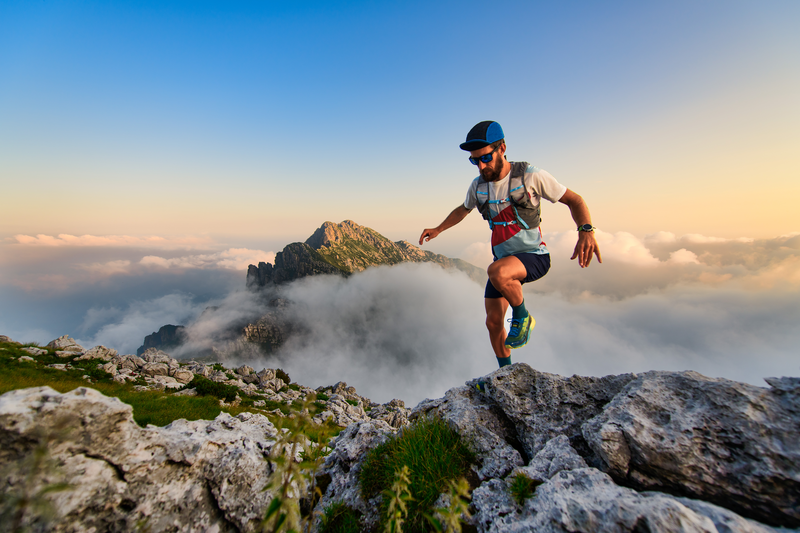
95% of researchers rate our articles as excellent or good
Learn more about the work of our research integrity team to safeguard the quality of each article we publish.
Find out more
BRIEF RESEARCH REPORT article
Front. Mar. Sci. , 19 September 2024
Sec. Marine Pollution
Volume 11 - 2024 | https://doi.org/10.3389/fmars.2024.1425221
This article is part of the Research Topic Endocrine Disruption in Marine Species: Unraveling Pollution and Climate Change Effects View all 5 articles
Due to the expanding oil-related activities, the arctic and sub-arctic marine environments are increasingly vulnerable to oil-related pollution such as accidental oil spills. These cold-water ecosystems harbor many fish species that are both ecologically and economically important such as the pelagic polar cod (Boreogadus saida), capelin (Mallotus villosus), and benthic long rough dab (Hippoglossoides platessoides). The latter two are much less studied and it is crucial to characterize their responses to oil-related contaminants and develop molecular biomarkers and genomic resources for future monitoring. In this study, liver slice preparation and culture methods were used to characterize the transcriptome responses (using RNA-seq) in capelin and long rough dab to exposures of the polycyclic aromatic hydrocarbon (PAH) compound benzo[a]pyrene (BaP). The liver slice culture and exposure experiments were performed onboard a research vessel in the Barents Sea. Strong up-regulation of genes involved in biotransformation, particularly the aryl hydrocarbon receptor signaling pathway was observed in both species. A comparison of the number of differentially expressed genes (DEGs) with previously published polar cod exposures indicates that the latter responded more strongly (higher number of genes), suggesting higher uptake and bioconcentration of BaP in the fatty liver tissue, although other factors such as differences in clearance rate could potentially affect the responses. This study provides new genomic resources and gene expression biomarkers in capelin and long rough dab, enhancing our understanding of their response mechanism to oil-related contaminants.
Oil-related activities are expanding into the arctic and sub-arctic marine environments, making them increasingly vulnerable to oil-related pollution, such as accidental oil spills. Globally, an estimated 13% of the undiscovered oil resources are in the Arctic and tens of oil and gas fields have been identified in the region (Harsem et al., 2011). The Barents Sea is an area of particular interest, as the Norwegian Offshore Directorate estimates 61% of undiscovered resources in Norwegian territorial waters to be found there and has awarded 78 new production licenses in the past 10 years (Norwegian Offshore Directorate, 2024). Increased traffic and industrial activities in the harsh climate of the Arctic region may increase the likelihood of an oil spill, posing considerable risk to the sensitive Arctic ecosystem (Nevalainen et al., 2017; Nong et al., 2018).
In addition to the risk of accidental oil spill, PAH contaminations in the Arctic region may come from atmospheric deposition, increased traffic, and crude-oil contaminated produced water discharges from offshore petroleum installations (Beyer et al., 2020). Although baseline levels of PAHs such as BaP in fish from Arctic and sub-Arctic regions are low or undetectable (Nahrgang et al., 2013; Jörundsdóttir et al., 2014), PAH contaminations have been detected in fish species near offshore installations in the North Sea, causing concerns about the possible effect of crude oil contamination in the sub-Arctic regions due to increasing oil-related activities (Beyer et al., 2020).
The Arctic and sub-Arctic cold-water environments are home to a wide array of fish species, many of which have both ecological and commercial significance. Among these species are the pelagic polar cod (Boreogadus saida) and capelin (Mallotus villosus) as well as the demersal long rough dab (Hippoglossoides platessoides). Polar cod and capelin are fish species in the arctic and sub-arctic food web, linking lower trophic levels to higher-level predators such as sea birds and marine mammals (Hop and Gjøsæter, 2013). While the Polar cod is an arctic species, capelin is the most abundant in sub-arctic regions (Hop and Gjøsæter, 2013). Long rough dab is a bottom-dwelling species that is among the most abundant in the Barents Sea of the sub-Arctic region (Walsh, 1996).
Although the effects of pollutants on temperate fish species have been widely studied, fewer studies have focused on how cold-water species respond to pollutants such as oil spills (Beyer et al., 2020). These fish species also have different life history traits (slower growth, later maturation, greater allocation of energy resources towards reproduction than growth) that may affect how they respond to contaminants. Moreover, some Arctic and sub-Arctic species store fat in the liver (e.g. polar cod and Atlantic cod), which may affect the accumulation and responses to lipophilic contaminants (Dabrowska et al., 2009). It is thus important to develop robust methods and genomic resources that can effectively characterize the responses of the Arctic and sub-Arctic species to relevant contaminants.
Transcriptome analyses have previously been useful in toxicological studies of crude oil-related contaminants in polar cod (Nahrgang et al., 2009, 2010; Song et al., 2019; Yadetie et al., 2021) and Atlantic cod (Yadetie et al., 2018; Aranguren-Abadía et al., 2022). However, transcriptomic resources and biomarkers that facilitate the study of contaminant effects have not been established for the less-studied capelin and long rough dab. Physiologically, capelin eggs respond to heavy fuel oil with decreased hatching success and malformations such as craniofacial abnormalities and reduced body size (Frantzen et al., 2012; Tairova et al., 2019). Such toxicological studies will benefit from the use of molecular biomarkers that may better detect sub-lethal effects and link molecular initiating events to observed apical endpoints in the adverse outcome pathway framework (Ankley et al., 2010; Sørhus et al., 2017). Little is known on the response of long rough dab to oil pollution but being a bottom-dwelling fish, it can be vulnerable to the harmful effects of contaminated sediments (Jonsson and Björkblom, 2011). Crude oil is more persistent in sediments as shown for the Deepwater Horizon oil spill (Liu et al., 2012) and bottom-dwelling fish are useful for evaluating toxicological effects. For example, the flatfish Southern flounder has been useful to study the effects of sediments contaminated with Deepwater Horizon oil (Brown-Peterson et al., 2015). Thus, developing genomic resources and molecular biomarkers for long rough dab will facilitate its use in environmental pollution monitoring in the sub-Arctic region.
The primary objectives of this study were threefold: first, to sequence liver transcriptomes for capelin and dab; second, to elucidate the transcriptome responses elicited by benzo[a]pyrene (BaP) in these species and identify candidate biomarkers; and third to compare the transcriptome responses observed in capelin and dab with previously published data from polar cod (Yadetie et al., 2021), which store fat in the liver. To this end, liver slices (Eide et al., 2014; Yadetie et al., 2021) of the fish species were prepared and exposed to BaP, on board a research vessel in the northern Barents Sea, and transcriptome responses were investigated using RNA-seq and qPCR. PAHs such as BaP that can activate the aryl hydrocarbon receptor (AhR) are among the most toxic components of crude oil and most biomarker assays for oil contaminants are based on the AhR activity (Hylland et al., 2006; Pampanin and Sydnes, 2013; Honda and Suzuki, 2020). Therefore, we used BaP as a model compound representing one of the most toxic components of crude oil to map transcriptome responses and develop biomarkers of crude oil contamination.
All fish were sampled by trawling (permitted by the Directorate of Fisheries, Norway) on board R/V Kronprins Haakon during the Nansen Legacy research cruise to the Northern Barents Sea in August 2019. Capelin and long rough dab were sampled opportunistically at station P2 (77.5156°N, 33.9343°E) during a trawl that served multiple sampling purposes for other projects. See Supplementary Figure S1 for location details and Supplementary Table S1 for biometric details. Female polar cod were collected at station P3 (78.7°N, 34.0°E) and the data of this species was previously published (Yadetie et al., 2021). After trawling, the fish were either kept in 500 L tanks with running seawater at ambient seawater temperature or kept on ice. One by one, live fish were euthanized by a quick blow to the head before dissecting the liver aseptically for slicing. This procedure does not require ethical approval as the fish are killed before the experiment.
The precision-cut liver slices (PCLS) were prepared as described previously (Yadetie et al., 2021). For long rough dab, liver samples were collected from six female fish but one replicate was excluded from the analysis due to insufficient cell viability as assessed by MTT and LDH assay (n = 5 per group). The liver was rinsed and placed in ice-cold PCLS buffer (Supplementary Table S2). A small block (ca. 2x2x2 cm) of liver sample from each fish was cut and glued (Loc-tite® Super Glue) on the specimen plate of a Leica vibratome VT1200 (Leica, Wetzlar, Germany) and sliced into 300 μm thick slices at a speed of 0.9 mm/s and amplitude 3 mm. The slices were then transferred to a petri dish with ice-cold culture medium (Leibowitz-15 medium, Life Technologies™ Gibco®, Paisley, UK) supplemented with 10% charcoal-stripped and heat-inactivated fetal bovine serum and 1% penicillin-streptomycin-amphotericin (10,000 U/mL potassium penicillin, 10,000 μg/mL streptomycin and 25 μg/mL amphotericin B, Sigma-Aldrich). The slices from each fish were cut into smaller (approximately 4 × 4 mm) pieces using a sterile razor blade and divided into 12-well culture plates containing the growth medium. For capelin, the liver was too small to slice with a vibratome. Instead, 24 liver samples were manually cut into approximately 1 mm3 pieces using a sterile razor blade and put into 12-well culture plates (all slices from a single liver per well, n = 6 per group). All liver pieces were subsequently preincubated at 10°C for 2 h in the culture medium (Supplementary Table S3) before exposure.
A paired sample design was chosen for long rough dab as described previously (Yadetie et al., 2021), with 5 biological replicates per treatment group. For capelin, the liver was too small for paired sample design, and thus an independent sample design was chosen where replicates in each treatment group represented different individual fish. The slices were treated with dimethyl sulfoxide (DMSO; solvent control), low, medium, and high concentrations of BaP (0, 0.1, 1, and 10 μM, respectively) with an equal concentration of DMSO (0.2%) across treatment groups. The BaP concentrations were selected based on our experience with ex vivo exposures covering concentration ranges that enable to identify biomarker candidates and are beyond the naturally occurring BaP concentrations in arctic sediments (Lakhmanov et al., 2022; Stich and Dunn, 1980). All samples were cultured in 12-well plates (Costar, Corning, New York, USA) in 2 mL of culture medium and incubated at 10°C on a horizontal shaker (50 rpm) for 72 h. After 72 h in culture, slices were collected and either used directly in an MTT assay or snap-frozen in liquid nitrogen for RNA extraction. The samples as well as the media (for measurement of lactate dehydrogenase; LDH) were stored at −80°C until further processing.
Total RNA was extracted from frozen slices in TRI Reagent using a motorized handheld tissue homogenizer according to the manufacturer’s protocol (Sigma-Aldrich, St. Louis, MO, USA). RNA purity and concentration were confirmed using a NanoDrop ND-1000 spectrophotometer (NanoDrop Technologies, Wilmington, DE, USA) and Agilent 2100 bioanalyzer where all samples passed a RIN value of ≥ 6.8 (Agilent Technologies, Santa Clara, CA, USA). Library preparation using Poly(A)+RNA and sequencing (Illumina NovaSeq 6000) were performed at Novogene (Cambridge, UK). Briefly, for each sample, Poly(A)+RNA was purified from 1 μg total RNA using poly-T oligo-attached magnetic beads and fragmented using divalent cations under elevated temperature. After first strand and second strand cDNA synthesis, size selected cDNA library (150–200 bp) was PCR amplified and sequenced to generate 150-bp paired-end sequences using the Illumina NovaSeq 6000 platform.
For long rough dab and capelin, de novo transcriptome assembly and quantification were performed by Novogene as described before (Yadetie et al., 2022). Briefly, after clean raw RNA-seq reads and quality filtering, de novo transcriptome assembly was performed by combining all reads (from both control and exposed samples) for each species, using the Trinity software (Grabherr et al., 2011). The longest transcript from each cluster was selected as “unigene”. Subsequent analyses were performed with the unigene reference assembly for each species. The unigene assemblies were annotated using BLAST+ searches in the databases NT, NR, KO, SwissProt, Pfam, GO, and KOG. For each species, the clean reads from individual samples were mapped to the respective reference assembly and quantified using Bowtie2 and RSEM (Li and Dewey, 2011). The summary of the quality controls and assembly statistics can be found in Supplementary Tables S4–S6. The distribution of transcriptome lengths for each species are depicted in Supplementary Figures S2 and S3.
Differential expression analysis between DMSO control and 10 μM BaP-treated groups was performed using the edgeR package (Robinson et al., 2010). Differential expression analysis was performed using paired tests for liver slices from long rough dab, as described previously (Yadetie et al., 2021), whereas an unpaired test was used for capelin groups with statistically independent samples. Genes with adjusted p-values (padj) < 0.05 and fold-change (FC) ≥ 1.5 for up-regulated and FC ≤ 0.67 for down-regulated were considered differentially expressed. The venn diagram was plotted using Plotly. The RNA-seq raw and processed data files have been deposited in the ArrayExpress database at EMBL-EBI (http://www.ebi.ac.uk/arrayexpress) under accession numbers E-MTAB-13934 (capelin) and E-MTAB-13932 (long rough dab).
Quantitative polymerase chain reaction (qPCR) assays were performed as described previously (Yadetie et al., 2018) using primers targeting cytochrome P450 family 1 subfamily A (cyp1a), aryl hydrocarbon receptor repressor b (ahrrb), and fibroblast growth factor 7 (fgf7) protein-coding genes (Supplementary Table S7). Briefly, cDNA was synthesized from 1.0 μg of each total RNA sample in 20 μL reactions using iScript cDNA Synthesis Kit (Bio-Rad, Hercules, CA, USA). qPCR was performed with diluted (1:20) cDNA and 0.5 μM of the forward and reverse primers using a BioRad CFX96 real-time PCR detection system (Bio-Rad Laboratories). All quality controls were run as previously described and the expression levels were analyzed by comparing treated samples to controls using the ΔΔCq method (Schmittgen and Livak, 2008) followed by log2 transformation. The data was plotted using GraphPad PRISM 10.
The supernatants of the PCLS cultures were frozen in liquid nitrogen for subsequent viability analysis based on LDH activity using the Cytotoxicity Detection Kit (Roche Molecular Diagnostics, Mannheim, Germany). The results are depicted in Supplementary Figure S4. For the long rough dab PCLS cultures, additionally, MTT assays were performed following the protocol described in Yadetie et al., 2018. For capelin, no MTT assay was possible as the liver was too small for slicing appropriate sizes for this assay. As no spectrophotometer was available onboard the research vessel, the color change was evaluated by eye and pictures were taken.
In capelin, a total of 82 genes (with known human orthologs) were identified as differentially expressed genes (DEGs; Figure 1). Since independent samples (biological replicates) were used in the case of capelin, an unpaired independent test was applied to identify DEGs. For long rough dab, since slices from the same fish could be used in both the control and BaP-treated groups, a paired test was applied in the statistical analysis, as described before (Yadetie et al., 2021). The number of DEGs identified for long rough dab (with the same cutoff described above for capelin) was 189 (Figure 1).
Figure 1. Venn diagram of the differentially expressed genes in polar cod, long rough dab, and capelin liver slices exposed to 10 μM BaP. Only DEGs of human orthologs were included. Previously published data from polar cod was included for comparison (Yadetie et al., 2021).
A comparison of the DEGs shows 14 shared genes between capelin and long rough dab including the Ahr-pathway genes cyp1a and ahrrb (Figure 1). The cyp1a and ahrrb genes are among the top induced genes used as biomarkers for Ahr pathway-activating compounds such as BaP and crude oil (Yadetie et al., 2018, 2021; Strople et al., 2023). A higher number of DEGs was identified for the long rough dab, which might be related to the use of paired design, which reduces confounding factors providing better statistical power. It is also possible that the manual capelin slicing resulting in thicker liver tissue was less optimal for culture and exposure. Furthermore, in the case of capelin, the whole liver was used per sample which may contain more diverse cell types such as endothelial cells from larger blood vessels and biliary ducts that are less likely to be included in the thin liver slices of long rough dab.
Comparison of the DEGs from the two species with DEGs from polar cod PCLS from experiments with a similar design (10 μM BaP exposure; Yadetie et al., 2021) shows that the two species in the current experiment have a much lower number of DEGs. This is possibly related to the higher lipid content of the polar cod liver, although other factors such as differences in metabolic rate may affect the responses. Alternatively, species differences in AhR sensitivity may contribute to the differences in DEGs.
The RNA sequencing was performed only from the highest concentration of BaP (10 μM) and DMSO groups. To evaluate concentration responses, the expression of selected genes (cyp1a, ahrrb, and fgf7) that were highly upregulated by BaP in this and other experiments (Yadetie et al., 2018, 2021; Strople et al., 2023) was quantitated using qPCR. Figure 2 shows that the three genes were significantly upregulated in the highest BaP concentration groups in both species. The cyp1a gene was significantly upregulated also in the lower (0.1 μM and 1 μM BaP) concentration groups for capelin. None of the other capelin qPCR analyzed genes were significantly affected in the 0.1 μM and 1 μM BaP groups (Figure 2A). In long rough dab, none of the genes were significantly affected in the 0.1 μM and 1 μM BaP concentration groups (Figure 2B), which may suggest lower responses to BaP than capelin, although a higher number of DEGs were detected in the former (Figure 1).
Figure 2. qPCR analysis of cyp1a, ahrrb, and fgf7 mRNA levels in (A) capelin (n = 6) and (B) long rough dab liver slices (n = 5) exposed to 0.1-10 μM BaP as indicated. Error bars indicate the mean ± SD of the individual values. Asterisks indicate significant differences to DMSO controls (*p < 0.05; **p < 0.01; ***p < 0.001).
Pathway enrichment analysis of the DEGs showed that the Ahr pathway was activated in capelin (Table 1). In addition to the Ahr pathway, only two other WikiPathways, namely Glycerophospholipid Biosynthetic Pathway and B Cell Receptor Signaling Pathway, were significantly enriched (Table 1). In long rough dab, the Ahr pathway was not among the top list, although the top enriched Nuclear Receptors Meta-Pathway includes the Ahr-signaling pathway (Fijten et al., 2023), also evidenced by the presence of cyp1a and ahrrb in the gene list populating this enriched pathway (Table 2). Other enriched pathways in long rough dab include the NRf2-signaling and Cysteine and methionine catabolism related to oxidative stress responses (Ma, 2013; Bin et al., 2017). BaP is known to induce oxidative stress that may lead to activation of the NRf2 pathway (Ma, 2013). Overall, the pathways enriched in both species in this study relate to the activation of Ahr-signaling pathways and are consistent with known broad toxicological effects of this pathway that include oxidative stress responses, immune responses, and lipid metabolism (Lee et al., 2010; Ma, 2013; Rothhammer and Quintana, 2019). Comparison of enriched Wikipathways for capelin, long rough dab (Tables 1, 2), and polar cod (Yadetie et al., 2021), shows that the main Ahr-related pathways were enriched in all three fish, while there are pathways that are affected exclusively in each organism (Supplementary Figure S5). For example, oxidative stress and various signaling pathways are exclusive to long rough dab and polar cod, respectively (Supplementary Figure S5). Induction of oxidative stress in long rough dab is consistent with the well-known effects of BaP (Briedé et al., 2004). Although a much longer list of genes was affected in polar cod, oxidative stress-related pathways were not significantly enriched.
Enriched pathways may indicate disturbed physiological processes and may provide a mechanistic link to apical endpoints. The Ahr pathway is emblematic of the detoxification of xenobiotic compounds and its enrichment has been linked to developmental abnormalities such as cardiac malformation (Incardona et al., 2009; Tang et al., 2020) and circadian rhythm disturbances (Shen et al., 2019). In long rough dab, the enriched pathways are often related to oxidative stress which can have various adverse physiological outcomes or be resolved at the cellular level as adaptive responses. Thus, some of the activated pathways can suggest physiological consequences for exposed fish, and genes from these pathways may be used as biomarker genes once the link is established, for example by an AOP.
In summary, we have characterized the liver transcriptome response in the two cold-water species capelin and long rough dab, providing transcriptomic resources and biomarkers for PAH exposures, previously unavailable for these species. These resources can be useful in future monitoring of the effects of Ahr-agonist contaminants such as high molecular weight PAHs from crude oil, a possible source of pollution due to increasing petroleum-related activities in the Arctic region. Toxicological studies of crude oil exposure have revealed harmful effects such as reduced hatching success of capelin eggs and increased developmental malformations (Frantzen et al., 2012; Tairova et al., 2019). However, according to a recent exposure study of capelin embryos to a water-soluble fraction (WSF) of crude oil had no observable effects on embryo development (Nahrgang et al., 2023). Combining phenotypic analysis such as developmental endpoints with gene expression analysis may reveal mechanistic information underlying observed phenotypic changes in such toxicological studies (Sørhus et al., 2016; Aranguren-Abadía et al., 2022). Comparison of the transcriptome analysis results with previously published polar cod exposures (Yadetie et al., 2021) indicated that polar cod responded more strongly (higher number of genes), suggesting higher up-take of BaP in the fatty liver tissue or species differences in AhR sensitivity. The main enriched pathways in polar cod indicated effects on cell signaling while pathways enriched in capelin and long rough dab indicate immune response and oxidative stress. In all three fish species, pathways related to Ahr signaling were dysregulated. Future studies need to investigate the factors that resulted in the apparent differences in the responses to BaP.
The sub-Arctic fish species capelin and long rough dab responded to the exposure of a petroleum-related contaminant with the upregulation of biomarker genes in the liver, particularly cyp1a and ahrrb of the Ahr pathway. This study thus provides new liver transcriptome resources and gene expression biomarkers in capelin and long rough dab. This is relevant for biomonitoring in these lesser-studied environments, particularly in the case of oil spills.
The datasets presented in this study can be found in online repositories. The names of the repository/repositories and accession number(s) can be found in the article/Supplementary Material.
Ethical review and approval were not required for the animal study because the killing of animals to use their organs or tissues for scientific purposes is not considered an animal experiment under the Norwegian Animal Experimentation Act. The use of a species (Hippoglossoides platessoides) listed as Endangered by the IUCN Red List is justified according to § 10(e): The experiment is for research aimed at environmental protection.
NB: Funding acquisition, Conceptualization, Investigation, Methodology, Visualization, Writing – original draft, Writing – review & editing. FY: Conceptualization, Formal analysis, Investigation, Methodology, Supervision, Writing – original draft, Writing – review & editing. HL: Methodology, Writing – review & editing. OK: Funding acquisition, Writing – review & editing. AG: Funding acquisition, Project administration, Writing – review & editing.
The author(s) declare financial support was received for the research, authorship, and/or publication of this article. The study was supported by the Research Council of Norway through the Nansen Legacy Project (276730) and the European Union’s Horizon 2020 program through the MSCA NRgCod project (101023171) granted to NB. The fees for open access publication were covered by the University of Bergen.
The authors would like to thank Faezeh Mohammadi for assistance during laboratory experiments and the Nansen Legacy seasonal Q3 2019 cruise organizers and team members for the support onboard during sampling and exposure experiments.
The authors declare that the research was conducted in the absence of any commercial or financial relationships that could be construed as a potential conflict of interest.
All claims expressed in this article are solely those of the authors and do not necessarily represent those of their affiliated organizations, or those of the publisher, the editors and the reviewers. Any product that may be evaluated in this article, or claim that may be made by its manufacturer, is not guaranteed or endorsed by the publisher.
The Supplementary Material for this article can be found online at: https://www.frontiersin.org/articles/10.3389/fmars.2024.1425221/full#supplementary-material
Ankley G. T., Bennett R. S., Erickson R. J., Hoff D. J., Hornung M. W., Johnson R. D., et al. (2010). Adverse outcome pathways: a conceptual framework to support ecotoxicology research and risk assessment. Env. Toxicol. Chem. 29, 730–741. doi: 10.1002/etc.34
Aranguren-Abadía L., Yadetie F., Donald C. E., Sørhus E., Myklatun L. E., Zhang X., et al. (2022). Photo-enhanced toxicity of crude oil on early developmental stages of Atlantic cod (Gadus morhua). Sci. Total Environ. 807, 150697. doi: 10.1016/J.SCITOTENV.2021.150697
Beyer J., Goksøyr A., Hjermann D.Ø., Klungsøyr J. (2020). Environmental effects of offshore produced water discharges: A review focused on the Norwegian continental shelf. Mar. Environ. Res. 162, 105155. doi: 10.1016/J.MARENVRES.2020.105155
Bin P., Huang R., Zhou X. (2017). Oxidation resistance of the sulfur amino acids: Methionine and cysteine. BioMed. Res. Int. 2017, 1–6. doi: 10.1155/2017/9584932
Briedé J. J., Godschalk R. W. L., Emans M. T. G., De Kok T. M. C. M., Van Agen E., Van Maanen J. M. S., et al. (2004). In vitro and in vivo studies on oxygen free radical and DNA adduct formation in rat lung and liver during benzo[a]pyrene metabolism. Free Radic. Res. 38, 995–1002. doi: 10.1080/10715760400000976
Brown-Peterson N. J., Krasnec M., Takeshita R., Ryan C. N., Griffitt K. J., Lay C., et al. (2015). A multiple endpoint analysis of the effects of chronic exposure to sediment contaminated with Deepwater Horizon oil on juvenile Southern flounder and their associated microbiomes. Aquat. Toxicol. 165, 197–209. doi: 10.1016/J.AQUATOX.2015.06.001
Dabrowska H., Bernard E., Barska I., Radtke K. (2009). Inter-tissue distribution and evaluation of potential toxicity of PCBs in Baltic cod (Gadus morhua L.). Ecotoxicol. Environ. Saf. 72, 1975–1984. doi: 10.1016/J.ECOENV.2009.07.007
Eide M., Karlsen O. A., Kryvi H., Olsvik P. A., Goksøyr A. (2014). Precision-cut liver slices of Atlantic cod (Gadus morhua): An in vitro system for studying the effects of environmental contaminants. Aquat. Toxicol. 153, 110–115. doi: 10.1016/j.aquatox.2013.10.027
Fijten R., Willighagen E., Ehrhart F., Summer-Kutmon M., Cirillo E., Weitz E., et al. Nuclear receptors meta-pathway (WP2882) [Internet]. Available online at: https://www.wikipathways.org/instance/WP2882.
Frantzen M., Falk-Petersen I. B., Nahrgang J., Smith T. J., Olsen G. H., Hangstad T. A., et al. (2012). Toxicity of crude oil and pyrene to the embryos of beach spawning capelin (Mallotus villosus). Aquat. Toxicol. 108, 42–52. doi: 10.1016/J.AQUATOX.2011.09.022
Grabherr M. G., Haas B. J., Yassour M., Levin J. Z., Thompson D. A., Amit I., et al. (2011). Full-length transcriptome assembly from RNA-Seq data without a reference genome. Nat. Biotechnol. 2011 297 29, 644–652. doi: 10.1038/nbt.1883
Harsem Ø., Eide A., Heen K. (2011). Factors influencing future oil and gas prospects in the Arctic. Energy Policy 39, 8037–8045. doi: 10.1016/J.ENPOL.2011.09.058
Honda M., Suzuki N. (2020). Toxicities of polycyclic aromatic hydrocarbons for aquatic animals. Int. J. Environ. Res. Public Health 17, 1363. doi: 10.3390/ijerph17041363
Hop H., Gjøsæter H. (2013). Polar cod (Boreogadus saida) and capelin (Mallotus villosus) as key species in marine food webs of the Arctic and the Barents Sea. Mar. Biol. Res. 9, 878–894. doi: 10.1080/17451000.2013.775458
Hylland K., Ruus A., Børseth J. F., Bechmann R., Barsiene J., Grung M., et al. (2006). Biomarkers in monitoring-a review.
Incardona J. P., Carls M. G., Day H. L., Sloan C. A., Bolton J. L., Collier T. K., et al. (2009). Cardiac arrhythmia is the primary response of embryonic pacific herring (Clupea pallasi) exposed to crude oil during weathering. Environ. Sci. Technol. 43, 201–207. doi: 10.1021/ES802270T/SUPPL_FILE/ES802270T_SI_004.PDF
Jonsson H., Björkblom C. (2011). Biomarker Bridges – Biomarker responses to dispersed oil in four marine fish species. Rep. IRIS - 2011/113. (IRIS Miljø). Available online at: https://norceresearch.brage.unit.no/norceresearch-xmlui/handle/11250/2631906.
Jörundsdóttir H.Ó., Jensen S., Hylland K., Holth T. F., Gunnlaugsdóttir H., Svavarsson J., et al. (2014). Pristine Arctic: Background mapping of PAHs, PAH metabolites and inorganic trace elements in the North-Atlantic Arctic and sub-Arctic coastal environment. Sci. Total Environ. 493, 719–728. doi: 10.1016/J.SCITOTENV.2014.06.030
Kuleshov M. V., Jones M. R., Rouillard A. D., Fernandez N. F., Duan Q., Wang Z., et al. (2016). Enrichr: a comprehensive gene set enrichment analysis web server 2016 update. Nucleic Acids Res. 44, W90–W97. doi: 10.1093/NAR/GKW377
Lakhmanov D. E., Kozhevnikov A. Y., Pokryshkin S. A., Semiletov I. P., Kosyakov D. S. (2022). Polycyclic aromatic hydrocarbons in the Siberian Arctic seas sediments. Mar. pollut. Bull. 180, 113741. doi: 10.1016/J.MARPOLBUL.2022.113741
Lee J. H., Wada T., Febbraio M., He J., Matsubara T., Lee M. J., et al. (2010). A novel role for the dioxin receptor in fatty acid metabolism and hepatic steatosis. Gastroenterology 139, 653–663. doi: 10.1053/j.gastro.2010.03.033
Li B., Dewey C. N. (2011). RSEM: Accurate transcript quantification from RNA-Seq data with or without a reference genome. BMC Bioinf. 12, 1–16. doi: 10.1186/1471-2105-12-323/TABLES/6
Liu Z., Liu J., Zhu Q., Wu W. (2012). The weathering of oil after the Deepwater Horizon oil spill: insights from the chemical composition of the oil from the sea surface, salt marshes and sediments. Environ. Res. Lett. 7, 35302. doi: 10.1088/1748-9326/7/3/035302
Ma Q. (2013). Role of Nrf2 in oxidative stress and toxicity. Annu. Rev. Pharmacol. Toxicol. 53, 401–426. doi: 10.1146/ANNUREV-PHARMTOX-011112-140320/1
Nahrgang J., Brooks S. J., Evenset A., Camus L., Jonsson M., Smith T. J., et al. (2013). Seasonal variation in biomarkers in blue mussel (Mytilus edulis), Icelandic scallop (Chlamys islandica) and Atlantic cod (Gadus morhua)—Implications for environmental monitoring in the Barents Sea. Aquat. Toxicol. 127, 21–35. doi: 10.1016/J.AQUATOX.2012.01.009
Nahrgang J., Camus L., Gonzalez P., Goksøyr A., Christiansen J. S., Hop H. (2009). PAH biomarker responses in polar cod (Boreogadus saida) exposed to benzo(a)pyrene. Aquat. Toxicol. 94, 309–319. doi: 10.1016/J.AQUATOX.2009.07.017
Nahrgang J., Camus L., Gonzalez P., Jönsson M., Christiansen J. S., Hop H. (2010). Biomarker responses in polar cod (Boreogadus saida) exposed to dietary crude oil. Aquat. Toxicol. 96, 77–83. doi: 10.1016/J.AQUATOX.2009.09.018
Nahrgang J., Granlund C., Bender M. L., Sørensen L., Greenacre M., Frantzen M. (2023). No observed developmental effects in early life stages of capelin (Mallotus villosus) exposed to a water-soluble fraction of crude oil during embryonic development. J. Toxicol. Environ. Heal. Part A 86, 404–419. doi: 10.1080/15287394.2023.2209115
Nevalainen M., Helle I., Vanhatalo J. (2017). Preparing for the unprecedented — Towards quantitative oil risk assessment in the Arctic marine areas. Mar. pollut. Bull. 114, 90–101. doi: 10.1016/J.MARPOLBUL.2016.08.064
Nong D., Countryman A. M., Warziniack T. (2018). Potential impacts of expanded Arctic Alaska energy resource extraction on US energy sectors. Energy Policy 119, 574–584. doi: 10.1016/J.ENPOL.2018.05.003
Norwegian Offshore Directorate. (2024). FactMaps. Available online at: https://factmaps.sodir.no/factmaps/3_0/ (Accessed 02.08.2024).
Pampanin D. M., Sydnes M. O. (2013). Polycyclic aromatic hydrocarbons a constituent of petroleum: presence and influence in the aquatic environment. Hydrocarbon. InTech. doi: 10.5772/48176
Robinson M. D., McCarthy D. J., Smyth G. K. (2010). edgeR: a Bioconductor package for differential expression analysis of digital gene expression data. Bioinformatics 26, 139–140. doi: 10.1093/BIOINFORMATICS/BTP616
Rothhammer V., Quintana F. J. (2019). The aryl hydrocarbon receptor: an environmental sensor integrating immune responses in health and disease. Nat. Rev. Immunol. 2019 193 19, 184–197. doi: 10.1038/s41577-019-0125-8
Schmittgen T. D., Livak K. J. (2008). Analyzing real-time PCR data by the comparative CT method. Nat. Protoc. 3, 1101–1108. doi: 10.1038/nprot.2008.73
Shen X., Chen Y., Zhang J., Yan X., Liu W., Guo Y., et al. (2019). Low-dose PCB126 compromises circadian rhythms associated with disordered glucose and lipid metabolism in mice. Environ. Int. 128, 146–157. doi: 10.1016/J.ENVINT.2019.04.058
Song Y., Nahrgang J., Tollefsen K. E. (2019). Transcriptomic analysis reveals dose-dependent modes of action of benzo(a)pyrene in polar cod (Boreogadus saida). Sci. Total Environ. 653, 176–189. doi: 10.1016/J.SCITOTENV.2018.10.261
Sørhus E., Incardona J. P., Furmanek T., Goetz G. W., Scholz N. L., Meier S., et al. (2017). Novel adverse outcome pathways revealed by chemical genetics in a developing marine fish. Elife 6, 1–30. doi: 10.7554/ELIFE.20707
Sørhus E., Incardona J. P., Karlsen Ø., Linbo T., Sørensen L., Nordtug T., et al. (2016). Crude oil exposures reveal roles for intracellular calcium cycling in haddock craniofacial and cardiac development. Sci. Rep. 6, 1–21. doi: 10.1038/srep31058
Stich H. F., Dunn B. P. (1980). The carcinogenic load of the environment: benzo(a) pyrene in sediments of arctic waters. Arctic. 33, 807–814. Available online at: http://www.jstor.org/stable/40509083.
Strople L. C., Vieweg I., Yadetie F., Odei D. K., Thorsen A., Karlsen O. A., et al. (2023). Spawning time in adult polar cod (Boreogadus saida) altered by crude oil exposure, independent of food availability. J. Toxicol. Environ. Heal. Part A. 1–24 doi: 10.1080/15287394.2023.2228535
Tairova Z., Frantzen M., Mosbech A., Arukwe A., Gustavson K. (2019). Effects of water accommodated fraction of physically and chemically dispersed heavy fuel oil on beach spawning capelin (Mallotus villosus). Mar. Environ. Res. 147, 62–71. doi: 10.1016/J.MARENVRES.2019.03.010
Tang C., Shen C., Zhu K., Zhou Y., Chuang Y. J., He C., et al. (2020). Exposure to the AhR agonist cyprodinil impacts the cardiac development and function of zebrafish larvae. Ecotoxicol. Environ. Saf. 201, 1–8. doi: 10.1016/J.ECOENV.2020.110808
Walsh S. J. (1996). Life history and ecology of long rough dab Hippoglossoides platessoides (F) in the Barents Sea. J. Sea Res. 36, 285–310. doi: 10.1016/S1385-1101(96)90797-2
Yadetie F., Brun N. R., Giebichenstein J., Dmoch K., Hylland K., Borgå K., et al. (2022). Transcriptome responses in copepods Calanus finmarchicus, Calanus glacialis and Calanus hyperboreus exposed to phenanthrene and benzo[a]pyrene. Mar. Genomics 65, 100981. doi: 10.1016/J.MARGEN.2022.100981
Yadetie F., Brun N. R., Vieweg I., Nahrgang J., Karlsen O. A., Goksøyr A. (2021). Transcriptome responses in polar cod (Boreogadus saida) liver slice culture exposed to benzo[a]pyrene and ethynylestradiol: insights into anti-estrogenic effects. Toxicol. Vitr. 75, 0–9. doi: 10.1016/j.tiv.2021.105193
Yadetie F., Zhang X., Hanna E. M., Aranguren-Abadía L., Eide M., Blaser N., et al. (2018). RNA-Seq analysis of transcriptome responses in Atlantic cod (Gadus morhua) precision-cut liver slices exposed to benzo[a]pyrene and 17α-ethynylestradiol. Aquat. Toxicol. 201, 174–186. doi: 10.1016/J.AQUATOX.2018.06.003
Keywords: capelin, long rough dab, American plaice, oil spill, aryl hydrocarbon receptor
Citation: Brun NR, Yadetie F, Liestøl HT, Karlsen OA and Goksøyr A (2024) Transcriptome responses to benzo[a]pyrene in liver slices of sub-arctic fish species. Front. Mar. Sci. 11:1425221. doi: 10.3389/fmars.2024.1425221
Received: 29 April 2024; Accepted: 29 August 2024;
Published: 19 September 2024.
Edited by:
Carlos Gravato, University of Lisbon, PortugalReviewed by:
David Hala, Texas A&M University at Galveston, United StatesCopyright © 2024 Brun, Yadetie, Liestøl, Karlsen and Goksøyr. This is an open-access article distributed under the terms of the Creative Commons Attribution License (CC BY). The use, distribution or reproduction in other forums is permitted, provided the original author(s) and the copyright owner(s) are credited and that the original publication in this journal is cited, in accordance with accepted academic practice. No use, distribution or reproduction is permitted which does not comply with these terms.
*Correspondence: Nadja R. Brun, bmFkamEuYnJ1bkB1aWIubm8=
†These authors share first authorship
Disclaimer: All claims expressed in this article are solely those of the authors and do not necessarily represent those of their affiliated organizations, or those of the publisher, the editors and the reviewers. Any product that may be evaluated in this article or claim that may be made by its manufacturer is not guaranteed or endorsed by the publisher.
Research integrity at Frontiers
Learn more about the work of our research integrity team to safeguard the quality of each article we publish.