- 1Coastal Survey Solutions LLC, Lajas, Puerto Rico
- 2Department of Biological Sciences, University of Rhode Island, Kingston, RI, United States
- 3Department of Natural Resources Sciences, University of Rhode Island, Kingston, RI, United States
The queen snapper, Etelis oculatus, is experiencing a growing fishery presence across the wider Caribbean, yet our understanding of its distribution, biology, and ecology remains limited. To address this gap, we investigated the feeding ecology of this demersal deep-water snapper using two molecular approaches: single-species barcoding and multispecies metabarcoding. Between November 2019 and July 2020, we collected 157 queen snapper from seven locations along the west coast of Puerto Rico. Our analysis identified a diverse array of prey items in the stomachs, comprising 61 species from 31 families, 18 orders, and 38 genera. Our findings suggest that E. oculatus is a large carnivore, primarily preying on squids, shrimps, and deep-water fishes. Notably, common fish prey included Diaphus brachycephalus, D. dumerilii, Dasyscopelus selenops, Coccorella atlantica, Sigmops elongatus, and Zaphotias pedaliotus. In contrast, prevalent invertebrate prey consisted of Abralia veranyi, Doryteuthis pealeii, Abralia redfieldi, Oplophorus gracilirostris, and Systellaspis debilis. Although our data suggest potential variation in diet composition across locations, definitive conclusions remain elusive. However, we observed heightened prey richness at seamount Pichincho, possibly attributable to the high structural complexity of the karst terrain on the seafloor. Additionally, we noted variation in prey species composition across size classes, with certain species more prevalent in larger or smaller queen snapper. Given the challenge of examining the prey of deep-water fish species, our study showcases the utility of both single-species barcoding and multispecies metabarcoding methodologies in characterizing the dietary range of queen snapper and similar demersal deep-water carnivorous fishes. The multispecies metabarcoding approach, in particular, offers a rapid, comprehensive, and effective means of identifying prey species. As the commercial value of the queen snapper continues to rise, our investigation into its feeding behavior provides critical baseline information for future species management efforts. By enhancing our understanding of its ecological dynamics, we aim to contribute to informed conservation and sustainable fisheries practices in the region.
Introduction
Commercial fisheries provide many local US Caribbean populations with a vital source of employment and sustenance. Like many other Caribbean countries, commercial fishing in Puerto Rico and the US Virgin Islands is artisanal. It occurs mainly on the insular shelf with small boats. The catch consists primarily of shellfish (lobster– Panilirus argus), conch (Aliger gigas), and finfish, with snappers and groupers being the most essential finfish landed by weight. From 2007 to 2011, Lutjanus vivanus (silk snapper) and Etelis oculatus (queen snapper) were the most landed species (Matos-Caraballo, 2012). Many snappers are caught by recreational and commercial fishers on the west and east coasts in waters deeper than 200m.
Over the years, L. vivanus was the main target for deep-water fisheries in Puerto Rico. However, changes in fishing techniques and gear types, management initiatives, and seasonal closures of L. vivanus have led to revenue growth for E. oculatus landings. E. oculatus fishery has become an increasingly important fishery throughout Puerto Rico. Matos-Caraballo (2012) reported E. oculatus commercial landings reached 174,969 lbs in 2011, valuing around $750,617, representing 13% of the entire fishery landings that year. Even though E. oculatus is a vital component essential to Puerto Rico’s commercial fishing industry (CMFC, 2016); little is known –with some recent exceptions– about this species’ biology and ecology, such as life history (Overly and Shervette, 2023), habitat preferences (Overly and Lecours, 2024), or prey diversity. The lack of available information has made it challenging to manage this species.
Queen snapper are members of the family Lutjanidae, one of the largest fish families in the Caribbean. The distribution of E. oculatus is extensive in the western Atlantic, from North Carolina to eastern Brazil, and is also found in the Gulf of Mexico. E. oculatus is one of the deepest-dwelling snapper species, contributing to the lack of information about this species. The depth range of E. oculatus was previously reported to range from 130 to 450 m (Allen, 1985). In the most recent NOAA Okeanos Expedition in 2018, scientists observed E. oculatus as deep as 539m, and a fisher in Guadeloupe reported catching E. oculatus in depths from 100 to 550 m (Gobert et al., 2005).
Observing recruits and juveniles of the queen snapper in the wild has been challenging due to the technical difficulties following or collecting small (< 2 cm) individuals. Similar to L. vivanus (Boardman and Weiler, 1980; South Atlantic Fishery Management Council, 2005), it is uncertain if E. oculatus displays a depth and ontogenetic relationship, where recruits and juveniles are found at shallower depths and adults move to deeper habitats (Overly and Shervette, 2023). Individuals smaller than 45- 50 cm have been caught by fishers near the shore or at the shelf edge in Roatán, Honduras (Gobert et al., 2005) and in the Straits of Florida as deep as 100m (D’Alessandro et al., 2010). Juveniles have been sighted close to the shelf in less than 30 m (Appeldoorn et al., 1987), and observed at mesophotic depths (59m) of the southeastern United States (Cuellar et al., 1996).
Most of the research on E. oculatus has occurred along the west coast of Puerto Rico and has focused on describing the benthic habitat at snapper fishing sites (García-Sais, 2015; Garcia-Sais et al., 2018). However, E. oculatus distribution around Puerto Rico is extensive (Wagner et al., 2018). E. oculatus was observed at six of the 19 sites during the Okeanos Expedition, spanning the bathymetry island-wide. Most of the sites with E. oculatus observations were along the west coast. However, queen snapper were also observed in Ponce and south of St. Croix, US Virgin Islands. Fishers catch E. oculatus with weighted vertical longlines.
High-relief submerged reefs, like the Mona Passage and Desecheo Ridge, are targeted by fishers because they are the main habitats of the E. oculatus (Tonioli and Agar, 2011). Garcia-Sais et al. (2018) AUV study, which characterized the habitat and benthic composition of targeted queen snapper sites, found the density and relative composition of sessile-benthic organisms to be highly varied along the west coast of Puerto Rico. Submerged areas with substrate discontinuities and high topographic relief were hotspots of increased abundance of E. oculatus (Garcia-Sais et al., 2018; Wagner et al., 2018). Many areas in the Mona Passage were characterized by a high on coral cover (43%). Like their shallow-water counterparts, high topographic relief areas of deep-sea habitats enhance benthic productivity, microhabitat availability, and ecosystem biodiversity (Garcia-Sais et al., 2018).
Understanding the potential prey in these areas of high productivity and how E. oculatus feeds in different environments is crucial to generating management plans. Developing robust and efficient methods to study a species’ diet is an area of continuous research and growing interest for managers (Thuo et al., 2019). Traditionally, prey were identified through morphological identification of the stomach contents found in stomachs, guts, or feces of the species of interest, in some cases using compound microscopes (De Sousa et al., 2019). While we have learned a substantial amount utilizing this approach, identifications through morphological characteristics require taxonomic expertise of various groups (i.e., fishes, crustaceans, mollusks, etc.), which is difficult to obtain through visual inspection alone. Having only undigested parts of individuals often results in misidentifying prey, poor resolution, and inconclusive results. In addition, soft-bodied taxa, such as some mollusks or polychaetes, digest quite fast, leaving only small traces in the stomachs of predators (Randall, 1967). As such, DNA amplicon-based approaches have complemented traditional methods to understand prey diversity, especially of species living in habitats that are challenging to monitor, such as highly mobile deep-water fishes. DNA-based analyses to explore the breadth of dietary items have emerged as a valuable tool in ecological research (Shokralla et al., 2012). This methodology enables the analysis of taxon-specific variants of standardized genomic regions, also known as DNA barcodes amplified from DNA mixtures using universal PCR primers. Barcoding and metabarcoding are two predominant approaches for identifying prey in stomach contents. Barcoding is typically utilized when intact tissue is present in the stomach. Each tissue item is individually removed, washed, and subjected to DNA extraction and identification, often employing conventional PCR and Sanger sequencing methods. This method yields high confidence in species presence and prey identification, yet it may overlook fast-digesting prey due to the requirement for undigested tissue.
Conversely, digested prey DNA persists longer in predator’s stomachs, prompting the application of a multispecies approach known as metabarcoding. This method enables the simultaneous identification of prey from DNA mixtures, a common occurrence in the stomach contents of most species. In the metabarcoding approach, DNA is extracted from these mixtures, followed by amplifying a molecular marker (e.g., 12S for fishes) and sequencing using parallel sequencing platforms (e.g., Illumina sequencing). Subsequently, potential prey are identified bioinformatically using curated databases as long as the sequence is present in the database. This DNA-based approach significantly enhances prey identification (Thuo et al., 2019).
In our study, we employed both single-species barcoding and multispecies 12S metabarcoding to identify prey species in the stomach contents of E. oculatus. Furthermore, we investigated differences in prey composition across size ranges and fishing locations of E. oculatus. This comprehensive identification of prey items in deep-water snappers offers a novel perspective and serves as a promising avenue for understanding the feeding ecology of these commercially valuable species.
Materials and methods
Sample collection
We collected the stomachs of queen snapper from fishes landed by commercial fishers on the west coast of Puerto Rico between November 2019 and July 2020. Therefore, this study did not need to be reviewed or approved by an ethics committee because it was a byproduct of the fishing industry. The fishers recorded the name, general geographical location, and depth of the site or area. Given that this species is in high demand and the fishing area is sensitive, the fishers did not report the exact geographical location. Therefore, we only report the name of the area and not the geographical coordinates. See Figure 1 for the general area of specimen collections.
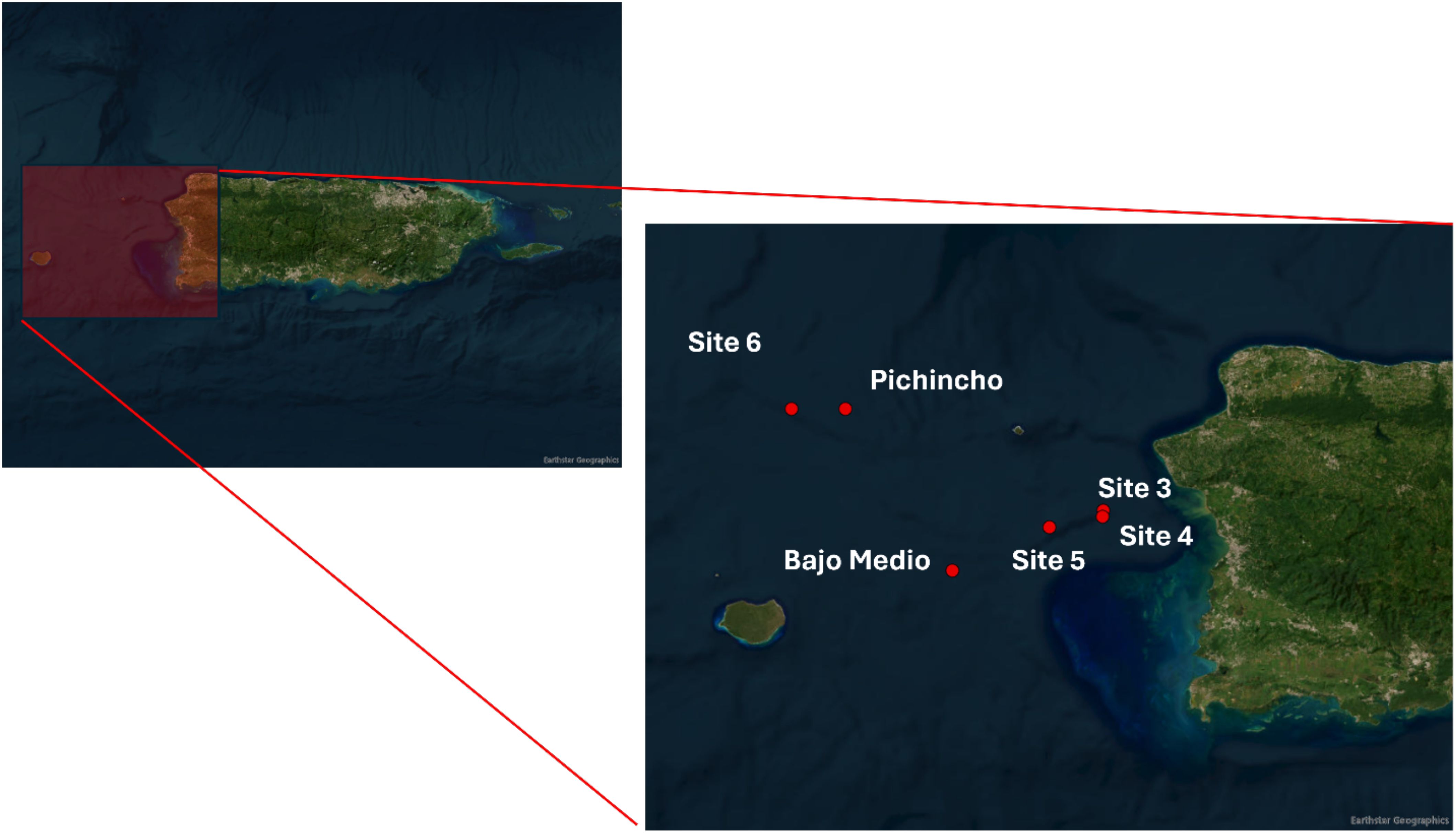
Figure 1. Map with the sampling locations of Etelis oculatus in the west of Puerto Rico. Map source ESRI ArcGIS online Pro 2024.
We stored the queen snapper in coolers on ice until processed. Most fish were processed within 24 hours. Queen snapper specimens were uniquely labeled with a code and photographed next to a metric ruler (Figure 2). We weighed each fish to the nearest gram (g), except for fish caught South of Pichincho because there was a malfunction in the scale. Total, fork and standard length were recorded for each fish to the nearest millimeter (mm). We removed stomachs and any remaining prey items in the mouth and placed them in a sterile Whirl-pack bag. Whirl-pack bags were stored immediately in a freezer at -20°C. We cleaned knives, tweezers, and processing tables with water and a 10% bleach solution before and after collecting each stomach sample. We also disposed of gloves after each sample to avoid cross-contamination between samples.
Stomach analysis
To identify potential prey in the samples, we thawed the stomachs of each fish and placed them on a disinfected laboratory surface. Using sterile gloves and autoclaved blades, tweezers, and spatulas, we dissected each fish’s stomach. To minimize any potential cross contamination across samples, we cleaned the area using a bleach solution and used autoclaved new utensils and sterile new gloves before and after processing each fish stomach. We observed both undigested pieces and digested liquid material throughout the gastrointestinal tract. All tissue pieces were individually separated, cleaned with deionized water (DI) water and preserved in 2 mL sterile tubes, and the digested liquid material was also preserved in a 2 mL tube. Thus, from each fish, we collected various 2 mL tubes, one for each potential prey, and a single 2 mL tube with the digested liquid material. Once stomach contents were cleaned and placed in 2 mL tubes, these tubes were stored in -80°C. Because individual muscle pieces should come from single individual prey, we implemented a traditional single species barcoding using standard PCR procedures and a regular Sanger sequencing approach. The liquid digested material, however, could potentially come from a mixture of species that are potential prey, and thus, we implemented a multi-species metabarcoding approach.
We extracted the genomic DNA from the muscle tissue of each prey following the DNeasy Kit protocol (QIAGEN, Canada) to identify the prey species in individual undigested items (Figure 3) using a single species barcoding approach. Each prey item was genotyped for the mitochondrial protein-coding gene cytochrome oxidase I (COI) using two primer sets, one for fish (Baldwin et al., 2009) and one for invertebrates (Folmer et al., 1994) (see Table 1). To amplify the DNA in each sample, we used a 4000 thermal cycler (Bio-Rad, USA) for PCR amplifications following standard PCR conditions with 35 amplification cycles and an annealing temperature of 50–52°C. A cycle-sequencing reaction was produced in both directions to add fluorescent labels and analyzed on an ABI 3130xl (Applied Biosystems, USA) using the amplification primers. Sequences were assembled, edited, and aligned using Geneious R8 8.1.4 (USA) (Kearse et al., 2012). Each COI sequence was manually blasted on the NCBI website using the Blastx algorithm, and sequence similarity was used to identify each prey species.
To identify the prey species in the digested liquid material using a multispecies metabarcoding approach, we used an autoclaved spatula to homogenize the liquid in the 2 mL tube. We then extracted the DNA from the digested liquid using a Macherely Nagil DNA extraction kit. After DNA was extracted, we targeted the mitochondrial 12S gene using the TELEO2 primers with Illumina overhangs (Taberlet et al., 2018; Table 1). Standard PCR conditions were followed with 35 amplification cycles and an annealing temperature of 54°C. We performed three individual PCR replicates for each sample and then combined the replicates. We then performed a second PCR using the Illumina overhangs to add barcodes to each sample using the Nextera XT Index system (Illumina, USA). After barcoding all samples from all fish stomachs, we pooled the samples together and sequenced them in one 2 X 300 bp Illumina MiSeq run using the V3 Illumina kit (Illumina, USA). In addition to processing the samples, we also included negative controls across all steps, from extraction to DNA sequencing in bhk'set of DNA extractions and PCRs to capture and minimize any potential contamination across the process.
We demultiplexed reads from the sequencing center using the Anacapa Toolkit (Curd et al., 2019) with the default parameters and the Anacapa 2020 Vert12S reference database. To identify species from each metabarcoding sample. Anacapa incorporates the Cutadapt (v. 1.9.1) (Martin, 2011) and DADA2 algorithms (Callahan et al., 2016) to denoise and perform error corrections on the raw sequence data, merge paired-end reads, and assign high-quality reads to Amplicon Sequence Variants (ASVs). We quality-filtered reads (minimum quality = 35), sorted them, and assigned them to ASVs. Within DADA2, we removed reads shorter than 100 bases, and reads with more than 10 consecutive errors. In the final step of the pipeline, Anacapa used Bowtie 2 (Langmead and Salzberg, 2012) and the Bayesian Lowest Common Ancestor (BLCA) algorithm to assign ASVs to taxa, using a default likelihood threshold value as suggested by Gao et al. (2017). This approach produced taxonomic classification summaries with a Bayesian Confidence Cutoff, following Curd et al. (2019). We chose the 95% cutoff because it is an ideal trade-off between capturing a large portion of identified species while maintaining high taxonomic accuracy. The result was an ASV presence-absence matrix and a taxonomy file with the unique ASV IDs and associated taxonomy. We imported these files and sample metadata information into the R packages Ranacapa and Phyloseq for downstream analyses and visualization (R Core Team, 2017). We manually confirmed all unique sequences by blasting each sequence to the general NCBI database (Available from: https://www.ncbi.nlm.nih.gov/).
Statistical analysis
Given the unbalanced nature of the data, four one-way distance Permutational Multivariate Analyses of Variance (PERMANOVA) tests (Anderson, 2001) were performed to examine the difference in the fork length and weight between sample sites and sampling time. The similarity matrix was based on Euclidean distances since the fork length and weight are univariate measures. Euclidean distance measures for univariate PERMANOVA analyses produce sums-of-squares estimates equivalent to parametric ANOVA (Anderson, 2001) and allow the same methodological framework to be used for all community attributes.
One-way PERMANOVAs were performed to examine the distribution of prey items across locations and sizes. Species composition (presence/absence) was calculated with the Jaccard similarity index, which considers only the presence and absence of each species in each sample and allows comparisons of the proportion of species between locations (Anderson, 2001). P -values were obtained using 9999 permutations of residuals under a reduced model for all PERMANVOA analyses. Similarity percentage tests (SIMPER) were also performed to identify which prey species contributed to the difference in the factors (locations, sex, and size, Anderson, 2001). SIMPERs were also performed in PRIMER-E software.
Results
Sample demography
A total of 157 queen snapper were collected from November 2019 to July 2020 across seven locations (Table 2), ranging in depths from 256 m to 402 m (Table 1). Most fishers targeted Bajo Medio (55 samples) and Pichincho (29 samples) (Figure 1). The size and weight of queen snapper collected during this study varied between the date fishes were caught and location. However, from the estimates of the components of variation, sampling time had a more significant effect on size and weight than location.
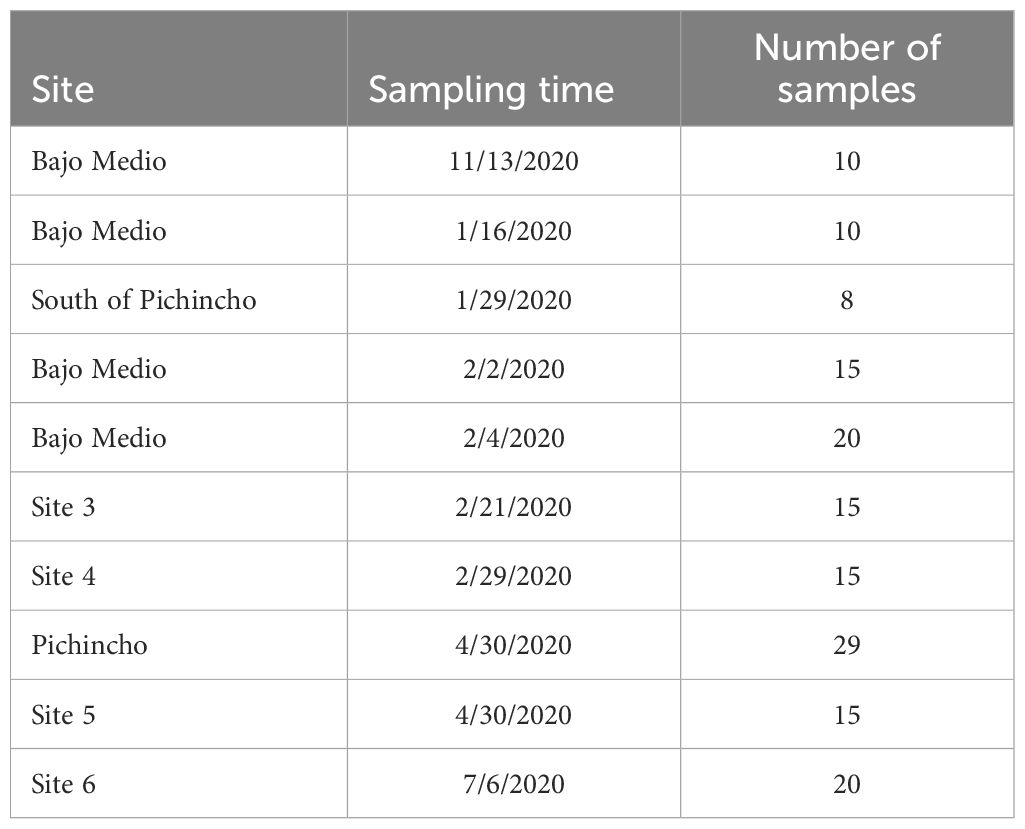
Table 2. The number of Etelis oculatus samples collected at each site and sampling time along the west coast of Puerto Rico.
The mean (± SE) standard, fork, and total length of queen snapper were 443 ± 12 mm, 472 ± 12 mm, and 595 ± 15 mm, respectively. The standard size ranged from 190 mm to 756 mm, fork length ranged from 220 mm to 808 mm, and total length ranged from 260 mm to 970 mm. The size distribution was skewed slightly towards larger fish (Figure 4). More fish were sampled with a fork length between 280–300 mm and 520–540 mm. The fork length size significantly varied between sampling time and locations (Table 3). Smaller fish were caught at Site 4 and Site 3, while larger fish were landed at Site 6 and South of Pichincho. Fishers landed the largest fish in July and the smallest fish in November.
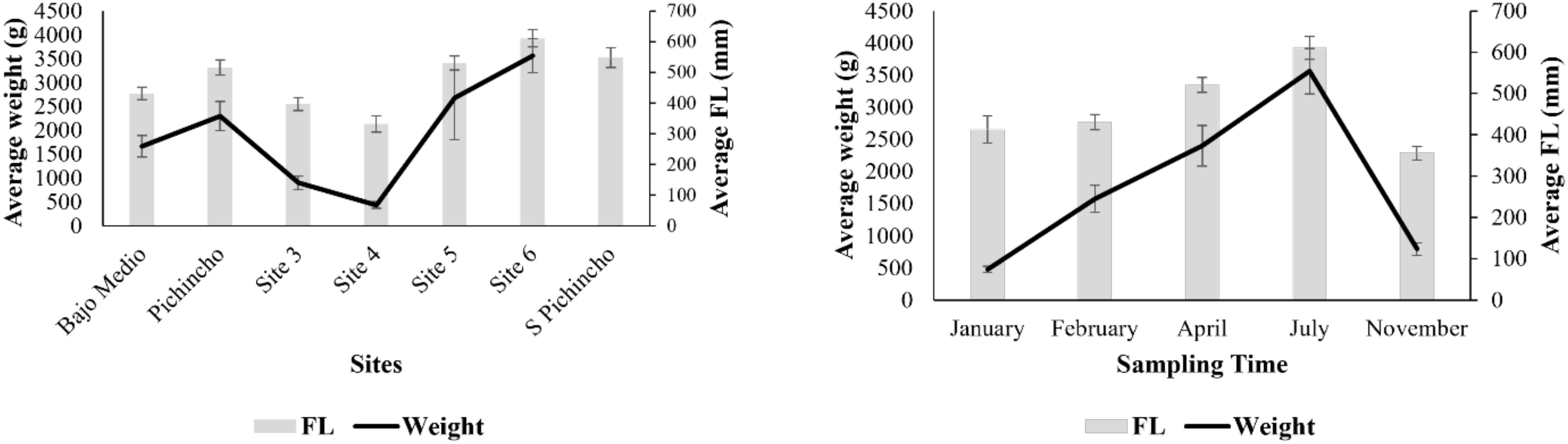
Figure 4. Average weight (g) and fork length (mm) of Etelis oculatus landed at the locations (left) and different sampling times (right) in the western side of Puerto Rico. Bars denote standard errors.

Table 3. Permutational Multivariate ANOVA results of the difference of fork length (mm) and weight (g) of the queen snappers between sites and sampling times for the west coast of Puerto Rico.
The mean (± SE) weight of the queen snapper was 1,948 ± 144 g, ranging from 195 g to 10,568 g. Given the size differences across sites, it was unsurprising that fish’s weight also significantly varied across locations (Table 3). Weight variation within the site was more extreme than length variation, especially at Site 5. For sampling time, mean fish weight was the lowest during January, followed by November. The highest weights were observed during July (Figure 4).
Stomach analyses
A total of 61 species belonging to 31 families, 18 orders, and 38 genera were observed in the stomach contents of queen snapper using the two molecular approaches (Table 4). All species found in the stomachs were reported, which includes those most commonly used as baits, such as skipjacks (Katsuwonus pelamis), little tunny (Euthynnus alletteratus), blackfin tunas (Thunnus atlanticus), herring (Opisthonema oglinum), bonefish (Albula vulpes), and squids (N. Crespo pers comm). Most prey species were fish; however, five squid species were found, including the midwater squid (Abralia veranyi), longfin inshore squid (Doryteuthis sp. (possible pealeii)), wonderful firefly squid (Lampadioteuthis megaleia), and Redfield’s enope squid (Abralia redfieldi), and also two species of shrimps Oplophorus gracilirostris, and Systellaspis debilis (Table 4).
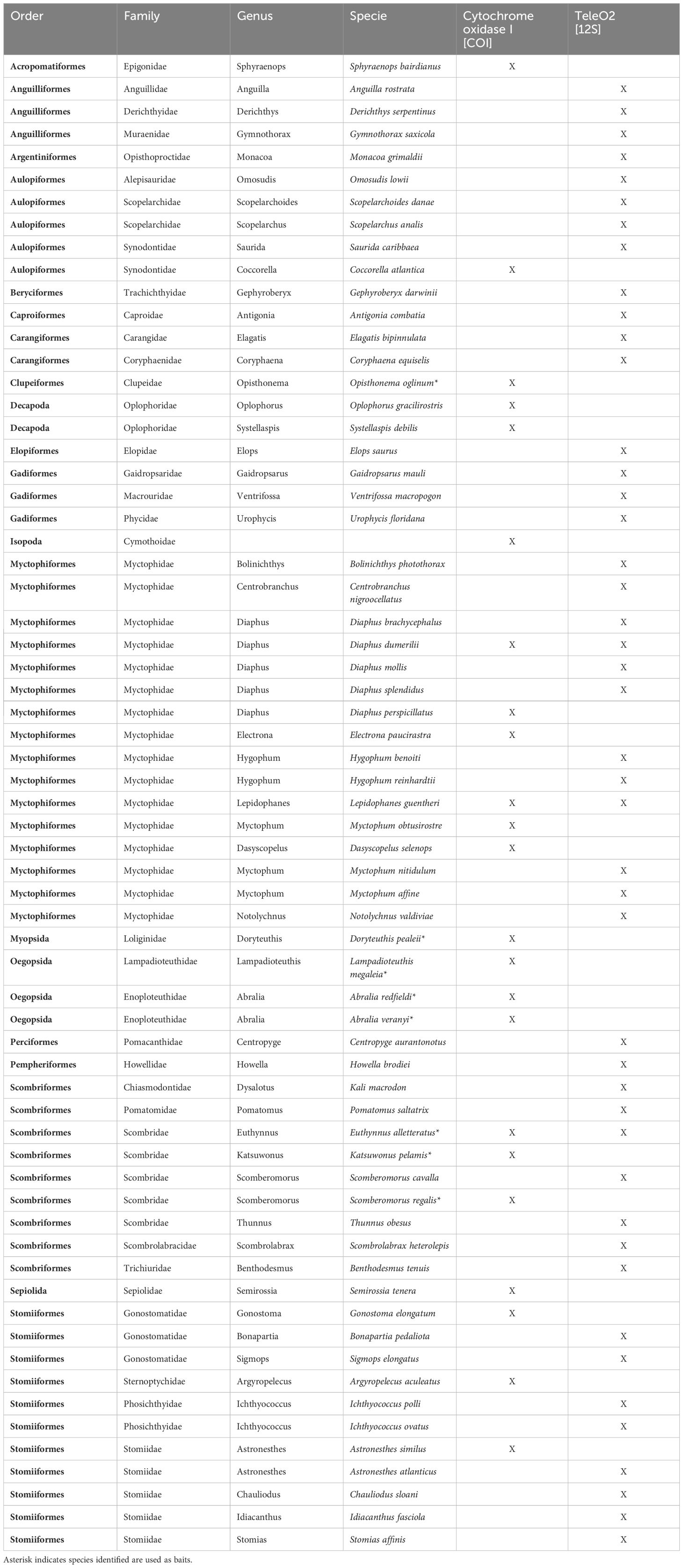
Table 4. Prey species of Etelis oculatus using two molecular approaches, single species Cytochrome oxidase I (COI) barcoding and multispecies metabarcoding (Tele02).
The species of prey and their quantities varied between molecular approaches. Out of the 157 fish collected, 144 had a stomach, 36 stomachs had undigested items (25% of the captures), and two stomachs were fully empty and no longer processed (2.7%). A total of 144 fish stomachs were processed using the 12S metabarcoding approach. Thirty-six of them were also processed using the COI barcoding approach. Seven species of invertebrates (two arthropods, four mollusks, and one isopod) and 16 fish species were identified within those undigested items using the COI barcoding approach. A total of 45 prey species were detected. The total number of species using both approaches was 67 (45 with the 12S metabarcoding and 22 with the COI barcoding) across 34 genera, and 45 families (some samples were only identified to family level). Diaphus dumerilii, E. alletteratus, Lepidophanes guentheri detected with both approaches (Table 4).
Myctophiformes was the most common order among the identified prey (59 stomach samples), with 16 species recognized within the family Myctophidae. Stomiiformes were the second most common order observed, represented by eleven species. Prey from the families Gonostomatidae and Stomiidae were also common and identified in 34 and 17 stomach samples, respectively. The most common prey was the Diaphus brachycephalus, the short-headed lantern fish, which was observed in 25 stomach samples, followed by Sigmops elongatus in 23 and Zaphotias pedaliota in 19 (Figure 5). The two other common species, S. elongatus, elongated bristlemouth fish, and Z. pedaliota, longgray fangjaw were common in stomach samples.
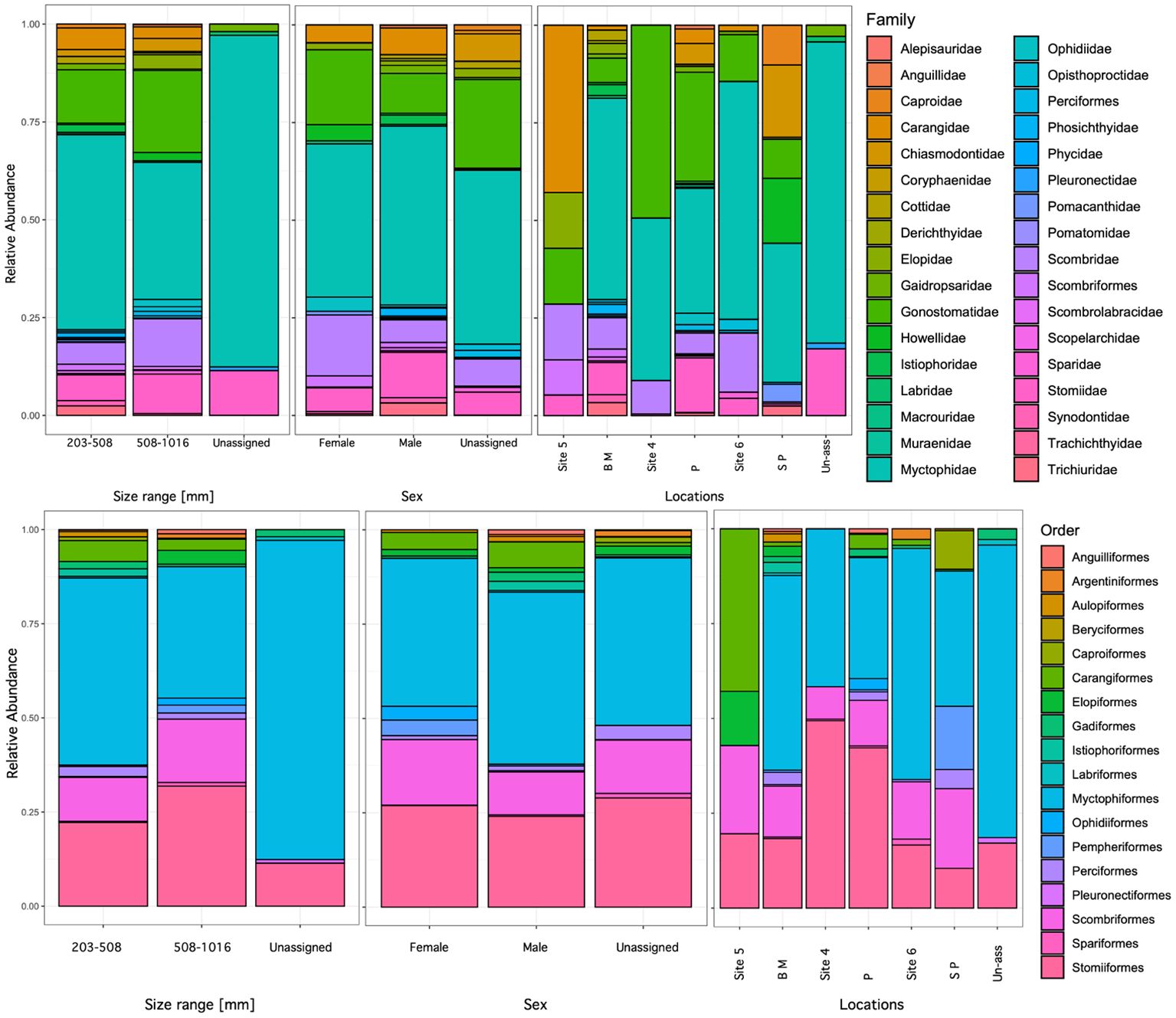
Figure 5. Relative abundance of species in stomach samples of Etelis oculatus in Puerto Rico, order level in top panel and family level in the bottom panel. Locations with abbreviations: B M (Bajo Medio), P (Pinchinchos), and S P (South of Pichinchos).
Prey composition significantly differed among locations (Table 5); however, these results should be taken with caution as the sample numbers greatly varied per site. Pichincho exhibited the highest prey richness with 33 different prey species. We also observed high prey richness at Bajo Medio, another seamount and popular fishing ground for many fish species, especially queen snapper. The most common prey species identified at Bajo Medio were two species of Diaphus, D. brachycephalus (11 samples) and D. dumerilii (8 samples). At Pichincho, S. elongatus (11 samples) was the most common prey item, followed by Z. pedaliota (8 samples). Hypogeum benoiti and L. guentheri (8 samples), were the most common prey at Site 6.
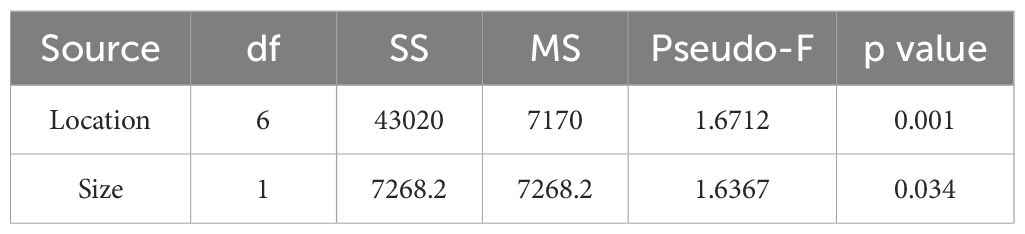
Table 5. The one-way Permutational Analysis of Variance tests examining the difference of prey composition between the locations and fork length sizes of Etelis oculatus along the west coast of Puerto Rico.
Smaller (<203 mm fork length) and larger fish (>1,016 mm fork length) were not landed during this project. Therefore, stomach samples were absent from these ranges. However, the species composition significantly differed between the size ranges 203–508 mm and 508–1,016 mm fork length (Table 5), even though species richness was similar between the two ranges, 45 species and 46 species, respectively. Z. pedaliota, S. elongatus, and H. benoiti were more common in the stomach contents of larger queen snapper (508–1016 mm FL), while D. brachycephalus, Myctophum nitidulum, D. dumerilii, Elagatis bipinnulata, and L. guentheri contributed the most to the prey composition of smaller queen snapper (203–508 mm FL, P < 0.05, SIMPER test).
Discussion
Our study is the first attempt to use the barcoding and metabarcoding approaches to uncover the diet composition in queen snapper. Unlike previous cases with analysis of fish gut contents (Haight et al., 1993), most of the fish prey we found were identified to the species level, and some of the most common invertebrate species were also identified to the species level. Identifiable prey was diverse systematically and ecologically and included Myctophid fishes with some benthic species like shrimps, fishes with demersal habits at a variety of depths, and fishes of the water column. Both molecular approaches suggest E. oculatus is a large carnivore that mainly feeds on squids, shrimps, and deep-water fishes. It coincides with earlier studies of its sister species, E. coruscans, and E. carbunculus that have also been reported as piscivorous fishes (i.e., primary piscivorous feeding guild; Haight et al., 1993). E. oculatus is classified as a 4.2 on the trophic level on FishBase, suggesting that only larger top predators, such as striped marlin or some sharks, are above it in the trophic pyramid (Froese and Pauly, 2002). The data provided in this study for E. oculatus, as well as the diet analysis of their Pacific congeners support the idea that E. oculatus likely represents a top predator in deep-water environments in the US Caribbean.
The most common prey of E. oculatus were D. brachycephalus, S. elongatus, Z. pedaliota, L. guentheri, M. nitidulum, H. benoiti, A. atlanticus, D. dumerilii, D. selenops, C. atlantica, and A. veranyi. These species belong to three orders of deep-sea fishes: Myctophiformes, Stomiiformes, and Aulopiformes. The myctophids, commonly known as lanternfishes, are a diverse group with over 240 species (Helfman et al., 2009). They occur in all seas and are the prey of numerous other fishes and marine mammals. Myctophiformes make up a significant fraction of the deep scattering layer – a diverse assemble of the fishes and invertebrates that live at mesopelagic depths (200–1000m) during the day and migrate towards the surface at dusk (Helfman et al., 2009). The Stomiiforms, commonly known as dragonfishes and allies, are characterized by inhabiting the mesopelagic and bathypelagic regions in the open water, between 200 and 4,000 m. During the day, Stomiiforms stay in deep water, and at night, migrate to the surface following zooplankton migration patterns, taking advantage of the plentiful food in the shallow areas of the ocean. Lastly, Aulopiforms are largely found in the open water in mesopelagic habitats. All these species are considered deep-water dwellers of the mesopelagic, bathypelagic, and benthic regions of the ocean (Helfman et al., 2009); however, we cannot identify which exact habitat E. oculatus targets these prey items.
Despite the lack of studies on the diet of deep-water snappers, we found an overlap with the only other study on Etelis species (Haight et al., 1993). Haight et al. (1993) found that the main prey of E. coruscans and E. carbunculus were mesopelagic lanternfishes and deep-water demersal cardinalfish. In Hawai’i, those mesopelagic prey species are a component of the “mesopelagic boundary community” (100-700 m), a band surrounding the islands or banks. Although, E. oculatus is feeding mainly on mesopelagic fishes, results from this study also suggest they feed on other fish species from families Scombridae, Phosichthyidae, Scopelarchidae, Gonostomatidae, Alepisauridae, Anguillidae, Caproidae, Carangidae, Chiasmodontidae, Coryphaenidae, Derichthyidae, Epigonidae, Evermamellidae, Howellidae, Macrouridae, Muraenidae, Opisthoproctidae, Phycidae, Pomacanthidae, Pomatomidae, Scombrolabracidae, Sternoptychidae, Synodontidae, Trachichthyidae, and Trichiuridae. Mychtophidae and Synodonidae were detected in stomach contents from deep-water snappers in previous studies in the Indo Pacific (Parrish, 1987), and were also identified in this study.
The diet analysis of E. oculatus also contained invertebrate prey such as three species of squids (A. veranyi, D. pealeii, and A. redfieldi), two shrimps (O. gracilirostris and S. debilis), and one isopod species. We must note that one squid species listed and six species of Scombriformes fishes were used as bait. Squids such as the ommastrepid (Humbolt squid) and chiroteuthis have been previously reported as prey in other Etelis species from the Pacific (Parrish, 1987; Haight et al., 1993). In addition, large crustaceans such as lobsters, shrimps, crabs, amphipods, euphausiids, isopods, and stomatopods have been found to be part of the diet of the deep snappers in the Pacific (Parrish, 1987; Seki and Callahan, 1988; Haight et al., 1993).
Our study results strongly suggest that E. oculatus can feed on benthic vertebrate and invertebrate species and capture mid-water fishes. We suggest that deep-water snappers are a key link between shallow, highly productive environments and demersal, mostly unproductive areas. The reason is that many E. oculatus prey were mesopelagic, like the Myctophiformes and Stomiiforms that we found. These fishes migrate daily from deep, unproductive areas to shallow, rich, productive areas following the zooplankton migration. The zooplankton in the upper layers of the ocean maintain a major fish community in the mesopelagic environment and, by doing so, also support a highly productive demersal community, thus supporting queen snapper.
Our data indicate that the diet composition of species of E. oculatus is relatively constant across locations. We noted some locations with higher prey richness in the stomach contents. However, these locations had more specimens collected, which prevented us from extricating if higher diversity was due to larger sample sizes or a location effect. The highest prey richness was observed at Pichincho, a seamount on the far western ridge of Desecheo Island, and a known recreational fishing site for highly migratory pelagic fishes (Appeldoorn et al., 2015). As observed during the Okeanos Expedition, high structural relief of the karstic terrain characterizes the seafloor geomorphology at Pichincho (Wagner et al., 2018), and strong bottom currents have eroded and molded some of this karst terrain (Chaytor et al., 2015). Given recent habitat models, this area might be a hotspot for queen snapper (Overly and Lecours, 2024). The variation in structural relief has allowed for the high diversity and abundance of benthic organisms such as deep-sea corals and sponges. During the expedition, small invertebrates and deep-water fishes, such as the misty grouper (Hyporthodus mystacinus) and queen snapper, inhabited caverns and overhangs that dominate this site. A more balanced sampling approach needs to be conducted to understand if there are site differences in prey items of E. oculatus.
We observed variation in the species composition of prey across size classes. Z. pedaliota, S. elongatus and H. benoiti were more common in larger queen snapper, while D. brachycephalus, Myctophum nitidulum, D. dumerilii, E. bipinnulata, and L. guentheri were more common in smaller E. oculatus. However, the limitation of sample size of smaller <203 mm FL) and larger (>1,016 mm FL) fishes in this study inhibits us to validate if the difference of diet may be related to an ontogenetic change of E. oculatus in the exploitation of different habitats as it changes in size. A more thoroughly designed study needs to be developed to confirm if the diet varies between size ranges in queen snapper.
Our study demonstrates that metabarcoding analysis is a powerful method for studying trophic interactions in deep-ocean species. This method provides fish species’ diet’s breadth and food web structure at a better taxonomical resolution than conventional analysis (Berry et al., 2015). For example, we detected that queen snapper diet is comprised of species in over 31 families. By providing a comprehensive view of the food web structure and dynamics and identifying prey species at risk of overexploitation, metabarcoding of fish stomach contents can inform management decisions about fisheries, habitat protection, and restoration efforts.
In ecosystem-based fish management (EBFM), metabarcoding of fish stomach contents provides valuable information by revealing relative abundance of prey items and identifying keystone species critical for ecosystem function, especially in difficult-to-access systems like the deep-ocean. In this study, myctophids and stomiiforms are essential players in the EBFM of mesophotic and deep- ocean areas around Puerto Rico by connecting deep-benthic habitats with shallow plankton-rich areas. We must address the limitations of this study. Close to 75% of the fish exhibited signs of barotrauma, meaning that most of the gut contents were expelled before being brought on the boat. Therefore, this study may not have captured the entire diversity of prey items for the queen snapper. In addition, due to experimental design (fishery-independent study), and the low sample size of this study, our conclusions about spatial and temporal variation of prey further needs investigation.
Our results from the metabarcoding approach could have been influenced by the database and the primers we used. It is likely that the database that we used does not contain all species of fish in the studied area, which could hamper our ability to identify some fish species. We, however, had very few sequences unassigned to species level and in all cases, we were able to ID them to family level. We did not notice any systematic absence of fish species related to a particular family, and in all cases, we double-checked the assignments from the database with a curated blastx search in NCBI, and a validation of the presence of the species in the studied area. It is unquestionably desirable to develop the database de-novo, but such effort is beyond most metabarcoding analyses given the effort and the expense associated. In addition, it is likely that the primers we used could have preferentially amplified some species within a family or an order. However, our results suggest that this is not the case because we amplified species from Anguilliformes to Scombriformes, which are on opposite sides of the Actinopterygii tree of life.
In summary, our study suggests that the diet of E. oculatus is broad and includes fishes with different behaviors that occupy different habitats of the ocean. The connectivity across layers of the sea permits the transfer of energy from shallow, highly productive areas to deeper less productive mesopelagic ones, which harbors an astonishing biomass of commercially-important species like the queen snapper. The new information we provided in this study will be vital to improve the function of ecosystem models across PR and consequently facilitate best practice of ecosystem-based fish management for these deep-ocean marine systems.
Data availability statement
The raw data and the summary tables for the metabarcoding supporting the conclusions of this article are available at https://doi.org/10.5281/zenodo.13838547. Sequences reported in the barcoding section have been submitted to GeneBank under accession number PQ376114-PQ376136.
Ethics statement
Ethical approval was not required for the study involving animals in accordance with the local legislation and institutional requirements because the samples taken were byproducts of industry.
Author contributions
SW: Conceptualization, Data curation, Formal analysis, Funding acquisition, Investigation, Methodology, Project administration, Supervision, Visualization, Writing – original draft, Writing – review & editing. CP: Conceptualization, Data curation, Formal analysis, Funding acquisition, Investigation, Methodology, Writing – original draft, Writing – review & editing. DB: Conceptualization, Data curation, Formal analysis, Investigation, Methodology, Writing – original draft, Writing – review & editing.
Funding
The author(s) declare financial support was received for the research, authorship, and/or publication of this article. This project was founded by the Caribbean Fisheries Management Council (NA15NMF4410012; NOAA CRCP CFMC Grant NA17NMF4410270), the Florida Wildlife Federation, Inc and The Pew Charitable Trusts Conserving Marine Life in the United States. CP was funded by grants from USDA National Institute of Food and Agriculture (1017848).
Acknowledgments
We would first like to thank Luis Roman and Nelson Crespo for their dedication and knowledge that they shared of the queen snapper fishery. We would also like to thank Braulio Quintero, Katie Flynn, Orian Tzadik, Fernando Melendez Vazquez, Manuel Nieves, Leysa Lopez Gonzalez, and Maria del Pilar Gonzalez Garcia for their help in processing the samples. We would also like to thank Wilson Santiago and Noemi Pena from the Department of Natural and Environmental Resources (DNER) for their help in the logistical support. Juliane Mora helped with the molecular work to ID preys and the HPC Center at URI provided all the server support to analyze the metabarcoding data. Also, we would like to thank Dr. Virginia Shervette for allowing us to collect gut contents and size measurements from her queen snapper samples. SW and DB have presented part of the content of this manuscript at the 178th Caribbean Fisheries Council Meeting and in technical report Williams, S. M., Prada, C., Beltrán, D. M. (2022) Characterization of Prey Diversity of the Commercially-Important. Caribbean Fisheries Management Council.
Conflict of interest
Author SW was employed by Coastal Survey Solutions LLC.
The remaining authors declare that the research was conducted in the absence of any commercial or financial relationships that could be construed as a potential conflict of interest.
Publisher’s note
All claims expressed in this article are solely those of the authors and do not necessarily represent those of their affiliated organizations, or those of the publisher, the editors and the reviewers. Any product that may be evaluated in this article, or claim that may be made by its manufacturer, is not guaranteed or endorsed by the publisher.
References
Allen G. R. (1985). FAO species catalogue. Vol. 6. Snappers of the world. An annotated and illustrated catalogue of lutjanid species known to date. FAO Fisheries Synopsis, No. 125, Vol. 6: 61–208. (Rome, Italy: Food and Agriculture Organization of the United Nations (FAO)).
Anderson M. J. (2001). Permutation tests for univariate or multivariate analysis of variance and regression. Can. J. Fish. Aquat. Sci. 58, 626–639. doi: 10.1139/f01-004
Appeldoorn R. S., Dennis D., Monterrosa Lopez O. (1987). Review of shared demersal resources of Puerto Rico and the Lesser Antilles region. FAO Fish. Rep. 383, 36–106.
Appeldoorn R. S., Sanders I. M., Färber L. (2015). A 51 year reconstruction of fisheries catch in Puerto Rico (Columbia, Vancouver, Canada: Final Report Fisheries Centre, University of British).
Baldwin C., Mounts J., Smith D., Weigt L. (2008). Genetic identification and color descriptions of early life-history stages of Belizean Phaeoptyx and Astrapogon (Teleostei: Apogonidae) with Comments on identification of adult Phaeoptyx. Zootaxa. 1, 2009. doi: 10.5281/zenodo.185742
Baldwin C. C., Weigt L. A., Smith D. G., Mounts J. H. (2009). Reconciling genetic lineages with species in western atlantic coryphopterus (Teleostei: Gobiidae). Smithsonian Contributions to Mar. Sci., 112–138.
Berry O., Bulman C., Bunce M., Coghlan M., Murray D. C., Ward R. D. (2015). Comparison of morphological and DNA metabarcoding analyses of diets in exploited marine fishes. Mar. Ecol. Prog. Ser. 540, 167–181. doi: 10.3354/meps11524
Boardman C., Weiler D. (1980). Aspects of the life history of three deepwater snappers around Puerto Rico. Proc. Gulf. Carib. Fish. Inst. 32, 158–172.
Callahan B., McMurdie P., Rosen M., Han A. W., Johnson A. J., Holmes S. P. (2016). DADA2: High-resolution sample inference from Illumina amplicon data. Nat. Methods 13, 581–583. doi: 10.1038/nmeth.3869
Chaytor J., Demopoulos A., Ten Brink U., Quattrini A. (2015). Ecology and geology of the MonaPassage Region- The view from D2 and Seirios (Silver Spring, Maryland, USA: NOAA Océano Profundo 2015: Exploring Puerto Rico’s Seamounts, Trenches, and Troughs. National Oceanic and Atmospheric Administration (NOAA)).
CMFC (2016). Comprehensive amendment to the U.S. caribbean fishery management plans: Application of accountability measures, including final environmental assessment, regulatory impact review, and regulatory flexibility act analysis [Technical report] (San Juan, Puerto Rico).
Cuellar N., Sedberry G. R., Machowski D. J., Collins M. R. (1996). Species composition, distribution and trends in abundance of snappers of the southeastern USA, based on fishery independent sampling. ICLARM Conf. Proc. 48, 59–73.
Curd E. E., Gold Z., Kandlikar G. S., Gomer J., Ogden M., O'Connell T., et al. (2019). Anacapa Toolkit: An environmental DNA toolkit for processing multilocus metabarcode datasets. Methods Ecol. Evol. 10, 1469–1475. doi: 10.1111/2041-210X.13214
D’Alessandro E. K., Sponaugle S., Serafy J. E. (2010). Larval ecology of a suite of snappers (family: Lutjanidae) in the straits of florida, western atlantic ocean. Mar. Ecol. Prog. Ser. 410, 159–175. doi: 10.3354/meps08632
De Sousa L. L., Silva S. M., Xavier R. (2019). DNA metabarcoding in diet studies: Unveiling ecological aspects in aquatic and terrestrial ecosystems. Environ. DNA. 1, 199–214. doi: 10.1002/edn3.27
Folmer O., Black M., Hoeh W., Lutz R., Vrijenhoekand R. (1994). DNA primers for amplification of mitochondrial cytochrome c oxidase subunit I from diverse metazoan invertebrates. Mol. Mar. Biol. Biotech. 3, 294–299. doi: 10.1071/MB9940294
Froese R., Pauly D. (2022). FishBase (World Wide Web electronic publication). Available at: www.fishbase.org.
Gao X., Lin H., Revanna K., Dong Q. (2017). A Bayesian taxonomic classification method for 16S rRNA gene sequences with improved species-level accuracy. BMC Bioinf 18, 1–10. doi: 10.1186/s12859-017-1670-4
García-Sais J. R. (2015). Characterization of Deep Reef Benthic Habitats of Queen Snapper in Mona Passage, Puerto Rico. Final Report submitted to the Caribbean Fisheries Management Council, 85 pp. (San Juan, Puerto Rico: Caribbean Fisheries Management Council).
Garcia-Sais J. R., Williams S., Sabater J., Garcia-Moliner G. (2018). Characterization of benthic habitats associated with deep-water snapper fishing grounds of Desecheo Ridge and other seamounts of the west coast of Puerto Rico (Final Report submitted to Caribbean Fisheries Management Council) (San Juan, Puerto Rico: Caribbean Fisheries Management Council), 118.
Gobert B., Guillou A., Murray P., Berthou P., Oqueli Turcios M. D., Lopez E., et al. (2005). Biology of queen snapper (Etelis oculatus: Lutjanidae) in the Caribbean. Fish. Bull. 103, 417–425.
Haight W., Parrish J., Hayes T. A. (1993). Feeding ecology of deepwater lutjanid snappers at penguin bank, Hawaii. Trans. Am. Fish. 122, 328–347. doi: 10.1577/1548-8659(1993)122<0328:FEODLS>2.3.CO;2
Helfman G., Collette B., Facey D., Bowen B. (2009). The diversity of fishes: Biology, Evolution, and Ecology. 2nd ed. (New Jersey: Wiley-Blackell).
Kearse M., Moir R., Wilson A., Stones-Havas S., Cheung M., Sturrock S., et al. (2012). Geneious Basic: an integrated and extendable desktop software platform for the organization and analysis of sequence data. Bioinformatics 28, 1647–1649. doi: 10.1093/bioinformatics/bts199
Langmead B., Salzberg S. (2012). Fast gapped-read alignment with Bowtie 2. Nat. Methods 9, 357–359. doi: 10.1038/nmeth.1923
Martin M. (2011). Cutadapt removes adapter sequences from high-throughput sequencing reads EMBnet. journal. 17, 1. doi: 10.14806/ej.17.1.200
Matos-Caraballo (2012). DRNA commercial fisheries statistics 2007-2011 (Puerto Rico NMFS Cooperative Fisheries Statistics Program).
Overly K. E., Lecours V. (2024). Mapping queen snapper (Etelis oculatus) suitable habitat in Puerto Rico using ensemble species distribution modeling. PLoS One 19, e0298755. doi: 10.1371/journal.pone.0298755
Overly K. E., Shervette V. R. (2023). Caribbean deepwater snappers: Application of the bomb radiocarbon age estimation validation in understanding aspects of ecology and life history. PLoS One 18, e0295650. doi: 10.1371/journal.pone.0295650
Parrish J. D. (1987). “The trophic biology of snappers and groupers, in Tropical snappers and groupers,” in Biology and fisheries management. Eds. Polovina J. J., Ralston S. (Westview Press, Boulder), 405–463.
Randall J. E. (1967). Food habits of reef fishes of the west indies. Stud. Trop. Oceanography Miami 5, 665–847.
R Core Team (2017). R: A language and environment for statistical computing (Vienna, Austria: R Foundation for Statistical Computing). Available at: https://www.R-project.org/.
Seki M. P., Callahan M. W. (1988). The feeding habits of two deep slope snappers, pristipomoides zonatus and p. auricilla, at pathfinder reef, mariana archipelago. Fish. Bull. 86 (4), 807–811.
Shokralla S., Spall J. L., Gibson J. F., Hajibabaei M. (2012). Next-generation sequencing technologies for environmental DNA research. Mol. Ecol. 21, 1794–1805. doi: 10.1111/j.1365-294X.2012.05538.x
South Atlantic Fishery Management Council (2005). Stock assessment and fishery evaluation report for the snapper grouper fishery of the south atlantic [Technical report] (Florida, USA).
Taberlet P., Bonin A., Coissac E., Zinger L. (2018). Environmental DNA: For biodiversity research and monitoring. (Oxford, UK: Oxford University Press).
Thuo D., Furlan E., Broekhuis F., Kamau J., Mcdonald K., Gleeson D. M. (2019). Food from faeces: Evaluating the efficacy of scat DNA metabarcoding in dietary analyses. PLoS One 14, e0225805. doi: 10.1371/journal.pone.0225805
Tonioli F. C., Agar J. J. (2011). Synopsis of Puerto Rican commercial fisheries (Vol. 69 NOAA Technical Memorandum NMFS-SEFSC-622). Silver Spring, MD: National Oceanic and Atmospheric Administration (NOAA).
Wagner D., Sowers D., Williams S. M., Auscavitch S., Blaney D., Cromwell M. (2018). EX 18-11 Expedition Report -Océano Profundo 2018: Exploring deep-sea habitats off Puerto Rico and the U.S. Virgin Islands (OER Expedition Report EX-18-11). (Silver Spring, MD: Office of Ocean Exploration and Research, Office of Oceanic & Atmospheric Research, NOAA), 171 pp. OER Expedition Report EX-18-11. doi: 10.25923/wc2n-qg29
Keywords: queen snapper, prey diversity, deep-sea, metabarcoding, Caribbean
Citation: Williams SM, Prada C and Beltrán DM (2024) Prey diversity in the deep ocean: metabarcoding feeding ecology of the commercially important queen snapper in the US Caribbean. Front. Mar. Sci. 11:1409336. doi: 10.3389/fmars.2024.1409336
Received: 29 March 2024; Accepted: 10 September 2024;
Published: 11 October 2024.
Edited by:
Tomaso Fortibuoni, Istituto Superiore per la Protezione e la Ricerca Ambientale (ISPRA), ItalyReviewed by:
Arsalan Emami-Khoyi, University of Johannesburg, South AfricaKatherine Overly, Southeast Fisheries Science Center (NOAA), United States
Copyright © 2024 Williams, Prada and Beltrán. This is an open-access article distributed under the terms of the Creative Commons Attribution License (CC BY). The use, distribution or reproduction in other forums is permitted, provided the original author(s) and the copyright owner(s) are credited and that the original publication in this journal is cited, in accordance with accepted academic practice. No use, distribution or reproduction is permitted which does not comply with these terms.
*Correspondence: Stacey M. Williams, c3RjbXdpbGxpYW1zQGdtYWlsLmNvbQ==
†These authors have contributed equally to this work