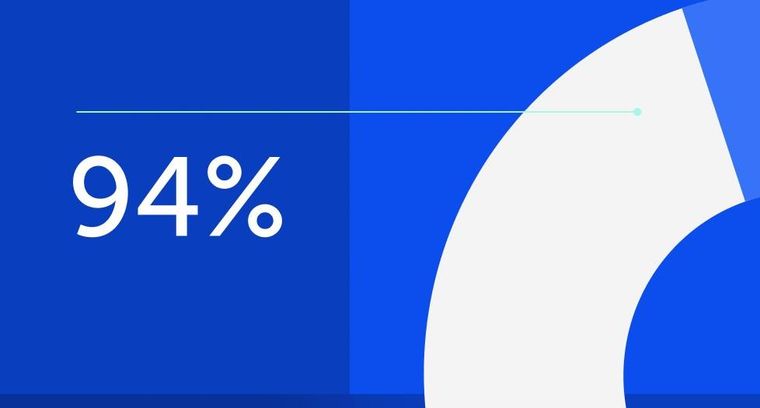
94% of researchers rate our articles as excellent or good
Learn more about the work of our research integrity team to safeguard the quality of each article we publish.
Find out more
ORIGINAL RESEARCH article
Front. Mar. Sci., 15 July 2024
Sec. Marine Affairs and Policy
Volume 11 - 2024 | https://doi.org/10.3389/fmars.2024.1418435
This article is part of the Research TopicAdvances in Marine Environmental Protection: Challenges, Solutions and PerspectivesView all 41 articles
Area-based management tools (ABMTs), including marine protected areas (MPAs) are often static and fail to reflect the dynamic realities of marine ecosystems. Marine ecosystems are characterized by their embodiment of constant change, which is further amplified by anthropogenic stressors, particularly climate change. ABMTs and MPAs are, however, premised on an implicit assumption of environmental equilibrium as their boundaries and management framework are often fixed and difficult to adjust. This article seeks to lay bare the tension between static conservation strategies and the deeply and inherently dynamic nature of marine ecosystems. It further seeks to advance the notion of dynamic ABMTs, proposing an integrated conceptualization of ABMT governance, one that is more apt to respond to the type of challenges that the dynamism of complex marine ecosystems presents. Dynamism, in this context, is broadly construed as encompassing three dimensions: spatial, with fluid and adjustable conservation measures; normative, denoting a volatile and adaptive management framework that utilizes ecological and management thresholds as an initiator for adaptive, timely, and prospective approaches to enhance management outcomes; and institutional, i.e., sufficiently flexible and dynamic institutional machinery overseeing ABMT implementation. Following a comprehensive conceptualization of dynamic ABMTs, the article addresses the question whether the legal frameworks governing the ocean can sustain such a dynamic mode of ocean governance.
The contemporary environmental epoch is characterized by change and uncertainty (Ortuño Crespo et al., 2020). Ever-intensifying climate change and biodiversity loss are rapidly altering the environmental conditions, putting the resilience of marine ecosystems to the test and forcing them to adapt. These changes are often non-linear, adding to the already complex and dynamic nature of ecosystems. Marine ecosystems, in particular, are characterized by their embodiment of constant change, which is further amplified by anthropogenic pressures. Our endeavours to protect these ecosystems should account for this dynamism, however, these considerations are largely absent in the design of area-based management tools (ABMTs), specifically for existing marine protected areas (MPAs). Rather, they are premised on an implicit assumption of environmental equilibrium (Allee et al., 1974; Cuddington, 2001)1. These spatial management tools are in virtually all instances static in their design; their spatial delineation and conservation measures are often fixed and difficult to amend due to strenuous decision-making processes and rigid institutional arrangements.
The present paper puts forward an alternative conceptual framework for ABMTs in a way that better reflects the dynamic nature of the ocean. A dynamic ABMT (hereinafter: dABMT) design is proposed that encapsulates three spheres of dynamism: spatial, normative, and institutional. It further utilizes ecological and management thresholds as an initiator for adaptive, timely, and prospective approaches to enhance management outcomes. The result is a macro-level conceptualization of dABMTs, that, although elements thereof require further research and elaboration, holds the potential for application across diverse marine regions and ecosystems. It moreover accounts for temporal variation in ecosystem processes, as it builds upon the temporal model developed by Ortuño Crespo et al. (2020). This model encompasses four temporal scales at which the movement of species, habitats, and ecosystems occur. Their framework facilitates the development of management strategies that appropriately match the spatial and temporal scales of ocean changes (Simmons et al., 2021).
In light of existing literature on dynamic ocean governance (Craig, 2012; Hobday et al., 2014; Maxwell et al., 2015; Ortuño Crespo et al., 2020), particularly regarding ABMTs and MPAs (Cashion et al., 2020; Maxwell et al., 2020), this article distinguishes itself by offering a novel synthesis of spatial, normative, and institutional dynamism. In doing so, the paper builds upon existing research by drawing from existing theories and concepts such as adaptive management, the use of thresholds in ocean management, adaptive governance, and social-ecological resilience, utilizing and applying them in the context of dABMT governance. This integrated approach aims to bridge monodisciplinary theoretical concepts by merging various theoretical insights, thereby advancing the discourse on dABMTs.
At the outset, a few terminological remarks are in order. Firstly, the term ‘dynamic’ denotes “characterized by constant change, activity, or progress” when referring to a process or system (Oxford English Dictionary, 2021). This choice of nomenclature is adopted since the proposed ABMT design primarily aims to incorporate the ever-changing nature of marine ecosystems, and it corresponds with the established jargon in the MPA literature. Secondly, the terms AMBTs and MPA warrant further clarification. While there is no agreed-upon definition of either term, ABMTs are generally understood to be geographically defined areas within which human activities, such as shipping or fishing, are regulated for one or more purposes in order to minimize their (potential) adverse impacts on the ecosystem and its biodiversity (BBNJ, 2023, art. 1(1))2. ABMTs simply denote the management of processes and human activities in a given area, whether for conservation purposes or other objectives. There are a variety of ABMTs available ranging from single-sector tools (e.g., vulnerable marine ecosystems (VMEs); fisheries closures) to cross-sectoral tools (e.g., marine reserves), with the degree of protection varying from low to highly protected. They thus incorporate a high degree of flexibility due to their broad range of application, spanning a spectrum ranging from sectoral management restrictions on a single activity to more integrated approaches where restraints are imposed on all human interferences. As we will see later on, this flexibility leaves room for dynamism in their application, which is why the present article builds on the concept of ABMTs.
However, this does not preclude MPAs, which are part of the wider portfolio of tools encapsulated within the overarching concept of ABMTs. MPAs are distinct from most other ABMTs as they tend to be more holistic and cross-sectoral in nature. In addition, they put a stronger emphasis on conservation, which is aptly illustrated by the definition contained in the Agreement for the Conservation and Sustainable Use of Biological Diversity in Areas Beyond National Jurisdiction (BBNJ Agreement). Therein, MPAs are defined in article 1(9) as “a geographically defined marine area that is designated and managed to achieve specific long-term biological diversity conservation objectives and may allow, where appropriate, sustainable use provided it is consistent with the conservation objectives”. This emphasis on conserving components of biodiversity necessitates a more integrated approach to mitigating human activities (Klerk, 2021)3. which has been shown to be a key element in successful conservation strategies (Sala and Giakoumi, 2017). Consequently, it comes as no surprise that MPAs are widely regarded as indispensable instruments in our endeavors to mitigate ocean threats, conserve biodiversity, and enhance resilience to environmental impacts (UNEP-WCMC et al., 2021)4.
The remainder of this article is structured as follows. The subsequent section presents an overview of the relevant literature depicting the intrinsic as well as human-induced complexity and dynamism of marine ecosystems. It moreover juxtaposes this dynamism to the static nature of ABMTs, thereby laying the foundation for section 3, which forms the core of this article as it comprehensively introduces the concept of dABMTs, discussing their spatial, normative and institutional elements, including the use of thresholds in ocean management. Section 4 addresses the question of whether the legal frameworks governing the ocean can sustain such a dynamic ABMT design, and finally, section 5 provides a summary of the key findings and offers concluding remarks.
The approach to area-based management as outlined in this article seeks to better align governance of human activities with the fluid and dynamic nature of the marine environment. It thus emanates from scientific considerations, a brief discussion of which is in place before delving into the substantive issues relating to ABMT design.
The marine environment is, indeed, deeply and inherently dynamic—even more so than terrestrial ecosystems, from which our current static approach to protection is derived. Although the ocean and the atmosphere are both subject to the same laws of fluid dynamics, they have very different physical properties such as density, thermal expansion coefficient, and viscosity (Steele et al., 2019). These different properties manifest in various ways. For instance, plankton and most fish, unlike insects and birds, exhibit near-neutral buoyancy, minimizing the energy expended to remain suspended (Steele et al., 2019). This buoyancy and dissolved nutrients allow for primary production in the upper, illuminated sections of the water column, enabling suspended food webs without a solid foundation. Thus, whole ecosystem components can float and flow with the marine currents as seen in rivers. Take, for instance, the Sargasso Sea, a rich and diverse ecosystem providing essential habitat for many endangered species, hailed as the ‘golden floating rainforest of the Atlantic Ocean’ as it is based upon the floating Sargassum macroalgae (Laffoley et al., 2011).
The ocean’s inhabitants are in constant motion due to the currents, tides, and waves, and marine animals dwelling in the water column must actively orient and swim to maintain their position or travel to distant foraging areas (Putman, 2018). In temperate coastal ecosystems, tidal currents serve as the primary drivers of ecosystem dynamics, influencing various levels of the food chain, from primary productivity to zooplankton, fish, and top predators (Embling et al., 2012). Currents play a pivotal role in pelagic ecosystem dynamics inter alia by facilitating the dispersal of marine species, transporting eggs and larvae over vast distances. Oceanic circulation further influences the success of spawning, dispersal, and settlement processes (Dubois et al., 2016).
Marine species are thus generally more mobile and subject to passive transport compared to those living on land5. This elevated mobility is particularly evident on a larger spatial scale, as marine mammals are capable of undertaking migrations spanning far greater distances than terrestrial species. For instance, Western North Pacific Gray Whales demonstrated the longest documented mammalian migration in a single year, with one female completing a round trip of 22,511 kilometers (Mate et al., 2015). It is not just marine mammals that migrate across the ocean. Fish, too, are known to engage in transoceanic migrations. The Atlantic bluefin tuna offers a well-documented example, as they migrate seasonally between the Gulf of Mexico and the eastern Atlantic and Mediterranean Sea (Luschi, 2013). Sharks and turtles are known to undertake similar journeys (Boyle et al., 2009). These migrations do not only occur horizontally but also vertically through different depths of the water column, known as diel vertical migration (DVM). It is said to be the largest synchronized movement of biomass, observed for a number of marine and freshwater species (Sims et al., 2005; Berge et al., 2009; Mehner and Kasprzak, 2011). Zooplankton, for instance, normally remains in deeper waters during the day and rises at dusk towards the surface to feed (Sims et al., 2005).
Human interference, and climate change in particular adds another layer of dynamism. The ocean has absorbed more than 93% of the excess heat from greenhouse gas emissions since the 1970s, causing their temperatures to rise (IPCC, 2021). Marine life responds to these changes in the environment by shifting their geographical range, generally towards the poles (Lenoir and Svenning, 2014)6. Many marine species have already done so, moving at an average rate of up to ~70 km per decade (Poloczanska et al., 2016). Notably, these range shifts occur at different speeds and depths, impacting all layers of ocean space. Deep-sea species’ velocities are already impacted by changes in ocean temperature, even more so than those living close to the surface (Brito-Morales et al., 2020).
These human-driven changes in spatial distribution also have an impact on how ecosystems function, as well as on their composition. In a warming ocean, warm-water species populations are expected to increase, and cold-water species populations to decline (Poloczanska et al., 2016). In the Arctic, for instance, marine food web structures and ecosystem functionality are projected to undergo substantial changes as large piscivorous species are rapidly replacing smaller bottom-dwelling species (Kortsch et al., 2020; Ingvaldsen et al., 2021). Species respond to changes in their environment in different ways, leading to disruptions in their interactions and ultimately to the emergence of new biotic communities and rapid shifts in ecosystem functioning. Ecosystem functioning is further altered by climate-induced changes in species abundance (Sutton-Grier and Sandifer, 2018).
Some ecosystems may even vanish completely, such as warm-water coral reefs, which are likely to be eliminated by 2040-2050, even in lower greenhouse gas emission scenarios (Hoegh-Guldberg et al., 2017). Such cases qualify as regime shifts, which are shifts between the states of different systems and are defined as large, persistent, and often unexpected changes that might occur on different temporal and spatial scales (Cooper et al., 2010; Sguotti and Cormon, 2018). Another example of a regime shift is the weakening of thermohaline circulation (THC) in the ocean, also described as an overturning of the world’s ocean, such as the slowing down of the Gulf Stream as a result of the weaker circulation of warmer surface waters and colder water in deeper layers of the ocean (Rahmstorf, 2006; Rocha et al., 2014). While the latter example is hypothesized to be an effect of climate change, other regime shifts may be traced back to a failure in marine management.
For instance, a regime shift in a marine food web may occur with the overexploitation of a key species (e.g. Atlantic cod or Antarctic krill). The status of (over)exploitation of a species is determined on an ecological threshold, a concept akin to the notion of tipping points, that have been crossed. Such shifts may be difficult to foresee, as the underlying drivers and internal feedback of a system can be subject to gradual change with minor impacts on the overall system state (Rahmstorf, 2006). For the purpose of this paper, ecological thresholds are broadly understood as a point, level, or value, that when crossed initiates or triggers an abrupt change or considerable response of the ecosystem in regards to the ecosystem quality or characteristics, including regime shifts or a state after an impact has occurred which may lead to a profound change not necessarily defined as a regime shift (Groffman et al., 2006; Foley et al., 2015).
While the high degree of complexity and dynamism in marine ecosystems is by no means a scientific novelty and a large body of scientific literature is devoted in particular to the impacts of climate change on marine life, these considerations seem to be largely absent in the current modus operandi of marine environmental protection, which primarily centers around designating long-term ABMTs with static boundaries. However, the above discussion has a number of fundamental implications for ABMT governance. First of all, the apparent differences between marine and terrestrial ecosystems (with the former exhibiting a higher degree of dynamism) raises the question of whether our current approach to environmental protection, which is derived from nature preservation on land is appropriate (Peters, 2020)7. These concerns are even stronger in relation to migratory species engaging in transoceanic journeys, which need to be considered when developing protection mechanisms. They moreover require protection in their spawning and feeding grounds, the location of which may vary seasonally.
When considering the impacts of climate change and other human interference with marine ecosystems, the merit of static approaches diminishes even further, as ABMT boundaries become void when species shift their geographical range (Tittensor et al., 2019; Ortuño Crespo et al., 2020; Goodman et al., 2022). Moreover, significant changes in ecosystem functioning and composition are occurring, effectively transforming ecosystems. Over time, they may bear little resemblance to their past state, leading to a potential mismatch between the protective measures put in place and the protective needs 8 require a more dynamic mode of protection.
The present section seeks to answer the question of how ABMTs can be conceptualized to optimally reflect the ocean’s dynamism. An integrated conceptualization of ABMT governance is put forward, one that is more apt to respond to the type of challenges that the dynamism of complex marine ecosystems presents. Dynamism, in this context, is broadly construed as encompassing three dimensions: spatial, normative, and institutional. It should be noted, however, that although conceptually separated, in practice these spheres are interactive and deeply intertwined.
Geographical, or spatial dynamism, denotes ABMTs with fluid, non-static boundaries, allowing them to respond to ecological, oceanographic, and atmospheric variability (Ortuño Crespo et al., 2020). As described in the foregoing section, variability exists not only in horizontal movements of marine life, but also vertically through different depths of the water column. Depending on the scope and objectives of existing ABMTs, they typically distinguish between activities in the water column (e.g. fishing, pelagic sampling), on the surface (e.g. shipping, recreational boating), and on the seabed (e.g. seabed mining, laying submarine cables). This limited vertical differentiation suffices, given that vertical migrations typically only occur within the water column.
Thus, horizontal boundary adjustments are adequate to attain a sufficient degree of spatial dynamism to account for the highly mobile nature of marine life. These adjustments should be aligned with the spatial as well as the temporal scales at which the movement of species, habitats, and ecosystems occur (Ortuño Crespo et al., 2020). Both of these variables are contingent upon the dynamic characteristics of the ecosystem in question. That is, which dynamic processes does the ABMT seek to address, and over which spatial and temporal scales do they unfold?
The spatial delineation of a geographically dynamic ABMT is fundamentally contingent upon its scope. This scope may concentrate on specific species, ecosystems, or activities, with the corresponding boundaries established accordingly. For example, dABMTs targeting a particular species are demarcated based on the geographical distribution of that species. An illustrative instance is the implementation of seasonal fisheries closures, designed to safeguard designated species within their feeding and breeding habitats, the location of which may vary9. In some cases, dABMTs may even target individual (often critically endangered) species. Examples of these extremely targeted and technologically intensive dABMTs are discussed in more detail below. Conversely, when a dABMT focuses on components of biodiversity, its spatial configuration mirrors that of a static ABMT, aligning with the location of the ecosystem in question. Depending on the extent to which that ecosystem is spatially dynamic, boundary adjustments can be implemented. Take, for instance, Australia’s Great Barrier Reef (GBR), which is subject to rapid coral bleaching as well as ongoing reef restoration projects (Ainsworth et al., 2016; Great Barrier Reef Foundation, 2024). The spatial location of its ABMT network could be adjusted to accommodate these changes.
As can be inferred from the above discussion, the temporal dimension of an ABMT is intricately linked to its spatial dimension, both being contingent upon accommodating dynamic processes. If changes are occurring rapidly, as is the case with the GBF, spatial adjustments must be implemented regularly10. Conversely, if a process unfolds over longer periods (e.g. changes in oceanic circulation), boundary adjustments can be implemented at longer intervals. While oceanic processes are deeply intertwined and nonlinear, Ortuño Crespo et al. (2020) identified four key temporal scales at which such changes occur: contemporary; intra-annual; multi-annual; and multi-decadal. Such a segmented conceptualization of temporality is useful, as it allows us to develop management strategies that appropriately match both the spatial and temporal scales of ocean changes. For example: to respond to the changes occurring on the contemporary scale (i.e., normal variability in species distributions, such as aggregations of prey and predators at productive ocean features), ABMT boundaries could be adjusted in near real-time. This requires continuous monitoring of the marine environment through satellite-based remote sensing and tracking technologies, as well as real-time integration of this information into rapid management responses (Hobday et al., 2014). On the other side of the temporal spectrum, the multi-decadal scale – which concerns redistributions of marine life in the face of climate change – requires adjustments at a far lower frequency, given that such movements occur at a relatively slow rate (Poloczanska et al., 2016).
The idea of protected areas with adjustable boundaries has been around since the early 2000s (Agardy et al., 2003; Tompkins and Adger, 2004) and quickly gained traction in scientific spheres, leading to a number of studies being conducted to assess their potential viability. Nonetheless, practical examples thereof are limited, and available studies are primarily using environmental modeling techniques. For example, modeling techniques, such as complex dynamic species distribution models (SDMs), which are traditionally static, are increasingly investigated for their use and as a more apt approach to management. While these are still in their infancy and are only seen as a waypoint in the advancement of climate-adapted management (Barnes et al., 2022), these have shown that dynamic approaches to environmental protection and resource management could yield a number of potential benefits. For one, dynamic spatial management is more focused: instead of protecting entire migration routes or habitats of (endangered) species year-round, real-time or seasonal adjustments for relevant areas could be implemented, which require significantly less spatial coverage. This was aptly illustrated in a modeling study, using a habitat prediction model conditioned with temperature preference data, which would be adjusted and updated frequently to match the real time conditions of the ecosystem (i.e. occurrence of targeted species in a predicted time-period) to allocate fishing effort and reduce by-catch. As such, it simulated a single temperature-dependent species’ habitat using a 12°C – 15°C temperature range (Maxwell et al., 2015). Across these temperature scenarios, the annual restricted fisheries time-area was between 62.1 and 86.1% less in a dynamic management approach, compared to a static one. Compared to traditional seasonal closures, between 34.2 and 82.0% less space was required (Maxwell et al., 2015).
In a similar vein, fisheries closures could be 2 to 10 times smaller than existing static closures, while still providing adequate protection of endangered species (Hazen et al., 2018). This higher degree of spatial efficiency could result in significant economic benefits. For instance, it has been estimated that in New England cod fisheries alone, the economic benefits of a dynamic approach, in comparison to a static one could be up to a third of the value of all of the cod landed, that is, USD 52,750,000 (Hazen et al., 2018).
Spatially dynamic ABMTs are not just economically attractive, they also bear the potential to yield substantial ecological benefits. One ecosystem modeling study, focusing specifically on the effects of climate change-induced redistributions and the effects thereof on MPA effectiveness, compared six MPA designs in a hypothetical ecosystem with 4°C warming at the end of the 21st century (Cashion et al., 2020). As such, the study used a static mass-balanced model modified by a temporal scenario and spatialized to a certain habitat, meaning that changing nature of the chosen (theoretical) ecosystem, in a designated spatial scale, was incorporated by including sea surface temperature change for a designated timeframe of 100 years. The results suggest that there is a significant difference in biomass in only one MPA design scenario, the ‘square shifting’ (i.e., dynamic) MPA, compared to the no-MPA scenario. This MPA design outperforms network MPA designs, as well as the ‘square static’ MPA design on all aggregate measures. The study shows that there may be some, albeit modest, benefits to dynamic MPAs for some species and fishing fleets (Cashion et al., 2020). Even in ecosystems that consist of fixed structures and are thus not an obvious choice for dynamic management, dynamic MPAs can bring about considerable conservation benefits. A study looking at dynamic MPAs that rotate among an appropriate subset of the entire reef system (e.g., rotating 10 protected areas between only 20 reefs in a 100-reef system) found that dynamic closures always lead to increased mean herbivore biomass (Game et al., 2009).
Although few in number, there are some real-life examples of ABMTs that embody some form of spatial dynamism. A well-known example of real-time dynamic spatial management, also referred to as dynamic ocean management (DOM)11, is the protection of the quota-managed southern bluefin tuna (Thunnus maccoyii) off the coast of south-eastern Australia. Southern bluefin tuna make annual winter migrations, during which they interact with a year-round tropical tuna longline fishery (Eastern Tuna and Billfish fishery) (Hobday and Hartmann, 2006). A temperature-based habitat model for southern bluefin tuna was developed to mitigate the bycatch of the non-target species. This model enables managers to estimate the distribution of tuna in real-time, providing a basis for decision-making regarding the placement of management boundaries. The projected location of the tuna is determined by matching temperature preferences to satellite-derived sea surface temperature data and vertical temperature data obtained from an oceanographic model. Based on this data, fishing access to areas where southern bluefin tuna are believed to be present is restricted. In a retrospective study on the efficacy of this approach, it was found that it is successfully mitigating the bycatch of southern bluefin tuna (Hobday and Hartmann, 2006).
A similar, albeit voluntary, program is the TurtleWatch project in Hawaii-based pelagic longline fishery. Based on data from satellite-tagged turtles, as well as ocean surface temperatures, maps were generated that indicate the area that fishers should avoid. The TurtleWatch program has successfully reduced the bycatch of the endangered loggerhead turtle from bycatch in the Hawaii-based longline fishery (Howell et al., 2008). However, significant bycatch continued to occur, which can primarily be attributed to the voluntary nature of the program. The majority of all loggerhead turtle bycatch occurred in the area where fishing was discouraged, which shows that the program was successful in indicating the area where bycatch is most likely to occur (Howell et al., 2008).
The above-outlined illustrates that the development of dynamic ecological modelling techniques is increasing and a number of ecological indicators, such as sea surface temperature, are used to identify and develop apt approaches to changing conditions. Yet, these models are highly complex and, in instances, still in their infancy, subject to high uncertainty and depend on oftentimes large, high-quality datasets. More research is certainly required, but these studies and examples indicate that there may be some considerable benefits to spatially dynamic ABMTs. Against the backdrop of the persistent acceleration of climate change, it can reasonably be expected that these benefits will increase even further, given the disruptive effects thereof on marine ecosystems. However, to account for the full extent of oceanic dynamism, mere adjustment of boundaries is insufficient.
Normative dynamism, i.e., the regular readjustment of ABMT measures can occur structurally through adaptive management (hereinafter: AM) of ABMTs, which is a thread that runs through and ties together all three spheres of dynamism. It can be described as “an approach to policy implementation in which ecological responses to management actions are monitored and compared to expected responses, then differences between observations and expectations are used to refine management in an iterative process” (Walters, 2010). AM provides a useful and comprehensive theoretical framework that, when applied in the context of area-based management, can result in a high degree of normative dynamism. Its first articulations date back to the mid-twentieth century, and AM has now matured to the extent that it can be considered a discipline of its own (Beverton and Holt, 1957).
Given the wealth of available literature, many different definitions and applications exist, however, three elements can be said to be universal: (i) planning, i.e., identifying and setting management objectives and identifying key uncertainties; (ii) designing and implementing actions to achieve the objectives; (iii) monitoring management effectiveness and reviewing the management program to improve outcomes (Fuentes et al., 2014). Importantly, AM differs from a trial-and-error approach due to its structural nature, with each step informing and guiding the others iteratively to improve the understanding of the socio-ecological system being managed.
AM is a conceptually robust approach to management. However, it is also under constant scrutiny in the scientific literature and has often been criticized (Lee, 2001; Allen and Gunderson, 2011; Månsson et al., 2023). It is well-established that its feasibility is heavily contingent on the socio-ecological context of a given region. More specifically, commentators found that AM is ill-suited for application to large, complex problems, as well as in contexts wherein very rapid or very slow changes in ecosystem states occur (Rist et al., 2012; Månsson et al., 2023). Indeed, in systems of intricate complexity, or where changes occur over an extended timespan, it becomes extremely difficult to distinguish the impacts of management interventions from the myriad other factors at play. Conversely, if changes occur very rapidly, the stepwise learning that is at the core of AM may be too slow to keep pace (Månsson et al., 2023). Within these parameters, however, AM has proven to be a potent framework to address uncertainty and predictability in complex systems (Westgate et al., 2013; Johnson et al., 2015).
Another often-hailed criticism of AM is the “inherent tension between its acknowledgment of complexity, uncertainty, and emergence and its presumption that goals and system metrics can be established to assess and adapt interventions” (Rist et al., 2012). Indeed, there is an implicit assumption inherent in AM that ecosystem responses can, at least to some degree, be predicted. Management is treated as an ‘adaptive learning process’ (Walters, 1986), and hence rests on an assumed predictability, i.e., “that the structural features of the resource system and the underlying environment are dynamically stable” (Williams, 2011). The predictability of marine ecosystems is a subject of considerable debate within ecological discourse. Gaüzère et al. (2018), for instance, posit that “ecological systems might intrinsically be unpredictable due to their complexity and the amount of chaotic, neutral, or stochastic processes impairing their dynamics”. When, in addition, the environmental conditions and ecological processes influenced by them are themselves changing, for example, due to climate change, further complications arise in the application of AM (Petchey et al., 2015). The impacts of climate change on the dynamics of marine biodiversity, as described previously, impair our ability to predict these impacts and to forecast the responses of biodiversity to climate change (Gaüzère et al., 2018). Even under gradual climate change, ecological systems demonstrate non-linear responses. As a consequence of the nonlinearity in the external factors influencing ecosystems, their dynamics may increase in complexity and we are only beginning to understand these changes (Saros et al., 2019).
Thus, in an increasingly unpredictable and dynamic world, AM may not always be the appropriate means to achieve normative dynamism. Indeed, concerns have been raised around AM’s excessive reliance on linear system models (McLain and Lee, 1996), and the “tendency among scientists to overstate their capability to measure complex functional relationships through experimentation.” (Allen and Gunderson, 2011). The adequacy of AM should be carefully considered on a case-by-case basis, which depends on myriad factors12. AM appears ill-suited for application to changes occurring on the multi-annual to multi-decadal scales, as well as to processes unfolding on large spatial scales. In such cases, management changes should be informed by observed, rather than projected changes. Indeed, a reactive approach to management that relies on adaptive monitoring may be more appropriate in the uncertain times we find ourselves in and could be used to supplement AM programs, making them more robust to unexpected or rapid ecosystem changes (Lindenmayer and Likens, 2009). A potential component of a reactive management approach is the (designation and) application of ecological and management thresholds. Therein, thresholds serve as tools applicable on a case-by-case basis and may provide a basis for the designation and implementation of adequate management of changing ecosystems.
In this context, two types of thresholds (see section 2), which are tools borrowed from existing management approaches that make use of thresholds, can be employed to address these challenges and foster a high degree of normative dynamism.
Ecological thresholds may serve as a tool to tackle environmental problems and as an initiator for adaptive, timely, and prospective approaches to enhance management outcomes (Foley et al., 2015). To this end, it is critical to understand the conditions leading to the crossing of thresholds, which is generally an undesirable outcome (Groffman et al., 2006). While it is true that ecological thresholds presuppose predictability of an ecosystem or ecosystem component and are context-specific, there may be ways to use thresholds as a tool to support an anticipatory and precautionary approach for the management and conservation of a dynamic ecosystem.
Thresholds are predictive when considering changes that occur on a multidecadal scale, as their establishment is based on historical data and modelling. Their interpretation relates to future scenarios. For more dynamic, rapid changes on shorter temporal scales, thresholds may be developed and based on real-time or near-real time data, which allow and support a reactive and more dynamic approach to ocean management. Therein, ecological thresholds can trigger management tools on a shorter time scale.
The (sudden) crossing of an ecological threshold initiates a reaction following an undesirable event, which ideally should have been avoided and may lead to a fundamental change that may not be easily restored or returned to the initial state. To avoid such outcome and allow for the implementation of management measures, an additional and different type of threshold may be operationalized.
This refers to the concept of a regulatory limit, which may also be called decision or management threshold (Hitchin et al., 2023). It is established on a level indicating the limit of human disturbance after which undesirable ecological change would occur and through which a response before the crossing of the tipping point is initiated. This level, further referred to as management threshold, is determined by decision-makers based on existing scientific information provided through and determined with the guidance of the ecological thresholds (see Figure 1). By creating a buffer zone between the level of the management threshold and the crossing of the ecological threshold, the aspect of uncertainty, which is inherent within ecological systems, may be accounted for. With the crossing of the management threshold a level of the last acceptable state of a resource, ecosystem or habitat is set. Thus, a designated period of time is created, allowing for the implementation of management measures to avoid the crossing of the ecological threshold. While the concept of thresholds arguably is predictive, it still represents a tool, when used strategically, that may support normative dynamism by contributing to the understanding of the marine ecosystem, its species, and complex interrelations. Such understanding is key for decision-making.
Figure 1 Simplified illustration of the proposed placement of management and ecological thresholds on a timescale in regard to ecological indicators indicating a potential regime shift.
The utilization of ecological and management thresholds to support decision-making processes concerning environmental problems is not new. For example, the use and integration of thresholds has been discussed previously in the context of deep-sea mining under the regulatory regime of the International Seabed Authority (ISA) (Hitchin et al., 2023). Therein, their value as part of an early warning system has been emphasized, especially referring to their ability to support the fulfilment of the legal obligations under the UN Convention on the Law of the Sea (UNCLOS), as well as to provide flexibility in the management of the activity in line with the present legal obligations.
Moreover, the use of thresholds is also highlighted in respect of the EU Marine Strategy Framework Directive (EU MSFD). Thresholds are established and used based on defined MSFD criteria, which reflect the predominant pressures and impacts on the environment. The criteria and their linked thresholds contribute to the achievement of a Good Environmental Status (GES). Accordingly, they serve as tools for the management of EU waters through cooperation on a Union, regional, or subregional scale (e.g. in the framework of Regional Seas Conventions) and ultimately lead to an “environmental status of marine waters where these provide ecologically diverse and dynamic oceans and seas which are clean, healthy and productive within their intrinsic conditions” (GES) (MFSD, 2008, art. 3(5)).
The UN Fish Stocks Agreement (UNFSA, 1995) is another example for the use of thresholds, on both ecological as well as management scales. Article 6 provides for the establishment and utilization of so-called stock-specific and region-specific reference points to be established by Regional Fisheries Management Organizations (RFMOs), which are designated as the relevant institutional bodies. The article is given further substance through the guidelines for the application of precautionary reference points provided in Annex II, defining the precautionary reference point as an “estimated value derived through an agreed scientific procedure, which corresponds to the state of the resource and of the fishery, and which can be used as a guide for fisheries management.” (UNFSA, 1995, art. 1). Moreover, two possible types of reference points are set out. These are: (i) conservation, or limit, reference points and (ii) management, or target, reference points, which in both cases shall be stock specific and include sources of uncertainty (UNFSA, 1995, art. 2 and 3). The first type aims to set boundaries intended to constrain the harvesting within safe biological limits, referring to the maximum sustainable yield (MSY), which is at the same time determined to be the minimum standard of reference points to be set by the responsible organization/entity (UNFSA, 1995, art. 7). While the first type is akin to the concept of ecological thresholds which are purely scientific, the second type is intended to meet management objectives and falls under the concept of management thresholds. Notably, management reference points shall account for both, ecological and socio-economic aspects (i.e. the type of fisheries) of the stock. What is more, they serve as a trigger for pre-agreed conservation and management activities, such as “harvest control rules” meaning that the taking of fish stocks shall be kept within safe biological limits (UNFSA, 1995, art.2; Baez et al., 2016). This may be understood as a mechanism allowing for automatic protection of the targeted resource (UNFSA, 1995, Annex II, art.4). The Agreement further deals with uncertainty and the possibility of data gaps, in which case provisional reference points shall be set. However, these may be revised based on monitoring and improved data availability (UNFSA, 1995, art. 6).
While several uncertainties regarding the development of reference points under the UNFSA remain, an example of the application of thresholds, in practice, are real-time closures based on ‘move-on rules’, which is a form of DOM. Accordingly, a threshold triggers a move-on rule and determines the shift of a fishing activity and the implementation of a targeted closure of an area for a temporary period. Relevant thresholds are determined based on the fishing or bycatch quota, aiming to reduce bycatch of juveniles and non-target species (Dunn et al., 2013). The closure may be implemented on a broader scale, with closures lasting from days to weeks over various distances, thus embodying the consolidation of the temporal and spatial scale (Dunn et al., 2016). In the case of the Eastern Australian long-line fishery, which has been highlighted in section 4.1, another form of a DOM approach constitutes an oceanographic closure, which is defined by environmental conditions, and may be implemented daily or bi-weekly (Lewison et al., 2019).
The management strategies outlined in the foregoing sections, that is, aligning the spatial and normative fabric of ABMTs with the inherent complexity and dynamism of the ecosystems they seek to protect, are contingent upon sufficiently flexible and indeed, dynamic, institutional machinery for their implementation. Institutional dynamism denotes a governance structure that is capable of sustaining the required degree of dynamism for the implementation of dABMTs.
There is, however, no one-size-fits-all institutional approach to achieve this. Ostrom and Cox argue that we should ‘move beyond panaceas’, that is, proposing oversimplified institutional prescriptions for complex environmental issues (Ostrom and Cox, 2010). Rather, institutional processes should be matched to the scale and characteristics of the particular social-ecological system, with a view to avoid mismatches between ecosystems and institutions (Young, 2002). Young refers to this as the problem of ‘fit’, that is, the “congruence or compatibility between ecosystems and institutional arrangements created to manage human activities affecting these systems”. He proposes an approach of institutional diagnostics, which starts from the premise that one size does not fit all when it comes to designing institutions bestowed with a conservation mandate and stresses the need to identify critical features of a specific problem, subsequently undertaking an endeavor to determine institutional arrangements that are most adept at addressing those aspects effectively (Young, 2002). He further argues that the effectiveness of institutional frameworks protecting the environment is “determined in considerable measure by the degree to which they are compatible with the biogeophysical systems with which they interact”. As such, it is key to acknowledge and internalize the human component of coupled human-natural systems when designing institutional models to govern them.
It is thus essential to be cognizant of the differences among ecosystems for which dABMTs are designated and carefully study their characteristics to align the institutional machinery overseeing their implementation accordingly. The aforementioned temporal scales are a useful framework to identify dynamic processes targeted by ABMTs, determining the frequency at which spatial and normative adaptations should occur, and subsequently calibrating the institutional structures accordingly (e.g., by structurally incorporating monitoring, review and decision-making processes at the required intervals).
This process of mirroring and alignment is key to fostering social-ecological resilience of intertwined natural-human systems, that is, the capacity of social-ecological systems to adapt or transform in the face of change without shifting to a new regime with a different set of processes and structures (Walker and Salt, 2006). Like ecosystems, social-ecological systems are complex systems with non-linear, dynamic processes that occur at various spatial and temporal scales. To govern them effectively and sustainably, their institutional components should, in parallel with ecological paradigms, embody a high degree of diversity and redundancy of institutions at multiple organizational levels, from individual actors to actor groups, organizations and governments (Jones et al., 2013).
The need of institutions to operate in sync with the dynamics of ecosystems finds resonance with adaptive governance scholarship, a theoretical framework for “addressing uncertainty and change with institutions and policy processes that reflect the social context” (Quimby, 2023). Adaptive governance theory stresses the importance of adaptability as a key component of resilience in social-ecological systems (Dietz et al., 2003; Folke et al., 2005). Folke et al. (2005) identify the need for multilevel governance systems to not only synchronize with ecosystem dynamics but also to anticipate changes and develop the capacity to endure external perturbations. This requirement constitutes one of the four key factors necessary to foster adaptive governance. They further stress the importance of acquiring reliable ecological data to feed into adaptive management processes, and, in a similar vein, to “build knowledge and understanding of resource and ecosystem dynamics” (Folke et al., 2005). Lastly, institutional flexibility and multilevel governance systems are identified as an important aspect of adaptive governance. Young (2002) echoes the importance of institutional flexibility, which he finds is crucial to the success of efforts to alleviate or eliminate mismatches. This is particularly true, he argues, “when knowledge regarding dynamics of key ecosystems is limited at the time of regime creation and where the ecosystems are prone to nonlinear and even transformative changes” (Young, 2002), as is often the case with ecosystems befitting protection through dABMTs.
Adaptive governance is particularly applicable to marine contexts, effectively addressing the spatial scale and unpredictability inherent in these environments (Quimby, 2023), as discussed previously, and appears well-suited for overseeing dABMT implementation and management. However, specific challenges emerge when applying adaptive governance in marine settings. For instance, Quimby stresses the need to “recognize existing social and political dimensions, support social learning and equity”, rather than conceptualizing the oceans as empty and ahistorical. These concerns are even stronger in relation to dABMTs due to their spatially dynamic nature and heavy reliance on scientific data. Therefore, it is crucial that decision-making processes consider the historical and cultural significance of the ocean areas designated for dABMTs. Similarly, stakeholder participation, which Quimby identifies as a vital component of adaptive governance, may be complicated by the varying presence and activities of individuals involved in marine fisheries. The spatially dynamic nature of dABMTs necessitates continuous stakeholder engagement rather than intermittent consultations solely prior to dABMT designation.
Building and sustaining an institutional structure that fully reflects the complexity, diversity, and non-linearity occurring at various spatial and temporal scales within marine ecosystems poses a significant challenge, if not an insurmountable one. In an international context, achieving institutional dynamism as portrayed here is especially challenging. International legal institutions often have rigid and time-intensive decision-making processes13, and in a transboundary context, fostering cooperation among States and international organizations is imperative but also entails considerable time investments.
There are, however, some international legal frameworks that leave ample scope for institutional flexibility and dynamism, such as the UNFSA, which seeks to foster greater cooperation between States in the conservation and management straddling and highly migratory fish stocks. To this end, RFMOs – the appropriate institutions to implement management measures – are required by virtue of 8 to “tak[e] into account the specific characteristics of the subregion or region”, as well as to agree on “the area of application … and the characteristics of the subregion or region, including socio-economic, geographical and environmental factors” (article 9). These provisions resonate with the above discussion on aligning institutional machinery with the characteristics of socio-ecological systems.
Institutional flexibility can moreover be observed in that, while States are under an obligation to cooperate, they are free to shape their cooperation as they see fit; they can do so through an organization (RFMO) or arrangements (RFMA). It is not specified how such an organization or arrangement must be structured. Thus, while there are undoubtedly many pitfalls on the way to achieving institutional dynamism in an international context, it should not be dismissed as an unattainable goal from the outset. Ultimately, a lot will boil down to whether enough political will can be harnessed to restructure and align institutions with the dynamic nature of the ocean. As Holling and Anderson aptly put it, “until modern human institutions are built on ecological dynamism, and designed to flex with natural variability, their principal impact will be to impede nature, not to sustain it” (Holling and Anderson, 1996).
Having clarified the conceptual intricacies of dABMTs, the question arises whether there is scope for implementing such dynamic modes of ABMT governance under the existing legal constellation governing the ocean. This question can, broadly speaking, be answered affirmatively; the law of the sea, although not mentioning ABMTs or MPAs anywhere (notwithstanding the BBNJ Agreement, to which we turn shortly) leaves ample room for implementing such a tool. Given its framework nature, among the main functions of UNCLOS is to allocate jurisdiction and sovereign rights in coastal States’ maritime zones, which are balanced against the rights and duties of foreign States14. Within the parameters of this jurisdictional framework, States are free to give substance to their duty to protect the marine environment (UNCLOS, 1994, art. 192 et seq)15.
Thus, given that UNCLOS does not contain any specific obstacles to the implementation of dABMTs, States are free to include this tool in their marine environmental strategies. The examples of spatially dynamic ABMTs discussed earlier demonstrate that some States have already done so. However, domestic legal and institutional contexts vary significantly and may often impede the successful implementation of dABMTs. Distinct legal frameworks may apply to different activities, and coordination can be complicated by a fragmented institutional landscape where, in addition to the State, various regional and local agencies and bodies hold competencies.
An illustrative example of these complexities is the case of the California Current System (CCS) offshore California, in the United States, where seasonal upwelling events take place. The timing, evolution, intensity, and duration of coastal upwellings in the CCS exhibit significant seasonal variation (Bograd et al., 2009), making this area well-suited for dynamic conservation strategies. However, the legal and institutional landscape of the CCS is highly fragmented with at least 20 federal agencies tasked with implementing over 140 federal ocean-related legal instruments. In addition, the States of California, Oregon and Washington have jurisdiction within 3 nautical miles off the coast, each with their own legislative frameworks, further adding to the legal complexity of the region, leading to transboundary issues and spatial mismatches between scales of ecosystems and governance systems (Crowder et al., 2006).
Similar issues may arise in other countries and regions, although the CCS presents an extreme example. Notwithstanding these challenges, a dynamic management program was developed within the CCS to mitigate whale-ship collisions16. While this program remains voluntary, the collaborative effort involving NGOs, universities, and the National Oceanic and Atmospheric Administration (NOAA) demonstrates that even in particularly challenging normative and institutional contexts like the CCS, opportunities for dABMT implementation exist.
In an international context, similar transboundary issues arise, albeit on a larger scale. While UNCLOS indeed does not prohibit the designation of dABMTs, one significant limitation lies in the jurisdictional architecture of the ocean, i.e., the fragmentation thereof in different maritime zones, subsuming each fragment into a different legal regime17. This is problematic for many reasons, which have been extensively discussed elsewhere (Tanaka, 2004), and can impede implementing dABMTs since the ecosystems and species they seek to protect are not bound by these artificial boundaries. The zonal and sectoral fragmentation of ocean space and the activities taking place therein make establishing dABMTs complex – especially if they straddle between two or more maritime zones – but not impossible. It would, however, require substantial political commitment and a high degree of international cooperation among States and international organizations.
Whereas UNCLOS does not mention ABMTs or MPAs, the 1992 Convention on Biological Diversity (CBD) in article 8 provides for the establishment of MPAs. This must be read in conjunction with the Kunming-Montreal Global Biodiversity Framework, adopted in December 2022, which replaces the Strategic Plan for Biodiversity 2011-2020 including the Aichi Targets and provides for AMBTs, including MPAs, as a key tool to protect at least 30% of the marine and coastal areas18v The CBD explicitly acknowledges the dynamic nature of ecosystems19, and defines protected areas broadly enough to encompass dABMTs and can hence be said to provide ample scope for their implementation.
In areas beyond national jurisdiction (ABNJ), i.e., the high seas and the Area, a distinct legal regime applies by virtue of Parts V and XI. The high seas are governed by Part V of UNCLOS, of which article 87, setting forth the ‘high seas freedoms’, forms the centre of gravity. Recently, however, this regime was supplemented by the new BBNJ Agreement, which seeks to “ensure the conservation and sustainable use of marine biological diversity of [ABNJ]” through, inter alia, the “establishment of a comprehensive system of [ABMTs], with ecologically representative and well-connected networks of [MPAs]” (BBNJ, 2023, art. 14(a)). Although the BBNJ Agreement does not explicitly allow for the designation of dABMTs, there appears to be some scope for their implementation. MPAs, in article 1(12), are described as “a geographically defined marine area that is designated and managed to achieve specific long-term biodiversity conservation objectives and may allow, where appropriate, sustainable use provided it is consistent with the conservation objective”. The definition is broad enough to encompass ABMTs with fluid boundaries, as long as the boundaries are geographically defined at any given point in time. Furthermore, normative dynamism, i.e., volatile conservation measures subject to regular review, also falls within the ambit of this definition, as it merely requires the area to be ‘managed’. Nothing in the Agreement explicitly precludes the possibility of designating dABMTs, and there are even some dynamic elements that could facilitate their implementation, such as the creation of a Scientific and Technical Body (BBNJ, 2023, art. 49) and the periodic monitoring and review of ABMTs (BBNJ, 2023, art. 26(3)).
Thus, it can be concluded that the principal legal frameworks governing the ocean do not contain any specific impediments for designating dABMTs, nor do they explicitly foster their implementation. Within these frameworks, however, there is ample space for flexibility and, indeed, dynamism, to designate these tools. The complexities in implementing these tools intensify when one ventures further offshore, where sovereignty erodes and is replaced by extensive rights and freedoms of third States, making international cooperation a prerequisite for their implementation. The newly adopted BBNJ Agreement provides a potential platform through which such cooperation can take place (Westholm and Argüello, 2024).
Equilibrium thinking is deeply ingrained in the way we conceptualize, manage, and design ocean space. This mode of thinking is pervasively present in all levels of ocean governance, from the jurisdictional architecture of the ocean to marine spatial management and conservation strategies. The present article commenced by unpacking ocean space, highlighting its inherently and deeply dynamic nature. Rather than a static equilibrium, the ocean is a movescape20, and hence our efforts to conserve the ocean and sustainably manage its resources should reflect these fundamental properties. Against this background, an integrated conceptualization of dABMTs was outlined in the main body of this article. In addition to spatial, normative and institutional dynamism, a set of ecological and management thresholds may be incorporated to trigger (pre-agreed) conservation measures, which prevent ecologically undesirable outcomes such as regime shifts.
It is worth noting, however, that the implementation of dABMTs, as proposed herein, is contingent upon specific contexts and should ideally complement rather than replace static MPAs. Indeed, dAMBTs present certain limitations that make them ill-suited for application in certain contexts. For instance, their successful implementation is heavily contingent upon the availability of reliable scientific data, thus necessitating the establishment of robust and comprehensive monitoring programs—a costly endeavor fraught with concerns regarding the politicization of scientific findings21. Similarly, setting up the required institutional and technological machinery requires substantial investments.
Conversely, spatially and normatively static MPAs, in addition to being less costly to implement, undeniably possess a higher degree of legal certainty, clarity and stability—qualities generally considered desirable in ocean governance. The present paper sought to highlight the (potential) ecological and economic benefits of dABMTs, that will ultimately need to be weighed against any potential downsides to their utilization, taking into account specific social-ecological context of a given region or ecosystem.
On the other hand, one must not forget the dire and ever-worsening state of our climate and biodiversity, in the midst of which we need to rethink and reshape the way in which we relate to and govern the natural world. We may need to venture into unknown and uncomfortable territory. After all, to safeguard our ocean and address the pressing challenges it faces, more than mere tinkering at the margins is required. We must embrace innovation, adaptability, and cooperation in the governance of our planet’s most vital and vulnerable ecosystems. Governance of marine activities should embody change, rather than resist it.
The original contributions presented in the study are included in the article/supplementary material. Further inquiries can be directed to the corresponding author.
BK: Writing – original draft. KH: Writing – original draft. RP: Writing – review & editing.
The author(s) declare that no financial support was received for the research, authorship, and/or publication of this article.
We would like to thank Professor Joanna Mossop for her insightful comments on an earlier version of this article.
The authors declare that the research was conducted in the absence of any commercial or financial relationships that could be construed as a potential conflict of interest.
All claims expressed in this article are solely those of the authors and do not necessarily represent those of their affiliated organizations, or those of the publisher, the editors and the reviewers. Any product that may be evaluated in this article, or claim that may be made by its manufacturer, is not guaranteed or endorsed by the publisher.
Agardy T., Bridgewater P., Crosby M. P., Day J., Dayton P. K., Kenchington R., et al. (2003). Dangerous targets? Unresolved issues and ideological clashes around marine protected areas. Aquat. Conservation: Mar. Freshw. Ecosystems. 13, 353–367. doi: 10.1002/aqc.583
Ainsworth T. D., Heron S. F., Ortiz J. C., Mumby P. J., Grech A., Ogawa D., et al. (2016). Climate change disables coral bleaching protection on the Great Barrier Reef. Science. 352, 338–342. doi: 10.1126/science.aac7125
Allee W. C., Emerson A. E., Park O., Al E. (1974). Principles of animal ecology (Philadelphia Etc: Saunders).
Allen C. R., Gunderson L. H. (2011). Pathology and failure in the design and implementation of adaptive management. J. Environ. Manage. 92, 1379–1384. doi: 10.1111/ele.12443
Alves-Pinto H., Geldmann J., Jonas H., Maioli V., Balmford A., Ewa Latawiec A., et al. (2021). Opportunities and challenges of other effective area-based conservation measures (OECMs) for biodiversity conservation. Perspect. Ecol. Conserv. 19, 115–120. doi: 10.1016/j.pecon.2021.01.004
Baez S., Fabra A., Friedman A., Galland G., Nickson A., Warwick L. (2016). Global progress toward implementing the united nations fish stocks agreement (PEW Charitable Trust). Available online at: https://www.pewtrusts.org/-/media/assets/2016/05/un-review-conf-brief-mar2016-final.pdf (Accessed 28 January 2023).
Barnes C. L., Essington T. E., Pirtle J. L., Rooper C. N., Laman E. A., Holsman K. K., et al. (2022). Climate-informed models benefit hindcasting but present challenges when forecasting species–habitat associations. Ecography 10, e06189. doi: 10.1111/ecog.06189
Bastille-Rousseau G., Wittemyer G. (2020). Characterizing the landscape of movement to identify critical wildlife habitat and corridors. Conserv. Biol. 35:1, 346–359. doi: 10.1111/cobi.13519
BBNJ. (2023). Agreement under the United Nations convention on the law of the sea on the conservation and sustainable use of marine biological diversity of areas beyond national jurisdiction, New York, 19 June 2023 (adopted 19 June 2023).
Berge J., Cottier F., Last K. S., Varpe Ø, Leu E., Søreide J., et al. (2009). Diel vertical migration of Arctic zooplankton during the polar night. Biol. Letters. 5, 69–72. doi: 10.1098/rsbl.2008.0484
Beverton R. J. H., Holt S. J. (1957). On the dynamics of exploited fish populations (Her Majesty’s Stationery Office: London). doi: 10.2307/1440619
Bograd S. J., Schroeder I., Sarkar N., Qiu X., Sydeman W. J., Schwing F. B. (2009). Phenology of coastal upwelling in the California Current. Geophysical Res. Lett. 36, 2. doi: 10.1029/2008GL035933
Boyle M. C., FitzSimmons N. N., Limpus C. J., Kelez S., Velez-Zuazo X., Waycott M. (2009). Evidence for transoceanic migrations by loggerhead sea turtles in the southern Pacific Ocean. Proc. R. Soc. B: Biol. Sci. 276, 1993–1999. doi: 10.1098/rspb.2008.1931
Brito-Morales I., Schoeman D. S., Molinos J. G., Burrows M. T., Klein C. J., Arafeh-Dalmau N., et al. (2020). Climate velocity reveals increasing exposure of deep-ocean biodiversity to future warming. Nat. Climate Change. 10, 576–581. doi: 10.1038/s41558-020-0773-5
Cashion T., Nguyen T., ten Brink T., Mook A., Palacios-Abrantes J., Roberts S. M. (2020). Shifting seas, shifting boundaries: Dynamic marine protected area designs for a changing climate. PloS One 15, e0241771. doi: 10.1371/journal.pone.0241771
Cooper G. S., Willcock S., Dearing J. A. (2010). Regime shifts occur disproportionately faster in larger ecosystems. Nat. Commun. 11, 1–10. doi: 10.1038/s41467-020-15029-x
Craig R. K. (2012). Ocean governance for the 21st century: making ocean zoning climate change adaptable. Harvard Environ. Law Rev. 36, 305.
Crowder L. B., Osherenko G., Young O. R., Airamé S., Norse E. A., Baron N., et al. (2006). Science policy forum 313, 617–618. doi: 10.1126/science.1129706
Cuddington K. (2001). The “Balance of nature” Metaphor and equilibrium in population ecology. Biol. Philosophy. 16, 463–479. doi: 10.1023/A:1011910014900
Dervovic M., Heinrich K. (2023). Law-science nexus in international law-making: perspectives from arctic fisheries governance. Nordic J. Int. Law 92, 599–622. doi: 10.1163/15718107-bja10073
Dietz T., Ostrom S., Stern P. C. (2003). The struggle to govern the commons. Science 302, 1907–1912. doi: 10.1126/science.1091015
Dubois M., Rossi V., Ser-Giacomi E., Arnaud-Haond S., López C., Hernández-García E. (2016). Linking basin-scale connectivity, oceanography and population dynamics for the conservation and management of marine ecosystems. Global Ecol. Biogeography. 25, 503–515. doi: 10.1111/geb.12431
Dunn D. C., Boustany A. M., Roberts J. A., Brazer E. O., Sanderson M., Gardner B., et al. (2013). Empirical move-on rules to inform fishing strategies: a New England case study. FISH and FISHERIES 15 (3), 359–375. doi: 10.1111/faf.12019
Dunn D. C., Maxwell S. M., Boustany A. M., Halpin P. N. (2016). Dynamic ocean management increases the efficiency and efficacy of fisheries management. Proc. Natl. Acad. Sci. 113, 668–673. doi: 10.1073/pnas.1513626113
Egevang C., Stenhouse I. J., Phillips R. A., Petersen A., Fox J. W., Silk J. R. (2010). Tracking of Arctic terns Sterna paradisaea reveals longest animal migration. Proc. Natl. Acad. Sci. 107, 2078–2081. doi: 10.1073/pnas.0909493107
Embling C. B., Illian J., Armstrong E., van der Kooij J., Sharples J., Camphuysen K. C. J., et al. (2012). Investigating fine-scale spatio-temporal predator-prey patterns in dynamic marine ecosystems: a functional data analysis approach. J. Appl. Ecology. 49, 481–492. doi: 10.1111/j.1365-2664.2012.02114.x
Foley M. M., Martone R. G., Fox M. D., Kappel C. V., Mease L. A., Erickson A. L., et al. (2015). Using ecological thresholds to inform resource management: current options and future possibilities. Front. Mar. Sci. 2. doi: 10.1007/s10021-003-0142-z
Folke C., Hahn T., Olsson P and Norberg J. (2005). Adaptive governance of social ecological systems. Annu. Rev. Environ. Resour. 30, 441–473. doi: 10.1146/annurev.energy.30.050504.144511
Fuentes M. M. P. B., Chambers L., Chin A., Dann P., Dobbs K., Marsh H., et al. (2014). Adaptive management of marine mega-fauna in a changing climate. Mitigation Adaptation Strategies Global Change. 21, 209–224. doi: 10.1007/s11027-014-9590-3
Game E. T., Bode M., McDonald-Madden E., Grantham H. S., Possingham H. P. (2009). Dynamic marine protected areas can improve the resilience of coral reef systems. Ecol. Letters. 12, 1336–1346. doi: 10.1111/j.1461-0248.2009.01384.x
Gaüzère P., Iversen L. L., Barnagaud J. Y., Svenning J. C., Blonder B. (2018). Empirical predictability of community responses to climate change. Front. Ecol. Evolution. 23. doi: 10.3389/fevo.2018.00186
Goldsworthy L., Press T., Haward M. (2023). China and Russia are blocking the creation of a third Antarctic marine protected area. Polar J. Available online at: https://polarjournal.ch/en/2023/06/19/china-and-russia-are-blocking-creation-of-a-third-antarctic-marine-protected-area/. (Accessed 2 July 2024).
Goodman C., Davis R., Azmi K., Bell J. D., Galland G. R., Gilman E., et al. (2022). Enhancing cooperative responses by regional fisheries management organisations to climate-driven redistribution of tropical Pacific tuna stocks. Front. Mar. Sci. 9. doi: 10.3389/fmars.2022.1046018
Great Barrier Reef Foundation. (2024). Reef restoration. Available online at: https://www.barrierreef.org/what-we-do/projects/reef-restoration (Accessed 28 January 2024).
Groffman P. M., Baron J. S., Blett T., Gold A. J., Goodman I., Gunderson L. H., et al. (2006). Ecological thresholds: the key to successful environmental management or an important concept with no practical application? Ecosystems. 9, 1–13. doi: 10.1007/s10021-003-0142-z
Hazen E. L., Scales K. L., Maxwell S. M., Briscoe D. K., Welch H., Bograd S. J., et al. (2018). A dynamic ocean management tool to reduce bycatch and support sustainable fisheries. Sci. Adv. 4, eaar3001. doi: 10.1126/sciadv.aar3001
Hitchin B., Smith S., Kröger K., Jones D., Jaeckel A., Mestre N., et al. (2023). Thresholds in deep-seabed mining: A primer for their development. Marine Policy. 149, 105505–105505. doi: 10.1016/j.marpol.2023.105505
Hobday A. J., Hartmann K. (2006). Near real-time spatial management based on habitat predictions for a longline bycatch species. Fisheries Manage. Ecology. 13, 365–380. doi: 10.1111/j.1365-2400.2006.00515.x
Hobday A. J., Maxwell S. M., Forgie J., McDonald J., Darby M., Seto K., et al. (2014). Dynamic ocean management: integrating scientific and technological capacity with law, policy, and management. Stanford Environ. Law J. 33:2, 125–165.
Hoegh-Guldberg O., Poloczanska E. S., Skirving W., Dove S. (2017). Coral reef ecosystems under climate change and ocean acidification. Front. Mar. Sci. 4. doi: 10.3389/fmars.2017.00158
Holling C. S., Anderson S. (1996). ““Dynamics of (dis)harmony in ecological systems”,” in Rights to nature. Eds. Hanna S. S., Folke K., Mäler K. G. (Washington D.C.: Island Press).
Howell E., Kobayashi D., Parker D., Balazs G., Polovina J. J. (2008). TurtleWatch: a tool to aid in the bycatch reduction of loggerhead turtles Caretta caretta in the Hawaii-based pelagic longline fishery. Endangered Species Res. 5, 267–278. doi: 10.3354/esr00096
Hughes T. P., Kerry J. T., Álvarez-Noriega M., Álvarez-Romero J. G., Anderson K. D., Baird A. H., et al. (2017). Global warming and recurrent mass bleaching of corals. Nature 543, 373–377. doi: 10.1038/s41586-018-0041-2
Ingvaldsen R. B., Assmann K. M., Primicerio R., Fossheim M., Polyakov I. V., Dolgov A. V. (2021). Physical manifestations and ecological implications of Arctic Atlantification. Nat. Rev. Earth Environ. 2, 874–889. doi: 10.1038/s43017-021-00228-x
IPCC (2021). Climate change 2021 working group I contribution to the sixth assessment report of the intergovernmental panel on climate change. Available online at: https://www.ipcc.ch/report/ar6/wg1/downloads/report/IPCC_AR6_WGI_SPM_final.pdf (Accessed 15 April 2024).
Johnson F. A., Boomer G. S., Williams B. K., Nichols J. D., Case D. J. (2015). Multilevel learning in the adaptive management of waterfowl harvests: 20 years and counting. Wildlife Soc. Bull. 39, 9–19. doi: 10.1002/wsb.518
Jones P. J. S., Qiu W., De Santo E. M. (2013). Governing marine protected areas: Social–ecological resilience through institutional diversity. Marine Policy. 41, 5–13. doi: 10.1016/j.marpol.2012.12.026
Klerk B. E. (2021). From undermining to strengthening. Int. J. Mar. Coast. Law 38, 107–139. doi: 10.1163/15718085-bja10112
Kortsch S., Primicerio R., Fossheim M., Dolgov A. V., Aschan M. (2020). Climate change alters the structure of arctic marine food webs due to poleward shifts of boreal generalists. Proc. R. Soc. B: Biol. Sci. 282, 20151546. doi: 10.1098/rspb.2015.1546
Laffoley D.d’A, Roe H. S. J., Angel M. V., Ardron J., Bates N. R., Boyd I. L. (2011). The protection and management of the sargasso sea. Summary science and supporting evidence case. Available online at: http://www.sargassoseacommission.org/storage/documents/Sargasso.Report.9.12.pdf. (Accessed 2 July 2024).
Lee K. N. (2001). “Appraising adaptive management,” in Biological diversity. Ed. Buck L. E. (Boca Raton: CRC Press).
Lenoir J., Svenning J. C. (2014). Climate-related range shifts - a global multidimensional synthesis and new research directions. Ecography 38, 15–28. doi: 10.1111/ecog.00967
Lewison R., Hobday A. J., Maxwell S., Hazen E., Hartog J. R., Dunn D. C., et al. (2019). Dynamic ocean management: identifying the critical ingredients of dynamic approaches to ocean resource management. BioScience 65, 486–498. doi: 10.1093/biosci/biv018
Lindenmayer D. B., Likens G. E. (2009). Adaptive monitoring: a new paradigm for long-term research and monitoring. Trends Ecol. Evol. 24, 482–486. doi: 10.1016/j.tree.2009.03.005
Luschi P. (2013). Long-distance animal migrations in the oceanic environment: orientation and navigation correlates. ISRN Zoology 2013, 1–23. doi: 10.1155/2013/631839
Månsson J., Eriksson L., Hodgson I., Elmberg J., Bunnefeld N., Hessel R., et al. (2023). Understanding and overcoming obstacles in adaptive management. Trends Ecol. Evol. 38, 55–71. doi: 10.1016/j.tree.2022.08.009
Mate B. R., Ilyashenko V. Y., Bradford A. L., Vertyankin V. V., Tsidulko G. A., Rozhnov V. V., et al. (2015). Critically endangered western gray whales migrate to the eastern North Pacific. Biol. Lett. 11, 20150071. doi: 10.1155/2013/631839
Maxwell S. M., Gjerde K. M., Conners M. G., Crowder L. B. (2020). Mobile protected areas for biodiversity on the high seas. Science Policy Forum. 367 (6475), 252–254. doi: 10.1126/science.aaz9327
Maxwell S. M., Hazen E. L., Lewison R. L., Dunn D. C., Bailey H., Bograd S. J., et al. (2015). Dynamic ocean management: Defining and conceptualizing real-time management of the ocean. Mar. Policy 58, 42–50. doi: 10.1016/j.marpol.2015.03.014
McLain R. J., Lee R. G. (1996). Adaptive management: Promises and pitfalls. Environ. Manage. 20, 437–448. doi: 10.1007/BF01474647
Mehner T., Kasprzak P. (2011). Partial diel vertical migrations in pelagic fish. J. Anim. Ecol. 80, 761–770. doi: 10.1111/j.1365-2656.2011.01823.x
MFSD. (2008). Directive 2008/56/EC of the European Parliament and of the Council of 17 June 2008 establishing a framework for community action in the field of marine environmental policy . OJ L 164, 25.6 (Marine Strategy Framework Directive).
Ortuño Crespo G., Mossop J., Dunn D., Gjerde K., Hazen E., Reygondeau G., et al. (2020). Beyond static spatial management: Scientific and legal considerations for dynamic management in the high seas. Mar. Policy 122, 104102. doi: 10.1016/j.tree.2011.04.007
Ostrom E., Cox M. (2010). Moving beyond panaceas: a multi-tiered diagnostic approach for social-ecological analysis. Environ. Conserv. 37, 451–463. doi: 10.1017/S0376892910000834
Petchey O. L., Pontarp M., Massie T. M., Kéfi S., Ozgul A., Weilenmann M., et al. (2015). The ecological forecast horizon, and examples of its uses and determinants. Ecol. Letters. 18, 597–611. doi: 10.1111/ele.12443
Peters K. (2020). The territories of governance: unpacking the ontologies and geophilosophies of fixed to flexible ocean management, and beyond. Philos. Trans. R. Soc. B: Biol. Sci. 375, 20190458. doi: 10.1098/rstb.2019.0458
Poloczanska E. S., Burrows M. T., Brown C. J., García Molinos J., Halpern B. S., Hoegh-Guldberg O., et al. (2016). Responses of marine organisms to climate change across oceans. Front. Mar. Sci. 3. doi: 10.3389/fmars.2016.00062
Quimby B. (2023). ““Adaptive governance for marine environments: methods, challenges, and lessons for ocean fisheries”,” in Handbook on adaptive governance. Ed. Juhola S. (Cheltenham: Edgar Elgar Publishing).
Rahmstorf S. (2006). ““Thermohaline ocean circulation”,” in Encyclopedia of quaternary science. Ed. Elias S. A. (Amsterdam: Elsevier).
Rist L., Campbell B. M., Frost P. (2012). Adaptive management: where are we now? Environ. Conserv. 40, 5–18. doi: 10.1017/S0376892912000240
Rocha J., Biggs R., Peterson G. (2014). Regime Shifts: What are they and why do they matter. Available online at: https://zahachtah.github.io/ItSS/assets/images/Regime_Shifts_Intro1.pdf (Accessed 15 April 2024).
Rosenberg D. (2023). The legal fight over deep-sea resources enters a new and uncertain phase (EJIL: Talk!). Available online at: https://www.ejiltalk.org/the-legal-fight-over-deep-sea-resources-enters-a-new-and-uncertain-phase/ (Accessed 15 April 2024).
Sala E., Giakoumi S. (2017). No-take marine reserves are the most effective protected areas in the ocean. ICES J. Mar. Sci. 75, 1166–1168. doi: 10.1093/icesjms/fsx059
Saros J. E., Anderson N. J., Juggins S., McGowan S., Yde J. C., Telling J., et al. (2019). Arctic climate shifts drive rapid ecosystem responses across the West Greenland landscape. Environ. Res. Letters. 14, 074027. doi: 10.1088/1748-9326/ab2928
Sguotti C., Cormon X. (2018). “Regime shifts – A global challenge for the sustainable use of our marine resources,” in Jungblut S., Liebich V., Bode M. eds. YOUMARES 8 – Oceans Across Boundaries: Learning from each other, 155–166. doi: 10.1007/978-3-319-93284-2_11
Simmons B. I., Blyth P. S. A., Blanchard J. L., Clegg T., Delmas E., Garnier A., et al. (2021). Refocusing multiple stressor research around the targets and scales of ecological impacts. Nat. Ecol. Evol. 5, 1478–1489. doi: 10.1038/s41559-021-01547-4
Sims D. W., Southall E. J., Tarling G. A., Metcalfe J. D. (2005). Habitat-specific normal and reverse diel vertical migration in the plankton-feeding basking shark. J. Anim. Ecology. 74, 755–761. doi: 10.1111/j.1365-2656.2005.00971.x
Steele J. H., Brink K. H., Scott B. E. (2019). Comparison of marine and terrestrial ecosystems: suggestions of an evolutionary perspective influenced by environmental variation. ICES J. Mar. Sci. 76, 50–59. doi: 10.1093/icesjms/fsy149
Sutton-Grier A. E., Sandifer P. A. (2018). Conservation of wetlands and other coastal ecosystems: a commentary on their value to protect biodiversity, reduce disaster impacts, and promote human health and well-being. Wetlands 39, 1297. doi: 10.1126/science.aai9214
Tanaka Y. (2004). Zonal and integrated management approaches to ocean governance: reflections on a dual approach in international law of the sea. Int. J. Mar. Coast. Law. 19, 483–514. doi: 10.4324/9781315564678
Tittensor D. P., Beger M., Boerder K., Boyce D. G., Cavanagh R. D., Cosandey-Godin A., et al. (2019). Integrating climate adaptation and biodiversity conservation in the global ocean. Sci. Adv. 5, eaay9969. doi: 10.1126/sciadv.aay9969
Tompkins E. L., Adger W. N. (2004). Does adaptive management of natural resources enhance resilience to climate change? Ecol. Soc. 9, 1–14. doi: 10.5751/ES-00667-090210
UNCLOS. (1994). (Montego Bay: United Nations Convention on the Law of the Sea). 1833 UNTS 3. Available at: https://www.un.org/depts/los/convention_agreements/texts/unclos/unclos_e.pdf.
UNEP-WCMC, IUCN, NGAS (2021). Protected planet report 2020. Available online at: https://livereport.protectedplanet.net/ (Accessed 9 November 2023).
UNFSA. (1995). Agreement for the Implementation of the Provisions of the United Nations Convention on the Law of the Sea of 10 December 1982 relating to the Conservation and Management of Straddling Fish Stocks and Highly Migratory Fish Stocks, New York, 4 August 1995 (adopted 4 August 1995, in force 21 December 2001) 2167 UNTS 3.
Walker B. H., Salt D. (2006). Resilience thinking: sustaining people and ecosystems in a changing world (Washington, DC: Island Press).
Westgate M. J., Likens G. E., Lindenmayer D. B. (2013). Adaptive management of biological systems: A review. Biol. Conserv. 158, 128–139. doi: 10.1016/j.biocon.2012.08.016
Westholm A., Argüello G. (2024). Dynamic ocean management in areas beyond national jurisdiction. Ocean Dev. Int. Law 4, 1–21. doi: 10.1080/00908320.2023.2296392
Williams B. K. (2011). Adaptive management of natural resources—framework and issues. J. Environ. Management. 92, 1346–1353. doi: 10.1016/j.jenvman.2010.10.041
Keywords: area-based management tools (ABMTs), marine protected areas (MPAs), biodiversity conservation, dynamic management, adaptive management, climate change
Citation: Klerk BE, Heinrich K and Primicerio R (2024) Beyond equilibrium thinking: dynamic area-based management tools in a changing ocean. Front. Mar. Sci. 11:1418435. doi: 10.3389/fmars.2024.1418435
Received: 16 April 2024; Accepted: 25 June 2024;
Published: 15 July 2024.
Edited by:
Mehran Idris Khan, University of International Business and Economics, ChinaReviewed by:
Robin Kundis Craig, University of Southern California, United StatesCopyright © 2024 Klerk, Heinrich and Primicerio. This is an open-access article distributed under the terms of the Creative Commons Attribution License (CC BY). The use, distribution or reproduction in other forums is permitted, provided the original author(s) and the copyright owner(s) are credited and that the original publication in this journal is cited, in accordance with accepted academic practice. No use, distribution or reproduction is permitted which does not comply with these terms.
*Correspondence: Bastiaan E. Klerk, YmFzdGlhYW4uZS5rbGVya0B1aXQubm8=
Disclaimer: All claims expressed in this article are solely those of the authors and do not necessarily represent those of their affiliated organizations, or those of the publisher, the editors and the reviewers. Any product that may be evaluated in this article or claim that may be made by its manufacturer is not guaranteed or endorsed by the publisher.
Research integrity at Frontiers
Learn more about the work of our research integrity team to safeguard the quality of each article we publish.