- 1College of Fisheries, Guangdong Ocean University, Zhanjiang, China
- 2Key Laboratory of Marine Ecology and Aquaculture Environment, Zhanjiang, China
- 3Guangdong Evergreen Feed Industry Co., Ltd., Zhanjiang, Guangdong, China
- 4Zhanjiang Ocean and Fishery Development Research Center, Zhanjiang, China
- 5South China University of Technology, School of Biomedical Science and Engineering, Guangzhou, China
- 6Zhanjiang Ocean and Fishery Development Research Centre, Zhanjiang, China
Introduction: Shrimp is an important aquaculture species worldwide. Vibrio parahaemolyticus (VP) is an opportunistic pathogen of Litopenaeus vannamei that can cause diseases such as acute hepatopancreatic necrotic disease (AHPND), resulting in significant losses to the shrimp farming industry.
Methods: In this study, We analyzed two shrimp populations by transcriptomics and non-targeted metabolomics, which exhibited significant differences in resistance to VP. Through integrated analysis, we identified genes and metabolites linked to the development of shrimp's resistance to VP.
Results and discussion: The analysis revealed that the differential metabolism of flavonoid compounds, especially quercetin, significantly influences the expression of shrimp's resistance to VP. Supplementing feed with an appropriate quantity of quercetin has the potential to increase the expression of crucial genes in the NF-κB pathway, including TLR and AP1, along with the expression of the antibacterial peptide crustin, resulting in a decreased mortality rate. Together, these results indicate that an appropriate amount of quercetin can strengthen the immune response of shrimp to VP, thereby reducing the incidence of AHPND.
1 Introduction
Litopenaeus vannamei is the most extensively cultivated crustacean species globally, contributing to over 80% of the total shrimp production (FAO, 2022). Vibrio parahaemolyticus (VP) is the most detrimental bacterium to shrimp aquaculture. Currently, there are no highly effective preventive and control strategies for VP. Similar to other crustaceans, L. vannamei primarily depends on its innate immune system to counteract pathogen intrusion. The hepatopancreas, a pivotal organ within the immune system, plays a critical role in orchestrating the immune response of shrimp (Ren et al., 2019). VP, harboring the pirA and pirB toxin genes on a plasmid, is causally implicated in the onset of acute hepatopancreatic necrotic disease (AHPND) in L. vannamei, also known as early mortality syndrome (EMS) (Lee et al., 2015; Kewcharoen and Srisapoome, 2019). The clinical signs of AHPND include jejunum, pale watery hepatopancreas, and a soft shell. The mortality rate of AHPND is almost 100% in shrimp (Lai et al., 2015; Soto-Rodriguez et al., 2015). Therefore, there is an urgent need to develop an effective control method for AHPND.
The integration of genomics, transcriptomics, proteomics, metabolomics, and various other omics technologies has emerged as a crucial approach for the screening of biomarkers, facilitating disease diagnosis and increasing our understanding of pathogenic mechanisms (Bujak et al., 2015; Shah et al., 2015; Khan et al., 2020). Specifically, the integration of metabolomics with transcriptomics offers a powerful approach to understanding the dynamic shifts in the metabolic processes of organisms (Saito and Matsuda, 2010; Patti et al., 2012). In a majority of instances, metabolites are downstream functional entities resulting from the activities of genes and proteins (Ye et al., 2016). In the context of aquatic animals, liquid chromatography-tandem mass spectrometry (LC-MS) has proven to be a valuable tool for investigating potential mechanisms underlying diseases (Zhang et al., 2019; De Los Santos et al., 2022). In one study, a comprehensive analysis of transcriptome and metabolome data indicated that infection of molting crabs with Staphylococcus aureus or Vibrio alginolyticus altered the metabolome and transcriptome levels of the crabs and resulted in hormonal and immune dysfunction (Li et al., 2023). At present, the investigation of metabolic alterations in shrimp during bacterial infestations is still in a nascent stage.
Quercetin is a biologically active flavonoid found in more than 20 plant materials (Anand David et al., 2016; Hai et al., 2020). It has anti-inflammatory, anti-oxidation (Lesjak et al., 2018), anti-cancer (Baghel et al., 2012), and other characteristics. Previous studies have shown that the addition of polyphenols and polyphenol-rich additives to aquatic animal feed can increase growth performance, immune responses, antioxidant status, and disease resistance (Ahmadifar et al., 2021, Ahmadifar et al., 2022). Quercetin is known to promote immunostimulant properties, antioxidant indices, and disease resistance in zebrafish (Danio rerio) (Wang et al., 2020b). Additionally, as a dietary supplement, quercetin has been shown to enhance growth, antioxidant capacity, immune response, and regulate immune-related gene expression in Channa argus (Kong et al., 2022). However, reports of quercetin’s antibacterial activity in L. vannamei are rare. In this study, we conducted a comprehensive analysis using transcriptomics and metabolomics to compare different genes and metabolites between two populations of L. vannamei with different resistance to VP. The findings revealed a significant impact of flavonoids, particularly quercetin metabolism, on the resistance of L. vannamei to VP. Supplementation of shrimp feed with quercetin at a dosage of 1g per 100 g substantially enhanced their resistance against VP, resulting in reduced mortality due to VP infection. This study aims to uncover the critical metabolites involved in the resistance of L. vannamei to VP, offering diverse perspectives on the mechanisms underlying resistance to pathogenic microbial infections.
2 Materials and methods
2.1 Animals, pathogens, and sampling
Shrimp (L. vannamei; average weight 4.65 ± 1.97 g each) were obtained from the 863 bases of Guangdong Hengxing Feed Co., Ltd. In Zhanjiang City, China. In the pathogenicity tests on different shrimp populations, we utilized six populations sourced from different origins, with 120 shrimp in each population. The Mx and Mx01 populations were introduced from Mexico, while the TH04, TH06, CTLL, and CTS were bred domestically through independent selection. The six populations were divided into experimental and control groups of 60 shrimp each. The experimental group was injected with VP, while the control group was injected with an equal volume of sterile PBS. Based on the survival rate curves, the Mx population with the highest survival rate and the TH04 population with the lowest survival rate were chosen for transcriptional and metabolome sample collection and sequencing. The water in the culture tank was maintained at a temperature of 27 ± 0.5°C, salinity at 30-33‰, and pH at 7.2 ± 0.2 and one-third was replaced every day. Aeration was provided to maintain dissolved oxygen at a concentration of approximately 8 mg/L. Water quality indicators during the test period can meet the requirements of shrimp growth. The shrimp were fed with a formula feed at 5% of their body weight at 9:00 AM and 5:00 PM every day.
VP was obtained from the Guangdong Microbiological Collection Center, GDMCC No. 1.306. We used VP carrying pirA and pirB plasmids (as shown in Supplementary Figure S1) and cultured in Luria broth (LB) medium overnight at 37°C. The bacteria were harvested by centrifugation (5000 g, 5 min) and washed twice in phosphate buffer saline (PBS) to remove the growth medium. According to the prior study, the final injection concentration was around 2.5 × 107 CFU/mL of solution, with each shrimp receiving 50 μL (Li et al., 2013). The shrimp count was recorded every 4 h, and the surviving shrimp pairs were tallied after 5 days (120 h) to calculate the survival rate. On the 5th day (120 h) after infection, all shrimp were collected and marked to estimate cumulative mortality.
Hepatopancreas tissues from the Mx and TH04 populations of shrimp were collected at 0, 4, 24 hours post-VP infection, with the hepatopancreas of six shrimp serving as one mixed sample. Six samples were collected at each time point, and the samples were immediately immersed in liquid nitrogen after sampling and stored at -80°C.
2.2 Transcriptome and metabolome sequencing and analysis
2.2.1 Transcriptomic sequencing and analysis
Total RNA was extracted from the hepatopancreas using a Trizol reagent. Then, a NanoDrop ND-1000 (NanoDrop, Wilmington, DE, USA) and Bioanalyzer 2100 (Agilent, CA, USA) were used to analyze the amount and purity of total RNA with a RIN value > 7.0 and to detect the integrity of RNA. Two rounds of purification were performed using oligo (dT) magnetic beads. The purified mRNA was used to fragment RNA into short fragments at a high temperature using divalent cations. Then, the cleaved RNA fragments were reverse-transcribed to create the final cDNA library, by the protocol of the mRNASeq sample preparation kit (Illumina, San Diego, USA). The average insert size for the paired-end libraries was 300 bp (± 50 bp). Finally, 2×150bp paired-end sequencing (PE150) was performed on an Illumina Novaseq™ 6000 (LC-Bio Technology CO., Ltd., Hangzhou, China), according to the manufacturer’s protocol. The raw mRNA sequencing data has been uploaded to the NCBI Sequence Read Archive (SRA) submission: PRJNA1063533.
All assembled unigenes were aligned against the non-redundant (Nr) protein, Gene Ontology (GO), and Kyoto Encyclopaedia of Genes and Genomes (KEGG) databases using DIAMOND, with a threshold of Evalue<0.00001. Next, GO and KEGG enrichment analyses were performed on the differentially expressed unigenes using in-house Perl scripts. The reference genome of the transcription group is ASM378908V1.
2.2.2 Metabolomics sequencing and analysis
The sample stored at -80°C refrigerator was thawed on ice. Subsequently, the thawed sample was homogenized using a grinder (30 HZ) for 20 s. A 400 μL solution (Methanol: Water = 7:3, V/V) containing internal standard was added into a 20 mg ground sample and shaken at 1500 rpm for 5 minutes. After placing it on ice for 15 minutes, the sample was centrifuged at 12000 rpm for 10 minutes at 4°C. A 300 μL aliquot of the supernatant was collected and kept at -20°C for 30 minutes. The sample was then centrifuged again at 12000 rpm for 3 minutes at 4°C. Finally, 200 μL of the supernatant was transferred for LC-MS analysis. All samples were acquired by the LC-MS system following machine orders. The analytical conditions were as follows, UPLC: column, Waters ACQUITY UPLC HSS T3 C18 (1.8 µm, 2.1 mm*100 mm); column temperature, 40°C; flow rate, 0.4 mL/min; injection volume, 2 μL; solvent system, water (0.1% formic acid): acetonitrile (0.1% formic acid); The column was eluted with 5% mobile phase B (0.1% formic acid in acetonitrile) at 0 minute followed by a linear gradient to 90% mobile phase B (0.1% formic acid in acetonitrile) over 11 minutes, held for 1 minute, and then come back to 5% mobile phase B within 0.1 minute, held for 1.9 minutes.
The LC-MS raw data files were converted to mzXML format using ProteoWizard software. The corrected and filtered peaks were queried for metabolite identification based on laboratory-created databases, comprehensive public databases, artificial intelligence prediction databases, and metDNA methods. Principal component analysis (PCA) and orthogonal partial least squares discriminant analysis (OPLS-DA) were performed on the processed data. The projected variable importance (VIP) was calculated based on the OPLS-DA model (at least three biological replicates). Metabolites with statistically significant differences between populations were screened based on a VIP value greater than 1 and a P value less than 0.05. Metabolomics data has been deposited in the MetaboLights database: MTBLS9507.
2.2.3 Integrated analysis of transcriptomic and metabolomic variability
To ascertain the relationship between the transcriptomic and metabolomic data, the Pearson Correlation Coefficient (PCC) was computed and the corresponding p-values for genes and metabolites were calculated using the ‘cor’ function in R. This enabled us to assess the connection between differentially expressed metabolites (DMs) and differentially expressed genes (DEGs), only those with a PCC greater than 0.80 and a p-value less than 0.05 were considered significant. Given the significance of DEGs at 4 h in both populations, we focused on scrutinizing the correlations between DEGs and DMs in the TH04-4 h versus Mx-4 h comparison.
2.3 Quercetin feeding method
The quercetin (CAS number: 6151-25-3) used in this experiment had a purity of 97% and was purchased from Shanghai McLean Biotechnology Co., Ltd. The quercetin was prepared in dimethyl sulfoxide (DMSO). For the quercetin feeding experiment, a total of 480 shrimps (L. vannamei; mean weight 3.65 ± 0.93 g, n=480) were divided into three experimental groups and one control group, each containing 120 shrimps. After one week of acclimation, the shrimp were evenly distributed across 12 buckets designated for the quercetin feeding experiment. The barrels were organized into four groups, each representing a different concentration of quercetin feeding experiment, along with one blank control. Each group consisted of three replicates and housed 40 shrimps per barrel. The experimental groups involved supplementation of 0.1 g, 1 g, and 2 g of quercetin per 100 g of commercial feed. The control group was provided commercial feed without quercetin supplementation. The experiment lasted for 14 days. During this period, shrimp were fed three times daily (at 9:00 AM, 1:00 PM, and 6:00 PM) with feed constituting 5% of their body weight per day. Waste removal was conducted 3-4 hours after each feeding session, and one-third of the water was replaced daily. The water conditions in the culture tank were maintaining a temperature of 27 ± 0.5°C, a salinity level between 30-33‰, and a pH value of 7.2 ± 0.2, with a third of the water being refreshed daily. To ensure optimal water quality, aeration was provided to keep the dissolved oxygen levels at approximately 8 mg/L. These water quality parameters were sustained throughout the test period to fulfill the growth requirements of the shrimp. The statistical method of VP challenge and survival rate is the same as in 2.1. The hepatopancreas tissue of each shrimp was obtained after 24 h of VP challenge and was pre-treated with liquid nitrogen and stored at -80°C for subsequent RNA extraction. The death of shrimp was recorded every 4 h after the challenge, and the mortality rate was calculated. To verify the effect of quercetin on VP infection in L. vannamei, primers were designed to detect the expression of immune-related genes by RT-qPCR (Supplementary Table S1).
2.4 RT-qPCR assays
Total RNA was isolated from the hepatopancreas, and the expression of immune-related genes was detected by RT-qPCR to improve the reliability and accuracy of the transcriptome data. The designed primers are shown in Supplementary Table S1. In the RT-qPCR analysis, β-actin was used as an internal reference gene. The RT-qPCR reaction mixture (20 μL) was carefully prepared from 10 μL 2 × Power SYBR Green PCR Master mixture, 400 nM forward and reverse primers, and 1 μL template cDNA. Subsequently, PCR amplification was carried out through a series of 40 cycles, including initial denaturation at 95°C for 2 min, denaturation at 95°C for 15 s, annealing at 60°C for 20 s, extension at 72°C for 30 s, and finally, cooling at 4°C for 5 min. The 2-ΔΔCT method was employed to quantify the relative expression of the target genes and the β-actin gene (Michaelidou et al., 2013). The data are reported in the form of relative mRNA expression levels.
2.5 Statistical analysis
All data are presented as the mean ± SD. One-way ANOVA and Duncan multiple tests were used to conduct between-group comparisons of numerical data. Origin software was used to generate a Kaplan ± Meier plot (log-rank χ2 test) for the analysis of the mortality or survival rates.
3 Results
3.1 Differences in the survival rates of the Mx and TH04 populations
To select shrimp populations with significant differences in resistance to VP, infection experiments were carried out by the artificial injection method. The results showed that the survival rates of the Mx, TH04, TH06, Mx01, CTLL, and CTS populations were 71.38%, 0%, 23.72%, 39.14%, 18.82%, and 14.49%, respectively (Figure 1). The survival rate of the Mx population was significantly higher than that of the TH04 population. Thus, among the six populations, Mx exhibited the strongest resistance against VP with the highest survival rate, while TH04 showed the weakest resistance and lowest survival rate. Accordingly, these two populations with the greatest difference in VP resistance were chosen as the focus of the transcriptional and metabolome analyses.
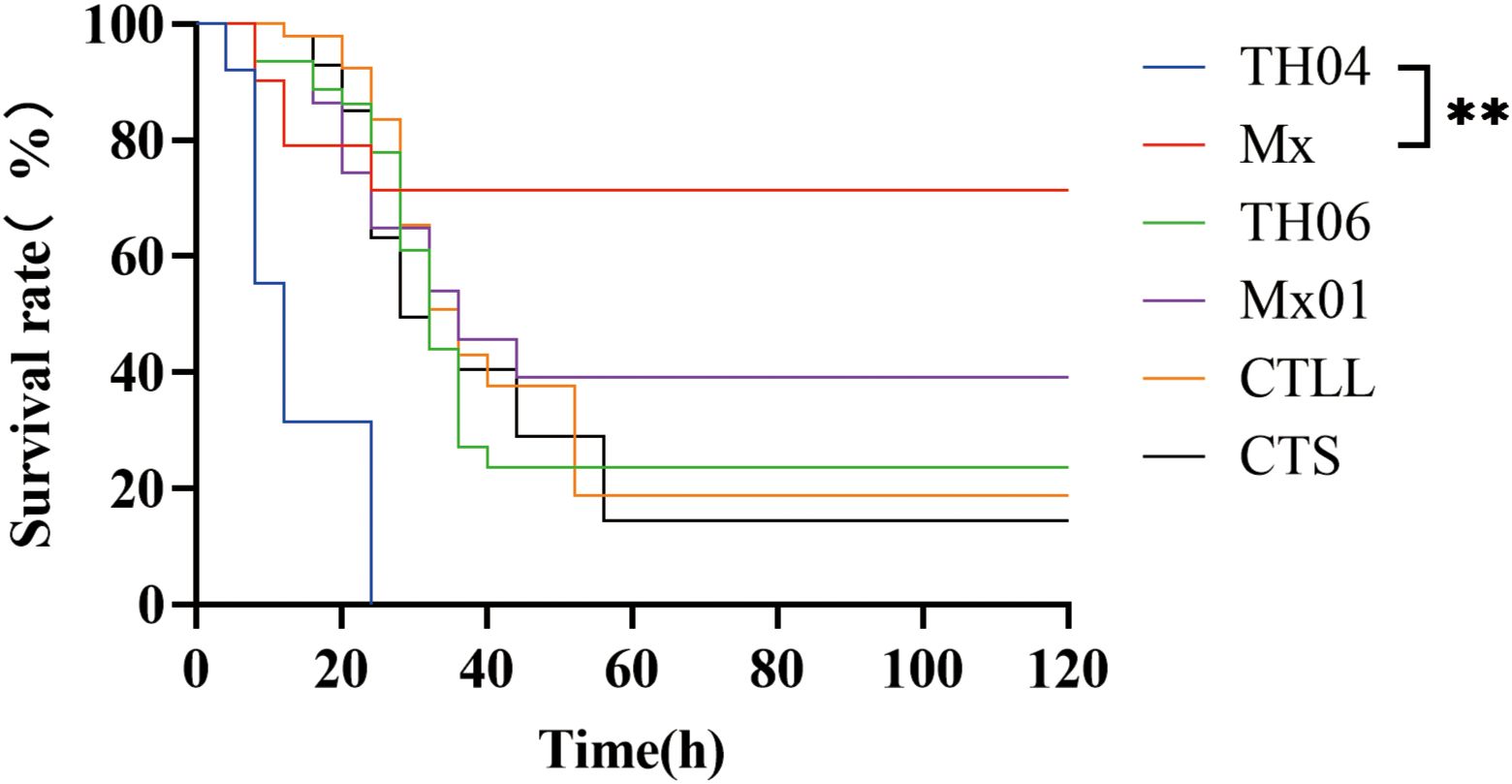
Figure 1 Survival rates of six L. vannamei populations infected with VP. “**” indicate significant differences, and the significance levels were P<0.01.
3.2 Identification of differential genes and metabolites following VP infection
3.2.1 Differential gene and metabolite analysis
Transcriptome analysis of the hepatopancreas tissue of TH04 and Mx shrimp infected with VP was performed using an Illumina Novaseq™ 6000 sequencer. The resulting dataset comprised a total of 859,258,916 raw reads from the shrimp samples. Following stringent quality control procedures, 20,948 expressed genes were retained for further analysis (Supplementary Table S2). In the TH04 population, 530 upregulated genes and 3,020 downregulated genes were identified after 4 h of VP infection, and 534 upregulated genes and 1,013 downregulated genes were identified after 24 h of VP infection. In the Mx population, 512 upregulated genes and 175 downregulated genes were identified after 4 h of VP infection, and 396 upregulated genes and 45 downregulated genes were identified after 24 h of VP infection (Figure 2A). The analysis revealed that there were more downregulated genes in the TH04 population than in the Mx population after 4 h of VP challenge. A Venn diagram was used to analyze the differential genes in the Mx and TH04 populations after 4 h of VP infection. 115 downregulated DEGs were overlapping between the TH04 and Mx populations, whereas 60 downregulated DEGs in Mx population were distinct from 2,905 downregulated DEGs in TH04 population (Figure 2B). 79 upregulated DEGs were overlapping between the TH04 and Mx populations, while the 433 upregulated DEGs in Mx population differed from the 451 upregulated DEGs in TH04 population (Figure 2C). To validate the sequencing data, the upregulated DEGs in the Mx population and the downregulated DEGs in the TH04 population were selected for RT-qPCR analysis. The results (Supplementary Figure S2) showed that the expression profiles of the transcriptome data and the RT-qPCR data were consistent.
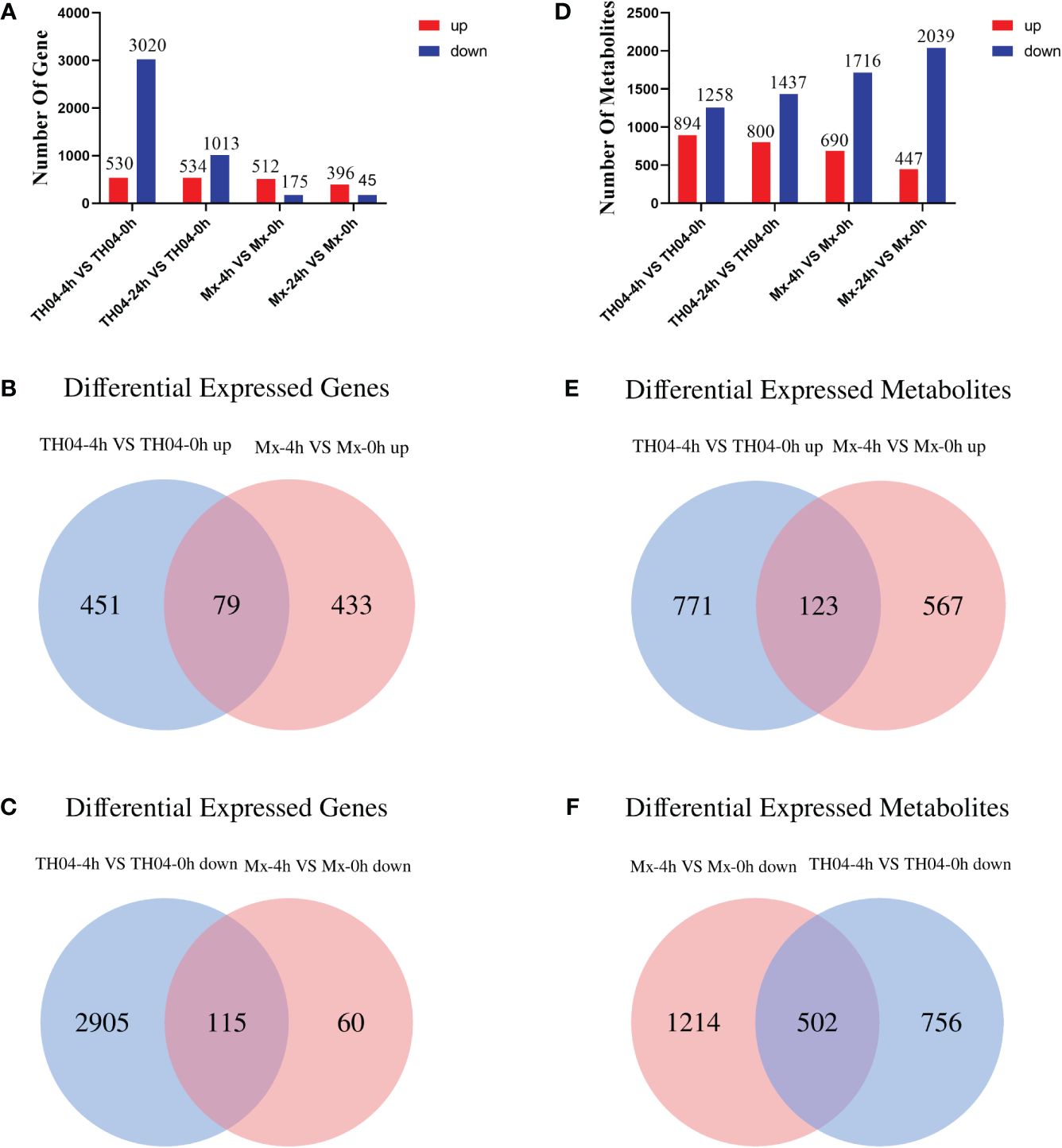
Figure 2 Statistical analysis of DEGs and DMs. (A, D) The numbers of DEGs and DMs at 4 h and 24 h of VP infection in the TH04 and Mx populations. Red color represents upregulation and blue color represents downregulation. (B, C) Venn diagram showing differentially upregulated and downregulated DEGs in the TH04 and Mx populations at 4 h after VP infection. (E, F) Venn diagram showing differentially upregulated and downregulated DMs in the TH04 and Mx populations at 4 h after VP infection. Significant differences were p < 0.05.
LC-MS was used to analyze the non-targeted metabolomics of the hepatopancreas tissue samples of L. vannamei, a total of 5,980 metabolites were detected. The PCA clustering results show that there are differences in metabolites between TH04 and Mx populations after VP infection (Supplementary Figure S3). In the TH04 population, 894 upregulated metabolites and 1,258 downregulated metabolites were identified after 4 h of VP infection while 800 upregulated metabolites and 1,437 downregulated metabolites were identified after 24 h of VP infection. In the Mx population, 690 upregulated metabolites and 1,716 downregulated metabolites were identified after 4 h of VP infection while 447 upregulated metabolites and 2,039 downregulated metabolites were identified after 24 h of VP infection (Figure 2D). A Venn diagram was used to analyze the differential metabolites in the Mx and TH04 populations after 4 h of VP infection. 502 downregulated DMs were overlapping between the TH04 and Mx populations, whereas 1,214 downregulated DMs in Mx population were distinct from 756 downregulated DMs in TH04 population (Figure 2E). 123 upregulated DMs were overlapping between the TH04 and Mx populations, while the 567 upregulated DMs in Mx population differed from the 771 upregulated DMs in TH04 population (Figure 2F).
3.2.2 Differential gene and metabolite annotation
To explore the immune-related genes and pathways in the hepatopancreas of L. vannamei after VP infection, KEGG pathway enrichment analysis was performed. Compared with the control group, after 4 h of VP infection, the amoeba disease and phagosome pathways were significantly enriched in the 79 genes co-upregulated in the two populations (TH04, Mx), while the 433 genes specifically upregulated in the Mx population were related to fructose and mannose metabolism and lysosome, and the 451 genes specifically upregulated in the TH04 population were related to ribosome and purine metabolism. Compared with the control group, after 4 h of VP infection, the lysosomal and arachidonic acid pathways were significantly enriched in the 115 genes co-downregulated in the two populations (TH04, Mx), and the significantly enriched pathways in the 60 genes downregulated in the Mx population included purine metabolism and metabolism of xenobiotics by cytochrome P450. Among the 2,905 genes specifically downregulated in the TH04 population, the significantly enriched pathways included phagosome and endocytosis (Supplementary Table S3).
Non-targeted metabolomics was also used to analyze the DMs in the hepatopancreas of L. vannamei 4 h after VP infection. To further explore the potential metabolic pathways involved in VP infection of L. vannamei at 4 h, KEGG pathway enrichment analysis was performed. Compared with the control group, metabolic pathways and nucleotide metabolism were significantly enriched in the 123 metabolites co-upregulated in the Mx population and TH04 population 4 h after VP infection, while metabolic pathways and biosynthesis of cofactors were significantly enriched in the 567 metabolites specifically upregulated in the Mx population. Metabolic pathways and ABC transporters were significantly enriched in the 771 metabolites specifically upregulated in the TH04 population. Compared with the control group, 4 h after VP infection, the KEGG pathways significantly enriched in the 502 metabolites co-downregulated in the Mx and TH04 populations were metabolic pathways and ABC transporters, while the KEGG pathways significantly enriched in the 756 metabolites specifically downregulated in the Mx population and the 1,214 metabolites specifically downregulated by the TH04 population were metabolic pathways and ABC transporters (Supplementary Table S4).
3.2.3 Integration of transcriptomics and metabolomics analyses
Metabolomics analysis identified a total of 12 flavonoid compounds, including quercetin, formononetin, and ipriflavone. Differential metabolite analysis revealed that, except for Rebaudioside B (MW0169755), the levels of 11 flavonoid compounds, including quercetin (MW0009623), formononetin (MW0124161) and ipriflavone (MW0124440), were markedly higher in the Mx population, which exhibited strong resistance against VP, compared with the TH04 population, which exhibited weak resistance traits. Notably, quercetin displayed the most pronounced difference in metabolite levels (Figure 3A). A correlation analysis of genes and metabolites revealed that 128 genes were related to flavonoid metabolism, including 11 genes related to quercetin metabolism (Figure 3B). The transcriptome differential analysis revealed that, among the 128 genes associated with flavonoid metabolism, 66 genes displayed significantly higher expression levels in the Mx population than in the TH04 population (Supplementary Table S5). Among them, 11 genes related to quercetin showed a significant upregulation trend in the Mx population (Figure 3C). The integrated transcriptome and metabolome analysis suggested that the metabolic levels of quercetin could impact the resistance traits of shrimp against VP.
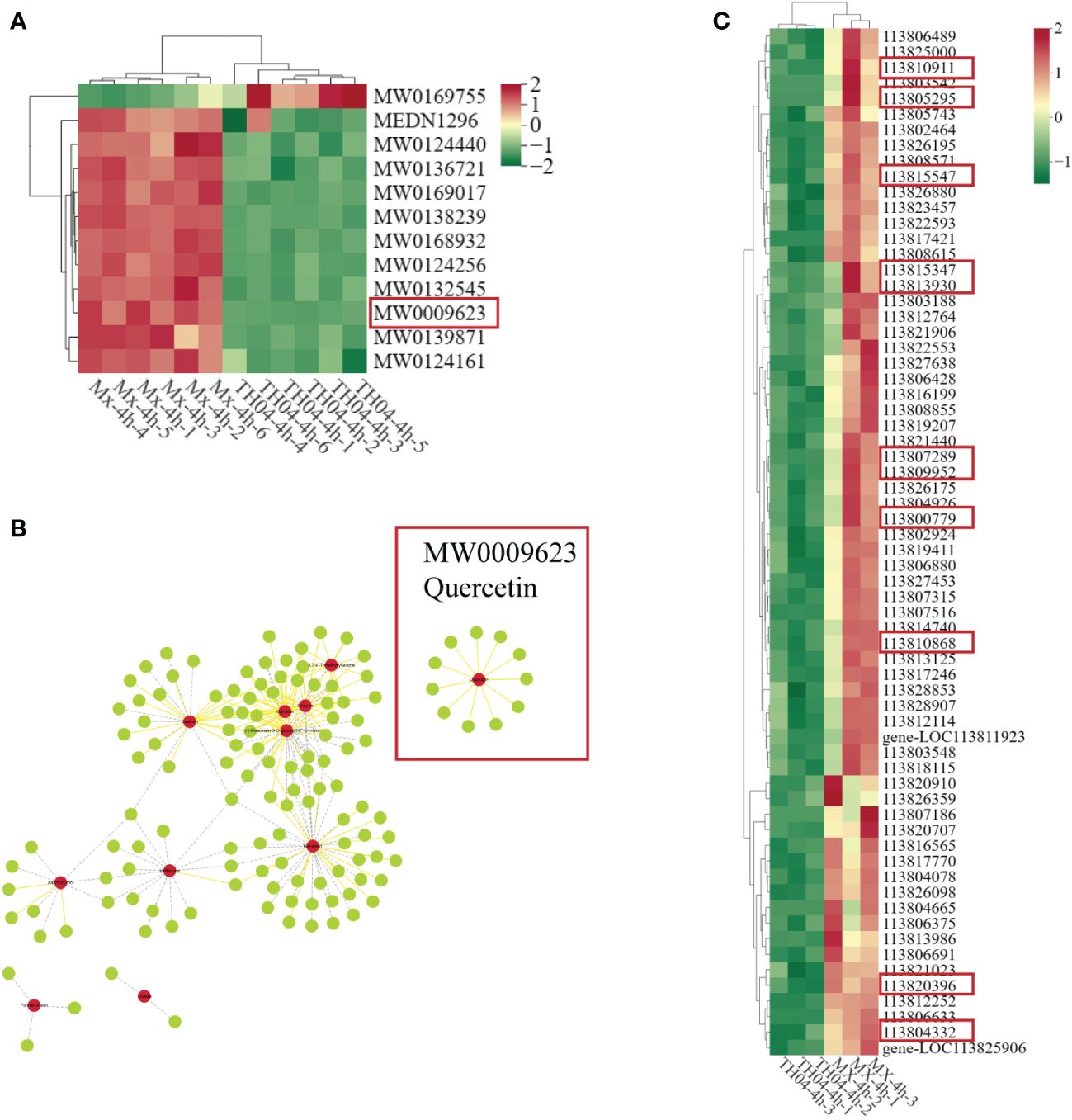
Figure 3 Correlation analysis of metabolomic and transcriptomic data. (A) Metabolomic analysis of heat maps of DMs associated with flavonoids in the TH04 and Mx populations (quercetin is in the red box). (B) Network diagram of genes associated with flavonoids (genes associated with quercetin are in red boxes). Solid yellow lines indicate positive correlations and dashed grey lines indicate negative correlations; red circles are metabolites and green circles are genes. (C) Transcriptomics analysis of heat maps of DEGs associated with flavonoids in the TH04 and Mx populations.
3.3 Effect of quercetin on L. vannamei infected with VP
Further experiments were conducted to verify the effect of quercetin on VP infection in L. vannamei. Quercetin was added to commercial shrimp feed at 0.1 g, 1 g, and 2 g of quercetin per 100 g of feed. Shrimp were fed twice per day, with a feeding rate of 5% of body weight. After continuous feeding for 14 days, on the 15th day, all shrimp were infected with VP for challenge testing (Figure 4A). The survival rate over five days was calculated, and the results showed that the control group without quercetin supplementation had a survival rate of 14.79%. The experimental groups that received 1 g of quercetin added to 100 g of feed had a higher survival rate of 78.95%, compared to the 0.1 g and 2 g quercetin supplementation groups (37.61% and 25.08%, respectively). Kaplan-Meier analysis revealed that the experimental group that received 1 g of quercetin added to 100 g of feed had a significantly higher survival rate than the control group, while there were no significant differences between the control group and the experimental groups that received 0.1 g and 2 g of quercetin (Figure 4B).
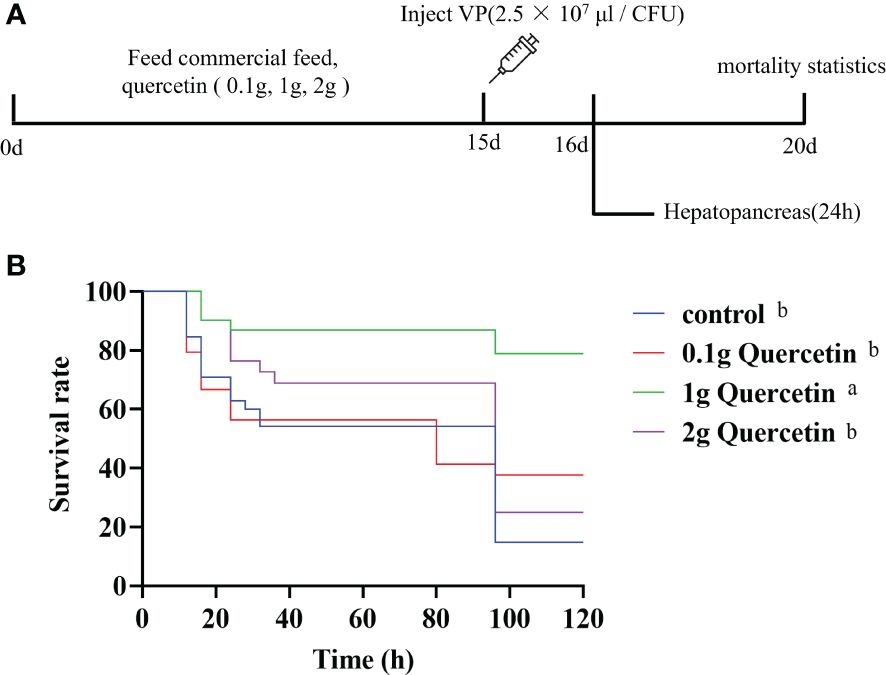
Figure 4 Effect of quercetin on L. vannamei infected with VP. (A) Course of the quercetin feeding experimental program. (B) The survival rate of the different experimental groups. Different letters show significant differences by ANOVA (p < 0.05).
The effect of quercetin on the anti-Vibrio traits of shrimp was verified by RT-qPCR. The relative expression levels of immune-related genes (Toll-like receptor, anti-lipopolysaccharide factor, crustin, lysozyme, cathepsin B) and quercetin-related genes (hypothetical protein C7M84_016793, zinc/cadmium resistance protein-like, transcription factor activator protein-1 and holotricin-3) were verified. The results showed that compared to the control group, the experimental group fed quercetin had high expression of Toll-like receptor, anti-lipopolysaccharide factor, crustin, lysozyme, cathepsin B, hypothetical protein C7M84_016793, zinc/cadmium resistance protein-like, transcription factor activator protein-1 and holotricin-3-like. The expression of the genes in the 1 g quercetin group was significantly higher than that in the groups fed 0.1 g and 2 g quercetin (Figure 5). These findings indicate that the addition of quercetin to the diet of L. vannamei had a positive impact on their resistance to VP infection; quercetin supplementation at an appropriate concentration enhanced the immune response of the shrimp.
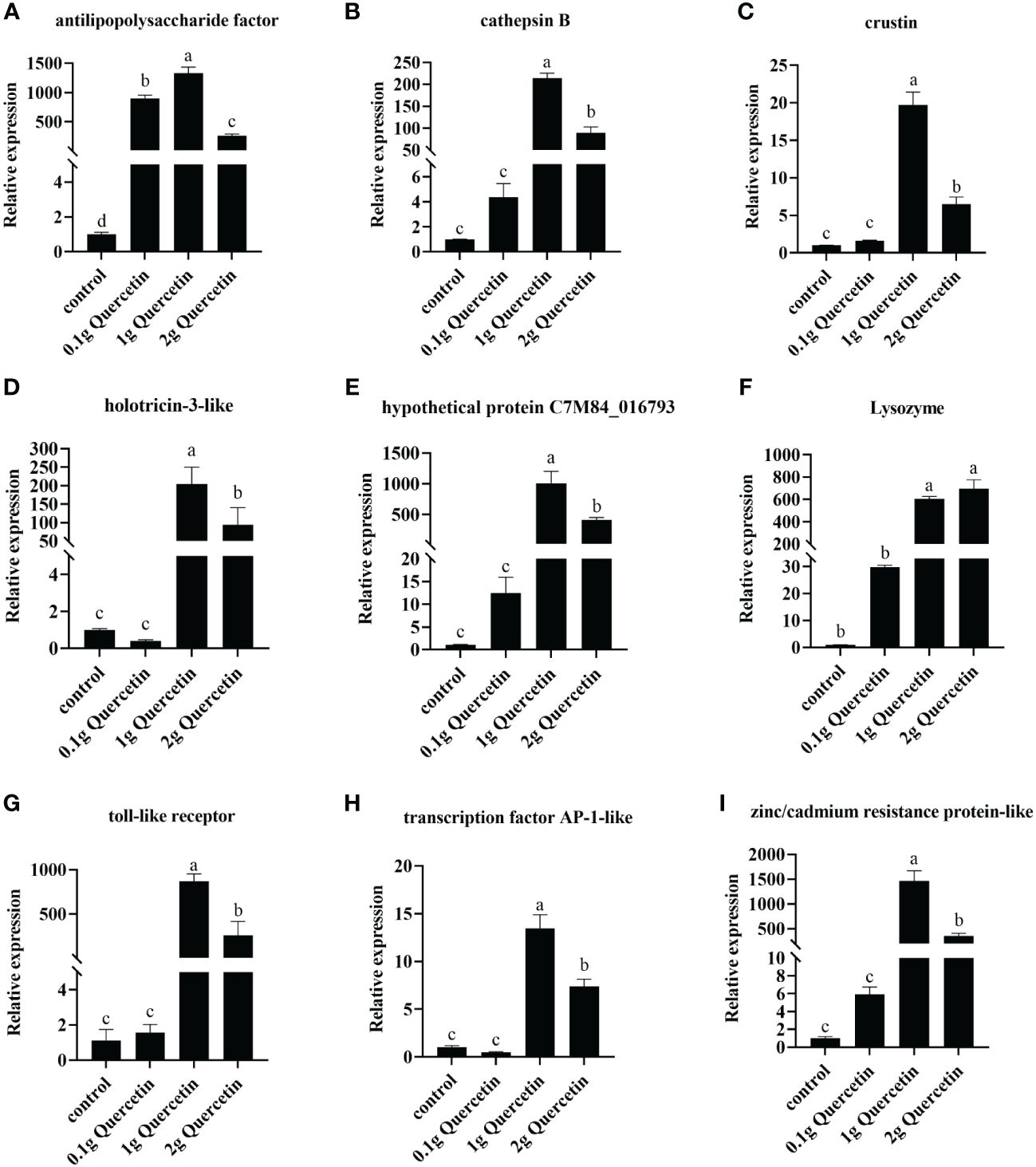
Figure 5 Gene expression of immune-related factors in L. vannamei after feeding with different levels of quercetin and challenge with Vibrio. (A) Anti-lipopolysaccharide factor gene, (B) cathepsin B, (C) crustin, (D) holotricin-3-like, (E) hypothetical protein C7M84_016793, (F) lysozyme, (G) Toll-like receptor, (H) transcription factor AP-1-like, and (I) zinc/cadmium resistance protein-like in the hepatopancreas of L. vannamei infected with VP for 24 h, under the action of different concentrations of quercetin. One-way ANOVA and Duncan’s multiple comparisons test were used; Different letters show significant differences by ANOVA (p< 0.05). Expression levels were obtained for all three biological replicates (n=3). Error bars indicate the standard error of the mean of the three replicates of the organism.
4 Discussion
VP is a commonly encountered opportunistic pathogen capable of causing AHPND, leading to mortality in shrimp. In this study, transcriptomics and metabolomics were used to explore the differential gene expression and metabolites in Mx and TH04 populations, which exhibited significant differences in resistance to VP. Transcriptome and metabolome sequencing identified 20,948 expressed genes and 5,980 metabolites. Following a 4 h VP infection, 687 genes and 2,406 metabolites exhibited significant changes in the Mx population, while 3,550 genes and 2,152 metabolites showed significant alterations in the TH04 population. The transcriptomic analysis of Exopalaemon carinicauda infected with VP highlighted a marked predominance of downregulated differential genes compared to upregulated genes following the infection (Ge et al., 2017). Moreover, families of L. vannamei with weak resistance to VP showed a higher count of downregulated DEG (Liu et al., 2023). This study’s transcriptomic analysis revealed that, after VP exposure, the TH04 population, with weaker resistance, exhibited significantly more downregulated genes than the highly resistant Mx population. This observation aligns with findings from previous research. This outcome could stem from variables like gene expression patterns across populations, the activation of immune pathways, or variations in how hosts recognize pathogens. Earlier research has demonstrated that infection with Vibrio harveyi notably enhances metabolic pathways related to energy, amino acids, and carbohydrates in shrimp (Ma et al., 2020). There are notable disparities in resistance to VPAHPND among various L. vannamei families (Sun et al., 2023a). In this study, metabolomics analysis showed that after exposure to VP, both TH04 and Mx populations exhibited significant enrichment in metabolic pathways, indicating considerable alterations in host metabolism potentially linked to the severity of VPAHPND. These findings suggest that metabolic dysregulation may play a role in facilitating Vibrio proliferation (Lai et al., 2015). To explore the critical metabolites influencing shrimp resistance to VP, further correlation analyses between differentially expressed genes and metabolites in the Mx and TH04 populations revealed notable differences in the expression of flavonoids and their related genes.
To explore the critical metabolites influencing shrimp resistance to VP, further correlation analyses between differentially expressed genes and metabolites in the Mx and TH04 populations revealed notable differences in the expression of flavonoids and their related genes. Particularly, quercetin, among the 11 types of flavonoid compounds, displayed significantly higher metabolic levels and gene activity in the Mx population compared to the TH04 population, suggesting a potential impact of quercetin and other flavonoids on shrimp resistance to VP. Flavonoids play vital roles in the antioxidant and antibacterial effects (Aisiah et al., 2022), flavonoids intervention leads to the disintegration of cell walls and membranes, disruption of metabolic processes and redox balance, and nucleic acid damage in Shewanella putrefaciens, ultimately culminating in cellular demise (Wang et al., 2020a). In addition, transcriptome and metabolomics research indicated that flavonoid biosynthesis may be key to Camellia oleifera’s resistance against Anthracnose (Yang et al., 2022).
Quercetin as a typical representative of flavonoids and the most important bioflavonoid in the human diet, is widely found in plants (Petersen et al., 2016; Hai et al., 2020; Wang et al., 2020b). In recent years, Quercetin has become a popular feed additive because of its properties of growth-promoting, anti-oxidation, lipid metabolism-regulating, anti-carcinogenicity, immunity-enhancing, anti-virus, and anti-bacterial (Wang et al., 2016). In this study, the integrated analysis of the transcriptomic and metabolomic unveiled flavonoid compounds, specifically quercetin, as crucial metabolites underlying the disparities in VP resistance between the Mx and TH04 populations. Furthermore, the gradient feeding experiment revealed that the inclusion of 1 g of quercetin per 100 g of feed enhanced the ability of shrimp to resist VP and reduced the mortality rate. Notably, feeding 0.1 g and 2 g of quercetin per 100 g of shrimp feed did not significantly improve the immune capacity of shrimp against VP, indicating that there is an optimal dosage range for the utilization of quercetin. Previous studies have shown that the combination of quercetin and florfenicol can enhance disease resistance, survival, and immune activity in VPAHPND-infected shrimp (Zhai et al., 2022), notably, the most significant enhancement in immune factor activity for Cyprinus carpio occurred when 100 mg of quercetin was added per 1 kg of feed (Sun et al., 2023b). Shi et al. reported that quercetin could enhance immunity by regulating the gene expression of the NF-κB signaling pathway and AMPs in Bombyx mori (Shi et al., 2021). Following 24 hours of VP infection, the expression levels of genes including Toll-like receptor, crustin, and transcription factor activation protein 1 were significantly enhanced after the incorporation of quercetin into the feed. Furthermore, the peak activity of the shrimp immune pathway was observed with a quercetin feeding concentration of 1 g/100 g of feed. The NF-κB pathway plays a vital role in the innate immunity of shrimp and includes genes such as Toll-like receptor, crustin, and transcription factor activation protein, which contribute to the antibacterial immune response (Yang et al., 2008; Li and Xiang, 2013). In shrimp, crustin is predominantly expressed in haemocytes and gills, where it demonstrates antibacterial or antiviral activities. These activities are commonly regulated by the NF-κB pathway (Toll and IMD pathways) (Liu et al., 2015; Sun et al., 2017). Research indicates that increasing levels of quercetin supplementation in the feed result in an initial increase followed by a decrease in the activities of serum acid phosphatase (ACP), lysozyme (LZM), and the content of immunoglobulin M (IgM) in Cyprinus carpio (Sun et al., 2023b).
5 Conclusions
This study aimed to investigate the underlying reasons for phenotypic differences in the resistance of L. vannamei to VP using transcriptome and metabolome sequencing techniques. Joint analysis of transcriptome and metabolome data revealed that flavonoid compounds, particularly quercetin, play a role in the varying degrees of resistance to VP among the Mx and TH04 populations. Supplementation of shrimp feed with 1 g of quercetin per 100 g of feed enhanced the expression of the Toll-like receptor, crustin, and transcription factor activation protein genes in the NF-κB pathway, leading to enhanced resistance against VP and a decreased mortality rate. These findings indicate that the incorporation of an appropriate amount of quercetin into shrimp feed can effectively boost the immune capacity of shrimp against VP and lower the incidence of AHPND.
Data availability statement
The raw mRNA sequencing data has been uploaded to the NCBI Sequence Read Archive (SRA) submission: PRJNA1063533. Metabolomics data has been deposited in the MetaboLights database: MTBLS9507.
Ethics statement
The animal study was approved by China, the Animal Ethics Committee’s scope of approval is limited to studies involving vertebrates (Laboratory Animal Act, Chapter 5Article 25.Our study is on invertebrate species, and does not need ethical approval in China. The study was conducted in accordance with the local legislation and institutional requirements.
Author contributions
YS: Resources, Writing – review & editing. JJ: Conceptualization, Investigation, Methodology, Software, Writing – original draft. NC: Formal analysis, Writing – review & editing. YH: Formal analysis, Writing – review & editing. YC: Formal analysis, Writing – review & editing. BY: Formal analysis, Writing – review & editing. XW: Formal analysis, Writing – review & editing. SL: Resources, Supervision, Writing – review & editing.
Funding
The author(s) declare financial support was received for the research, authorship, and/or publication of this article. This research was funded by National Key Research and Development Program of China, grant number 2022YFD2400204, the open competition program of top ten critical priorities of Agricultural Science and Technology Innovation for the 14th Five-Year Plan of Guangdong Province, grant number 2022SDZG01. We are especially grateful for the critical comments from the anonymous reviewers.
Conflict of interest
Authors NC, YH, YC, and SL were employed by the company Guangdong Evergreen Feed Industry Co., Ltd.
The remaining authors declare that the research was conducted in the absence of any commercial or financial relationships that could be construed as a potential conflict of interest.
Publisher’s note
All claims expressed in this article are solely those of the authors and do not necessarily represent those of their affiliated organizations, or those of the publisher, the editors and the reviewers. Any product that may be evaluated in this article, or claim that may be made by its manufacturer, is not guaranteed or endorsed by the publisher.
Supplementary material
The Supplementary Material for this article can be found online at: https://www.frontiersin.org/articles/10.3389/fmars.2024.1400082/full#supplementary-material
References
Ahmadifar E., Eslami M., Kalhor N., Zaretabar A., Mohammadzadeh S., Moghadam M. S., et al. (2022). Effect of a diet enriched with sodium propionate on growth performance, antioxidant property, innate-adaptive immune response, and growth-related genes expression in critically endangered beluga sturgeon (Huso huso). Fish Shellfish Immunol. 125, 101–108. doi: 10.1016/j.fsi.2022.04.031
Ahmadifar E., Yousefi M., Karimi M., Fadaei Raieni R., Dadar M., Yilmaz S., et al. (2021). Benefits of dietary polyphenols and polyphenol-rich additives to aquatic animal health: an overview. Rev. Fisheries Sci. Aquaculture 29, 478–511. doi: 10.1080/23308249.2020.1818689
Aisiah S., Rini R. K., Tanod W. A., Fatmawati F., Fauzana N. A., Olga O., et al. (2022). Metabolomic profiling of Jeruju (Acanthus ilicifolius) leaf extract with antioxidant and antibacterial activity on Aeromonas hydrophila growth. J. Appl. Pharm. Sci. 12, 057–069. doi: 10.7324/JAPS.2022.120807
Anand David A. V., Arulmoli R., Parasuraman S. (2016). Overviews of biological importance of quercetin: A bioactive flavonoid. Pharmacogn Rev. 10, 84–89. doi: 10.4103/0973-7847.194044
Baghel S., Shrivastava N., Baghel P., Rajput S. (2012). A review of quercetin: Antioxidant and anticancer properties. World J. Pharm. Pharm. Sci. 1, 146–160.
Bujak R., Struck-Lewicka W., Markuszewski M. J., Kaliszan R. (2015). Metabolomics for laboratory diagnostics. J. Pharm. BioMed. Anal. 113, 108–120. doi: 10.1016/j.jpba.2014.12.017
De Los Santos M. V., Sánchez-Salgado J. L., Pereyra A., Zenteno E., Vibanco-Pérez N., Ramos-Clamont Montfort G., et al. (2022). The Vibrio parahaemolyticus subunit toxin PirB recognizes glycoproteins on the epithelium of the Penaeus vannamei hepatopancreas. Comp. Biochem. Physiol. Part B: Biochem. Mol. Biol. 257, 110673. doi: 10.1016/j.cbpb.2021.110673
FAO (2022). “The state of world fisheries and aquaculture 2022,” in Towards blue transformation (Beijing: China Agricultural Publishing House).
Ge Q., Li J., Wang J., Li J., Ge H., Zhai Q. (2017). Transcriptome analysis of the hepatopancreas in Exopalaemon carinicauda infected with an AHPND-causing strain of Vibrio parahaemolyticus. Fish Shellfish Immunol. 67, 620–633. doi: 10.1016/j.fsi.2017.06.047
Hai Y., Zhang Y., Liang Y., Ma X., Qi X., Xiao J., et al. (2020). Advance on the absorption, metabolism, and efficacy exertion of quercetin and its important derivatives: Absorption, metabolism and function of quercetin. Food Front. 1, 420–434. doi: 10.1002/fft2.50
Kewcharoen W., Srisapoome P. (2019). Probiotic effects of Bacillus spp. from Pacific white shrimp (Litopenaeus vannamei) on water quality and shrimp growth, immune responses, and resistance to Vibrio parahaemolyticus (AHPND strains). Fish Shellfish Immunol. 94, 175–189. doi: 10.1016/j.fsi.2019.09.013
Khan S., Ince-Dunn G., Suomalainen A., Elo L. L. (2020). Integrative omics approaches provide biological and clinical insights: examples from mitochondrial diseases. J. Clin. Invest. 130, 20–28. doi: 10.1172/JCI129202
Kong Y., Tian J., Niu X., Li M., Kong Y., Li R., et al. (2022). Effects of dietary quercetin on growth, antioxidant capacity, immune response and immune-related gene expression in snakehead fish, Channa argus. Aquaculture Rep. 26, 101314. doi: 10.1016/j.aqrep.2022.101314
Lai H.-C., Ng T. H., Ando M., Lee C.-T., Chen I.-T., Chuang J.-C., et al. (2015). Pathogenesis of acute hepatopancreatic necrosis disease (AHPND) in shrimp. Fish Shellfish Immunol. 47, 1006–1014. doi: 10.1016/j.fsi.2015.11.008
Lee C.-T., Chen I.-T., Yang Y.-T., Ko T.-P., Huang Y.-T., Huang J.-Y., et al. (2015). The opportunistic marine pathogen Vibrio parahaemolyticus becomes virulent by acquiring a plasmid that expresses a deadly toxin. Proc. Natl. Acad. Sci. U.S.A. 112, 10798–10803. doi: 10.1073/pnas.1503129112
Lesjak M., Beara I., Simin N., Pintać D., Majkić T., Bekvalac K., et al. (2018). Antioxidant and anti-inflammatory activities of quercetin and its derivatives. J. Funct. Foods 40, 68–75. doi: 10.1016/j.jff.2017.10.047
Li F., Xiang J. (2013). Signaling pathways regulating innate immune responses in shrimp. Fish Shellfish Immunol. 34, 973–980. doi: 10.1016/j.fsi.2012.08.023
Li S., Li W., Chen F., Zhu X., Chen H.-Y., Hao H., et al. (2023). Metabolomic and transcriptomic analysis reveals immune and hormone modulation at the molting stage of juvenile mud crabs challenged with Staphylococcus aureus and Vibrio alginolyticus. Aquaculture 575, 739775. doi: 10.1016/j.aquaculture.2023.739775
Li S., Zhu S., Li C., Zhang Z., Zhou L., Wang S., et al. (2013). Characterization of microRNAs in mud crab Scylla paramamosain under Vibrio parahaemolyticus infection. PloS One 8, e73392. doi: 10.1371/journal.pone.0073392
Liu N., Lan J.-F., Sun J.-J., Jia W.-M., Zhao X.-F., Wang J.-X. (2015). A novel crustin from Marsupenaeus japonicus promotes hemocyte phagocytosis. Dev. Comp. Immunol. 49, 313–322. doi: 10.1016/j.dci.2014.11.021
Liu Y., Yu Y., Li S., Sun M., Li F. (2023). Comparative transcriptomic analysis of gill reveals genes belonging to mTORC1 signaling pathway associated with the resistance trait of shrimp to VPAHPND. Front. Immunol. 14. doi: 10.3389/fimmu.2023.1150628
Ma S., Kim A., Lee W., Kim S., Lee S., Yoon D., et al. (2020). Vibrio harveyi Infection Significantly Alters Amino Acid and Carbohydrate Metabolism in Whiteleg Shrimp, Litopenaeus vannamei. Metabolites 10, 265. doi: 10.3390/metabo10060265
Michaelidou K., Tzovaras A., Missitzis I., Ardavanis A., Scorilas A. (2013). The expression of the CEACAM19 gene, a novel member of the CEA family, is associated with breast cancer progression. Int. J. Oncol. 42, 1770–1777. doi: 10.3892/ijo.2013.1860
Patti G. J., Yanes O., Siuzdak G. (2012). Innovation: Metabolomics: the apogee of the omics trilogy. Nat. Rev. Mol. Cell Biol. 13, 263–269. doi: 10.1038/nrm3314
Petersen B., Egert S., Bosy-Westphal A., Müller M. J., Wolffram S., Hubbermann E. M., et al. (2016). Bioavailability of quercetin in humans and the influence of food matrix comparing quercetin capsules and different apple sources. Food Res. Int. 88, 159–165. doi: 10.1016/j.foodres.2016.02.013
Ren X., Liu P., Li J. (2019). Comparative transcriptomic analysis of Marsupenaeus japonicus hepatopancreas in response to Vibrio parahaemolyticus and white spot syndrome virus. Fish Shellfish Immunol. 87, 755–764. doi: 10.1016/j.fsi.2019.02.030
Saito K., Matsuda F. (2010). Metabolomics for functional genomics, systems biology, and biotechnology. Annu. Rev. Plant Biol. 61, 463–489. doi: 10.1146/annurev.arplant.043008.092035
Shah N. J., Sureshkumar S., Shewade D. G. (2015). Metabolomics: a tool ahead for understanding molecular mechanisms of drugs and diseases. Indian J. Clin. Biochem. 30, 247–254. doi: 10.1007/s12291-014-0455-z
Shi G., Kang Z., Liu H., Ren F., Zhou Y. (2021). The effects of quercetin combined with nucleopolyhedrovirus on the growth and immune response in the silkworm (Bombyx mori). Arch. Insect Biochem. Physiol. 108, e21839. doi: 10.1002/arch.21839
Soto-Rodriguez S. A., Gomez-Gil B., Lozano-Olvera R., Betancourt-Lozano M., Morales-Covarrubias M. S. (2015). Field and experimental evidence of Vibrio parahaemolyticus as the causative agent of acute hepatopancreatic necrosis disease of cultured shrimp (Litopenaeus vannamei) in Northwestern Mexico. Appl. Environ. Microbiol. 81, 1689–1699. doi: 10.1128/AEM.03610-14
Sun B., Wang Z., Zhu F. (2017). The crustin-like peptide plays opposite role in shrimp immune response to Vibrio alginolyticus and white spot syndrome virus (WSSV) infection. Fish Shellfish Immunol. 66, 487–496. doi: 10.1016/j.fsi.2017.05.055
Sun M., Yu Y., Li S., Liu Y., Zhang X., Li F. (2023a). Integrated application of transcriptomics and metabolomics provides insights into acute hepatopancreatic necrosis disease resistance of Pacific white shrimp Litopenaeus vannamei. mSystems 8, e0006723. doi: 10.1128/msystems.00067-23
Sun R., Cheng Y., Zhang B., Jing S., Wang B., Qi Q., et al. (2023b). Effects of dietary quercetin on growth performance, digestive enzyme activities, immune and antioxidant functions of Cyprinus carpio. Chin. J. Anim. Nut. 35, 2514–2523. doi: 10.5555/20230204672
Wang J., Chi Z., Zhao K., Wang H., Zhang X., Xu F., et al. (2020a). A transcriptome analysis of the antibacterial mechanism of flavonoids from Sedum aizoon L. against Shewanella putrefaciens. World J. Microbiol. Biotechnol. 36, 94. doi: 10.1007/s11274-020-02871-w
Wang W., Sun C., Mao L., Ma P., Liu F., Yang J., et al. (2016). The biological activities, chemical stability, metabolism and delivery systems of quercetin: A review. Trends Food Sci. Technol. 56, 21–38. doi: 10.1016/j.tifs.2016.07.004
Wang J., Zhang C., Zhang J., Xie J., Yang L., Xing Y., et al. (2020b). The effects of quercetin on immunity, antioxidant indices, and disease resistance in zebrafish (Danio rerio). Fish Physiol. Biochem. 46, 759–770. doi: 10.1007/s10695-019-00750-2
Yang C., Wu P., Yao X., Sheng Y., Zhang C., Lin P., et al. (2022). Integrated Transcriptome and Metabolome Analysis Reveals Key Metabolites Involved in Camellia oleifera Defense against Anthracnose. Int. J. Mol. Sci. 23, 536. doi: 10.3390/ijms23010536
Yang C., Zhang J., Li F., Ma H., Zhang Q., Jose Priya T. A., et al. (2008). A Toll receptor from Chinese shrimp Fenneropenaeus chinensis is responsive to Vibrio Anguillarum infection. Fish Shellfish Immunol. 24, 564–574. doi: 10.1016/j.fsi.2007.12.012
Ye Y., Xia M., Mu C., Li R., Wang C. (2016). Acute metabolic response of Portunus trituberculatus to Vibrio alginolyticus infection. Aquaculture 463, 201–208. doi: 10.1016/j.aquaculture.2016.05.041
Zhai Q., Chang Z., Li J., Li J. (2022). Protective effects of combined utilization of quercetin and florfenicol on acute hepatopancreatic necrosis syndrome infected litopenaeus vannamei. Antibiotics 11, 1784. doi: 10.3390/antibiotics11121784
Keywords: Litopenaeus vannamei, quercetin, Vibrio parahaemolyticus, transcriptomic, metabolomic
Citation: Jin J, Chen N, Hu Y, Chen Y, Yin B, Wang X, Shen Y and Li S (2024) Transcriptome and metabolome for identifying key metabolites impacting the Vibrio parahaemolyticus in Litopenaeus vannamei. Front. Mar. Sci. 11:1400082. doi: 10.3389/fmars.2024.1400082
Received: 13 March 2024; Accepted: 09 April 2024;
Published: 26 April 2024.
Edited by:
Ngoc Tuan Tran, Shantou University, ChinaReviewed by:
Yafei Duan, South China Sea Fisheries Research Institute, ChinaQuan Xin Gao, Huzhou University, China
Copyright © 2024 Jin, Chen, Hu, Chen, Yin, Wang, Shen and Li. This is an open-access article distributed under the terms of the Creative Commons Attribution License (CC BY). The use, distribution or reproduction in other forums is permitted, provided the original author(s) and the copyright owner(s) are credited and that the original publication in this journal is cited, in accordance with accepted academic practice. No use, distribution or reproduction is permitted which does not comply with these terms.
*Correspondence: Yuchun Shen, c2hlbnl1Y2h1bkAxNjMuY29t; Sedong Li, bGlzZWRvbmdAMTYzLmNvbQ==