- 1Co-Innovation Center of Jiangsu Marine Bio-industry Technology, Jiangsu Ocean University, Lianyungang, China
- 2Jiangsu Key Laboratory of Marine Biotechnology, Jiangsu Ocean University, Lianyungang, China
- 3Jiangsu Key Laboratory of Marine Bioresources and Environment, Jiangsu Ocean University, Lianyungang, China
- 4Jiangsu Institute of Marine Resources Development, Jiangsu Ocean University, Lianyungang, China
- 5College of Bio-systems Engineering and Food Science, Zhejiang University, Hangzhou, China
- 6State Environmental Protection Key Laboratory of Land and Sea Ecological Governance and Systematic Regulation, Shandong Academy for Environmental Planning, Jinan, China
Development of environment-friendly and efficient aquaculture effluent treatment system is crucial for sustainable intensification of aquaculture, in the face of the rapidly increasing environmental pressure in the mariculture industry. In this study, mariculture wastewater was treated by the anoxic-anaerobic-oxic biochemical treatment system (A2O system) with traditional activated sludge replaced by nitrifying bacteria, denitrification bacteria and phosphorus accumulating bacteria absorbed on PBS carrier biofilms suitable for saline/brackish water. The results showed that biofilm-enhanced A2O system can effectively remove pollutants from aquaculture wastewater. The removal efficiencies of CODMn, NH4+-N, TN and TP in A2O system were approximately 86.3%-90.8%, 97.7%-99.5%, 94.6%-95.2% and 97.0%-98.1%. The results further showed that CODMn, NH4+-N, and TN were mainly removed in anaerobic tank and anoxic tank, while TP was mainly removed in the anoxic tank and oxic tank. The biofilm-enhanced A2O system by adding nitrifying bacteria and phosphorus accumulating bacteria biofilms using PBS as carriers instead of conventional activated sludge could be applied to the treatment of circulating aquaculture wastewater. This study provides a feasible scheme for enhancing the efficiency of A2O system in the treatment of aquaculture tail water, and provides a reference for the immobilization of microorganisms.
Introduction
With the rapid development of population and economy, seafood such as fish and shrimp from aquaculture plays a very important role in China’s food supply (Yaseen et al., 2022; Fong et al., 2023). Statistically, China is the only country in the world where aquaculture production exceeds fishery production, accounting for more than 30% of the world’s total aquatic products. However, the rapid development of aquaculture industry produced a large amount of aquaculture wastewater containing organic matter and ammonia-nitrogen. The direct discharge of marine aquaculture wastewater caused serious environmental pollution, such as eutrophication (Zhang et al., 2023b). Furthermore, it has been confirmed that marine aquaculture wastewater was an oligotrophic wastewater, with low concentrations of ammonia-nitrogen (NH4+-N) and organic matter, but also with high salinity (about 30). At present, the environmental pollution caused by the direct discharge of aquaculture wastewater has attracted more and more attention. Many treatment methods of aquaculture wastewater have been explored and reported. For instance, Ma et al. (2021) realized effective treatment of aquaculture wastewater by the constructed wetland system (CWs) and Zhou et al. (2022) found that macroalga could adsorb nutrients in aquaculture water. Although the CWs has a good treatment effect on aquaculture wastewater, it has the disadvantages of large area and high investment cost (Ren et al., 2021). Aerobic denitrifying bacteria isolated from aquaculture farms, such as Vibrio spp. AD2. These bacteria can effectively remove ammonia and nitrate from aquaculture wastewater under aerobic conditions. However, aerobic denitrifying bacteria are heterotrophic microorganisms, and their growth and nitrogen removal performance depend on high C/N ratio. The growth and energy metabolism of aerobic denitrifying bacteria require sufficient supply of carbon sources to meet their growth and energy metabolism needs (ref). Aquaculture wastewater was an oligotrophic wastewater, which further limited the application of aerobic denitrification in aquaculture wastewater treatment (Tang et al., 2020). These characteristics of marine aquaculture wastewater are not conducive to the growth and nitrogen removal performance of traditional nitrifying bacteria, such as Nitrosomonas, Nitrosospira, and Nitrospira (Li et al., 2020; Nunzia et al., 2021). Therefore, it is difficult for marine aquaculture wastewater to be effectively treated by traditional biological methods.
However, faced with the rapidly increasing environmental pressure of the seawater aquaculture industry, there is an urgent need for efficient and low-cost wastewater treatment technologies. The A2O process has been used for urban sewage treatment. By adding an anoxic tank to the AO process, the organic matter, nitrogen and phosphorus in the water can be removed while denitrification. The process has a simple structure and the total hydraulic retention time is shorter than other similar processes. Currently, research on the A2O process has shifted from improving wastewater treatment efficiency to treating special wastewater, such as high-strength, highly recalcitrant wastewater from the production of polyphenylene sulfide (PPS) resins and their composite chemicals (Guo et al., 2023), brewery wastewater, meat processing wastewater, pharmaceutical wastewater, etc (Sun et al., 2015; Gallardo-Altamirano et al., 2019). Alternatively, research can improve the treatment efficiency of polluted wastewater by modifying the A2O process or combining it with other processes. For example, Congcong Zhang (Zhang C. et al., 2023) combined the side flow sludge fermenter with the A2O system. Yongqing Gao et al. (2011) combined two-step alkaline sludge fermentation with A2O system. Likun Huang et al. (2022) studied the A2O -MBR -BAF -O3 combined process. The A2O-MBR combined process has also been studied by many scholars (Na et al., 2017; Abyar et al., 2018; Li et al., 2019). Chunhong Na (Na et al., 2017) studied the combination of anaerobic-anoxic-oxic (A2O) and deep oxidation processes. IFAS (Gallardo-Altamirano et al., 2021) studied an integrated fixed membrane activated sludge system. A full-scale biofilm system using fluidized-carriers integrated with anaerobic-anoxic–aerobic process was used for municipal wastewater treatment (Xiao et al., 2016).The sludge age (residence time of biological solids) is the control objective of wastewater nitrification management. In order for the nitrifying bacterial community to survive in a continuous flow system, the SRT of the system must be greater than the specific growth rate of autotrophic nitrifying bacteria. Short sludge age can lead to the loss of nitrifying bacteria or a decrease in nitrification rate. In actual denitrification projects, the sludge age generally selected should be greater than the actual SRT. Research has shown that for activated sludge denitrification, the sludge age is generally not less than 15 days. However, excessive sludge age was not conducive to phosphorus removal (Wang et al., 2019). The A2O system was relatively mature in the treatment of freshwater wastewater. But there are few reports on its use in saline/semisaline water treatment, which might have a significant relationship with activated sludge (Xiao et al., 2020; Ma et al., 2023). Additionally, the traditional A2O system was not suitable for the treatment of the nutrient-poor seawater aquaculture wastewater (Rajesh Banu et al., 2009). The traditional A2O system mainly removes nitrogen and phosphorus by microorganisms in the activated sludge. The existence of activated sludge in the system is limited, and when the activated sludge exists in the high-salt and poor-nutritional water body, the microorganisms in the sludge will also limit the efficiency of nitrogen and phosphorus removal due to the lack of carbon source. Therefore, strengthening the A2O system by taking some measures may be an alternative approach. A suitable treatment system for saline/brackish water aquaculture wastewater was conducted by combined the A2O system with nitrifying bacteria, denitrification bacteria and phosphorus accumulating bacteria biofilm systems suitable for saline/brackish water, i.e. Biofilm-enhanced A2O system. Biofilm-enhanced A2O system has the advantage of providing organic carbon source independently and has strong salt tolerance, which can solve the shortcomings of traditional A2O system in the treatment of mariculture tail water, small floor area, high treatment efficiency, free operation time, and low investment cost. Biofilm-enhanced A2O system has extensive potential in the treatment of aquaculture wastewater.
At present, the main limitation of applying A2O system to treat the marine aquaculture wastewater with the characteristics of oligotrophic was that the organic carbon source required by denitrifying bacteria is insufficient, which limits their normal growth and energy metabolism (Wu et al., 2023). It is believed that denitrifying microorganisms are heterotrophic microorganisms, which not only require sufficient carbon sources for their growth, but also require carbon sources as electron donors to achieve complete nitrogen removal in denitrification (Zhang et al., 2023a). The effluent from PBS carrier contained mainly protein-like and soluble microbial product-like substances, which can be used as the growth carrier of microorganisms and the source of organic carbon (Díaz et al., 2014). The proportion of methyl and hydroxyl functional groups in PBS materials decreased, while other functional groups did not change significantly (Zhang C. et al., 2023). Starch and ethylene, the main monomer components of PBS, could be used as carbon sources by denitrification microorganisms. Scanning electron microscope observation showed that after the attachment and growth of denitrification biofilm, holes would appear on the surface of PBS particles, expanding the surface area of biofilm biological attachment and growth, which was conducive to the formation of dense denitrification biofilm, Protection against denitrifying bacteria (Wang et al., 2012). The application of PBS particles as a denitrification carbon source and biofilm carrier in a packed bed reactor for A2O system has certain feasibility (Zeng et al., 2010; Tang et al., 2021). Therefore, it is of great significance to use PBS as biofilm carrier to strengthen the A2O system to remove the marine aquaculture wastewater with the characteristics of oligotrophic wastewater.
In this study, the A2O system was strengthened by biofilms of microorganisms adsorbed on PBS carriers to evaluate the treatment effect of A2O system on aquaculture wastewater, including chemical oxygen demand (CODMn), NH4+-N, total nitrogen (TN), total phosphorus (TP), etc. This study provides a practical method for aquaculture wastewater treatment.
Experimental materials and methods
Experimental setup and operation
An A2O urban domestic sewage treatment (A2O UDST) system with five units was set up in the laboratory, including inlet sedimentation tank, anaerobic tank, anoxic tank, oxic tank, and outlet sedimentation tank (Figure 1). The working volume of the inlet tank, anaerobic tank, anoxic tank, oxic tank, and outlet sedimentation tank were 1000 L, 20 L, 25 L, 53 L, and 10 L, respectively. The bottom of outlet sedimentation tank was provided with a return pipe to return the sludge to the anaerobic tank and the return ratio was about 300%. A circular distribution bucket with a working volume of 1000 L was used to hold simulated aquaculture wastewater. A peristaltic pump connected to a distribution bucket and an inlet settling tank was used to pump aquaculture waste into the A2O system. Besides, an aeration pump was installed at the bottom of the oxic tank for continuous aeration to maintain a high dissolved oxygen concentration.
The entire operation of the A2O system consisted of two phases: initial startup phase (0−15 days) and biofilm strengthening phase (16−180 days). In the initial startup phase, 200 g of the mature PBS biofilm was further added to anaerobic tank, anoxic tank, oxic tank every 5 days (i.e., 5 days of sludge age), respectively. After 15 days of stable operation, the A2O system entered the second phase, i.e. the biofilm strengthening stage. The peristaltic pump continuously pumps aquaculture waste into the A2O system, and controls the HRT time to 8 h. The effluent flow rate was 10 L/h. A2O system was equipped with agitator, and the shaking speed was controlled at 1000 rpm/min. Water samples were collected every 7 days from each reaction tank of the A2O system for the determination of CODMn. The collected samples were filtered through a 0.45 μm filter membrane to determine the concentration of NH4+-N, NO2–N, NO3–N, and TP.
Biofilm culture
To obtain mature biofilms, the enhanced microorganisms were placed in the same container with PBS and cultured for approximately 14 days and biofilms were formed on PBS. Anaerobic biofilm and oxic biofilm were cultured in oxic and anaerobic environments respectively. Hypoxia: phosphorus-accumulating bacteria, nitrifying bacteria, and PBS were added to a sealed container. Oxic: phosphorus-accumulating bacteria, nitrifying bacteria, and PBS were added to the open container. In this experiment, a microorganism isolated from the sea area of Haizhou Bay by laboratory separation and purification and send to 16s rRNA sequence, at last confirmed that the microorganism is named Halomonas sp.; nitrifying bacteria (rich in nitrifying bacteria and denitrification bacteria) were purchased from Beihai Yeshengwang Biotechnology Co., Ltd., liquid (Biozym); PBS (polybutylene succinate) (HO-(CO-(CH2)2-CO-O-(CH2)4-O) n-H) was a white or light yellow crystalline cylinder with a diameter of approximately 2 mm and a height of approximately 4 mm.
Analytical method
The aquaculture wastewater was taken from aquaculture farms and originated from Haizhou Bay. The characteristics of aquaculture wastewater were as follows: the concentrations of COD, NH4+-N, TN/NOx and TP were 1.2 mg/L, 0.89 mg/L, 0.98 and 0.1 mg/L. The concentration of CODMn, NH4+-N, NO2–N, NO3–N, and TP were measured using the method specified in the seawater monitoring standard GB/T 17378.4-1998.
Results
Water quality characteristics change
The characteristics of water quality change in A2O system were measured. As shown in Figure 2A, the influent CODMn concentration of the A2O process were 1.13 ± 0.04 mg/L, the effluent CODMn concentration of the anaerobic tank was 0.65 ± 0.02 mg/L, the effluent CODMn concentration of the anaerobic tank was 0.21 ± 0.03 mg/L, and the effluent CODMn concentration of the oxic tank was 0.13 ± 0.02 mg/L. In the first three months, the total CODMn removal efficiencies of A2O system were 86.3-87.7%. In the last three months, the total CODMn removal efficiencies of A2O system were rose to 90.7-90.8%. The removal of NH4+-N in the A2O system was shown in Figure 2B. The concentrations of influent water in the A2O system were 0.75-0.89 mg/L, the effluent from the anaerobic tank was 0.18-0.21 mg/L, the effluent from the anaerobic tank was 0.05-0.13 mg/L, and the effluent from the oxic tank was 0.004-0.02 mg/L. The total removal efficiencies were approximately 97-99.5%. The removal of TN/NOx in the A2O system were shown in Figure 2C. The influent concentrations of TN in the A2O system were 0.82-0.98 mg/L. the NO3–N concentrations in the anaerobic tank outlet were 0.64-0.7 mg/L, the NO3–N concentrations in the anaerobic tank outlet were 0.02-0.03 mg/L, the NO3–N concentrations in the oxic tank outlet were 0.03-0.04 mg/L, the NO2–N concentrations in the oxic tank outlet were 0.001-0.01 mg/L, the TN concentrations in the oxic tank outlet were 0.04-0.05 mg/L, and the TN removal efficiencies were 94.7-95.2%. The removal of TP in the A2O system was shown in Figure 2D. The influent concentrations of TP in the system were 0.07-0.1 mg/L, the concentrations of TP in the effluent of the anaerobic tank were 0.06-0.09 mg/L, the concentrations of TP in the effluent of the anaerobic tank were 0.003-0.04 mg/L, and the concentrations of TP in the effluent of the oxic tank were 0.001-0.002 mg/L. The TP removal efficiencies were 97-98%.
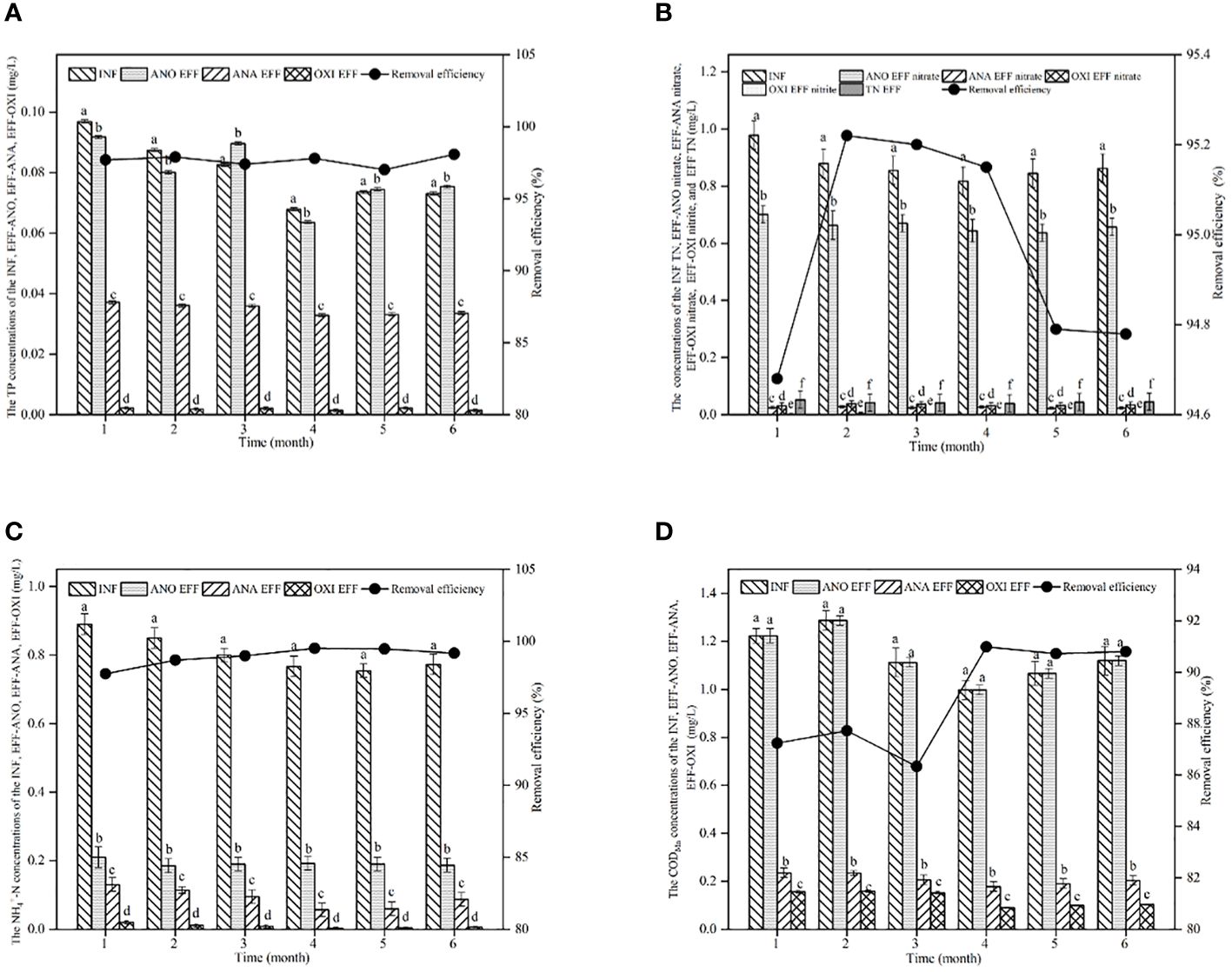
Figure 2 Concentrations and total removal efficiency of CODMn, NH4+-N, TN/NOx, and TP in each unit of the A2O system, including: anaerobic tank, ANA; anoxic tank, ANO; oxic tank, OXI; influent, INF; effluent, EFF. (A) Concentrations and total removal efficiency of CODMn in each unit of the A2O system; (B) Concentrations and total removal efficiency of NH4+-N in each unit of the A2O system; (C) Concentrations and total removal efficiency of TN/NOx in each unit of the A2O system; (D) Concentrations and total removal efficiency of TP in each unit of the A2O system. The same letter indicates no significant difference(P>0.05), while different letters indicate significant difference(P<0.05).
The removal efficiencies of CODMn, NH4+-N, TN/NOx, and TP in the A2O system
The removal efficiencies of CODMn, NH4+-N, TN/NOx, and TP in the A2O system were shown in Figure 3. As shown in Figure 3A, the removal efficiency of CODMn in the anaerobic tank and the anoxic tank remained stable at 39.2-46.9% and 65.7-70.6%, respectively. The removal efficiency of CODMn in oxic tank gradually decreased in the first three months and the removal efficiencies were about 26.2-33.6%. After that, the removal efficiency of CODMn increased significantly to 49.4% in the fourth month. The removal efficiencies of NH4+-N were showed in Figure 3B. The removal efficiencies of NH4+-N in anaerobic tank gradually increased from 38.1% to 70.3% in the first four months and then gradually decreased to approximately 53.5% in the sixth month. The removal efficiencies of NH4+-N in anoxic tank remained stable, approximately 76.4%. The removal efficiencies of NH4+-N in oxic tank maintained high level, approximately 91.1%. The removal efficiencies of TN were showed in Figure 3C. The removal efficiencies of TN in anaerobic tank, and anoxic tank remained stable, approximately 62.6% and 21.1%. The removal efficiencies of TP were showed in Figure 3D. The removal efficiencies of TP in anaerobic tank and oxic tank were 55.7% and 94.7%, respectively.
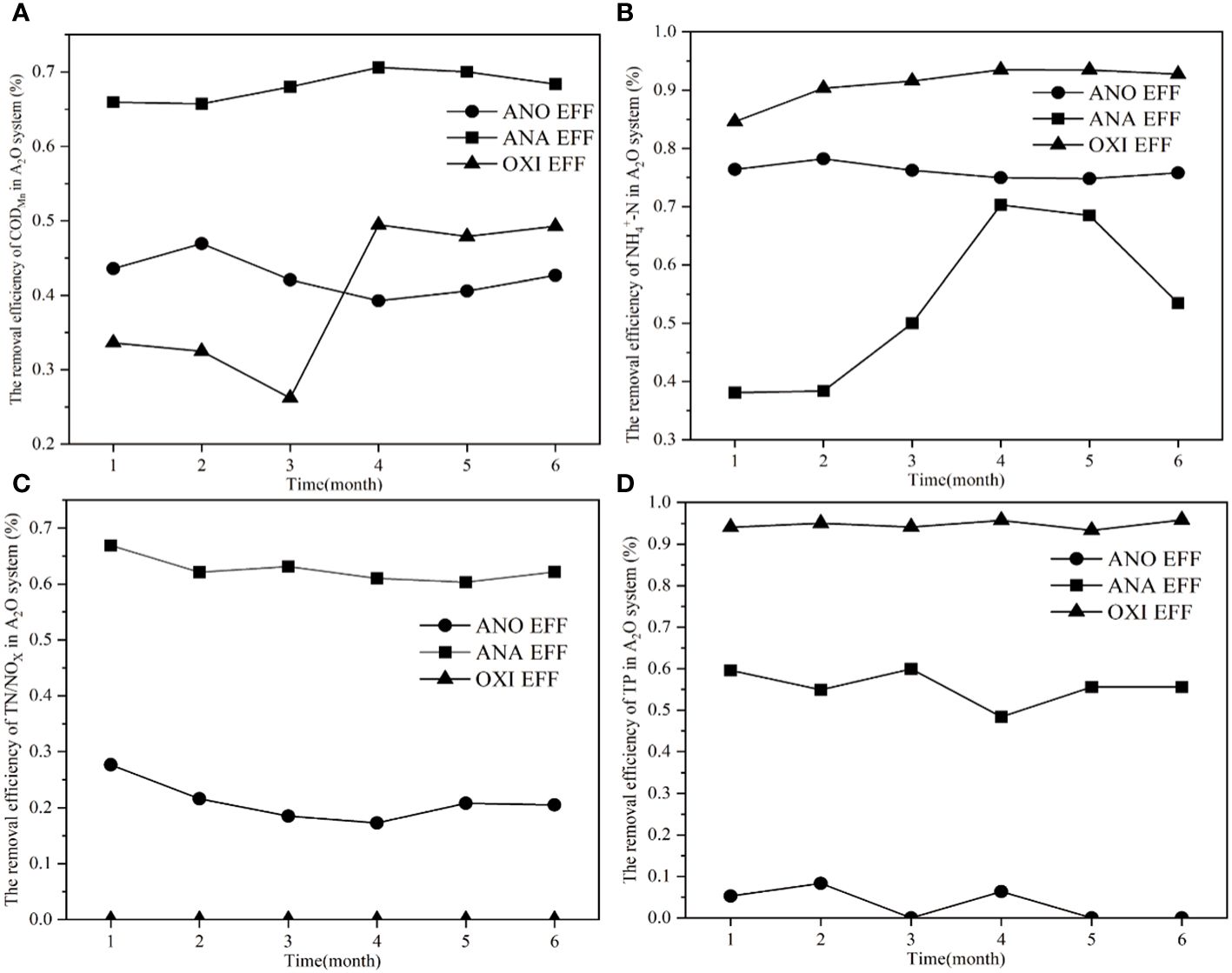
Figure 3 The removal efficiencies of CODMn, NH4+-N, TN/NOx, and TP in each unit of the A2O system, including: anaerobic tank, ANA; anoxic tank, ANO; oxic tank, OXI. (A) The removal efficiency of CODMn in each unit of the A2O system; (B) The removal efficiency of NH4+-N in each unit of the A2O system; (C) The removal efficiency of TN/NOx in each unit of the A2O system; (D) The removal efficiency of TP in each unit of the A2O system.
Water quality removal analysis of anoxic, anaerobic and oxic tanks
The removal distribution of different indicators (CODMn, NH4+-N, TN and TP) in anaerobic tank, anoxic tank, and oxic tank were shown in Figure 4. The A2O system can remove a total of 88.9% of CODMn, where anaerobic tank, anoxic tank, and oxic tank can remove approximately 39%, 42.7%, and 7.2%, respectively. The A2O system can remove a total of 98.9% of NH4+-N, where anaerobic tank, anoxic tank, and oxic tank can remove approximately 12.6%, 76.1%, and 10.2%, respectively. The A2O system can remove a total of 99.8% of TN, where anaerobic tank, anoxic tank, and oxic tank can remove approximately 24.1%, 73%, and 2.7%, respectively. The A2O system can remove a total of 99.8% of TP, where anaerobic tank, anoxic tank, and oxic tank can remove approximately 3.5%, 55.3%, and 41%, respectively.
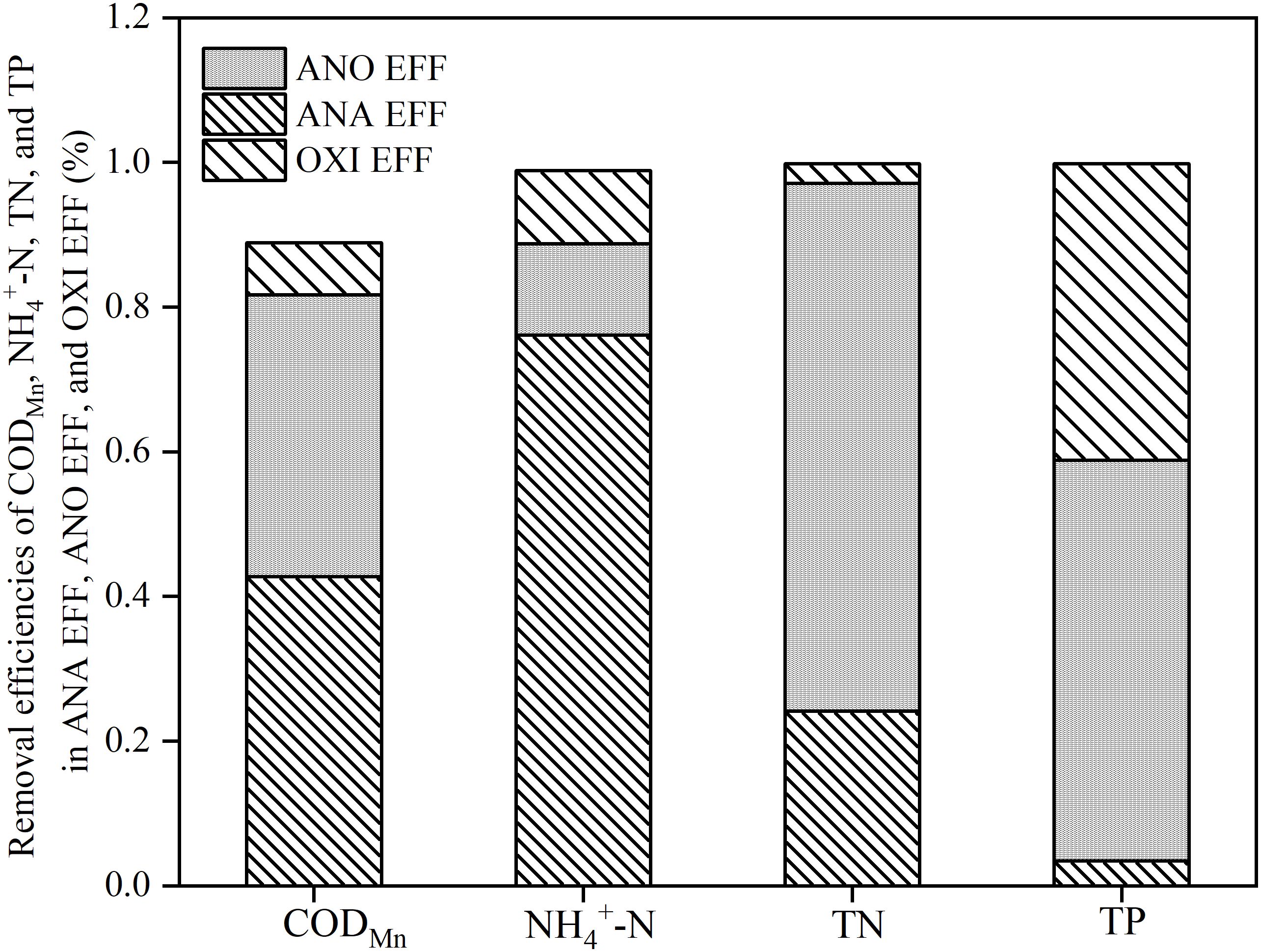
Figure 4 The removal distribution of different indicators (CODMn, NH4+-N, TN and TP) in anaerobic tank (ANA EFF), anoxic tank (ANO EFF), and oxic tank (ANO EFF).
Discussion
In this study, the results showed that the A2O system by adding nitrifying bacteria and phosphorus accumulating bacteria biofilms using PBS as carriers instead of conventional activated sludge could effectively treat aquaculture wastewater. Compared to the application of the A2O system in freshwater wastewater treatment (Ravishankar et al., 2019; Chen et al., 2022; Choi et al., 2022) or the combination of A2O with MBR (Hao et al., 2022), BAF (Xi et al., 2022), SBR (Dai et al., 2022), the application in saline/semisaline water could achieve relatively ideal results.
As shown in Figure 2, biofilm-enhanced A2O system can effectively remove CODMn. In the first three months, the CODMn removal efficiency of the A2O system was about 87.2%. After three months of PBS biofilm enhancement of A2O system, the removal efficiency of CODMn was further improved to approximately 91%. The results in Figure 3A further also showed that the removal efficiency of CODMn in the oxic tank gradually decreased in the first three months and gradually increased thereafter. This may be due to the gradual growth of heterotrophic microorganism after 3 months of A2O system operation, which further improved the utilization of organic matters (Choi et al., 2022). Nevertheless, the results of Figure 4 further showed that CODMn was mainly removed in anaerobic tank and anoxic tank, accounting for about 92.7% of the total CODMn removal. Simultaneously, the removal efficiency of CODMn in the anaerobic tank and the anoxic tank remained stable (Figure 3A). It has been proved that heterotrophic nitrifying bacteria was more sensitive to oxygen, and heterotrophic nitrifying bacteria was mainly distributed in anaerobic and anoxic environment in A2O systems (Ravishankar et al., 2019). Heterotrophic nitrifying bacteria require organic matter as an energy source for growth and metabolism (Chen et al., 2022). Moreover, it has been confirmed that many autotrophic nitrifying bacteria were mainly distributed in the oxic tank of the A2O system, such as Nitrosomonas, Nitrosospira, Nitrospira, and Nitrotoga (Hao et al., 2022). These bacteria mainly rely on an autotrophic lifestyle for growth and metabolism resulting in a low need for organic matter (Xi et al., 2022). Therefore, the removal of CODMn in the A2O system may be mainly due to the growth of heterotrophic nitrifying bacteria. The results in Figure 2, the removal efficiencies of NH4+-N and TP remained stable, and the removal efficiencies were 98.9% and 97.7%, respectively. This indicated that biofilm-enhanced A2O system had a good performance in removing NH4+-N and TP from aquaculture wastewater. Autotrophic nitrifying bacteria can use oxygen as electron acceptor to oxidize ammonia to nitrite or nitrate under oxic conditions by autotrophic nitrification (NH4+-N→NH2OH→NO2–N→NO3–N) (Dai et al., 2022). Therefore, the nitrification of autotrophic nitrifying bacteria may be one of the reasons for the increased removal efficiency of NH4+-N. As shown in Figure 3B, the results showed that the removal efficiency of NH4+-N in the anaerobic tank of the A2O system decrease from 70.3% to 53.5%, which may be due to the release of intracellular nitrogen after the ganglionic formation of some dead bacterial cells, resulting in an increase in NH4+-N concentration (Yue et al., 2023). Nevertheless, some studies also believed that this may also be due to the reduction of nitrate and nitrite to ammonia caused by the reduction of ammonia nitrogen removal rate (Xiang et al., 2023). The results in Figure 4 showed that nearly 76.1% of NH4+-N was removed under anoxic conditions, which was similar to Peng et al. (2020). This was mainly due to the existence of a large number of facultative anaerobic and oxic microorganisms in the anoxic environment, which can effectively use ammonia nitrogen (Marazuela et al., 2023).
As shown in Figure 2, the results showed that CODMn and NH4+-N decreased significantly in the anaerobic tank, while the reduction of nitrate nitrogen and TP were not significant and even slightly increased. The reason was that the aquaculture wastewater in the tank was raw sewage after sedimentation and returned phosphorus-containing sludge. Dissolved organic matter and NH4+-N were absorbed by microorganisms for their own cell synthesis and respiratory metabolism (Zhang et al., 2023a), but the change in NO3–N content was not significant (Figure 2C). The characteristics of phosphorus released by phosphorus-accumulating bacteria in the anaerobic tank made the concentration of TP in wastewater not significantly reduced (Figure 3D). The results in Figure 4 also confirmed that approximately 96.3% of TP was removed in anoxic tank and oxic tank. The results in Figure 2 also showed that the concentration of NO3–N and phosphorus decreased significantly. However, as the nitrification process increased the concentration of NO3–N, TP also decreased at a faster rate with excessive uptake by phosphorus-accumulating bacteria. In the anoxic tank, PBS could not only be used as a carrier of microorganisms to help the growth of nitrifying bacteria/denitrification bacteria/phosphorus accumulating bacteria but could also properly supplement the carbon source required for microbial growth due to its own degradation (Zhang et al., 2019). The denitrification bacteria used the organic matter in the sewage and part of the products degraded by PBS as the carbon source to bring a large amount of NO2–N and NO3–N into the reflux mixture and reduced them to N2 or released NXO to the air.
Nitrogen and phosphorus removal mechanisms in three different phases of the reactor were shown in Figure 5. In anaerobic period, the sludge returned from the oxic zone entered the anaerobic zone. NH4+-N in the oxic zone was converted by AOB and NOB to nitrite and nitrate through nitrification. NO2–N and NO3–N were further removed to NOX/N2 heterotrophic nitrification bacteria (HNB). Simultaneously, it has been reported that HNB can use carbon source as electron donor and energy source to achieve denitrification (Abyar et al., 2018; Li et al., 2020). Therefore, nitrogen and COD are simultaneously removed by organic matter during the anaerobic phase of the reactor. Besides, phosphorus accumulating bacteria release phosphorus from the cell into the activated sludge (Ravishankar et al., 2019). Phosphorus and nitrogen released into the sludge entered the anoxic zone and were further removed by phosphorous-accumulating bacteria (PAOs) and Glycanogen organisms (GAOs). Some studies have confirmed that PAOS and GAOS can store carbon sources in the form of PHB in microbial cells, and achieve nitrogen and phosphorus removal through endogenous denitrification (ED).
In this study, by adding nitrifying bacteria and phosphorus accumulating bacteria biofilms using PBS as carriers instead of conventional activated sludge, the A2O system could effectively solve the inherent contradiction between nitrogen (long HRT) and phosphorus (short HRT) on HRT and improve treatment efficiency. In contrast to the methods mentioned above, biofilm-enhanced A2O system had the advantages of good treatment effect, low cost and easy practical application. Therefore, the biofilm-enhanced A2O system has wide application potential in aquaculture wastewater treatment.
Conclusion
The nitrifying bacteria, denitrifying and phosphorus accumulating bacteria biofilms were used to enhance the A2O system to evaluate the removal efficiency for the aquaculture wastewater. The results showed that Biofilm-enhanced A2O system can effectively remove pollutants from aquaculture wastewater. The removal efficiencies of CODMn, NH4+-N, TN and TP in A2O system were approximately 86.3%-90.8%, 97.7%-99.5%, 94.6%-95.2% and 97.0%-98.1%. The results further showed that CODMn, NH4+-N, and TN were mainly removed in anaerobic tank and anoxic tank, while TP was mainly removed in the anoxic tank and oxic tank. This study provides a practical method for aquaculture wastewater treatment.
Data availability statement
The original contributions presented in the study are included in the article/supplementary material. Further inquiries can be directed to the corresponding author.
Author contributions
XM: Writing – review & editing, Data curation, Project administration. RuY: Formal analysis, Writing – review & editing. CY: Formal analysis, Writing – review & editing. CC: Writing – original draft, Writing – review & editing. JZ: Resources, Writing – review & editing. CL: Writing – review & editing. XW: Writing – review & editing. SC: Writing – review & editing. ReY: Writing – review & editing. BZ: Writing – original draft.
Funding
The author(s) declare financial support was received for the research, authorship, and/or publication of this article. This research was supported by the Project Funded by the Priority Academic Program Development of Jiangsu Higher Education Institutions (PAPD), Study on Removing Heavy Metal Pollution in Porphyra Cultivation Areas (Z2009050); Ecological Effects of Characteristic Pollutants in Typical Seafood in Haizhou Bay (2013HS011); the Natural Science Foundation of Jiangsu Province (No. BK20220682); the Project funded by China Postdoctoral Science Foundation (2022M721398); the Project funded by Postdoctoral Science Foundation of Lianyungang (LYG20230005); Postgraduate Research & Practice Innovation Program of Jiangsu Province; the Doctoral Program of Entrepreneurship and Innovation in Jiangsu Province (JSSCBS20221618).
Conflict of interest
The authors declare that the research was conducted in the absence of any commercial or financial relationships that could be construed as a potential conflict of interest.
Publisher’s note
All claims expressed in this article are solely those of the authors and do not necessarily represent those of their affiliated organizations, or those of the publisher, the editors and the reviewers. Any product that may be evaluated in this article, or claim that may be made by its manufacturer, is not guaranteed or endorsed by the publisher.
References
Abyar H., Younesi H., Bahramifar N., Zinatizadeh A. (2018). Biological CNP removal from meat-processing wastewater in an innovative high rate up-flow A2O bioreactor. Chemosphere 213, 197–204. doi: 10.1016/j.chemosphere.2018.09.047
Chen X., Zhang Q., Zhu Y., Zhao T. (2022). Response of rotating biological contactor started up by heterotrophic nitrification-aerobic denitrification bacteria to various C/N ratios. Chemosphere 291, 133048. doi: 10.1016/j.chemosphere.2021.133048
Choi S., Yoom H., Son H., Seo C., Kim K., Lee Y., et al. (2022). Removal efficiency of organic micropollutants in successive wastewater treatment steps in a full-scale wastewater treatment plant: Bench-scale application of tertiary treatment processes to improve removal of organic micropollutants persisting after secondary treatment. Chemosphere 288, 132629. doi: 10.1016/j.chemosphere.2021.132629
Dai H., Sun Y., Wan D., Abbasi H., Guo Z., Geng H., et al. (2022). Simultaneous denitrification and phosphorus removal: a review on the functional strains and activated sludge processes. Sci. Total Environ. 835, 155409. doi: 10.1016/j.scitotenv.2022.155409
Díaz A., Franco L., Estrany F., Delvalle L., Puiggalí J. (2014). Poly(butylene azelate-co-butylene succinate) copolymers: Crystalline morphologies and degradation. Polymer Degradation Stability 99, 80–91. doi: 10.1016/j.polymdegradstab.2013.11.022
Fong C., Smith T., Muthukrishnan R., Fong P. (2023). A persistent green macroalgal mat shifts ecological functioning and composition of associated species on an Eastern Tropical Pacific coral reef. Mar. Environ. Res. 188, 105952. doi: 10.1016/j.marenvres.2023.105952
Gallardo-Altamirano M. J., Maza-Márquez P., Montemurro N., Rodelas B., Osorio F., Pozo C. (2019). Linking microbial diversity and population dynamics to the removal efficiency of pharmaceutically active compounds (PhACs) in an anaerobic/anoxic/aerobic (A2O) system. Chemosphere 233, 828–842. doi: 10.1016/j.chemosphere.2019.06.017
Gallardo-Altamirano M. J., Maza-Márquez P., Pérez S., Rodelas B., Pozo C., Osorio F. (2021). Fate of pharmaceutically active compounds in a pilot-scale A2O integrated fixed-film activated sludge (IFAS) process treating municipal wastewater. J. Environ. Chem. Eng. 9, 105398. doi: 10.1016/j.jece.2021.105398
Gao Y., Peng Y., Zhang J., Wang S., Guo J., Liu Y. (2011). Biological sludge reduction and enhanced nutrient removal in a pilot-scale system with 2-step sludge alkaline fermentation and A2O process. Bioresource Technol. 102, 4091–4097. doi: 10.1016/j.biortech.2010.12.051
Guo H., Yao H., Huang Q., Li T., Show D., Ling M., et al. (2023). Anaerobic–anoxic–oxic biological treatment of high-strength, highly recalcitrant polyphenylene sulfide wastewater. Bioresource Technol. 371, 128640. doi: 10.1016/j.biortech.2023.128640
Hao Z., Ali A., Ren Y., Su J., Wang Z. (2022). A mechanistic review on aerobic denitrification for nitrogen removal in water treatment. Sci. Total Environ. 847, 157452. doi: 10.1016/j.scitotenv.2022.157452
Huang L., Li Z., Wang G., Hou Y., Han J., Yi F. (2022). A2O–MBR–BAF–O3 process for treating high organic wastewater with high ammonia nitrogen. Biochem. Eng. J. 186, 108574. doi: 10.1016/j.bej.2022.108574
Li J., Xu K., Liu T., Bai G., Liu Y., Wang C., et al. (2020). Achieving stable partial nitritation in an acidic nitrifying bioreactor. Environ. Sci. Technol. 54, 456–463. doi: 10.1021/acs.est.9b04400
Li L., Zhang J., Tian Y., Sun L., Zuo W., Li H., et al. (2019). A novel approach for fouling mitigation in anaerobic-anoxic-oxic membrane bioreactor (A2O-MBR) by integrating worm predation. Environ. Int. 127, 615–624. doi: 10.1016/j.envint.2019.02.041
Ma J., Cao Y., Fan L., Xie Y., Zhou X., Ren Q., et al. (2023). Degradation characteristics of polybutylene adipate terephthalic acid (PBAT) and its effect on soil physicochemical properties: A comparative study with several polyethylene (PE) mulch films. J. Hazard. Mater. 456, 131661.
Ma X., Li X., Li J., Ren J., Chi L., Cheng X. (2021). Iron-carbon could enhance nitrogen removal in Sesuvium portulacastrum constructed wetlands for treating mariculture effluents. Bioresource Technol. 325, 124602. doi: 10.1016/j.biortech.2020.124602
Marazuela M., Formentin G., Erlmeier K., Hofmann T. (2023). Seasonal biodegradation of the artificial sweetener acesulfame enhances its use as a transient wastewater tracer. Water Res. 232, 119670. doi: 10.1016/j.watres.2023.119670
Na C., Zhang Y., Quan X., Chen S., Liu W., Zhang Y. (2017). Evaluation of the detoxification efficiencies of coking wastewater treated by combined anaerobic-anoxic-oxic (A2O) and advanced oxidation process. J. Hazard. Mater. 338, 186–193. doi: 10.1016/j.jhazmat.2017.05.037
Nunzia P., Arjan P., Rob M., Maartje A., Greet C., Antonie H., et al (2021). Ammonia oxidation at pH 2.5 by a new gammaproteobacterial ammonia-oxidizing bacterium. ISME J. 15, 1150–1164. doi: 10.1038/s41396-020-00840-7
Peng S., Deng S., Li D., Xie B., Yang X., Lai C., et al. (2020). Iron-carbon galvanic cells strengthened anaerobic/anoxic/oxic process (Fe/C-A2O) for high-nitrogen/phosphorus and low-carbon sewage treatment. Sci. Total Environ. 722, 137657. doi: 10.1016/j.scitotenv.2020.137657
Rajesh Banu J., Uan D. K., Yeom I.-T. (2009). Nutrient removal in an A2O-MBR reactor with sludge reduction. Bioresource Technol. 100, 3820–3824. doi: 10.1016/j.biortech.2008.12.054
Ravishankar H., Moazzem S., Jegatheesan V. (2019). Performance evaluation of A2O MBR system with graphene oxide (GO) blended polysulfone (PSf) composite membrane for treatment of high strength synthetic wastewater containing lead. Chemosphere 234, 148–161. doi: 10.1016/j.chemosphere.2019.05.264
Ren J., Ma H., Liu Y., Ruan Y., Han R. (2021). Characterization of a novel marine aerobic denitrifier Vibrio spp. AD2 for efficient nitrate reduction without nitrite accumulation. Environ. Sci. Pollut. Res. 28, 30807–30820. doi: 10.1007/s11356-021-12673-8
Sun F., Sun B., Hu J., He Y., Wu W. (2015). Organics and nitrogen removal from textile auxiliaries wastewater with A2O-MBR in a pilot-scale. J. Hazard. Mater. 286, 416–424. doi: 10.1016/j.jhazmat.2015.01.031
Tang G., Zheng X., Li X., Liu T., Pan B. (2020). Variation of effluent organic matter (EfOM) during anaerobic/anoxic/oxic (A2O) wastewater treatment processes. Water Res. 178, 115830. doi: 10.1016/j.watres.2020.115830
Tang L., Su C., Chen Y., Xian Y., Hui X., Ye Z., et al. (2021). Influence of biodegradable polybutylene succinate and non-biodegradable polyvinyl chloride microplastics on anammox sludge: Performance evaluation, suppression effect and metagenomic analysis. J. Hazard. Mater. 401, 123337. doi: 10.1016/j.jhazmat.2020.123337
Wang H., Cui D., Han J., Cheng H., Wang A. (2019). A2O-MBR as an efficient and profitable unconventional water treatment and reuse technology: A practical study in a green building residential community. Resources Conserv. Recycling 150, 104418. doi: 10.1016/j.resconrec.2019.104418
Wang Z., Xu X., Gong Z., Yang F. (2012). Removal of COD, phenols and ammonium from Lurgi coal gasification wastewater using A2O-MBR system. J. Hazard. Mater. 235-236, 78–84. doi: 10.1016/j.jhazmat.2012.07.012
Wu T., Yang S. S., Zhong L., Pang J., Zhang L., Xia X., et al. (2023). Simultaneous nitrification, denitrification and phosphorus removal: What have we done so far and how do we need to do in the future? Sci. Total Environ. 856, 158977. doi: 10.1016/j.scitotenv.2022.158977
Xi H., Zhou X., Arslan M., Luo Z., Wei J., Wu Z., et al. (2022). Heterotrophic nitrification and aerobic denitrification process: Promising but a long way to go in the wastewater treatment. Sci. Total Environ. 805, 150212. doi: 10.1016/j.scitotenv.2021.150212
Xiang Z., Chen X., Bai J., Li B., Li H., Huang X. (2023). Bioaugmentation performance for moving bed biofilm reactor (MBBR) treating mariculture wastewater by an isolated novel halophilic heterotrophic nitrification aerobic denitrification (HNAD) strain (Zobellella B307). J. Environ. Manage. 325, 116566. doi: 10.1016/j.jenvman.2022.116566
Xiao L., Zhao Z., Ma Z., Chen J., Song Z. (2020). Immobilization of Rhodopseudomonas palustris P1 on glass pumice to improve the removal of NH4+ -N and NO2- -N from aquaculture pond water. Biotechnol. Appl. Biochem. 67, 323–329.
Xiao K., Zhou L., He B., Qian L., Wan S., Qu L. (2016). Nitrogen and phosphorus removal using fluidized-carriers in a full-scale A 2 O biofilm system. Biochem. Eng. J. 115, 47–55. doi: 10.1016/j.bej.2016.08.004
Yaseen A., Assad I., Sofi M., Hashmi M., Bhat S. (2022). A global review of microplastics in wastewater treatment plants: Understanding their occurrence, fate and impact. Environ. Res. 212, 113258. doi: 10.1016/j.envres.2022.113258
Yue X., Liu H., Wei H., Chang L., Gong Z., Zheng L., et al. (2023). Reactive and microbial inhibitory mechanisms depicting the panoramic view of pH stress effect on common biological nitrification. Water Res. 231, 119660. doi: 10.1016/j.watres.2023.119660
Zeng W., Li L., Yang Y., Wang S., Peng Y. (2010). Nitritation and denitritation of domestic wastewater using a continuous anaerobic–anoxic–aerobic (A2O) process at ambient temperatures. Bioresource Technol. 101, 8074–8082. doi: 10.1016/j.biortech.2010.05.098
Zhang C., Guisasola A., Oehmen A., Baeza J. (2023). Benefits and drawbacks of integrating a side-stream sludge fermenter into an A2O system under limited COD conditions. Chem. Eng. J. 468, 143700. doi: 10.1016/j.cej.2023.143700
Zhang H., Li H., Ma M., Ma B., Liu H., Niu L., et al. (2023a). Nitrogen reduction by aerobic denitrifying fungi isolated from reservoirs using biodegradation materials for electron donor: Capability and adaptability in the lower C/N raw water treatment. Sci. Total Environ. 864, 161064. doi: 10.1016/j.scitotenv.2022.161064
Zhang H., Yang W., Ma B., Liu X., Huang T., Niu L., et al. (2023b). Aerobic denitrifying using actinobacterial consortium: Novel denitrifying microbe and its application. Sci. Total Environ. 859, 160236. doi: 10.1016/j.scitotenv.2022.160236
Zhang L., Zhang M., Guo J., Zheng J., Chen Z., Zhang H., et al. (2019). Effects of K+ salinity on the sludge activity and the microbial community structure of an A2O process. Chemosphere 235, 805–813. doi: 10.1016/j.chemosphere.2019.06.137
Keywords: biofilm-enhanced A2O system, mariculture wastewater, total nitrogen, total phosphorus, PBS
Citation: Ma X, Yin R, Yang C, Cai C, Zhao J, Lv C, Wang X, Chen S, Ren J and Zhang B (2024) Application of a biofilm-enhanced A2O system in the treatment of wastewater from mariculture. Front. Mar. Sci. 11:1408774. doi: 10.3389/fmars.2024.1408774
Received: 28 March 2024; Accepted: 19 April 2024;
Published: 22 May 2024.
Edited by:
Stephen J. Newman, Western Australian Fisheries and Marine Research Laboratories, AustraliaReviewed by:
Chun Wang, Beijing Technology and Business University, ChinaCe Shi, Ningbo University, China
Copyright © 2024 Ma, Yin, Yang, Cai, Zhao, Lv, Wang, Chen, Ren and Zhang. This is an open-access article distributed under the terms of the Creative Commons Attribution License (CC BY). The use, distribution or reproduction in other forums is permitted, provided the original author(s) and the copyright owner(s) are credited and that the original publication in this journal is cited, in accordance with accepted academic practice. No use, distribution or reproduction is permitted which does not comply with these terms.
*Correspondence: Bingzhi Zhang, zhangbz@jou.edu.cn