- Department of Genetics and Marine Biotechnology, Institute of Oceanology, Polish Academy of Sciences, Sopot, Poland
The diverse and changing pigmentation of the skin allows fish to adapt to environmental conditions for survival and communication with conspecifics. However, various physical and chemical environmental factors, including pollutants, affect fish coloration. Therefore, the implementation of an analysis of skin pigmentation has been considered in fish well-being and ecotoxicological studies. A physiological color change is achieved by the motility of melanin-containing organelles: they aggregate into the perikaryon or disperse throughout the cytoplasm of melanophores in response to various stimuli. In our study, we addressed the issue of implementing the analysis of pigment dispersion in melanophores in stickleback skin to assess the response of fish to oxidative stress. We examined pigment dispersion in day and night skin samples collected from the dorsal, lateral and ventral regions. The degree of pigment dispersion we assessed by the melanophore index. The total number of melanophores counted in the defined skin area was significantly higher in the night samples than in the day samples. Only in day samples of dorsal skin we observed the significant changes in pigment dispersion after exposure to stress: melanin was predominantly in the aggregated state. In the night samples, we did not report any response to stress in any part of the skin. Examination of pigment dispersion in melanophores in stickleback skin can be useful for assessing the welfare of fish and detecting toxic agents in the environment, but under specified conditions: in sticklebacks, it is analysis of dorsal skin during the day.
Overall
The diverse and changing hues and patterns of the skin allow fish to adapt to environmental conditions for survival and communication with conspecifics. It is substantial for its camouflage, self-defense, courtship and mating, thermoregulation, and protection from UV radiation (Kulkeaw et al., 2011; Sköld et al., 2012, 2016; Nüsslein-Volhard and Singh, 2017; Chauhan and Gretz, 2021; Vissio et al., 2021). The coloration depends on pigment cells (chromatophores) in the skin. It is well-established that various physical and chemical environmental factors interfere with fish pigmentation (Fujii and Oshima, 1994; Sköld et al., 2012, 2016; Choi et al., 2020), and growing evidence has shown that various environmental pollutants can affect it (Allen et al., 2004; Kaur and Dua, 2012; Daiwile et al., 2015; Hou et al., 2022). This information is crucial for the potential implementation of an analysis of skin coloration in fish well-being and ecotoxicological studies. For example, Kittilsen and colleagues (2009) showed the association between melanin-based pigmentation and individual stress responsiveness (stress coping style) in salmonid fish. A good example are fish chromatophores from Betta splendens applied as cytosensors for detecting environmental toxins and bacterial pathogens by monitoring changes in pigment distribution (Chaplen et al., 2002). In addition, Channa punctatus chromatophores are considered as a biomarker of arsenic exposure in aquatic ecosystems (Allen et al., 2004). Dukovcic and collaborators (2010) reported that Oncorhynchus tshawytscha melanophores can also be used as environmental toxicity sensors for the detection of mercuric chloride and sodium arsenite. Furthermore, the skin, which is directly exposed to environmental stressors, is a site of the synthesis of biologically active components involved in the stress response (Kulczykowska, 2019). These components of the so-called cutaneous stress response system may affect melanin dispersion in melanophores (Kulczykowska et al., 2018; Gozdowska et al., 2022; Pomianowski et al., 2023).
Now, a brief reminder: various types of chromatophores are involved in the pigmentation of teleosts; among them are the most universal black or brown melanophores primarily located in the dermis, although they can also be observed in the epidermis (Fujii, 2000). Rapid and transient color change (physiological color change) is achieved by the motility of pigment vesicles in cells. The motile activities of melanophores rely on intracellular motor proteins, namely tubulin, dynein, and kinesin (Sköld et al., 2002). Melanin-containing organelles (melanosomes) can aggregate into the perikaryon or disperse throughout the cytoplasm of melanophores in response to various stimuli and the corresponding nervous and hormonal signals; some external physical and chemical factors can also directly affect melanophores. This subject has been discussed in several valuable papers in detail (Fujii and Oshima, 1994; Fujii, 2000; Yamaguchi and Hearing, 2009; Sköld et al., 2012, 2016). In recent years, complementary data on the physiological regulation of pigmentation in fish have been increasing (Kobayashi et al., 2012; Vissio et al., 2021; Wen et al., 2022).
Lately, we conducted an analysis of the dispersion of melanin in melanophores after a short-term exposure of three-spined stickleback to oxidative stress (Gozdowska et al., 2022). To evoke oxidative stress, we have used a potassium dichromate solution (K2Cr2O7) well identified as an anthropogenic water pollutant that induces oxidative stress in aquatic organisms, including fish (Velma et al., 2009; Ko et al., 2019). In that preliminary study, we collected and examined pigment dispersion in night skin samples and observed the responses of melanophores from different locations (dorsal, lateral, and ventral skin samples), but the pattern of changes was not definite. However, these data hinted at the potential use of pigment dispersion as a mark of oxidative stress in three-spined stickleback (Gozdowska et al., 2022). Therefore, in the present study, we have revisited the question of implementing the analysis of pigment dispersion in melanophores to assess the response to oxidative stress in this species. We aim to specify under what conditions this is relevant.
Why the three-spined stickleback and melanophores?
The three-spined stickleback is one of the most ubiquitous and adaptable species widely distributed in coastal waters (fresh, brackish, or salt) of the northern hemisphere. It is a well-recognized species for monitoring the biological effects of chemical contamination (Katsiadaki et al., 2012; Von Hippel et al., 2016), and consequently is a model fish in many ecotoxicological studies. Therefore, we have also selected this species in our study. We have analysed a pigment dispersion in melanophores because, among all chromatophores, the melanophores exhibit the highest motile activities and consequently play a leading role in the rapid color change in many fish species, including sticklebacks. In this species, a rapid pigmentation change can occur in minutes or hours. The ornamental coloration of male sticklebacks during breeding is a good example; the physiological regulation of this well-known phenomenon has been thoroughly described by Franco-Belussi et al. (2018).
Experimental conditions
Adult three-spined sticklebacks of both sexes (total length: 50−66 mm; total weight: 1.206−2.485 g) were caught in the Gulf of Gdańsk (southern Baltic Sea) in October 2020, out of the breeding, at an environmental water temperature of approximately 10°C and a photoperiod of 12L:12D. After being transported to the Institute of Oceanology PAS (Sopot, Poland), the fish were acclimatized in 40-L aerated aquaria under laboratory conditions corresponding to those in the environment (10 ± 2°C, 12L:12D, brackish water with a salinity of 7ppt) for four weeks. Sticklebacks fed on frozen chironomids once a day at 13:00; feeding was finished three days before the experiment. Prior to experiment, the fish were randomly assigned to two groups: the control (kept in water without K2Cr2O7) and the tested group (kept in water with K2Cr2O7: 50 mg Cr/1 L for 6 hours). The concentration of chromium added to the water was expressed as Cr6+ ion in milligrams per liter of water. The fish were kept at a temperature of 10 ± 2 °C and 12L:12D photoperiod (light phase: 6.00−18.00). The fish were sacrificed by decapitation after a six-hour exposition. The daytime sampling started at 10:00, four hours after turning on the light (n = 9, control fish = 5, K2Cr2O7-treated fish = 4). Overnight sampling began at 22.00, four hours after turning off the light (n = 18, control fish = 10, K2Cr2O7-treated fish = 8). Overnight samples were collected under red light. Skin samples of 9 mm × 4 mm were taken from the left body flank from the dorsal (above the midline), lateral and ventral body regions (in total 3 samples from each fish). The samples were fixed in 4% buffered formalin, mounted on Eukitt slides (Sigma-Aldrich, USA), scanned with an Olympus BX60 light microscope (Olympus, Japan) and photographed using transillumination with an Olympus XC10 digital camera (Olympus, Japan) coupled with the Olympus microscope. The photographs were loaded into ImageJ 1.53e. image analysis software. The number of melanophores of different pigment dispersion degrees was counted on the area of 5 mm × 2 mm. The degree of pigment dispersion was assessed by the melanophore index (MI) based on the scale given by Aspengren et al. (2003), with modification: MI 1: melanophores with completely aggregated melanin, MI 2: melanophores with partially dispersed melanin, MI 3: melanophores with completely dispersed melanin.
Statistical analysis of data was performed using Statistica 13.3 software. Values were presented as mean ± standard error of the mean (SEM). The differences in the number of three types of melanophores in three regions of the body between day and night in control (non-stressed) fish and fish exposed to oxidative stress were examined with the Multifactorial ANOVA statistics followed by Tukey’s HSD post hoc test for unequal number of fish. Statistical significance was considered at P < 0.05.
Observations
We have examined the pigment dispersion in melanophores in day and night skin samples collected from three different regions (dorsal, lateral, and ventral) of the three-spined stickleback exposed to oxidative stress. Interestingly, the total number of melanophores counted in the defined skin area appears to be significantly higher in night samples than in day samples (P < 0.05) (Figure 1). It is evident that the time of day (or presence or absence of light), but not stress exposure, makes a difference, because a total number does not change significantly after stress exposure, either during the day or at night (Figure 1).
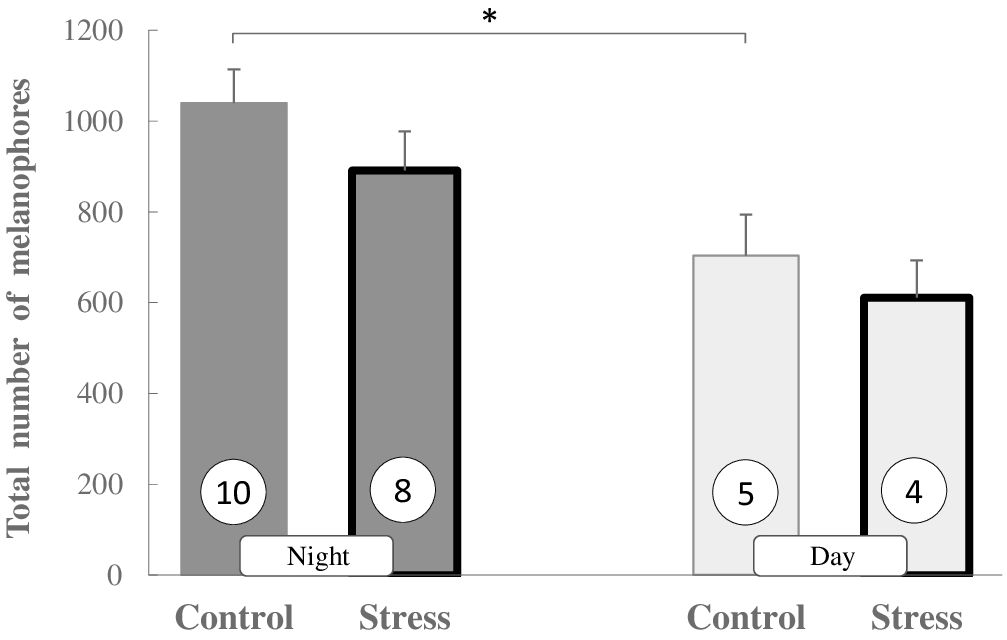
Figure 1 The total number of melanophores (MI 1 + MI 2 + MI 3) in skin sections in non-stressed and stressed (bold bars) three-spined sticklebacks during the day and night. Values are presented as means ± SEM. Significant differences are indicated as *P < 0.05 (Multifactorial ANOVA, Tukey HSD post hoc test). The number of fish is given in circles.
The question arises what is going on with melanophores? Do they migrate between different skin layers during the day? They may be exposed during the night in the external skin layer as they migrate to this layer during the night; on the other hand, they may be hidden in deeper layers during the day, so they are not visible. The phenomenon of melanophores migration in the skin was addressed by Takahashi and Kondo (2008), who suggested that melanophores possess the migration ability in adult zebrafish. However, to the authors’ knowledge, there are no papers dealing with melanophores migration between skin layers during the day/night. Generally, melanophores density variation in the skin implies both differentiation and apoptosis processes, but it is not possible that changes in day/night could be related to these processes. On the other hand, we can also speculate that light can change the structure of the pigment (melanin) in the cell (losing color), making melanophores undetected that can impact the final count. It is worth mentioning that melanin is highly sensitive to light (Korytowski and Sarna, 1990; Meredith and Sarna, 2006; Chauhan and Gretz, 2021). For now there is only conjecture and the question reminds unanswered but should be addressed in a future study.
In Figure 2, we present the effects of exposure of fish to oxidative agent during the day and night. Only in day samples of dorsal skin we observe the significant changes in pigment dispersion after stress exposure: melanin is predominantly in aggregated state (MI 1: P < 0.01). This effect is slightly visible in lateral skin samples, but not in ventral. In the night samples, we have not reported any response to oxidative stress in any region of the skin. It is evident that not every part of the skin is suitable for research: the same is true with the time of day.
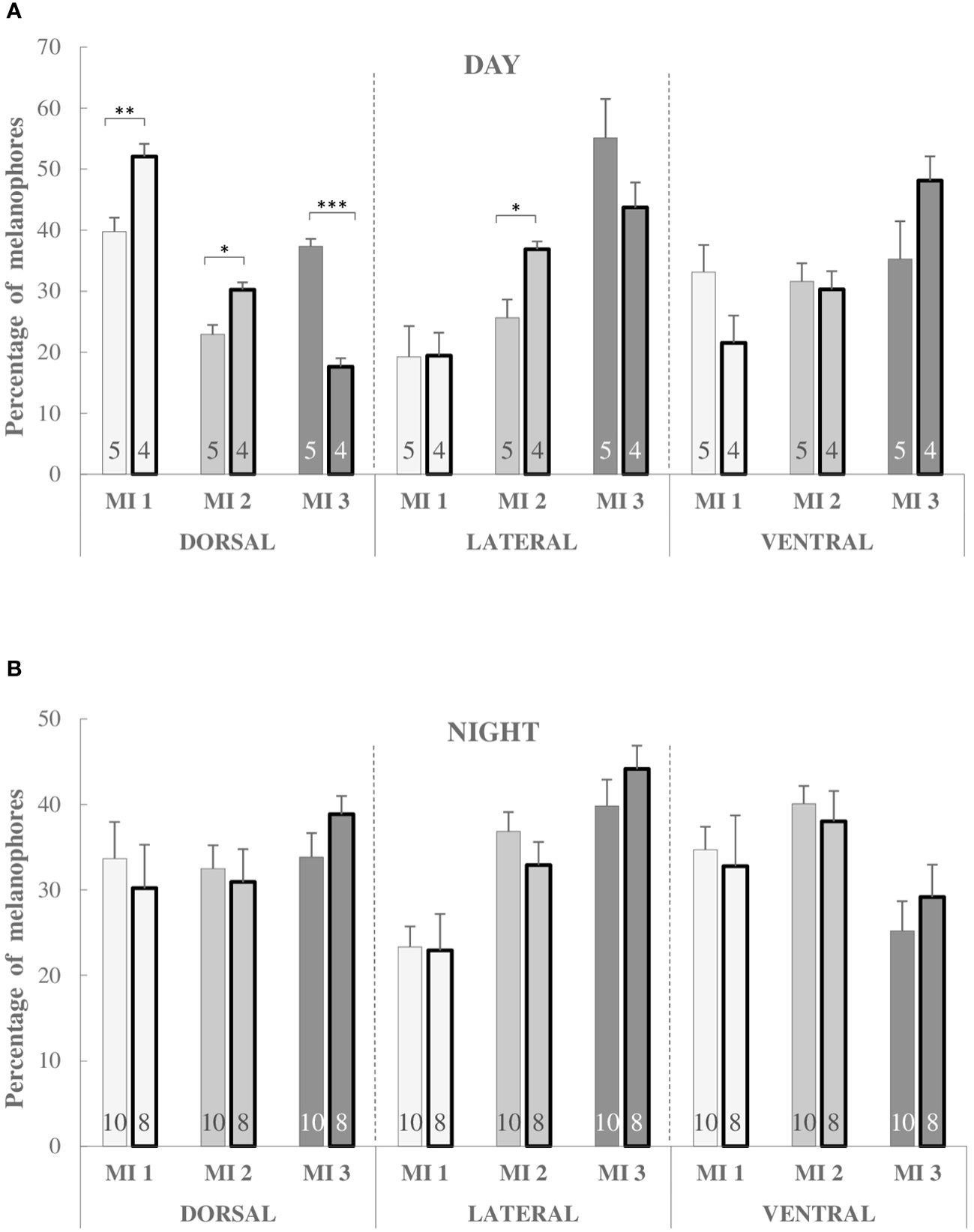
Figure 2 Percentage of melanophores of MI 1, MI 2 and MI 3 types during the day (A) and night (B) in the dorsal, lateral and ventral skin regions in non-stressed and stressed (bold bars) three-spined sticklebacks. Significant differences are indicated as *P < 0.05, **P < 0.01 and ***P < 0.001 (Multifactorial ANOVA, Tukey HSD post hoc test). The number of fish is given at the bottom of the bars. MI 1: melanophores with completely aggregated melanin
MI 2: melanophores with partially dispersed melanin
MI 3: melanophores with completely dispersed melanin.
What can we learn from studying melanin dispersion in melanophores?
Finding a proper way to assess the response of fish to the changing environment is still a topic of debate; many questions remain because the performance of different species of fish and their reactions to various kinds of stress or awry environments differ significantly. There is still a need to develop adequate biosensors for the measurement of different types of stress, including oxidative stress induced by environmental pollutants. Therefore, we have addressed the issue of implementing the pigment dispersion analysis in melanophores to assess oxidative stress in the model fish, the three-spined stickleback. Examination of pigment dispersion in melanophores in stickleback skin can be useful for both assessing fish welfare and detecting biologically toxic agents in the environment, but under specified conditions. Here, we point out the conditions that must be met to perform analyses and interpret data accurately. It appears that an analysis of dorsal skin samples collected during the day is recommended. However, these conditions have been emphasized as relevant in terms of sticklebacks and oxidative stress, but may be different in other species of fish or types of stress.
Data availability statement
The raw data supporting the conclusions of this article will be made available by the authors, without undue reservation.
Ethics statement
The animal study was approved by Ethics Committee for Animal Experimentation of Bydgoszcz University of Science and Technology, ul. Mazowiecka 28, 85-084 Bydgoszcz, Poland, Protocol No 27/2018 and 01/2022. The study was conducted in accordance with the local legislation and institutional requirements.
Author contributions
ES: Conceptualization, Data curation, Formal analysis, Investigation, Methodology, Visualization, Writing – original draft, Writing – review & editing. EK: Conceptualization, Data curation, Funding acquisition, Investigation, Project administration, Resources, Visualization, Writing – original draft, Writing – review & editing.
Funding
The author(s) declare financial support was received for the research, authorship, and/or publication of this article. This research was supported by the National Science Centre (Poland) grant UMO-2017/27/B/NZ4/01259. The publication was supported by a statutory task of the Institute of Oceanology Polish Academy of Sciences, Sopot, Poland.
Conflict of interest
The authors declare that the research was conducted in the absence of any commercial or financial relationships that could be construed as a potential conflict of interest.
The author(s) declared that they were an editorial board member of Frontiers, at the time of submission. This had no impact on the peer review process and the final decision.
Publisher’s note
All claims expressed in this article are solely those of the authors and do not necessarily represent those of their affiliated organizations, or those of the publisher, the editors and the reviewers. Any product that may be evaluated in this article, or claim that may be made by its manufacturer, is not guaranteed or endorsed by the publisher.
Supplementary material
The Supplementary Material for this article can be found online at: https://www.frontiersin.org/articles/10.3389/fmars.2024.1401537/full#supplementary-material
References
Allen T., Awasthi A., Rana S. V. S. (2004). Fish chromatophores as biomarkers of arsenic exposure. Environ. Biol. Fishes 71, 7–11. doi: 10.1023/B:EBFI.0000043145.58953.86
Aspengren S., Sköld H. N., Quiroga G., Mårtensson L., Wallin M. (2003). Noradrenaline- and melatonin-mediated regulation of pigment aggregation in fish melanophores. Pigment Cell Res. 16, 59–64. doi: 10.1034/j.1600-0749.2003.00003.x
Chaplen F. W. R., Upson R. H., McFadden P. N., Kolodziej W. (2002). Fish chromatophores as cytosensors in a microscale device: detection of environmental toxins and bacterial pathogens. Pigment Cell Res. 15, 19–26. doi: 10.1034/j.1600-0749.2002.00069.x
Chauhan A., Gretz N. (2021). Role of visible light on skin melanocytes: a systematic review. Photochem. Photobiol. 97, 911–915. doi: 10.1111/php.13454
Choi S.-H., Kim B.-H., Lee C.-H., Lee Y.-D. (2020). Response of body color change rearing under different light intensity conditions in farmed red spotted grouper, Epinephelus akaara. Fish. Aquat. Sci. 23, 29. doi: 10.1186/s41240-020-00173-8
Daiwile A. P., Naoghare P. K., Giripunje M. D., Rao P. D. P., Ghosh T. K., Krishnamurthi K., et al. (2015). Correlation of melanophore index with a battery of functional genomic stress indicators for measurement of environmental stress in aquatic ecosystem. Environ. Toxicol. Pharmacol. 39, 489–495. doi: 10.1016/j.etap.2014.12.006
Dukovcic S. R., Hutchison J. R., Trempy J. E. (2010). Conservation of the chromatophore pigment response. J. Appl. Toxicol. 30, 574–581. doi: 10.1002/jat.1528
Franco-Belussi L., De Oliveira C., Sköld H. N. (2018). Regulation of eye and jaw colouration in three-spined stickleback Gasterosteus aculeatus. J. Fish Biol. 92, 1788–1804. doi: 10.1111/jfb.13620
Fujii R. (2000). The regulation of motile activity in fish chromatophores. Pigment Cell Res. 13, 300–319. doi: 10.1034/j.1600-0749.2000.130502.x
Fujii R., Oshima N. (1994). “Factors influencing motile activities of fish chromatophores”, in Advances in Comparative and Environmental Physiology, vol. 20. Ed. Gilles R. (Springer-Verlag, Berlin Heidelberg), 2–54. doi: 10.1007/978-3-642-78598-6-1
Gozdowska M., Sokołowska E., Pomianowski K., Kulczykowska E. (2022). Melatonin and cortisol as components of the cutaneous stress response system in fish: Response to oxidative stress. Comp. Biochem. Physiol. Part A 268, 111207. doi: 10.1016/j.cbpa.2022.111207
Hou Y., Wang L., Jin Y., Guo R., Yang L., Li E., et al. (2022). Triphenyltin exposure induced abnormal morphological colouration in adult male guppies (Poecilia reticulate). Ecotoxicol. Environ. Saf. 242, 113912. doi: 10.1016/j.ecoenv.2022.113912
Katsiadaki I., Sanders M. B., Henrys P. A., Scott A. P., Matthiessen P., Pottinger T. G. (2012). Field surveys reveal the presence of anti-androgens in an effluent-receiving river using stickleback-specific biomarkers. Aquat. Toxicol. 15, 75–85. doi: 10.1016/j.aquatox.2012.05.006
Kaur R., Dua A. (2012). Fish scales as indicators of wastewater toxicity from an international water channel Tung Dhab drain. Environ. Monit. Assess. 184, 2729–2740. doi: 10.1007/s10661-011-2147-y
Kittilsen S., Schjolden J., Beitnes-Johansen I., Shaw J. C., Pottinger T. G., Sørensen C., et al. (2009). Melanin-based skin spots reflect stress responsiveness in salmonid fish. Horm. Behav. 56, 292–298. doi: 10.1016/j.yhbeh.2009.06.006
Ko H. D., Park H. J., Kang J. C. (2019). Change of growth performance, haematological parameters, and plasma component by hexavalent chromium exposure in starry flounder, Platichthys stellatus. Fish Aqua. Sci. 22, 9. doi: 10.1186/s41240-019-01245
Kobayashi Y., Mizusawa K., Saito Y., Takahashi A. (2012). Melanocortin systems on pigment dispersion in fish chromatophores. Front. Endocrinol. 3. doi: 10.3389/fendo.2012.00009
Korytowski W., Sarna T. (1990). Bleaching of melanin pigments. Role of copper ions and hydrogen peroxide in autooxidation and photooxidation of synthetic dopa-melanin. J. Biol. Chem. 265, 12410–12416. doi: 10.1016/S0021-9258(19)38362-0
Kulczykowska E. (2019). Stress response system in the fish skin - welfare measures revisited. Front. Physiol. 10. doi: 10.3389/fphys.2019.00072
Kulczykowska E., Kalamarz-Kubiak H., Gozdowska M., Sokołowska E. (2018). Cortisol and melatonin in the cutaneous stress response system of fish. Comp. Biochem. Physiol. Part A 218, 1–7. doi: 10.1016/j.cbpa.2018.01.003
Kulkeaw K., Ishitani T., Kanemaru T., Ivanovski O., Nakagawa M., Horio Y., et al. (2011). Cold exposure down-regulates zebrafish pigmentation. Genes Cells 16, 358–367. doi: 10.1111/gtc.2011.16.issue-4
Meredith P., Sarna T. (2006). The physical and chemical properties of eumelanin. Pigment Cell Res. 19, 549–659. doi: 10.1111/j.1600-0749.2006.00345.x
Nüsslein-Volhard Ch., Singh A. P. (2017). How fish color their skin: a paradigm for development and evolution of adult patterns. Multipotency, plasticity, and cell competition regulate proliferation and spreading of pigment cells in zebrafish coloration. Bioessays: Prospects and Overviews. 39, 1600231. doi: 10.1002/bies.201600231
Pomianowski K., Gozdowska M., Sokołowska E., Kulczykowska E. (2023). The cutaneous stress response system in three-spined stickleback and European flounder exposed to oxidative stress: different mode of action. Comp. Biochem. Physiol. Part A 285, 111493. doi: 10.1016/j.cbpa.2023.111493
Sköld H. N., Aspengren S., Cheney K. L., Wallin M. (2016). “Fish chromatophores–from molecular motors to animal behaviour”, in International Review of Cell and Molecular Biology, vol. 321. Ed. Jeon K. W. (Academic Press, Chennai), 171–220. doi: 10.1016/bs.ircmb.2015.09.005
Sköld H. N., Aspengren S., Wallin M. (2012). Rapid color change in fish and amphibians - function, regulation, and emerging applications. Pigment Cell Melanoma Res. 26, 29–38. doi: 10.1111/pcmr.12040
Sköld H. N., Norström E., Wallin M. (2002). Regulatory control of both microtubule- and actin-dependent fish melanosome movement. Pigment Cell Res. 15, 357–366. doi: 10.1034/j.1600-0749.2002.02048.x
Takahashi G., Kondo S. (2008). Melanophores in the stripes of adult zebrafish do not have the nature to gather, but disperse when they have the space to move. Pigment Cell Melanoma Res. 21, 677–686. doi: 10.1111/j.1755-148X.2008.00504.x
Velma V., Vutukuru S. S., Tchounwou P. B. (2009). Ecotoxicology of hexavalent chromium in freshwater fish: a critical review. Rev. Environ. Health 24, 129–145. doi: 10.1515/REVEH.2009.24.2.129
Vissio P. G., Darias M. J., Di Yorio M. P., Pérez Sirkin D. I., Delgadin T. H. (2021). Fish skin pigmentation in aquaculture: the influence of rearing conditions and its neuroendocrine regulation. Gen. Comp. Endocrinol. 301, 113662. doi: 10.1016/j.ygcen.2020.113662
Von Hippel F. A., Trammell E. J., Merilla J., Sanders M. B., Schwarz T., Postlethwait J. H., et al. (2016). The ninespine stickleback as a model organism in arctic ecotoxicology. Evol. Ecol. Res. 17, 487–504.
Wen X., Yang M., Zhou K., Huang J., Fan X., Zhang W., et al. (2022). Transcriptomic and proteomic analyses reveal the common and unique pathway(s) underlying different skin colors of leopard coral grouper (Plectropomus leopardus). J. Proteomics 266, 104671. doi: 10.1016/j.jprot.2022.104671
Keywords: fish, melanin aggregation, melanophores, melanosomes, pigment dispersion, Gasterosteus aculeatus, oxidative stress
Citation: Sokołowska E and Kulczykowska E (2024) A new insight into the pigmentation of the three-spined stickleback exposed to oxidative stress: day and night study. Front. Mar. Sci. 11:1401537. doi: 10.3389/fmars.2024.1401537
Received: 15 March 2024; Accepted: 15 April 2024;
Published: 30 April 2024.
Edited by:
Jose Fernando Lopez-Olmeda, University of Murcia, SpainReviewed by:
Sofia Priyadarsani Das, National Taiwan Ocean University, TaiwanCopyright © 2024 Sokołowska and Kulczykowska. This is an open-access article distributed under the terms of the Creative Commons Attribution License (CC BY). The use, distribution or reproduction in other forums is permitted, provided the original author(s) and the copyright owner(s) are credited and that the original publication in this journal is cited, in accordance with accepted academic practice. No use, distribution or reproduction is permitted which does not comply with these terms.
*Correspondence: Ewa Sokołowska, ewasokol@iopan.gda.pl
†These authors have contributed equally to this work