- Key Laboratory of Freshwater Fish Reproduction and Development (Ministry of Education); Key Laboratory of Aquatic Science of Chongqing; School of Life Sciences, Southwest University, Chongqing, China
Distant hybridization is a well-established technique in genetic breeding for cultivation of superior traits. Previous studies have shown that, hybrids derived from the cross between Schizothorax wangchiachii (♀) and Percocypris pingi (♂) are significantly different from their parents in terms of growth performance, feeding behavior and disease resistance. Both cellular and plasma components of blood are integral to immune system, and erythrocytes serve as the sole cells for transporting oxygen which makes energy available for the growth, development, immune responses and all other physiological activities. In order to understand ecological adaptive strategies and also the underlying physiological and biochemical mechanisms of fish with different characteristics, the present study used offsprings and hybrids produced by artificial reproduction of the same batch of domesticated S. wangchiachii, P. pingi broodstock as the experimental fish, and analyses of biochemical indexes, differential leucocytes counts and histological examination of liver sections were performed. Results showed that the proportions of lymphocytes and thrombocytes in P. pingi were significantly higher than those in S. wangchiachii (P<0.05), while the proportions of granulocytes and monocytes in S. wangchiachii were significantly higher than those in P. pingi (P<0.05). In contrast, all blood cell values of hybrids fell between those of its parent species, with the exception of the percentage of thrombocytes, which was the highest of the three. The erythrocyte area, perimeter and short diameter of hybrid fish were significantly higher than those of S. wangchiachii and P. pingi (P<0.0001), while P. pingi exhibited the smallest erythrocyte values. Hepatocyte area, perimeter and short diameter were also significantly higher in hybrids than in S. wangchiachii and P. pingi, and P. pingi had the smallest hepatocyte area, perimeter, long diameter and short diameter. However, size and perimeter of hepatic nucleus of hybrids were the smallest. The number of Kupffer cells per unit area of liver was significantly greater in S. wangchiachii than in hybrids and Percocypris pingi (P<0.05). In terms of biochemical markers, plasma C-reactive protein level was nearly undetectable in S. wangchiachii, but it could reach 10 mg/L in both P. pingi and hybrids. Besides, plasma total bile acids was significantly higher in S. wangchiachii than in P. pingi (P<0.01), and hybrids fell in intermediate level. These results suggest that there may be differences in the immune functions among S. wangchiachii, P. pingi, and their hybrids, as well as in their liver metabolism and protein synthesis capabilities. These findings here might provide some crucial clues in understanding the biodiversity and fish adaptation, further promote the development of wild fish cultivation.
1 Introduction
Schizothorax wangchiachii, a species endemic to the upper reaches of the Yangtze River, belongs to the order Cypriniformes, family Cyprinidae, subfamily Schizothoracinae. Percocypris pingi, also wildly distributed in the upper reaches of Yangtze River and has long been recognized for its delectable flesh and nutritional value, shares the same order and family, but is distinct in its subfamily, the Barbinae (Ding, 1994), and its wild population has been listed in China’s Class II National Key Protection Fish since 2021. Due to overfishing and habitat destruction, which is largely caused by anthropogenic activities such as extensive water conservancy projects, wild populations of both S. wangchiachii and P. pingi have declined markedly over recent decades. In response to these conservation concerns, while also aiming to satisfy human demands, advancements have been made in the artificial propagation of these two species. However, S. wangchiachii prefers cooler water temperatures, exhibits slower growth rate and weak disease resistance, particularly against infections from pathogens such as Ichthyophthirius multifiliis and Saprolegnia (Li et al., 2014). To address these challenges, our laboratory team initiated a hybridization endeavor between S. wangchiachii and P. pingi in 2017, and successfully obtained hybrid fish progeny (Chen et al., 2015; Yang et al., 2018). Subsequent researches revealed that, these hybrid fish possess different ecological habits, dietary preferences, gut structure and microflora, setting them apart from their parental species (Gu et al., 2019; Wang et al., 2022). Most notably, the hybrid fish demonstrated a significantly higher resistance to I. multifiliis compared to S. wangchiachii (Gu et al., 2023), suggesting a different immune system of hybrids from their parental fish and also providing a novel mode for further researches on the underlying mechanism of fish disease resistance and other differential characteristics.
Adapted to the local environment, different fish exhibit varied functional traits, which determines where they live, how they interact with each other (Cadotte et al., 2011). Physiological traits is a subset of functional traits, measured at levels of molecular, biochemical and cellular, inevitably affecting the performance of fish, and these can arise through a variety of processes including acute responses to environmental change, developmental programs across ontogeny, and even evolutionary adaptation (Schulte and Healy, 2022). Fish blood, as one of the most important tissues, plays a multifaceted roles in maintaining the health and vitality of these aquatic creatures. It is generally acknowledged that the various cellular components of fish blood, including leukocytes, thrombocytes and erythrocytes, contribute to a range of critical functions such as immune defense, gas delivery and exchange, coagulation and so on. Leukocytes are primarily recognized for their immune functions, forming a crucial line of defense against pathogens and foreign substances, by identifying and neutralization. Thrombocytes, on the other hand, are pivotal in regulating blood coagulation, ensuring that any injuries to the fish are effectively managed by preventing excessive bleeding and facilitating clot formation. Recent research has expanded the role of thrombocytes in immune responses. They are capable of phagocytosing small molecules and bacteria, expressing immune-related genes, and processing and presenting antigens (Harikrishnan et al., 2003; Nagasawa et al., 2014; Ferdous and Scott, 2023). Erythrocytes, the most abundant cells in fish blood, are traditionally known for their oxygen-carrying capacity. However, emerging studies have shed light on their multifunctional nature. Fish erythrocytes are nucleated, and they contain organelles such as mitochondria and lysosomes (Shen et al., 2018). These organelles enable erythrocytes to express genes related to immunity and stress responses (Puente-Marin et al., 2019), produce interleukins and related specific signaling molecules, and even recognize and clear pathogens (Ortega-Villaizan et al., 2022). Therefore, all cellular components of blood contribute to fish immune response. In addition, physiological and biochemical indices of fish blood serves as valuable indicators of the fish health status. For instance, significant differences in the long and short diameter of erythrocyte have been observed between wild and domesticated individuals of Schizothorax prenanti and Schizothorax davidi (Chen et al., 2009). The blood indices are also highly sensitive to external environmental changes. For example, it has shown that Mugil cephalus exhibits changed blood parameters under arsenic stress (Fazio et al., 2019). Similarly, altered blood indices have been reported in Sinibrama taeniatus under starvation (Shi et al., 2023), fish such as Epinephelus coioides experiencing low-temperature and bacterial infections (Harikrishnan et al., 2003; Nagasawa et al., 2014; Sun et al., 2019). Thus, the blood of fish is a complex and vital tissue that not only facilitates essential physiological processes but also serves as important biomarkers for fish health, activities and life strategy assessment.
Combined with the above-mentioned information, we hypothesized that blood physiological and biochemical indexes might reflect fish ecological traits such as dietary preferences and disease resistance. In this study, we comparatively analyzed the erythrocyte morphology, the leukocytes compositions, liver structure and its resident immune cells, alongside the blood physiological and biochemical parameters in S. wangchiachii, P. pingi and their hybrids, with the aim to investigate physiological and cellular basis of the ecological characteristics of these fish, and to unravel the underlying mechanism of their differential in disease resistance and diet preference, and thus to provide the theoretical basis for the conservation and utilization of the wild resources of S. wangchiachii and P. pingi, as well as for the aquaculture practice of their hybrid progeny.
2 Materials and methods
2.1 Fish husbandry
The experimental fish were reared in the Key Laboratory of Freshwater Fish Resources and Reproductive Development of the Ministry of Education, Southwest University, China. All fish used in this study were offsprings or hybrids produced by artificial reproduction of the same batch of domesticated S. wangchiachii, P. pingi broodstock. After artificial reproduction, juveniles were transferred to our laboratory and raised separately in 300L water-circulating system for 2 years, with the same condition as illustrated by the previous study in our laboratory (Gu et al., 2023). In brief, the fish were raised under water temperature of 20 ± 1°C, pH=8.1 ± 0.5, dissolved oxygen of 6.8 mg/L, and photoperiod of 12h light/12h dark. Corresponding to different developmental stages, fish were fed with commercial diet (Shandong Shengsuo Co, Ltd.) in different diameters at a ratio of 1% body weight twice a day, at 9:00 and 18:00 respectively. In the present study, a total of 45 2-year healthy fish were used, with 15 individuals of each species. In order to avoid any growth or health related impacts on the results, fish were all at a basic level of health, with no observed clinical signals of disease, malformation or injuries, nor altered behaviors, and also in an equivalent size.
2.2 Declaration of Ethics
All procedures were submitted and authorized by the Ethical Committee of the Southwest University (IACUC-20200710–02), and performed according to the accepted standards for animal care of Southwest University, which is in accordance with the National Research Council (US) Committee for the Update of the Guide for the Care and Use of Laboratory Animals (2011).
2.3 Measurement of biometrics
After anesthetization by MS222 (Sigma, USA), basic biometric parameters such as body mass, body length, full length, weight of liver, weight of visceral organs of eight healthy individuals of each S. wangchiachii, P. pingi and hybrid fish were measured, and the condition factor (CF), hepatosomatic index (HSI), and viscerosomatic index (VSI) were calculated according to the following formulas.
2.4 Peripheral blood smear preparation and differential leucocytes counts
After rapid measurement of the above biometric indexes, blood was drawn from the caudal vein of each individual fish using sterilized 1 mL syringes and stored into separate heparinized plastic 1.5 mL tube. Three blood smears per individual were made, and these smears were fixed by methanol for 3 min, followed by staining with Swiss-Giemsa solution (DM0007, Leagene Biotechnology, Beijing, China) for 60 min. The stained smears were then dried in an oven at 37°C before being sealed with neutral resin (E675007, BBI Life Science Corporation, Shanghai, China). The blood smears were examined and photographed with a microscopic imaging system (Nikon, Japan). Differential leucocytes counts were performed using the Image-Pro Plus 6.0 software (Media Cybernetics, USA). Specifically, the number of lymphocytes, granulocytes, monocytes, and thrombocytes in 50 randomly selected high-powered (40×) fields of vision were counted for each fish, and their percentages were calculated as well. In parallel, cellular indices including size, long and short diameter, size and perimeter of nucleus of 100 erythrocytes per fish were measured.
2.5 Liver histology and counting of resident immune cells
After blood sampling, the liver of each individual were rapidly dissected, followed by fixation in Bouin’s solution (LA0976, Fuzhou Feijin Biotechnology Co., Fujian, China) for 24 h. Preparation of liver sections and observation of histological traits were based on our previous study (Liu et al., 2023). In brief, after fixation, the liver tissues were then subjected to a series of dehydration steps: transitioning through increasing concentrations of alcohol (from 70% to 100%), followed by treatment with xylene, and paraffin embedding. Embedded tissues were then sectioned in 5 μm, stained with hematoxylin (BA4097, BaSo Diagnostics Lnc. Zhuhai, Guangdong, China) and eosin (G1100, Beijing Solarbio Technology Co., Ltd, Beijing, China) (H.E.) solution, and then photographed under a microscope (Nikon, Japan). Using Image-Pro Plus 6.0 software (Media Cybernetics, USA), a total of 100 hepatocytes were randomly selected for each fish species and measured for parameters including perimeter, area, long and short diameters of hepatocytes, and nuclear perimeter and area. Furthermore, to assess the liver-resident immune cell populations, 20 equal-area (167×223 μm2) fields of vision per individual were examined, focusing on Kupffer cells and melanomacrophages.
2.6 Plasma isolation and determination of biochemical indices
Plasma was separated from the blood by centrifugation at 2000g for 10 min at 4°C, and then transferred to a clean tube and stored at -20°C until analyses. Superoxide dismutase (SOD, #A001–3-2), and catalase (CAT, #A007–1-1) activities were measured using the corresponding commercial kits (Nanjing Jiancheng Biological Research Institute, Jiangsu, China) according to the user’s manual.
Contents or activities of albumin transaminase (ALT, #CH0101201), albumin transaminase (AST, #CH0101202), total serum protein (TP, #CH0101008), alkaline phosphatase (ALP, #CH0101203), total bile acids (TBA, #CH0101005), blood glucose (GLU, #CH0101102), creatinine (CREA, #CH0101053), lactate dehydrogenase (LDH, #CH0101208), and C-reactive protein (CRP, #CH0101005) were determined using a fully automated biochemical analyzer (Hitachi 7100, Japan) according to the instructions of the corresponding kits (Mcccura Biotechnology Co.Ltd., Chengdu, China).
2.7 Statistics
All experimental data in this study were presented as Mean ± Standard Error (Mean ± SE). One-way Anova was performed using GraphPad Prism (GraphPad Software Inc, San Diego, CA) and followed by Tukey’s post hoc multiple tests. In case of heterogeneity of variance was assumed, the nonparametric Kruskal-Wallis test was selected, and followed by Dunn’s method for subsequent post-hoc multiple comparisons. A threshold of P<0.05 was applied to denote statistically significant differences.
3 Results
3.1 Comparison of basic biological indices of S. wangchiachii, P. pingi and their hybrids
The weight, body length and full length of hybrids were slightly larger than those of S. wangchiachii and P. pingi, but without significance. Condition factors of S. wangchiachii, hybrids, and P. pingi were 1.62 ± 0.05%, 1.41 ± 0.03%, and 1.28 ± 0.03% respectively, and the difference between the former two fish was significant (P<0.05). The liver of S. wangchiachii was 0.53 ± 0.06 g, which was significant weightier than that of hybrids (0.32 ± 0.04 g, P<0.01), whereas P. pingi had the smallest liver weight (0.15 ± 0.01 g). Hepatosomatic index, on the other hand, was significantly greater in the S. wangchiachii than in P. pingi and hybrids (P<0.05). There was no significant difference in either weight of visceral mass or viscerosomatic index among the three (Table 1).
3.2 Leucocyte composition and erythrocyte morphology in S. wangchiachii, P. pingi and their hybrids
Morphology of various white blood cells in these three fish were similar (Figure 1). However, the composition ratios of various white blood cells were different (Figure 2). Based on differential leucocytes counts, the percentage of each cell type in blood of S. wangchiachii was, in descending order, lymphocytes, monocytes, thrombocytes and granulocytes. In hybrid fish, the sequence was thrombocytes, lymphocytes, monocytes and granulocytes. For P. pingi, the order was lymphocytes, thrombocytes, monocytes and granulocytes. Among these three fish, P. pingi, exhibited the highest proportion of lymphocytes, with a value of 52.75 ± 2.92%, which was slightly larger than the value of S. wangchiachii (40.24 ± 4.32%) and significantly higher than that of hybrid fish (35.54 ± 2.65%, P< 0.01). The percentage of granulocytes was highest in the S. wangchiachii, with a value of 6.27 ± 1.90%, followed by hybrid fish (2.10 ± 0.82%), and then P. pingi (0.18 ± 0.03%), the values between S. wangchiachii and P. pingi was significant different (P< 0.01). The percentage of monocytes was similar to the results of granulocytes, showing a highest proportion in S. wangchiachii (26.59 ± 3.35%), followed by hybrid fish (17.47 ± 1.56%), and the lowest in P. pingi (4.24 ± 0.49%), and differences among these three fish were significant (P<0.05). Whereas, thrombocytes in hybrid fish and P. pingi accounted for 44.89 ± 2.06% and 42.83 ± 2.55%, respectively, which were significantly higher that in S. wangchiachii (26.90 ± 4.68%) (P<0.05, Figure 2).
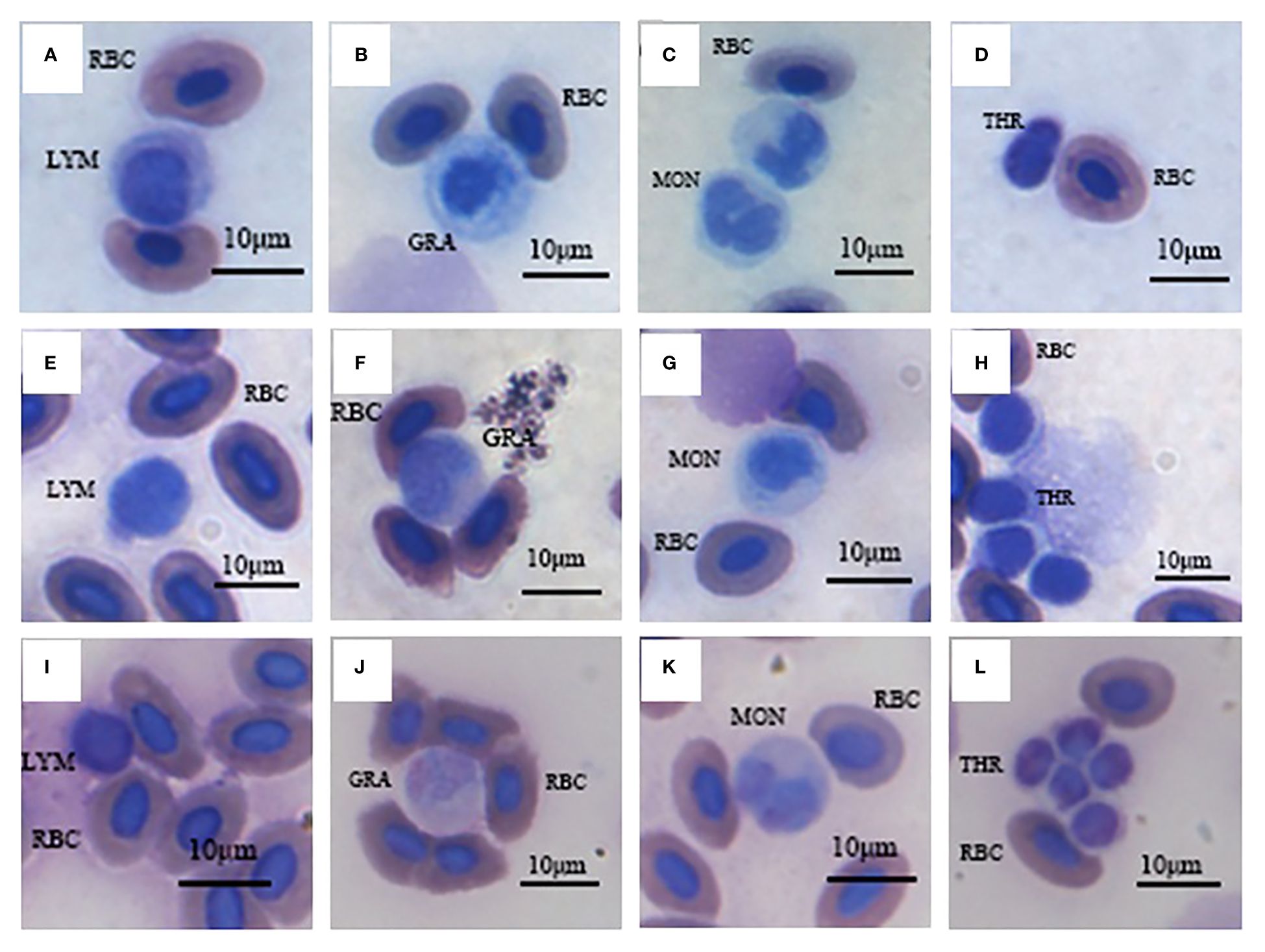
Figure 1 Various blood cells of S. wangchiachii (A-D), P. pingi (I-L) and their hybrids (E-H). LYM, lymphocyte; GRA, granulocyte; MON, monocyte; THR, thrombocyte; RBC, red blood cell.
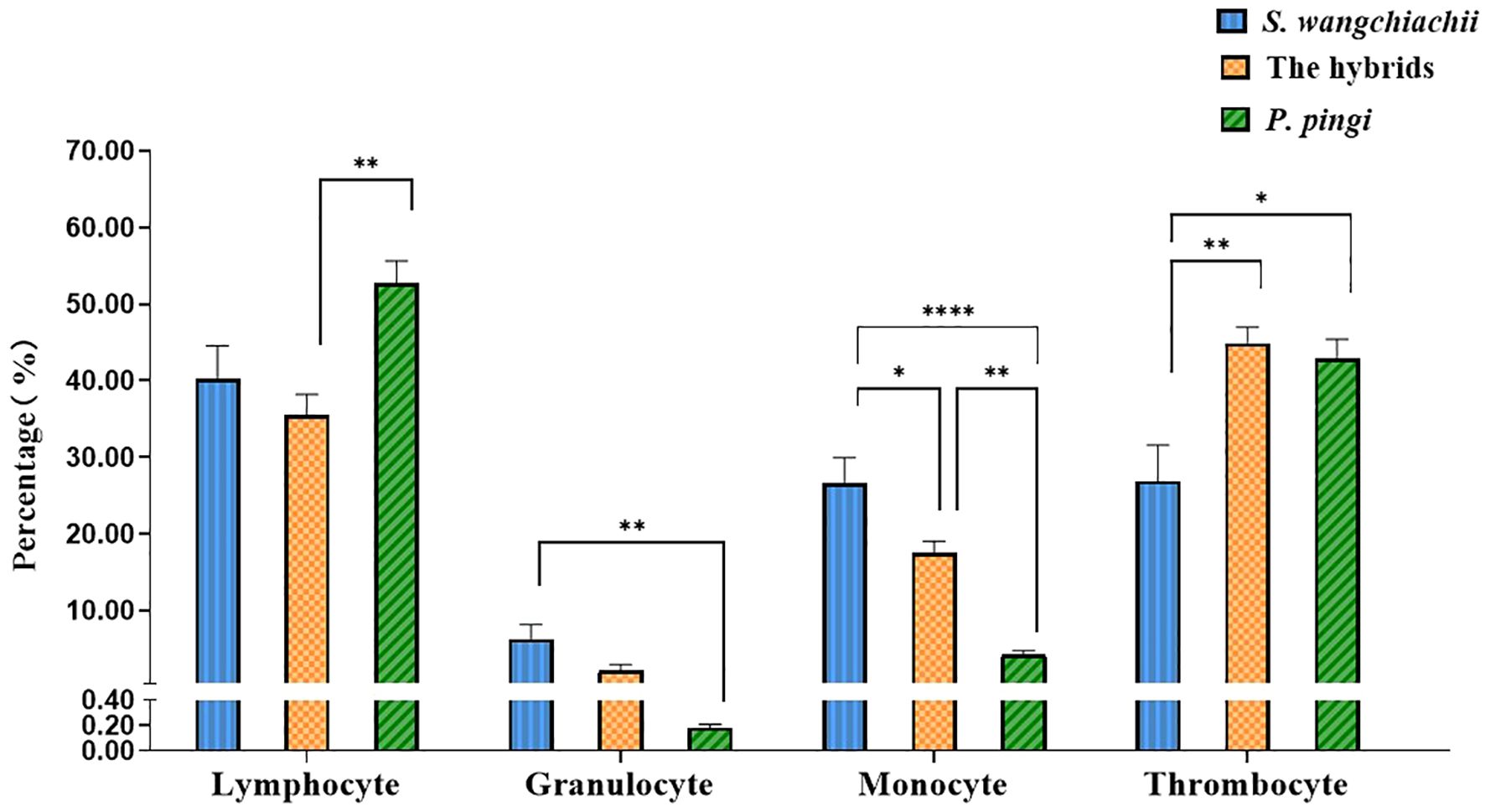
Figure 2 Percentage of lymphocyte, granulocyte, monocyte and thrombocyte in peripheral blood among S. wangchiachii, P. pingi and their hybrids. *:P<0.05; **:P<0.01; ****:P<0.0001.
In addition, we also measured the morphological indexes of 100 erythrocytes from each of the three fish (Figure 3), and the results showed that the erythrocyte area, perimeter and short diameter of the hybrid fish reached 111.80 ± 1.02 μm2, 41.17 ± 0.38 μm and 9.70 ± 0.06 μm, respectively, all of which were significantly higher than those of S. wangchiachii and P. pingi, and values in P. pingi were significant lower than those in S. wangchiachii as well (P<0.01). The long diameter of erythrocyte in S. wangchiachii and hybrid fish were comparable, and were significantly higher than that in P. pingi (P<0.0001). S. wangchiachii and hybrid fish also showed comparable size and perimeter of erythrocyte nucleus, which were significantly higher than those of P. pingi (P<0.0001).
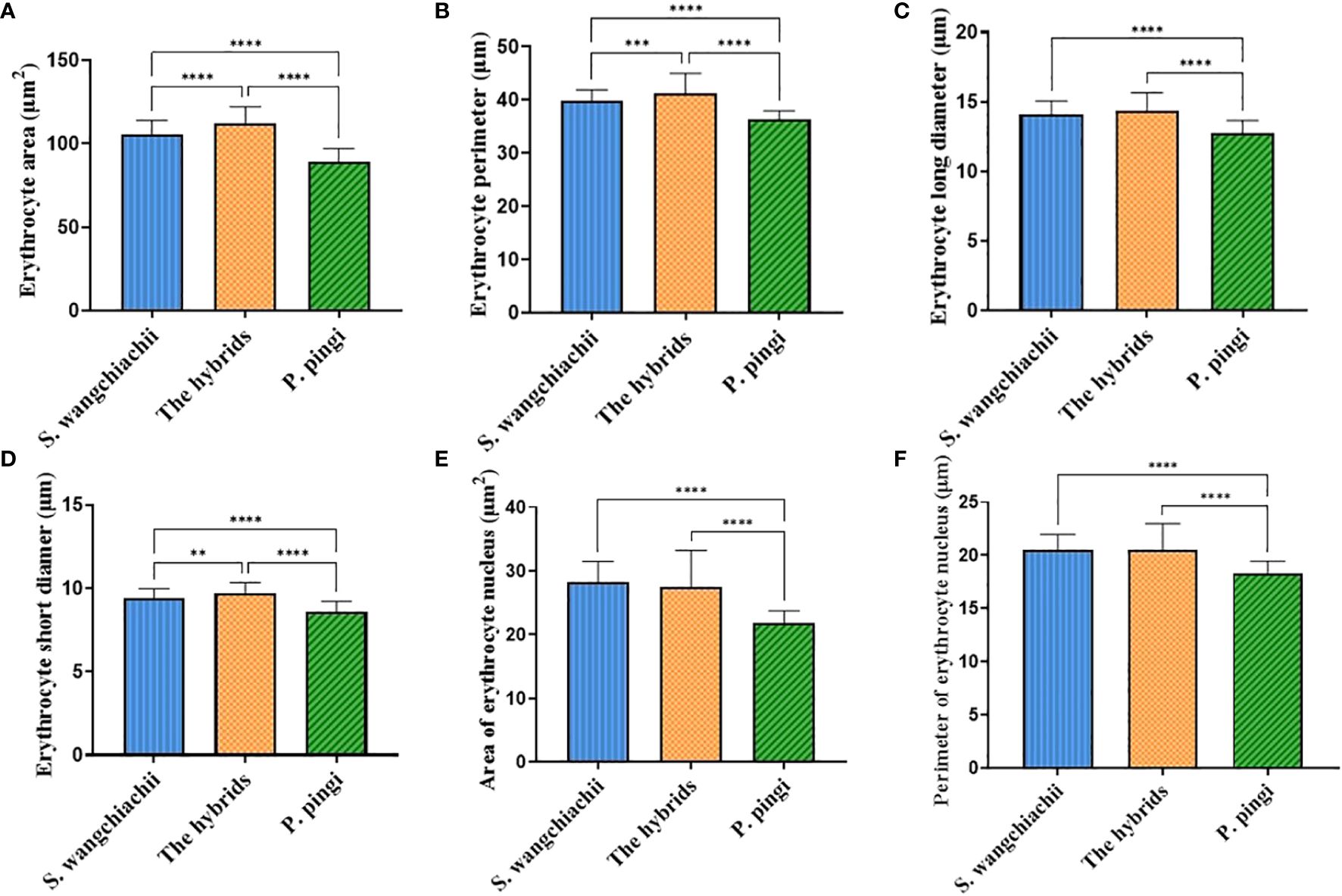
Figure 3 Comparison of erythrocyte morphologic indexes among S. wangchiachii, P. pingi and their hybrids. (A) area of erythrocyte; (B) perimeter of erythrocyte; (C) long diameter of erythrocyte; (D) short diameter of erythrocyte; (E) area of erythrocyte nucleus; (F) perimeter of erythrocyte nucleus. **:P<0.01; ***:P<0.001; ****:P<0.0001.
3.3 Blood biochemical indices of S. wangchiachii, P. pingi and their hybrids
There were no significant differences in most of the blood physiological and biochemical indices of S. wangchiachii, P. pingi and their hybrids, but the levels of TBA and CRP were noteworthy (Table 2). Plasma TBA content was 14.78 ± 6.27 μmol/L, being significant higher than that of hybrid fish and P. pingi (P<0.05). Plasma CRP in S. wangchiachii could hardly be detected in all samples, but reached 11.70 ± 3.11 mg/L and 11.44 ± 2.50 mg/L in hybrids and P. pingi, respectively.
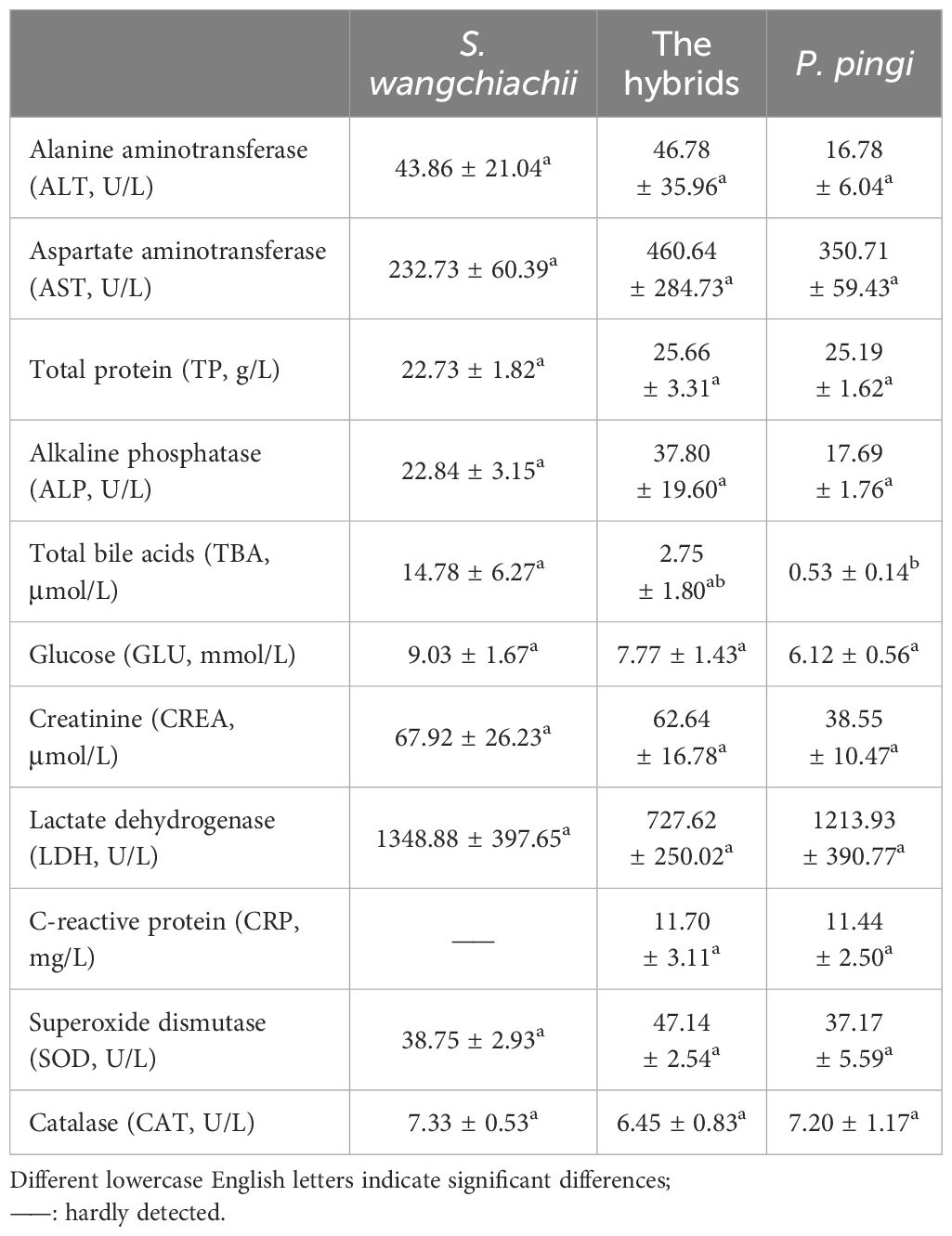
Table 2 Comparison of blood biochemical indexes among S. wangchiachii, P. pingi and their hybrids ( ± SE).
3.4 Comparison of hepatocytes morphology and resident immune cells in S. wangchiachii, P. pingi and their hybrids
Since the weight of liver, and HSI of these three fish showed significant differences, liver histology was observed to check the cellular difference. In general, the liver structures of all three fish were similar, with abundant big hepatocytes, some small Kupffer cells, and scattered oval melanomacrophages in orange (Figure 4). However, in terms of the hepatocytes morphology, difference existed. The size, perimeter and long diameter of hepatocytes of hybrid fish reached 184.00 ± 3.87 μm2, 51.69 ± 0.58 μm and 19.26 ± 0.28 μm, respectively, which were significantly higher than those of S. wangchiachii and P. pingi (P<0.0001). While the area and perimeter of hepatic nucleus of hybrid fish were just 17.45 ± 0.35 μm2 and 16.91 ± 0.22 μm, respectively, both of which were extremely significantly lower than those of S. wangchiachii and P. pingi (P< 0.0001). P. pingi exhibited the smallest hepatocyte cell area (94.75 ± 3.12 μm2), perimeter (36.70 ± 0.65 μm), long diameter (13.57 ± 0.28 μm), and short diameter (8.47 ± 0.14 μm, P< 0.0001), and the area (25.1 ± 0.48 μm2) and perimeter (20.74 ± 0.29 μm) of nucleus were intermediate among the three fish. Area (161.00 ± 4.11 μm2), perimeter (47.69 ± 0.67 μm), and long diameter (17.25 ± 0.28 μm) of S. wangchiachii were intermediate for all three fish, whilst the area (29.84 ± 0.45 μm2) and perimeter (23.50 ± 0.25 μm) of nucleui of this fish were significantly higher than those of hybrid fish and P. pingi (P<0.0001, Figure 5).
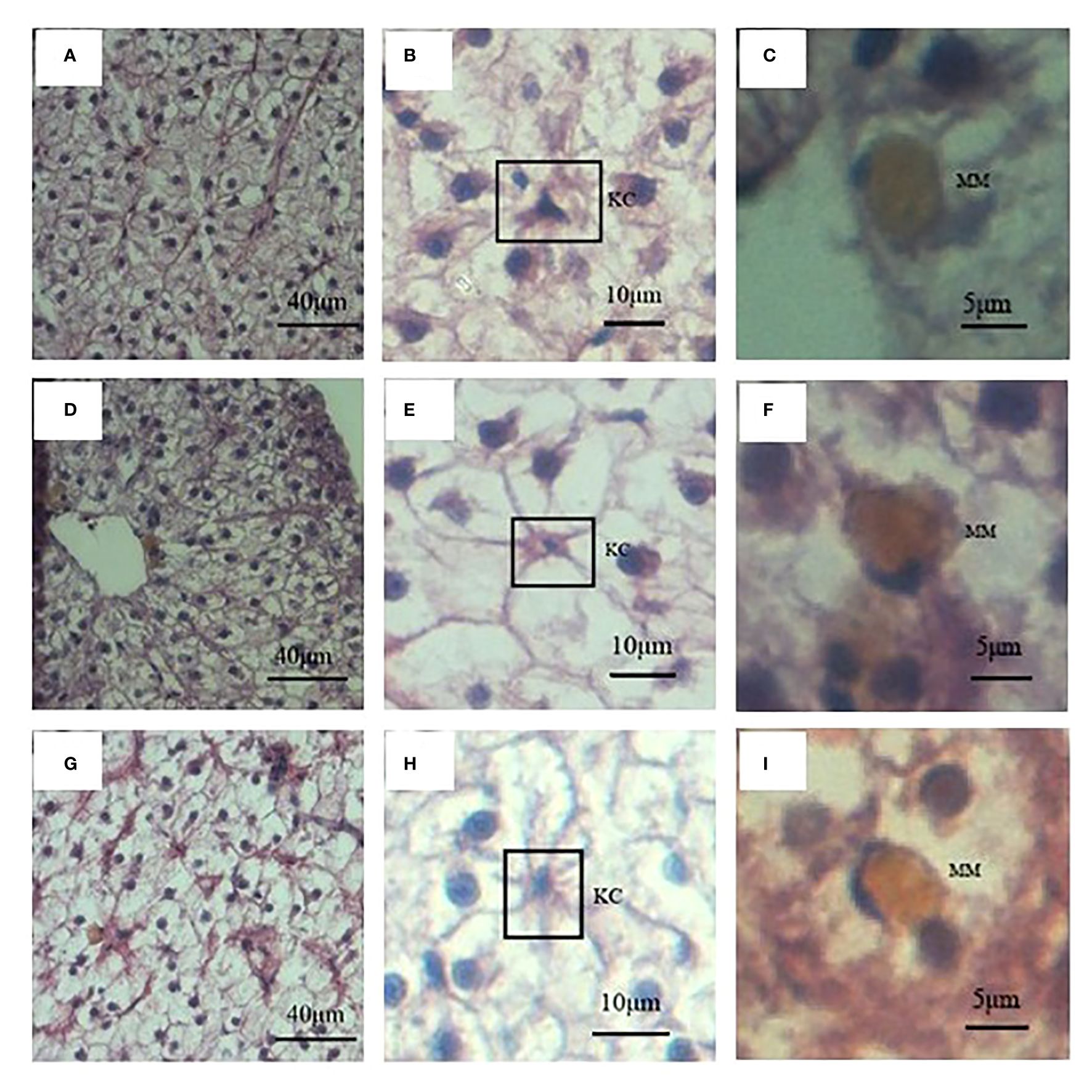
Figure 4 Liver histology and immune cells of S. wangchiachii, P. pingi and their hybrids. (A) liver histology of S. wangchiachii; (B) amplification of A, the box indicates Kupffer cell of S. wangchiachii; (C) amplification of A, showing melanomacrophage of S. wangchiachii; (D) liver histology of P.pingi; (E) amplificaiton of D, the box indicates Kupffer cell of P.pingi; (F) amplification of (E), showing melanomacrophage of P.pingi; (G) liver histology of the hybrids; (H) amplification of (G), the box indicates Kupffer cell of the hybrids; (I) amplification of (G), showing melanomacrophage of the hybrids. KC, Kupffer cell; MM, melanomacrophage.
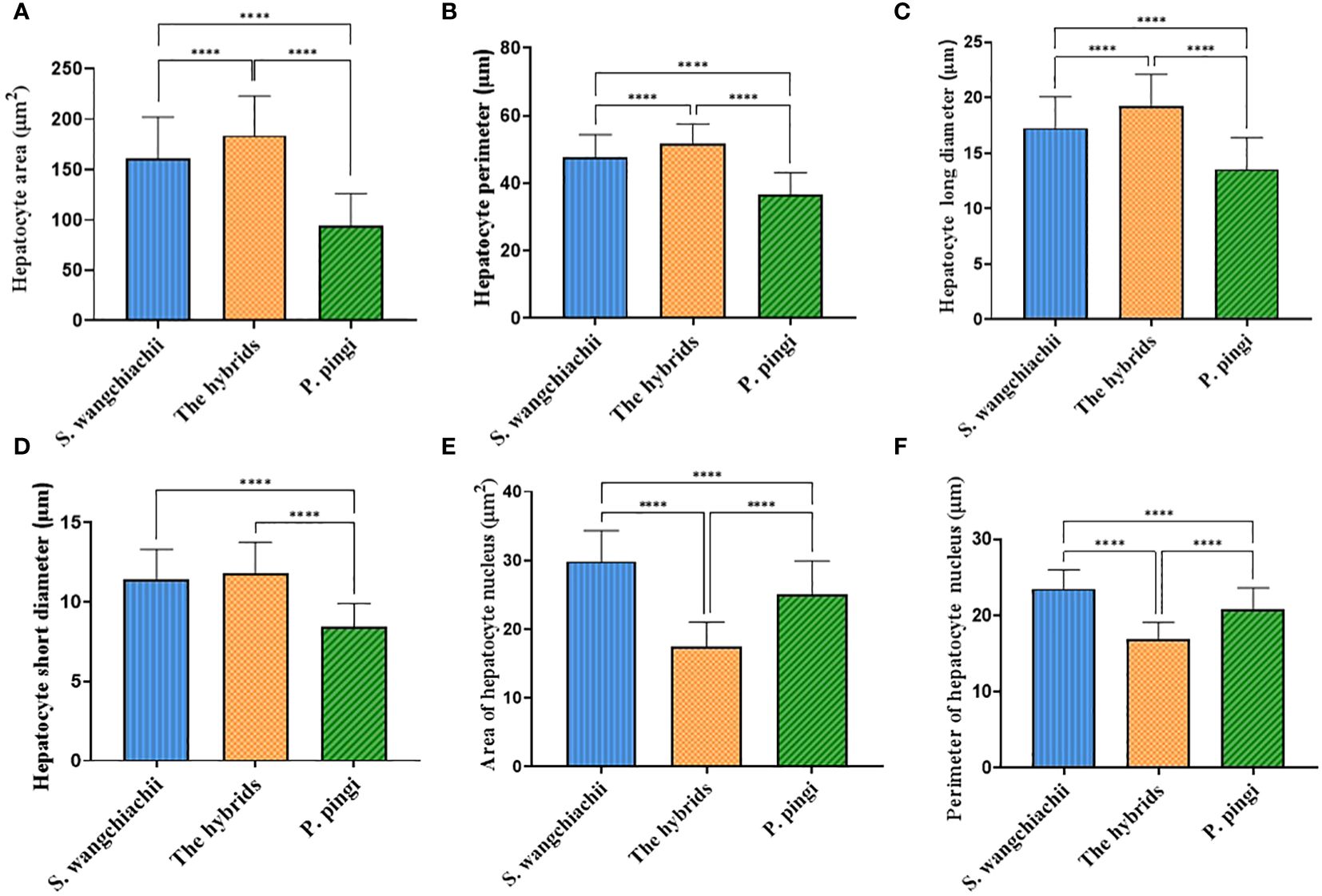
Figure 5 Comparison of hepatocyte morphologic indexes among S. wangchiachii, P. pingi and their hybrids. (A) area of hepatocyte; (B) perimeter of hepatocyte; (C) long diameter of hepatocyte; (D) short diameter of hepatocyte; (E) area of hepatocyte nucleus; (F) perimeter of hepatocyte nucleus. *:P<0.05; **:P<0.01; ****:P<0.0001.
Besides, the resident immune cells in liver of these three fish were also counted. In a same 20 fields of vision, 156 immune cells were counted in the liver of S. wangchiachii, of which 81.22 ± 2.25% were Kupffer cells. In hybrid fish liver, 127 immune cells were counted and Kupffer cells accounted for 72.80 ± 3.96%. While in P. pingi lvier, 113 immune cells were observed, and 77.24 ± 8.16% were Kupffer cells. Regarding the number of Kupffer cells in a certain area, S. wangchiachii exhibited a significantly higher value than hybrid fish and P. pingi (P<0.05), but there was no significant difference in the number of melanomacrophages per unit area among the three fish (Table 3).
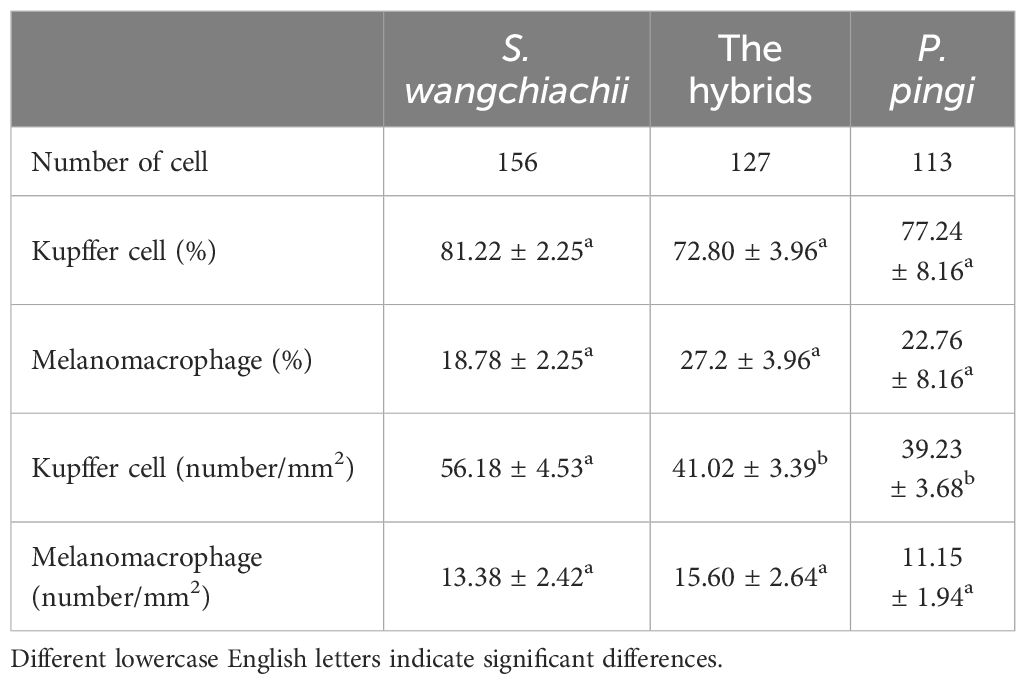
Table 3 Comparison of differential immunocyte count among S. wangchiachii, P. pingi and their hybrids ( ± SE).
4 Discussion
Erythrocytes, the most abundant cells in the blood, play a paramount role in oxygen transportation. The characteristics of erythrocytes, particularly the size and shape, serves indicators of fish life history strategies (Snyder and Sheafor, 1999). For instance, there is a reverse relationship between the size of erythrocytes and both the metabolic rate and swimming capabilities of fish (Lay and Baldwin, 1999; Witeska, 2013). The average size of erythrocytes in fish is approximately 76.64 μm2 (Martins et al., 2021). When comparing reported species within the same order, the erythrocyte size of P. pingi measured here is comparable to that of the crucian carp (Carassius auratus, 83.41μm2), while S. wangchiachii erythrocytes here resemble to that of Tinca tinca (111.50 μm2, Martins et al., 2021). In terms of critical swimming speed, S. wangchiachii around 4 cm can achieve 65 cm/s (Lu et al., 2017), while that of P. pingi around 5 cm can surpass 80 cm/s (Lu et al., 2019), suggesting that P. pingi possesses a slightly greater locomotor ability than S. wangchiachii. Fish locomotor ability is also crucial for predation and predator avoidance. S. wangchiachii, a benthic omnivore, mainly consumes on anchored algae. While P. pingi, being a ferocious carnivore, predominantly preys on smaller fish. Hybrids display intermediate characteristics (Gu et al., 2019), and their inferior mouth is akin to that of S. wangchiachii, which indicates a greater adaptation for consuming anchored algae. Therefore, feeding process of P. pingi’s requires a higher degree of locomotor proficiency. In addition, S. wangchiachii inhabits in streams with low water temperatures and a scarcity of large carnivorous predators, whereas P. pingi thrives in the water environment with broader temperature variations, wider surface and greater depth, where the prevalence of large predators is higher, necessitating a more sophisticated avoidance strategy and, by extension, a greater reliance on locomotion. Thus, the smaller red blood cells of P. pingi are instrumental in meeting the energy demands associated with rapid movements during both predatory and evasive actions.
The size of vertebrate erythrocyte nuclei is positively correlated with genomic size, as a larger nucleus facilitates increased space for protein synthesis in larger cells, there by catering to the heightened metabolic demands (Gregory, 2001). Furthermore, the DNA content of the erythrocyte nucleus links to the process of hematopoiesis, which in turn affects the animal’s environmental adaptations and life-history strategies (Martins et al., 2021). A previous study showed that, the erythrocyte DNA content of S. wangchiachii, hybrid and P. pingi was 7.42 ± 0.38 pg, 5.53 ± 0.2 pg and 4.28 ± 0.25 pg, respectively (Gu et al., 2019), with P. pingi erythrocyte nucleus being the smallest among the three fish, which is also in accordance with its relatively lower DNA content.
Besides erythrocytes, the blood contains a large number of thrombocytes and leukocytes, and all of which equipped with immune or immunomodulatory functions. I. multifiliis, a parasitic protozoon, hosts on the skin and gills of fish, feeding on the host’s erythrocytes and leading to anemia, infection and even death of the host (Tavares-Dias et al., 2002; Abdel-Hafez et al., 2014). In response to I. multifiliis infection, fish exhibit different manifestations of leucocyte profiles. Specifically, infected Oncorhynchus mykiss showed a decreased percentage of lymphocytes and increased levels of neutrophils, monocytes, eosinophils, and thrombocytes (Azimzadeh, 2016). While only lymphocyte and thrombocyte counts were significantly elevated in Chub (Leuciscus cephalus) after infection (Abdel-Hafez et al., 2014). But tilapia exhibited a significant decrease of lymphocytes and increase of neutrophils and monocytes (Tavares-Dias et al., 2002). Furthermore, upon injection with I. multifiliis antigens, Carassius auratus langsdorfii showed an increase in T4 and T8 lymphocytes but a decrease in the number of macrophages and granulocytes at the injection site (Shiota et al., 2021). And Rhamdia quelen experienced an increase in the number of thrombocytes following both I. multifiliis infection and vaccination (Tancredo et al., 2015). Besides, the transcriptomic analysis of gill tissues of O. mykiss infected with I. multifiliis identified 3352 differentially expressed genes, with 268 of these genes being enriched in 16 immune-related pathways involved in chemokine signaling, platelet activation pathway, etc (Syahputra et al., 2019). These results suggest that various types of leukocytes, including thrombocytes may play crucial fish immune responses upon I. multifiliis infection.
In terms of resistance to I. multifiliis infection, a previous report has revealed that, P. pingi had the strongest resistance when compared with Culter alburnus, S. prenanti, Silurus meridionalis, and Ctenopharyngdon idella, and this was evidenced by the lowest pathogen titers, highest survival rate, and fastest resilience within the same timeframe after infection with an equivalent dose (Li et al., 2023). The hybrid offspring of S. wangchiachii and P. pingi also exhibits high resistance to I. multifiliis (Gu et al., 2023). Tilapia is also considered to have high resistance to I. multifiliis (Xu et al., 2009), with a peripheral blood thrombocyte count of 50.24×103 cells/μL, surpassing the total number of leukocytes (46.91×103 cells/μL), and approaching twice the number of lymphocytes (28.92×103 cells/μL) (Souza et al., 2020). Upon an injury, thrombocytes in fish not only congregate promptly at the injury site to engage in thrombus formation, which in turn participates in the inflammatory response, but they also express and release proteins and substances that facilitate the repair of wound (Koupenova et al., 2018). Thrombocytes in some fish have reported to possess a phagocytic function, thus they play important roles in both innate and acquired immunity processes (Stosik et al., 2002; Kollner et al., 2004; Nagasawa et al., 2014; Zhao et al., 2016; Koupenova et al., 2018). In addition, CRP is an acute phase protein synthesized by the liver, plays pivotal role in the early stages of infection by binding to injured tissues, recruiting monocytes, activating the complement system, regulating the function of thrombocytes, and triggering coagulation (Salazar et al., 2014). In the present study, the results of differential leucocytes counts showed that the percentage of thrombocytes in hybrid fish and P. pingi were significantly higher than that of S. wangchiachii (P< 0.05). The plasma CRP content in S. wangchiachii was also lowest, nearly undetectable. Thus, we hypothesized that hybrid fish possess effective coagulation, and the high proportion of thrombocytes and lymphocytes, combined with high level of plasma CRP, may confer a protective advantage against initial infection of I. multifilli.
The liver is not a center of metabolism, protein synthesis, detoxification in animals, but also an important immune and hematopoietic organ in fish. Hepatocytes, the most essential parenchymal cells of the liver, secrete bile, synthesize various important proteins such as CRP, and facilitate substance metabolism. These cells also take part in immune responses through their phagocytotic capability and expression of complement (Dini et al., 2002; Zhou et al., 2016). In addition to hepatocytes, liver-resident macrophages, also known as Kupffer cells, are a cellular taxon that maintains liver homeostasis and normal function (Hossen et al., 2022). Fish liver contains melanomacrophage centers, which contribute to immune responses. As observed by liver tissue sections, the hepatocytes size of hybrid fish were similar to erythrocytes, both being the largest of the three. However, the nuclei of hybrid fish hepatocytes were the smallest. Hepatocyte nuclear-to-cytoplasmic ratio is related to cell size, cell cycle and intracellular substance synthesis (Balachandra et al., 2022). Disruption of this ration has occurred due to deposition of lipid droplets (Loneke et al., 2023). Deduced hepatocyte nuclear-to-cytoplasmic ratio is reported to be associated with lipid metabolism in mice (Kim et al., 2023), whereas exposure to copper contamination has led to an increase in this ration in Sylvaemus uralensis (Davydova et al., 2023). TBA, synthesized by hepatocytes, mainly associates with the digestion and absorption of lipids, as well as influencing the lipid content of the fish. The supplementation of plant components to the feed of a omnivorous fish has been observed to decreases the digestion and absorption of lipids, TBA, and the total lipid content of fish (Gu et al., 2017). As mentioned above, S. wangchiachii primarily consumes benthic diatoms, whereas P. pingi are carnivorous (Gu et al., 2023). The lipid dry weight percentage of diatoms ranges from 30.2–45.1% (Chen, 2012), which is higher than the 10–25% lipid content found in most fish (Anthony et al., 2000). Meanwhile, this is reflected in the muscle lipid content, with S. wangchiachii at 12.83% (Wang et al., 2017), and P. pingi at a notably lower level of 0.93% (Liu et al., 2011). These figures align with the results in the present study, that the TBA content was significantly higher in S. wangchiachii than in P. pingi. The hybrid fish TBA content resided between its two parents offsprings, but the hepatocyte nuclear-to-cytoplasmic ratio, and HSI were the smallest, suggesting that the metabolism of hepatocytes, especially lipid metabolism in hybrid fish may be more different from its two parents.
5 Conclusions
The proportion of lymphocytes and thrombocytes in the peripheral blood, plasma contents of CRP and TBA, size of erythrocytes and hepatocytes of different fish are physiological parameters that reflect the distinct ecological traits such as disease resistance, diet preference and locomotive capabilities. However, to thoroughly understand and take advantage of the physiological biodiversity of fish, it is essential to further explore the underlying molecular mechanism that contributes to formation of these differential ecological traits.
Data availability statement
The raw data supporting the conclusions of this article will be made available by the authors, without undue reservation.
Ethics statement
The animal study was approved by The Ethical Committee of the Southwest University. The study was conducted in accordance with the local legislation and institutional requirements.
Author contributions
JeH: Formal analysis, Investigation, Methodology, Validation, Visualization, Writing – original draft, Writing – review & editing. DZ: Data curation, Formal analysis, Investigation, Methodology, Validation, Visualization, Writing – original draft. MW: Formal analysis, Investigation, Methodology, Visualization, Writing – original draft. SY: Formal analysis, Investigation, Methodology, Visualization, Writing – original draft. JjH: Formal analysis, Investigation, Visualization, Writing – original draft. XQ: Formal analysis, Investigation, Visualization, Writing – original draft. ML: Formal analysis, Investigation, Visualization, Writing – original draft. ZW: Conceptualization, Methodology, Resources, Software, Validation, Writing – review & editing. XL: Conceptualization, Data curation, Funding acquisition, Project administration, Resources, Supervision, Validation, Writing – review & editing, Writing – original draft.
Funding
The author(s) declare financial support was received for the research, authorship, and/or publication of this article. This research was supported by Natural Science Foundation of Chongqing (CSTB2022NSCQ-MSX1505).
Acknowledgments
Thanks are given to Dr. Haoran Gu and Jinfeng Shi for their assistance on the fish husbandry and sample collection. Besides, grateful acknowledgments are made to Prof. Yaoguang Zhang for his generous help in the facilities and suggestions in the experiment.
Conflict of interest
The authors declare that the research was conducted in the absence of any commercial or financial relationships that could be construed as a potential conflict of interest.
Publisher’s note
All claims expressed in this article are solely those of the authors and do not necessarily represent those of their affiliated organizations, or those of the publisher, the editors and the reviewers. Any product that may be evaluated in this article, or claim that may be made by its manufacturer, is not guaranteed or endorsed by the publisher.
References
Abdel-Hafez G., Lahnsteiner F., Mansour N., Licek E. (2014). Pathophysiology of Ichthyophthirius multifiliis infection in rainbow trout (Oncorhynchus mykiss) and chub (Leuciscus cephalus). J. Comp. Pathol. 151, 394–399. doi: 10.1016/j.jcpa.2014.08.003
Anthony J. A., Roby D. D., Turco K. R. (2000). Lipid content and energy density of forage fishes from the northern Gulf of Alaska. J. Exp. Mar. Biol. Ecol. 248, 53–78. doi: 10.1016/S0022-0981(00)00159-3
Azimzadeh K. (2016). Comparative studies of some haematological and serological indices in rainbow trout (Oncorhynchus mykiss Walbaum 1792) with ichthyophthiriasis. Turkish J. Fish. Aquat. Sci. 16, 617–627. doi: 10.4194/1303–2712-v16_3_14
Balachandra S., Sarkar S., Amodeo A. A. (2022). The nuclear-to-cytoplasmic ratio: coupling DNA content to cell size, cell cycle, and biosynthetic capacity. Annu. Rev. Genet. 56, 165–185. doi: 10.1146/annurev-genet-080320-030537
Cadotte M. W., Carscadden K., Mirotchnick N. (2011). Beyond species: functional diversity and the maintenance of ecological processes and services. J. Appl. Ecol. 48, 1079–1087. doi: 10.1111/j.1365-2664.2011.02048.x
Chen X. S., Deng S. H., Li X. M., Tan Z. L., Wan Y. F., Yao L. (2015). Biological characteristics and culture technology of Percocypris pingi. Hebei Fish. 02), 32–34. doi: 10.3969/j.issn.1004–6755.2015.02.012
Chen Y. C. (2012). The biomass and total lipid content and composition of twelve species of marine diatoms cultured under various environments. Food Chem. 131, 211–219. doi: 10.1016/j.foodchem.2011.08.062
Chen Y. X., Xiao L. Y., Yan T. M., Zhao H. T., Shen S. J., Zhou D. G. (2009). Comparative study of hematological indices in wild and farmed schizothoracine fishes. J. Aquat. Biol. 33, 905–910. doi: 10.3724/sp.j.0000.2009.50905
Davydova Y. A., Nesterkova D. V., Mukhacheva S. V. (2023). Morphological parameters of hepatocytes in the European mole (Talpa europaea) and herb field mouse (Sylvaemus uralensis) under industrial pollution: qualitative and quantitative assessment. Environ. Monit. Assess. 195, 300. doi: 10.1007/s10661-022-10810-5
Ding R. H. (1994). The fishes of Sichuan (Chengdu: Sichuan Science and Technology Publishing House), 365–367.
Dini L., Pagliara P., Carla E. C. (2002). Phagocytosis of apoptotic cells by liver: a morphological study. Microsc. Res. Tech. 57 (6), 530–540. doi: 10.1002/jemt.10107
Fazio F., Saoca C., Ferranteili V., Cammilleri G., Capillo G., Piccione G. (2019). Relationship between arsenic accumulation in tissues and hematological parameters in mullet caught in Faro Lake: a preliminary study. Environ. Sci. pollut. Res. 26, 8821–8827. doi: 10.1007/s11356-019-04343-7
Ferdous F., Scott T. (2023). The immunological capacity of thrombocytes. Int. J. Mol. Sci. 24, 12950. doi: 10.3390/ijms241612950
Gregory T. R. (2001). The bigger the C- value, the larger the cell: genome size and red blood cell size in vertebrates. Blood Cells Molecules Dis. 27, 830–843. doi: 10.1006/bcmd.2001.0457
Gu M., Bai N., Kortner T. M. (2017). Taurocholate supplementation attenuates the changes in growth performance, feed utilization, lipid digestion, liver abnormality and sterol metabolism in turbot (Scophthalmus maximus) fed high level of plant protein. Aquaculture 468, 597–604. doi: 10.1016/j.aquaculture.2016.11.022
Gu H. R., Wan Y. F., Yang Y., Ao Q., Chen W. L., Deng S. H., et al. (2019). Genetic and morphology analysis among the pentaploid F1 hybrid fishes (Schizothorax wangchiachii ♀ × Percocypris pingi ♂) and their parents. Animal 13, 2755–2764. doi: 10.1017/S1751731119001289
Gu H. R., Wang H. Y., Deng S. H., Dai X. Y., He X. F., Wang Z. J. (2023). Hybridization with Percocypris pingi male can improve the cultivation characteristics of snow trout (Schizothorax wangchiachii), especially the resistance to white spot disease (Ichthyophthirius multifiliis). Aquaculture 562, 738805. doi: 10.1016/j.aquaculture.2022.738805
Harikrishnan R., Rani M. N., Balasundaram C. (2003). Hematological and biochemical parameters in common carp, Cyprinus carpio, following herbal treatment for Aeromonas hydrophila infection. Aquaculture 221, 41–50. doi: 10.1016/S0044-8486(03)00023-1
Hossen M. J., Matin M. A., Sikder M. H., Ahmed M. S., Rahman M. (2022). "Kupffer cells and liver", Recent Advacements in Microbial Diversity, Macrophages and Their Role in Inflammation, ed. Cho JY, (Academic Press), 361–395. doi: 10.1016/B978–0-12–822368–0.00016–5
Kim K. E., Lee J., Shin H. J., Jeong E. A., Jiang H. M., Ahn Y. J., et al. (2023). Lipocalin-2 activates hepatic stellate cells and promotes nonalcoholic steatohepatitis in high-fat diet–fed Ob/Ob mice. Hepatology 77, p888–p901. doi: 10.1002/hep.32569
Kollner B., Fischer U., Rombout J., Taverne-Thiele J. J., Hansen J. D. (2004). Potential involvement of rainbow trout thrombocytes in immune functions: a study using a panel of monoclonal antibodies and RT-PCR. Dev. Comp. Immunol. 28, 1049–1062. doi: 10.1016/j.dci.2004.03.005
Koupenova M., Clancy L., Corkrey H A., Corkrey H. A., Freedman J. E. (2018). Circulating platelets as mediators of immunity, inflammation, and thrombosis. Circ. Res. 122, 337–351. doi: 10.1161/CIRCRESAHA.117.310795
Lay P. A., Baldwin J. (1999). What determines the size of teleost erythrocytes? Correlations with oxygen transport and nuclear volume. Fish Physiol. Biochem. 20, 31–35. doi: 10.1023/A:1007785202280
Li G. H., Leng Y., Wu J. D., Liu Y. T., Li S. H., Yang S. L., et al. (2014). Research on large-scale artificial propagation technology of Schizothorax wangchiachii. Modern Agric. Sci. Technol. 10), 259–261 + 270.
Li L. Z., Li M. H., Zegn R. K., Deng L. J., Yang K., Song Z. B. (2023). High resistance to Ichthyophthirius multifiliis in Percocypris pingi, an endemic fish in upper Yangtze River. Aquaculture 562, 738767. doi: 10.1016/j.aquaculture.2022.738767
Liu B. S., Li J. G., Li Z. Y., Zhan H. X., Zhang L. T., Huang H. (2011). Fish rate, muscle nutrition compostion and quality of Percocypris pingi pangi. Guizhou Agric. Sci. 39, 166–170.
Liu X. H., Pang X., Jin L., Pu D. Y., Wang Z. J., Zhang Y. G. (2023). Exposure to acute waterborne cadmium caused severe damage on lipid metabolism of freshwater fish, revealed by nuclear lipid droplet deposition in hepatocytes of rare minnow. Aquat. Toxicol. 257, 106433. doi: 10.1016/j.aquatox.2023.106433
Loneke A. E., Alisafaei F., Kant A., Li D., Janmey P. A., Shenoy V. B., et al. (2023). Lipid droplets are intracellular mechanical stressors that impair hepatocyte function. Proc. Natl. Acad. Sci. United States America 120, e2216811120. doi: 10.1073/pnas.2216811120
Lu Y., Li M. H., Gan W. X., Zeng R. K., Song Z. B. (2019). Effects of exercise training and suspension on swimming ability of juvenile Percocypris pingi. Sichuan Zool. 38, 361–367. doi: 10.11984/j.issn.1000–7083.20190057
Lu Y., Wu H., Deng L. J., Li T. C., Yang K., Fu S. J., et al (2020). Improved aerobic and anaerobic swimming performance after exercise training and detraining in Schizothorax wangchiachii: Implications for fisheries releases. Comp. Biochem. Physiol. A: Mol. Integr. Physiol., 245, 110698. doi: 10.1016/j.cbpa.2020.110698
Martins B. O., Franco-Belussi L., Siqueira M. S., Fernandes C. E., Provete D. B. (2021). The evolution of red blood cell shape in fishes. J. Evolution. Biol. 34, 537–548. doi: 10.1111/jeb.13757
Nagasawa T., Nakayasu C., Rieger A. M., Barreda D. R., Somamoto T., Nakao M. (2014). Phagocytosis by thrombocytes is a conserved innate immune mechanism in lower vertebrates. Front. Immunol. 5. doi: 10.3389/fimmu.2014.00445
Ortega-Villaizan M. D. M., Coll J., Rimstad E. (2022). The role of red blood cells in the immune response of fish. Front. Immunol. 13. doi: 10.3389/fimmu.2022.1005546
Puente-Marin S., Thwaite R., Mercado L., Coll J., Roher N., Ortega-Villaizan M. D. M. (2019). Fish red blood cells modulate immune genes in response to bacterial inclusion bodies made of TNFα and a G-VHSV fragment. Front. Immunol. 10. doi: 10.3389/fimmu.2019.01055
Salazar J., Martínez M. S., Chávez-Castillo M., Núñez V., Añez R., Torres Y., et al. (2014). C-reactive protein: an in-depth look into structure, function, and regulation. Int. Scholar. Res. Notices 2014, 653045. doi: 10.1155/2014/653045
Schulte P. M., Healy T. M. (2022). Physiological diversity and its importance for fish conservation and management in the Anthropocene. Fish Physiol. 39, 435–477. doi: 10.1016/bs.fp.2022.04.009
Shen Y. W., Wang D., Zhao J. L., Chen X. W. (2018). Fish red blood cells express immune genes and responses. Aquacult. Fish. 3, 14–21. doi: 10.1016/j.aaf.2018.01.001
Shi J., Zhuo D., Lu M., Wang H., Gu H., Liu X., et al. (2023). Partial immune responses in Sichuan bream (Sinibrama teaniatus) after starvation. Front. Immunol. 14. doi: 10.3389/fimmu.2023.1098741
Shiota K., Sukeda M., Prakash H., Kondo M., Nakanishi T., Nagasawa T., et al. (2021). Local immune responses to two stages of Ichthyophthirius multifiliis in ginbuna crucian carp. Fish Shellf. Immunol. 118, 19–24. doi: 10.1016/j.fsi.2021.08.013
Snyder G. K., Sheafor B. A. (1999). Red blood cells: Centerpiece in the evolution of the vertebrate circulatory system. Am. Zool. 39, 189–198. doi: 10.1093/icb/39.2.189
Souza F., Lima E., Urrea-Rojas A. M., Suphoronski S. A., Facimoto C. T., Bezerra Júnior J., et al. (2020). Effects of dietary supplementationwith a microalga (Schizochytrium sp.) on thehemato-immunological, and intestinal histologicalparameters and gut microbiota of Nile tilapia in netcages. PloS One 15, e0226977. doi: 10.1371/journal.pone.0226977
Stosik M. P., Deptula W., Travnicek M., Baldy-Chudzik K. (2002). Phagocytic and bactericidal activity of blood thrombocytes in carps (Cyprinus carpio). Veterinarni Medicina 47, 21–25. doi: 10.17221/5798-VETMED
Sun Z., Tan X., Liu Q., Ye H. Q., Zou C. Y., Xu M. L., et al. (2019). Physiological, immune responses and liver lipid metabolism of orange-spotted grouper (Epinephelus coioides) under cold stress. Aquaculture 498, 545–555. doi: 10.1016/j.aquaculture.2018.08.051
Syahputra K., Kania P. W., Al-Jubury A., Jafaar R. M., Dirks R. P., Buchmann K. (2019). Transcriptomic analysis of immunity in rainbow trout (Oncorhynchus mykiss) gillsinfected by Ichthyophthirius multifiliis. Fish Shellf. Immunol. 86, 486–496. doi: 10.1016/j.fsi.2018.11.075
Tancredo K. R., Goncalves E. T., Brum A., AcChile M., Hashimoto G. S. O., Pereira S. A., et al. (2015). Hemato-immunological and biochemical parameters of silver catfish Rhamdia quelen immunized with live theronts of Ichthyophthirius multifiliis. Fish Shellf. Immunol. 45, 689–694. doi: 10.1016/j.fsi.2015.05.024
Tavares-Dias M., de Moraes F. R., Martins M. L., Santana A. E. (2002). Haematological changes in Oreochromis niloticus (Osteichthyes: Cichlidae) with gill ichthyophthiriasis and saprolegniosis Vol. 28 (Sao Paulo: Boletim do Instituto da Pesca), 1–9.
Wang H. Y., Gu H. R., Wang Z. J. (2022). Hybrids with heterosis possess a more diverse intestinal microbiome than that of carnivorous and herbivorous parental fishes in controlled conditions. Aquacult. Rep. 30, 101581. doi: 10.1016/j.aqrep.2023.101581
Wang C., Liang Y. Q., Zhang Y., Shen Z. J., Jiang Z. W. (2017). Nutrient content and quality of Schizothorax wangchiachii. J. Hydroecol. 38, 96–100. doi: 10.15928/j.1674–3075.2017.04.014
Witeska M. (2013). Erythrocytes in teleost fishes:a review. Zool. Ecol. 23, 275–281. doi: 10.1080/21658005.2013.846963
Xu D. H., Shoemaker C. A., Klesius P. H. (2009). Enhanced mortality in Nile tilapia Oreochromis niloticus following coinfections with ichthyophthiriasis and streptococcosis. Dis. Aquat. Organ. 85, 187–192. doi: 10.3354/dao02073
Yang Y., Chen Y., Wan Y. F., Deng S. H., He X. F., Wang Z. J., et al. (2018). Observations on the development of embryos and littermates in the F1 generation of the cross between Schizothorax wangchiachii and Percocypris pingi. South. Aquat. Sci. 14, 66–73. doi: 10.12131/20180075
Zhao L. L., Chen Q. L., Yang S., Li S. J., Wang Y., Du Z. J. (2016). Morphological characteristics and phagocytosis of hemocytes in Micropterus salmoides L, Superior Perch 1. South. Aquat. Sci. 12, 43–50. doi: 10.3969/j.issn.2095–0780.2016.03.006
Keywords: biochemical indices, differential leucocytes counts, distant hybridization, erythrocyte morphology, shape of hepatocytes
Citation: Huang J, Zhuo D, Wang M, Yeerda S, Huang J, Qin X, Lu M, Wang Z and Liu X (2024) Comparative study on the blood physiological, biochemical indexes and liver histology of Schizothorax wangchiachii (♀), Percocypris pingi (♂) and their hybrids. Front. Mar. Sci. 11:1413965. doi: 10.3389/fmars.2024.1413965
Received: 08 April 2024; Accepted: 10 June 2024;
Published: 27 June 2024.
Edited by:
Vikash Kumar, Central Inland Fisheries Research Institute (ICAR), IndiaReviewed by:
Sofia Priyadarsani Das, National Taiwan Ocean University, TaiwanSoumya Prasad Panda, Central Inland Fisheries Research Institute (ICAR), India
Copyright © 2024 Huang, Zhuo, Wang, Yeerda, Huang, Qin, Lu, Wang and Liu. This is an open-access article distributed under the terms of the Creative Commons Attribution License (CC BY). The use, distribution or reproduction in other forums is permitted, provided the original author(s) and the copyright owner(s) are credited and that the original publication in this journal is cited, in accordance with accepted academic practice. No use, distribution or reproduction is permitted which does not comply with these terms.
*Correspondence: Xiaohong Liu, hongye-lxh@163.com