- 1Phycology Research Group, Department of Science, Ghent University, Ghent, Belgium
- 2ANEMOON Foundation, Bennebroek, Netherlands
- 3Meise Botanic Garden, Meise, Belgium
Using a DNA barcoding approach, we document an extensive number of inter-species cryptic introductions of bladed Bangiales (Pyropia) at an historic oyster aquaculture site in the Southern North Sea. We sampled the intertidal of 20 locations along the Belgian and Dutch coastline, ranging from sheltered to exposed, between February 2022 and April 2023 for bladed Bangiales. 230 individuals of bladed Bangiales were collected, morphological and ecological characteristics described and identified based on chloroplast rbcL gene sequences, revealing the presence of 13 species belonging to the genera Porphyra and Pyropia. While seven species (P. dioica, P. linearis, P. purpurea, P. umbilicalis, Py. collinsii, Py. elongata, Py. leucosticta) are regarded as native to the Northeast Atlantic, four species (Py. katadae, Py. koreana, Py. kinositae and Py. yezoensis) are considered non-indigenous. The records of Py. katadae, Py. kinositae and Py. koreana are the first confirmed observations for the Northeast Atlantic region. In addition, we identified two distinct rbcL clades, for which a definite species identification could not be established due to a lack of matching reference sequences. Species diversity was generally higher during winter and at exposed sites facing the open North Sea. The surprisingly high species diversity of the bladed Bangiales could only be revealed by molecular identification – a crucial tool for reliable species identification in this group of organisms.
1 Introduction
The proliferation of non-indigenous species can have long-lasting impacts on native communities and ecosystems by altering native species’ occurrence, interactions and compositions, which can result in disrupted ecosystem services and functions (Pejchar and Mooney, 2009; Simberloff et al., 2013; Buckley and Catford, 2016). Especially difficult to detect are cryptic invasions, including intraspecific (invasion of non-indigenous species lineage into genetically distinct population ranges) and interspecific cryptic invasions (unnoticed introduction and spread of non-indigenous species due to similar morphology within a native species range and therefore misidentification) (Morais and Reichard, 2018). Cryptic invasions go often undetected for extended times and may result in rapid range expansion of non-indigenous species (Morais and Reichard, 2018).
Introductions of non-indigenous species in the marine environment are common, mostly facilitated by direct or indirect human-mediated transport via shipping (e.g. fouling organisms settling on vessel hulls, release of ballast water), translocation and introduction of aquaculture related species including associated biota, removal of physical barriers (e.g. construction of the Suez Canal connecting the Mediterranean and the Red Sea) or the release of ornamental species (Ruiz et al., 1997; Nunes et al., 2014; Castro et al., 2017). Consequently, harbours and aquaculture sites are often hot spots of marine non-indigenous species (Soares et al., 2022). Throughout Europe, at least 317 species of animals, plants and algae have been introduced into marine and freshwater systems for aquaculture and stocking purposes, resulting in an additional 265 non-targeted species introductions (Olenin et al., 2008). Along the European Atlantic and North Sea coast, frequent introductions of the Pacific cupped oyster ‘Crassostrea gigas’ and Manila clam ‘Ruditapes philippinarum’ are responsible for the majority of reported introduction of non-indigenous invertebrate and macroalgae species (Savini et al., 2010; Lallias et al., 2015; Anglès d’Auriac et al., 2017). Often, these introductions occurred accidentally via attachment to packaging materials or the shells (Savini et al., 2010). Up to date, 140 non-indigenous algal species have been confirmed along the European coastlines, with five species (Sargassum muticum, Bonnemaisonia hamifera, Asparagopsis armata, Caulerpa cylindracea and Colpomenia peregrina) most frequently reported (van der Loos et al., 2023). In many cases however, cryptic invasions go undetected due to taxonomically unresolved species complexes or low morphological distinctiveness between indigenous and non-indigenous species (Díaz-Tapia et al., 2020a). Therefore, the detection of cryptic invasions relies on molecular assessments. Archived specimens, especially from historical collections, as well as phylogeographic studies, in case of the lack of historical records, can be crucial in deciphering cryptic invasions (Morais and Reichard, 2018).
A notable species complex characterized with few distinctive morphological traits and a great number of cryptic species are red macroalgae of the order Bangiales including foliose or bladed forms of the genera Porphyra and Pyropia (Sutherland et al., 2011; Kucera and Saunders, 2012). Bladed Bangiales are a diverse and ancient order, found globally in intertidal and subtidal habitats, and include some of the most economically important and valuable macroalgal species (Sahoo et al., 2002; Blouin et al., 2011; Yang et al., 2017). Traditionally, the order Bangiales consisted of only one family Bangiaceae, including two genera – Bangia Lynbg. (filamentous habitus) and Porphyra C. Agardh (leafy thallus habitus in gametophyte stage). The development, increased availability and cost effectiveness of molecular methods allowed for detailed regional taxonomic studies revealing high cryptic diversity with few morphological characteristics but distinctive geographic species distributions (Stiller and Waaland, 1996; Nelson et al., 2001; Lindstrom et al., 2015; Guillemin et al., 2016; Meynard et al., 2019; Reddy et al., 2020). As a result, the order has undergone several taxonomic revisions and new species are frequently described. Sutherland et al. (2011) divided the order into 15 genera, consisting of seven filamentous (Bangia, ‘Bangia’ 1, ‘Bangia’ 2, ‘Bangia 3’, Dione, Minerva, Pseudobangia) and eight foliose genera (Boreophyllum, Clymene, Fuscifolium, Lysithea, Miuraea, Porphyra, Pyropia, Wildemania). Sánchez et al. (2014) added another genus Neothemis to the Bangiales. Yang et al. (2020) redefined the genus Pyropia and proposed to split it into Calidia, Neoporphyra, Neopyropia and Uedaea, although this latest taxonomic revision is currently under discussion (Zuccarello et al., 2022).
Bladed Bangiales display a simple morphology. Foliose thalli typically arise from a small, sometimes discoid holdfast, and consist of only one or a few cell layers with generally low morphological complexity (Brodie and Irvine, 2003; Sutherland et al., 2011). While the morphology is simple, life histories are complex, involving alternation or cycling between the foliose macroscopic blade phase (gametophyte) and the microscopic filamentous conchocelis (sporophyte) phase (Drew, 1949), with further aspects concerning ploidy and a third life history stage (the conchosporophyte) still being actively investigated. While the bladed gametophyte usually grows attached to a hard substrate or epiphytically on other seaweeds, the filamentous conchocelis stage can burrow into calcium shells, e.g. of bivalves or other molluscs, most likely to escape grazing pressure (Lubchenco and Cubit, 1980). However, the conchocelis stage is not dependent on a shell substrate for germination and development (Knoop et al., 2020). Given its microscopic filamentous appearance, the sporophyte is hard to detect and its association with oyster shells facilitates accidental transportation alongside historic oyster transfers, mainly originating in the Pacific towards other marine environments.
The frequent introduction of C. gigas for aquaculture purposes to France, Belgium and the Netherlands from the Pacific started in the 1960s (Miossec et al., 2009; Martínez-García et al., 2022). Since then, C. gigas established non-indigenous populations throughout the North Atlantic and North Sea, with a range of associated negative and positive effects on local ecosystems (Martínez-García et al., 2022). Furthermore, the extensive transfers of Pacific C. gigas into European waters, have been linked to the introduction of several pacific macroalgae species into the North Atlantic and Mediterranean (e.g. Grateloupia subpectiniata and G. turuturu, Gelidium vagum, Gracilaria vermiculophylla, Saccharina japonica, Undaria pinnatifida) including the introduction of Pyropia suborbiculata to the Spanish Atlantic coast (Mineur et al., 2014). Given the lack of diagnostic morphological characteristics between bladed Bangiales species, it is likely that additional and unnoticed introductions of bladed Bangiales of Pacific origin into the North Atlantic occurred.
DNA-assisted taxonomic studies confirming the species identity of bladed Bangiales species along the Southern North Sea coast of Belgium and the Netherlands are lacking, where currently five bladed Bangiales (P. dioica, P. linearis, P. purpurea, P. umbilicalis and Pyropia leucosticta) are known to commonly occur (Stegenga and Mol, 1983; Coppejans, 1998; van der Loos et al., 2021). The northern Atlantic and southern North Sea are hot spots of non-indigenous seaweed species (Maggs and Stegenga, 1998; Stegenga and Karremans, 2015), especially in locations with a long history of extensive shellfish cultivation, in particular of C. gigas. Examples are the semi-closed deltas of the Netherlands, such as the Eastern Scheldt, or artificial inshore waters in Belgium, such as the Spuikom, where further non-indigenous and possibly cryptic species introductions have likely occurred.
Therefore, this study aims to give a detailed and updated overview of the distribution and abundance of bladed Bangiales along the Belgian and Dutch intertidal North Sea coast confirmed by molecular identification. Sample locations ranged from sheltered to exposed sites to account for a wide variety of habitats and included historic aquaculture sites and hot spots of occurring non-indigenous species to detect the presence of cryptic non-indigenous species.
2 Materials and methods
2.1 Sampling design
Bladed Bangiales were collected from in total 20 sampling locations (Table 1) along the Belgian and Dutch North Sea coast (Figure 1). Samples were collected in winter (20/– 23/02/2022) and summer (12/– 14/07/2022) from nine sampling locations. Two sampling sites were located in Belgium in Ostend along the breakwater and harbour wall (Figure 1B) and seven sites in the Netherlands (Figure 1C) along the Eastern Scheldt, covering different habitats from sheltered (Yerseke, Wemeldinge harbour, Wemeldinge stairs, Goes, Kats, Neeltje Jans pontoon) to exposed (Neeltje Jans tidal pool) conditions, rocky, artificial and sand dominated habitats. Each sampling site was thoroughly investigated for bladed Bangiales from the high tide line towards the low tide line around the date of spring tides. Samples were taken from all distinctive bladed Bangiales patches or individuals detected, targeting as many different habitats (e.g. high versus mid or low intertidal, growing in rock pools and different substrates or epiphytically) and morphological differences (considering e.g. colour, shape, size and thickness) as possible. Pictures of the sample habitat were taken, location within the intertidal and substrate noted, before representative individuals – in case of dense patches – or distinctive individuals were collected and transported back to the laboratory for further investigation and processing.
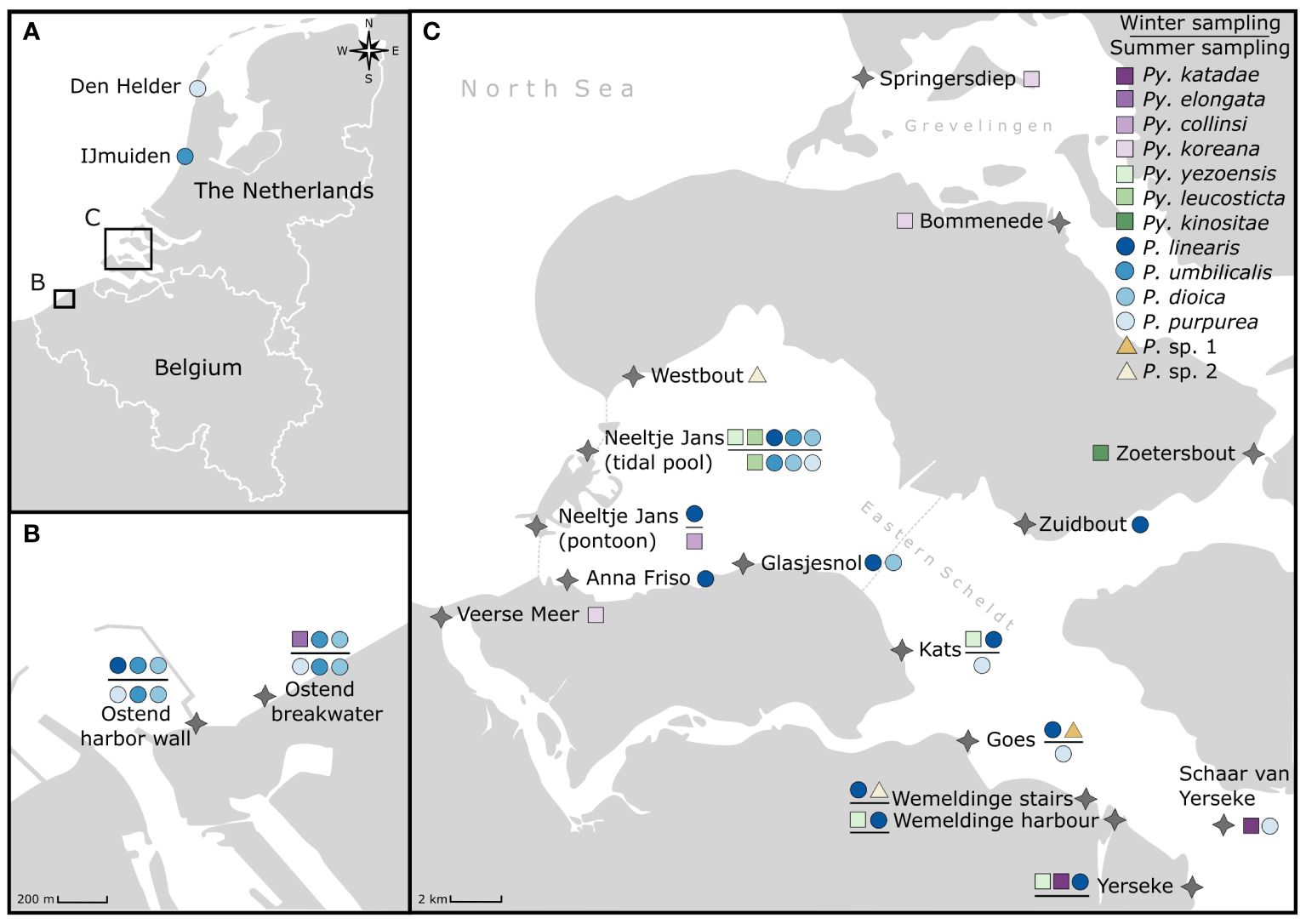
Figure 1. Overview of the study area in the Southern North Sea (A) and more detailed overview of sampling locations in Belgium (B) and the Netherlands (C). Identified species found per location are displayed, differentiating between the winter sampling (top of black line) and summer sampling (bottom of black line). Locations without a black line were only sampled once between the winter and summer sampling.
Besides these nine locations that were thoroughly investigated and resampled in winter and summer 2022, further samples were analysed from eleven additional sampling locations in the Netherlands (Anna Friso, Bommenede, Den Helder, Glasjesnol, IJmuiden, Schaar van Yerseke, Springersdiep, Veerse Meer, Westbout, Zoetersbout and Zuidbout) where distinct bladed Bangiales were observed.
In the laboratory, samples consisting of several individuals collected from the same patch were sorted into different morphotypes (if applicable) that possibly could represent different species, based on previous knowledge and literature considering shape, size, colouration, thickness and distribution of reproductive cells (Coppejans, 1998; Brodie and Irvine, 2003; van der Loos et al., 2021). In case of different morphotypes, one representative per morphotype was chosen, and analysed further for morphological characteristics (length, width, colour, thallus shape, presence and distribution of reproductive tissue and cell size). Vegetative and, where possible, reproductive tissue areas were investigated microscopically (40x objective, Observer A1, Zeiss, Germany) and pictures taken to measure maximum cell diameter of 10 representative vegetative and reproductive (spermatogonia, zygotosporangia/neutral sporangia) cells. A ~1 cm2 tissue sample was taken, rinsed in fresh water and dried in silica gel for genetic analysis (see 3.2). Pictures were taken of all individuals, before samples were preserved as herbarium specimens. Herbarium vouchers from specimens collected in Belgium were deposited in the Meise Botanic Garden herbarium, Belgium (BR). Specimens collected in the Netherlands were deposited at the herbarium of Naturalis Biodiversity Centre, the Netherlands (L). A full list of individuals, sampling date, location and habitat, voucher numbers and GenBank accession numbers is presented in the Supplementary Material S1.
2.2 DNA extraction and PCR
Total DNA was extracted from silica dried samples using a CTAB method described by Doyle and Doyle (1987). In short, samples were ground with liquid nitrogen before DNA was extracted in 500 µL cetyl-trimethyl-ammonium-bromide (CTAB) and Proteinase-K (20 mg/mL) followed by two cleaning cycles with 24:1 chloroform:isoamyl alcohol and final washing in 70% ethanol before extracted DNA was dissolved in 50 µL 1x TE buffer. The gene encoding large subunit of ribulose-1,5-bisphosphate carboxylase/oxgenase (rbcL) was amplified in two segments – rbcL 1 and rbcL 2 using respectively the forward primers F8 (5’-GGT GAA TTC CAT ACG CTA AAA TG-3’) and F481 (5’-GTA GAA CGT GAG CGT ATG GA-3’) and the reverse primers R646 (5’-AAT CTT TCT TTC CAT CGC AT -3’) and R1381 (5’-ATC TTT CCA TAA ATC TAA AGC-3’) (Wang et al., 2000). PCR mixtures consisted of 2.5 µL PCR buffer, 2.5 µL dNTP’s (200 µM), 1.25 µL forward primer (10 µM), 1.25 µL reverse primers (10 µL), 1 µL BSA (10 µg µL-1), 1.25 µL Taq (1U µL-1) and 1 µL sample template. PCRs were run by denaturation for 5 min at 94°C followed by 35 cycles (1 min denaturation at 94°C, 1 min annealing at 46°C, 2 min elongation at 75°C) and a final elongation for 5 min at 72°C on an Applied Biosystems Veriti 96 Well Thermal Cycler. All PCR products were verified by gel electrophoresis before being sequenced by Macrogen (Amsterdam, NL). Chemicals were sourced from Sigma, VWR and Chemlab. Sequences were edited and the rbcL 1 and rbcL 2 fragments combined using BioNumerics7 (Applied Maths NV, Sint-Martens-Latem, Belgium). The sequences from this study were aligned with sequences downloaded from GenBank. In total, 48 reference sequences were included. The full overview of reference genes including GenBank accession numbers are provided in the Supplementary Material S2.
2.3 Phylogenetic analysis
The sequences were aligned with MAFFT v7.154b (Katoh et al., 2002) and trimmed with trimAl v1.2rev59 (using gappyout settings) (Capella-Gutiérrez et al., 2009). The phylogenetic analysis was performed using IQ-TREE v1.6.12 with 1000 ultrafast bootstrap iterations (Nguyen et al., 2015). The phylogenetic tree was mid-point rooted, visualised in FigTree 1.4.4 (http://tree.bio.ed.ac.uk/software/figtree) and further edited using Inkscape.
Species names used in the phylogenetic tree were retrieved from AlgaeBase (Guiry and Guiry, 2024), following the taxonomy of Sutherland et al. (2011). The full phylogenetic tree including all sequences generated in this study is available in the Supplementary Material S3. For visual purposes, one sequence per species per location was included in the visualised tree.
All sequences, together with herbarium scans and further information, were deposited in BOLD (Ratnasingham and Hebert, 2007, https://boldsystems.org/) and can be accessed under Project ID ‘PORNB - Porphyra diversity of the Netherlands and Belgium’.
3 Results
3.1 Species diversity
In total, we sampled, characterised and identified 230 individuals from the different sampling locations and sampling dates (Supplementary Material Table S1 for complete sample list, GenBank accession numbers and further information). 112 samples were analysed during the winter campaign and 97 samples during the summer campaign, while further 21 samples were collected and analysed from the additional sampling points between March 2022 and April 2023. Based on rbcL sequences (1195 bp) we identified thirteen different bladed Bangiales species clustering within the two genera Porphyra (P. dioica, P. linearis, P. purpurea, P. umbilicalis) and Pyropia (Py. collinsii, Py. elongata, Py. katadae, Py. kinositae, Py. koreana, Py. leucosticta, Py. yezoensis) (Figures 1, 2). Four sequences were unidentified as they did not match any sequences found on GenBank (Figure 2). The unknown sequences formed a clade separated into two species within the genus Porphyra (‘Porphyra sp. 1’; ‘Porphyra sp. 2’). Most of the detected Pyropia samples were collected in the Netherlands, except for one small individual of Py. elongata (Figure 2) that was collected growing epiphytically on a mussel shell at the Ostend breakwater sampling location. Pyropia species were mainly observed in the inshore waters in the Netherlands (Eastern Scheldt and Grevelingen, Figure 1). Py. yezoensis and Py. katadae were abundant at Yerseke, where they frequently occurred on and around C. gigas oyster beds. In total, 10 specimens of Py. yezoensis and 22 of Py. katadae were collected. Py. yezoensis was further observed at three other sampling locations (Kats, Neeltje Jans tidal pool, Wemeldinge harbour) in the Eastern Scheldt. Both species were only observed during the winter sampling campaign and were absent from the sampling locations during summer. While we also detected Py. collinsii, Py. elongata and Py. kinositae, we recorded and sequenced only one specimen per species. Four specimen of Py. koreana were observed, although at very different sampling locations (Springersdiep, Bommenede and Veerse Meer) within the study area (Figure 1).
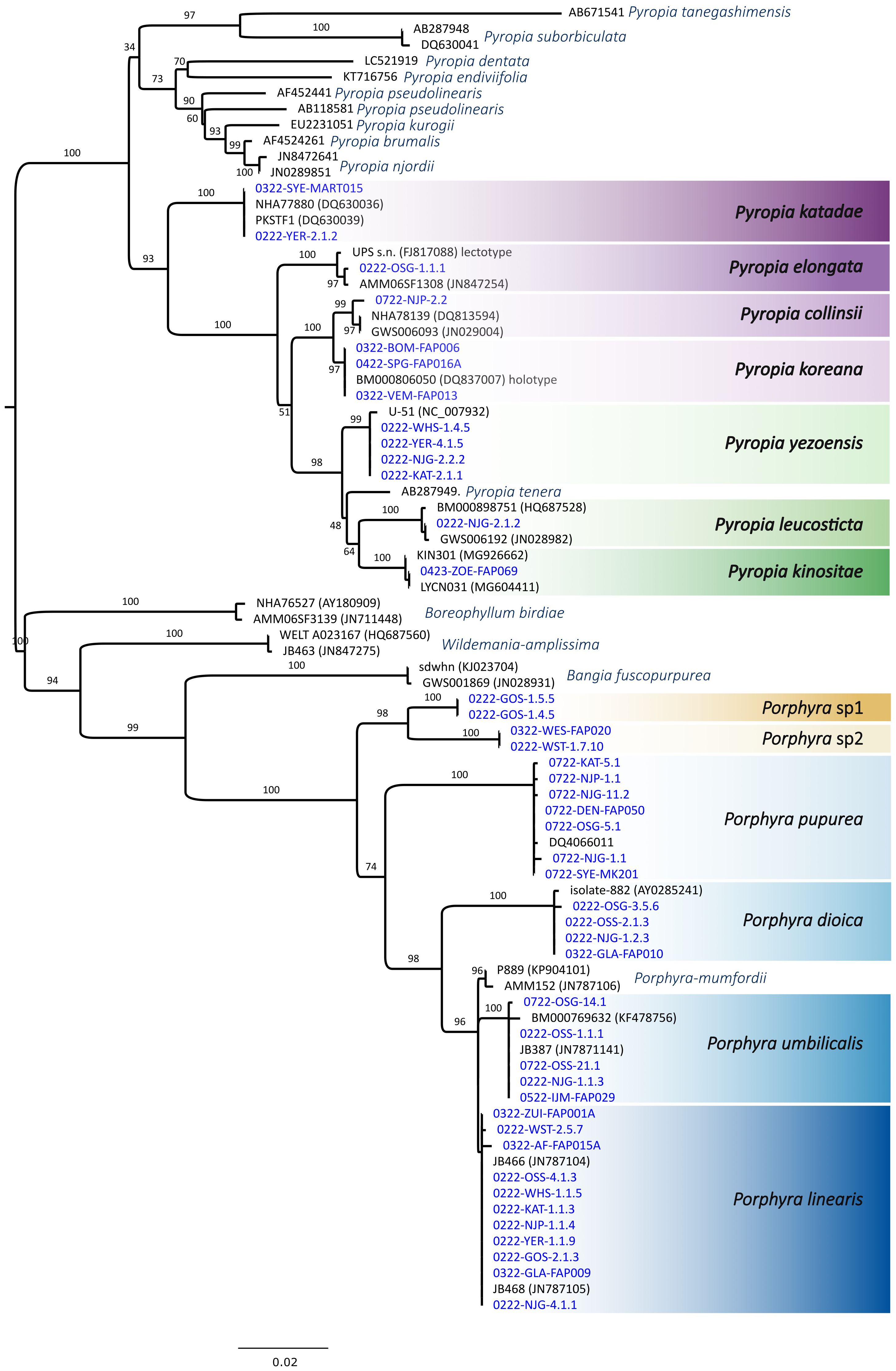
Figure 2. Maximum likelihood (IQ-TREE) phylogenetic tree based on rbcL gene sequences, including one sequence per location and species as well as representative sequences from GenBank. Sequences from this study are written in blue while sequences retrieved from GenBank are indicated in black. Branch support values are UFBoot2 supports (1000 replicates). Branch lengths are scaled according to the number of amino acid substitutions per site.
Observed species richness of detected bladed Bangiales per sampling location decreased with increasing distance from the North Sea, with the highest species richness observed at Neeltje Jans tidal pool, were six bladed Bangiales species were observed over the two sampling campaigns.
3.2 Seasonality
During the winter sampling, 52% of the samples (58 out of 112 records) were identified as P. linearis, which was widespread throughout the study area and abundant at ten of the 20 sampled sites (Figure 1). In summer, P. linearis was not observed and 64% (62 out of 97 records) of collected samples were identified as P. purpurea, present at seven sampling locations. Only three species (P. dioica, P. umbilicalis and Py. leucosticta) were detected during both, the winter and summer sampling campaigns, although only very small specimens (0.5 cm) of Py. leucosticta were observed at Neeltje Jans tidal pool during winter. The remaining species were either observed in winter (Py. elongata, Py. katadae, Py. yezoensis) or during summer (P. purpurea, Py. collinsii). In summer, no bladed Bangiales could be found at sampling locations situated in the South-Eastern Eastern Scheldt (Wemeldinge, Yerseke) even though plenty records were observed during the winter sampling campaign. In general, the more exposed locations facing the open North Sea (Neeltje Jans and locations in Ostend) harboured a greater number of bladed Bangiales species during summer (P. dioica, P. purpurea, P. umbilicalis), while only P. purpurea was observed during the summer sampling event at locations in the inshore sampling sites of the Eastern Scheldt.
3.3 Habitat
Bladed Bangiales were found growing in diverse intertidal habitats, ranging from artificial breakwaters to mussel and oyster beds and even sandy as well as muddy habitats (Figure 3). While some species displayed no preference for a specific habitat and were observed growing throughout the intertidal (e.g. P. dioica), other species were observed to occupy distinct areas within the intertidal (Table 1). P. linearis for example was only observed during winter in the high intertidal, forming a distinctive belt on rocks, wave breakers and harbour walls along the high tide line. Pyropia spp. specimens were more often observed growing epiphytical on other seaweeds in comparison to Porphyra species. P. umbilicalis could form distinct patches in the high intertidal growing on bare rocks, or artificial substrate or intermixed with filamentous Ulva species. Py. katadae and Py. yezoensis were abundant especially at Yerseke along the established C. gigas oyster reefs but also observed on more sandy parts. Py. leucosticta was only observed in the low intertidal and shallow subtidal. The majority of P. purpurea specimens were collected in the lower intertidal, but a few specimens were also collected in the high intertidal. Both unidentified Porphyra species grew in winter intermixed with P. linearis in the high intertidal.
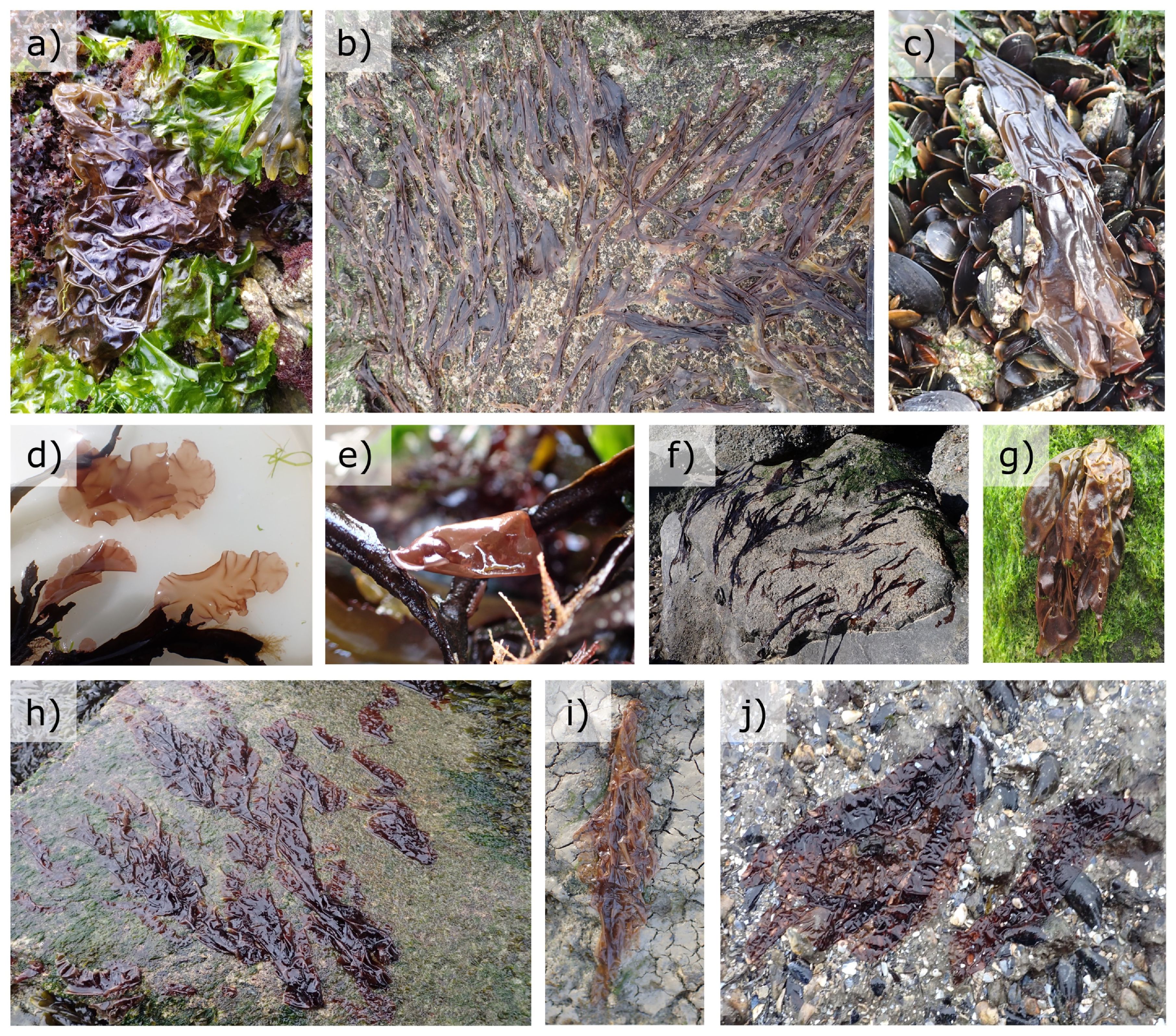
Figure 3. Examples of bladed Bangiales (Porphyra purpurea (A, C), Porphyra linearis (B, H), Pyropia leucosticta (D, E), Porphyra dioica (F), Porphyra umbilicalis (G), Porphyra sp. 2 (I), Pyropia yezoensis (J)) growing on various substrates.
3.4 Morphology
Observed species morphology was generally very diverse between but also within species (Table 2, Figure 4). While some morphological features were commonly observed within a species, morphologically aberrant individuals were always present. As an example, P. linearis usually displayed a linear or lanceolate habitus. However, we also recorded individuals with wide and nearly round thalli that were not assumed to be P. linearis based on morphological characteristics. P. umbilicalis could be identified by the umbilicate shape and often central discoid holdfast. Often, several blades grew in the shape of a rosette, but also individuals with only a single flat blade were observed. When displayed, the rosette and umbilicate growth form of P. umbilicalis was a good distinction setting it apart from P. dioica, P. purpurea and P. linearis.
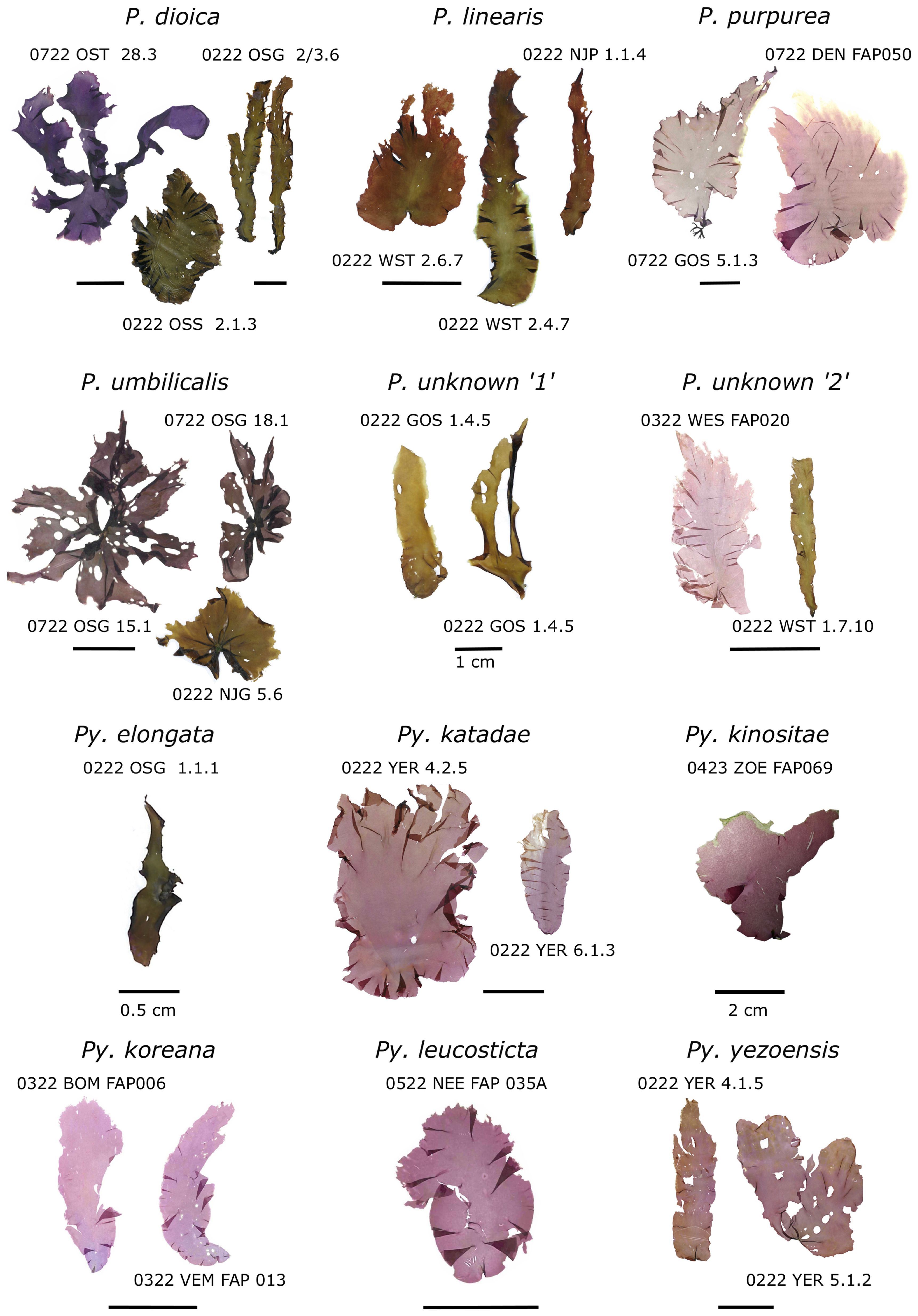
Figure 4. Morphological overview of selected (dried) Porphyra (P.) and Pyropia (Py.) species collected and identified within this study. Scale bars indicate 5 cm, unless indicated otherwise.
Generally, the colour of Pyropia species was more red-brown (e.g. Pyropia yezoensis) to pink, while Porphyra species (e.g. P. dioica, P. purpurea, P. umbilicalis) displayed more olive-green to dark purple-brown colours. However, colour was variable especially between different sampling locations and seasons when e.g. P. dioica turned pale olive-green in summer in comparison to samples collected in winter or P. umbilicalis which was in general dark olive-green to leathery brown in winter and greener in summer (Figure 4). Colouration of thalli changed when dried. While the colour change was sometimes consistent within species, we noticed that colouration of the herbarium preserved specimens was not stable and changed over time.
A distinct characteristic was observed for P. purpurea, which commonly displayed a clear separation of female zygostosporangial and male gametangial tissue areas and often a slightly curved thallus shape towards the tip. Py. katadae also often displayed a clear separation of male gametangial tissue, especially prevalent in samples from the winter campaign, although without the curved thallus as often observed in P. purpurea.
4 Discussion
Cryptic interspecific invasions often go unnoticed and can result in rapid range expansions of non-indigenous species. Through extensive genetic analysis, this study uncovers a remarkable diversity of bladed Bangiales and cryptic interspecific invasion of non-indigenous species at a historic aquaculture site in the Southern North Sea. We reveal the presence of thirteen bladed Bangiales species – four Porphyra, seven Pyropia and two unidentified Porphyra species – in contrast to the commonly assumed five species (P. dioica, P. linearis, P. umbilicalis, P. purpurea and Py. leucosticta) to occur along the Belgian and Dutch coastline (Stegenga et al., 1997; Coppejans, 1998; van der Loos et al., 2021). Notably, four of these species (Py. katadae, Py. koreana, Py. kinositae and Py. yezoensis) are considered as non-indigenous. The records of Py. katadae, Py. kinositae and Py. koreana are the first observations for the Northeast Atlantic region, updating the number of confirmed species records of foliose Bangiales observed in the Northeast Atlantic to 20 (Mols-Mortensen et al., 2012), excluding the two unidentified Porphyra species reported in this study.
The native distribution of the here reported non-indigenous bladed Bangiales species lies in the Northwest Pacific. Py. katadae and Py. kinositae were originally described from Japan but are also abundant in Korea and, in case of Py. katadae, China (Tanaka, 1952; Miura, 1968; Kim et al., 2018). Py. katadae grows in the intertidal or upper subtidal on various substrates, such as mollusc shells, rocks or epiphytically on other seaweeds from January to April (Miura, 1968; Wang et al., 2016). It has also been recorded from New England in the northwestern Atlantic, where rbcL sequences were identical with culture material originating from Qingdao, China, as well as Japan (Neefus et al., 2008).
We only observed a single record of Py. kinositae in the mid-intertidal, but given its preference for subtidal habitats, it might be more widespread in the study area. As we did not sample the subtidal range except what is within reachable distance during spring tides and rock pools, it is possible that we missed out on additional subtidal species. Additionally, we might have missed further subtidal species that have been reported to occur in the Northeast Atlantic, such as Pyropia drachii or Wildemania amplissima (Bunker et al., 2017). Following the high species diversity and non-indigenous abundance of bladed Bangiales, efforts to sample the subtidal range should be undertaken.
Py. koreana is an epiphytic species, originally described from the east coast of Korea, where it grows in the lower intertidal or shallow subtidal and can be distinguished from similar species due to its vibrant bright red or brown-red colour and delicate thallus (Hwang and Lee, 1994). It has been reported from several locations in the Mediterranean (partly identified as Pyropia olivii), such as Greece, Italy and the Balearic Islands, as well as from the Northwest Atlantic (Brodie et al., 2007; Vergés et al., 2013; Sánchez et al., 2014). The presence of Py. koreana in the Netherlands is, to our knowledge, the first confirmed record from the Northeast Atlantic and North Sea region. We also noticed the bright brown-red colour of fresh individuals growing epiphytically on other seaweeds (in our case Sargassum muticum, Chondrus crispus, Scytosiphon lomentaria) in the shallow stagnant water. In the Mediterranean and Northwestern Atlantic, Py. koreana has been observed from winter to spring with seawater temperatures below 20°C and daylength lower than 14 h (Orfanidis, 2001; Brodie et al., 2007), which also fits with our observations, finding the small red thalli in march. With herbarium records of Py. koreana from the Mediterranean dating back to the late 19th century (Brodie et al., 2007) more detailed population studies are required to understand the true native range and origin of this species.
From the group of observed non-indigenous species, only Py. yezoensis has so far been reported from the study area during an extensive effort to document the presence of non-indigenous species within the coastal waters of the Netherlands (Gittenberger et al., 2023). Other confirmed European records of Py. yezoensis exist from the Mediterranean in Italy (Armelli Minicante et al., 2014) and France (Thau Lagoon). While Py. yezoensis has also been reported from the British Isles and Helgoland in Germany, these records concern material described by Kornmann (1986), which did not match sequence data from Py. yezoensis from Japan (Brodie and Irvine, 2003; Brodie et al., 2015). Therefore, these records are currently referred to as Py. yezoensis sensu Kornmann. A record of Py. yezoensis from the Thau Lagoon – a historic aquaculture site in the western Mediterranean Sea – goes back to 1982 (ICES, 1999) and is regarded as established (Verlaque et al., 2015).
Py. collinsii and Py. elongata have until now not been recorded from the southern North Sea along the Belgian and Dutch coastline (Coppejans, 1998; van der Loos et al., 2021) but are considered native to the North Atlantic. Py. collinsii, originally described from Connecticut, USA, has also been recorded in New England (Mathieson and Dawes, 2017) and Denmark (Nielsen and Lundsteen, 2019). Py. elongata was described from Sweden (Kylin, 1907) with several records spanning the North Atlantic and Mediterranean (e.g. Brodie et al., 2007; Mols-Mortensen et al., 2012; Sánchez et al., 2014). As we only observed one record of each species, and both were very small individuals that could have been easily overlooked, further sampling effort should be undertaken to establish a clearer picture of their abundance and distribution. In Denmark, Py. collinsii has been observed to occur only in a short time window from February to May (Nielsen and Lundsteen, 2019). Given that the majority of sampling took place in February and July we might have missed the peak abundance of this species.
Our phylogenetic analysis of the rbcL gene sequence revealed two additional distinct clades, which we failed to identify, here referred to as ‘Porphyra sp. 1’ and ‘Porphyra sp. 2’. Both lineages were reported during the winter sampling, occurring within the belt of P. linearis in the high intertidal zone. At present we cannot be conclusive on their identity. It is possible that both clades correspond to previously described species, but for which a matching rbcL sequence has not been deposited in a public repository. While known European Porphyra species have been barcoded, this is not the case of the non-European diversity. However, in the absence of a comprehensive global DNA barcoding framework, a non-indigenous origin of these lineages remains speculative. Considering the formation of the two well-supported clades based on the here presented rbcL sequences, hybridization, as observed to occur between closely related Bangiales species (Kim, 2011; Varela-Álvarez et al., 2022), is rather unlikely, but should be further investigated using gene sequencing from other genomic compartments such as mitochondrial and nuclear genomes. Further samples should be acquired to support and add more detailed morphological, ecological and molecular information to these records.
The coastal waters of the Netherlands are a hot spot of introduced non-indigenous species with over 215 non-indigenous species recorded (Gittenberger et al., 2023). As an historic aquaculture site with frequent introductions of for example C. gigas originating from the Northwest Atlantic and Pacific since the 1960’s, the high abundance of non-indigenous species is not surprising (Miossec et al., 2009; Martínez-García et al., 2022). The calcareous shells of oysters can act as vectors enabling frequent and unnoticed introductions of especially fouling organisms and are associated with many unintentional introductions of non-indigenous species (Savini et al., 2010). Given the well-reported introduction from C. gigas from the Northwest Atlantic and Pacific into the study area, it is a likely explanation for the presence of the here observed non-indigenous bladed Bangiales Py. katadae, Py. koreana, Py. kinositae and Py. yezoensis.
The lack of distinctive morphological characteristics between bladed Bangiales species makes species identification based on morphological observations difficult and unreliable unless molecular methods – as in this study – are used to confirm species identity. Consequently, there is the possibility that the introduction of non-indigenous bladed Bangiales species have been present for several decades or longer without detection.
The taxonomy of bladed Bangiales has undergone several revisions following cryptic diversity revealed by molecular methods (Díaz-Tapia et al., 2020; Sutherland et al., 2011). A combination of mitochondrial and nuclear markers is crucial in reliable species delimitation, especially in closely related bladed Bangiales such as P. dioica, P. linearis and P. umbilicalis (Klein et al., 2003; Mols-Mortensen et al., 2012) which can also hybridise (Varela-Álvarez et al., 2018). This is reflected in the poorly supported clade of P. linearis within this study. However, while we only relied on species identification on rbcL, we have high confidence in our species identification based on compliance with the included reference sequences.
Temporal variation in abundance, as observed in the case of Py. katadae, Py. koreana and Py. yezoensis, which were only abundant during winter and early spring, demonstrate the necessity for repeated sampling campaigns to account for seasonal-dependent abundances. While Py. katadae and Py. yezoensis were abundantly observed at historic oyster aquaculture sites within this study at the location Yerseke and Wemeldinge in winter and spring, they were absent when the sampling locations were revisited in summer. A more detailed temporal sampling effort is required to establish time of occurrence of these non-indigenous species and to help assess whether they might have any harmful or negative effects at the ecosystem level. The here observed temporal abundance of the non-indigenous bladed Bangiales is in accordance with their native distribution in the temperate Pacific region, where optimum temperatures of the gametophyte phase of e.g. Py. yezoensis are around 15°C (Hwang and Lee, 1994; Wang et al., 2016; Watanabe et al., 2016) and 8°C for Py. katadae (Neefus et al., 2008; Wang et al., 2016; Liang et al., 2022). In the study area, sea surface temperature varies from around 3°C in winter up to 20°C in summer (van Aken, 2010; Horn et al., 2023). The sporophyte phase in bladed Bangiales often has a higher temperature tolerance (Watanabe et al., 2016) which could explain the high abundance of Py. katadae and Py. yezoensis gametophytes during winter and the here observed absence in summer.
While bladed Bangiales in general display a simple thallus morphology with little specification, high phenotypic plasticity results in the lack of morphological characteristics required for reliable species identifications. As an example, the gametophyte of P. linearis usually grows in the form of a single foliose thallus with an elongated, sometimes lanceolate shape. However, we also observed individuals displaying a round thallus morphology, leading at a first glance to a different preliminary species identification. Taking into account the seasonality, habitat and microscopic observations of reproductive tissue can assist with identification (Brodie and Irvine, 2003). In case of P. linearis, individuals only occurred in winter and early spring and grew in the very high intertidal zone, sometimes forming a distinct band of red to red-brown individuals. We also observed P. umbilicalis in the very high intertidal, although larger individuals mostly displayed a darker, olive-green to brown colour in contrast to P. linearis. Most individuals of P. umbilicalis could also be differentiated from P. linearis by their thallus shape, while P. linearis mainly forms a single, narrow and elongated thallus which can be observed lying flat against the rock during low tide, P. umbilicalis contrastingly displays the typical rosette growth with a more central holdfast, in most cases, resulting in individuals not sticking flat on the substrate as P. linearis. Nonetheless, P. umbilicalis could be confused as P. linearis during the early growth, when individuals are still small. While the consideration of seasonality, habitat, thallus morphology and colouration can narrow down or even lead to correct species identification, ultimately, molecular methods should be taken into account for accurate species identification of bladed Bangiales.
5 Conclusion and outlook
This study revealed a high diversity of a total of 13 species of bladed Bangiales in the study area of the southern North Sea – including four identified and two unidentified Porphyra species as well as seven Pyropia species. Of the seven detected Pyropia species, four are regarded as non-indigenous and three (Py. katadae, Py. kinositae and Py. koreana) represent the first records for the Northeast Atlantic. While morphology, habitat and temporal occurrence can aid in species identification, molecular methods are required for reliable species identification. Further effort should be directed towards investigating the broader distribution of the here recorded non-indigenous bladed Bangiales, including additional aquaculture sites in the Northeast Atlantic and Mediterranean which might reveal an even greater occurrence of non-indigenous species. Furthermore, additional records including further gene sequences of the two unidentified Porphyra species should be sampled for a more detailed species description.
Data availability statement
The datasets presented in this study can be found in online repositories. The names of the repository/repositories and accession number(s) can be found in the article/Supplementary Material.
Author contributions
JK: Conceptualization, Data curation, Formal analysis, Investigation, Methodology, Project administration, Supervision, Visualization, Writing – original draft, Writing – review & editing. SB: Data curation, Formal analysis, Investigation, Visualization, Writing – review & editing. LV: Conceptualization, Data curation, Investigation, Methodology, Supervision, Writing – review & editing. SD: Methodology, Supervision, Writing – review & editing. MK: Data curation, Writing – review & editing. FP: Data curation, Writing – review & editing. FL: Project administration, Resources, Writing – review & editing. OD: Conceptualization, Data curation, Funding acquisition, Methodology, Resources, Supervision, Visualization, Writing – review & editing.
Funding
The author(s) declare financial support was received for the research, authorship, and/or publication of this article. This study received funding from Flanders Innovation & Entrepreneurship (VLAIO) under the ICON project BlueMarine³.Com (BLUEprint for a viable multispecies hatchery of 3 MARINE COMmercially attractive species groups) under the grant agreement AIOSBO2019001503. The research was facilitated with infrastructure provided by EMBRC Belgium and the Research Foundation Flanders (I001621N) and by the FWO DiSSCo Flanders project (I001721N).
Acknowledgments
We would like to thank Prof. Juliet Brodie from the London Natural History Museum for cross checking the sequences of the here presented two unidentified Porphyra species.
Conflict of interest
The authors declare that the research was conducted in the absence of any commercial or financial relationships that could be construed as a potential conflict of interest.
Publisher’s note
All claims expressed in this article are solely those of the authors and do not necessarily represent those of their affiliated organizations, or those of the publisher, the editors and the reviewers. Any product that may be evaluated in this article, or claim that may be made by its manufacturer, is not guaranteed or endorsed by the publisher.
Supplementary material
The Supplementary Material for this article can be found online at: https://www.frontiersin.org/articles/10.3389/fmars.2024.1415034/full#supplementary-material
Supplementary Material S1 | Full sample list including herbarium vouchers and GenBank accession numbers.
Supplementary Material S2 | GenBank accession numbers of reference sequences used in the phylogenetic analysis.
Supplementary Material S3 | Full maximum likelihood (IQ-TREE) phylogenetic tree based on all rbcL gene sequences.
References
Anglès d’Auriac M. B., Rinde E., Norling P., Lapègue S., Staalstrøm A., Hjermann D. Ø., et al. (2017). Rapid expansion of the invasive oyster Crassostrea gigas at its northern distribution limit in Europe: Naturally dispersed or introduced? PloS One 12, e0177481. doi: 10.1371/journal.pone.0177481
Armelli Minicante S., Genovese G., Morabito M. (2014). Two new alien macroalgae identified by the DNA barcoding, in: 45°Congressa Della Societá Italiana Di Biologia Marina. Presented at the 45°Congressa della Societá Italiana di Biologia Marina, Venezia. 21 (1), 70–72.
Blouin N. A., Brodie J. A., Grossman A. C., Xu P., Brawley S. H. (2011). Porphyra: A marine crop shaped by stress. Trends Plant Sci. 16, 29–37. doi: 10.1016/j.tplants.2010.10.004
Brodie J. A., Irvine L. M. (2003). Seaweeds of the British isles. P. 3B: Vol. 1. Rhodophyta Bangiophycidae (London: Natural History Museum).
Brodie J., Bartsch I., Neefus C., Orfanidis S., Bray T., Mathieson A. C. (2007). New insights into the cryptic diversity of the North Atlantic–Mediterranean ‘Porphyra leucosticta’ complex: P. olivii sp. nov. and P. rosengurttii (Bangiales, Rhodophyta). Eur. J. Phycol. 42, 3–28. doi: 10.1080/09670260601043946
Brodie J., Wilbraham J., Pottas J., Guiry M. D. (2015). A revised check-list of the seaweeds of Britain. J. Mar. Biol. Ass. 96, 1005–1029. doi: 10.1017/S0025315415001484
Buckley Y. M., Catford J. (2016). Does the biogeographic origin of species matter? Ecological effects of native and non-native species and the use of origin to guide management. J. Ecol. 104, 4–17. doi: 10.1111/1365-2745.12501
Bunker F. S. D., Brodie J. A., Maggs C., Bunker A. R. (2017). Seaweeds of Britain and Ireland, 2nd edition. ed, Seasearch (Plymton St. Maurice, Plymouth: Wild Nature Press).
Capella-Gutiérrez S., Silla-Martínez J. M., Gabaldón T. (2009). trimAl: a tool for automated alignment trimming in large-scale phylogenetic analyses. Bioinformatics 25, 1972–1973. doi: 10.1093/bioinformatics/btp348
Castro M. C. T. D., Fileman T. W., Hall-Spencer J. M. (2017). Invasive species in the Northeastern and Southwestern Atlantic Ocean: A review. Mar. pollut. Bull. 116, 41–47. doi: 10.1016/j.marpolbul.2016.12.048
Coppejans E. (1998). Flora van de Noord-Franse en Belgische zeewieren (Meise: Nationale Plantentuin van België. Koninklijke Nederlandse Natuurhistorische Vereniging).
Díaz-Tapia P., Ly M., Verbruggen H. (2020). Extensive cryptic diversity in the widely distributed Polysiphonia scopulorum (Rhodomelaceae, Rhodophyta): Molecular species delimitation and morphometric analyses. Mol. Phylogenet. Evol. 152, 106909. doi: 10.1016/j.ympev.2020.106909
Doyle J. J., Doyle J. L. (1987). A rapid DNA isolation procedure for small quantities of fresh leaf tissue. Phytochem. Bull. 1, 11–15.
Drew K. M. (1949). Conchocelis-phase in the life-history of Porphyra umbilicalis (L.) Kütz. Nature 164, 748–749. doi: 10.1038/164748a0
Gittenberger A., Rensing M., Faasse M., van Walraven L., Smolders S., Keeler Perez H., et al. (2023). Non-Indigenous species dynamics in time and space within the coastal waters of The Netherlands. Diversity 15, 719. doi: 10.3390/d15060719
Guillemin M.-L., Contreras-Porcia L., Ramírez M. E., Macaya E. C., Contador C. B., Woods H., et al. (2016). The bladed Bangiales (Rhodophyta) of the South Eastern Pacific: Molecular species delimitation reveals extensive diversity. Mol. Phylogenet. Evol. 94, 814–826. doi: 10.1016/j.ympev.2015.09.027
Guiry M. D., Guiry G. M. (2024). AlgaeBase: AlgaeBase (World-wide electronic publication, University of Galway). Available online at: https://www.algaebase.org (Accessed April 4, 2024).
Horn H. G., van Rijswijk P., Soetaert K., van Oevelen D. (2023). Drivers of spatial and temporal micro- and mesozooplankton dynamics in an estuary under strong anthropogenic influences (The Eastern Scheldt, Netherlands). J. Sea Res. 192, 102357. doi: 10.1016/j.seares.2023.102357
Hwang M. S., Lee I. K. (1994). Two Species of Porphyra (Bangiales, Rhodophyta), P. koreana sp. nov. and P. lacerata Miura from Korea. Algae 9, 169–177.
ICES (1999). Status of Introductions of Non-Indigenous marine species to North Atlantic waters 1981-1991. Int. Council Explor. Sea. doi: 10.17895/ICES.PUB.5362
Katoh K., Misawa K., Kuma K., Miyata T. (2002). MAFFT: a novel method for rapid multiple sequence alignment based on fast Fourier transform. Nucleic Acids Res. 30, 3059–3066. doi: 10.1093/nar/gkf436
Kim N.-G. (2011). Culture study on the hybrid by interspecific crossing between Porphyra pseudolinearis and P. dentata (Bangiales, Rhodophyta), two dioecious species in culture. Algae 26, 79–86. doi: 10.4490/algae.2011.26.1.079
Kim S.-M., Choi H.-G., Hwang M.-S., Kim H.-S. (2018). Biogeographic pattern of four endemic Pyropia from the east coast of Korea, including a new species, Pyropia retorta (Bangiaceae, Rhodophyta). ALGAE 33, 55–68. doi: 10.4490/algae.2018.33.2.26
Klein A. S., Mathieson A. C., Neefus C. D., Cain D. F., Taylor H. A., Teasdale B. W., et al. (2003). Identification of north-western Atlantic Porphyra (Bangiaceae, Bangiales) based on sequence variation in nuclear SSU and plastid rbcL genes. Phycologia 42, 109–122. doi: 10.2216/i0031-8884-42-2-109.1
Knoop J., Griffin J. N., Barrento S. (2020). Cultivation of early life history stages of Porphyra dioica from the British Isles. J. Appl. Phycol. 32, 459–471. doi: 10.1007/s10811-019-01930-6
Kornmann P. (1986). Porphyra yezoensis bei Helgoland — eine entwicklungsgeschichtliche Studie. Helgolander Meeresunters 40, 327–342. doi: 10.1007/BF01983740
Kucera H., Saunders G. W. (2012). A survey of Bangiales (Rhodophyta) based on multiple molecular markers reveals cryptic diversity. J. Phycol. 48, 869–882. doi: 10.1111/j.1529-8817.2012.01193.x
Kylin H. (1907). Studien über die Algenflora der Schwedischen Westküste (Upsala: K.W. Appelbergs buchdr).
Lallias D., Boudry P., Batista F., Beaumont A., King J., Turner J., et al. (2015). Invasion genetics of the Pacific oyster Crassostrea gigas in the British Isles inferred from microsatellite and mitochondrial markers. Biol. Invasions 17. doi: 10.1007/s10530-015-0896-1
Liang Z., Liu F., Wang W., Zhang P., Yuan Y., Liu Y., et al. (2022). Physiological and biochemical responses to light and temperature stress in free-living conchocelis of Neopyropia katadae (Bangiales, Rhodophyta). J. Appl. Phycol. 34, 1059–1072. doi: 10.1007/s10811-022-02691-5
Lindstrom S. C., Hughey J. R., Rosas L. E. A. (2015). Four new species of Pyropia (Bangiales, Rhodophyta) from the west coast of North America: the Pyropia lanceolata species complex updated. PhytoKeys 52, 1–22. doi: 10.3897/phytokeys.52.5009
Lubchenco J., Cubit J. (1980). Heteromorphic life histories of certain marine algae as adaptations to variations in herbivory. Ecology 61, 676–687. doi: 10.2307/1937433
Maggs C. A., Stegenga H. (1998). Red algal exotics on North Sea coasts. Helgolander Meeresunters 52, 243–258. doi: 10.1007/BF02908900
Martínez-García M. F., Ruesink J. L., Grijalva-Chon J. M., Lodeiros C., Arreola-Lizárraga J. A., de la Re-Vega E., et al. (2022). Socioecological factors related to aquaculture introductions and production of Pacific oysters (Crassostrea gigas) worldwide. Rev. Aquac. 14, 613–629. doi: 10.1111/raq.12615
Mathieson A. C., Dawes C. J. (2017). Seaweeds of the northwest Atlantic (Amherst & Boston: University of Massachusetts Press).
Meynard A., Zapata J., Salas N., Betancourtt C., Pérez-Lara G., Castañeda F., et al. (2019). Genetic and morphological differentiation of Porphyra and Pyropia species (Bangiales, Rhodophyta) coexisting in a rocky intertidal in Central Chile. J. Phycol. 55, 297–313. doi: 10.1111/jpy.12829
Mineur F., Le Roux A., Maggs C. A., Verlaque M. (2014). Positive feedback loop between introductions of non-native Marine Species and cultivation of oysters in Europe. Conserv. Biol. 28, 1667–1676. doi: 10.1111/cobi.12363
Miossec L., Deuff R.-M. L., Goulletquer P. (2009). Alien species alert: Crassostrea gigas (Pacific Oyster). ICES Cooperative Res. Rep. 299.
Miura A. (1968). Porphyra katadai, a new species from Japanese coast. J. Tokyo Univ. Fish 54, 55–59.
Mols-Mortensen A., Neefus C. D., Nielsen R., Gunnarsson K., Egilsdóttir S., Pedersen P. Mø., et al. (2012). New insights into the biodiversity and generic relationships of foliose Bangiales (Rhodophyta) in Iceland and the Faroe Islands. Eur. J. Phycol. 47, 146–159. doi: 10.1080/09670262.2012.666678
Morais P., Reichard M. (2018). Cryptic invasions: A review. Sci. Total Environ. 613–614, 1438–1448. doi: 10.1016/j.scitotenv.2017.06.133
Neefus C. D., Mathieson A. C., Bray T. L., Yarish C. (2008). The distribution, morphology, and ecology of three introduced Asiatic species of Porphyra (bangiales, Rhodophyta) in the Northwestern Atlantic1. J. Phycol. 44, 1399–1414. doi: 10.1111/j.1529-8817.2008.00607.x
Nelson W. A., Broom J. E., Farr T. J. (2001). Four new species of Porphyra (Bangiales, Rhodophyta) from the New Zealand region described using traditional characters and 18S rDNA sequence data. Cryptogamie Algologie 22, 263–284. doi: 10.1016/S0181-1568(01)01060-1
Nguyen L.-T., Schmidt H. A., von Haeseler A., Minh B. Q. (2015). IQ-TREE: A fast and effective stochastic algorithm for estimating maximum-likelihood phylogenies. Mol. Biol. Evol. 32, 268–274. doi: 10.1093/molbev/msu300
Nielsen R., Lundsteen S. (2019). Seaweeds of Denmark: vol. 1, Red algae (Rhodophyta), Series B, Biologica 7. ed (Copenhagen: Scientia Danica).
Nunes A. L., Katsanevakis S., Zenetos A., Cardoso A. C. (2014). Gateways to alien invasions in the European seas. AI 9, 133–144. doi: 10.3391/ai.2014.9.2.02
Olenin S., Didziulis V., Ovčarenko I., Olenina I., Nunn A. D., Cowx G. (2008). Review of introductions of aquatic species in Europe. Coordination Action IMPASSE No. EC FP6 (Klaipėda: Coastal Research and Planning Institute, Klaipėda University). doi: 10.13140/RG.2.1.4504.7449
Orfanidis S. (2001). Culture studies of Porphyra leucosticta (Bangiales, Rhodophyta) from the Gulf of Thessaloniki, Greece. Botanica Marina. 44, 533–539. doi: 10.1515/BOT.2001.059
Pejchar L., Mooney H. (2009). “Chapter 12 The impact of invasive alien species on ecosystem services and human well-being,” in Bioinvasions and Globalization. Eds. Perrings C., Mooney H., Williamson M. (Oxford: Oxford University PressOxford), 161–182. doi: 10.1093/acprof:oso/9780199560158.003.0012
Ratnasingham S., Hebert P. D. N. (2007). BOLD: the barcode of life data system (www.barcodinglife.org). Mol. Ecol. Notes 7, 355–364. doi: 10.1111/j.1471-8286.2006.01678.x
Reddy M. M., De Clerck O., Leliaert F., Anderson R. J., Bolton J. J. (2020). An appraisal of the genus Pyropia (Bangiales, Rhodophyta) from southern Africa based on a multi-gene phylogeny, morphology and ecology, including the description of Pyropia meridionalis sp. nov. South Afr. J. Bot. 131, 18–32. doi: 10.1016/j.sajb.2019.12.027
Ruiz G. M., Carlton J. T., Grosholz E. D., Hines A. H. (1997). Global invasions of marine and estuarine habitats by non-indigenous species: Mechanisms, extent, and consequences. Am. Zool. 37, 621–632. doi: 10.1093/icb/37.6.621
Sahoo D., Tang X., Yarish C. (2002). Porphyra – the economic seaweed as a new experimental system. Curr. Sci. 83, 1313–1316.
Sánchez N., Vergés A., Peteiro C., Sutherland J. E., Brodie J. (2014). Diversity of bladed Bangiales (Rhodophyta) in western Mediterranean: recognition of the genus Themis and descriptions of T. ballesterosii sp. nov., T. iberica sp. nov., and Pyropia parva sp. nov. J. Phycol. 50, 908–929. doi: 10.1111/jpy.12223
Savini D., Occhipinti–Ambrogi A., Marchini A., Tricarico E., Gherardi F., Olenin S., et al. (2010). The top 27 animal alien species introduced into Europe for aquaculture and related activities. J. Appl. Ichthyol. 26, 1–7. doi: 10.1111/jai.2010.26.issue-s2
Simberloff D., Martin J.-L., Genovesi P., Maris V., Wardle D. A., Aronson J., et al. (2013). Impacts of biological invasions: what’s what and the way forward. Trends Ecol. Evol. 28, 58–66. doi: 10.1016/j.tree.2012.07.013
Soares M. O., Xavier F. L., Dias N. M., Silva M. Q. M. da, Lima J. P. de, Barroso C. X., et al. (2022). Alien hotspot: Benthic marine species introduced in the Brazilian semiarid coast. Mar. pollut. Bull. 174, 113250. doi: 10.1016/j.marpolbul.2021.113250
Stegenga H., Karremans M. (2015). Overzicht van de roodwier-exoten in de mariene wateren van Zuidwest-Nederland. Gorteria Dutch Botanical Arch. 37, 141–157.
Stegenga H., Mol I. (1983). Flora van de Nederlandse zeewieren, Bibliotheek van de Koninklijke Nederlandse Natuurhistorische Vereniging (Zeist: Koninklijke Nederlandse Natuurhistorische Vereniging).
Stegenga H., Mol I., Prud’homme van Reine W. F., Lokhorst G. M. (1997). Checklist of the marine algae of the Netherlands (Leiden: Gorteria Dutch Botanical Archives).
Stiller J. W., Waaland J. R. (1996). Porphyra rediviva sp. nov. (Rhodophyta): A new species from northeast Pacific salt marshes. 1. J. Phycol. 32, 323–332. doi: 10.1111/j.0022-3646.1996.00323.x
Sutherland J. E., Lindstrom S. C., Nelson W. A., Brodie J., Lynch M. D. J., Hwang M. S., et al. (2011). A new look at an ancient order: generic revision of the Bangiales (Rhodophyta)1. J. Phycol. 47, 1131–1151. doi: 10.1111/j.1529-8817.2011.01052.x
Tanaka T. (1952). The systematic study of the Japanese protoflorideae Vol. 2 (Kagoshima: Memoirs of the Faculty of Fisheries, Kagoshima University), 1–92.
van Aken H. M. (2010). Meteorological forcing of long-term temperature variations of the Dutch coastal waters. J. Sea Res. 63, 143–151. doi: 10.1016/j.seares.2009.11.005
van der Loos L. M., Bafort Q., Bosch S., Ballesteros E., Bárbara I., Berecibar E., et al. (2023). Non-indigenous seaweeds in the Northeast Atlantic Ocean, the Mediterranean Sea and Macaronesia: a critical synthesis of diversity, spatial and temporal patterns. Eur. J. Phycol. 0, 1–30. doi: 10.1080/09670262.2023.2256828
van der Loos L., Karremans M., Perk F. (2021). Veldgids Zeewieren, 1e druk. ed, Veldgids (Zeist: KNNV Uitgeverij).
Varela-Álvarez E., Loureiro J., Paulino C., Serrão E. A. (2018). Polyploid lineages in the genus Porphyra. Sci. Rep. 8, 1–15. doi: 10.1038/s41598-018-26796-5
Varela-Álvarez E., Meirmans P. G., Guiry M. D., Serrão E. A. (2022). Biogeographic population structure of chimeric blades of Porphyra in the Northeast Atlantic reveals southern rich gene pools, introgression and cryptic plasticity. Front. Plant Sci. 13. doi: 10.3389/fpls.2022.818368
Vergés A., Comalada N., Sánchez N., Brodie J. (2013). A reassessment of the foliose Bangiales (Rhodophyta) in the Balearic Islands including the proposed synonymy of Pyropia olivii with Pyropia koreana. Botanica Marina 56, 229–240. doi: 10.1515/bot-2013-0003
Verlaque M., Ruitton S., Mineur F., Boudouresque C. F. (2015). CIESM Atlas of exotic macrophytes in the Mediterranean Sea Vol. 38 (Monaco: CIESM Publisher).
Wang H. W., Kawaguchi S., HOriguchi T., Masuda M. (2000). Reinstatement of Grateloupia catenata (Rhodophyta, Halymeniaceae) on the base of morphology and rbcL sequences. Phycologia 39, 228. doi: 10.2216/i0031-8884-39-3-228.1
Wang W.-J., Sun X.-T., Liu F.-L., Liang Z.-R., Zhang J.-H., Wang F.-J. (2016). Effect of abiotic stress on the gameophyte of Pyropia katadae var. hemiphylla (Bangiales, Rhodophyta). J. Appl. Phycol. 28, 469–479. doi: 10.1007/s10811-015-0579-4
Watanabe Y., Yamada H., Mine T., Kawamura Y., Nishihara G. N., Terada R. (2016). Photosynthetic responses of Pyropia yezoensis f. narawaensis (Bangiales, Rhodophyta) to a thermal and PAR gradient vary with the life-history stage. Phycologia 55, 665–672. doi: 10.2216/16-25.1
Yang L.-E., Deng Y.-Y., Xu G.-P., Russell S., Lu Q.-Q., Brodie J. (2020). Redefining Pyropia (Bangiales, Rhodophyta): Four new genera, resurrection of Porphyrella and description of Calidia pseudolobata sp. nov. From China. J. Phycol. 56, 862–879. doi: 10.1111/jpy.12992
Yang L.-E., Lu Q.-Q., Brodie J. (2017). A review of the bladed Bangiales (Rhodophyta) in China: history, culture and taxonomy. Eur. J. Phycol. 52, 1–13. doi: 10.1080/09670262.2017.1309689
Keywords: rbcL, Porphyra, Pyropia, diversity, non-indigenous species, invasive species
Citation: Knoop J, Bouckenooghe S, van der Loos LM, D’hondt S, Karremans M, Perk F, Leliaert F and De Clerck O (2024) DNA barcoding reveals an extensive number of cryptic introductions of bladed Bangiales (Rhodophyta) in the Southern North Sea. Front. Mar. Sci. 11:1415034. doi: 10.3389/fmars.2024.1415034
Received: 09 April 2024; Accepted: 22 July 2024;
Published: 09 August 2024.
Edited by:
Zhe Qu, Ocean University of China, ChinaReviewed by:
Kathryn Schoenrock, University of Galway, IrelandNarongrit Muangmai, Kasetsart University, Thailand
Copyright © 2024 Knoop, Bouckenooghe, van der Loos, D’hondt, Karremans, Perk, Leliaert and De Clerck. This is an open-access article distributed under the terms of the Creative Commons Attribution License (CC BY). The use, distribution or reproduction in other forums is permitted, provided the original author(s) and the copyright owner(s) are credited and that the original publication in this journal is cited, in accordance with accepted academic practice. No use, distribution or reproduction is permitted which does not comply with these terms.
*Correspondence: Jessica Knoop, SmVzc2ljYS5Lbm9vcEB1Z2VudC5iZQ==