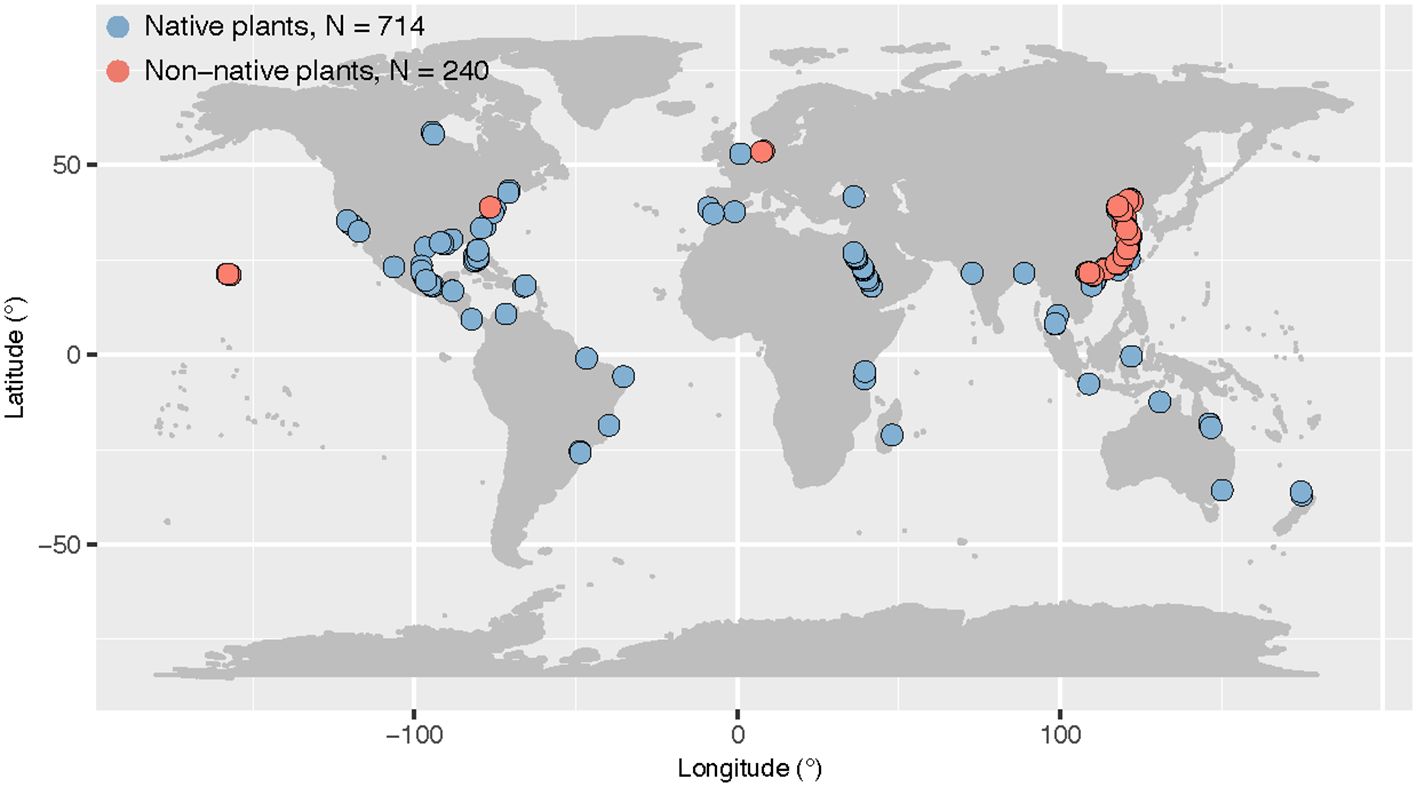
Figure 1 Map of original study sites of native and non-native plant leaf stoichiometry across global coastal wetlands. Several studies that only provided latitude without longitude were not included in the map. The sample size (N) was reported.
Statistical analyses
As the sample size (N) of the six leaf stoichiometric traits is inconsistent, we performed separate analyses for the six leaf stoichiometric traits in subsequent data analyses. We used the average of the values of leaf element contents (C, N and P) and their ratios (C:N, C:P and N:P) for each species within each site. We then tested the normality of the distributions for these plant characteristics and found that none followed a normal distribution. To normalize the distribution of residuals, all leaf characteristics were log10-transformed to meet the assumption of normality. To determine whether leaf stoichiometries were affected by plant identity (native and non-native) and latitude, we used the lme4 package for linear mixed models (LMMs) to analyze each leaf stoichiometric character (C, N, P, C:N, C:P and N:P). LMMs could accurately assess the effects of different factors on the response variables by including interactions (Bolker et al., 2009). Each model included plant identity, latitude, and their interaction as fixed effects and plant species as a random effect (Bates et al., 2015). Inclusion of plant species as a random effect to separately consider correlations for the same plant species and to account for these interdependencies when fitting the model. The significance of fixed effects in a model was assessed by the Type II Wald chi-squared test using the CAR package (Fox and Weisberg, 2019). To further compare differences in latitudinal patterns of leaf stoichiometry between native and non-native plants, linear regression was used to fit the relations between latitude and leaf stoichiometry for each plant type. To investigate the response of leaf stoichiometry to mean annual temperature (MAT), we used linear regression to fit the relations between MAT and leaf stoichiometry for each plant type. All analyses were performed using R v3.6.2 (R Core Team, 2019).
Results
Effects of plant identity and latitude on leaf stoichiometry
In the LMM models, the effect of plant identity was significant for leaf C, N, and P (Table 1), indicating significant differences in leaf stoichiometry between native and non-native plants in coastal wetlands. Latitude was significant for leaf C and N and C:N ratio, indicating significant relations of those variables with latitude (Table 1). Plant identity × latitude interactions were significant for leaf C and C:N ratio, indicating significant latitudinal differences in the relations of the two characteristics between native and non-native plants.
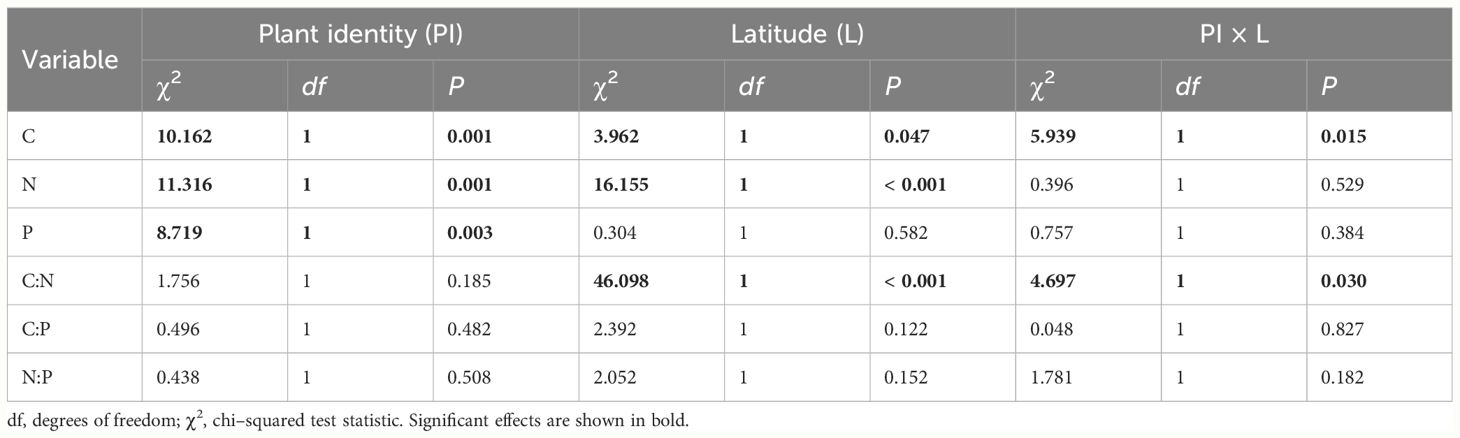
Table 1 Linear mixed models of the effects of plant identity (PI, native or non-native), latitude (L), and their interaction on leaf stoichiometry in global coastal wetlands.
In separate analyses of the relations between leaf stoichiometry and latitude for native and non-native plants, leaf C, N, and P and C:N and C:P ratios of native plant species were significantly correlated with latitude (Figure 2), with leaf C and C:N and C:P ratios decreasing with increasing latitude and leaf N and P increasing. For non-native plants, leaf N and C:P and N:P ratios were significantly related with latitude (Figure 2), with leaf N and N:P ratio increasing with increasing latitude and leaf C:P ratio decreasing.
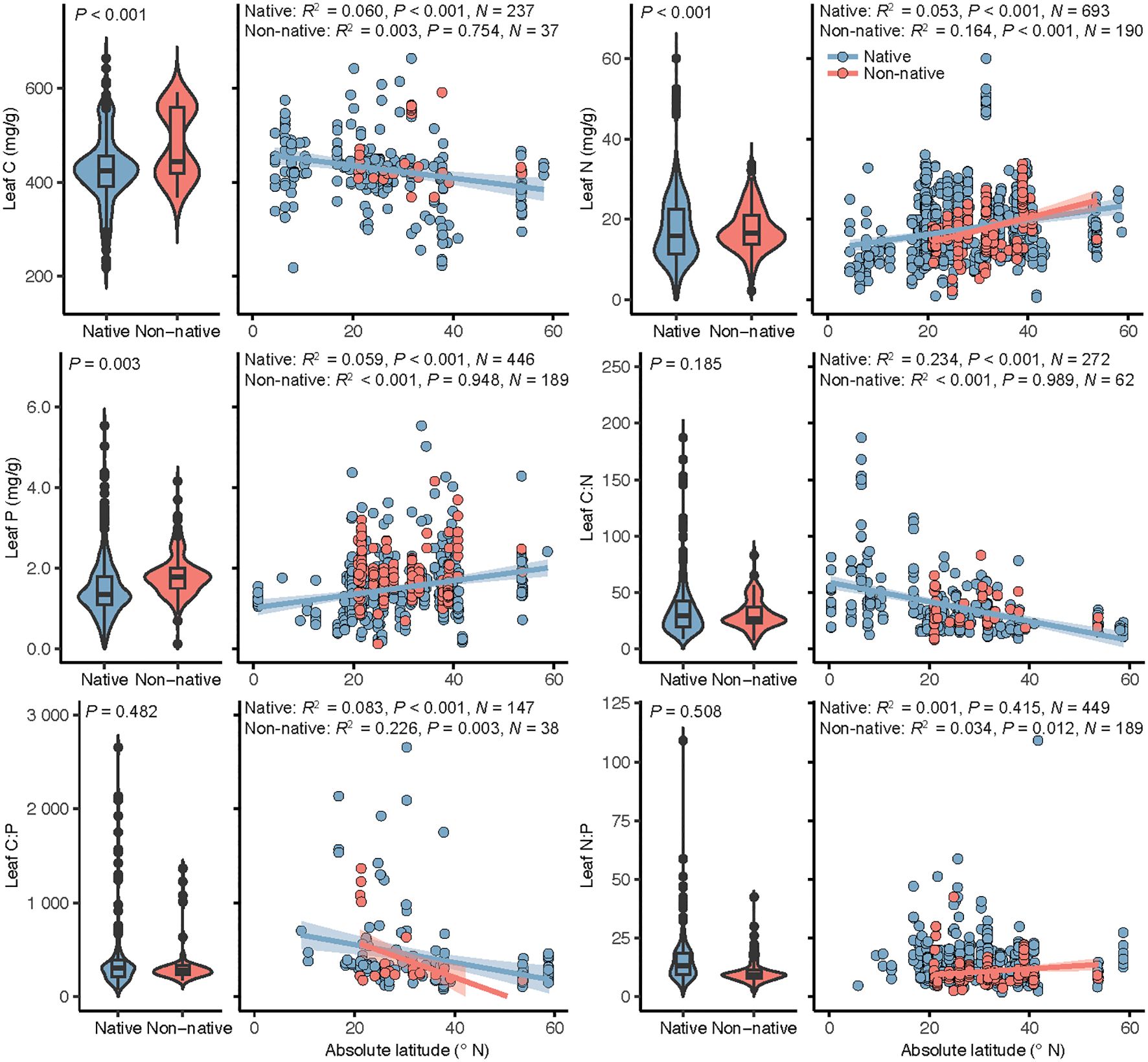
Figure 2 Leaf stoichiometry of native and non-native plant species in coastal wetlands and its relation with latitude. Panels, left side: leaf nutrient contents and stoichiometric ratios of native and non-native plant species, with box plots indicating the median and interquartile ranges and violin plots indicating distribution of the data with black dots outliers in the data set. The P-values indicate the significance of the effect of plant identity in linear mixed models. Panels, right side: linear regressions of the relations between leaf stoichiometry and latitude for native and non-native plants. When the latitude effect was significant, regression lines were plotted and associated R2, P-values and sample size (N) reported.
Relations between leaf stoichiometry and mean annual temperature
For native plants, leaf C, N, and P and C:N and C:P ratios were significantly related with MAT (Figure 3), with leaf C and C:N and C:P ratios increasing with increasing MAT and leaf N and P decreasing. For non-native plants, leaf N and C:P ratio were significantly related with MAT (Figure 3), with leaf N decreasing and leaf C:P ratio increasing with increasing MAT.
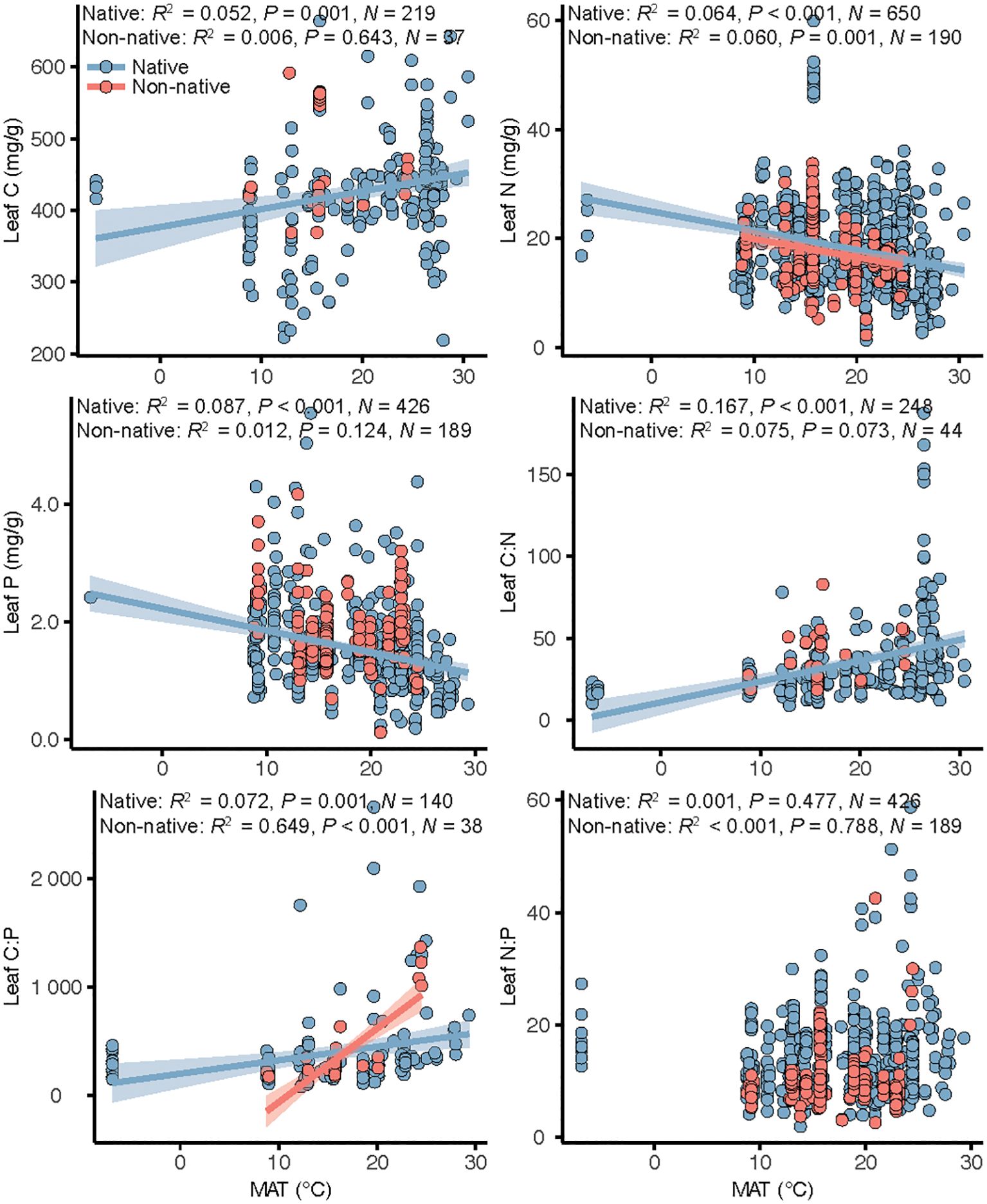
Figure 3 Linear regressions of the relations between mean annual temperature (MAT) and leaf stoichiometry in native and non-native plants in global coastal wetlands. When the temperature effect was significant, regression lines were plotted and associated R2, P-values and sample size (N) reported.
Discussion
We investigated the spatial heterogeneity of leaf nutrient contents and stoichiometric ratios between native and non-native plants across global coastal wetland ecosystems. And we found significant latitudinal patterns in leaf C and N and C:N ratio (Table 1). For native plants, latitudinal patterns were significant for most leaf nutrient contents and stoichiometric ratios, whereas for non-native plants, latitudinal patterns were only significant for leaf N and C:P and N:P ratios. In spite of this, striking differences were also found between native and non-native plants in the responses of leaf nutrient contents and stoichiometric ratios to temperature.
According to several studies, non-native plants typically have higher resource acquisition capacity than that of native plants, resulting in significantly higher C, N, and P contents in non-native plants than in native species (Leishman et al., 2007; Funk, 2013; Funk et al., 2017. This general conclusion is consistent with our findings. Although there were significant differences in leaf nutrient contents between native and non-native plants, there were no significant differences in stoichiometric ratios (Table 1; Figure 2). This result may be because leaf stoichiometric ratios are calculated from leaf element contents, which can mask potential differences between native and non-native plants. Nevertheless, our results indicated that non-native plants have higher nutrient acquisition capacity and thus nutrient levels than those of native plants. Such increases in leaf C and N contents may also indicate an increase in contents of structural substances and nitrogenous compounds, which are most likely related to an increase in plant defenses (Kitajima et al., 2012; Waller, 2012; Aljbory and Chen, 2018). However, the results of this study do not allow an accurate assessment of leaf palatability of coastal plants. Therefore, because leaf stoichiometry may affect multiple plant performances, further bioassay experiments are needed to determine leaf palatability.
Significant latitudinal patterns were detected in leaf C and N contents and C:N ratio of coastal wetland plants but not in leaf P content and C:P and N:P stoichiometric ratios (Table 1), which are results partially consistent with those of previous findings in coastal wetlands (Hu et al., 2021). This was mainly because in this study, plant identity was set as a random effect in LMMs, and species dependence was accounted for when assessing the significance of fixed effects. Furthermore, our results for leaf P are not fully consistent with those based on terrestrial ecosystems, mainly because of the strong P limitation in terrestrial ecosystems, which gradually increases with increasing latitude and leads to similar gradients of leaf P content (Reich and Oleksyn, 2004; Tian et al., 2018). When we further differentiated plant identities, significant differences were detected between native and non-native plants in latitudinal patterns of leaf nutrient contents and stoichiometric ratios (Figure 2). With the exception of the N:P ratio, all leaf stoichiometries of native plants showed significant latitudinal patterns, whereas in non-native plants, only leaf stoichiometries of N content C:P and N:P ratios showed significant latitudinal gradients. Such non-parallel latitudinal gradients have also been observed with native and invasive lineages of Phragmites australis, a model species in North American coastal marshes (Cronin et al., 2015; Bhattarai et al., 2017). Thus, our results extend the applicability of such findings from the level of single species to that of multiple species.
Temperature is one of the most important factors included in the complex factor of latitude. Environmental factors can influence plant traits independently of biotic factors and control the accumulation and removal rates of C, N, and P in leaves at the plant level and therefore also strongly influence plant development and resource use strategies (Moles et al., 2014; Abdala-Roberts et al., 2016; Tian et al., 2018; Wang et al., 2022). We found significant differences in the responses of native and non-native plants to MAT (Figure 3). Except for leaf N:P ratio, all native plants responded significantly to the variation in temperature with latitude, whereas only leaf N and C:P ratio of non-native plants responded to the variation in temperature (Figure 3). This difference in response of leaf stoichiometry to MAT between native and non-native plant species is mainly because non-native plants have been introduced for a short period of time and have not had enough time to adapt to MAT of the introduced range (Guo et al., 2023, 2024).
Temperature directly affects plant physiology and thus regulates plant traits, but it also indirectly mediates biotic factors, such as herbivory, which can cause changes in plant traits (Pennings et al., 2009; Moreira et al., 2018). The latitudinal gradient hypothesis of biotic interactions suggests that biotic interactions are more intense at low latitudes than at high latitudes (Zvereva and Kozlov, 2022; Guo et al., 2024). The increase in intensity of interactions would result in long-term natural selection pressure on plants at lower latitudes for lower palatability and thus resistance to herbivorous consumers, whereas the opposite would be true at higher latitudes (Anstett et al., 2015; Kooyers et al., 2017). We found that compared with non-native plants, native plants had higher leaf C and C:N and C:P ratios and lower leaf P and N at low latitudes (Figure 2). These results suggest that native coastal plants are less palatable at lower latitudes than at higher latitudes, supporting the latitudinal gradient hypothesis of biotic interactions. However, a similar latitudinal pattern was not detected for non-native coastal plants. This result is mainly because non-native plants have only a short history of coevolution with local herbivores in introduced areas and have not yet developed spatial patterns similar to those of native plants. One caveat is our study only focus the climatic factor as MAT, we suggest future study may have more on exploring the multifactor effect on the leaf stoichiometry in coastal wetlands (e.g. tide undulation, deposit sediment chemistry).
Our study is the most comprehensive study to date comparing the latitudinal patterns of leaf stoichiometry of native and non-native plants in coastal wetland ecosystems. We found significant spatial heterogeneity between native and non-native plants, supporting the previous hypothesis that plant traits of native and exotic plants show non-parallel latitudinal gradients (Cronin et al., 2015; Bhattarai et al., 2017). The differences in spatial patterns in this study may be due to differences in the responses of the two types of plants to temperature drivers. These findings support our first and second hypotheses. Leaf C, N, P and their ratios are the vital indicators of leaf palatability, our results imply there are differences in leaf palatability of coastal wetland plants between high and low latitudes, which indicate that biotic interactions (e.g. herbivory intensity) may vary across spatial scales. Thus, this study can help increase understanding of biotic interactions and nutrient cycling in coastal wetlands, and improve predictions of plant growth rates and vegetation productivity across large scales, as well as be useful in the parameterization of vegetation climate models under plant invasion scenarios (Ågren & Andersson, 2011; Tang et al., 2018). In addition, differences in leaf stoichiometry between native and non-native plants can help to study the effects of exotic plants on native plant communities and improve understanding of plant adaptation and evolution (Kerkhoff et al., 2006).
Data availability statement
The original contributions presented in the study are included in the article/Supplementary Material. Further inquiries can be directed to the corresponding author.
Author contributions
YZ: Conceptualization, Data curation, Formal analysis, Funding acquisition, Methodology, Writing – original draft, Writing – review & editing. YG: Conceptualization, Data curation, Formal analysis, Methodology, Writing – original draft, Writing – review & editing. HW: Writing – original draft, Writing – review & editing. NL: Writing – review & editing. HX: Writing – original draft, Writing – review & editing. DZ: Writing – original draft, Writing – review & editing. JQ: Writing – original draft, Writing – review & editing. YH: Data curation, Writing – original draft, Writing – review & editing.
Funding
The author(s) declare financial support was received for the research, authorship, and/or publication of this article. This study was supported by National Natural Science Foundation of China (grant no. 32301368), the Zhejiang Province Commonwealth Projects (grant no. ZCLQ24C0301 and LQ23C030003), the Scientific Research Fund of the Second Institute of Oceanography, SOA (Grant No. JG2216), and the Cooperation of Zhejiang Province and the Chinese Academy of Forestry (grant no. 2023SY11).
Conflict of interest
The authors declare that the research was conducted in the absence of any commercial or financial relationships that could be construed as a potential conflict of interest.
Publisher’s note
All claims expressed in this article are solely those of the authors and do not necessarily represent those of their affiliated organizations, or those of the publisher, the editors and the reviewers. Any product that may be evaluated in this article, or claim that may be made by its manufacturer, is not guaranteed or endorsed by the publisher.
Supplementary material
The Supplementary Material for this article can be found online at: https://www.frontiersin.org/articles/10.3389/fmars.2024.1425587/full#supplementary-material
References
Abdala-Roberts L., Rasmann S., Berny-Mier y Terán J. C., Covelo F., Glauser G., Moreira X. (2016). Biotic and abiotic factors associated with altitudinal variation in plant traits and herbivory in a dominant oak species. Am. J. Bot. 103, 2070–2078. doi: 10.3732/ajb.1600310
Ågren G. I. (2008). Stoichiometry and nutrition of plant growth in natural communities. Annu. Rev. Ecol. Evol. S 39, 153–170. doi: 10.1146/annurev.ecolsys.39.110707.173515
Ågren G. I., Andersson F. O. (2011). Terrestrial ecosystem ecology: principles and applications. (Cambridge: Cambridge University Press).
Aljbory Z., Chen M. S. (2018). Indirect plant defense against insect herbivores: a review. Insect Sci. 25, 2–23. doi: 10.1111/1744-7917.12436
Anstett D. N., Ahern J. R., Glinos J., Nawar N., Salminen J. P., Johnson M. T. (2015). Can genetically based clines in plant defence explain greater herbivory at higher latitudes? Ecol. Lett. 18, 1376–1386. doi: 10.1111/ele.12532
Anstett D. N., Naujokaitis-Lewis I., Johnson M. T. (2014). Latitudinal gradients in herbivory on Oenothera biennis vary according to herbivore guild and specialization. Ecology 95, 2915–2923. doi: 10.1890/13-0932.1
Banks L. K., Frost P. C. (2017). Biomass loss and nutrient release from decomposing aquatic macrophytes: effects of detrital mixing. Aquat. Sci. 79, 881–890. doi: 10.1007/s00027-017-0539-y
Bates D., Mächler M., Bolker B., Walker S. (2015). Fitting linear mixed-effects models using lme4. J. Stat. Software 67, 1–48. doi: 10.18637/jss.v067.i01
Bhattarai G. P., Meyerson L. A., Anderson J., Cummings D., Allen W. J., Cronin J. T. (2017). Biogeography of a plant invasion: genetic variation and plasticity in latitudinal clines for traits related to herbivory. Ecol. Monogr. 87, 57–75. doi: 10.1002/ecm.1233
Bolker B. M., Brooks M. E., Clark C. J., Geange S. W., Poulsen J. R., Stevens M. H. H., et al. (2009). Generalized linear mixed models: a practical guide for ecology and evolution. Trends Ecol. Evol. 24, 127–135. doi: 10.1016/j.tree.2008.10.008
Bradley B. A., Blumenthal D. M., Wilcove D. S., Ziska L. H. (2010). Predicting plant invasions in an era of global change. Trends Ecol. Evol. 25, 310–318. doi: 10.1016/j.tree.2009.12.003
Chen Y., Han W., Tang L., Tang Z., Fang J. (2013). Leaf nitrogen and phosphorus concentrations of woody plants differ in responses to climate, soil and plant growth form. Ecography 36, 178–184. doi: 10.1111/j.1600-0587.2011.06833.x
Cronin J. T., Bhattarai G. P., Allen W. J., Meyerson L. A. (2015). Biogeography of a plant invasion: plant–herbivore interactions. Ecology 96, 1115–1127. doi: 10.1890/14-1091.1
Ehrenfeld J. G. (2010). Ecosystem consequences of biological invasions. Annu. Rev. Ecol. Evol. S. 41, 59–80. doi: 10.1146/annurev-ecolsys-102209-144650
Elser J. J., Acharya K., Kyle M., Cotner J., Makino W., Markow T., et al. (2003). Growth rate–stoichiometry couplings in diverse biota. Ecol. Lett. 6, 936–943. doi: 10.1046/j.1461-0248.2003.00518.x
Funk J. L. (2013). The physiology of invasive plants in low-resource environments. Conserv. Physiol. 1, cot026. doi: 10.1093/conphys/cot026
Funk J. L., Cornwell W. K. (2013). Leaf traits within communities: context may affect the mapping of traits to function. Ecology 94, 1893–1897. doi: 10.1890/12-1602.1
Funk J. L., Nguyen M. A., Standish R. J., Stock W. D., Valladares F. (2017). Global resource acquisition patterns of invasive and native plant species do not hold at the regional scale in Mediterranean type ecosystems. Biol. Invasions 19, 1143–1151. doi: 10.1007/s10530-016-1297-9
Guo Y., Parepa M., Wang H., Wang M., Wu J., Li B., et al. (2024). Global heterogeneity of latitudinal patterns in herbivory between native and exotic plants. bioRxiv. doi: 10.1101/2024.01.24.576872
Guo Y., Yan Z., Gheyret G., Zhou G., Xie Z., Tang Z. (2020). The community-level scaling relationship between leaf nitrogen and phosphorus changes with plant growth, climate and nutrient limitation. J. Ecol. 108, 1276–1286. doi: 10.1111/1365-2745.13369
Guo Y., Zhang Y., Wu J., Richards C. L., Bossdorf O., Li B., et al. (2023). Geographic variation of litter chemistry and palatability in an invasive plant versus its native competitor. J. Biogeogr 50, 1139–1150. doi: 10.1111/jbi.14604
Güsewell S. (2004). N: P ratios in terrestrial plants: variation and functional significance. New Phytol. 164, 243–266. doi: 10.1111/j.1469–8137.2004.01192.x
Harvey J. T., Leffler A. J. (2020). Differential stoichiometric homeostasis and growth in two native and two invasive C3 grasses. Oecologia 193, 857–865. doi: 10.1007/s00442-020-04734-5
Heberling J. M., Fridley J. D. (2013). Resource-use strategies of native and invasive plants in Eastern North American forests. New Phytol. 200, 523–533. doi: 10.1111/nph.12388
Hu Y. K., Liu X. Y., He N. P., Pan X., Long S. Y., Li W., et al. (2021). Global patterns in leaf stoichiometry across coastal wetlands. Global Ecol. Biogeogr 30, 852–869. doi: 10.1111/geb.13254
Huang W., Siemann E., Wheeler G. S., Zou J., Carrillo J., Ding J. (2010). Resource allocation to defence and growth are driven by different responses to generalist and specialist herbivory in an invasive plant. J. Ecol. 98, 1157–1167. doi: 10.1111/j.1365-2745.2010.01704.x
Joshi J., Vrieling K. (2005). The enemy release and EICA hypothesis revisited: incorporating the fundamental difference between specialist and generalist herbivores. Ecol. Lett. 8, 704–714. doi: 10.1111/j.1461-0248.2005.00769.x
Ju R.-T., Li H., Shang L., Qiu S., Li J., Nie M., et al. (2017). “Saltmarsh cordgrass Spartina alterniflora Loisel,” in Biological invasions and its management in China, vol. 2 . Eds. Wan F. H., Jiang M., Zhan A. (Springer Nature Singapore Private Ltd, Singapore), 187–198.
Ju R. T., Ma D., Siemann E., Liu X., Wu J. H., Li B. (2019). Invasive Spartina alterniflora exhibits increased resistance but decreased tolerance to a generalist insect in China. J. Pest Sci. 92, 823–833. doi: 10.1007/s10340-018-1020-y
Kattge J., Knorr W., Raddatz T., Wirth C. (2009). Quantifying photosynthetic capacity and its relationship to leaf nitrogen content for global-scale terrestrial biosphere models. Global Change Biol. 15, 976–991. doi: 10.1111/j.1365-2486.2008.01744.x
Kerkhoff A. J., Fagan W. F., Elser J. J., Enquist B. J. (2006). Phylogenetic and growth form variation in the scaling of nitrogen and phosphorus in the seed plants. Am. Nat. 168, E103–E122. doi: 10.1086/507879
Kinlock N. L., Prowant L., Herstoff E. M., Foley C. M., Akin-Fajiye M., Bender N., et al. (2018). Explaining global variation in the latitudinal diversity gradient: meta-analysis confirms known patterns and uncovers new ones. Global Ecol. Biogeogr 27, 125–141. doi: 10.1111/geb.12665
Kitajima K., Llorens A. M., Stefanescu C., Timchenko M. V., Lucas P. W., Wright S. J. (2012). How cellulose-based leaf toughness and lamina density contribute to long leaf lifespans of shade-tolerant species. New Phytol. 195, 640–652. doi: 10.1111/j.1469-8137.2012.04203.x
Koerselman W., Meuleman A. F. (1996). The vegetation N:P ratio: a new tool to detect the nature of nutrient limitation. J. Appl. Ecol. 33, 1441–1450. doi: 10.2307/2404783
Kooyers N. J., Blackman B. K., Holeski L. M. (2017). Optimal defense theory explains deviations from latitudinal herbivory defense hypothesis. Ecology 98, 1036–1048. doi: 10.1002/ecy.1731
Kurokawa H., Peltzer D. A., Wardle D. A. (2010). Plant traits, leaf palatability and litter decomposability for co-occurring woody species differing in invasion status and nitrogen fixation ability. Funct. Ecol. 24, 513–523. doi: 10.1111/j.1365-2435.2009.01676.x
Leishman M. R., Haslehurst T., Ares A., Baruch Z. (2007). Leaf trait relationships of native and invasive plants: community-and global-scale comparisons. New Phytol. 176, 635–643. doi: 10.1111/j.1469-8137.2007.02189.x
Li B., Liao C. H., Zhang X. D., Chen H. L., Wang Q., Chen Z. Y., et al. (2009). Spartina alterniflora invasions in the Yangtze River estuary, China: an overview of current status and ecosystem effects. Ecol. Eng. 35, 511–520. doi: 10.1016/j.ecoleng.2008.05.013
Liu C., Li Y., Yan P., He N. (2021). How to improve the predictions of plant functional traits on ecosystem functioning? Front. Plant Sci. 12. doi: 10.3389/fpls.2021.622260
Liu Y., Xu X., Liu H., Li B., Nie M. (2020). Latitude gradient variations of leaf functional traits of Spartina alterniflora and Phragmites australis along the coastal saltmarshes of China. J. Fudan Univ. (Nat. Sci.) 59, 381–389.
Minchinton T. E., Simpson J. C., Bertness M. D. (2006). Mechanisms of exclusion of native coastal marsh plants by an invasive grass. J. Ecol. 94, 342–354. doi: 10.1111/j.1365-2745.2006.01099.x
Moles A. T., Perkins S. E., Laffan S. W., Flores-Moreno H., Awasthy M., Tindall M. L., et al. (2014). Which is a better predictor of plant traits: temperature or precipitation? J. Veg. Sci. 25, 1167–1180. doi: 10.1111/jvs.12190
Moreira X., Castagneyrol B., Abdala-Roberts L., Berny-Mier y Teran J. C., Timmermans B. G., Bruun H. H., et al. (2018). Latitudinal variation in plant chemical defences drives latitudinal patterns of leaf herbivory. Ecography 41, 1124–1134. doi: 10.1111/ecog.03326
Ning Z., Chen C., Xie T., Zhu Z., Wang Q., Cui B., et al. (2021). Can the native faunal communities be restored from removal of invasive plants in coastal ecosystems? A global meta-analysis. Global Change Biol. 27, 4644–4656. doi: 10.1111/gcb.15765
Ning Z., Cui B., Chen C., Xie T., Gao W., Zhang Y., et al. (2024). Tidal channel meanders serve as stepping-stones to facilitate cordgrass landward spread by creating invasion windows. Ecol. Appl. 34, e2813. doi: 10.1002/eap.2813
Pennings S. C., Ho C. K., Salgado C. S., Więski K., Davé N., Kunza A. E., et al. (2009). Latitudinal variation in herbivore pressure in Atlantic Coast salt marshes. Ecology 90, 183–195. doi: 10.1111/j.1365–2486.2011.02636.x
Pyšek P., Jarošík V., Hulme P. E., Pergl J., Hejda M., Schaffner U., et al. (2012). A global assessment of invasive plant impacts on resident species, communities and ecosystems: the interaction of impact measures, invading species' traits and environment. Global Change Biol. 18, 1725–1737. doi: 10.1111/j.1365-2486.2011.02636.x
R Core Team (2019). R: a language and environment for statistical computing (Vienna: R for Statistical Computing).
Reese A. T., Ames G. M., Wright J. P. (2016). Variation in plant response to herbivory underscored by functional traits. PloS One 11, e0166714. doi: 10.1371/journal.pone.0166714
Reich P. B., Oleksyn J. (2004). Global patterns of plant leaf N and P in relation to temperature and latitude. P. Natl. Acad. Sci. 101, 11001–11006. doi: 10.1073/pnas.040358810
Rogers A., Allen D. J., Davey P. A., Morgan P. B., Ainsworth E. A., Bernacchi C. J., et al. (2004). Leaf photosynthesis and carbohydrate dynamics of soybeans grown throughout their life-cycle under free-air carbon dioxide enrichment. Plant Cell Environ. 27, 449–458. doi: 10.1111/j.1365-3040.2004.01163.x
Sala O. E., Stuart Chapin F. I. I. I., Armesto J. J., Berlow E., Bloomfield J., Dirzo R., et al. (2000). Global biodiversity scenarios for the year 2100. Science 287, 1770–1774. doi: 10.1126/science.287.5459.1770
Schädler M., Jung G., Auge H., Brandl R. (2003). Palatability, decomposition and insect herbivory: patterns in a successional old-field plant community. Oikos 103, 121–132. doi: 10.1034/j.1600–0706.2003.12659.x
Silliman B. R., Bertness M. D. (2004). Shoreline development drives invasion of Phragmites australis and the loss of plant diversity on New England salt marshes. Conserv. Biol. 18, 1424–1434. doi: 10.1111/j.1523-1739.2004.00112.x
Tang X., Zhao X., Bai Y., Tang Z., Wang W., Zhao Y., et al. (2018). Carbon pools in China’s terrestrial ecosystems: New estimates based on an intensive field survey. Proc. Natl. Acad. Sci. 115, 4021-4026. doi: 10.1073/pnas.1700291115
Tian D., Yan Z., Niklas K. J., Han W., Kattge J., Reich P. B., et al. (2018). Global leaf nitrogen and phosphorus stoichiometry and their scaling exponent. Natl. Sci. Rev. 5, 728–739. doi: 10.1093/nsr/nwx142
Vilà M., Corbin J. D., Dukes J. S., Pino J., Smith S. D. (2007). “Linking plant invasions to global environmental change,” in Terrestrial ecosystems in a changing world (Springer Berlin Heidelberg, Berlin, Heidelberg), 93–102.
Vilà M., Espinar J. L., Hejda M., Hulme P. E., Jarošík V., Maron J. L., et al. (2011). Ecological impacts of invasive alien plants: a meta-analysis of their effects on species, communities and ecosystems. Ecol. Lett. 14, 702–708. doi: 10.1111/ele.2011.14.issue-7
Waller G. R. (2012). Alkaloid biology and metabolism in plants. (New York and London: Springer Science & Business Media).
Wang J., Wang X., Ji Y., Gao J. (2022). Climate factors determine the utilization strategy of forest plant resources at large scales. Front. Plant Sci. 13. doi: 10.3389/fpls.2022.990441
Xu X., Liu H., Liu Y., Zhou C., Pan L., Fang C., et al. (2020). Human eutrophication drives biogeographic salt marsh productivity patterns in China. Ecol. Appl. 30, e02045. doi: 10.1002/eap.2045
Xu X., Wei S., Chen H., Li B., Nie M. (2022). Effects of Spartina invasion on the soil organic carbon content in salt marsh and mangrove ecosystems in China. J. Appl. Ecol. 59, 1937–1946. doi: 10.1111/1365-2664.14202
Zhang Y., Pennings S. C., Liu Z., Li B., Wu J. (2021). Consistent pattern of higher lability of leaves from high latitudes for both native Phragmites australis and exotic Spartina alterniflora. Funct. Ecol. 35, 2084–2093. doi: 10.1111/1365-2435.13826
Zhu D., Hui D., Wang M., Yang Q., Yu S. (2020). Light and competition alter leaf stoichiometry of introduced species and native mangrove species. Sci. Total Environ. 738, 140301. doi: 10.1016/j.scitotenv.2020.140301
Keywords: biogeography, coastal wetlands, leaf stoichiometry, latitudinal spatial patterns, mean annual temperature, native plants, non-native plants
Citation: Zhang Y, Guo Y, Wang H, Li N, Xu H, Zhang D, Qian J and Hu Y (2024) Divergence in spatial patterns of leaf stoichiometry between native and non-native plants across coastal wetlands. Front. Mar. Sci. 11:1425587. doi: 10.3389/fmars.2024.1425587
Received: 30 April 2024; Accepted: 18 June 2024;
Published: 03 July 2024.
Edited by:
Qing Wang, Beijing Normal University, Zhuhai, ChinaReviewed by:
Fengrun Wu, Xiamen University of Technology, ChinaZhonghua Ning, Beijing Normal University, China
Copyright © 2024 Zhang, Guo, Wang, Li, Xu, Zhang, Qian and Hu. This is an open-access article distributed under the terms of the Creative Commons Attribution License (CC BY). The use, distribution or reproduction in other forums is permitted, provided the original author(s) and the copyright owner(s) are credited and that the original publication in this journal is cited, in accordance with accepted academic practice. No use, distribution or reproduction is permitted which does not comply with these terms.
*Correspondence: Youzheng Zhang, emhhbmd5ekBzaW8ub3JnLmNu
†These authors share first authorship