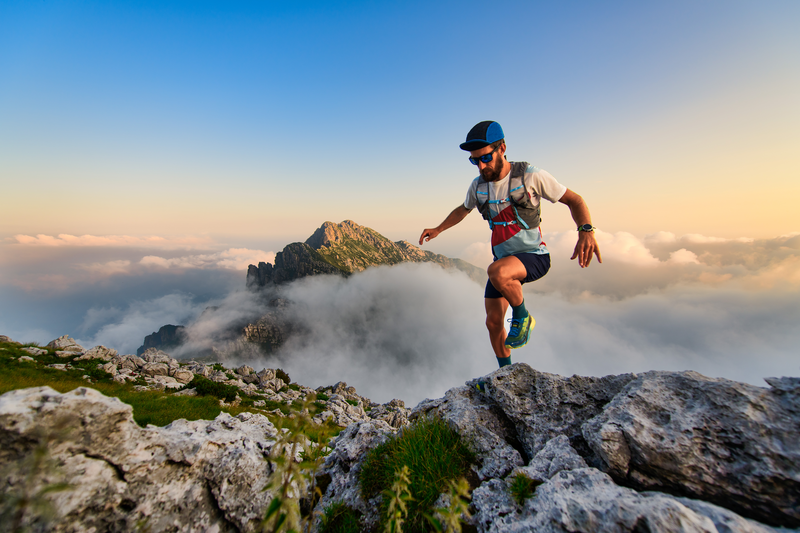
95% of researchers rate our articles as excellent or good
Learn more about the work of our research integrity team to safeguard the quality of each article we publish.
Find out more
ORIGINAL RESEARCH article
Front. Mar. Sci. , 07 January 2025
Sec. Marine Ecosystem Ecology
Volume 11 - 2024 | https://doi.org/10.3389/fmars.2024.1416546
Biofouling, the colonization of submerged surfaces by organisms including microorganisms, plants, algae, and animals, involves both natural and artificial environments. The serpulid worm Hydroides elegans, known as an invasive species in global port areas, creates extensive aggregations of calcareous tubes. In 2019, H. elegans was found attached to the research vessel ISABU in Korea, and a phylogenetic analysis was conducted to identify the species and determine its genetic characteristics. Following its detection, mesocosm experiments with plastic panels (PP, PE, PET, and EPS) were undertaken to investigate its recruitment and adaptation processes within new ecosystems. During the 12-week experiment, the biomass of H. elegans increased on all plastic types, significantly contributing to the overall biomass accumulation. This invasive species has replaced the previously prevalent native species Hydroides ezoensis, achieving dominance over other taxonomic groups in biomass. These findings demonstrate the significant ecological disruptions caused by non-indigenous species introduced through maritime activities, highlighting the urgent need for enhanced preventative and monitoring strategies
Marine biofouling, the accumulation of organisms on wet surfaces, poses challenges for maritime industries (Sarà et al., 2007; Fitridge et al., 2012; Bannister et al., 2019). Initiated by the adsorption of organic molecules such as proteins and polysaccharides, it leads to the attachment of microalgae, diatoms, and bacteria (Landoulsi et al., 2011; Mieszkin et al., 2013; Zeriouh et al., 2017; Nagaraj et al., 2018). These microorganisms form a protective biofilm that supports the colonization of larger organisms (Lappin-Scott and Bass, 2001; Ramsey and Whiteley, 2004). On ship hulls, this biological buildup increases weight and frictional resistance, leading to higher fuel consumption and maintenance costs due to reduced speed (Schultz, 2007). Additionally, biofouling facilitates the spread of invasive and non-indigenous species (NIS), with ports and harbors particularly at risk (Jaafar et al., 2012). These artificial substrates offer ideal conditions for invasive species to thrive, often to the detriment of native species (Ralston and Swain, 2014). The transportation of invasive species by ships, through hull fouling and ballast water, poses significant risks to coastal and estuarine environments (Piola and Johnston, 2006; Schwan et al., 2016). Globally, the impact of invasive species on native biodiversity and economic losses in sectors such as agriculture are escalating (Daigle and Herbinger, 2009; Castro et al., 2017).
Hydroides elegans is a tube-building worm recognized as one of the most prevalent biofouling organisms globally (Arenas-Mena and Li, 2014). Its presence has been recorded in numerous harbors, including Sydney Harbour, Australia; Pearl Harbor, Hawaii, USA; the southern coast of China; Hong Kong; and southeastern Brazil (Bastida-Zavala and ten Hove, 2002; Harder et al., 2002; Pettengill et al., 2007; Bao et al., 2010; Zhang et al., 2010; Chen et al., 2012; Schwan et al., 2016; Lema et al., 2019; Meng et al., 2019; Vijayan et al., 2019). The free-swimming planktotrophic larvae of Hydroides species, including H. elegans, are easily transported via ballast water, ship hulls, and aquaculture infrastructure, where they settle and mature into sessile adults (Xu et al., 2009; Zhang et al., 2014; Lema et al., 2019). The reproductive cycle of Hydroides species is defined by sexual dimorphism, external fertilization, and planktotrophic larval development from relatively small eggs (40–60 µm in diameter) (Kupriyanova, 2003). The transformation of H. elegans larvae into juveniles is influenced by various induction mechanisms, including contact with single-species bacterial biofilms or mature mixed biofilms (Unabia and Had, 1999; Toonen and Pawlik, 2001).
H. elegans is regarded as a highly problematic fouling organism and is noted for its efficient colonization of new submerged surfaces, a remarkable growth rate of up to 1.5 mm per day, and the ability to reach sexual maturity within a very brief period (9 days) in some tropical harbor conditions (Carpizo-Ituarte and Hadfield, 1998). In Pearl Harbor, Hawaii, H. elegans demonstrated the capability to densely colonize plastic mesh within 1–2 months; successful larval colonization hinges on a certain bacterial density, with neither larval settlement nor bacterial adherence being influenced by changes in surface wettability (Walters et al., 1997; Huggett et al., 2009). Additionally, this species exhibits a relatively short larval stage, leading to dense aggregation of its calcified tubes on submerged surfaces that can increase in thickness by several centimeters within 1–2 months, as observed in Pearl Harbor, Hawaii (Huang and Hadfield, 2003; Pettengill et al., 2007; Vijayan et al., 2019).
This serpulid worm defends itself from predation and environmental threats by forming calcareous tubes through a complex and regulated calcification process (Vinn et al., 2008; Tanur et al., 2010; Li et al., 2016). The planktotrophic larval stage of Hydroides is brief, with settlement triggered by chemical and bacterial signals allowing metamorphosis to complete within 4–5 days (Bryan et al., 1998; Lau and Qian, 2001; Toonen and Pawlik, 2001; Zhang et al., 2010; Vijayan et al., 2019). Adults of H. elegans produce calcareous tube material that can accumulate to several centimeters in thickness, potentially causing structural damage to ships and increasing frictional resistance during navigation (Zardus et al., 2008). These tubeworms adhere to surfaces and construct their calcareous tubes by incorporating seawater ions into a sophisticated inorganic–organic matrix through physiological transformations (Vinn et al., 2009; Vinn and Kupriyanova, 2011; Meng et al., 2018, 2019). The significant build-up of H. elegans tubes on man-made structures impacts the marine industry by obstructing aquaculture operations and clogging seawater cooling or intake systems in coastal facilities (ten Hove, 1974; Hadfield, 1998). The distribution of the species is limited to subtropical and tropical waters, with temperature being a crucial factor in its temperature tolerance, indicative of cryptic diversity within marine species (Qiu and Qian, 1997; Miller et al., 2009; Dennis and Hellberg, 2010; Sun et al., 2017).
Biofouling species such as H. elegans increase the surface roughness of ships, resulting in increased frictional resistance and reduced vessel speed, which inevitably lead to increased greenhouse gas emissions (Champ and Seligman, 1996). Various methods are employed to manage biofouling on ships, including prevention and removal. The most common method is to apply antifouling coatings containing copper or Irgarol 1051 (2-methythiol-4-tert-butylamino-6-cyclopropylamino-s-triazine) to the hull surface (Cima and Ballarin, 2012). Another approach is to directly remove hull-fouling organisms, either during dry docking of the vessel using water jets and scrapers, or underwater by divers or robots designed for hull cleaning (Park et al., 2022). Although technologies are being developed to capture debris generated by underwater robotic cleaning and prevent its release into the marine environment, there are limitations to using robots in less accessible areas such as thruster tunnels and seawater intake gratings. Among these, fouling release coatings, primarily silicon-based, stand out for their environmentally friendly profile, offering the benefits of low surface roughness and modulus (Hu et al., 2020).
As an invasive biofouling member of the Serpulidae family, H. elegans rapidly replaces indigenous species (Çinar, 2013). However, eradicating invasive species poses a complex challenge, requiring a prolonged commitment to keep populations low and mitigate ecosystem damage (Simberloff, 2014). While several projects have managed to reduce invasive populations, their long-term success is often hampered by discontinuous efforts, largely due to the financial burdens of ongoing projects (Simberloff, 2021). In response to the ecological threats posed by biofouling invasive species, the International Maritime Organization (IMO) has established guidelines for managing ship biofouling, with many nations actively working to identify and eliminate invasive biofouling organisms (IMO, 2011).
The ability of invasive species to adapt and prosper in new environments is often detrimental to native species and ecosystems. It is crucial to prevent the introduction of invasive species and to control their expansion once established (Simberloff and Rejmánek, 2011). Although several initiatives have successfully reduced invasive populations, they frequently fail due to high costs and a lack of sustainability (Simberloff, 2021). To address ecological disruptions caused by biofouling invasive species, the International Maritime Organization (IMO) formulated guidelines for biofouling management on ships (IMO [International Maritime Organization], 2011). In 2018, the IMO established the 5-year GloFouling Partnerships project, which aimed to minimize aquatic biofouling in developing countries. Within this period, the IMO 2020 policy was enacted to reduce pollutant emissions from ships in marine environments (De Castro et al., 2018; Sáez Álvarez, 2021). This policy left most pollutants (e.g., carbon dioxide, nitrogen oxides, sulfur oxides, and particulate matter) unregulated; consequently, global emissions from ships are projected to increase more than threefold between 2020 and 2050 (IMO, 2019). Overall, international attention to ship-based pollution is increasing, regulations are being strengthened, and there is a demand for efficient, environmentally friendly ship-management methods.
Invasive species can significantly affect coastal ecosystems and their management strategies. In 2019, H. elegans was detected for the first time on the hull and propeller of a research vessel in South Korea, displaying higher abundance than other typical fouling organisms, such as barnacles and mussels. Following this initial observation, a series of experiments using plastic panels was carried out to evaluate the adaptability of H. elegans to the local marine environment, focusing on attachment and settlement behaviors. The present study aimed to identify the preferred plastic substrate of this species by testing four different types of plastic plates. It also investigated the competitive interactions between H. elegans and native species, as well as their relationships with environmental factors in their habitats. This research enhances our understanding of the introduction, adaptation, and settlement of biofouling invasive species in Korea’s coastal waters, thereby supporting effective invasive species management strategies.
Individuals of H. elegans were collected from the research vessel ISABU in Jangmok Bay, Korea (34˚59.581 N, 128˚40.547 W) on August 23, 2019. The R/V ISABU journeyed from Korea, through the East China Sea and Taiwan, into the South China Sea (Figure 1), before passing Singapore and crossing the Strait of Malacca into the Indian Ocean. The vessel anchored in Sri Lanka and then in Mauritius. Samples of H. elegans, found in clusters on the ship’s side and propeller, were collected using a chisel and knife. These specimens were preserved with 70% alcohol for DNA analysis in situ and 10% formalin for morphological studies, and were identified to the species level in the laboratory.
Figure 1. Sailing route of research vessel ISABU. The red circle with a cross indicates the anchorage location and the black circle indicates the distribution area of H. elegans.
Morphological examinations were conducted using a DMC 4500 camera attached to a Leica M205C stereomicroscope (Leica, Wetzlar, Germany). Helicon Focus software (version 6; Helicon Soft Ltd., Kharkiv, Ukraine) facilitated the creation of composite images by stacking and merging multiple photographs. For scanning electron microscopy (SEM), samples were rinsed in absolute ethanol, dehydrated, coated with gold, and photographed using a Hitachi S-4300 SEM.
DNA was amplified through polymerase chain reaction (PCR) conducted using the DNeasy Blood and Tissue kit (Qiagen, Hilden, Germany), targeting 18S rRNA (18S), mitochondrial cytochrome c oxidase subunit 1 (COI) gene, and cytochrome b (cyt b) using the primers listed in Table 1. PCR was performed in 35-µL reaction mixtures. The genes were amplified with an initial denaturation at 94°C for 5 min, followed by five cycles of 94°C for 30 s, 47°C for 30 s, and 72°C for 40 s, then 30 cycles of 94°C for 30 s, 51°C for 30 s, and 72°C for 40 s, with a final 7-min elongation at 72°C. The PCR products were visualized on 1% agarose gels in 1× TAE buffer via electrophoresis.
Phylogenetic analysis was conducted to confirm species identity. This method determines evolutionary relationships among organisms by analyzing their genetic information. For phylogenetic reconstructions, mitochondrial COI sequences of Hydroides species were obtained from the National Center for Biotechnology Information (NCBI) database, using Scalibregma inflatum as an outgroup. And, phylogenetic tree of the Hydroides complex constructed using a combined dataset of the 18S, cytb, and COI genes, generated through MrBayes analysis. Sequences were aligned using ClustalW, and poorly aligned regions were trimmed using MEGA X (Kumar et al., 2018). The sequences were then compared against the GenBank database using the BLAST tool for species identication.
The optimal model for phylogenetic tree reconstruction was determined to be the K2+G evolutionary model according to the Akaike information criterion (AIC) in jModelTest v2.1.10 (Darriba et al., 2012). A maximum likelihood (ML) phylogenetic tree was constructed using RAxML (Stamatakis, 2014). The GTR+G+I model was selected as the best-fit nucleotide substitution model based on the AIC. Bootstrap analysis was performed with 1000 replicates to assess the robustness of the tree topology. Bayesian inference (BI) was conducted using MrBayes v3.2.7 (Ronquist et al., 2012). The analysis was run under the GTR+G+I model, with two independent runs of four Markov Chain Monte Carlo (MCMC) chains for 1,000,000 generations, sampling every 1000 generations. Convergence was assessed by ensuring an average standard deviation of split frequencies of < 0.01, with the first 25% of trees discarded as burn-in. The resulting phylogenetic trees were visualized and annotated using FigTree v1.4.4, and clade credibility values (posterior probability values for BI and bootstrap values for ML) were mapped onto tree branches to indicate statistical support for each clade.
A network analysis was performed to visualize the geographical distribution and connectivity of biological samples collected from various regions. Nodes represented sample groups, with node size proportional to the number of samples from each region, and color-coded pie charts indicating geographical origins (Europe, Oceania, America, Asia). The COI haplotype network (excluding outgroups) was reconstructed using POPART v.1.7 to investigate the population structure (Leigh and Bryant, 2015).
The Korea Institute of Ocean Science and Technology is located.in Jangmok Bay, which houses a floating mesocosm laboratory. This bay is off-limits to foreign ships, maintaining a relatively pristine marine ecosystem (Figures 1, 2). Using this mesocosm setup, the impact of microplastics on ecology, physiology, and eutrophication has been studied targeting mesozooplankton, phytoplankton, and bacterioplankton (Lee et al., 2005; Kim and Jang, 2008; Jang et al., 2010b, a; Shin et al., 2010; Baek et al., 2011; Park et al., 2011).
Figure 2. H. elegans: (A, B) encrusted on the hull of the research vessel underwater; (C) piece detached from the hull of the research vessel; (D) specimen; (E) operculum under scanning electron microscopy.
Our experimental design was adapted from the method used by Choi et al. (2011). Sampling frames consisted of four plastic panels composed of polypropylene (PP), polyethylene (PE), polyethylene terephthalate (PET), and expanded polystyrene (EPS), each measuring 30 cm × 30 cm, suspended from a polyvinyl chloride frame (Figure 3). For each sampling event, three frames were collected to serve as replicates. Sites in Jangmok Bay were sampled six times between July 20 and October 12, 2021, at 2-week intervals. The experimental period and interval were determined based on the observed settlement period of serpulid polychaetes (Choi et al., 2011; Schwan et al., 2016). To examine settlement patterns, the composition ratio and biomass of fouling organisms were analyzed by taxonomic group.
Figure 3. Schematic diagram of the recruitment experiment using four types of plastic (PP, polypropylene; PE, polyethylene; PET, polyethylene terephthalate; EPS, expanded polystyrene).
A conductivity–temperature–depth (CTD) profiler (YSI-ProDSS) was used to measure environmental parameters, including water temperature, salinity, turbidity (in nephelometric turbidity units [NTU], mg/L), dissolved oxygen (DO, mg/L), and pH, prior to sampling. These environmental parameters were incorporated into the analysis to evaluate potential correlations between habitat conditions and the recruitment, biomass growth, and distribution patterns of Hydroides elegans.
Data were analyzed using three-way analysis of variance (ANOVA), with the experiment (plastic panel type: PP, PE, PET, and EPS), time (levels: 2, 4, 6, 8, 10, and 12 weeks), and taxon (Hydroides elegans, Balanomorpha, Ascidiacea, Bivalvia, Anthozoa, and Bryozoa) as factors. Prior to conducting ANOVA, normality and equal variance assumptions were tested. The Shapiro-Wilk normality test indicated a deviation from normality (P < 0.050), while the equal variance test confirmed homoscedasticity (P = 1.000). Despite the failed normality test, the ANOVA procedure was applied as the design was robust to minor deviations from normality. A three-way ANOVA was performed to assess the effects of plastic type, time, and group on biomass. Post-hoc pairwise comparisons were conducted using the Holm-Sidak method at an overall significance level of 0.05 to isolate specific differences between groups.
Family Serpulidae Linnaeus, 1758.
Genus Hydroides Gunnerus, 1768.
Species Hydroides elegans (Haswell, 1883).
In total, 80 specimens were collected from the hull of research vessel ISABU by diving. These samples were promptly fixed in a 70% ethanol solution.
The branchiae of the specimens have up to 20 radioles on each lobe. The peduncle is long and funnel-shaped, with an interradial groove extending half its length featuring up to 10 spines. The operculum is equipped with up to 25 smooth verticil spines (Figure 2E). These verticil spines are pointed, uniform in shape and size, and have 0–4 pairs of lateral spinules, but they lack external spinules. At the base, all spines converge to form a conical shape, appearing slender and smooth beyond the interradial groove. The central teeth of the operculum have small spinules (Figure 2E). Collar chaetae are serrated, bayonet-shaped, have a well-developed breast, and have two to four shorts, pointed teeth that are denticulated in the proximal zone. A pseudoperculum is short and was observed in only five specimens (Figure 2D). The thorax comprises six chaetigers with limbate and capillary chaetae, and thoracic uncini are saw-shaped with four to five-pointed, fang-like teeth. The abdominal chaetiger count ranges from 20 to 62, featuring flat, trumpet-shaped chaetae; the width of the 3rd chaetiger reaches up to 0.9 mm. Uncini possess three to five teeth in a saw-shaped configuration. The total length can reach up to 18 mm, with the thorax measuring up to 1 mm in length. Operculum and branchiae constitute one-third of the total length (Figure 2D).
The holotype measures 5 mm in length and 0.9 mm in width. Paratypes vary, with lengths ranging from 2–10 mm and widths from 0.6–1.6 mm. All specimens were examined after the removal of their calcareous tubes.
H. elegans has often been confused with other species in previous studies due to morphological similarities. Notably, the shape of the operculum is similar to those of H. norvegica Gunnerus, 1768, H. longispinosa Imajima, 1976b, and H. multispinosa Marenzeller, 1884 (Gunnerus, 1768; Imajima, 1976; Marenzeller, 1884). H. elegans is distinguished by the number of teeth on the collar, the number of side spines, and the length of the central teeth.
Phylogenetic trees were reconstructed used mitochondrial COI sequences (Figure 4). The ML tree and BI analyses inferred from Hydroides mitochondrial COI sequences yielded a consistent topology for each region (Figure 4). The analysis provided strong support, with Hydroides species from this study aligning closely with existing H. elegans sequences (ML: 100%, BI: 85). The phylogenetic relationships within the Hydroides complex were inferred from a combined dataset of the 18S, cyt b, and COI genes, using MrBayes for analysis (Figure 5). The tree illustrates the evolutionary relationships among various Hydroides species, with posterior probability values indicated at the nodes. The study sample clusters closely with Hydroides elegans (Haswell, 1883) with a high posterior probability of 1, indicating strong support for this relationship. Other species in the tree include Hydroides amri, Hydroides sanctaecrucis, Hydroides minax, Hydroides trivesiculosa, and Hydroides norvegica. The close clustering of the study sample with H. elegans suggests it belongs to the same species or a closely related lineage.
Figure 4. Maximum Likelihood (ML) phylogenetic tree based on mitochondrial COI sequences of Hydroides species. Node labels support from ML and Bayesian inference.
Figure 5. MrBayes tree of Hydroides-complex constructed from combined dataset of 18S, cytb, and COI.
A network analysis using the mitochondrial COI gene of Hydroides elegans was conducted to examine regional variation (Figure 6). The results showed distinct clustering by region. European samples (green) formed a cohesive cluster, indicating high similarity and suggesting a shared environmental influence or species distribution. Oceania samples (pink) were fewer and relatively isolated, indicating unique species composition or ecological conditions. American samples (teal) were more dispersed, showing connections with both Europe and Asia, implying ecological connectivity or shared species. Asian samples (orange) showed overlap with both European and American samples, possibly due to historical biogeographical exchanges or similar environmental pressures. Strong connectivity within European and Asian clusters indicated internal homogeneity, while weaker, dispersed connections among American and Oceanian nodes suggested greater diversity.
Water environmental variables measured over 12 weeks provide valuable insights into the recruitment patterns of organisms (Table 2). Temperature was initially highest at 25.9°C, rising to 30°C in week 2. It then gradually decreased to 23.5°C in week 12, reflecting the transition from summer to fall. Salinity levels generally remained within a narrow range of 27.21–30.92 psu, with a low of 27.21 psu in week 6. Turbidity remained low, peaking at 0.5 NTU on 17 August. DO decreased from 135.8% (9.3 mg/L) initially to 79% (5.57 mg/L) in week 12. pH showed a slight downward trend from 8.22 to 7.83 during the study period, indicating slight acidification. Temperature and DO showed seasonal trends, while salinity, turbidity and pH remained relatively stable with minor fluctuations.
Table 2. Environmental variables in the experimental area (NTU, nephelometric turbidity units; TSS, total suspended solids; DO, dissolved oxygen).
In the 12-week experiment, initial observations revealed biofilm coverage on the panels by the second week, with few macro-organisms (Figures 7, 8). The average biomass increased over time across all four types of plastic. The taxa observed included Cnidaria, Echinodermata, Mollusca, Arthropoda, and Chordata. Cnidaria had the highest biomass, averaging around 60 g/m². Polychaeta also had a considerable biomass of approximately 30 g/m². By contrast, the biomass of taxa such as Echinodermata, Mollusca, and Bryozoa was much lower, often below 10 g/m² (Figure 9).
Figure 7. Photos of the plastic panel experiment (PP, polypropylene; PE, polyethylene; PET, polyethylene terephthalate; EPS, expanded polystyrene) at different experimental durations: (A) 2 weeks, (B) 4 weeks, (C) 6 weeks, (D) 8 weeks, (E) 10 weeks, (F) 12 weeks.
Figure 8. Recruitment and growth of H. elegans on PET (polyethylene terephthalate) panels at different experimental durations: (A) 6 weeks, (B) 8 weeks, (C) 10 weeks, (D) 12 weeks.
Figure 9. Average biomass and standard deviation of invertebrate taxa attached to plastic panel experiments.
The three-way ANOVA results for the biomass of invertebrates on plastic panels (Substrate: PP, PE, PET, EPS; Time: 2, 4, 6, 8, 10, 12 weeks; Taxon: Hydroides elegans, Balamorpha, Ascidiacea, Bivalvia, Anthozoa, Bryozoa) indicated that substrate type (Su) had no significant effect on biomass (F = 0.653, P = 0.583) (Table 3). However, time (Ti) and taxon (Ta) both significantly influenced biomass (F = 36.56, P < 0.001 and F = 46.788, P < 0.001, respectively). There was no significant interaction between substrate and time (Su x Ti, F = 0.185, P = 1) or between taxon and substrate (Ta x Su, F = 0.493, P = 0.94). However, the interaction between time and taxon (Ti x Ta) was significant (F = 5.576, P < 0.001), indicating varying biomass accumulation patterns among taxa over time.
Table 3. Results of three-way ANOVA of the biomass of invertebrates recruited on plastic panels (Substrate; PP, PE, PET, EPS/Time; 2, 4, 6, 8, 10, 12/Taxon; Hydroides elegans, Balamorpha, Ascidiacea, Bivalvia, Anthozoa, Bryozoa).
H. elegans exhibited an increase in biomass across all plastic types as the experiment progressed (Figure 10). Until the 10th week, biomass accumulation was comparable across all materials. However, by the 12th week, PP recorded the highest increase, followed by PE, with PET and EPS showing no significant changes between the 10th and 12th weeks. The growth of calcareous polychaete tubes contributed to an increase in overall biomass over the duration of the experiment.
Figure 10. Biomass of invertebrate taxa attached to plastic panels according to experiment duration (2, 4, 6, 8, 10, 12 weeks. (A) PP panels (polypropylene), (B) PE panels (polyethylene), (C) PET panels (polyethylene terephthalate), (D) EPS panels (expanded polystyrene).
This study marks the first observation of the biofouling invasive species H. elegans attached to a ship in Korea. Previously documented species of the Hydroides genus in Korea include H. albiceps, H. ezoensis, and H. uncinatus, with most of these species being well-established, particularly H. ezoensis, which is commonly found in coastal waters (Paik, 1989). Carpizo-Ituarte and Hadfield (1998) noted that H. elegans quickly becomes predominant on artificial substrates following its settlement (Carpizo-Ituarte and Hadfield, 1998). Given its quick settlement, dense populations, and the construction of robust calcareous tubes, H. elegans is recognized as a crucial contributor to fouling communities on man-made structures, which has significant ecological and economic repercussions (Chandra Mohan and Aruna, 1994; Nedved and Hadfield, 2009; Tovar-Hernández et al., 2009). While this study was limited to ship-based occurrences, the potential for tube-building worms such as H. elegans to spread across various man-made structures is evident. In other nations, this species has been identified on aquaculture farms and power stations, where it impairs energy efficiency, highlighting the necessity for effective countermeasures and anti-biofouling solutions.
Marine biological surveys in the northwestern Pacific Ocean have revealed the invasion of nonindigenous species (NIS) into certain regions, including the coastal waters of Korea (Chavanich et al., 2010). Notably, the South China Sea (SCS) has experienced a significant influx of NIS, which has had detrimental effects on biodiversity and local economies (Xiong et al., 2017). Several studies on hull-fouling organisms have been conducted in Korea, focusing on taxa ranging from bacteria to large invertebrates (Lee et al., 2008, 2024; Kim et al., 2021; Park et al., 2023; Hyun et al., 2024). For example, 15 invasive marine species (1 sponge, 2 bryozoans, 3 mollusks, 1 polychaete, 4 cirripedes, and 4 ascidians) were identified in a survey conducted in major Korean ports (Park et al., 2017). Among these, the serpulid tubeworm Ficopomatus enigmaticus resembles Hydroides elegans, which was found in the present study. Korean populations of F. enigmaticus originated from coastal waters of the Indian Ocean, including those off Australia (Dittmann et al., 2009), and were introduced to shallow seas worldwide, where they alter habitats and cause biofouling (Schwindt et al., 2004; McQuaid and Griffiths, 2014). This species also acts as a bioengineering organism by modifying the physical characteristics of the invaded environment and providing refuge for other species (Dittmann et al., 2009; Yee et al., 2019).
The research vessel involved in this study previously made anchorages in Sri Lanka and Mauritius in the Indian Ocean. The journey traversed several critical maritime regions, including the East China Sea, Taiwan, the South China Sea, Singapore, the Strait of Malacca, and Malaysia, before reaching Sri Lanka and Mauritius. In the vicinity of these routes, specifically in India near Sri Lanka, H. elegans was identified off the east coast in 2015, although Sri Lanka itself has not officially reported the species (Raghunathan et al., 2019). Singapore, known as a major global shipping and logistics center with numerous ports and extensive artificial marinas, faces a high risk of invasions by exotic marine species, including H. elegans and various invasive species such as fish, mollusks, dinoflagellates, and polychaetes (Jaafar et al., 2012; Seebens et al., 2013; Lim et al., 2017; Wells et al., 2019). A recent review of NIS in estuarine and marine environments of Singapore reported 17 invasive species, including 2 bivalve molluscs, 1 polychaete worm, 2 dinoflagellates, and 12 fish species (Jaafar et al., 2012); however, the authors did not discuss the potential negative impacts of these species on the marine environment. Similarly, the presence of H. elegans has been confirmed in Taiwan, Hong Kong, and China, regions that have experienced a rapid increase in the number of non-indigenous species and biofouling organisms (Mak, 1982; Yang and Sun, 1988; Paxton and Chou, 2000). This situation highlights the need for increased ship inspections and broader investigations along these international maritime routes, demonstrating the importance of managing bioinfestations at a national level.
Temperature levels are key factors influencing biofouling patterns, with the dominance of specific taxa suggesting favorable ecological conditions (Liu et al., 2020b). These findings underscore the need for further research on marine organisms’ interactions with plastic materials, especially concerning marine pollution and ecological impacts. In previous studies in the Jangmok mesocosm, calcareous polychaetes were identified as the dominant group in October, with evidence suggesting that accession occurred in August when water temperatures exceeded 25°C (Choi et al., 2011). In this study, water temperatures remained above 25°C from early July and surpassed 30°C in August before gradually decreasing, while the biomass of Hydroides elegans on various plastic substrates increased. At approximately 25°C, H. elegans larvae commence feeding 12 h post-fertilization and achieve metamorphic competence by the fifth day (Nedved and Hadfield, 2009). Many Hydroides species have temperature-restricted distributions; for example, H. elegans inhabits tropical and subtropical waters (Qiu and Qian, 1997), H. ezoensis is found in temperate waters, and H. dianthus is reported across a broad temperature range, from temperate to tropical regions (Zibrowius and Thorp, 1989). The declining trend in DO towards the end of this study, coupled with stable pH levels, may have favored certain organisms, such as Hydroides elegans, that are more tolerant of lower oxygen conditions. These environmental factors, particularly temperature and DO, likely influenced the overall biomass accumulation and the specific taxa that colonized the plastic substrates. These observations suggest that the water column environment in the study area is transitioning to a subtropical state, potentially leading to more frequent invasions by non-indigenous species.
Before this study, the research vessel ISABU was cleaned and treated with a copper-based antifouling paint and coating. Subsequently, it navigated through the South China Sea and Indian Ocean, docking in Mauritius and Sri Lanka. Calcareous polychaetes attached in patches where biofilms formed. Extended voyages will result in the increased accumulation of fouling organisms, which settle and adhere to substrates due to the influence of ocean currents (Callow and Callow, 2002). Species associated with the research vessel ISABU are widespread globally (Park et al., 2023), which increases the likelihood of their attachment to anchorages when the vessel enters Korean waters. Moreover, these species are resistant to copper-based antifouling coatings, which presents challenges in managing biofouling (Park et al., 2023). Copper stress is thought to have a significant effect on the settlement of H. elegans larvae, with the effect likely increasing with the exposure period (Bao et al., 2010). Recent efforts have aimed at enhancing the efficacy of fouling-release coatings and various studies have examined the settlement patterns of Hydroides larvae in relation to biofilms (Lau and Qian, 2001; Shikuma and Hadfield, 2005; Xu et al., 2009; Hu et al., 2020).
A previous study investigated the colonization patterns of sessile benthic organisms using artificial settlement plates, and revealed that the dominant species varied seasonally; in October, Ulva pertusa (Chlorophyta) and Hydroides ezoensis (tube worm) were the dominant species attached to the plates (Choi et al., 2011). Comparing experiments conducted in 2007 and 2011 to the current study, the major taxa observed did not differ. However, the dominant species shifted from H. ezoensis to H. elegans (Choi et al., 2011; Park et al., 2011). The present study was conducted from July to September, which coincides with the settlement period of tubeworms. Therefore, we infer that tubeworm settlement occurred earlier in our study compared to previous studies. The timing of settlement is closely linked to the inherent life cycle of a marine organism; it may vary among species but is generally fixed within species (Chung and Youn, 2012). Therefore, it is important to evaluate the influence of the settlement substrate on the larval settlement patterns of various marine species (Choi et al., 2011).
Invasive species may outperform native species; for example, Halophila stipulacea competes with native seagrasses, often replacing species such as Syringodium filiforme, Halodule wrightii, and Halophila decipiens (Christianen et al., 2019; Alidoost Salimi et al., 2021). In the Mediterranean Sea, the invasive seaweed Caulerpa taxifolia has replaced native seagrasses (Montefalcone et al., 2015). Hydroides elegans has a survival advantage because it can live in various environments differing in temperatures, salt concentrations, and oxygen levels (Mak and Huang, 1982; Link et al., 2009). Invasive species can outcompete native species for resources such as food, habitat, and light, which can lead to declines in the populations of native species (Montefalcone et al., 2015). These factors contribute to the ability of H. elegans to outcompete other species and survive in various environments (Shikuma and Hadfield, 2005; Nedved and Hadfield, 2009; Shikuma et al., 2016). The native calcareous polychaete in Jangmok Bay, H. ezoensis, appears to have been displaced by the invasive H. elegans; further experiments are needed to investigate the relationship between these two species.
Calcareous tube worms are causing many economic and ecological problems in many ports and countries, and their spread is increasing. Hydroides elegans is a notable biofouling invasive species frequently encountered in new ecosystems, such as on ship hulls (Bastida-Zavala et al., 2017). It adheres to artificial substrates, causing economic damage. Both H. elegans and H. ezoensis have been documented in Roman marinas, where they were identified as non-indigenous species, highlighting their status as long-established invaders in the Mediterranean (Ferrario et al., 2016). Recent discoveries include the newly identified Hydroides species H. amri, H. dolabrus, H. glasbyi, H. gracilis, H. lirs, H. nikae, and H. qiui, each posing potential biofouling threats (Kupriyanova et al., 2015; Sun et al., 2015, 2016; Tovar-Hernández et al., 2016; Read et al., 2017). The calcareous lugworm most frequently compared to H. elegans is Hydroides dirampha, which has greater tolerance of low salinity, with adult numbers exceeding those of H. elegans during the rainy season when salinity levels decrease markedly (Liu et al., 2020a). This species has not been reported in Korea, but continues to be monitored because it has appeared in many countries. The present study focused on identifying biofouling on an international research vessel, and further research is required for container ships traversing multiple nations. With thousands of vessels operational worldwide, local marine ecosystems and native species face ongoing threats from invasive species.
The serpulid worm H. elegans was identified in Korea for the first time in 2019, likely introduced via maritime vessels. A mesocosm experiment using plastic panels was conducted to investigate the recruitment and adaptation of this species within a new ecosystem. The findings revealed that H. elegans experienced an increase in biomass on all types of plastic materials, with the most significant growth observed on PP and PE by the 12th week. By this time, the biomass of H. elegans had surpassed that of barnacles, establishing its dominance over other taxonomic groups in biomass terms. These outcomes emphasize the importance of strengthening preventative actions and monitoring efforts to prevent the invasion of non-indigenous species through shipping activities.
The original contributions presented in the study are included in the article/supplementary material. Further inquiries can be directed to the corresponding author/s.
SK: Conceptualization, Data curation, Formal analysis, Investigation, Writing – original draft, Writing – review & editing. OY: Conceptualization, Funding acquisition, Supervision, Writing – review & editing.
The author(s) declare financial support was received for the research, authorship, and/or publication of this article. This research was supported by the Korea Institute of Ocean Science and Technology (PEA0204) and Korea Institute of Marine Science & Technology Promotion (KIMST) funded by the Ministry of Oceans and Fisheries (20210651).
We would like to thank R/V ISABU (Korea Institute of Ocean and Technology) for their cooperation.
The authors declare that the research was conducted in the absence of any commercial or financial relationships that could be construed as a potential conflict of interest.
All claims expressed in this article are solely those of the authors and do not necessarily represent those of their affiliated organizations, or those of the publisher, the editors and the reviewers. Any product that may be evaluated in this article, or claim that may be made by its manufacturer, is not guaranteed or endorsed by the publisher.
Alidoost Salimi P., Creed J. C., Esch M. M., Fenner D., Jaafar Z., Levesque J. C., et al. (2021). A review of the diversity and impact of invasive non-native species in tropical marine ecosystems. Mar. Biodivers. Rec. 14, 1–11. doi: 10.1186/s41200-021-00206-8
Arenas-Mena C., Li A. (2014). Development of a feeding trochophore in the polychaete Hydroides elegans. Int. J. Dev. Biol. 58, 575–583. doi: 10.1387/ijdb.140100ca
Baek S. H., Shin H. H., Choi H. W., Shimode S., Hwang O. M., Shin K., et al. (2011). Ecological behavior of the dinoflagellate Ceratium furca in Jangmok harbor of Jinhae Bay, Korea. J. Plankton. Res. 33, 1842–1846. doi: 10.1093/plankt/fbr075
Bannister J., Sievers M., Bush F., Bloecher N. (2019). Biofouling in marine aquaculture: a review of recent research and developments. Biofouling 35, 631–648. doi: 10.1080/08927014.2019.1640214
Bao W. Y., Lee O. O., Chung H. C., Li M., Qian P. Y. (2010). Copper affects biofilm inductiveness to larval settlement of the serpulid polychaete hydroides elegans (Haswell). Biofouling 26, 119–128. doi: 10.1080/08927010903329680
Bastida-Zavala J. R., McCann L. D., Keppel E., Ruiz G. M. (2017). The fouling serpulids (Polychaeta: Serpulidae) from United States coastal waters: An overview. Eur. J. Taxon. 2017, 1–76. doi: 10.5852/ejt.2017.344
Bastida-Zavala J. R., ten Hove H. A. (2002). Revision of hydroides gunnerus 1768 (Polychaeta: Serpulidae) from the western Atlantic region. Beaufortia 52, 103–179.
Boore J. L., Brown W. M. (2000). Mitochondrial genomes of galathealinum, helobdella, and platynereis: sequence and gene arrangement comparisons indicate that pogonophora is not a phylum and annelida and arthropoda are not sister taxa. Mol. Biol. Evol. 17, 87–106. doi: 10.1093/oxfordjournals.molbev.a026241
Bryan P. J., Kreider J. L., Qian P. Y. (1998). Settlement of the serpulid polychaete Hydroides elegans (Haswell) on the arborescent bryozoan Bugula neritina (L.): Evidence of a chemically mediated relationship. J. Exp. Mar. Bio. Ecol. 220, 171–190. doi: 10.1016/S0022-0981(97)00105-6
Burnette A. B., Struck T. H., Halanych K. M. (2005). Holopelagic Poeobius meseres (“Poeobiidae,” Annelida) is derived from benthic flabelligerid worms. Biol. Bull. 208, 213–220. doi: 10.2307/3593153
Carpizo-Ituarte E., Hadfield M. G. (1998). Stimulation of metamorphosis in the polychaete Hydroides elegans Haswell (Serpulidae). Biol. Bull. 194, 14–24. doi: 10.2307/1542509
Castro M. C. T., Fileman T. W., Hall-Spencer J. M. (2017). Invasive species in the Northeastern and Southwestern Atlantic Ocean: A review. Mar. pollut. Bull. 116, 41–47. doi: 10.1016/j.marpolbul.2016.12.048
Champ M., Seligman P. (1996). An introduction to organotin compounds and their use in antifouling coatings. Eds. Ma C., PF S. (Organotin: Springer, Dordrecht).
Chandra Mohan P., Aruna C. H. (1994). “The biology of serpulid worms in relation to biofouling control,” in Recent Developments in Biofouling control. Oxford & IBH. Eds. : Thompson M. F., Nagabhushanam ,. R., Sarojini R. (Oxford & IBH. Pub1 CO, New Delhi).
Chavanich S., Tan L., Vallejo B., Viyakam V. (2010). Report on the current status of marine non-indigenous speceis in the Western Pacific Region (Bangkok: Intergovernmental Oceanographic Commission Sub-Commission for the Western Pacific (IOC/WESTPAC)), 25.
Chen Z. F., Wang H., Qian P. Y. (2012). Characterization and expression of calmodulin gene during larval settlement and metamorphosis of the polychaete Hydroides elegans. Comp. Biochem. Physiol. - B. Biochem. Mol. Biol. 162, 113–119. doi: 10.1016/j.cbpb.2012.04.001
Choi J.-W., Park S.-H., Seo J.-Y. (2011). Recruitment patterns of sessile organisms on the artificial PVC panels in Jangmok Bay, southern coast of Korea. Korean. J. Malacol. 27, 29–33. doi: 10.9710/kjm.2011.27.1.029
Christianen M. J. A., Smulders F. O. H., Engel M. S., Nava M. I., Willis S., Debrot A. O., et al. (2019). Megaherbivores may impact expansion of invasive seagrass in the Caribbean. J. Ecol. 107, 45–57. doi: 10.1111/1365-2745.13021
Chung M. H., Youn S.-H. (2012). Ecological characteristics of the epiphytes on seagrass - II. Effects of physico-chemical factors on eelgrass (Zostera marina L.) and epiphytes. Korean. J. Environ. Biol. 30, 272–279.
Cima F., Ballarin L. (2012). Immunotoxicity in ascidians: Antifouling compounds alternative to organotins. III - The case of copper(I) and Irgarol 1051. Chemosphere 89, 19–29. doi: 10.1016/j.chemosphere.2012.04.007
Çinar M. E. (2013). Alien polychaete species worldwide: Current status and their impacts. J. Mar. Biol. Assoc. United. Kingdom. 93, 1257–1278. doi: 10.1017/S0025315412001646
Daigle R. M., Herbinger C. M. (2009). Ecological interactions between the vase tunicate (Ciona intestinalis) and the farmed blue mussel (Mytilus edulis) in Nova Scotia, Canada. Aquat. Invasions. 4, 177–187. doi: 10.3391/ai.2009.4.1.18
Darriba D., Taboada G. L., Doallo R., Posada D. (2012). JModelTest 2: More models, new heuristics and parallel computing. Nat. Methods 9, 772. doi: 10.1038/nmeth.2109
De Castro M. C. T., Vance T., Yunnie A. L. E., Fileman T. W., Hall-Spencer J. M. (2018). Low salinity as a biosecurity tool for minimizing biofouling on ship sea chests. Ocean. Sci. 14, 661–667. doi: 10.5194/os-14-661-2018
Dennis A. B., Hellberg M. E. (2010). Ecological partitioning among parapatric cryptic species. Mol. Ecol. 19, 3206–3225. doi: 10.1111/j.1365-294X.2010.04689.x
Dittmann S., Rolston A., Benger S., Kupriyanova E. (2009). “Habitat requirements, distribution and colonisation of the tubeworm Ficopo,” in Adelaide: matus enigmaticus in the Lower Lakes and Coorong (Adelaide: Report for the South Australian Murray-Darling Basin Natural Resources Management Board), 99.
Ferrario J., Ulman A., Marchini A., Saracino F., Occhipinti-Ambrogi A. (2016). Non-indigenous fouling species in the marina of Rome. Biol. Mar. Mediterr. 23, 224–225.
Fitridge I., Dempster T., Guenther J., de Nys R. (2012). The impact and control of biofouling in marine aquaculture: A review. Biofouling 28, 649–669. doi: 10.1080/08927014.2012.700478
Gunnerus J. E. (1768). Om Nogle Norske Coraller. Det Kongelige Norske Videnskabers Selskabs Skrifter. 4, 38–73, Available online at: https://gdz.sub.uni-goettingen.de/dms/load/img/?PPN=PPN481641912_0004&IDDOC=273812.
Hadfield M. G. (1998). The D P Wilson lecture. Research on settlement and metamorphosis of marine invertebrate larvae: Past, present and future. Biofouling 12, 9–29. doi: 10.1080/08927019809378343
Harder T., Lam C., Qian P. Y. (2002). Induction of larval settlement in the polychaete Hydroides elegans by marine biofilms: An investigation of monospecific diatom films as settlement cues. Mar. Ecol. Prog. Ser. 229, 105–112. doi: 10.3354/meps229105
Haswell W. A. (1883). On some new Australian tubicolous annelids. Proc. Linn. Soc. N. S. W. 7 (4), 633–638. Available online at: http://biodiversitylibrary.org/page/6462370.
Hu P., Xie Q., Ma C., Zhang G. (2020). Silicone-based fouling-release coatings for marine antifouling. Langmuir 36, 2170–2183. doi: 10.1021/acs.langmuir.9b03926
Huang S., Hadfield M. G. (2003). Composition and density of bacterial biofilms determine larval settlement of the polychaete Hydroides elegans. Mar. Ecol. Prog. Ser. 260, 161–172. doi: 10.3354/meps260161
Huggett M. J., Nedved B. T., Hadfield M. G. (2009). Effects of initial surface wettability on biofilm formation and subsequent settlement of Hydroides elegans. Biofouling 25, 387–399. doi: 10.1080/08927010902823238
Hyun B., Jang P. G., Jang M. C., Kang J. H., Kim J. H., Ki J. S., et al. (2024). Development of biological risk assessment protocols for evaluating the risks of in-water cleaning of hull-fouling organisms. J. Mar. Sci. Eng. 12, 234. doi: 10.3390/jmse12020234
Imajima M. (1976). Serpulinae (Annelida, Polychaeta) from Japan I. the genus Hydroides. Bulletin of the National Science Museum, Tokyo, Ser. A (Zoology). 2 (4), 229–248, Available online at: https://www.kahaku.go.jp/research/publication/zoology/download/02_4/BNSM020403.pdf.
IMO (2019). Note by the international maritime organization to the fifty-first session of the subsidiary body for scientific and technological advice (SBSTA 51) (Madrid, Spain). Available online at: https://www4.unfccc.int/sites/SubmissionsStaging/Documents/201911261754—IMO%20submission%20to%20SBSTA%2051_with%20annex.pdf (accessed January 11, 2021).
IMO (2011). Guidelines for the control and management of ships’ biofouling to minimize the transfer of invasive aquatic species (London, UK), 25.
Jaafar Z., Yeo D. C. J., Tan H. H., O’Riordan R. M. (2012). Status of estuarine and marine non-indigenous species in Singapore. Raffles. Bull. Zool. 14, 79–92.
Jang M. C., Shin K., Jang P. G., Lee W. J. (2010a). Relationship between environmental factors and short-term variations of mesozooplankton during summer in Jangmok Bay, South Coast of Korea. Ocean. Polar. Res. 32, 41–52. doi: 10.4217/OPR.2010.32.1.041
Jang M. C., Shin K., Lee T., Noh I. (2010b). Feeding selectivity of calanoid copepods on phytoplankton in jangmok bay, south coast of Korea. Ocean. Sci. J. 45, 101–111. doi: 10.1007/s12601-010-0009-0
Kim Y., Jang M. (2008). Temporal distribution of planktonic Ciliates in Jangmok Bay, South coast of Korea. Ocean. Polar. Res. 30, 419–426. doi: 10.4217/OPR.2008.30.4.419
Kim H. J., Park J. S., Lee T. K., Kang D., Kang J. H., Shin K., et al. (2021). Dynamics of marine bacterial biofouling communities after initial Alteromonas genovensis biofilm attachment to anti-fouling paint substrates. Mar. pollut. Bull. 172, 112895. doi: 10.1016/j.marpolbul.2021.112895
Kumar S., Stecher G., Li M., Knyaz C., Tamura K. (2018). MEGA X: Molecular evolutionary genetics analysis across computing platforms. Mol. Biol. Evol. 35, 1547–1549. doi: 10.1093/molbev/msy096
Kupriyanova E. K. (2003). "Live history evolution in Serpulimorph polychaetes: a phylogenetic analysis," in Proceedings of the 7th International Polychaete Conference held in Reykjavik (Iceland), 105–114.
Kupriyanova E. K., Sun Y., Harry A. T. H., Wong E., Greg W. R. (2015). Serpulidae (Annelida) of lizard island, great barrier reef, Australia. Zootaxa 4019, 275–353. doi: 10.11646/zootaxa.4019.1.16
Landoulsi J., Cooksey K. E., Dupres V. (2011). Review - Interactions between diatoms and stainless steel: Focus on biofouling and biocorrosion. Biofouling 27, 1105–1124. doi: 10.1080/08927014.2011.629043
Lappin-Scott H. M., Bass C. (2001). Biofilm formation: Attachment, growth, and detachment of microbes from surfaces. Am. J. Infect. Control. 29, 250–251. doi: 10.1067/mic.2001.115674
Lau S. C. K., Qian P. Y. (2001). Larval settlement in the serpulid polychaete Hydroides elegans in response to bacterial films: An investigation of the nature of putative larval settlement cue. Mar. Biol. 138, 321–328. doi: 10.1007/s002270000453
Lee J. W., Nam J. H., Kim Y. H., Lee K. H., Lee D. H. (2008). Bacterial communities in the initial stage of marine biofilm formation on artificial surfaces. J. Microbiol. 46, 174–182. doi: 10.1007/s12275-008-0032-3
Lee W. J., Shin K., Jang P.-G., Jang M.-C., Park N. J. (2005). Summer pattern of phytoplankton distribution at a station in jangmok bay. Ocean. Sci. J. 40, 1–9. doi: 10.1007/bf03022605
Lee H., Yu O., Kim S., Kang J. (2024). Species composition and distribution of hull-fouling macroinvertebrates differ according to the areas of research vessel operation. J. Mar. Sci. Eng. 12, 613. doi: 10.3390/jmse12040613
Leigh J. W., Bryant D. (2015). POPART: Full-feature software for haplotype network construction. Methods Ecol. Evol. 6, 1110–1116. doi: 10.1111/2041-210X.12410
Lema K. A., Constancias F., Rice S. A., Hadfield M. G. (2019). High bacterial diversity in nearshore and oceanic biofilms and their influence on larval settlement by Hydroides elegans (Polychaeta). Environ. Microbiol. 21, 3472–3488. doi: 10.1111/1462-2920.14697
Li C., Meng Y., He C., Chan V. B. S., Yao H., Thiyagarajan V. (2016). Mechanical robustness of the calcareous tubeworm Hydroides elegans: warming mitigates the adverse effects of ocean acidification. Biofouling 32, 191–204. doi: 10.1080/08927014.2015.1129532
Lim C. S., Leong Y. L., Tan K. (2017). Managing the risk of non-indigenous marine species transfer in Singapore using a study of vessel movement. Mar. pollut. Bull. 115, 332–344. doi: 10.1016/j.marpolbul.2016.12.009
Link H., Nishi E., Tanaka K., Bastida-Zavala R., Kupriyanova E. K., Yamakita T. (2009). Hydroides dianthus (Polychaeta: Serpulidae), an alien species introduced into Tokyo Bay, Japan. Mar. Biodivers. Rec. 2, e87. doi: 10.1017/s1755267209000931
Liu T. X., Kinn-Gurzo S., Chan K. Y. K. (2020a). Resilience of invasive tubeworm (Hydroides dirampha) to warming and salinity stress and its implications for biofouling community dynamics. Mar. Biol. 167, 1–10. doi: 10.1007/s00227-020-03758-y
Liu W., Xu M., Zhang J., Zhang T. (2020b). Survival and attachment of biofouling freshwater mussel (Limnoperna fortunei) to environmental conditions: potential implications in its invasion, infection and biofouling control. Limnology 21, 245–255. doi: 10.1007/s10201-020-00607-1
Mak P. M. S. (1982). The coral associated polychaetes of Hong Kong, with special reference to the serpulids. Eds. Morton B., Tseng C. K. (Hong Kong: Hong Kong University Press).
Mak P., Huang Z. (1982). The salinity tolerance of the serpulid polychaete, Hydroides elegans (Haswell 1883), and its possible applications in bio-antifouling In The marine flora and fauna of Hong Kong and southern China. Eds. Morton B., Tseng C. (Hong Kong: Hong Kong University Press).
Marenzeller E. von. (1884). Südjapanische Anneliden. II. Ampharetea, Terebellacea, Sabellacea, Serpulacea. Denkschriften der Akademie der Wissenschaften, Wien. 49 (2), 197–224, 4 plates. Available online at: https://biodiversitylibrary.org/page/7102598
McQuaid K. A., Griffiths C. L. (2014). Alien reef-building polychaete drives long-term changes in invertebrate biomass and diversity in a small, urban estuary. Estuar. Coast. Shelf. Sci. 138, 101–106. doi: 10.1016/j.ecss.2013.12.016
Meng Y., Guo Z., Fitzer S. C., Upadhyay A., Chan V. B. S., Li C., et al. (2018). Ocean acidification reduces hardness and stiffness of the Portuguese oyster shell with impaired microstructure: A hierarchical analysis. Biogeosciences 15, 6833–6846. doi: 10.5194/bg-15-6833-2018
Meng Y., Li C., Li H., Shih K., He C., Yao H., et al. (2019). Recoverable impacts of ocean acidification on the tubeworm, Hydroides elegans: implication for biofouling in future coastal oceans. Biofouling 35, 945–957. doi: 10.1080/08927014.2019.1673376
Mieszkin S., Callow M. E., Callow J. A. (2013). Interactions between microbial biofilms and marine fouling algae: A mini review. Biofouling 29, 1097–1113. doi: 10.1080/08927014.2013.828712
Miller L. P., Harley C. D. G., Denny M. W. (2009). The role of temperature and desiccation stress in limiting the local-scale distribution of the owl limpet, lottia gigantea. Funct. Ecol. 23, 756–767. doi: 10.1111/j.1365-2435.2009.01567.x
Montefalcone M., Morri C., Parravicini V., Bianchi C. N. (2015). A tale of two invaders: divergent spreading kinetics of the alien green algae Caulerpa taxifolia and Caulerpa cylindracea. Biol. Invasions. 17, 2717–2728. doi: 10.1007/s10530-015-0908-1
Nagaraj V., Skillman L., Li D., Ho G. (2018). Review – Bacteria and their extracellular polymeric substances causing biofouling on seawater reverse osmosis desalination membranes. J. Environ. Manage. 223, 586–599. doi: 10.1016/j.jenvman.2018.05.088
Nedved B., Hadfield M. (2009). Hydroides elegans (Annelida: Polychaeta): a model for biofouling research. Mar. Ind. Biofouling. Springer. Ser. Biofilms. 4, 203–207. doi: 10.1007/7142
Norén M., Jondelius U. (1999). Phylogeny of the Prolecithophora (Platyhelminthes) inferred from 18S rDNA sequences. Cladistics 15, 103–112. doi: 10.1111/j.1096-0031.1999.tb00252.x
Paik E. I. (1989). Illustrated encyclopedia of fauna & flora of Korea Vol. 31 Polychaeta. (SEOUL), 764.
Park J. K., Hoe C. H., Kim H. P., Cho Y. K. (2022). Study on the biofouling management of international ships entering South Korea. J. Korean. Soc Mar. Environ. Saf. 28, 10–18. doi: 10.7837/kosomes.2022.28.1.010
Park C., Kim S. T., Hong J. S., Choi K. H. (2017). A rapid assessment survey of invasive species of macrobenthic invertebrates in Korean waters. Ocean. Sci. J. 52, 387–395. doi: 10.1007/s12601-017-0024-5
Park J., Kim T., Lawan B., Jang M., Ki S. (2023). Ship hull − Fouling diatoms on korean research vessels revealed by morphological and molecular methods, and their environmental implications. J. Microbiol. 61, 615–626. doi: 10.1007/s12275-023-00055-3
Park S., Seo J., Choi J. (2011). Community structure of sessile organismas on PVC plates according to different submerged timings and durations in Jangmok Bay, Korea. Korean. J. Malacol. 27, 99–105. doi: 10.9710/kjm.2011.27.2.099
Paxton H., Chou L. M. (2000). Polychaetous annelids from the south China sea. Raffles. Bull. Zool. 48, 209–232.
Pettengill J. B., Wendt D. E., Schug M. D., Hadfield M. G. (2007). Biofouling likely serves as a major mode of dispersal for the polychaete tubeworm Hydroides elegans as inferred from microsatellite loci. Biofouling 23, 161–169. doi: 10.1080/08927010701218952
Piola R. F., Johnston E. L. (2006). Differential resistance to extended copper exposure in four introduced bryozoans. Mar. Ecol. Prog. Ser. 311, 103–114. doi: 10.3354/meps311103
Qiu P. Y., Qian J. W. (1997). Combined effects of salinity, temperature and food on early development of the polychaete Hydroides elegans. Marine Ecology Progress Series, 152(1-3): 79-88, 5 figures, 3 tables. Mar. Ecol. Prog. Ser. 152, 79–88. doi: 10.3354/meps152079
Raghunathan C., Gopi K. C., Chandra K. (2019). Invasive alien faunal species in India. J. Gov. 18, 124–140.
Ralston E. A., Swain G. W. (2014). The ghost of fouling communities past: The effect of original community on subsequent recruitment. Biofouling 30, 459–471. doi: 10.1080/08927014.2014.894984
Ramsey M. M., Whiteley M. (2004). Pseudomonas aeruginosa attachment and biofilm development in dynamic environments. Mol. Microbiol. 53, 1075–1087. doi: 10.1111/j.1365-2958.2004.04181.x
Read G. B., ten Hove H. A., Sun Y., Kupriyanova E. K. (2017). Hydroides gunnerus 1768 (Annelida, Serpulidae) is feminine: A nomenclatural checklist of updated names. Zookeys 2017, 1–52. doi: 10.3897/zookeys.642.10443
Ronquist F., Teslenko M., van der Mark P., Ayres D. L., Darling A., Höhna S., et al. (2012). Mrbayes 3.2: Efficient bayesian phylogenetic inference and model choice across a large model space. Syst. Biol. 61, 539–542. doi: 10.1093/sysbio/sys029
Sáez Álvarez P. (2021). From maritime salvage to IMO 2020 strategy: Two actions to protect the environment. Mar. pollut. Bull. 170, 112590. doi: 10.1016/j.marpolbul.2021.112590
Sarà G., Lo Martire M., Buffa G., Mannino A. M., Badalamenti F. (2007). The fouling community as an indicator of fish farming impact in Mediterranean. Aquac. Res. 38, 66–75. doi: 10.1111/j.1365-2109.2006.01632.x
Schultz M. P. (2007). Effects of coating roughness and biofouling on ship resistance and powering. Biofouling 23, 331–341. doi: 10.1080/08927010701461974
Schwan I. D. S., Brasil A. C. D. S., Neves D., Dias G. M. (2016). The invasive worm Hydroides elegans (Polychaeta – Serpulidae) in southeastern Brazil and its potential to dominate hard substrata. Mar. Biol. Res. 12, 96–103. doi: 10.1080/17451000.2015.1080370
Schwindt E., Iribarne O. O., Isla F. I. (2004). Physical effects of an invading reef-building polychaete on an Argentinean estuarine environment. Estuar. Coast. Shelf. Sci. 59, 109–120. doi: 10.1016/j.ecss.2003.06.004
Seebens H., Gastner M. T., Blasius B. (2013). The risk of marine bioinvasion caused by global shipping. Ecol. Lett. 16, 782–790. doi: 10.1111/ele.12111
Shikuma N. J., Antoshechkin I., Medeiros J. M., Pilhofer M., Newman D. K. (2016). Stepwise metamorphosis of the tubeworm Hydroides elegans is mediated by a bacterial inducer and MAPK signaling. Proc. Natl. Acad. Sci. U. S. A. 113, 10097–10102. doi: 10.1073/pnas.1603142113
Shikuma N. J., Hadfield M. G. (2005). Temporal variation of an initial marine biofilm community and its effects on larval settlement and metamorphosis of the tubeworm Hydroides elegans. Biofilms 2, 231–238. doi: 10.1017/S1479050506002018
Shin K., Jang P. G., Jang M. C., Lee W. J. (2010). Effects of nutrient property changes on summer phytoplankton community structure of jangmok bay. Ocean. Polar. Res. 32, 97–111. doi: 10.4217/OPR.2010.32.2.097
Simberloff D. (2014). Biological invasions: what’s worth fighting and what can be won? Ecol. Eng. 65, 112–121. doi: 10.1016/j.ecoleng.2013.08.004
Simberloff D. (2021). Maintenance management and eradication of established aquatic invaders. Hydrobiologia 848, 2399–2420. doi: 10.1007/s10750-020-04352-5
Simberloff D., Rejmánek M. (2011). Encyclopedia of Biological Invasions (California: University of California Press). doi: 10.1525/california/9780520264212.001.0001
Stamatakis A. (2014). RAxML version 8: A tool for phylogenetic analysis and post-analysis of large phylogenies. Bioinformatics 30, 1312–1313. doi: 10.1093/bioinformatics/btu033
Sun Y., Kupriyanova E. K., Qiu J. W. (2012). COI barcoding of Hydroides: A road from impossible to difficult. Invertebr. Syst. 26, 539–547. doi: 10.1071/IS12024
Sun Y., Wong E., Keppel E., Williamson J. E., Kupriyanova E. K. (2017). A global invader or a complex of regionally distributed species? Clarifying the status of an invasive calcareous tubeworm Hydroides dianthus (Verrill 1873) (Polychaeta: Serpulidae) using DNA barcoding. Mar. Biol. 164, 1–12. doi: 10.1007/s00227-016-3058-9
Sun Y., Wong E., Ten Hove H. A., Hutchings P. A., Williamson J. E., Kupriyanova E. K. (2015). Revision of the genus Hydroides (Annelida: Serpulidae) from Australia. Zootaxa. 4009, 1–99. doi: 10.11646/zootaxa.4009.1.1
Sun Y., Wong E., Tovar-Hernández M. A., Williamson J. E., Kupriyanova E. K. (2016). Is Hydroides brachyacantha (Serpulidae: Annelida) a widespread species? Invertebr. Syst. 30, 41–59. doi: 10.1071/IS15015
Tanur A. E., Gunari N., Sullan R. M. A., Kavanagh C. J., Walker G. C. (2010). Insights into the composition, morphology, and formation of the calcareous shell of the serpulid Hydroides dianthus. J. Struct. Biol. 169, 145–160. doi: 10.1016/j.jsb.2009.09.008
ten Hove H. (1974). Notes on Hydroides elegans (Haswell 1883) and Mercierella enigmatica Fauvel 1923, alien serpulid polychaetes introduced into the Netherlands. Bull. Xool. Mus. Univ. Amster- dam 4, 45–51.
Toonen R., Pawlik J. R. (2001). Settlement of the gregarious tube worm Hydroides dianthus (Polychaete: Serpulidae). II. Testing the desperate larva hypothesis. Mar. Ecol. Prog. Ser. 224, 115–131. doi: 10.3354/meps224115
Tovar-Hernández M. A., Méndez N., Villalobos-Guerrero T. F. (2009). Fouling polychaete worms from the southern Gulf of California: Sabellidae and Serpulidae. Syst. Biodivers. 7, 319–336. doi: 10.1017/S1477200009990041
Tovar-Hernández M. A., Villalobos-Guerrero T. F., Kupriyanova E. K., Sun Y. (2016). A new fouling Hydroides (Annelida, Sabellida, Serpulidae) from southern Gulf of California. J. Mar. Biol. Assoc. United. Kingdom. 96, 693–705. doi: 10.1017/S0025315415000764
Unabia C. R. C., Had M. G. (1999). Role of bacteria in larval settlement and metamorphosis of the polychaete Hydroides elegans. Mar. Biol. 133, 55–64. doi: 10.1007/s002270050442
Vijayan N., Lema K. A., Nedved B. T., Hadfield M. G. (2019). Microbiomes of the polychaete Hydroides elegans (Polychaeta: Serpulidae) across its life-history stages. Mar. Biol. 166, 1–13. doi: 10.1007/s00227-019-3465-9
Vinn O., Kirsimäe K., ten Hove H. A. (2009). Pomatoceros americanus’e (Polychaeta, Serpulidae) koja peenstruktuur ja selle tähendus serpuliidide koja moodustumise mõistmisel. Est. J. Earth Sci. 58, 148–152. doi: 10.3176/earth.2009.2.05
Vinn O., Kupriyanova E. K. (2011). Evolution of a dense outer protective tube layer in serpulids (Polychaeta, Annelida). Carnets. Géol. (Notebooks. Geol. 05, 137–147. doi: 10.4267/2042/43896
Vinn O., Ten Hove H. A., Mutvei H., Kirsimäe K. (2008). Ultrastructure and mineral composition of serpulid tubes (Polychaeta, Annelida). Zool. J. Linn. Soc 154, 633–650. doi: 10.1111/j.1096-3642.2008.00421.x
Walters L. J., Hadfield M. G., Carmen K. A. (1997). The importance of larval choice and hydrodynamics in creating aggregations of hydroides elegans (Polychaeta: Serpulidae). Invertebr. Biol. 102–114. doi: 10.2307/3226974
Wells F. E., Tan K. S., Todd P. A., Jaafar Z., Yeo D. C. J. (2019). A low number of introduced marine species in the tropics: A case study from Singapore. Manage. Biol. Invasions. 10, 23–45. doi: 10.3391/MBI.2019.10.1.03
Xiong W., Shen C., Wu Z., Lu H., Yan Y. (2017). A brief overview of known introductions of non-native marine and coastal species into China. Aquat. Invasions. 12, 109–115. doi: 10.3391/ai.2017.12.1.11
Xu Y., Li H., Li X., Xiao X., Qian P. Y. (2009). Inhibitory effects of a branched-chain fatty acid on larval settlement of the polychaete hydroides elegans. Mar. Biotechnol. 11, 495–504. doi: 10.1007/s10126-008-9161-2
Yang D., Sun R. (1988). Polychaetous annelids commonly seen from the Chinese waters (Beijing: Agriculture Publishing).
Yee A., Mackie J., Pernet B. (2019). The distribution and unexpected genetic diversity of the non-indigenous annelid ficopomatus enigmaticus in California. Aquat. Invasions. 14, 250–266. doi: 10.3391/ai.2019.14.2.06
Zardus J. D., Nedved B. T., Huang Y., Tran C., Hadfield M. G. (2008). Microbial biofilms facilitate adhesion in biofouling invertebrates. Biol. Bull. 214, 91–98. doi: 10.2307/25066663
Zeriouh O., Reinoso-Moreno J. V., López-Rosales L., Cerón-García M., del C., Sánchez-Mirón A., et al. (2017). Biofouling in photobioreactors for marine microalgae. Crit. Rev. Biotechnol. 37, 1006–1023. doi: 10.1080/07388551.2017.1299681
Zhang Y., Sun J., Xiao K., Arellano S. M., Thiyagarajan V., Qian P. Y. (2010). 2D gel-based multiplexed proteomic analysis during larval development and metamorphosis of the biofouling polychaete tubeworm hydroides elegans. J. Proteome Res. 9, 4851–4860. doi: 10.1021/pr100645z
Zhang Y., Sun J., Zhang H., Chandramouli K. H., Xu Y., He L. S., et al. (2014). Proteomic profiling during the pre-competent to competent transition of the biofouling polychaete Hydroides elegans. Biofouling 30, 921–928. doi: 10.1080/08927014.2014.951341
Keywords: biofouling, tube worm, non-indigenous species, biodiversity, ecological
Citation: Kim SL and Yu OH (2025) Invasion and ecological impact of the biofouling tube worm Hydroides elegans (Polychaeta: Serpulidae) in Korean coastal waters. Front. Mar. Sci. 11:1416546. doi: 10.3389/fmars.2024.1416546
Received: 12 April 2024; Accepted: 25 November 2024;
Published: 07 January 2025.
Edited by:
Bernardo Antonio Perez Da Gama, Fluminense Federal University, BrazilReviewed by:
Joachim Langeneck, Consorzio Nazionale Interuniversitario per le Scienze del Mare (CoNISMa), ItalyCopyright © 2025 Kim and Yu. This is an open-access article distributed under the terms of the Creative Commons Attribution License (CC BY). The use, distribution or reproduction in other forums is permitted, provided the original author(s) and the copyright owner(s) are credited and that the original publication in this journal is cited, in accordance with accepted academic practice. No use, distribution or reproduction is permitted which does not comply with these terms.
*Correspondence: Ok Hwan Yu, b2h5dUBraW9zdC5hYy5rcg==
Disclaimer: All claims expressed in this article are solely those of the authors and do not necessarily represent those of their affiliated organizations, or those of the publisher, the editors and the reviewers. Any product that may be evaluated in this article or claim that may be made by its manufacturer is not guaranteed or endorsed by the publisher.
Research integrity at Frontiers
Learn more about the work of our research integrity team to safeguard the quality of each article we publish.