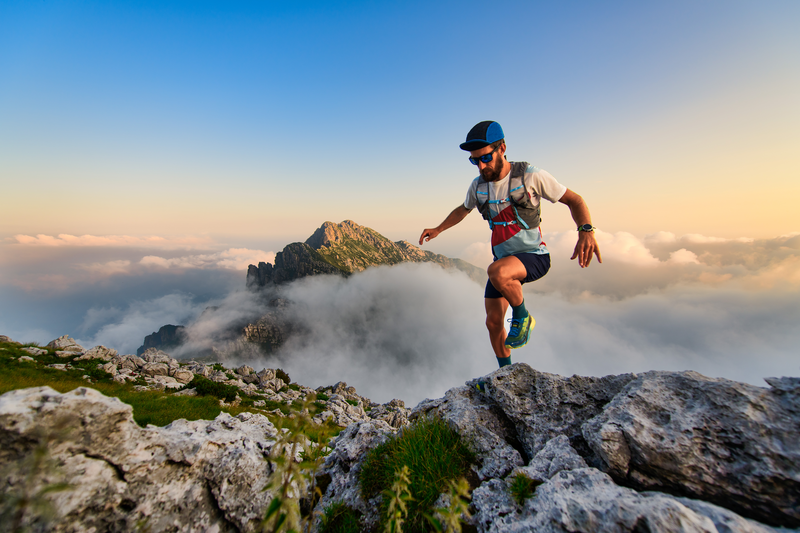
94% of researchers rate our articles as excellent or good
Learn more about the work of our research integrity team to safeguard the quality of each article we publish.
Find out more
ORIGINAL RESEARCH article
Front. Mar. Sci. , 04 October 2024
Sec. Marine Biology
Volume 11 - 2024 | https://doi.org/10.3389/fmars.2024.1418506
Globally, key predators such as lobsters are thought to control urchins. In south-eastern Australia, the role of Sagmariasus verreauxi (eastern rock lobster) as a key predator of the native urchin Centrostephanus rodgersii (long-spined urchin) has been questioned while the sympatric Heliocidaris erythrogramma (short-spined urchin) may be eaten more frequently. To test this, we tethered and filmed 100 urchins (50 C. rodgersii and 50 H. erythrogramma) outside of a lobster den over 25 nights to identify predators and quantify predation rates, time to feeding onset and handling time. Sagmariasus verreauxi exhibited very low predation rates (4%), despite being filmed walking past urchins repeatedly whereas Heterodontus galeatus (crested horn shark) was the main predator (45%). Predation rates by all predators (lobsters and sharks) were influenced primarily by tethering night but not urchin size or species. Predation increased throughout the study and while H. erythrogramma and C. rodgersii were eaten at similar rates, there was a trend for lobsters to eat H. erythrogramma and sharks to eat C. rodgersii. Feeding onset had no significant predictors though large C. rodgersii took longer to handle during feeding for both predators. Importantly, sharks readily consumed 25 C. rodgersii up to 121 mm Test Diameter (TD) while lobsters only ate one C. rodgersii (58 mm TD). These findings, although from only one test location, provide important insights into the species eating urchins and highlight the potential significance of overlooked predators such as H. galeatus.
Lobsters are considered to be key urchin predators and have been linked with reduced urchin densities in many places around the world (Scheibling, 1996; Shears and Babcock, 2003; Lafferty, 2004; Pederson and Johnson, 2006; Kawamata and Taino, 2021). As such, there is an assumption that lobsters regulate urchin numbers (Pederson and Johnson, 2006; Babcock et al., 1999; Edgar et al., 2009; Day et al., 2021; Smith et al., 2022, 2023; Peleg et al., 2023). Regulating urchin numbers via predator control is thought to be a method of limiting urchin grazing effects on macroalgae, preventing the formation of “barrens”. Barrens are bare-rock areas denuded of macroalgae (Ling et al., 2009; Filbee-Dexter and Scheibling, 2014) and are represented in the mosaic of habitats on temperate rocky reefs (Underwood et al., 1991; Kingsford and Byrne, 2023). Predatory control of urchins by lobsters has been quantitatively demonstrated in South Africa (Blamey and Branch, 2012; Blamey et al., 2013), Japan (Kawamata and Taino, 2021) and Tasmania (Pederson and Johnson, 2006). In south eastern Australia, New South Wales (NSW) barrens attributed to the native species Centrostephanus rodgersii (long-spined urchin) and to a lesser extent Heliocidaris erythrogramma (short-spined urchin) are naturally occurring and have remained stable over the last 40 years though it is suggested barrens may have increased in southern NSW (Andrew and Underwood, 1989; Barrett et al., 2008a, b; Glasby and Gibson, 2020; Kingsford and Byrne, 2023). Unlike other places in the world (Kawamata and Taino, 2021) NSW barrens persist even with lobster population recovery to 34% of unfished biomass since past overfishing (Montgomery and Liggins, 2013; Woodings et al., 2021). However, it remains uncertain whether the current extent of C. rodgersii barrens occurred due to a paucity of predators (Evans et al., 2017; Layton et al., 2020) or whether barrens represent a natural ecological state (Kingsford and Byrne, 2023) as it is difficult to re-imagine historic lobster populations.
Recent tethering experiments in the same region suggested S. verreauxi consumed few (~ 30%) C. rodgersii and H. erythrogramma (Day et al., 2023a). This differed to the Palinurids Jasus edwardsii in Tasmania (Pederson and Johnson, 2006; Ling et al., 2019) and Palinurus japonicus in Japan (~ 90%) (Kawamata and Taino, 2021). Importantly, dissections of lobster gut contents showed C. rodgersii was present in only 1 – 2% of wild S. verreauxi guts (1%, N = 115 Day et al., 2021; 2%, N = 125 J Day unpublished data). These results have brought the potential for S. verreauxi to control C. rodgersii into question, highlighting the need to consider other predators. Sagmariasus verreauxi frequently moves between nearshore coastal and deep water environments, are highly mobile inshore and can spend portions of their lives in deep offshore waters where urchins are not present (Jeffs et al., 2013) so their impact on local urchin populations may be less than lobsters with smaller home ranges like J. edwardsii and P. japonicus (Pederson and Johnson, 2006; Ling et al., 2019; Kawamata and Taino, 2021). Given urchin tethers are generally placed in open environments where predators encounter them by chance during foraging (Boada et al., 2015; Pederson and Johnson, 2006; Ling et al., 2019; Day et al., 2023a) and S. verreauxi can avoid ingesting urchin spines during feeding (Day et al., 2021), the recent tethering experiments and gut contents (Day et al., 2021, 2023a) might not have provided an accurate predation rate compared with other work (Pederson and Johnson, 2006; Ling et al., 2019; Kawamata and Taino, 2021). It is expected that predation rates would increase if encounters for lobsters and tethered urchins were guaranteed and lobsters were not disturbed or handled (Day et al., 2021; Smith et al., 2022). This could be achieved by placing tethered urchins within the vicinity of an inhabited “den” where lobsters and other predators congregate, where the density of lobsters is higher than generally encountered in nearshore NSW.
Larger urchins can be more difficult prey to handle than smaller ones due to their longer spines, and this may deter some predators from feeding or cause them to avoid urchins completely. Once larger than ~ 120 mm TD, C. rodgersii is thought to experience little predation (Edgar et al., 2009; Ling and Johnson, 2012; Ling, 2013) and across its natural NSW range, body sizes of > 100 mm TD are typical (Andrew and Underwood, 1989; Andrew and Byrne, 2007; Day et al., 2023a). While lobsters in NSW have been reported to eat large C. rodgersii (up to ~ 98 mm TD, Day et al., 2021) other predators such as Achoerodus viridis (eastern blue groper) and potentially Chrysophyrus auratus (pink snapper) effectively handle large C. rodgersii (> 100 mm TD, Day et al., 2023a). However, both species have shown historical stock declines in NSW due to overfishing (Stewart et al., 2010; Young et al., 2014; Kingsford and Byrne, 2023). The main fish predator of C. rodgersii in NSW is thought to be A. viridis (Andrew, 1999; Layton et al., 2020; Day et al., 2023a) because it is known for removing urchins from their holes and turning them over to feed (Andrew, 1993, 1999) in a similar fashion to lobsters (Ling and Johnson, 2012; Day et al., 2021; Kawamata and Taino, 2021). Although C. auratus is abundant in New Zealand (Denny et al., 2004; Jones, 2013; Shears and Babcock, 2002; Balemi and Shears, 2023), it does not seem to control C. rodgersii (Balemi and Shears, 2023) and possibly prefers to prey on smaller, native species (Babcock et al., 1999; Jones, 2013; Schiel et al., 2018). While C. auratus is also present in Tasmania due to a recent southward range extension (Morgan et al., 2018; Wolfe, 2021), they only occur in low densities and are therefore unlikely to exert trophic control of C. rodgersii (Smith et al., 2023). Smaller teleosts like Acanthopagrus australis, Ophthalmolepis lineolatus, Notolabrus gymnogenis and Pictilabrus laticlavius (Morton et al., 2008; Puskic and Coghlan, 2021) have also been found with urchin spines in their guts and Gymnothorax prasinus has shown interest in C. rodgersii remains in Baited Remote Underwater Video (BRUV) studies (Wraith, 2007) and whole urchins in tethering experiments (Day et al., 2023a). Invertebrate predators including the cephalopods Sepia and Octopodes species have also been speculated to eat C. rodgersii because octopus can eat urchins (Ambrose, 1986) and both species interact with tethered C. rodgersii (Day et al., 2023a) but neither have been observed feeding. Compared to other parts of south east Australia it is likely that some level of “trophic redundancy” (Eisaguirre et al., 2020) exists in NSW with multiple predators providing similar functional roles. However, some predators may potentially be more important than others as seen in other urchin-dominated systems (Trowbridge et al., 2019; Eisaguirre et al., 2020).
Tethering experiments involve removing urchins from their protective holes and restraining them from returning to record rates of urchin predation (Boada et al., 2015; Pederson and Johnson, 2006; Ling et al., 2019; Kawamata and Taino, 2021; Day et al., 2023a). This is meant to simulate conditions where C. rodgersii is more vulnerable for predation, similar to when urchins leave their holes at night to graze macroalgae (Byrne and Andrew, 2020). In recent experiments conducted in NSW, the shark Heterodontus portusjacksonii (Port Jackson shark) was observed readily feeding on large C. rodgersii > 100 mm TD (Day et al., 2023a) although the frequency and timing of these events were not quantified, which is a limitation of traditional tethering methods (Baker and Waltham, 2020). Previously, there had been speculation that H. portusjacksoni ate C. rodgersii based on its dentition (Powter et al., 2010), gut contents (McLaughlin and O’Gower, 1971) and fatty-acid stable isotopes (Beckmann et al., 2013; Hoopes et al., 2020). Given the similar dentition and tough skull of the sympatric crested horn shark (Heterodontus galeatus) it is also anticipated that H. galeatus preys on C. rodgersii. These sharks are frequently observed in and around lobster dens in south east Australia, often cohabiting with other sharks and S. verreauxi. Both H. portusjacksoni and H. galeatus are reported with bright, purple-stained mouths which is thought to result from C. rodgersii predation (Taylor, 1972; Elliott and Bounds, 1987; Powter et al., 2010) because C. rodgersii carries purple dye within its tissues. Other Chondrichthyans like Batoid stingrays are also suggested to eat urchins (Grun, 2016; Day et al., 2023a). However, there are no recorded instances of feeding or the characteristic purple dye and urchin spines in Batoid guts like there are in Heterodontus species (McLaughlin and O’Gower, 1971; Taylor, 1972; Elliott and Bounds, 1987; Powter et al., 2010). Restraining urchins from returning to their holes and filming predator-prey interactions without manipulating predators (Day et al., 2021; Smith et al., 2022) would provide empirical evidence of the species that eat urchins and the extent to which urchins survive due to size refugia (Edgar et al., 2009; Ling and Johnson, 2012; Ling, 2013).
In this study, we use an orthogonal arrangement of filmed urchin tethers to determine predators of the barrens-forming urchins C. rodgersii and H. erythrogramma and to record predation rates. We used a single study site with habitats representative of rocky reefs occurring in temperate NSW waters because we chose to do more rigorous temporal sampling rather than focusing on spatial replication. The site was an S. verreauxi lobster den within Wollongong, NSW, and was selected as lobsters and other potential predators are abundant at this location and urchins are naturally available. Caution is warranted to extrapolating beyond the experimental region of a single lobster den used here as lobsters and other urchin predators can show differences at the level of sites and individuals (Day et al., 2021, 2023a; Byrnes and Brown, 2016) and predation near a lobster den is likely not representative for broader NSW. We considered that if lobsters regularly eat urchins, then placing tethered urchins at multiple entrances of a lobster den would ensure encounters, resulting in high rates of urchin predation. Our experiments occurred under natural conditions, with the caveat that we had removed urchins from their crevices and made them more available for feeding. We expected that smaller urchins would experience more predation than larger urchins, be eaten sooner and take less time to handle.
Our study was conducted in Wollongong, New South Wales (34.42488°S, 150.8931°E; Figure 1A) on the southeast coast of Australia (Figure 1B). The study location contains a large, known lobster den which is estimated to regularly house > 20 large S. verreauxi including some individuals of up to > 180 mm CL until approximately December each year when most lobsters will have migrated offshore or further along the coast (Jeffs et al., 2013; Day, 2020; J Day pers obs). Other putative urchin predators including the fishes A. viridis, C. auratus, O. lineolatus, sharks H. portusjacksonii, H. galeatus and Orectolobus maculatus and cephalopods O. tetricus, Sepia apama and S. plangon as well as the scavengers N. gymnogenis, P. laticlavius and G. prasinus are often present within the den and surrounds, as well as the occasional sub-adult J. edwardsii and Panulirus spp. lobster of up to ~ 115 mm CL (Day et al., 2023a; J Day pers obs). Lobsters are nocturnal foragers (Jeffs et al., 2013; Day et al., 2021) and the study location was chosen because it allowed repeated safe access at sunrise and dusk for the divers. The den is ~ 5 – 8 m deep and has four entrances orientated at approximately North, East, South and West (Figures 1C–G). Although we only sampled urchin predation at one location in this study, we do so because this location has consistently high densities of eastern rock lobsters which should provide the best opportunity to observe lobster predation. In many other reef areas on the NSW coastline, the low density levels of lobsters may miss urchin predation in tethering experiments due to lobsters not occurring in that section of reef. At the location used in this study, lobsters were frequently observed and observed in high densities. The location also provided a safe location to present urchins to assess predation rates. We realize that the lack of spatial replication reduces the generality of our findings, but our aim here is to quantify urchin predation rates at a site where we would expect high rates of predation due to the high densities of lobsters (a best case scenario) to measure this potential ecosystem function. It also provides an opportunity to more naturally assess urchin predation than in comparison with laboratory studies and assess influence of urchin size and species in an area these species naturally occur at a wide range of urchin sizes. We selected this location as we knew that S. verreauxi and C. rodgersii would be abundant and that it contained a typical mix of habitats representative of contemporary temperate seascapes in SE Australia (Underwood et al., 1991; Kingsford and Byrne, 2023). Rather than undertaking a spatially replicated experiment (with increased risk to divers) we opted to undertake rigorous temporal sampling at this one location where we were confident that predation would occur. This provided an opportunity to intensively assess urchin predation by S. verreauxi with none of the typical human interference associated with lobster feeding experiments (Mayfield et al., 2001; Day et al., 2021; Smith et al., 2022). The urchins C. rodgersii and H. erythrogramma as well as sympatric non-barrens forming species H. tuberculata and Phyllacanthus parvispinus were present within the den and concealed during daylight. The den is situated within a diverse mosaic of rocky macroalgae, bare rock and cunjevoi (“sea-squirt”) habitats which are characteristic of NSW rocky reefs, i.e., the barrens-mosaic and fringe habitats described by Underwood et al. (1991). Rocky macroalgae patches contained the brown macroalgae Ecklonia radiata and Phyllospora comosa. The bare rock areas consisted of the articulated coralline algae Amphiroa anceps and Corallina officinalis as well as smaller fleshy macroalgae like Ulva, Padina, Lobophora, Zonaria sp. and Asparagopsis species. The top of the den and surroundings had small fleshy macroalgae, turban snails Lunella and Astralium species and crabs Guinusia chabrus. The ascidians Pyura praeputalis and P. gibbosa were present. Adjacent to the reef mosaic were areas of sand and seagrass meadows dominated by Zostera and Halophila species. The urchins C. rodgersii and H. erythrogramma were present everywhere except in seagrass, sand and shallow cunjevoi. Densities of C. rodgersii were shown to be below the barrens-forming threshold of ~ 6 – 8 individuals/m2 (Andrew and Byrne, 2007; Ling et al., 2019) by previous surveys in the experimental area (Day et al., 2023a) and other previously surveyed dense C. rodgersii barrens (i.e. areas with 1 – 2 individuals/m2) were situated > 500 m away and by multiple headlands.
Figure 1. Location of (A) tethering units or “blocks” 1 – 4 where Centrostephanus rodgersii (shown black) and Heliocidaris erythrogramma (shown purple) were tethered at four entrances to the one lobster den (shown with “den”) situated within Wollongong harbor and (B) the orientation of Wollongong on the South East coast (shown with a red “x”) of New South Wales (shown with “NSW”). Block positions 1 – 4 are shown (C–G), with the top and approximate center of the lobster den shown in (G).
To tether urchins, we used methods previously employed by Day et al. (2023a) to attach single C. rodgersii and H. erythrogramma to wire brackets weighted with concrete blocks. Tethering units consisted of a concrete block (Supplementary Figure S1.1A) attached to a piece of 60 x 1200 mm galvanized wire mesh with 50 x 50 mm panels. Panels were attached to the blocks using rope (5 mm diameter) and brackets constructed from steel wire (2 mm diameter). GoPro camera mounts were fitted to the top of the blocks using marine glue. To illuminate the tethered urchins a red-filtered light was fitted into a torch housing constructed from PVC pipe (10cm diameter) (Supplementary Figure S1.1B). A red-filtered light was used because Palinurid lobsters are sensitive to the white light spectrum and red-filtered lights have been used successfully in past night field studies using lobsters (Booth, 2001; Derby et al., 2001; Weiss et al., 2008; Day et al., 2021). GoPro ‘Hero 11 black’ cameras were set to take one photo every minute for ~ 12 hours (or until the battery ran out > 12 hours) from approximately sunset until sunrise using GoPro Labs firmware (https://community.gopro.com/s/article/GoPro-Labs?language=en_US). To standardize the hours and night cover each urchin would be tethered for, we were careful to tether urchins only at sunset which was approximately 6 – 7pm each evening as per daylight savings. Red-filtered lights were “Big Blue VL8000PTC” dive torches which had a piece of “Sun Tuf” red acrylic cut to the diameter of the torch lens and attached to the front using waterproof tape (Supplementary Figure S1.1C). Torches were cycled to the medium (4000 lumen) brightness light setting to provide ~ 12 hours of red light. Urchins were tethered using ~ 8 cm lengths of monofilament fishing line (15 kg tensile strength) to keep them within range of the light sources and camera frame. If the field of view was obstructed during feeding (e.g. by particulates or macroalgae) the footage was disregarded, and this occurred six times. The likely predator was inferred from the feeding remains left on tethers (Day et al., 2023a) but this was not included in analysis. Camera footage from each night was compared with field results recorded the next morning with four possible outcomes: H. erythrogramma (Supplementary Figure S1.1D) or C. rodgersii (Supplementary Figure S1.1E) urchin insides eaten with the test intact, urchin insides eaten with the test cracked or whole urchin absent (Supplementary Figure S1.1F) or the urchin insides not eaten with the test intact and the urchin still present. The presence of lobsters or other putative urchin predators like sharks or large fishes in the den was verified by sighting them or taking a photo using an iPhone 11 and “Wee-Fine WHF05” submersible housing. Observations of lobsters (Supplementary Figure S1.2) and sharks (Supplementary Figure S1.3) were undertaken each morning because we considered that observing or taking photos closer to sunset could disturb them (Booth, 2001; Derby et al., 2001; Weiss et al., 2008; Day et al., 2021). The presence of “urchin-fall”, i.e., identifiable remains of urchins (Day et al., 2023a; Supplementary Figure S1.4) was also recorded around the den vicinity for the duration of the experiment (Online Supplementary Material S1; Experimental Design). Field observations were recorded on a dive slate each morning when the cameras and lights were retrieved after being deployed overnight for < 24 hours.
Our study was conducted over 25 non-consecutive nights with 100 urchins (50 C. rodgersii and 50 H. eyrthrogramma) from September 14th until November 1st in 2023. Tethering occurred on successive nights on 12 occasions while on 15 occasions there was a gap of 2 – 7 nights due to rough weather preventing access or filming. Urchins were collected by hand via snorkeling at 2 – 5 m depth in nearshore environments. To standardize the potential of urchins as prey we only used urchins collected locally from dense macroalgal habitat, as past work has suggested that urchins taken from barrens are less attractive prey (Eurich et al., 2014; Smith et al., 2023). Since roe maturation can affect the rates that lobsters eat urchins (Eurich et al., 2014) the experiment was designed to give the best chance of C. rodgersii carrying plentiful roe, shown by Gonad Index (GI) which peaks in approximately September (King et al., 1994) with breeding ending after November, causing GI to decrease (Bentley et al., 1998). Since spawning regimes can vary between locations along the NSW coast (King et al., 1994; Bentley et al., 1998) we dissected 20 additional C. rodgersii and H. erythrogramma collected from within the study site at the approximate start (September 2023) and end (November 2023) of the experiments (N = 40 urchins total) to verify whether GI changed during the experimental period. Any uneaten tethered urchins were also retrieved and dissected for GI analysis each day for comparison (Online Supplementary Material S2; Gonad Index Analysis). Sagmariasus verreauxi are reported to molt their carapaces during seasonal inshore habitation (Jeffs et al., 2013) and this was an important consideration because molting has affected feeding on urchins by other lobsters (Mayfield et al., 2000; Ziegler et al., 2004). However, recent work undertaken in NSW showed no effect of molt stage on the presence of urchin parts in S. verreauxi guts with urchin parts found in both pre- and post-molt lobsters (Day et al., 2021). Photos were catalogued to identify predators and feeding events (Supplementary Material S3; Feeding Images) and notable photo sequences were turned into videos (Supplementary Material S4; Urchin Videos). On one occasion two tethering units were damaged during a storm and had an additional wire mesh bracket added for support.
To guarantee lobsters would encounter tethered C. rodgersii and H. erythrogramma, urchins were placed at four entrances to the lobster den, with the blocks numbered 1 – 4 (Figures 1C–G). Urchins were restricted within camera view to ensure that predators could be identified. To ensure randomly dispersed sizes and species of urchins, treatments were randomized across the nights so that all blocks received all treatments. We searched for urchins each day according to predetermined size bins of 20 mm which were randomized before the experiment started to determine the order of treatments (Supplementary Table S1.1, Supplementary Figure S1.5). The size of each collected urchin was recorded to the nearest millimeter (mm) using vernier calipers before tethering urchins at sunset. This design allowed urchins to be presented orthogonally four at a time (two C. rodgersii and two H. erythrogramma) across their naturally occurring size range, with C. rodgersii attaining larger body sizes > 100 mm TD compared to H. erythrogramma (Cook and Vanderklift, 2011; Day et al., 2023a) (Supplementary Figure S1.5). For logistical simplicity urchins were denoted as “small” or “large” once measured. Based on previous surveys within the experimental area (Day et al., 2023a) small and large C. rodgersii or H. erythrogramma were defined as being below and above the cutoff points of 80 mm and 70 mm TD for each species, respectively (Supplementary Table S1.1, Supplementary Figure S1.5).
In the laboratory, GoPro photo sequences were reviewed to identify predation and the identity of predators. Predation was recorded when a lobster or fish was eating an urchin or appearing in-frame with the urchin feeding remains visible or the urchin gone thereafter (Kawamata and Taino, 2021). These events occurred frequently, though instances of predators investigating or moving past tethered urchins without feeding were also recorded. Because one photo was taken per minute, we were able to ascertain periods for “feeding onset” (how many minutes it took tethered urchins to be attacked) and “predator handling” (how long it took to eat an urchin) when a feeding event was recorded, based on the number of images recorded. To calculate feeding onset, the number of images from when the tethered urchin was placed on benthos until a predator was seen to feed were counted (Lester and Harmsen, 2002; Kawamata and Taino, 2021). To calculate handling time, the number of images that the predator was present in and observed feeding until the urchin was eaten were counted (Kawamata and Taino, 2021). For lobsters this included time spent turning urchins over and feeding from the ventral surface (Day et al., 2021), while for potential shark or fish predators it included time spent attacking and chewing urchins, with chewing indicated by similar forceful head and gill slit movements after engulfing urchins or urchin remains as reported for H. portusjacksoni eating shark eggs (Powter and Gladstone, 2008). When lobsters were clearly visible in side-profile on cameras, body sizes were estimated. This was achieved by comparing the number of steel mesh links closest to the body with the whole length of the animal, with each mesh link being 50 mm in diameter. Therefore, lobsters of ~ 100 mm and ~ 150 mm CL would span approximately two and three links, whereas a ~ 125 mm CL lobster would span approximately two and a half links. When predators exited frame and re-appeared we assessed whether they were the same or different individuals based on their physical features. Predator identity was determined by observing body and head shape, distinctive patterning and general coloration for invertebrates, fish, and sharks, respectively (McLaughlin and O’Gower, 1971; Powter and Gladstone, 2008; Jeffs et al., 2013). We identified sharks primarily based on head shape and coloration, with crested H. galeatus showing a high crest and vague body patterning while H. portusjacksoni has a lower crest and strong patterning. Coloration was a factor because the in-situ nature of our experiment meant that a range of lighting and visibility conditions were possible, and in some cases the patterning on the shark bodies was clearer than others. We checked our identification of sharks to species with local experts in a double-blind test. Size of lobsters within the den was estimated based on extensive experience with lobsters on south east Australian reefs (Day et al., 2021, 2023a, b, Day et al., 2024). We chose to estimate lobster size when observing them within the den as handling lobsters may cause significant disturbance (Day et al., 2021; Smith et al., 2022).
We used Generalized Linear Models (GLMs) to test whether rates of predation would be greater for C. rodgersii than H. erythrogramma and whether small compared to large urchins would be eaten more frequently or with greater feeding onset and handling time. This was an important consideration, because lobsters have shown different feeding responses for urchins based on size and species in the past (Gnanalingam and Butler, 2018; Day et al., 2021, 2024). Interactions between urchin size and species were tested because we wanted to know whether effects would be general or idiosyncratic to a specific size or species of urchin. We also included the night that urchins were presented to test for effects of tethering night because lobsters (Jeffs et al., 2013; Day et al., 2021) and their co-predators (McLaughlin and O’Gower, 1971; Powter and Gladstone, 2008) can be highly mobile in NSW over September to November when the experiment was undertaken, meaning the presence of putative urchin predators and associated predation rates could change over consecutive nights. We validated the models by using the “DHARMa” package (Hartig, 2022) which showed non-significant dispersion after five outliers for “handling time” > 10 minutes were removed. One outlier occurred for lobsters eating C. rodgersii (48mm TD; 53 minutes handling time) and H. erythrogramma (79mm TD; 60 minutes handling time) whereas three occurred with sharks eating C. rodgersii (68, 105 and 120mm TD; 17, 12 and 15 minutes handling time). We suspect these outliers occurred because in the absence of rock refugia, urchins may occasionally have been able to wedge themselves into the metal grates of the tethering apparatus which could have made it harder for predators to dislodge and eat them. GLMs for predation rates used a binomial distribution with tethered urchins being eaten (1) or not eaten (0), while models for feeding onset and handling time assumed a gamma distribution and all models were structured using a log-link. “Size” and “night” were run as continuous variables whereas “species” was a factor. There was not strong collinearity between continuous predictors of urchin size and tethering night (Pearson’s correlation, r < 0.4).
Models were constructed using the “glmmTMB” package (Brooks et al., 2017) with statistical significance computed via likelihood ratio tests (LRTs) using the “Anova” function in the “car” package (Fox and Weisberg, 2019). Predictions and standard errors for the likelihood of urchins to be eaten as well as onset and handling periods were generated using the “predict” function in the “glmmTMB” package (Brooks et al., 2017). All statistical analyses and plots were developed using the statistical program R (R Core Team, 2021) and the associated packages mentioned above as well as “lme4” (Bates et al., 2015), “ggplot2” (Wickham, 2016) and “dplyr” (Wickham et al., 2023).
We recorded known and potential urchin predators within the den on the majority (80%) of inspections. Between two and ten S. verreauxi were sighted on 17 occasions (68% of site visits; Supplementary Figure S1.2) while one to six Heterodontus species were sighted on 15 occasions (60% of site visits; Supplementary Figure S1.3). This was confirmed by sighting predators on multiple cameras at once and counting them at different den positions during morning inspections. Large wrasses and juvenile snappers were sighted around the experimental area but were not present within the den. Smaller teleosts, including A. australis and G. prasinus and the wrasses O. lineolatus, N. gymnogenis and P. laticlavius were generally present around the den but were not counted. Heterodontus spp. sharks co-occurred with lobsters in the den on 14 occasions (48% of site visits) and there were five instances (20% of site visits) where only lobsters were sighted and three instances (12% of site visits) where we only saw sharks. There were four occasions (16% of site visits) where sharks were sighted within the den and did not eat urchins, and this occurred 13 times (52% of site visits) for lobsters. Conversely, there were 10 occasions (40% of site visits) where sharks were not sighted in the den at the morning but were recorded eating tethered urchins that night, but this never occurred for lobsters. Urchins co-occurred with predators on all occasions as there were multiple C. rodgersii present within the den (Supplementary Figures S1.2A–C). Many large, hard-shelled lobsters were sighted within the den at the start and during the experiment. A molted carapace of one small lobster was also encountered at the den surrounds at the start of the experiment (Supplementary Figures S1.2F). There were signs of urchin fall identified as H. erythrogramma around the vicinity and on top of the den (Supplementary Figures S1.4A–C). Some urchin tests were degraded which prohibited identification (Supplementary Figures S1.4 D, E). No C. rodgersii urchin fall was recorded.
Out of the 100 tethered urchins 55 were eaten (55%). However, six feeding events (6%) were disregarded and not analyzed due to camera obstruction. In total, 49 tethered urchins were eaten (49%): Four were eaten by lobsters (4%) and 45 by Heterodontus species (45%). Lobsters tended to eat small urchins, eating three H. erythrogramma (x̄ = 70 ± 5 mm TD, 61 – 79 mm TD) and one C. rodgersii (48 mm TD) over the course of the experiment. Lobsters ate only one large H. erythrogramma > 70 mm TD (79 mm TD) and no large C. rodgersii > 80 mm TD. In comparison, Heterodontus species ate 25 C. rodgersii (x̄ = 87 ± 5 mm TD, 32 – 121 mm TD) and 20 H. erythrogramma (x̄ = 65 ± 5 mm TD, 29 – 93 mm TD) which included 15 large C. rodgersii (x̄ = 105 ± 3 mm TD, 82 – 121 mm TD) and 9 large H. erythrogramma (x̄ = 84 ± 2 mm TD, 72 – 93 mm TD). There were 24 uneaten C. rodgersii (24%) and 27 H. erythrogramma (27%). One disregarded feeding event strongly suggested lobster predation of a small H. erythrogramma (58 mm TD) indicated by an urchin eaten with insides removed and the test intact (Day et al., 2021, 2023a).
We observed multiple sharks (up to six per day) and lobsters (up to 10 per day) within the den and surrounds from September to November of 2023 and this led to 45 feeding events with sharks but only four with lobsters. All lobsters were identified as S. verreauxi (Figures 2A–F) and most sharks as H. galeatus, with at least four different lobsters and six different sharks observed to feed (Figures 2G–L, Supplementary Figures S3.1.1-S3.1.4). One shark was tentatively identified as H. portusjacksoni based on body patterning (Figure 2G). On four other occasions, both lobsters and sharks were recorded on camera travelling past tethered urchins and not feeding. This occurred three times with C. rodgersii and once with H. erythrogramma for lobsters (Supplementary Figures S3.1.5A–S3.1.5C) and sharks (Supplementary Figures S3.1.5D–S3.1.5F) on different nights. Lobster eye-shine within the den and visible antennas on top of the den were seen on four and three other occasions where no feeding occurred, respectively, resulting in 11 occasions where lobsters were seen on camera but showed no interest in tethered urchins. There was no discernible effect of urchin roe maturation, which decreased for both C. rodgersii and H. erythrogramma over the experimental period while predation increased (Supplementary Figure S2.1, Supplementary Table S2.1). Lobsters recorded feeding on camera appeared to be ~ 100 – 125 mm CL though individuals estimated to be > 150 mm CL were sighted in the den on inspections (Supplementary Figure S1.2). Predator feeding fluctuated throughout the experiment with lobsters and sharks feeding at the beginning, but only sharks feeding at the end. Overall, C. rodgersii was eaten more often (26%) than H. erythrogramma (23%). Sharks showed a preference for C. rodgersii (25%) over H. erythrogramma (20%) while lobsters preferred to eat H. erythrogramma (3%) over C. rodgersii (1%). Up to three individuals of the shark Orectolobus maculatus were sighted inhabiting the den on seven inspections (28% of nights) and an individual appeared on camera once. While O. maculatus often co-occurred with H. galeatus and S. verreauxi they were not recorded eating any tethered urchins. The cephalopod S. plangon and various small fishes including Parma unifasciata, Ostorhinchus limenus and N. gymnogenis were seen to inspect tethered urchins but did not feed (Supplementary Figure S3.1.6). There were eight small fish species seen feeding on urchin remains left by larger predators (Supplementary Figure S3.1.7) including N. gymnogenis (Supplementary Figures S3.1.7A, B), Scobinichthys granulataus (Supplementary Figure S3.1.7B), A. strigatus (Supplementary Figures S3.1.7C, D), Eviota sp. (Supplementary Figure S3.1.7D), P. microlepis (Supplementary Figures S3.1.7E, F), Acanthistius ocellatus (Supplementary Figures S3.1.7F, H), Microcanthus strigatus (Supplementary Figure S3.1.7G) and Pictilabrus laticlavius (Supplementary Figure S3.1.7I). Individuals of the fishes C. auratus and A. viridis were observed within the vicinity sporadically but never appeared on cameras, and no fishes were observed attacking tethered urchins. Intact urchin tests left by lobsters provided noticeably greater opportunity for scavengers (Supplementary Figures S3.1.7 A–F) compared to fragments left by sharks (Supplementary Figures S3.1.7 G–I) because lobsters left more remains.
Figure 2. Predators feeding on tethered urchins including (A, B) Sagmariasus verreauxi attacking small Centrostephanus rodgersii, (C) small H. erythrogramma, (D–F) large H. erythrogramma by turning urchins over to feed from the ventral surface, as well as (G–I) Heterodontus species attacking large C. rodgersii from the dorsal surface and (J, K) H. erythrogramma from the dorsal and ventral surfaces while (L) shows a shark completely engulfing a small H. erythrogramma urchin. Images (G–L) depict shark feeding events that occurred approximately at sunset directly after urchins were tethered. H. portusjacksonii was tentatively identified in (G) while (H–L) were positively identified as H. galeatus..
The tethering of urchin species outside a lobster den with many S. verreauxi lobsters present revealed substantially low rates of urchin predation by lobsters, whereas Heterodontus sharks were responsible for most predation events. Sharks appeared to feed voraciously with vigorous head movements (Supplementary Videos S4.1, S4.3), attacking urchins from dorsal or lateral surfaces and consuming them quickly compared to lobsters which were more careful and methodical (Supplementary Video S4.2), turning urchins over to feed and hence taking longer to eat them.
One C. rodgersii that had its tether broken while being attacked by a shark was also not eaten, surviving until morning by escaping onto a nearby rock (Supplementary Video S4.4). This suggests either that sharks are only willing to expend a limited amount of energy on eating urchins or that tethering urchins makes them significantly more attractive prey, even compared to similar sized urchins sitting on nearby rocks.
On eight occasions sharks commenced feeding directly before cameras were attached to the block (Supplementary Figure S3.1.4), necessitating hand filming using a GoPro (Supplementary Video S4.1). In contrast, lobsters ceased feeding on tethered urchins mid-way through the experiment even though lobsters and non-tethered urchins were present within the den (Supplementary Figure S1.3) while sharks continued to feed. The abundance of predators did not change appreciably throughout the experiment as sharks and lobsters were present throughout, though predators did change den positions frequently. Intraspecific competition was observed once each for lobsters (night 5, Supplementary Video S4.2) and sharks (night 14, Supplementary Video S4.3). We did not record any competition, predation or scavenging by A. viridis or C. auratus though they were present within the general area. We confirm prior suggestions that various small teleosts including Notolabrus sp. and Parma sp. scavenge urchin remains left by larger predators.
Urchin predation was only significantly correlated with tethering night (LRT, X21 = 12.09, P = < 0.001) and there was no significant difference in predation rate between urchin species (LRT, X21 = 0.01, P = 0.979; Table 1A). Contrary to our predictions there was also no effect of urchin size on predation rates (LRT, X21 = 0.63, P = 0.427; Table 1A) as urchins of all sizes were eaten. The likelihood of C. rodgersii of any size being eaten increased from 21.5 ± 10% to 79.2 ± 10.3% from “night 1” to “night 25” and for H. erythrogramma these values were 19.7 ± 10.7% to 77.4 ± 11.2% (Figure 3A). We were not able to analyze shark and lobster predators separately due to the extremely low instances of lobster predation (4%) recorded throughout the experiment.
Table 1. Likelihoods Ratio Testing (LRT) for Generalized Linear Models (GLMs) for (A) urchin predation (whether urchins were eaten or not), (B) feeding onset (time for urchins to be attacked) and (C) handling time (time for urchins to be consumed).
Figure 3. Predicted values from Generalized Linear Models (GLMs) for (A) urchins eaten (%) (B) feeding onset (time to start feeding on urchins) and (C) handling time (how long it took urchins to be consumed). Outcomes in (A) are shown as a percentage across all trials while (B, C) are expressed as minutes. Urchin species in (A, C) are shown by different colors and the shaded areas of the plot represent standard error around the predicted mean.
Feeding onset from tethering to urchin consumption were generally shorter for sharks (1 – 587 minutes, x̄ = 104 minutes ± 20 minutes, n = 45) compared to lobsters (32 – 549 minutes, x̄ = 165 minutes ± 121 minutes, n = 4) although the latter had fewer observations for comparison. Some sharks initiated feeding immediately upon urchin tethering at sunset (~ 6:00 – 7:00 pm), while others delayed feeding for up to ~ 10 hours (~ 4:00 – 5:00 am). We found no effect of urchin size (LRT, X21 = 0.36, P = 0.547), species (LRT, X21 = 0.50, P = 0.479) or night (LRT, X21 = 3.15, P = 0.076) on feeding onset (Table 1B). It was notable, however, that there was a non-significant trend for feeding onset to become quicker as the experiment progressed, with feeding onset decreasing from 265 ± 160 minutes to 68.1 ± 35 minutes from “night 1” to “night 25” (Figure 3B).
There was a non-significant difference in prey handling time between urchin species (LRT, X21 = 3.57, P = 0.059) and urchin size (LRT, X21 = 3.73, P = 0.053) and there was no effect of night (LRT, X21 = 0.21, P = 0.644) (Table 1C, Figure 3C).
Despite tethering urchins outside of a den to ensure lobsters would encounter them, lobsters appeared generally uninterested in urchins across a range of sizes. This was unexpected because lobsters including S. verreauxi have been reported to prefer eating small urchins (Kintzing and Butler, 2014; Day et al., 2021, 2024; Smith et al., 2023). Past feeding trials (Gnanalingam and Butler, 2018; Day et al., 2021, 2024; Smith et al., 2022) and tethering experiments (Day et al., 2023a) have suggested that some lobsters can be reluctant to eat urchins, despite their predatory capability at various body sizes (Day et al., 2021, 2024) while in other studies lobsters ate urchins voraciously (Provost et al., 2017; Kawamata and Taino, 2021). Compared to lobsters, Heterodontus species readily ate tethered urchins up to 121 mm TD with no apparent feeding limitation, and large C. rodgersii required approximately equal handling effort (~ 4 minutes) compared to H. erythrogramma (~ 3 minutes). These findings are consistent with prior suggestions about Heterodontus species being urchin predators (McLaughlin and O’Gower, 1971; Andrew, 1999; Powter et al., 2010; Beckmann et al., 2013; Hoopes et al., 2020; Day et al., 2023a). Therefore, our study site of a lobster den in Wollongong, NSW may avail of diverse urchin predators and hence might not be as reliant on lobsters controlling urchins as has been suggested (Ling et al., 2009; Ling and Johnson, 2012; Evans et al., 2017; Layton et al., 2020).
Although more urchins were eaten as the experiment progressed, feeding on tethered urchins occurred at all times of night and early morning. It was notable that the cessation of lobster feeding seemed to coincide with the approximate start of shark feeding (nights 4 – 7) which continued for the remainder of the study period, suggesting potential interspecific competition. Interspecific competition provides another potential explanation for the large positive change in likelihoods of urchins being eaten over the duration, particularly for C. rodgersii which sharks appeared to eat readily. This is similar to previous feeding trials involving S. verreauxi and J. edwardsii, where S. verreauxi was consistently (80% of cases) outcompeted for food resources (Twiname et al., 2022). It is crucial to note the abundance of preferred lobster prey such as mollusks T. torquata, L. undulata and A. tentiformis (Day et al., 2021; Smith et al., 2022) which were present around and on top of the den during the experiment. Hence, although competition may have influenced the results (Twiname et al., 2022) the most straightforward explanation for the low rates of lobster feeding observed is that S. verreauxi simply prefers to consume items other than urchins. However, fishing pressure has affected the availability of lobster prey items in the past, changing rates of feeding on urchins (Guenther et al., 2012; Eddy et al., 2014) and this could have affected our results since S. verreauxi is a fished resource (Montgomery and Liggins, 2013; Woodings et al., 2021). In a previous feeding trial study conducted with the lobsters P. guttatus and P. argus and the urchin Diadema antillarum which is a relative of C. rodgersii, urchins showed a negative response to proximate cues of the keen urchin predator P. guttatus and no response to the more hesitant urchin predator P. argus (Kintzing and Butler, 2014). Our observation of multiple C. rodgersii within and around the S. verreauxi lobster den and little tethered urchin predation by lobsters here and in previous experiments (Day et al., 2023a) may suggest that C. rodgersii may not view S. verreauxi as a threat. Previous studies with J. edwardsii suggest that C. rodgersii size refuge from predation could be the reason urchins persist in the presence of lobsters (Ling and Johnson, 2012; Smith et al., 2023). More recent studies with S. verreauxi where small lobsters ate large C. rodgersii with no apparent size limitation, eating significantly more urchins than larger lobsters (Day et al., 2021, 2024) make this seem unlikely for S. verreauxi.
In four instances, sharks could not be identified on camera due to environmental conditions and light backscatter and these could have been H. portusjacksoni rather than H. galeatus. Although both sharks have the dentition and tough heads to handle large urchins (McLaughlin and O’Gower, 1971; Andrew, 1999) it is crucial to acknowledge that while they were present in Wollongong during our experiment, H. galeatus is fewer in number and has a less extensive southern distribution than H. portusjacksoni (McLaughlin and O’Gower, 1971). Therefore, results would likely differ outside of the location we tested. Unlike other urchin predators whose populations have fluctuated significantly over time (Ling et al., 2009; Ling and Johnson, 2012; Evans et al., 2017; Layton et al., 2020), Heterodontus species have remained stable across their range (Walker and Gason, 2007; Braccini et al., 2009) and both H. portusjacksoni (http://www.iucnredlist.org/details/39334/0) and H. galeatus (https://www.iucnredlist.org/species/41824/68625634) are listed as ‘least concern’ under IUCN red list criteria. It has been speculated that Heterodontus species may have been impacted by commercial fishing operations historically (Andrew and Byrne, 2007) but they are a hardy species that show survival rates > 96% when caught in nets (Reid and Krogh, 1992) and like S. verreauxi (Jeffs et al., 2013) are seasonally abundant across the study region (Reid and Krogh, 1992; Powter and Gladstone, 2008; Smoothey et al., 2016) meaning predators can show different abundances latitudinally. There is also the potential for animal habits at the level of individuals to play a role in rates of feeding on tethered urchins, and individual preferences have been reported for H. portusjacksonii in the past (Byrnes and Brown, 2016).
Potential artefacts of experimental procedures, like predators becoming attuned to experiment conditions, is a key factor to consider in ecological studies as it could cause feeding rates to change or increase (Minello, 1993; Boada et al., 2015; Heinrich et al., 2022). In this study, there was a significant increase in feeding rate as the trials progressed but no increase in the time taken for feeding onset, suggesting that sharks may have become attuned to the experiments and eaten more urchins over the progressive nights, though they did not start feeding sooner. In previous studies in Tasmania, increased feeding rates over time have suggested that predators were naïve to C. rodgersii (Smith et al., 2022). However, in NSW predators are exposed to C. rodgersii at various life stages and would not be considered naïve (Andrew, 1993, 1999; Day et al., 2021; Kingsford and Byrne, 2023). Since this experiment was intensively temporally rather than spatially replicated, it could also be that repeated human-induced behaviour had an impact, similar to when divers feed urchins to fishes. Hence, there is a need for the present study to be replicated spatially and temporally outside of the area used here to test whether the results we report are general or site-specific. Future tethering experiments should have greater temporal separation than the 25 nights our trial went for, of which 12 nights were non-consecutive. Separating individual trials by a week or more could prevent animals becoming attuned to repeated experimental treatments (i.e., offerings of food) over time (Underwood and Clarke, 2005). This is an important consideration, as the timing of feeding trials can impact on the tendency of predators to feed (Boada et al., 2015; Day et al., 2024). For example, if predators are recently satiated when they encounter tethered urchins, they may show no interest. Conversely, if predators are hungry and learn that a captive meal arrives at a certain location and time each day, they may show great interest and this may have occurred with the sharks in the current study (Minello, 1993; Boada et al., 2015; Heinrich et al., 2022). Importantly, our repeated tethering experiments could have exacerbated natural intercompetition between lobsters and sharks, in turn leading to low urchin predation rates by S. verreauxi (Twiname et al., 2022). Previous urchin tethering studies have suggested that tethering makes urchins more attractive prey because their bodies are pierced, inducing predation, or increasing detectability through olfactory stimuli (Boada et al., 2015). Previous pilot studies indicated that tethering did not inflate rates of urchin predation by lobsters or induce urchin mortality.
Video footage allows specific urchin predators and the timing and method of their attack to be identified, minimizing potential experimental artefacts (Minello, 1993; Boada et al., 2015; Baker and Waltham, 2020; Kawamata and Taino, 2021). Tethering is often used to assess predation pressure on urchins specifically (Pederson and Johnson, 2006; Boada et al., 2015; Ling et al., 2019; Kawamata and Taino, 2021; Day et al., 2023a). Past NSW tethering work attributes ~ 70% of predation to fish and sharks and only ~ 30% to lobsters (Day et al., 2023a). The absence of video footage in Day et al. (2023a) may have underestimated lobster predation since predator identities (lobster or fish/shark) were determined by observing urchin remains or the absence of remains. This was an important consideration, because while lobsters typically leave characteristic remains of an empty, intact urchin test after feeding (Pederson and Johnson, 2006; Kawamata and Taino, 2021; Day et al., 2021, 2023a) they can also consume urchins entirely leaving little or no remains (Day et al., 2024) which could have been confused with predation by fish or sharks (Day et al., 2023a). In this study using a filmed tethering array, we confirm low rates of urchin predation by S. verreauxi (4%) and moderate to high rates by Heterodontus species (45%). Sharks ate more than half of tethered urchins and handled large C. rodgersii ~ 120 mm TD with none of the apparent feeding limitations shown for other predators (Edgar et al., 2009; Ling and Johnson, 2012; Ling, 2013; Balemi and Shears, 2023; Smith et al., 2023). Previous feeding trials (Day et al., 2021) and tethering experiments (Day et al., 2023a) had the caveats that lobsters and urchins were restrained within mesocosms and on tethers, respectively. Here, we removed urchins from their protective crevices making them more accessible to predators and pierced them, potentially increasing their risk of predation or increasing detectability (Boada et al., 2015). The urchins were then placed near an inhabited lobster den and illuminated with red-filtered light overnight, significantly increasing lobster encounters compared to previous experiments where urchins were tethered in open habitats and were not illuminated (Day et al., 2023a). Despite these manipulations, we report considerably less predation by lobsters than in previous experiments with approximately half (51%) of tethered urchins going uneaten overnight.
Worldwide, the role of lobsters in controlling sea urchins is being reconsidered in some ways because the correlation between lobster predation and environmental phase shifts from macroalgae to barrens can be inconsistent (Ebeling et al., 1985; Garnick, 1989; Elner and Vadas, 1990; Norderhaug and Christie, 2009; Foster and Schiel, 2010; Guenther et al., 2012). In the USA and Canada, the role of Homarus americanus has been revised from a keystone-like predator which controls urchin numbers (Harding et al., 1983; Pringle, 1986) to that of a benthic generalist which eats urchins opportunistically (Elner and Campbell, 1987; Garnick, 1989; Elner and Vadas, 1990; Scheibling, 1996). Since then, many of the changes in macroalgal and urchin populations seen in the USA and Canada have been put down to environmental factors (Sheldon et al., 1982; Garnick, 1989; Elner and Vadas, 1990; Scheibling, 1996) rather than predator control of urchins, though this is only one hypothesis. Similarly in NSW, predator-prey dynamics between urchins and their predators appear more complex than traditionally thought (Ling et al., 2009; Ling and Johnson, 2012; Evans et al., 2017; Layton et al., 2020) and alternative hypotheses regarding environmental factors like storm activity and freshwater influx regulating C. rodgersii populations are increasingly being considered (Andrew, 1991; Edgar et al., 2017; Davis et al., 2023). Concurrent lines of evidence now indicate that the impact of lobsters on urchins in NSW is notably less than reported in Tasmania (Day et al., 2021; Smith et al., 2022; Day et al., 2023a; Smith et al., 2023). Comparatively, rather than consistently acting as a “key” predator it is suggested that J. edwardsii in New Zealand may have exhibited different feeding responses under historic levels of fishing pressure (Eddy et al., 2014). Similarly, in Southern California the influence of fishing lobsters on urchin barrens appears inconsistent (Guenther et al., 2012). For S. verreauxi, we cannot discount on current information that urchins may have had a higher dietary importance for lobsters and other natural predators in unfished ecosystems. If urchins are controlled by predators in NSW, then like other urchin dominated ecosystems this likely occurs with a diverse predator suite (Trowbridge et al., 2019). Here, we describe an overlooked group of urchin predators in Heterodontus species, which have been present long term in south east Australia (Reid and Krogh, 1992; Walker and Gason, 2007; Braccini et al., 2009; Smoothey et al., 2016) and eats C. rodgersii significantly more readily than lobsters with little apparent physical limitation. Importantly, our temporally replicated tethering experiments conducted over 25 non-consecutive nights at one site now need to be spatially replicated across south east Australia to determine how urchin predation rates change at different latitudes where urchin predators could potentially be more varied, abundant, or larger in size.
The datasets presented in this study can be found in online repositories. The names of the repository/repositories and accession number(s) can be found in the article/Supplementary Material.
The animal study was approved by remote sensing of marine communities. Approval No. A-2022-221 and Ecology and food web characteristics of estuarine and coastal systems. Approval No. A-2020-026. The study was conducted in accordance with the local legislation and institutional requirements.
JD: Conceptualization, Data curation, Formal analysis, Funding acquisition, Investigation, Methodology, Project administration, Resources, Software, Visualization, Writing – original draft, Writing – review & editing. NK: Conceptualization, Data curation, Formal analysis, Funding acquisition, Investigation, Methodology, Project administration, Resources, Supervision, Validation, Visualization, Writing – original draft, Writing – review & editing. DS: Conceptualization, Data curation, Formal analysis, Methodology, Software, Supervision, Validation, Visualization, Writing – original draft, Writing – review & editing. MH: Conceptualization, Funding acquisition, Methodology, Project administration, Resources, Supervision, Validation, Visualization, Writing – original draft, Writing – review & editing. TG: Writing – review & editing, Conceptualization, Formal analysis, Funding acquisition, Investigation, Methodology, Project administration, Resources, Supervision, Validation, Visualization, Writing – original draft.
The author(s) declare financial support was received for the research, authorship, and/or publication of this article. JD received funding from University of Newcastle under a Research Training Program scholarship (number 1032626).
The authors thank Ebene Montgomery, Jen Donoghue, Kody Heinlein and Ben Neaves who were the main fieldwork volunteers and Craig Taylor, Jack Radcliffe and Simon Kirgis who gave helpful advice. We also thank Sue Newson (Crest Diving Jervis Bay) and Georgia Hall (NSW DPI Fisheries) for their review of shark photos as a quality control measure, Dr. Dave Harasti, Professor Maria Byrne, Sian Liddy, and Sue Newson for their advice regarding sharks feeding on urchins and Dr. Matt Rees for his advice on statistics. Fieldwork was undertaken using a scientific collection permit number (P13/0037-2.0). The authors acknowledge the traditional Owners of the coastal marine environments within which this research was undertaken, the Dharawal people, and pay our respect to Elders past, present and future.
The authors declare that the research was conducted in the absence of any commercial or financial relationships that could be construed as a potential conflict of interest.
The author(s) declared that they were an editorial board member of Frontiers, at the time of submission. This had no impact on the peer review process and the final decision.
All claims expressed in this article are solely those of the authors and do not necessarily represent those of their affiliated organizations, or those of the publisher, the editors and the reviewers. Any product that may be evaluated in this article, or claim that may be made by its manufacturer, is not guaranteed or endorsed by the publisher.
The Supplementary Material for this article can be found online at: https://www.frontiersin.org/articles/10.3389/fmars.2024.1418506/full#supplementary-material
Ambrose R. F. (1986). Effects of octopus predation on motile invertebrates in a rocky subtidal community. Mar. Ecol. Prog. Ser. 30, 261–273. doi: 10.3354/meps030261
Andrew N. L. (1991). Changes in subtidal habitat following mass mortality of sea urchins in Botany Bay, New South Wales. Aus. Journ. Ecol. 16, 353–362. doi: 10.1111/j.1442-9993.1991.tb01063.x
Andrew N. L. (1993). Spatial heterogeneity, sea urchin grazing, and habitat structure on reefs in temperate Australia. Ecology 74, 292–302. doi: 10.2307/1939293
Andrew N. L., Byrne M. (2007). “Ecology of centrostephanus,” in Developments in aquaculture and fisheries science. Ed. Lawrence J. M. (Elsevier), 191–204. doi: 10.1016/s0167-9309(07)80074-3
Andrew N. L., Underwood A. J. (1989). Patterns of abundance of the sea urchin Centrostephanus rodgersii (Agassiz) on the central coast of New South Wales, Australia. J. Exp. Mar. Bio. Ecol. 131, 61–80. doi: 10.1016/0022-0981(89)90011-7
Babcock R. C., Kelly S., Shears N. T., Walker J. W., Willis T. J. (1999). Changes in community structure in temperate marine reserves. Mar. Ecol. Prog. Ser. 189, 125–134. doi: 10.3354/meps189125
Baker R., Waltham N. (2020). Tethering mobile aquatic organisms to measure predation: A renewed call for caution. J. Exp. Mar. Bio. Ecol. 523, 151270. doi: 10.1016/j.jembe.2019.151270
Balemi C. A., Shears N. T. (2023). Emergence of the subtropical sea urchin Centrostephanus rodgersii as a threat to kelp forest ecosystems in northern New Zealand. Front. Mar. Sci. 10. doi: 10.3389/fmars.2023.1224067
Barrett N. S., Edgar G. J., Polacheck A. S., Lynch T., Clements F. (2008a). Ecosystem monitoring of subtidal reefs in the Jervis Bay Marine Park 1996-2007. Tasmanian Aquaculture Fisheries Institute Internal Rep.
Barrett N. S., Stuart-Smith R. D., Zagal C. J., Polacheck A. S., Lynch T., Edgar G. J., et al. (2008b). Baseline surveys of the subtidal reef biota of the Batemans Bay Marine Park 2005-2007. Tasmanian Aquaculture Fisheries Institute Internal Rep.
Bates D., Maechler M., Bolker B., Walker S. (2015). Fitting linear mixed-effects models using lme4. J. Stat. Software 67, 1–48. doi: 10.18637/jss.v067.i01
Beckmann C. L., Mitchell J. G., Stone D. A. J., Huveneers C. A. (2013). A controlled feeding experiment investigating the effects of a dietary switch on muscle and liver fatty acid profiles in Port Jackson sharks Heterodontus portusjacksoni. J. Exp. Mar. Biol. Ecol. 448, 10–18. doi: 10.1016/j.jembe.2013.06.009
Bentley N., Chick R. C., Blount C. (1998). “Interactions between the abalone fishery and sea urchins in New South Wales,” in Final report to Fisheries and Research Development Corporation, Cronulla, Australia. Project No. 93/102.
Blamey L. K., Branch G. M. (2012). Regime shift of a kelp-forest benthic community induced by an ‘invasion’ of the rock lobster Jasus lalandii. J. Exp. Mar. Biol. Ecol. 420, 33–47. doi: 10.1016/j.jembe.2012.03.022
Blamey L. K., Plagányi É.E., Branch G. M. (2013). Modeling a regime shift in a kelp forest ecosystem caused by a lobster range expansion. Bull. Mar. Sci. 89, 347–375. doi: 10.5343/bms.2011.1127
Boada J., Sanmartí N., Selden R. L., Lucas A., Pérez M., Alcoverro T., et al. (2015). Evaluating potential artifacts of tethering techniques to estimate predation on sea urchins. J. Exp. Mar. Biol. Ecol. 471, 17–22. doi: 10.1016/j.jembe.2015.05.011
Booth J. D. (2001). Habitat preferences and behavior of newly settled Jasus edwardsii (Palinuridae). Mar. Freshw. Res. 52, 1055–1065. doi: 10.1071/MF01089
Braccini J. M., Walker T. I., Gason A. S. (2009). “GHATF shark survey of population abundance and population size composition for target, byproduct and bycatch species,” in Report to Australian Fisheries Management Authority (Fisheries Research Branch, Department of Primary Industries, Queenscliff, Victoria, Australia).
Brooks M. E., Kristensen K., van Benthem K. G., Magnusson A., Berg C. W., Nielsen A., et al. (2017). glmmTMB balances speed and flexibility among packages for zero-inflated generalized linear mixed modeling. R. J. 9, 378–400. doi: 10.3929/ethz-b-000240890
Byrne M., Andrew N. L. (2020). “Centrostephanus rodgersii and Centrostephanus tenuispinu,” in Developments in Aquaculture and Fisheries Science. Ed. Lawrence J. M. (Elservier), 379–396.
Byrnes E. E., Brown C. (2016). Individual personality differences in Port Jackson sharks Heterodontus portusjacksoni. J. Fish. Biol. 89, 1142–1157. doi: 10.1111/jfb.12993
Cook K., Vanderklift M. A. (2011). Depletion of predatory fish by fishing in a temperate reef ecosystem leads to indirect effects on prey, but not to lower trophic levels. Mar. Ecol. Prog. Ser. 432, 195–205. doi: 10.3354/meps09155
Davis T. R., Knott N. A., Champion C, Przeslawski R. (2023). Impacts of climate change on densities of the urchin Centrostephanus rodgersii vary among marine regions in eastern Australia. Diversity. 15, 419. doi: 10.3390/d15030419
Day J. (2020). Urchin predation and marine park residency in the eastern rock lobster (Sagmariasus verreauxi): an initial assessment of its potential to control urchin populations. (Wollongong, Australia: University of Wollongong). Available at: https://ro.uow.edu.au/thsci/198/.
Day J., Knott N. A., Ayre D., Byrne M. (2023a). Effects of habitat on predation of ecologically important sea urchin species on east coast Australian temperate reefs in tethering experiments. Mar. Ecol. Prog. Ser. 714, 71–86. doi: 10.3354/meps14348
Day J. K., Knott N. A., Swadling D. S., Ayre D. J. (2021). Dietary analysis and mesocosm feeding trials confirm the eastern rock lobster (Sagmariasus verreauxi) as a generalist predator that can avoid ingesting urchin spines during feeding. Mar. Freshw. Res. 72, 1220–1232. doi: 10.1071/MF20287
Day J. K., Knott N. A., Swadling D., Ayre D., Huggett M., Gaston T. (2023b). Non-lethal sampling does not misrepresent trophic level or dietary sources for Sagmariasus verreauxi (eastern rock lobster). R. C. M. 37, e9435. doi: 10.1002/rcm.9435
Day J. K., Knott N. A., Swadling D. S., Ayre D. J., Huggett M. J., Gaston T. F. (2024). Spiny lobster predation of barrens-forming sea urchins is not limited by body size, but may be overstated. Ecosphere. 15. doi: 10.1002/ecs2.v15.8
Denny C. M., Willis T. J., Babcock R. C. (2004). Rapid recolonisation of snapper Pagrus auratus: Sparidae within an offshore island marine reserve after implementation of no-take status. Mar. Ecol. Prog. Ser. 272, 183–190. doi: 10.3354/meps272183
Derby C. D., Steullet P., Horner A. J., Cate H. S. (2001). The sensory basis of feeding behaviour in the Caribbean spiny lobster, Panulirus argus. Mar. Freshw. Res. 52, 1339–1350. doi: 10.1071/MF01099
Ebeling A. W., Laur D. R., Rowley R. J. (1985). Severe storm disturbances and reversal of community structure in a southern California kelp forest. Mar. Biol. 84, 287–294. doi: 10.1007/BF00392498
Eddy T. D., Pitcher T. J., MacDiarmid A. B., Byfield T. T., Tam J. C., Jones T. T., et al. (2014). Lobsters as keystone: Only in unfished ecosystems? Ecol. Modell. 275, 48–72. doi: 10.1016/j.ecolmodel.2013.12.006
Edgar G. J., Barrett N. S., Stuart-Smith R. D. (2009). Exploited reefs protected from fishing transform over decades into conservation features otherwise absent from seascapes. Ecol. Appl. 19, 1967–1974. doi: 10.1890/09-0610.1
Edgar G. J., Stuart-Smith R. D., Thomson R. J., Freeman D. J. (2017). Consistent multi-level trophic effects of marine reserve protection across northern New Zealand. PloS One 12, e0177216. doi: 10.1371/journal.pone.0177216
Eisaguirre J. H., Eisaguirre J. M., Davis K., Carlson P. M., Gaines S. D., Caselle J. E. (2020). Trophic redundancy and predator size class structure drive differences in kelp forest ecosystem dynamics. Ecology 101, e02993.
Elliott D. K., Bounds S. D. (1987). Causes of damage to brachiopods from the Middle Pennsylvanian Naco Formation, central Arizona. Lethaia 20, 327–335. doi: 10.1111/j.1502-3931.1987.tb02052.x
Elner R. W., Campbell A. (1987). Natural diets of lobster Homarus americanus from barren ground and macroalgal habitats off southwestern Nova Scotia, Canada. Mar. Ecol. Prog. Ser. 37, 131–140. doi: 10.3354/meps037131
Elner R. W., Vadas S. R. L. (1990). Inference in ecology: the sea urchin phenomenon in the northwestern Atlantic. Am. Nat. 136, 108–125. doi: 10.1086/285084
Eurich J. G., Selden R. L., Warner R. R. (2014). California spiny lobster preference for urchins from kelp forests: implications for urchin barren persistence. Mar. Ecol. Prog. Ser. 498, 217–225. doi: 10.3354/meps10643
Evans K., Bax N., Smith D. C. (2017). “Australia state of the environment 2016: marine environment,” in Canberra: Australian Government Department of the Environment and Energy (Commonwealth Scientific and Industrial Research Organisation report).
Filbee-Dexter K., Scheibling R. E. (2014). Sea urchin barrens as alternative stable states of collapsed kelp ecosystems. Mar. Ecol. Prog. Ser. 495, 1–25. doi: 10.3354/meps10573
Foster M. S., Schiel D. R. (2010). Loss of predators and the collapse of southern California kelp forests ()?: Alternatives, explanations and generalizations. J. Exp. Mar. Bio. Ecol. 393, 59–70. doi: 10.1016/j.jembe.2010.07.002
Fox J., Weisberg S. (2019). An R Companion to Applied Regression, Third edition (Thousand Oaks CA: Sage). Available at: https://socialsciences.mcmaster.ca/jfox/Books/Companion/.
Garnick E. (1989). Lobster (Homarus americanus) population declines, sea urchins, and “barren grounds”: A space-mediated competition hypothesis. Mar. Ecol. Prog. Ser. 58, 23–28. doi: 10.3354/meps058023
Glasby T. M., Gibson P. T. (2020). Decadal dynamics of subtidal barrens habitat. Mar. Environ. Res. 154, 104869. doi: 10.1016/j.marenvres.2019.104869
Gnanalingam G., Butler IV, M.J. (2018). Examining the relationship between size and feeding preferences in the Caribbean spiny lobster Panulirus argus (Latreille 1804) (Decapoda: Achelata: Palinuridae). J. Crustacean Biol. 38, 245–249. doi: 10.1093/jcbiol/rux122
Grun T. B. (2016). Echinoid test damage by a stingray predator. Lethaia. 49, 285–286. doi: 10.1111/let.12165
Guenther C. M., Lenihan H. S., Grant L. E., Lopez-Carr D., Reed D. C. (2012). Trophic cascades induced by lobster fishing are not ubiquitous in Southern California kelp forests. PloS One 7, e49396. doi: 10.1371/journal.pone.0049396
Harding G. C., Drinkwater K. F., Vass W. P. (1983). Factors influencing the size of American lobster (Homarus americanus) stocks along the Atlantic coast of Nova Scotia, Gulf of St. Lawrence, and Gulf of Maine: a new synthesis. Can. J. Fish. Aquat. Sci. 40, 168–184. doi: 10.1139/f83-027
Hartig F. (2022). DHARMa: Residual Diagnostics for Hierarchical (Multi-Level/Mixed) Regression Models. R package version 0.4.6. Available online at: https://CRAN.Rproject.org/package=DHARMa.
Heinrich D. D., Huveneers C., Houslay T. M., Dhellemmes F., Brown C. (2022). Shark habituation to a food-related olfactory cue. Anim. Behav. 187, 147–165. doi: 10.1016/j.anbehav.2022.03.003
Hoopes L. A., Clauss T. M., Browning N. E., Delaune A. J., Wetherbee B. M., Shivji M., et al. (2020). Seasonal patterns in stable isotope and fatty acid profiles of southern stingrays (Hypanus americana) at Stingray City Sandbar, Grand Cayman. Sci. Rep. 10, 19753. doi: 10.1038/s41598-020-76858-w
Jeffs A. G., Gardner C., Cockcroft A. (2013). “Jasus and sagmariasus species,” in Lobsters: Biology, Management, Aquaculture and Fisheries. Ed. Phillips B. F.(Wiley: John Wiley and Sons), 259–288. doi: 10.1002/9781118517444
Jones G. P. (2013). Ecology of rocky reef fish of northeastern New Zealand: 50 years on. N. Z. J. @ Mar. Freshw. Res. 47, 334–359. doi: 10.1080/00288330.2013.812569
Kawamata S., Taino S. (2021). Trophic cascade in a marine protected area with artificial reefs: spiny lobster predation mitigates urchin barrens. Ecol. Appl. 31, e02364. doi: 10.1002/eap.2364
King C. K., Hoegh-Guldberg O., Byrne M. (1994). Reproductive cycle of Centrostephanus rodgersii (Echinoidea), with recommendations for the establishment of a sea urchin fishery in New South Wales. Mar. Biol. 120, 95–106. doi: 10.1007/bf00381945
Kingsford M. J., Byrne M. (2023). New South Wales rocky reefs are under threat. Mar. Freshw. Res. 74, 95–98. doi: 10.1071/MF22220
Kintzing M. D., Butler IV, M.J. (2014). Effects of predation upon the long-spined sea urchin Diadema antillarum by the spotted spiny lobster Panulirus guttatus. Mar. Ecol. Progr. Ser. 495, 185–191. doi: 10.3354/meps10568
Lafferty K. D. (2004). Fishing for lobsters indirectly increases epidemics in sea urchins. Ecol. Appl. 14, 1566–1573. doi: 10.1890/03-5088
Layton C., Coleman M. A., Marzinelli E. M., Steinberg P. D., Swearer S. E., Vergés A., et al. (2020). Kelp forest restoration in Australia. Front. Mar. Sci. 7, 74. doi: 10.3389/fmars.2020.00074
Lester P. J., Harmsen R. (2002). Functional and numerical responses do not always indicate the most effective predator for biological control: an analysis of two predators in a two-prey system. J. Appl. Ecol. 39, 455–468. doi: 10.1046/j.1365-2664.2002.00733.x
Ling S. D. (2013). Pushing boundaries of range and resilience: a review of range-extension by a barrens-forming sea urchin. (Hobart, Tasmania: University Of Tasmania). Available at: https://hdl.handle.net/102.100.100/534937.
Ling S. D., Johnson C. R. (2012). Marine reserves reduce risk of climate-driven phase shift by reinstating size-and habitat-specific trophic interactions. Ecol. Appl. 22, 1232–1245. doi: 10.1890/11-1587.1
Ling S. D., Johnson C. R., Frusher S. D., Ridgway K. (2009). Overfishing reduces resilience of kelp beds to climate-driven catastrophic phase shift. P. N. A. S. 106, 22341–22345. doi: 10.1073/pnas.0907529106
Ling S. D., Kriegisch N., Woolley B., Reeves S. E. (2019). Density-dependent feedbacks, hysteresis, and demography of overgrazing sea urchins. Ecology. 100, e02577. doi: 10.1002/ecy.2577
Mayfield S., Atkinson L. J., Branch G. M., Cockcroft C. (2000). Diet of the West Coast rock lobster Jasus lalandii: Influence of lobster size, sex, capture depth, latitude, and moult stage. Afr. J. Mar. Sci. 22, 57–69. doi: 10.2989/025776100784125690
Mayfield S., De Beer E., Branch G. M. (2001). Prey preference and the consumption of sea urchins and juvenile abalone by captive rock lobsters (Jasus lalandii). Mar. Freshw. Res. 52, 773–780. doi: 10.1071/MF00067
McLaughlin R. H., O’Gower A. K. (1971). Life history and underwater studies of a heterodont shark. Ecol. Monogr. 41, 271–289. doi: 10.2307/1948494
Minello T. J. (1993). Chronographic tethering: a technique for measuring prey survival time and testing predation pressure in aquatic habitats. Mar. Ecol. Prog. Ser. 101, 99–104. doi: 10.3354/meps101099
Montgomery S. S., Liggins G. W. (2013). Recovery of the eastern rock lobster Sagmariasus verreauxi off New South Wales, Australia. Mar. Biol. Res. 9, 104–115. doi: 10.1080/17451000.2012.727436
Morgan J. A. T., Sumpton W. D., Jones A. T., Campbell A. B., Stewart J., Hamer P., et al. (2018). Assessment of genetic structure among Australian east coast populations of snapper Chrysophrys auratus (Sparidae). Mar. Freshw. Res. 70, 964. doi: 10.1071/mf18146
Morton J. K., Platell M. E., Gladstone W. (2008). Differences in feeding ecology among three co-occurring species of wrasse (Teleostei: Labridae) on rocky reefs of temperate Australia. Mar. Biol. 154, 577–592. doi: 10.1007/s00227-008-0951-x
Norderhaug K. M., Christie H. G. (2009). Sea urchin grazing and kelp re-vegetation in the NE Atlantic. Mar. Biol. Res. 5, 515–528. doi: 10.1080/17451000902932985
Pederson H. G., Johnson C. R. (2006). Predation of the sea urchin Heliocidaris erythrogramma by rock lobsters (Jasus edwardsii) in no-take marine reserves. J. Exp. Mar. Bio. Ecol. 336, 120–134. doi: 10.1016/j.jembe.2006.04.010
Peleg O., Blain C. O., Shears N. T. (2023). Long-term marine protection enhances kelp forest ecosystem stability. Ecol. Appl. 33, e2895. doi: 10.1002/eap.2895
Powter D. M., Gladstone W. (2008). Habitat preferences of Port Jackson sharks, Heterodontus portusjacksoni, in the coastal waters of eastern Australia. Proc. Linn. Soc 129, 151–165.
Powter D. M., Gladstone W., Platell M. (2010). The influence of sex and maturity on the diet, mouth morphology and dentition of the Port Jackson shark, Heterodontus portusjacksoni. Mar. Freshw. Res. 61, 74–85. doi: 10.1071/MF09021
Pringle J. D. (1986). A review of urchin/macro-algal associations with a new synthesis for nearshore, eastern Canadian waters. Monogr. Biolog. 4, 191–218. Available at: https://cir.nii.ac.jp/crid/1571698599657712000.
Provost E. J., Kelaher B. P., Dworjanyn S. A., Russell B. D., Connell S. D., Ghedini G., et al. (2017). Climate‐driven disparities among ecological interactions threaten kelp forest persistence. Glob. Change Biol. 23, 353–361. doi: 10.1111/gcb.13414
Puskic P. S., Coghlan A. R. (2021). Minimal meso-plastics detected in Australian coastal reef fish. Mar. pollut. Bull. 173, 113074. doi: 10.1016/j.marpolbul.2021.113074
R Core Team (2021). R: A language and environment for statistical computing (Vienna, Austria: R Foundation for Statistical Computing). Available at: https://www.R-project.org/.
Reid D. D., Krogh M. (1992). Assessment of catches from protective shark meshing off NSW beaches between 1950 and 1990. Mar. Frew. Res. 43, 283–296. doi: 10.1071/MF9920283
Scheibling R. E. (1996). The role of predation in regulating sea urchin populations in eastern Canada. Oceanol. Acta 19, 421–430. Available at: https://archimer.ifremer.fr/doc/00094/20498/.
Schiel D. R., Ayling T., Kingsford M. J., Battershill C. N., Choat J. H., Andrew N. L., et al. (2018). Change in the rocky reef fish fauna of the iconic Poor Knights Islands Marine Reserve in north-eastern New Zealand over 4 decades. Mar. Freshw. Res. 69, 1496–1507. doi: 10.1071/mf18037
Shears N. T., Babcock R. C. (2002). Marine reserves demonstrate top-down control of community structure on temperate reefs. Oecologia. 132, 131–142. doi: 10.1007/s00442-002-0920-x
Shears N. T., Babcock R. C. (2003). Continuing trophic cascade effects after 25 years of no-take marine reserve protection. Mar. Ecol. Prog. Ser. 246, 1–16. doi: 10.3354/meps246001
Sheldon R. W., Sutcliffe W. H. Jr., Drinkwater K. (1982). “Fish production in multispecies,” in Multispecies approaches to fisheries management advice, Canadian Special Publication on Fisheries and Aquatic Science. (Quebec, Canada: Canadian Government Publishing Centre) Ed. Mercer M. C., 28–34.
Smith J. E., Keane J., Mundy C., Gardner C., Oellermann M. (2022). Spiny lobsters prefer native prey over range-extending invasive urchins. I. C. E. S. J. Mar. Sci. 79, 1353–1362. doi: 10.1093/icesjms/fsac058
Smith J. E., Keane J., Oellermann M., Mundy C., Gardner C. (2023). Lobster predation on barren-forming sea urchins is more prevalent in habitats where small urchins are common: a multi-method diet analysis. Mar. Freshw. Res. 74, 1493–1505. doi: 10.1071/MF23140
Smoothey A. F., Gray C. A., Kennelly S. J., Masens O. J., Peddemors V. M., Robinson W. A. (2016). Patterns of occurrence of sharks in Sydney Harbour, a large urbanised estuary. PloS One 11, e0146911. doi: 10.1371/journal.pone.0146911
Stewart J., Rowling K., Hegarty A. M., Nuttall A. (2010). Size and age at sexual maturity of snapper Pagrus auratus in New South Wales 2008/09 Vol. 27 (Industry and Investment NSW).
Taylor JR, L.R. (1972). A revision of the shark family Heterodontidae (Heterodontiformes, Selachii) (San Diego: University of California).
Trowbridge C. D., Little C., Plowman C. Q., Williams G. A., Pilling G. M., Morritt D., et al. (2019). No ‘silver bullet’: Multiple factors control population dynamics of European purple sea urchins in Lough Hyne Marine Reserve, Ireland. Estuar. Coast. Shelf Sci. 226, 106271. doi: 10.1016/j.ecss.2019.106271
Twiname S., Fitzgibbon Q. P., Hobday A. J., Carter C. G., Oellermann M., Pecl G. T. (2022). Resident lobsters dominate food competition with range-shifting lobsters in an ocean warming hotspot. Mar. Ecol. Prog. Ser. 685, 171–181. doi: 10.3354/meps13984
Underwood A. J., Clarke K. R. (2005). Solving some statistical problems in analyses of experiments on choices of food and associations with habitat. J. Exp. Mar. Biol. Ecol. 318, 227–237. doi: 10.1016/j.jembe.2004.12.014
Underwood A. J., Kingsford M. J., Andrew N. L. (1991). Patterns in shallow subtidal marine assemblages along the coast of New South Wales. Austral. Ecol. 16, 231–249. doi: 10.1111/j.1442-9993.1991.tb01050.x
Walker T. I., Gason A. S. (2007). “Shark and other chondrichthyan byproduct and bycatch estimation in the Southern and Eastern Scalefish and Shark Fishery,” in Final report to Fisheries and Research Development Corporation Project No. 2001/007. July 2007 (Primary Industries Research Victoria, Queenscliff, Victoria, Australia).
Weiss H. M., Lozano-Álvarez E., Briones-Fourzán P. (2008). Circadian shelter occupancy patterns and predator–prey interactions of juvenile Caribbean spiny lobsters in a reef lagoon. Mar. Biol. 153, 953–963. doi: 10.1007/s00227-007-0867-x
Wickham H. (2016). ggplot2: Elegant Graphics for Data Analysis (New York: Springer-Verlag). Available at: https://ggplot2.tidyverse.org.
Wickham H., François R., Henry L., Müller K., Vaughan D. (2023). Dplyr: A Grammar of Data Manipulation. R package version 1.1.1. Available online at: https://CRAN.R-project.org/package=dplyr.
Wolfe B. (2021). Physiological and behavioural mechanisms underpinning the climate-driven range extension of snapper Chrysophrys auratus in southeast Tasmania. (Hobart, Tasmania: University Of Tasmania). doi: 10.25959/23249579.v1
Woodings L. N., Murphy N. P., Liggins G. W., Miller M. E., Ballinger G. M., Lau S. C., et al. (2021). Population genomics of the Eastern Rock Lobster, Sagmariasus verreauxi, during spawning stock recovery from over-exploitation. I. C. E. S. J. Mar. Sci. 78, 2448–2459. doi: 10.1093/icesjms/fsab117
Wraith J. A. (2007). Assessing reef fish assemblages in a temperate marine park using baited remote underwater video. (Wollongong, Australia: University of Wollongong Thesis). Available at: https://ro.uow.edu.au/theses/12/.
Young M. A., Foale S., Bellwood D. R. (2014). Impacts of recreational fishing in Australia: historical declines, self-regulation and evidence of an early warning system. Environ. Conserv. 41, 350–356. doi: 10.1017/S0376892914000046
Keywords: Great Southern Reef, Palinuridae, Echinodermata, temperate reef, predator dynamics, feeding ecology
Citation: Day JK, Knott NA, Swadling DS, Huggett MJ and Gaston TF (2024) Assessing lobster and co-predator feeding rates on barrens-forming sea urchins in South East Australia. Front. Mar. Sci. 11:1418506. doi: 10.3389/fmars.2024.1418506
Received: 16 April 2024; Accepted: 02 September 2024;
Published: 04 October 2024.
Edited by:
Ana M. Faria, University of Porto, PortugalReviewed by:
Ben Gutzler, Wells National Estuarine Research Reserve, United StatesCopyright © 2024 Day, Knott, Swadling, Huggett and Gaston. This is an open-access article distributed under the terms of the Creative Commons Attribution License (CC BY). The use, distribution or reproduction in other forums is permitted, provided the original author(s) and the copyright owner(s) are credited and that the original publication in this journal is cited, in accordance with accepted academic practice. No use, distribution or reproduction is permitted which does not comply with these terms.
*Correspondence: Jeremy K. Day, SmVyZW15LkRheTEwQHVvbi5lZHUuYXU=
Disclaimer: All claims expressed in this article are solely those of the authors and do not necessarily represent those of their affiliated organizations, or those of the publisher, the editors and the reviewers. Any product that may be evaluated in this article or claim that may be made by its manufacturer is not guaranteed or endorsed by the publisher.
Research integrity at Frontiers
Learn more about the work of our research integrity team to safeguard the quality of each article we publish.