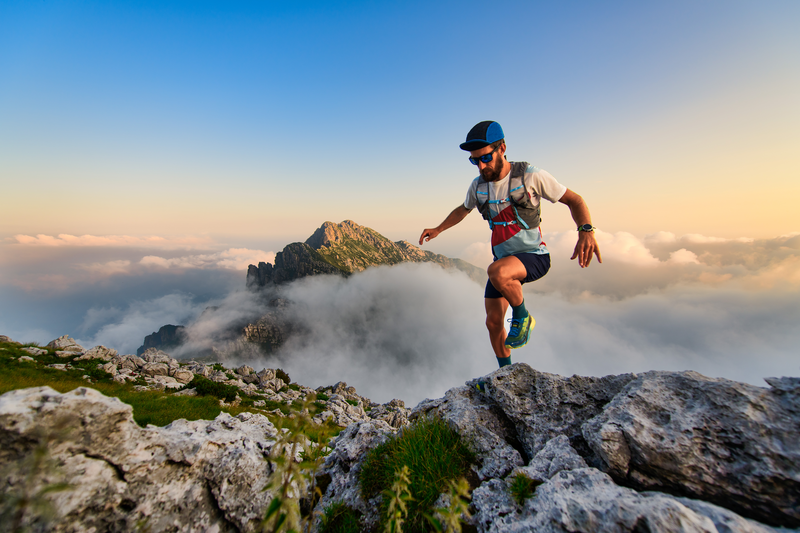
95% of researchers rate our articles as excellent or good
Learn more about the work of our research integrity team to safeguard the quality of each article we publish.
Find out more
ORIGINAL RESEARCH article
Front. Mar. Sci. , 05 September 2024
Sec. Marine Ecosystem Ecology
Volume 11 - 2024 | https://doi.org/10.3389/fmars.2024.1424638
This article is part of the Research Topic Understanding Interaction Among Diversity, Ecosystem Processes and Ocean & Human Health View all articles
This study investigates the biomass production and growth strategies of the relict seagrass Zostera marina, a species sensitive to high temperatures, within the Venice Lagoon (Italy). Monthly data collected from January to December 2019 and November 2020 to October 2021 in a single station in proximity of one lagoon port-entrance, along with seasonal data from 2018 and 2021 for the whole lagoon, were analyzed in conjunction with environmental parameters and macroalgal presence. The objective was to understand the impact of climate changes, particularly rising water temperatures, on the cover, standing crop and net primary production of Z. marina and the effect of the MoSE gate closures designed to protect Venice from high-water events. Despite expectations of a decline due to temperature increase and reduced water exchange, our findings reveal a notable adaptability of Z. marina, with increases in both cover and biomass. This resilience suggests that Z. marina can counteract environmental challenges, making this study relevant for broader ecological and conservation contexts beyond the Venice Lagoon.
Seagrasses, and more in general aquatic angiosperms, are one of the most important primary producers in coastal waters and transitional water systems, where their dominance is generally considered a good indicator of pristine-oligotrophic (undisturbed) or almost pristine environmental conditions (Orfanidis et al., 2003; Sfriso et al., 2007). On the contrary, the absence or replacement of angiosperm meadows by free-floating opportunistic macroalgae is a symptom of eutrophication and environmental degradation (Sfriso et al., 1987; Morand and Briand, 1996; Viaroli et al., 2008).
In the world 72 species of seagrasses are reported, compared to 5,000-6,000 species of seaweeds (https://www.wri.org/insights/understanding-seagrass).
Among them, Zostera is the most spread genus belonging to the class Monocotyledoneae, order Helobiae and family Zosteraceae. At present, Guiry and Guiry (2024) reported 5 accepted species names in the database (Algaebase.org) and the eelgrass Z. marina is the most widely distributed species, colonizing the intertidal and subtidal zones of shallow areas where it grows up to -12 m on the mean sea level (MSL), from temperate waters to northern Arctic regions, preferentially in muddy bottoms in the presence of clear waters (den Hartog, 1970; Short et al., 1993; Borum and Greve, 2004; Jarvis et al., 2012; Olesen et al., 2015).
However, since the 1900s, Z. marina populations were affected from a lot of anthropogenic impacts causing eutrophication and severe wasting diseases. In the 1930s the North Atlantic (the Great Bay Estuary, on the New Hampshire-Maine border) Z. marina populations were decimated by a massive epidemic of wasting disease, an infectious marine slimemold of genus Labyrinthula (Milne and Milne, 1951; Short et al., 1986; Gustafsson and Boström, 2014; Hughes et al., 2018). Then, since the 1960s it has recolonized, many areas characterized by water clarity, although other wasting diseases were recorded in 1980s and 1990s (Short et al., 1988; Burdick et al., 1993). However, other diseases caused by Aplanochytrium sp. affected Zostera populations also in more recent years, favored by herbicides and nitrate inputs which increased the susceptibility of Z. marina to infections (Hughes et al., 2018). In the late 1940s Wilson (1949) reported a strong Z. marina decrease due to macroalgal overgrowth whereas den Hartog (1994) found in Enteromorpha radiata J. Agardh blooms the decline of this species. In general, harmful algal blooms are considered to be one of the biggest threats to benthic vegetation (Gustafsson and Boström, 2014).
Z. marina is a relict species, present only in the boreal regions originated when the Tethys Sea covered much of the northern hemisphere (Setchel, 1935), with a wide North American and Eurasian distribution. In the past it was present also along the Italian coasts (Portal to the flora of Italy, 2023), even if it is currently in sharp decline or even disappearing almost everywhere due to eutrophication, pollution and water temperature increase. Some years ago Cecere et al. (2009) reported the presence of wide and dense populations of Z. marina in the lagoons of Venice and Marano-Grado. Sporadic populations were also recorded in some lakes of Sicily (Faro and Ganzirri), Sardinia (Santa Giusta, Tortoli, Boi Cerbus, Is Benas) (Cecere et al., 2009) and in the marine coats of the Gulf of Panzano (Monfalcone) in the northwestern Adriatic Sea (Curiel et al., 2021). In 2022-23 sods and rhizomes of Z. marina were also transplanted in some lagoons of the Po Delta in the framework of the still ongoing project Life TRANSFER (www.transfer.eu, Life 19 NAT/IT/000264) and small populations are now present in Caleri and Albarella, whereas other populations are expected to take root in Barbamarco and Goro.
The most extended eelgrass populations are present in the Venice Lagoon (Rismondo et al., 2003; Sfriso et al., 2007), where they have shown continuous changes over the time (Sfriso et al., 2022a). Indeed, before the 1960s-1970s, Z. marina, like other aquatic angiosperms, showed the highest distribution that drastically decreased in the 1980s during the period of excessive growth of macroalgae. In the following years it began to recolonize the lagoon but was hampered by the harvesting of the Manila clam Ruditapes philippinarum Adams & Reeve that disrupted the lagoon bottoms and resuspended large amounts of sediment increasing water turbidity (Sfriso et al., 2005b). When clam stocks declined, mainly due to overfishing, starting from the 2010s this eelgrass began to recolonize the lagoon.
The first map of Z. marina in the Venice Lagoon dates back to the early 1990s (Caniglia et al., 1990, 1992). Overall, in 1990 this species covered 36.4 Km2, of which 2.66 Km2 as pure populations and 33.8 km2 as mixed with N. noltei and C. nodosa. In 2002, this eelgrass displayed a cover of 34.4 km2, of which pure populations increased up to 22.0 Km2 (Sfriso et al., 2009).
In 2003, Sfriso and Facca (2007) mapped the presence of this species throughout the entire lagoon. Z. marina covered mostly the southern lagoon, especially the bottoms near Chioggia and some areas at north and south of the Malamocco-Marghera Canal, whereas it was negligible in the northern lagoon. The eelgrass covered approx. 26.1 Km2, accounting for a standing crop (SC) of 91 Ktonnes FWT and a net primary production (NPP) of 343 Ktonnes FWT (Sfriso et al., 2022a).
In the Venice Lagoon, the first studies on Z. marina morphology and production date back to February 1994-February 1995 (Sfriso and Marcomini, 1997, 1999; Sfriso and Ghetti, 1998; Zharova et al., 2001; Rigolett et al., 1999). In those investigations, the biomass variation and production of Z. marina, as well as of N. noltei and C. nodosa, were recorded monthly during one year in a southern lagoon station (Petta di Bò) (Figure 1).
In July 1998-June 1999, the growth of Z. marina was studied also in the central lagoon at Alberoni, a station near the Malamocco port-entrance (Sfriso et al., 2005a). Successively, the coverage of Z. marina was recorded by our team in 118 (in 2011) and 88 (in 2014, 2018 and 2021) stations, spread along the entire Lagoon (Mo. V.Eco. I, 2012, Mo.V.Eco. II, 2015, Mo.V.Eco. III, 2019, Mo.V.Eco. IV, 2022).
Climate changes, and in particular the increase of water temperatures, can severely affect the spread and growth of this species, putting its presence at risk. Among the Italian seagrasses, Z. marina is the less thermotolerant; indeed, the highest water temperatures tolerated by this cold-loving species are below 26-28°C (Höffle et al., 2011; Sfriso et al., 2019a), while above these temperatures it declines and dies.
This study aims to understand how Z. marina, a cold-loving seagrass species, responds to various environmental pressures. Our investigation mainly focused on two primary pressures: 1) the effect of different environmental parameters/macroalgal variables, especially the increase in water temperature due to climate changes; 2) the impact of the MoSE (Modulo Sperimentale Elettromeccanico, namely Experimental Electromechanical Module) mobile gates (autumn 2020-winter 2021), put into action in the three lagoon water inlets to contrast the Venice Lagoon high tide events.
By examining and comparing the obtained results, we aim to provide insights into the resilience and adaptability of Z. marina, with broader implications for ecological conservation.
The Venice Lagoon is a polyhedric shallow water body of approx. 549 Km2, located in the North-Western Adriatic Sea. It has a water surface of ca. 432 Km2, a mean depth of ca. 1.2 m, and a mean tidal excursion of ±31 cm (Figure 1).
The lagoon is connected to the sea through three large (400–900 m) and deep (15-20, up to 50 m) port-entrances, which allow a total water renewal of approx. 50% each tidal cycle (12 hr), although in the choked areas water renewal can take up to 40 days (Cucco and Umgiesser, 2006). The lagoon is subdivided into three hydrological basins, separated by Lido and Pellestrina watersheds that shift depending on tides and winds. They change with spring tides or neap tides, with higher tides in autumn and during strong wind events, and can move more than a kilometer, especially near the main channels. To better define basin boundaries, the present study refers to the three morphological basins as “northern”, “central” and “southern”, as depicted in Figure 1. The deep Malamocco-Marghera Canal marks the separation between the central and the southern basin, while Burano and Torcello tidal marshes divide the central and the southern ones.
The central basin has been the most studied for the multiple impacts of industrial waste, urban sewage and other anthropogenic pressures, such as commercial and touristic activities and the illegal harvesting of the Manila clam Ruditapes philippinarum Adams & Reeve by disruptive fishing gears (Pranovi and Giovanardi, 1994). Before the 1970s-1980s, it was mainly colonized by seagrasses and Ruppia cirrhosa. Then, between the 1980s and the early 2000s, aquatic angiosperms almost completely disappeared due to the trophic status increase and the abnormal growth of nuisance macroalgae, especially Ulva rigida C. Agardh (Sfriso et al., 1987). Z. marina started to recolonize the central basin only in the following years (Sfriso and Facca, 2007).
The northern basin, due to its high choked morphology and shallow waters, is characterized by low water exchanges. Seagrasses and R. cirrhosa completely disappeared in early 2000s (Sfriso and Facca, 2007) and started to recolonize the lagoon only in 2014. The recolonization was mostly due to the transplants of approx. 75.000 rhizomes with 1-3 shoots, carried out during the Life project SERESTO (Life12 NAT/IT/000331, www.lifeseresto.eu, Sfriso et al. (2021), and of approx. 26,000 rhizomes, during the project LAGOON REFRESH (Life 16 NAT/IT/000663, www.lagoonrefresh.eu).
The southern lagoon is the basin with the least anthropogenic impacts, lower pollutants and nutrient concentrations (Sfriso et al., 2005a, 2019); it has been always characterized by extensive populations of seagrasses, including Z. marina (Caniglia et al., 1990, 1992) which have changed little over time with the exception of the areas close to the salt marshes in the past densely colonized by N. noltei. Indeed, this species had almost disappeared due to the reconstruction of many artificial salt marshes, but it is currently showing a strong recovery (Sfriso et al., 2022a).
The standing crop (SC) and net primary production (NPP) of Z. marina have been measured on a temporal scale (24 monthly samples for the biomass and morphological parameters; 48 samples twice a month for the primary production) in a station: Ca’ Roman during 2019 and 2020-21, and on a spatial scale (88 stations and surrounding areas, in the entire lagoon in 2018 and 2021 (Figure 1).
The biomass (i.e. shoots and roots-rhizomes) of Z. marina was recorded by averaging 6 sub-samples collected within a frame of 20x25 cm (0.05 m2), according to the procedure of Sfriso and Ghetti (1998) that allows an accuracy >95%. Plants were collected with rhizomes in the first 10-15 cm of sediment top layer, washed in situ in a perforated thank (pore size approx. 1 cm) to eliminate sediment, benthic organisms and bivalve/gastropod shells, and stored in a refrigerator in plastic bags at 4°C, until the morphometric determinations occurred within 1-3 days. Before the analyses, samples were washed with tap water to eliminate salts, sorted in shoots, roots-rhizomes and blackened dead parts, dried with laboratory paper and weighed. The plant biomass was calculated as the sum of these three fractions (i.e. shoots, roots-rhizomes, blackened dead parts). At each monthly sampling, the 20 longest shoots were measured (precision 1 mm) to determine the maximum height of the prairie and the number of leaves per shoot.
The NPP was estimated twice a month by marking the leaf bundles above the leaf and rhizome meristems with a hole of 10 shoots/rhizomes and measuring the growth of leaves and rhizomes between subsequent samplings. The total production was obtained by the number of shoots per square meter. Results in cm per shoot and cm per rhizome were transformed into weight values by the weight determination of 10 linear meters of leaves and 1 meter of rhizomes.
The cover and SC of Z. marina were recorded by sampling 88 stations spread in the entire lagoon in 2018 and 2021 (Figure 1). Accurate monitoring of Z. marina presence around these stations, determined by using a GPS Garmin MAP78, allowed to draw its distribution in 4 cover (0-25, 25-50, 50-75, 75-100%) and weight ranges (0-1.5, 1.5-3.0, 3.0-4.5, 4.5-6.3 Kg FWT m-2) in a map with a scale 1:50,000, according to Sfriso and Facca (2007). The sum of the biomass of each range per its cover allowed to determine the mean and maximum SC. The use of the annual biomass Production/maximum annual Biomass ratio (P/B ratio), determined on yearly basis in many stations of the lagoon, allowed also to determine the total NPP.
Water column temperature (precision 0.1°C), water pH (pHw, precision 0.01 units), and water redox potential (Ehw, precision 1 mV) were determined by a portable Hanna pHmeter (mod. HI98190, Hanna Instruments Italia srl). Oxygen concentration was measured by a portable dissolved oxygen meter Oxi 3310 (Wissenschaftlich-Technische Werkstätten GmbH, Weilheim, Germany). Water depth and water transparency were measured by a Secchi Disk.
Water samples were obtained by mixing six sub-samples of the entire water column collected with a handmade bottle (diameter 4 cm, height 150 cm), and 0.25-1.0 L of the mixed sample were filtered with a Swinnex filter holder through Whatman GF/F glass microfiber filters (porosity 0.7 µm). Filters and approx. 250 ml of filtered water samples were stored at -20°C for nutrient (RP = reactive phosphorus; SiO44- = silicate, DIN = sum of ammonium, NH4+, nitrite: NO2-, nitrate: NO3-) and Chlorophyll-a (Chl-a) - Phaeophytin-a (Phaeo-a) determination, according to Strickland and Parsons (1984). Two water sub-samples (250-500 ml) were filtered through GF/F glass microfiber filters, after desiccation at 130°C for one hour, for the determination of total suspended solids (TSS). Filters were stored frozen until the laboratory analysis, after washing with Milli-Q water to remove salts. Additional 20 ml sub-samples were retained for the laboratory determination of salinity by titration method following Oxner (1962).
Three cores of surface sediments were collected with a Plexiglas corer (i.d. 10 cm), retaining the first 5 cm top-layers that were carefully mixed in a tank. Sediment pH (pHs) and redox potential (Ehs) were immediately measured with another portable Hanna pH-meter, only used for sediment measurements. Two sub-samples of 50-100 ml were kept frozen for the analyses of the main sediment parameters (i.e. Fines: fraction <63 µm, density, moisture) and the concentration of nutrients [i.e. total phosphorus (Ptot), inorganic phosphorus (Pinorg), organic phosphorus (Porg), total nitrogen (Ntot), total carbon (Ctot), inorganic carbon (Cinorg) and organic carbon (Corg)].
Sediment density was obtained in laboratory after sediment desiccation at 110°C in tared crucibles of approx. 30 ml. Fine percentage was determined by wet sieving approx. 50 g of dried sediment throughout Endecotts sieves (ENCO Scientific Equipment, Spinea, Italy). All analyses were performed in duplicate.
Total phosphorus (Ptot) was determined after 2 hours combustion at 550°C of 0.3-0.4 g of pulverized dry sediment, followed by 30 minute sonication of the combusted sample in 50 ml of 1N HCl. After making the sample to settle for at least an hour, 0.5 ml of the supernatant were taken with a graduated gas-chromatographic syringe and diluted to exactly 10 ml in a volumetric flask, in order to have a final dilution of 1 liter and the results expressed directly in µM. Phosphorus concentration was determined spectrophotometrically at 885 nm following Aspila et al. (1976). Inorganic phosphorus (Pinorg) was obtained with the same procedure used for Ptot, but without combustion at 550°C, whereas the concentration of organic phosphorus (Porg) was obtained by difference. All analyses were replicated twice in different days and values were considered reliable when the coefficient of variation (standard deviation/mean) was <5%.
The settled particulate matter (SPM) was obtained by pyramidal sedimentation traps (base: 20x20 cm, heigh 10 cm, mouth 15 cm), covered with a net to avoid fish entering and placed on the bottoms according to Sfriso et al. (2005b). Traps were emptied monthly and volumetric samples were retained for the weight determination and processed in order to have monthly and daily sedimentation rates per square meter, regardless of the sampling days.
The time-scale associations of 7 Z. marina variables (i.e. weight of dead parts, rhizomes, shoots, number and height of shoots, elongation of shoots and rhizomes) with 15 water parameters (i.e. RP, NH4+, NO3-, NO2-, SiO44-, pHw, Ehw, Chl-a, Phaeo-a, TSS, DO, Salinity, Temperature, Depth, Transparency), 11 sediment parameters (i.e. Pinorg, Porg, Cinorg, Corg, Ntot, pHs, Ehs, density, moisture, porosity, Fines), SPM and 5 macroalgal variables (i.e. macroalgal cover and biomass, number of macroalgal taxa, sensitive and calcareous species), recorded monthly at Ca’ Roman in 2019 and 2020-21 (24 samples) were obtained by applying the Principal Components Analysis (PCA).
The PCA analysis was also applied to the same 15 water parameters and the 5 macroalgal variables and to 8 sediment parameters (i.e. Pinorg, Porg, Cinorg, Corg, Ntot, density, moisture, porosity) recorded in 88 stations spread across the entire lagoon in 2018, in order to highlight those most closely associated with the presence/absence of the eelgrass although results can’t always prove cause and effect. In both cases the associations with Z. marina were highlighted by plotting the first two components on a plane.
Figure 2 shows the Z. marina monthly variation in biomass, shoot number and shoot height per square meter on an annual basis at Ca’ Roman.
The mean biomass recorded during the two years ranged from 2399 ± 993 (2019) to 3500 ± 1198 g FWT m-2 (2020-21), with peaks up to 5929 g FWT m-2. The biomass of shoots and rhizomes was quite similar in 2019, whereas rhizomes prevailed on shoots in 2020-21. On average, the biomass of the blackened and dead parts of shoots and rhizomes in 2019 (474 g FWT m-2) was two-fold higher than in 2020-21 (240 g FWT m-2). Plant height was very similar, ranging from 35.2 ± 8.5 to 39.2 ± 6.3 cm, with mean peaks up to 51.2 cm and single shoots up to 1 m high, whereas the number of shoots per square meter ranged between 696 ± 291 and 974 ± 317.
Figure 3 shows the monthly growth of shoots and rhizomes during the two sampling years. During 2020-21 the mean plant growth was higher than in 2019. On average, the mean growth was 2.73 (2019) - 3.27 cm day-1 (2020-21) per shoot and 0.077 (2019) - 0.090 cm day-1 (2020-21) per rhizome. Growth peaks were recorded in May, with 5.69-6.83 cm day-1 (for shoots) and 0.22-0.23 cm day-1 (for rhizomes), whereas the lowest growth values were on average recorded in August-September.
On a wet weight basis, the annual production accounted for 12,981 and 20,677 g FWT m-2 y-1 in 2019 and 2020-21, respectively. Shoots represented the 83.7-85.9% of total production, whereas rhizomes only the 14.1-16.3%. The annual biomass Production/maximum annual Biomass (P/B) ratio ranged from 2.69 in 2019 to 3.49 in 2020-21.
Z. marina colonized all the three lagoon basins (Figure 4), especially the deepest waters and the areas close to the main canals. Indeed, the highest cover was recorded in the southern and central lagoon, around the main canals (Malamocco-Marghera Canal and Poco Pesce Canal) that connect these basins with Malamocco and Chioggia port-entrances, respectively, whereas the presence of this species in the northern basin was negligible. However, in 2021 prairies were more extensive than in 2018, especially in the southern basin (Table 1).
Table 1. Distribution of the biomass in ranges, mean and maximum biomass values, cover, standing crop and NPP of Z. marina in the entire lagoon in 2018 and 2021.
The values of SC and NPP in the whole lagoon indicated that Z. marina colonized 38.2 Km2 in 2018 and had a significant increase in 2021, when the plant cover reached 49.4 Km2. The increase was mostly due to the colonization of new areas, where plant density had been not so high yet, as shown by the surface increase in the cover ranges between 0 and 3 Kg FWT m-2. However, because of a slight reduction in the highest range, the SC and NPP increases in 2021 were almost negligible, changing from 145 and 545 Ktonnes (FWT) in 2018 to 148 and 564 Ktonnes (FWT) in 2021, respectively, for the SC and NPP.
The principal component analysis (PCA) between different Z. marina variables and environmental parameters of water column, surface sediments and macroalgal variables recorded inside the same station at Ca’ Roman in 2019 and 2020-21, showed 11 components explaining 91.3% of the total variance. The first two components (variance 31%) are plotted in a plane (Figure 5). Results show two main groups of parameters/variables. On the left the first one highlights the strong associations between Zostera variables and temperature, pHs, pHw, Chl-a, sediment density, the number of sensitive and calcareous macroalgal taxa. On the right the second one shows the parameters that hinder eelgrass growth: i.e. sediment moisture, sediment porosity, DO, salinity and nutrient concentrations in water column. The other parameters showed intermediate patterns.
Figure 5. Principal component plot of Z. marina variables (dark green), nutrient parameters (black) and macroalgal variables (light green) at Ca’ Roman in 2019 and 2020-21. AlgBiom, Macroalgal Biomass; AlgCalc, Calcareous Macroalgae; AlgCov, Macroalgal Cover; AlgSens, Sensitive Macroalgae; AlgTot, Total Macroalgal Taxa; DO, Dissolved Oxygen; Chl-a, Chlorophyll-a; Cinorg, Inorganic Carbon; Corg, Organic Carbon; DeadPWT, Dead Part Weight; Moist., Moisture; Ntot, Total Nitrogen; Pinorg, Inorganic Phosphorus; Porg, Organic Phosphorus; RhizGr, Rhizome Growth; Poros., porosity; SDens, Sediment Density; pHs, Sediment pH; pHw, Water pH; Sal., Salinity; ShootGr, Shoot Growth; ShootH, Shoot Height; ShootN°, Shoot Number; ShootWT, Shoot Weight; Temp, Water temperature; Transp., water transparency; TSS, Total Suspended Solids. On the left, in the blue circle, parameters/variables associated to Z. marina variables, on the right, in the pink circle, parameters/variables that counteract its growth.
The PCA analysis of the data collected in late spring-early summer 2018 throughout the entire lagoon, with and without the presence of the eelgrass, is reported in Figure 6. Nine components explained a total variance of 73%. The plot of the first two components (variance 37%) highlighted two main groups. On the right, the presence of Z. marina was associated with sensitive, calcareous and total macroalgal taxa and in a minor extent with algal biomass and algal cover. Among the environmental parameters water transparency, DO, pHw, Ehw salinity, Pinorg and sediment density played a key role to explain the eelgrass presence. On the left, all the parameters that counteract its presence are grouped: i.e. nutrient concentrations in the water column (RP, NH4+, NO3-, NO2-, SiO44-), in surface sediments (Porg, Corg, Ntot, Cinorg), Chl-a, Phaeo-a, TSS, temperature, sediment porosity and moisture.
Figure 6. Principal component plot of Z. marina presence with water and sediment parameters and macroalgal variables in late spring – early summer 2021. On the right, in the blue circle, parameters/variables associated to Z. marina presence, on the left, in the pink circle, parameters/variables that counteract its presence. see Figure 5.
This study analyses the growth of Z. marina in a station where it forms a dense population and the presence/absence of this eelgrass throughout the entire lagoon to investigate both the environmental parameters and macroalgal variables that could affect its growth and its presence/absence.
In the Venice Lagoon, Z. marina, in contrast to many papers reported in literature (Short et al., 1988; Burdick et al., 1993; Wilson, 1949; den Hartog, 1994), is showing a significant recruitment. Indeed, the comparison between the data on Z. marina distribution, biomass and NPP obtained in this study (2018 and 2021) and the situation recorded in 2003 (Figure 7; Table 2) shows a strong increase in the spread and production of this species, especially in the central and in northern lagoon basins. This is probably due to the strong reduction of the trophic level and harvesting of the Manila clam Ruditapes philippinarum (Sfriso et al., 2005a; Sfriso et al., 2019b).
Table 2. Comparison between Z. marina cover, SC and NPP in 2003, 2018 and 2021 in the entire lagoon.
Between 2003 and 2018, the colonization of this species increased from 26.1 to 38.2 Km2, and in 2021 it even reached 49.4 Km2 (Table 2). In 2003, Z. marina was completely absent in the northern basin, showing only few populations in the central lagoon. In 2018, it colonized 2.91 and 12.7 Km2, in the northern and central basins respectively, increasing to 3.69 and 14.1 Km2 in 2021. Consequently, SC and NPP markedly increased from 2003 to 2018 and continued slightly to increase also in 2021. These data are in contrast with the expectation of the eelgrass decline in 2018 and 2021, based on the water temperature increase (Vilibić et al., 2019; Bonacci et al., 2021), and in 2021 also as a possible consequence of MoSE gate closures (Ghezzo et al., 2010; Baldassarre et al., 2023), which reduced the water exchange between the Venice Lagoon and the Northern Adriatic Sea.
Indeed, although water temperature shows a general increasing trend, in the Venice Lagoon the temperature changes have been observed mostly in winter (Amos et al., 2017), since no more frost events in surficial waters have occurred. Z. marina is a cold-loving species, which starts the production of new shoots at 5°C (Zharova et al., 2001) and shows a generative growth at 15-20°C (Rasmussen, 1973; Zharova et al., 2001), while its growth is inhibited above 25°C (Wetzel and Neckles, 1986). Therefore, the summer decrease of the eelgrass is usually followed by a winter recovery, which allows for a greater plant growth at temperatures higher than 5-6°C.
In addition, in the last 30 years, the lagoon has suffered of intense subsidence and eustatism phaenomena (Carbognin et al., 2004; Zanchettin et al., 2021) and has been affected by strong sediment erosion and grain-size changes, due to clam harvesting (Aspden et al., 2004; Sfriso et al., 2005b) and to wave motion caused by an increased naval traffic (Rapaglia et al., 2015; Parnell et al., 2016; Scarpa et al., 2019). Consequently, in many lagoon areas (e.g., in the bottoms around the Malamocco-Marghera Canal), water depth has markedly increased (Carniello et al., 2009), favouring the colonization of Z. marina. In fact, this species prefers deeper and cooler waters and colonizes the canal edges affected by high water renewal, disregarding choked and shallow areas where water temperature can easily exceed 30°C.
Finally, the closures of MoSE mobile gates, located at the three lagoon port-entrances to actively prevent flooding threats, began on October 2020 and, in Autumn 2020-Winter 2021, these barriers were raised 20 times, even for two days in a row (www.mosevenezia.eu/il-mose-in-funzione). A concern regarding MoSE activation was that, by reducing lagoon turnover, the gate closures would have had a negative impact on lagoon seagrass meadows. Instead, a study carried out in 2019, before MoSE activation, and in 2020-21, during the first closures, highlighted that autumn-winter interventions had no impact on seagrass growth (Mazzoldi and Sfriso, 2022). Indeed, in 2020-21, Z. marina has continued the positive trend observed between 2003 and 2018, further increasing its cover and biomass production.
The PCA analysis of environmental parameters that could impact on Z. marina growth at Ca’ Roman (Figure 5), where water temperature was never too high (max 26-27°C) has indicated that this is the parameter mostly associated with all the considered Z. marina variables (i.e. shoot/rhizome biomass, shoot number, shoot height and, shoot/rhizome growth), but also with the number of sensitive and calcareous macroalgae (i.e. Pneophyllum Kützing, Hydrolithon (Foslie) Foslie and Melobesia J.V. Lamouroux) which usually grow as epiphytes on the older plant leaves and on the thalli of other macroalgae). Positive associations were also recorded with high pHs values, low Chl-a concentrations and high sediment density. In contrast, lower values of salinity, Ehw, Ehs, DO, higher concentrations of nitrogen (i.e. NH4+, NO2-, NO3-), RP, SiO44- and Fines and high sediment moisture and porosity counteracted the growth of this eelgrass.
Instead, the PCA analysis to determine the parameters/variables which could favor the presence/absence of Z. marina in the extremely different environmental conditions recorded throughout the Venice Lagoon highlighted the significant role played by high water transparency, pHw and sediment density for eelgrass population development, in opposition to water temperature, RP, sediment moisture and nutrient concentrations in the water column and surface sediments (Figure 6), as previously reported also by Sfriso et al. (2023). In addition, the presence and abundance of Z. marina resulted strongly associated with the total number of macroalgae, especially with calcareous and sensitive taxa. Conversely, phytoplankton concentration (recorded as Chl-a and Phaeo-a), TSS and macroalgal cover had a negative impact on Z. marina populations.
The negative effect of water turbidity has recently been reported also by Li et al. (2021), who considered this parameter as the primary cause of seagrass meadow loss. Those authors found, in fact, that short-term periods of increased water turbidity led to long-term effects on Z. marina survival. Similarly, Wong et al. (2021) found that reduced light availability is a leading cause of seagrass declines worldwide. In particular, based on laboratory experiments, they found a chronic decline in shoot density and biomass, especially at 60 and 80% of pulsed light shading for periods of approx. ten days; when light availability was restored, reduction in shoot density, biomass, and morphology remained. Therefore, prolonged periods of phytoplankton and/or macroalgal blooms can severely affects Z. marina populations or even make it to completely disappear.
Similar results were recorded by Gustafsson and Boström (2014) who considered harmful algal blooms, triggered by eutrophication, one of the biggest threats to angiosperm vegetation. Indeed, the presence of algal mats significantly reduced eelgrass shoot and root biomass production and affected the leaf growth negatively. Hauxwell et al. (2001) found that a loss of Z. marina habitat from temperate estuaries worldwide often coincides with increased macroalgal accumulations, especially macroalgal canopy height, resulting from increased delivery of anthropogenic nitrogen affecting density, recruitment, growth rate, and primary production. The removal of macroalgae triggered an increase in shoot density, in summer growth (+55%), and in summer aboveground net production (+500%). Therefore, eelgrass recovery upon removal of macroalgae may be possible.
This is what happened in the 1980s in the Venice Lagoon, when the increase of nutrient availability and the consequent production of abnormal biomasses of nuisance macroalgae (especially Ulva rigida) lasted 2-3 months, often ending with the macroalgal complete degradation and the related triggering of anoxic crises and seagrass disappearance (Sfriso et al., 1987). In contrast, the decline of both nutrient concentrations and macroalgal biomass recorded since the early 1990s favored an eelgrass recruitment.
Due to the increase in global water temperatures, Zostera marina, a Tethis Sea relict cold-loving species only present in the boreal North American and Eurasian regions, is considered the most endangered seagrass in the Mediterranean Sea.
This study on eelgrass growth in a station with a dense prairie and on its presence/absence throughout the Venice Lagoon, simultaneously considering the most common environmental parameters and macroalgal variables that could counteract/trigger its presence and growth, indicates water transparency and water temperature as opposite key-parameters regulating the Z. marina presence/absence and growth. Indeed, the increase in water transparency, due to the reduction of phytoplankton (Bernardi-Aubry et al., 2021) and sediment resuspension, caused in turn by the drastic reduction in clam harvesting, and the significant decline of nutrients and nuisance macroalgae (Sfriso et al., 2019b) have favored Z. marina colonization on the deeper bottoms and the edges of the main canals, where water temperature is usually lower than in shallow areas.
In addition, the activation of MoSE gates to protect the Venice historical center from exceptional high tides, causing the reduction of water exchanges with the sea and raising concerns about the risk of a drastic decline in the eelgrass populations had no impact on Z. marina populations because, at present, closures occur in autumn-winter when water temperatures are low. On the contrary, Z. marina has shown unexpected adaptability by increasing both the coverage and the NPP since 2003, due to the decrease of nutrient concentrations and the Manila clam harvesting.
Therefore, this species has proven to react quickly to the reduction of anthropogenic impacts and the reduction of the trophic status in coastal areas can be a concrete solution not only for the recovery of good/high environmental conditions and to favour the general colonization by seagrasses (Sfriso et al., 2021), but also to increase the spread of the species more sensitive to water temperature, fostering plant rooting in areas before precluded to the presence of these taxa.
The raw data supporting the conclusions of this article will be made available by the authors, without undue reservation.
AS: Conceptualization, Data curation, Funding acquisition, Investigation, Methodology, Project administration, Validation, Writing – original draft. AB: Formal analysis, Writing – review & editing. YT: Formal analysis, Writing – review & editing. GS: Formal analysis, Writing – review & editing. MW: Formal analysis, Supervision, Writing – review & editing. KS: Formal analysis, Supervision, Writing – review & editing. AAS: Formal analysis, Investigation, Supervision, Writing – review & editing.
The author(s) declare financial support was received for the research, authorship, and/or publication of this article. The research and the publication of this paper was in part supported by ARPA Veneto (Regional Agency for Environmental Prevention and Protection of Veneto). Technical -scientific collaboration agreement pursuant to ART. 215 of law 2241/1990 for the definition of the status of Biological Quality Elements in the Venetian lagoons according to European Directive 2000/60/CE. Funding awarded with D.C.R.V. n. 81 of 07.21.2021
The recent scientific activity was performed in the framework of the Research Programmes: “Venezia 2021, coordinated by CORILA (Consortium for coordination of research activities concerning the Venice lagoon system), with the contribution of the Provveditorato for the Public Works of Veneto, Trentino Alto Adige and Friuli Venezia Giulia” and “Mo.V.Eco. I, II, III, IV (Ecological monitoring of the Venice Lagoon), between ARPA (Regional Agency for Environmental Protection) Veneto and Ca’ Foscari University of Venice - Department of Environmental Sciences, Informatics and Statistics”. The authors thank the reviewers who contributed to the improvement of the work with their observations and suggestions.
The authors declare that the research was conducted in the absence of any commercial or financial relationships that could be construed as a potential conflict of interest.
The author(s) declared that they were an editorial board member of Frontiers, at the time of submission. This had no impact on the peer review process and the final decision.
All claims expressed in this article are solely those of the authors and do not necessarily represent those of their affiliated organizations, or those of the publisher, the editors and the reviewers. Any product that may be evaluated in this article, or claim that may be made by its manufacturer, is not guaranteed or endorsed by the publisher.
Amos C. L., Umgiesser G., Ghezzo M., Kassem H., Ferrarin C. (2017). Sea surface temperature trends in venice lagoon and the adjacent waters. J. Coast. Res. 33, 385–395. doi: 10.2112/JCOASTRES-D-16-00017.1
Aspden R. J., Vardy S., Perkins R. G., Davoidson I. R., Bates R., Paterson D. M. (2004). The effect of clam fishing on the properties of surface sediments in the lagoon of Venice, Italy. Hydrol. Earth Syst. Sci. 8, 160–169. doi: 10.5194/hess-8-160-2004
Aspila K. I., Agemian H., Chau A. S. J. (1976). A semi-automated method for the determination of inorganic, organic and total phosphorus in sediments. Analyst 101, 187–197. doi: 10.1039/an9760100187
Baldassarre L., Natali V., De Pascale F., Vezzi A., Banchi E., Bazzaro M., et al. (2023). The impact of MOSE (Experimental electromechanical module) flood barriers on microphytobenthic community of the venice lagoon. Microorganisms 11, 936. doi: 10.3390/microorganisms11040936
Bernardi-Aubry F., Acri F., Finotto S., Pugnetti A. (2021). Phytoplankton dynamics and water quality in the venice lagoon. Water 13, 2780. doi: 10.3390/w13192780
Bonacci O., Bonacci D., Patekar M., Pola M. (2021). Increasing trends in air and sea surface temperature in the central adriatic sea (Croatia). J. Mar. Sci. Eng. 9, 358. doi: 10.3390/jmse9040358
Borum J., Greve T. M. (2004). “The four European seagrass species,” in European seagrasses: an introduction to monitoring and management. EU project Monitoring and Managing of European Seagrasses (M&MS) EVK3-CT-2000-00044. (Oostende, Belgium: European Project).
Burdick D. M., Short F. T., Wolf J. (1993). An index to assess and monitor the progression of wasting disease in eelgrass Zostera marina. Mar. Ecol.-Prog. Ser. 94, 83–90. Available at: http://www.int-res.com/articles/meps/94/m094p083.pdf.
Caniglia G., Borella S., Curiel D., Nascimbeni P., Paloschi F., Rismondo A., et al. (1992). Distribuzione delle fanerogame marine Zostera marina L., Zostera noltii Hornem, Cymodocea nodosa (Ucria) Asch. in Laguna di Venezia. Lav. Soc Ven. Sc. Nat. 17, 137–150.
Caniglia G., Borella S., Curiel D., Nascimbeni P., Paloschi F., Scarton F., et al. (1990). Cartografia computerizzata delle fanerogame marine della laguna di Venezia (Bollettino dell’A.I.C), 81–8, 129-132.
Carbognin L., Teatini P., Tosi L. (2004). Eustacy and land subsidence in the Venice Lagoon at the beginning of the new millennium. J. Mar. Syst. 51, 1–4, 345-353. doi: 10.1016/j.jmarsys.2004.05.021
Carniello L., Defina A., D’Alpaos L. (2009). Morphological evolution of the Venice lagoon: Evidence from the past and trend for the future. J. Geophys. Res. 114, F04002. doi: 10.1029/2008JF001157
Cecere E., Petrocelli A., Izzo G., Sfriso A. (2009). Flora and Vegetation of the Italian Transitional Water Systems (Multigraf, Spinea (Italy: CoRiLa).
Cucco A., Umgiesser G. (2006). Modeling the Venice Lagoon residence time. Ecol. Model. 193, 34–51. doi: 10.1016/j.ecolmodel.2005.07.043
Curiel D., Pavelić S. K., Kovačev A., Miotti C., Rismondo A. (2021). Marine seagrasses transplantation in confined and coastal Adriatic environments: Methods and results. Water 13, 2289. doi: 10.3390/w13162289
den Hartog C. (1994). Suffocation of a littoral Zostera bed by Enteromorpha radiata. Aquat. Bot. 47, 21–28 21. doi: 10.1016/0304-3770(94)90045-0
Ghezzo M., Guerzoni S., Cucco A., Umgiesser G. (2010). Changes in venice lagoon dynamics due to construction of mobile barriers. Coast. Eng. 57, 694–708. doi: 10.1016/j.coastaleng.2010.02.009
Guiry M. D., Guiry G. M. (2024). AlgaeBase. World-wide electronic publication, University of Galway. https://www.algaebase.org (accessed May 5, 2024).
Gustafsson C., Boström C. (2014). Algal mats reduce eelgrass (Zostera marina L.) growth in mixed and monospecific meadows. J. Exp. Mar. Bio. Ecol. 461, 85–92. doi: 10.1016/j.jembe.2014.07.020
Hauxwell J. H., Cebrian J., Furlong C., Valiela I. (2001). Macroalgal canopies contribute to eelgrass (Zostera marina) decline in temperate estuarine ecosystems. Ecology 82, 1007–1022. doi: 10.1890/0012-9658(2001)082[1007:MCCTEZ]2.0.CO;2
Höffle H., Thomsen M. S., Holme M. (2011). High mortality of Zostera marina under high temperature regimes but minor effects of the invasive macroalgae Gracilaria vermiculophylla. Estuar. Coast. Shelf Sci. 92, 35–96. doi: 10.1016/j.ecss.2010.12.017
Hughes R. G., Potouroglou M., Ziauddin Z., Nichol J. C. (2018). Seagrass wasting disease: Nitrate enrichment and exposure to a herbicide (Diuron) increases susceptibility of Zostera marina to infection. Mar. pollut. Bul. 134, 94–98. doi: 10.1016/j.marpolbul.2017.08.032
Jarvis J. C., Moore K. A., Kenworthy W. J. (2012). Characterization and ecological implication of eelgrass life history strategies near the species’ southern limit in the western North Atlantic. Mar. Ecol. Prog. Ser. 444, 43–56. doi: 10.3354/meps09428
Li C., Zhang Y. H., Wu X. X., Jiang Y. S., Li W.-T., Zhang P. D. (2021). Changes in survival and growth in response to different combinations of turbidity and duration in eelgrass Zostera marina plants. Estuar. Coast. Shelf Sci. 249, 107108. doi: 10.1016/j.ecss.2020.107108
Life12 NAT/IT/000331 -SERESTO- Habitat 1150* (Coastal lagoon) recovery by SEagrass RESTOration. A new strategic approach to meet HD & WFD objectives. Available online at: www.lifeseresto.eu.
Life16 NAT/IT/000663 - LAGOON REFRESH - Coastal Lagoon habitat, (1150*) and species recovery by REstoring the salt gradient increasing FRESH water input. Available online at: www.lagoonrefresh.eu.
Life19 NAT/IT/000264 -TRANSFER- seagrass TRANSplantation For transitional Ecosystem Recovery. Available online at: www.transfer.eu.
Mazzoldi C., Sfriso A. (2022). Scientific Research Program for a “Regulated” Lagoon. Line 3.3 Primary Production, Microbial, Benthic, planktonic and nektonic communities in the lagoon. VII Technical-Scientific Report, Period 01/07/2021 – 31/12/2021. CORILA (Consortium for Coordination of Research Activities Concerning the Venice Lagoon System), Provv. OOPP (Provveditorato for the Public Works of Veneto, Trentino Alto Adige and Friuli Venezia Giulia). (Venice, Italy), 1–103.
Milne L. J., Milne M. J. (1951). The eelgrass catastrophe. Sci. Am. Division. Nat. Scient. Am. 184, 52–55.
Morand P., Briand X. (1996). Excessive growth of macroalgae: A symptom of environmental disturbance. Bot. Mar. 39, 491–516. doi: 10.1515/botm.1996.39.1-6.491
Mo. V.Eco. I (2012). Monitoring plan for the Venice lagoon pursuant to Directive 2000/60/EC aimed at defining the ecological status (Legislative Decree N. 152/2006 s.m.i). Line 2: EQB: Macrophytes, Final Report, pp. 23 + Tables and Figures. (Venice, Italy).
Mo.V.Eco. II (2015). Monitoring plan for the Venice lagoon pursuant to Directive 2000/60/EC aimed at defining the ecological status (Legislative Decree N. 152/2006 s.m.i). EQB: Macrophytes, Final Report, pp. 27 + Tables and Figures. (Venice, Italy).
Mo.V.Eco. III (2019). Technical-scientific collaboration agreement pursuant to art. 15 of Law 241/1990 for the definition of the state of biological quality elements in the Venice Lagoon according to European Directive 2000/60/EC, EQB: Macrophytes, Final Report, pp. 26 + Tables and Figures. (Venice, Italy).
Mo.V.Eco. IV (2022). Technical-scientific collaboration agreement pursuant to art. 15 of Law 241/1990 for the definition of the state of biological quality elements in the Venice Lagoon according to European Directive 2000/60/EC, EQB: Macrophytes, Final Report, pp. 19 + Tables and Figures. (Venice, Italy).
Olesen B., Krause-Jensen D., Marbà N., Christensen P. B. (2015). Eelgrass Zostera marina in subarctic Greenland: dense meadows with slow biomass turnover in cold waters. Mar. Ecol. Prog. Ser. 518, 107–121. doi: 10.3354/meps11087
Orfanidis S., Panayotidis P., Stamatis N. (2003). An insight to the ecological evaluation index (EEI). Ecol. Indic. 3, 27–33. doi: 10.1016/S1470-160X(03)00008-6
Oxner M. (1962). The Determination of chlorinity by the Knudsen method and hydrographical tables (New York: G.M. Manufacturing Co), 63.
Parnell K. E., Zaggia L., Soomere T., Lorenzetti G., Scarpa G. M. (2016). Depression waves generated by large ships in the Venice Lagoon. J. Coast. Res. 75, 907–911. doi: 10.2112/SI75-182.1
Portal to the flora of Italy (2023). Available online at: https://dryades.units.it/florItaly (Accessed March 20, 2023).
Pranovi F., Giovanardi O. (1994). The impact of hydraulic dredging for short-necked clams, Tapes spp., on an infaunal community in the lagoon of Venice. Sci. Mar. 58, 345–353.
Rapaglia J., Zaggia L., Parnell K., Lorenzetti G., Vafeidis A. T. (2015). Ship-wake induced sediment remobilization: Effects and proposed management strategies for the Venice Lagoon. Ocean. Coast. Manag. 110, 1–11. doi: 10.1016/j.ocecoaman.2015.03.002
Rasmussen E. (1973). Systematics and ecology of the Isefjord marine fauna (Denmark). Ophelia 11, 1–495. doi: 10.1080/00785326.1973.10430115
Rigollet V., Laugier T., De Casabianca M. L., Sfriso A., Marcomini A. (1999). Seasonal biomass and nutrient dynamic of Zostera marina L. biomass in two mediterranean lagoons: Thau (France) and Venice (Italy). Bot. Mar. 41, 167–179. doi: 10.1515/botm.1998.41.1-6.167
Rismondo A., Curiel D., Scarton F., Mion D., Caniglia G. (2003). “A new seagrass map for the Venice lagoon,” in Proceedings of the Sixth International Conference on the Mediterranean Coastal Environment, MEDCOAST 2003, vol. Vol.II . Ed. Özhan E. (Ravenna, Italy: University of Ravenna), 843–852.
Scarpa G. M., Zaggia L., Manfè G., Lorenzetti G., Parnell K., Soomere T., et al. (2019). The effects of ship wakes in the Venice Lagoon and implications for the sustainability of shipping in coastal waters. Sci. Rep. 9, 19014. doi: 10.1038/s41598-019-55238
Setchel W. A. (1935). Geographic elements of the marine flora of the North Pacific Ocean. Am. Nat. 69, 560–577. doi: 10.1086/280625
Sfriso A., Buosi A., Mistri M., Munari C., Franzoi P., Sfriso A. A. (2019b). Long-term changes of the trophic status in transitional ecosystems of the northern Adriatic Sea, key parameters and future expectations: The lagoon of Venice as a study case. Nat. Conserv. 34, 193–215. doi: 10.3897/natureconservation.34.30473
Sfriso A., Buosi A., Sciuto K., Wolf M., Tomio Y., Juhmani A.-S., et al. (2022a). Effect of ecological recovery on macrophyte dominance and production in the Venice Lagoon. Front. Mar. Sci. 9. doi: 10.3389/fmars.2022.882463
Sfriso A., Buosi A., Tomio Y., Juhmani A.-S., Facca C., Sfriso A. A., et al. (2019a). Aquatic angiosperm transplantations: A Tool for Environmental Management and Restoring in Transitional Water Systems. Water 11, 2135. doi: 10.3390/w11102135
Sfriso A., Buosi A., Tomio Y., Juhmani A.-S., Facca C., Wolf M., et al. (2021). Environmental restoration by aquatic angiosperm transplants in transitional water systems: The Venice Lagoon as a case study. Sci. Tot. Environ. 795, 148859. doi: 10.1016/j.scitotenv.2021.148859
Sfriso A., Curiel D., Rismondo A. (2009). “The venice lagoon,” in Flora and Vegetation of the Italian Transitional Water Systems. Eds. Cecere E., Petrocelli A., Izzo G., Sfriso A. (CoRiLa, Multigraf, Spinea, Italy), 17–80.
Sfriso A., Facca C. (2007). Distribution and production of macrophytes in the lagoon of venice. Comparison of actual and past abundance. Hydrobiologia 577, 71–85. doi: 10.1007/s10750-006-0418-3
Sfriso A., Facca C., Ceoldo S., Marcomini A. (2005a). Recording the occurrence of trophic level changes in the lagoon of Venice over the ‘90s. Environ. Int. 31, 993–1001. doi: 10.1016/j.envint.2005.05.009
Sfriso A., Facca C., Ghetti P. F. (2007). Rapid Quality Index (R-MaQI), based mainly on macrophyte associations, to assess the ecological status of Mediterranean transitional environments. Chem. Ecol. 23, 1–11. doi: 10.1080/02757540701702918
Sfriso A., Facca C., Marcomini A. (2005b). Sedimentation rates and erosion processes in the lagoon of Venice. Environ. Int. 31, 983–992. doi: 10.1016/j.envint.2005.05.008
Sfriso A., Ghetti P. F. (1998). Seasonal variation in the biomass, morphometric parameters and production of rhizophytes in the lagoon of Venice. Aquat. Bot. 61, 207–223. doi: 10.1016/S0304-3770(98)00064-3
Sfriso A., Marcomini A. (1997). Macrophyte production in a shallow coastal lagoon. Part I. Coupling with physico-chemical parameters and nutrient concentrations in waters. Mar. Environ. Res. 44, 351–375. doi: 10.1016/S0141-1136(97)00012-3
Sfriso A., Marcomini A. (1999). Macrophyte production in a shallow coastal lagoon. Part II. Coupling with sediment, SPM and tissue nutrient concentrations. Mar. Environ. Res. 47, 285–309. doi: 10.1016/S0141-1136(98)00122-6
Sfriso A., Marcomini A., Pavoni B. (1987). Relationship between macroalgal biomass and nutrient concentrations in a hypertrophic area of the Venice Lagoon. Mar. Environ. Res. 22, 297–312. doi: 10.1016/0141-1136(87)90005-5
Sfriso A. A., Sciuto K., Mistri M., Munari C., Juhmani A.-S., Buosi A., et al. (2023). What, How, Where and When to transplant seagrasses to obtain long lasting results in Transitional Water Systems: the cases of Cymodocea nodosa, Zostera marina, Zostera noltei and Ruppia cirrhosa. Front. Mar. Sci. 10. doi: 10.3389/fmars.2023.1299428
Short F. T., Mathieson A. C., Nelson J. I. (1986). Recurrence of the eelgrass wasting disease at the border of New Hampshire and Maine, U.S.A. Mar. Ecol. Prog. Ser. 29, 88–92. doi: 10.3354/meps029089
Short F. T., Ibelings B. W., den Hartog C. (1988). Comparison of a current eelgrass disease to the wasting disease of the 1930's. Aquat. Bot. 30, 295–304. doi: 10.1016/0304-3770(88)90062-9
Short F. T., Porter D., Iizumi H., Aioi K. (1993). Occurrence of the eelgrass pathogen Labyrinthula zosterae in Japan. Dis. Aquat. Org. 16, 73–77. Available at: https://www.int-res.com/articles/dao/16/d016p073.
Strickland D., Parsons T. R. (1984). Practical Handbook of Seawater analysis. 2nd edition (Ottawa, Canada: Fisheries Research Board of Canada).
Viaroli P., Bartoli M., Giordani G., Naldi M., Orfanidis S., Zaldivar J. M. (2008). Community shifts, alternative stable states, biogeochemical controls and feedbacks and in eutrophic coastal lagoons: a brief review. Aquat. Conserv. Mar. Fresh. Ecosys. 18, 105–117. doi: 10.1002/aqc.956
Vilibić I., Zemunik P., Šepić J., Dunić N., Marzouk O., Mihanović H., et al. (2019). Present-climate trends and variability in thermohaline properties of the northern Adriatic shelf. Ocean. Sci. Discuss. 15, 1351–1362. doi: 10.5194/os-15-1351-2019
Wetzel R. L., Neckles H. A. (1986). A model of Zostera marina L. photosynthesis and growth: simulated physical-chemical variables and biological interactions. Aquat. Bot. 26, 307–323. doi: 10.1016/0304-3770(86)90029-X
Wilson D. P. (1949). The decline of Zostera marina L. at Salcombe and its effects on the shore. J. Mar. Biol. Assoc. U.K. 28, 395–412. doi: 10.1017/S0025315400023298
Wong M. C., Vercaemer B. M., Griffiths G. (2021). Response and Recovery of eelgrass (Zostera marina) to chronic and episodic light disturbance. Estuar. Coast. 44, 312–324. doi: 10.1007/s12237-020-00803-3
Zanchettin D., Bruni S., Raicich F., Lionello P., Adloff F., Androsov A., et al. (2021). Sea level rise in Venice: Historic and future trend. Nat. Hazards. Earth Syst. Sci. 21, 2643–2678. doi: 10.5194/nhess-21-2643-2021
Keywords: eelgrass cover, environmental variables, macroalgae, primary production, standing crop, Zostera marina
Citation: Sfriso A, Buosi A, Tomio Y, Silan G, Wolf MA, Sciuto K and Sfriso AA (2024) Biomass, production and growth strategies of the eelgrass Zostera marina, a relict cold-loving species: the Venice Lagoon as a study case. Front. Mar. Sci. 11:1424638. doi: 10.3389/fmars.2024.1424638
Received: 28 April 2024; Accepted: 13 August 2024;
Published: 05 September 2024.
Edited by:
Heliana Teixeira, University of Aveiro, PortugalReviewed by:
Marcos Rubal, University of Minho, PortugalCopyright © 2024 Sfriso, Buosi, Tomio, Silan, Wolf, Sciuto and Sfriso. This is an open-access article distributed under the terms of the Creative Commons Attribution License (CC BY). The use, distribution or reproduction in other forums is permitted, provided the original author(s) and the copyright owner(s) are credited and that the original publication in this journal is cited, in accordance with accepted academic practice. No use, distribution or reproduction is permitted which does not comply with these terms.
*Correspondence: Adriano Sfriso, c2ZyaXNvYWRAdW5pdmUuaXQ=
Disclaimer: All claims expressed in this article are solely those of the authors and do not necessarily represent those of their affiliated organizations, or those of the publisher, the editors and the reviewers. Any product that may be evaluated in this article or claim that may be made by its manufacturer is not guaranteed or endorsed by the publisher.
Research integrity at Frontiers
Learn more about the work of our research integrity team to safeguard the quality of each article we publish.