- 1Chioggia Hydrobiological Station “Umberto D’Ancona”, Department of Biology, University of Padova, Unità Operativa CoNISMa (Uo CoNISMa), Chioggia, Italy
- 2Dipartiment of Biological, Geological and Environmental Science (BIGEA), University of Bologna, Unità Organizzativa di Sede (UOS) Ravenna, Uo CoNISMa, Ravenna, Italy
- 3Dipartiment of Earth and Marine Sciences (DiSTeM), University of Palermo, Palermo, Italy
- 4National Research Foundation - South African Institute for Aquatic Biodiversity, Makhanda, South Africa
- 5Department of Ichthyology & Fisheries Science, Rhodes University, Makhanda, South Africa
- 6Interdipartimental Center of Research in Environmental Science, University of Bologna, Ravenna, Italy
- 7NBFC, National Biodiversity Future Center, Palermo, Italy
Marine coastal habitats are often characterized by strong gradients of anthropogenic disturbance such as pollution, typically most severe at urban waterfronts. These variations create stress for local organisms leading to their distribution along the disturbance as a function of their tolerance. non-indigenous species (NIS) are considered more tolerant to anthropogenic disturbances than their native counterparts, thriving in urban areas, where native species are sparser. It is however not yet entirely clear if these distribution patterns are due to larval behavior and preferential settlement or post-settlement processes. In the present study, we investigated the abundance of adults and settlers of two native (Ostrea edulis, Mytilus galloprovincialis) and two non-indigenous (Magallana gigas, Xenostrobus securis) bivalves along a strong marine urban environmental gradient. Oysters had sparse abundances of both adults and settlers at all sites, with no obvious distributional gradients. The two mussel species showed different settler-adult distributions along the gradient. Both settlers and adults of the native mussel M. galloprovincialis strongly decreased moving from the outermost periurban site to the innermost urban site, consistent with preferential settlement of larvae according to environmental conditions. The non-indigenous adult mussel X. securis showed a distribution pattern opposite to that of M. galloprovincialis, markedly increasing in abundance from the outermost to the innermost site. This was not paralleled by the distribution of X. securis settlers, which established over a larger area, including sites where adults were essentially absent. The mismatch between settler and adult distributions for X. securis suggests that post-settlement factors dictate spatial adult distribution.
1 Introduction
Coastal urbanization is on the rise, with urban and periurban marine areas currently making up more than 1.5% of the global exclusive economic zones (Airoldi et al., 2021; Bugnot et al., 2021). Urbanization results in the permanent modification of abiotic and biotic conditions around artificial structures (Dafforn et al., 2015; Bugnot et al., 2021), often generating a variety of disturbance sources (Todd et al., 2019). Marine urban areas and other anthropic (i.e. manmade) habitats are in fact typically characterized by high levels of chemical pollution (Lam and Todd, 2013; Gauff et al., 2022), physical disturbance (Atalah et al., 2007), sediment load and turbidity (Airoldi, 2003), and increased temperature variability (Menniti et al., 2020) compared to non-urban areas. This set of specific conditions largely influences the distribution and functionality of organisms in these habitats.
The fouling communities colonizing the most urbanized artificial habitats differ from those of less impacted natural habitats, being characterized by distinct faunal and floral communities, lower species and genetic diversity (Fauvelot et al., 2009; Megina et al., 2016), altered ecological interactions (Klein et al., 2011) and functions (Dafforn et al., 2015) and a notorious prevalence of non-indigenous species (NIS) over native species (Mineur et al., 2012; Rondeau et al., 2022). NIS thrive in marine urban habitats due to their often-higher performance in stressful environments compared to native species (McMahon, 2002; Lenz et al., 2011). The underlying drivers of native and NIS distributions are, however, not yet fully understood (Airoldi et al., 2015; Gauff et al., 2022; Rondeau et al., 2022). Identifying the ecological forces and processes shaping marine biodiversity along gradients of urbanization is considered a priority for a better planning, design, and management of the most urbanized marine spaces, such as ports and city waterfronts (Airoldi et al., 2021), especially since NIS are considered a critical biosecurity issue (Pyšek et al., 2020), causing loss of biodiversity and ecosystem services (Walsh et al., 2016), species extinctions (Blackburn et al., 2019), and significant economic loss (Diagne et al., 2021).
Disturbance in marine urban spaces could be attenuated at the most flushed areas, where the hydrodynamic conditions create dilution effects (Schiff et al., 2007; Menniti et al., 2020). Flush attenuation induces disturbance gradients potentially affecting NIS prevalence (Floerl and Inglis, 2003; Gauff et al., 2022; Rondeau et al., 2022) and propagule pressure, which tends to be correlated to greater settlement and invasion success (Simberloff, 2009). Many marine taxa show active larval behaviors ranging from substrate preferences (Pinochet et al., 2020) to actively seeking out biofilm and/or conspecifics (Scheltema et al., 1981; Von Der Meden et al., 2010), which could vastly impact recruitment patterns. However, whether the distribution of NIS and native species along urban gradients is related to factors affecting the settlement or subsequent recruitment of propagules is not clear yet.
We took advantage of the urban gradients along the channel harbor of Ravenna, North Adriatic, Italy to explore whether the distribution of target adult NIS and native species colonizing artificial structures is related or not to settlement patterns (Walters, 1992). We quantified how the gradient of urbanization affected a range of relevant environmental parameters, and then compared the distributions of adult and settlers of two native and two functionally similar NIS of the same family of bivalves along that environmental gradient. Based on previous knowledge (see above), we expected higher abundances of the native species at the most flushed periurban entrance of the harbor canal and higher abundance of the two NIS at the most confined inner waterfront. We hypothesized that these gradients in adult distribution would be paralleled by settler abundances as environmental constraints should lead to preferential settlement in suitable habitat. Alternatively, a larger settlement potential compared to the final adult distribution would suggest that other post-settlement factors such as predation or competition (often overlooked in marine urban areas) could better explain the NIS adult distribution.
2 Materials and methods
2.1 Sampling site
The Ravenna harbor (Figure 1) is one of the largest industrial and commercial ports in Italy (Airoldi et al., 2016). It is an 11 km long channel going from the highly urbanized Ravenna city center, through a large industrial complex to the Adriatic Sea, where urban influence is diluted by high water circulation. Its entrance is protected by 2 large 2.4 km long converging jetties. The connection with the lagoon increases the tidal water flow, reducing the risk of siltation at the harbor entrance. We selected five study sites (Figure 1) along the inner-outer gradient representative of the different levels of urbanization and anthropogenic pressures, as well as different levels of confinement: the innermost, most confined, fully urban site (S1); three intermediate sites with decreasing intensity of urbanization and increased flushing (S2, S3, S4); and the outermost, more flushed, periurban site S5, which is on a long breakwater leading out to the sea and thus more distant from the urban center and its disturbances (for a definition of urban and periurban, see Airoldi et al., 2021). Due to access prohibition at the industrial harbor S3 and S4 are more distantly spaced than other sites.
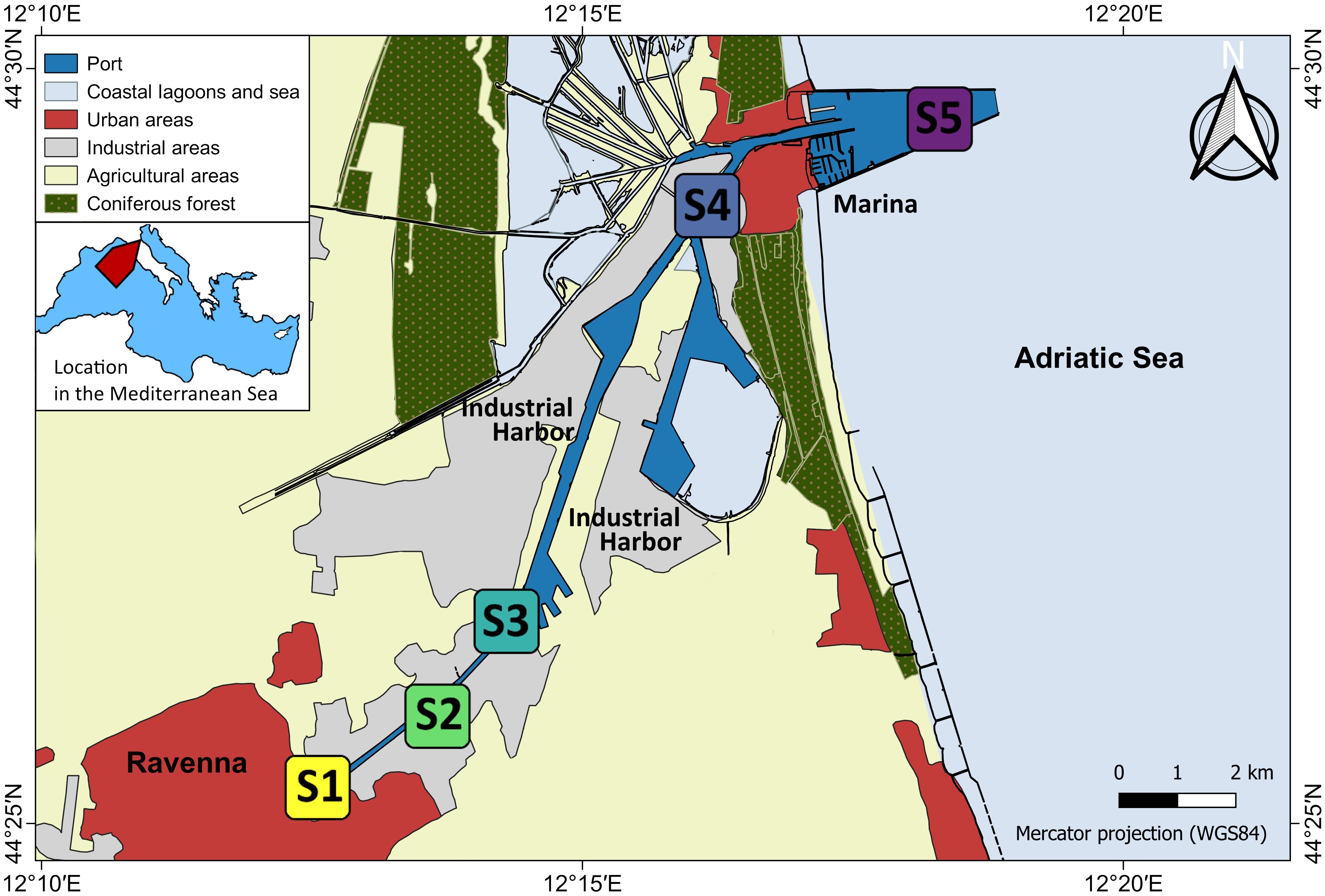
Figure 1 Map of Ravenna harbor, Italy. The five study sites S1 - S5 are indicated. Land use map source: 2017 land use of Emilia-Romagna Region; Corine Land Cover classification; coordinate system: WGS84. Geoportal of Emilia Romagna Region; Mediterranean vector map modified from Wikimedia Commons Mediterranean_map.svg.
2.2 Study species
We focused on four bivalve species with similar ecological niches and functions (mussels and oysters) occurring on the harbor seawalls, two of which were native and two of which were non-indigenous species (NIS). We expected the NIS to favor the innermost parts of the canal as seemingly specifically adapted to and dominant in urban habitats (Gauff et al., 2022; Rondeau et al., 2022). Mytilus galloprovincialis Lamarck, 1819 is a Mediterranean native species (Montes et al., 2021). Its non-indigenous counterpart Xenostrobus securis (Lamarck, 1819), is an Australian mussel species that was introduced in the north-western Adriatic Sea in 1994 (Garci et al., 2007). The native oyster Ostrea edulis Linnaeus, 1758 is a pan-European oyster species that was originally abundant from the low intertidal into water depths of about 80 m, but it was replaced by the Pacific oyster Magallana gigas (Thunberg, 1793) introduced for aquaculture purposes. At the end of the dispersal phase, all four species colonize intertidal and shallow subtidal habitats, including artificial ones (Bataller et al., 1999; Gomes et al., 2016; Stagličić et al., 2020; Montes et al., 2021).
2.3 Sampling design and treatment
At each study site, environmental conditions were monitored through time, mussel and oyster adults were sampled once, and their settlement was followed through the entire study. Environmental conditions were monitored at each site from March to July 2017 on a two-week basis, for a total of eight times (T0 – T7). Seawater pH, temperature, salinity, chlorophyl-a, dissolved oxygen, and turbidity were measured using a multiparametric probe (Hanna Instruments Hi 9828), and water samples were collected for subsequent analysis of ammonia, nitrate, nitrite, and phosphate in the laboratory. Six replicates of all variables were collected, except for T0, where only one replicate was taken at each site.
At T0 only, six replicated 20 × 20 cm samples were randomly scraped at each site to quantify the abundances of the four target species. Due to diving permitting constraints, sampling was conducted from a small boat, selecting days with low tidal conditions (average tidal amplitude is ~ 80 cm), to reach and sample the low intertidal seawall habitats (~0.1-0.3 m above Mean Low Waterlevel). Each sample was preserved in plastic bags and rapidly processed in the laboratory. Abundance was defined as the number of live specimens belonging to one of the four target species, identified under a steromicroscope (Garci et al., 2007).
The scraped surfaces at each site were used to deploy six replicated Artificial Substrate Units (ASU), consisting of polypropylene kitchen scouring pads serving as bivalve spat-collectors (see Porri et al., 2006). Each ASU was retrieved and replaced every 2 weeks from March (T1) to July (T7), for a total of n = 210 samples (6 replicates per 5 sites per 7 times). Upon retrieval, each ASU was placed into a sterile plastic bag and processed in the laboratory following the protocol described by Porri et al. (2006). Bivalve settlers (~ 360 µm; Porri et al., 2006) were sorted out and counted under a stereomicroscope (Nikon SMZ 15000), and further identified and quantified by molecular approaches. On replicates containing 1 to 4 settlers, individuals were barcoded for species identification, while on replicates with more than 4 settlers, we used a metabarcoding approach. In both cases, the cytochrome oxidase subunit I (COI) gene was targeted, and the number of reads was considered as proxy for settler abundance. The detailed protocol for molecular analyses is described in the Supplementary Material.
2.4 Statistical analysis
All statistical analyses were carried out in ‘R’ (R Core Team, 2020) and figures obtained via ‘ggplot2’ (Wickham, 2016). All logistic regressions were applied and interpreted according to Fox and Weisberg (2018).
Each environmental variable was plotted for each site and time (See Supplementary Material, Supplementary Figures 1–10A). To investigate potential environmental gradients across sites, the environmental variables were further scaled and analyzed for each time using Principal Component Analysis (PCA) via the ‘vegan’ package (Oksanen et al., 2018; Supplementary Figure 11). Some PCAs required flipping to align the site distribution among different time steps. The centroid PC1 value was then extracted for each site at each time, and the overall gradient was represented through this PC1 value as a function of the site. Further details can be found in the Supplementary Material. Subsequently, a logistic regression was employed to test for the effect of site (ordered discrete factor) on the PC1 value.
The abundances (number of adult individuals) of M. galloprovincialis (Native), X. securis (NIS), O. edulis (Native), and M. gigas (NIS) per sample were box-plotted for each site at T0, and logistic regressions (with site as ordered discrete factor) were used to identify their distributions among sites. If the overall model for any species was significant, the fitted model with all terms of the logistic regression (i.e., linear L, quadratic Q, cubic C, 4th degree) was superimposed onto the box plot distribution of the species.
Similarly, the numbers of newly settled larvae were plotted and analyzed as for the adults. As virtually no oyster settlers were found (see Results), these analyses were run only for mussel settlers. Since settlers were sampled seven times, we used a logistic linear mixed-effects model (LMM) via REML from the ‘lme4’ package (Bates et al., 2015) to account for time as random factor.
3 Results
Environmental factors varied across sites and times (Supplementary Figures 1–11). In general, the urban innermost site S1 was characterized by the highest temperature (1 to 3 degrees higher compared to the most external site S5, depending on the time of the year), salinity, and turbidity, which decreased moving towards the outermost periurban site, while nutrient levels tended to peak at the most industrial sites S3 and S4. When all the environmental data were combined, the PCAs for each time showed that overall, a temporally stable environmental gradient structure was present, with a high differentiation across sites from S1 to S5 (Supplementary Figure 11). The PC1, based on the 10 environmental variables, explained between 31.2% and 50.2% of the data distribution, and the boxplot of the PC1 centroid value showed an ordered structure of the sites (Figure 2). The logistic regression, testing for the effect of site on the PC1 centroid value, revealed a significant linear decrease (L: t-val. = -9.06; Pr (> |t|) < 0.001; Figure 2) with deceleration moving towards the outermost site (Q: t-val. = 2.714; Pr (> |t|) = 0.01; Figure 2). The overall model significance was high (Adj. R² = 0.7; F-value = 23.4; 35 DF; p < 0.001).
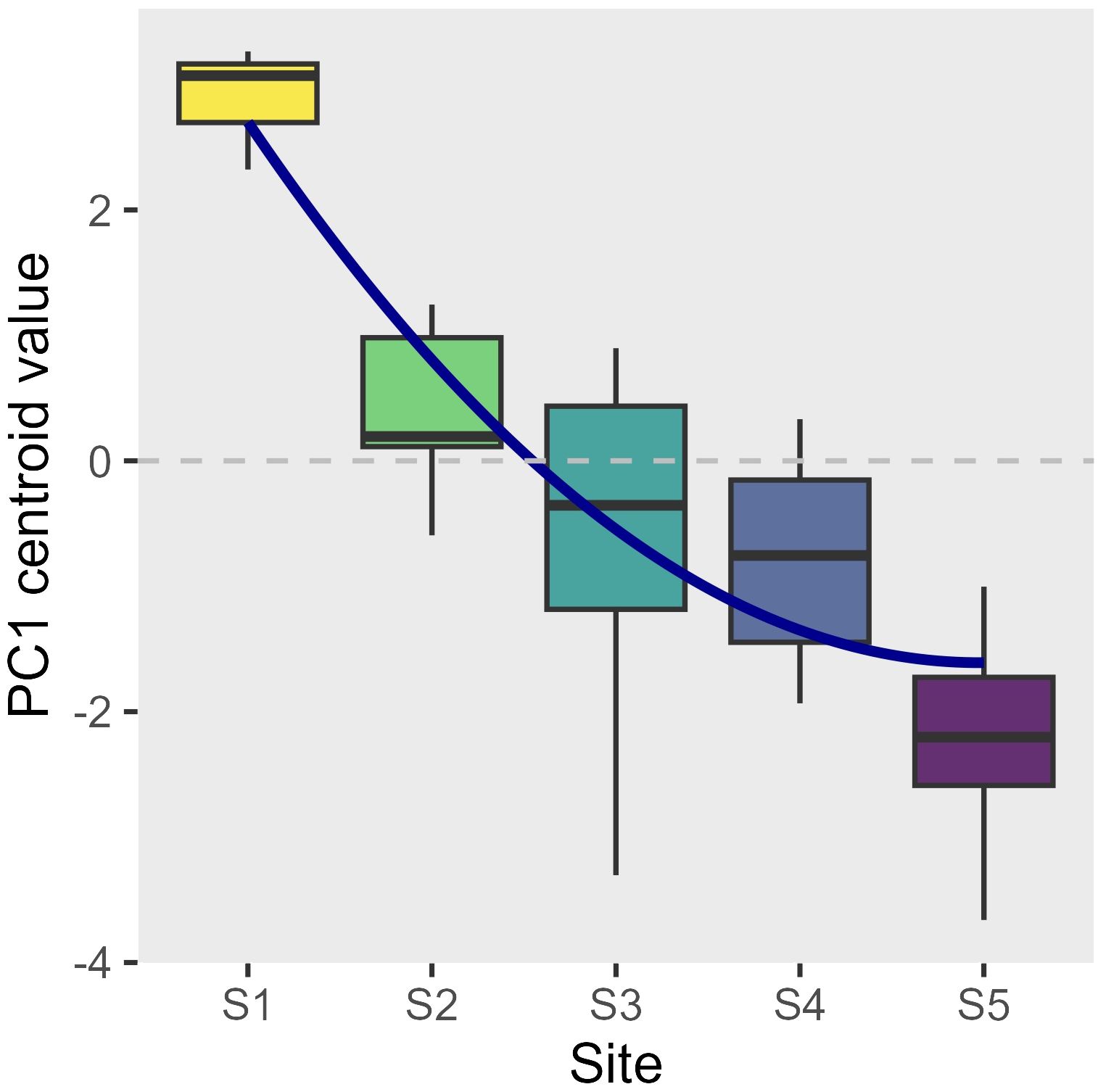
Figure 2 Boxplot of the PC1 centroid values (proxy of a comprehensive environmental gradient derived from 10 different parameters when projected into a PCA for each sampling time; see Supplementary Figure 11 for more detail) at each of 5 sites (S1 innermost urban to S5 outermost periurban). The logistic regression estimate (Supplementary Table 1A) is superimposed (Adj. R² = 0.7; p < 0.001).
Oysters were sparse at all study sites, with O. edulis virtually absent in most samples, and M. gigas sparsely occurring at only few plots at sites S2, S3, and S4 (Supplementary Figure 12). Like adult abundances, oyster settler abundances were unexpectedly low/absent based on molecular data. During the 7 sampling times, a total of only two settlers of M. gigas and no settlers of O. edulis were found on the ASUs, which prevented further analyses for these species.
The adults of the two mussel species (M. galloprovincialis and X. securis) significantly varied across sites, showing opposite patterns along the gradient (Adj. R² > 0.66; F-value > 15.27; 25 DF; p < 0.001; Figures 3A, B; Table 1). A significant increase of the native M. galloprovincialis was observed moving from the innermost waterfront to the outermost periurban areas (L: t-val. = 9.5; Pr (> |t|) < 0.001; Figure 3A; Table 1) accelerating (Q: t-val. = 6.47; Pr (> |t|) < 0.001; Figure 3A; Table 1) towards the outermost periurban site. In this model, the cubic term was significant (C: t-val. = 3.26; Pr (> |t|) = 0.003; Figure 3; Table 1) indicating the presence of a threshold at which the acceleration starts centered between S3 and S4. For the non-indigenous X. securis, the distribution of adults was opposite to that of M. galloprovincialis, with a significant linear decrease from the innermost waterfront to the outermost periurban areas (L: t-val. = -7.2; Pr (> |t|) < 0.001; Figure 3B; Table 1).
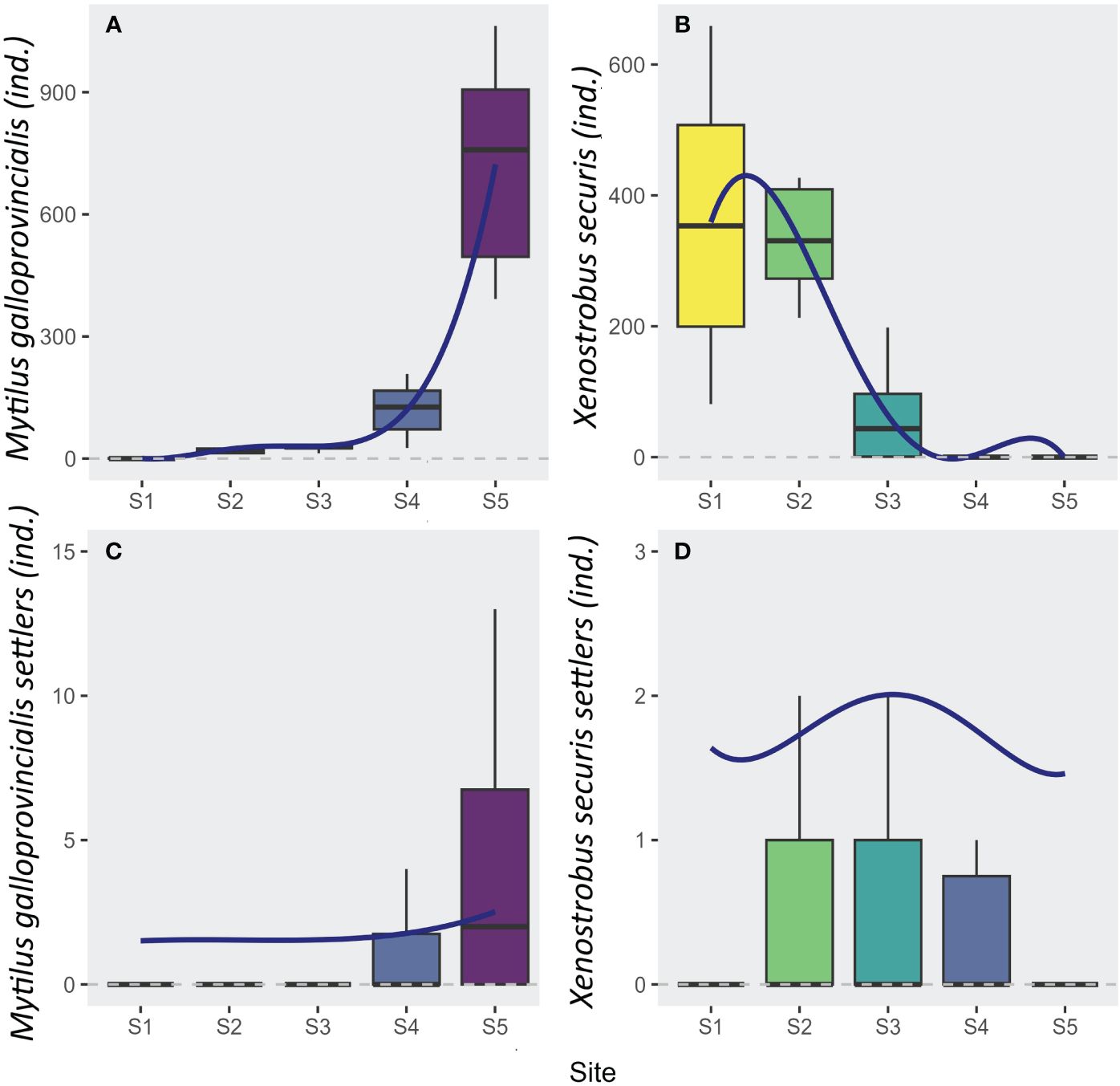
Figure 3 Boxplots of the abundances (number of individuals per sample) of (A) adults of Mytilus galloprovincialis (Native), (B) adults of Xenostrobus securis (NIS), (C) settlers of Mytilus galloprovincialis (Native), and (D) settlers of Xenostrobus securis (NIS) at each site. The settler abundances are an average over 7 sampling times, from March to July 2017. Logistic regression estimates (see Table 1) are superimposed when a model was significant.
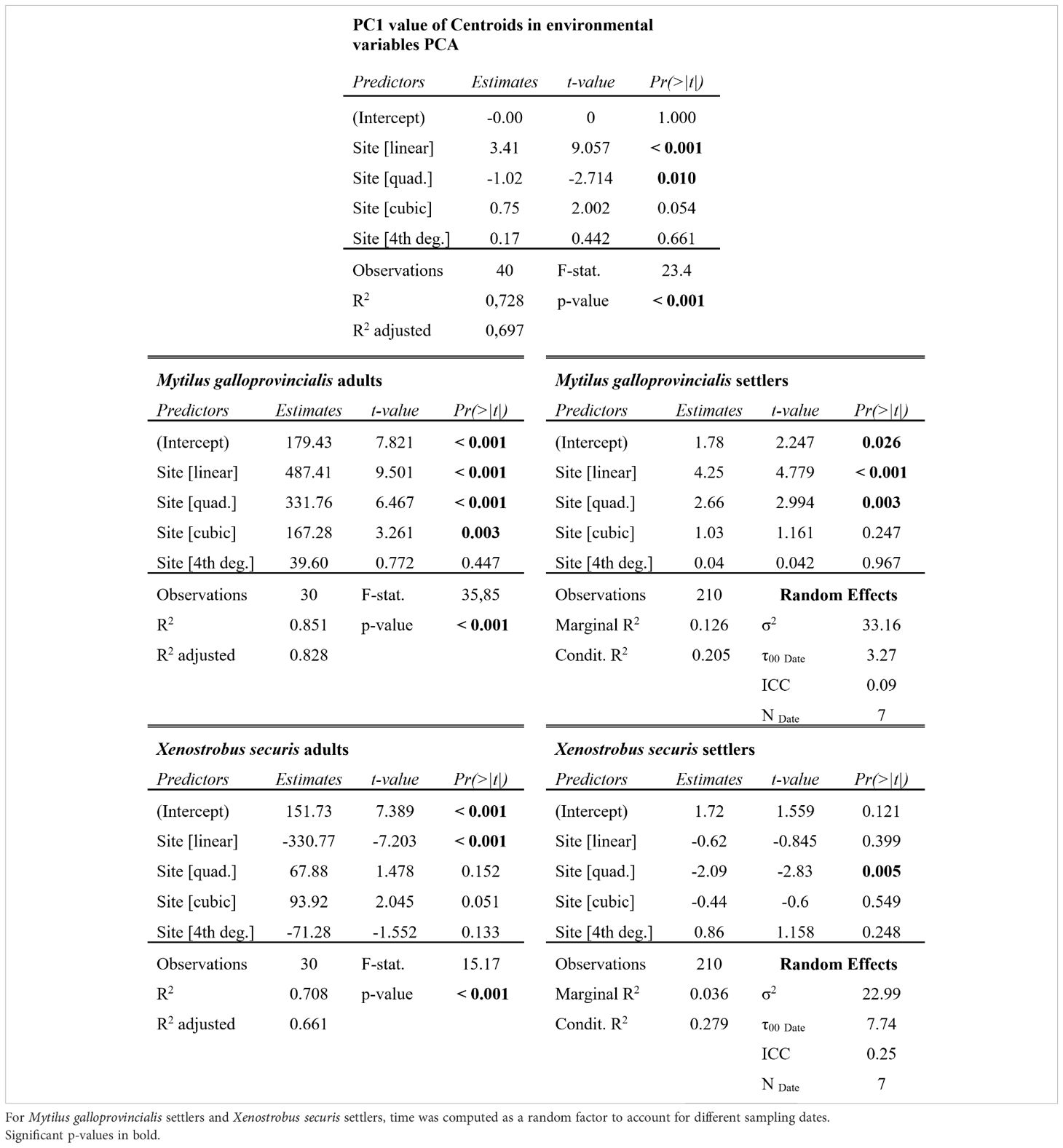
Table 1 Summary of the logistic regressions and the linear mixed effect models testing for the effect of site (ordered discrete factors) on different response variables of interest.
The distribution of M. galloprovincialis settlers over 7 sampling times paralleled the adult distribution, while there was a notable mismatch between adult and settler abundance for X. securis. Like for adults, M. galloprovincialis settlers had an increasing (L: t-val. = 4.78; Pr (> |t|) < 0.001; Figure 3C; Table 1) and accelerating (Q: t-val. = 2.99; Pr (> |t|) = 0.003; Figure 3C; Table 1) abundance towards the outermost periurban site. For X. securis, on the other hand, only the quadratic polynomial had significant effect (Q: t-val. = -2.83; Pr (> |t|) = 0.005; Figure 3D; Table 1) reflecting maximum abundances in the middle portion of the gradient and very low abundances at the periurban site. This notably deviates from the adult abundances, which were highest in the two innermost sites. This mismatch is especially striking for the innermost waterfront site, which had the highest adult abundances, but second lowest settler abundances (after the periurban site).
4 Discussion
In the present study, we investigated whether a gradient of increasing urbanization structured the distribution and abundances of adult populations of four target bivalves and their settlers. The periurban-urban gradient observed at the harbor of Ravenna was reflected in a temporally stable, significant gradient in relevant environmental parameters, including temperature, salinity, turbidity, and nutrient concentration, with consistent dissimilarities among the outermost and inner sites (Figure 2). This occurred despite strong temporal fluctuations in the individual parameters (Supplementary Figures 1–10). Only two of the four tested adult bivalve species were significantly influenced by this gradient, however, the distribution profiles align with our hypothesis: the NIS was more abundant in the inner parts of the canal, while the native species dominated the entrance. For settlers, only one species (M. galloprovincialis) exhibited a distribution profile that overlapped with adult distribution, indicating preferential settlement in habitats suitable for recruitment. Xenostrobus securis displayed a notable mismatch between adult and settler abundances, potentially linked to more indiscriminate recruitment owing to its resistance to disturbance.
Environmental conditions in the canal changed between March and July, as expected for both natural and urban areas (Lacroix et al., 2017). However, in accordance with hypotheses focusing on the importance of hydrodynamics and water exchange with the open sea (Floerl and Inglis, 2003; Schiff et al., 2007; Menniti et al., 2020), we observed a gradient structure in environmental conditions. This gradient was found to be temporally stable for individual parameters such as temperature (T S1 > T S5; Supplementary Figure 1), but also for the multivariate distribution of combined environmental conditions, with consistently high dissimilarity between S1 and S5 on the PC1 axis (Figure 2; Supplementary Figure 11). This gradient structure was however not entirely linear, as a slight deceleration trend for differences between sites was observed between the middle and the outermost site (Figure 2; Table 1), indicating that environmental conditions exhibit steeper spatial variations at the innermost part of the canal.
In Ravenna harbor, oysters showed surprisingly low abundance compared to similar marine urban areas in the northern Adriatic (see photographic comparison with an urban substrate from the Venice Lagoon: Supplementary Figure 12). In total, only 35 live adult oysters were sampled, with the majority (27) being M. gigas. Oyster settlers were virtually absent during the study, despite the study period coinciding with their spawning period (Bataller et al., 1999; Massapina et al., 1999). Both oyster species have been documented in estuaries, harbors, and marinas (Stagličić et al., 2020), including in the study area and nearby lagoons (Airoldi et al., 2016), and should be able to resist the environmental conditions in the area. Transient disturbances could have nevertheless led to mass mortality of oysters, possibly replaced by faster-growing mussels, X. securis, in the innermost sites, and M. galloprovincialis in the outermost sites respectively.
Expectedly, adult distributions reflected their status (NIS vs Native), with M. galloprovincialis (Native) increasing towards the periurban part of the canal (S5), and X. securis (NIS) decreasing. This dichotomous distribution aligns with observations made by Montes et al. (2021) in a Spanish harbor, where a combined effect of spatially organized features (i.e., environmental gradients) and seasonal dispersal barriers were hypothesized as underlying drivers. In our study, for M. galloprovincialis, a linear trend was accompanied by an acceleration setting at a threshold (Quadratic term; Table 1), centered between S3 and S4. Between S3 and the canal’s entrance, the abundance of adults increased at a high rate. The distribution pattern of its settlers closely mirrored this trend. Given that the length of the canal falls well within the typical dispersal distance of M. galloprovincialis (Gomes et al., 2016), this may indicate a preferential settlement as it tightly matched the adult distribution. Mytilus galloprovincialis adults and larvae are known to be controlled by their tolerance to environmental parameters, including temperature, salinity (Olabarria et al., 2016; Vasquez et al., 2022), and some sources of pollution (Serrano et al., 1995; Boukadida et al., 2016). Environmental conditions between S1 and S3 may not be favorable to M. galloprovincialis and may constitute a limiting factor for their settlement and survival. The distribution profile of M. galloprovincialis adults and settlers was similar to the PC1 value profile used as a proxy to outline the environmental gradient, with S4 and S5 markedly different from S3, S2 and S1 (Table 1; Figure 1). It is likely that M. galloprovincialis distribution was mostly influenced by its inability to thrive in the environmental conditions of inner urban areas and its preference for more suitable environments for settlement.
Decreasing from the innermost waterfront to the periurban site, the abundance of X. securis adults was consistently high at the two innermost sites, while it was almost absent at the outermost ones (Figure 2; Table 1). Unlike M. galloprovincialis, where the distribution of settlers closely mirrored that of the adults, X. securis displayed a significant discrepancy between the abundance of settlers and adults. Settlers of X. securis were consistently spread out in the canal, with the highest abundance found in the middle areas, where adult populations were already decreasing. The mismatch between adult and settler abundances could indicate that for this species, post-settlement processes could drive the adult population distribution patterns as settlers colonize a broader range than that to what the adult population was limited. One such post-settlement process could be predation from boring snails, crabs, and fish, which depending on the environmental conditions and characteristics of the mussel aggregates, can reduce X. securis survival (Gestoso et al., 2014), and consequently adult populations. Another post-settlement process affecting X. securis distribution could be competition with the native species M. galloprovincialis at the outermost part of the canal. Despite in aquaria experiments revealing a high resistance to a wide range of temperatures, pH, and salinities (Garci et al., 2007; Gestoso et al., 2016; Olabarria et al., 2016) in situ, X. securis seems restricted to the upper estuarine sites with low salinities (Garci et al., 2007; Montes et al., 2021). The general high resistance of NIS to multiple and variable environmental stressors (Lenz et al., 2011), which also applies to X. securis (Gestoso et al., 2016; Olabarria et al., 2016; Astudillo et al., 2017) could explain its high abundance at the inner canal sites. However, stress resistances come with costly metabolic trade-offs (Pook et al., 2009; Gauff et al., 2022). This metabolic challenge could lead to decreased competitivity in environments with lower disturbance and may explain why numerous NIS thrive in high disturbance habitats like anthropic habitats or estuaries but are far less common in nearby natural sites (Airoldi et al., 2015; Gauff, 2021). Other species could also compete with X. securis, but keystone bivalves were selected for this study because they are the most abundant species in the study area, thus it seems unlikely that other species compete too strongly with X. securis.
Overall, our data support the hypothesis that NIS are more prevalent in disturbed habitats (Piola and Johnston, 2008; Megina et al., 2016; Rondeau et al., 2022), potentially due to unfavorable post-settlement competitive matchups against similar native species in less disturbed environments (Montes et al., 2021; Gauff et al., 2022), and/or due to specialization into resistant life strategy traits implying certain metabolic trade-offs (Gauff, 2021; Gauff et al., 2022). Future work on larval stages and settlers of a greater range of species is worth pursuing to test the generality of this hypothesis and to identify the underlying drivers, by disentangling biotic interactions from abiotic parameters like temperature, salinity, and chlorophyl-a, which considerably varied in our experiment and could have impacted both species (Gestoso et al., 2014; Olabarria et al., 2016; Montes et al., 2021). This should include tests on competition between native and NIS with similar niches, as well as other post-settlement processes like predation or seasonal post-settlement mortality (Montes et al., 2021), which could also lead to the discrepancies observed here.
Data availability statement
The datasets are available within the Supplementary Material and scripts for this study will be provided upon reasonable request.
Ethics statement
Ethical approval was not required for the study involving animals in accordance with the local legislation and institutional requirements because not needed for these specimens.
Author contributions
RG: Data curation, Formal Analysis, Visualization, Writing – original draft, Writing – review & editing. FM: Data curation, Investigation, Methodology, Writing – original draft, Writing – review & editing. PM: Conceptualization, Methodology, Supervision, Writing – original draft, Writing – review & editing. FP: Conceptualization, Methodology, Writing – review & editing. FC: Conceptualization, Funding acquisition, Methodology, Project administration, Supervision, Writing – review & editing. LA: Conceptualization, Funding acquisition, Methodology, Project administration, Supervision, Writing – review & editing.
Funding
The author(s) declare financial support was received for the research, authorship, and/or publication of this article. This work was done within the World Harbor Project (www.worldharbourproject.org). During the writing LA was supported by the National Biodiversity Future Center - NBFC (Project code CN_00000033, Concession Decree No. 1034 of 17 June 2022, CUP D33C22000960007) under the National Recovery and Resilience Plan (PNRR), Mission 4 Component 2 Investment 1.4 - Call for tender No. 3138 of 16 December 2021, rectified by Decree n.3175 of 18 December 2021 of the Italian Ministry of University and Research funded by the European Union – NextGenerationEU and FC was supported by the RETURN Extended Partnership funded by the European Union Next-GenerationEU (National Recovery and Resilience Plan – NRRP, Mission 4, Component 2, Investment 1.3 – D.D. 1243 2/8/2022, PE0000005) and by the project “Ecosystem for Sustainable Transition in Emilia-Romagna” (ECOSISTER, Code: ECS_00000033 - CUP: B33D21019790006; Mission 04 Education and research - Component 2 From research to business Investment 1.5 - NextGenerationEU).
Acknowledgments
The authors are grateful to Autorità Portuale di Ravenna for allowing sampling along the Candiano Channel, Ravenna, to Paolo Comandini and Ferrante Grasselli who helped during sampling and lab processing of bivalve adults and to Owen S. Wangensteen who’s provided us a 96-well plate containing individual COI barcodes for sample multiplexing and metabarcoding sequencing. We wish to thank Massimo Ponti (Università di Bologna) for the establishment of the map in Figure 1.
Conflict of interest
The authors declare that the research was conducted in the absence of any commercial or financial relationships that could be construed as a potential conflict of interest.
The author(s) declared that they were an editorial board member of Frontiers, at the time of submission. This had no impact on the peer review process and the final decision.
Publisher’s note
All claims expressed in this article are solely those of the authors and do not necessarily represent those of their affiliated organizations, or those of the publisher, the editors and the reviewers. Any product that may be evaluated in this article, or claim that may be made by its manufacturer, is not guaranteed or endorsed by the publisher.
Supplementary material
The Supplementary Material for this article can be found online at: https://www.frontiersin.org/articles/10.3389/fmars.2024.1401552/full#supplementary-material
References
Airoldi L. (2003). The effects of sedimentation on rocky coast assemblages. Oceanography Mar. Biology: an Annu. Rev. 41, 161–236.
Airoldi L., Beck M. W., Firth L. B., Bugnot A. B., Steinberg P. D., Dafforn K. A. (2021). Emerging solutions to return nature to the urban ocean. Ann. Rev. Mar. Sci. 13, 445–477. doi: 10.1146/annurev-marine-032020-020015
Airoldi L., Ponti M., Abbiati M. (2016). Conservation challenges in human dominated seascapes: The harbour and coast of Ravenna. Reg. Stud. Mar. Sci. 8, 308–318. doi: 10.1016/j.rsma.2015.11.003
Airoldi L., Turon X., Perkol-Finkel S., Rius M. (2015). Corridors for aliens but not for natives: Effects of marine urban sprawl at a regional scale. Divers. Distrib 21, 755–768. doi: 10.1111/ddi.12301
Astudillo J. C., Bonebrake T. C., Leung K. M. Y. (2017). The recently introduced bivalve Xenostrobus securis has higher thermal and salinity tolerance than the native Brachidontes variabilis and established Mytilopsis sallei. Mar. pollut. Bull. 118, 229–236. doi: 10.1016/j.marpolbul.2017.02.046
Atalah J., Otto S. A., Anderson M. J., Costello M. J., Lenz M., Wahl M. (2007). Temporal variance of disturbance did not affect diversity and structure of a marine fouling community in north-eastern New Zealand. Mar. Biol. 153, 199–211. doi: 10.1007/s00227-007-0798-6
Bataller E., Burke K., Ouellette M., Maillet M.-J. (1999). Evaluation of spawning period and spat collection of the northernmost population of European oysters (Ostrea edulis L.) on the Canadian Atlantic coast. Can. Tech Rep. Fish Aquat Sci., 2630.
Bates D., Mächler M., Bolker B., Walker S. (2015). Fitting linear mixed-effects models using lme4. J. Stat. Softw 67, 1–48. doi: 10.18637/jss.v067.i01
Blackburn T. M., Bellard C., Ricciardi A. (2019). Alien versus native species as drivers of recent extinctions. Front. Ecol. Environ. 17. doi: 10.1002/fee.2020
Boukadida K., Banni M., Gourves P. Y., Cachot J. (2016). High sensitivity of embryo-larval stage of the Mediterranean mussel, Mytilus galloprovincialis to metal pollution in combination with temperature increase. Mar. Environ. Res. 122, 59–66. doi: 10.1016/j.marenvres.2016.09.007
Bugnot A. B., Mayer-Pinto M., Airoldi L., Heery E. C., Johnston E. L., Critchley L. P., et al. (2021). Current and projected global extent of marine built structures. Nat. Sustain 4, 33–41. doi: 10.1038/s41893-020-00595-1
Dafforn K. A., Glasby T. M., Airoldi L., Rivero N. K., Mayer-Pinto M., Johnston E. L. (2015). Marine urbanization: an ecological framework for designing multifunctional artificial structures. Front. Ecol. Environ. 13, 82–90. doi: 10.1890/140050
Diagne C., Leroy B., Vaissière A.-C., Gozlan R. E., Roiz D., Jarić I., et al. (2021). High and rising economic costs of biological invasions worldwide. Nature 592, 571–585. doi: 10.1038/s41586-021-03405-6
Fauvelot C., Bertozzi F., Costantini F., Airoldi L. (2009). Lower genetic diversity in the limpet Patella caerulea on urban coastal structures compared to natural rocky habitats. 156:2313–2323. doi: 10.1007/s00227-009-1259-1
Floerl O., Inglis G. J. (2003). Boat harbour design can exacerbate hull fouling. Austral Ecol. 28, 116–127. doi: 10.1046/j.1442-9993.2003.01254.x
Fox J., Weisberg S. (2018). An R companion to applied regression. 3rd ed. (Thousand Oaks CA: SAGE Publications, Inc).
Garci M. E., Trigo J. E., Pascual S., González A. F., Rocha F., Guerra A. (2007). Xenostrobus securis (Lamarck 1819) (Mollusca: Bivalvia): first report of an introduced species in Galician waters. Aquaculture Int. 15, 19–24. doi: 10.1007/s10499-006-9062-1
Gauff R. P. M. (2021). Diversity and functioning of harbor communities. Influence on non indigenous species in a climate change driven context (Sorbonne Université). Available online at: https://theses.hal.science/tel-03904604/ (Accessed May 8, 2023).
Gauff R. P. M., Davoult D., Greff S., Bohner O., Coudret J., Jacquet S., et al. (2022). Pollution gradient leads to local adaptation and small-scale spatial variability of communities and functions in an urban marine environment. Sci. Total Environ. 838, 155911. doi: 10.1016/j.scitotenv.2022.155911
Gestoso I., Arenas F., Olabarria C. (2014). Biotic resistance and facilitation of a nonindigenous mussel vary with environmental context. Mar. Ecol. Prog. Ser. 506, 163–173. doi: 10.3354/meps10828
Gestoso I., Arenas F., Olabarria C. (2016). Ecological interactions modulate responses of two intertidal mussel species to changes in temperature and pH. J. Exp. Mar. Biol. Ecol. 474, 116–125. doi: 10.1016/j.jembe.2015.10.006
Gomes I., Peteiro L. G., Albuquerque R., Nolasco R., Dubert J., Swearer S. E., et al. (2016). Wandering mussels: Using natural tags to identify connectivity patterns among Marine Protected Areas. Mar. Ecol. Prog. Ser. 552, 159–176. doi: 10.3354/meps11753
Klein J. C., Underwood A. J., Chapman M. G. (2011). Urban structures provide new insights into interactions among grazers and habitat. Ecol. Appl. 21, 427–438. doi: 10.1890/09-1940.1
Lacroix C., Duvieilbourg E., Guillou N., Guyomarch J., Bassoulet C., Moraga D. (2017). Seasonal monitoring of blue mussel (Mytilus spp.) populations in a harbor area: A focus on responses to environmental factors and chronic contamination. Mar. Environ. Res. 129, 24–35. doi: 10.1016/j.marenvres.2017.04.008
Lam K., Todd P. (2013). Spatial differences in subtidal epibiotic community structure in marina at Keppel Bay, Singapore. Nat. Singapore 6, 197–206.
Lenz M., da Gama B. A. P., Gerner N. V., Gobin J., Gröner F., Harry A., et al. (2011). Non-native marine invertebrates are more tolerant towards environmental stress than taxonomically related native species: Results from a globally replicated study. Environ. Res. 111, 943–952. doi: 10.1016/j.envres.2011.05.001
Massapina C., Joaquim S., Matias D., Devauchelle N. (1999). Oocyte and embryo quality in Crassostrea gigas (Portuguese strain) during a spawning period in Algarve, South Portugal. Aquat Living Resour 12, 327–333. doi: 10.1016/S0990-7440(99)00115-1
McMahon R. F. (2002). Evolutionary and physiological adaptations of aquatic invasive animals: r selection versus resistance. Can. J. Fisheries Aquat. Sci. 59, 1235–1244. doi: 10.1139/f02-105
Megina C., González-Duarte M. M., López-González P. J. (2016). Benthic assemblages, biodiversity and invasiveness in marinas and commercial harbours: an investigation using a bioindicator group. Biofouling 32, 465–475. doi: 10.1080/08927014.2016.1151500
Menniti C. M., Whitney M. M., Deignan-Schmidt S. R. (2020). The importance of offshore exchange for water temperatures in norwalk harbor. Estuaries Coasts 43, 787–800. doi: 10.1007/s12237-020-00710-7
Mineur F., Cook, Elizabeth J., Minchin D., Bohn K., Macleod A., et al. (2012). Changing coasts : marine aliens and artificial structures. Oceanography Mar. Biology: Annu. Rev. 50, 189–234.
Montes A., Vázquez E., Peteiro L. G., Olabarria C. (2021). Dynamics and processes influencing recruitment of the invasive mussel Xenostrobus securis and the coexisting indigenous Mytilus galloprovincialis in north-western Spain. Aquat Invasions 16, 391–414. doi: 10.3391/ai
Oksanen J., Blanchet F. G., Kindt R., Legendre P., Minchin P. R., O’hara R. B., et al. (2018). Vegan: community ecology package. R Package Version 2. 4-6. doi: 10.1093/molbev/msv334
Olabarria C., Gestoso I., Lima F. P., Vazquez E., Comeau L. A., Gomes F., et al. (2016). Response of two mytilids to a heatwave : the complex interplay of physiology, behaviour and ecological interactions. PloS Biol. 11, 1–23. doi: 10.1371/journal.pone.0164330
Pinochet J., Urbina M. A., Lagos M. E. (2020). Marine invertebrate larvae love plastics: Habitat selection and settlement on artificial substrates. Environ. pollut. 257, 113571. doi: 10.1016/j.envpol.2019.113571
Piola R. F., Johnston E. L. (2008). Pollution reduces native diversity and increases invader dominance in marine hard-substrate communities. Divers. Distrib 14, 329–342. doi: 10.1111/j.1472-4642.2007.00430.x
Pook C., Lewis C., Galloway T. (2009). The metabolic and fitness costs associated with metal resistance in Nereis diversicolor. Mar. pollut. Bull. 58, 1063–1071. doi: 10.1016/j.marpolbul.2009.02.003
Porri F., McQuaid C. D., Radloff S. (2006). Spatio-temporal variability of larval abundance and settlement of Perna perna: Differential delivery of mussels. Mar. Ecol. Prog. Ser. 315, 141–150. doi: 10.3354/meps315141
Pyšek P., Hulme P. E., Simberloff D., Bacher S., Blackburn T. M., Carlton J. T., et al. (2020). Scientists ‘ warning on invasive alien species. Biol. Rev. 95(6), 1151–1534. doi: 10.1111/brv.12627
Rondeau S., Davoult D., Lejeusne C., Kenworthy J. J. M., Bohner O., Loisel S., et al. (2022). Persistent dominance of non-indigenous species in the inner part of a marina highlighted by multi-year photographic monitoring. Mar. Ecol. Prog. Ser. 690, 15–30. doi: 10.3354/meps14052
Scheltema R. S., Williams I. P., Shaw M. A., Loudon C. (1981). Gregarious settlement by the larvae of hydroides dianthus (Polychaeta: serpulidae). Mar. Ecol. Prog. Ser. 5, 69–74. doi: 10.3354/meps005069
Schiff K., Brown J., Diehl D., Greenstein D. (2007). Extent and magnitude of copper contamination in marinas of the San Diego region, California, USA. Mar. pollut. Bull. 54, 322–328. doi: 10.1016/j.marpolbul.2006.10.013
Serrano R., Hernández F., Peña J. B., Dosda V., Canales J. (1995). Toxicity and bioconcentration of selected organophosphorus pesticides in Mytilus galloprovincialis and Venus gallina. Arch. Environ. Contam Toxicol. 29, 284–290. doi: 10.1007/BF00212491
Simberloff D. (2009). The role of propagule pressure in biological invasions. Annu. Rev. Ecol. Evol. Syst. 40, 81–102. doi: 10.1146/annurev.ecolsys.110308.120304
Stagličić N., Šegvić-Bubić T., Ezgeta-Balić D., Bojanić Varezić D., Grubišić L., Žuvić L., et al. (2020). Distribution patterns of two co-existing oyster species in the northern Adriatic Sea: The native European flat oyster Ostrea edulis and the non-native Pacific oyster Magallana gigas. Ecol. Indic 113(106233), 1–12. doi: 10.1016/j.ecolind.2020.106233
Todd P. A., Heery E. C., Loke L. H. L., Thurstan R. H., Kotze D. J., Swan C. (2019). Towards an urban marine ecology: characterizing the drivers, patterns and processes of marine ecosystems in coastal cities. Oikos 128, 1215–1242. doi: 10.1111/oik.05946
Vasquez M. C., Houston C. T., Alcantar C. Y., Milshteyn L., Brazil C. A., Zepeda O. G. (2022). Interactive effects of multiple stressors on the physiological performance of the invasive mussel Mytilus galloprovincialis. Mar. Environ. Res. 178, 105665. doi: 10.1016/j.marenvres.2022.105665
Von Der Meden C. E. O., Porri F., McQuaid C. D., Faulkner K., Robey J. (2010). Fine-scale ontogenetic shifts in settlement behaviour of mussels: Changing responses to biofilm and conspecific settler presence in Mytilus galloprovincialis and Perna perna. Mar. Ecol. Prog. Ser. 411, 161–171. doi: 10.3354/meps08642
Walsh J. R., Carpenter S. R., van der Zanden M. J. (2016). Invasive species triggers a massive loss of ecosystem services through a trophic cascade. Proc. Natl. Acad. Sci. U.S.A. 113, 4081–4085. doi: 10.1073/pnas.1600366113
Walters L. J. (1992). Field settlement locations on subtidal marine hard substrata: Is active larval exploration involved? Limnol Oceanogr 37, 1101–1107. doi: 10.4319/lo.1992.37.5.1101
Keywords: marine urbanization, propagule pressure, invasive species, post-settlement, recruitment, metabarcoding, larvae, pollution
Citation: Gauff RPM, Mugnai F, Mancuso FP, Porri F, Costantini F and Airoldi L (2024) Distribution of native and non-indigenous bivalves and their settlers along an urban gradient. Front. Mar. Sci. 11:1401552. doi: 10.3389/fmars.2024.1401552
Received: 15 March 2024; Accepted: 21 June 2024;
Published: 25 July 2024.
Edited by:
Jasmine Ferrario, University of Pavia, ItalyReviewed by:
Edson A. Vieira, Federal University of Rio Grande do Norte, BrazilGreta Srebaliene, Klaipėda University, Lithuania
Copyright © 2024 Gauff, Mugnai, Mancuso, Porri, Costantini and Airoldi. This is an open-access article distributed under the terms of the Creative Commons Attribution License (CC BY). The use, distribution or reproduction in other forums is permitted, provided the original author(s) and the copyright owner(s) are credited and that the original publication in this journal is cited, in accordance with accepted academic practice. No use, distribution or reproduction is permitted which does not comply with these terms.
*Correspondence: Federica Costantini, RmVkZXJpY2EuY29zdGFudGluaUB1bmliby5pdA==; Francesco Paolo Mancuso, ZnJhbmNlc2NvLm1hbmN1c29AdW5pcGEuaXQ=
†These authors have contributed equally to this work and share first authorship