- 1Key Laboratory of Inland Saline-alkaline Aquaculture, Ministry of Agriculture and Rural Affairs, East China Sea Fisheries Research Institute, Chinese Academy of Fishery Sciences, Shanghai, China
- 2Wenchang Innovation Research Center, Fengjiawan Modern Fishery Industry Park, Wenchang, Hainan, China
Syngnathid fishes often experience a reduction in brood size and an increase in immunity during pregnancy. Researchers have studied the polygamous broad-nosed pipefish (Syngnathus typhle) and revealed that some eggs from low-quality females in the male’s brood pouch serve as nurse eggs that are absorbed by the father. It is unclear whether nurse eggs also exist in other syngnathid fishes, especially in monogamous syngnathid fishes. In monogamous syngnathid fishes, the male pouch only carries eggs from a single female. Thus, the question remains: do some eggs of monogamous syngnathid fishes also serve as nurse eggs? And if so, are these nurse eggs impaired eggs, or are they viable eggs that are consumed by the brooding father? In the present study, we used the monogamous lined seahorse (Hippocampus erectus) and asked whether nurse eggs exist in this species. We also explored whether nurse eggs potentially originate from viable versus inviable eggs. Using isotope labeling, we found that nutrients from embryos could be transferred to brooding fathers. Furthermore, we also found that brooding fathers with limited food had a higher isotope content and a smaller brood size compared to individuals with sufficient food. These results have demonstrated that nurse eggs exist in the lined seahorse, and also suggested that the brooding fathers actively consume viable embryos to absorb nutrients in response to low food availability. These findings help us better understand parent–embryo conflict, filial cannibalism, and male-only care in teleost fishes.
1 Introduction
Male-only parental care, where the male is the sole carer of his offspring, is a rare strategy in most animals (Reynolds et al., 2002). However, in teleost fishes, male contributions to offspring care are unusually high, with males acting as the sole carer in more than 50% of families with any parental care (Gross and Sargent, 1985). Various non-mutually exclusive hypotheses have been proposed to explain the prevalence of male-only care in teleost fishes. These include 1) the gamete release order hypothesis, which suggests that the last parent to deposit gametes (usually the male in species showing external fertilization) is left in a “cruel bind” to care for the offspring (Trivers, 1972; Dawkins and Carlisle, 1976); 2) the costs hypothesis, which suggests that males pay less future fecundity costs than females for the same offspring fitness (Gross, 2005); and 3) the external fertilization hypothesis, which suggests that external fertilization increases male confidence in paternity (Kvarnemo, 2006; Sutton and Wilson, 2019). In recent years, the benefits hypothesis, which suggests that males gain benefits from caregiving activity through increased mating success associated with female preferences, has also been proposed (Goldberg et al., 2020). A number of studies have found that male fishes that provide high levels of paternal care are preferred in mate choice (Forsgren, 1997; Östlund and Ahnesjö, 1998; Lindström et al., 2006; Lin et al., 2023), suggesting that males gain the benefit of improving attractiveness from caregiving. Furthermore, in syngnathid fishes, male caregivers have been found to exhibit higher immune capacity during the care period (Roth et al., 2011; Whittington et al., 2015; Lin et al., 2016), suggesting that males gain the benefit of enhancing immunity while caregiving.
Providing care has always been considered a high-energy consuming activity. Care usually comes at the cost of engaging in other activities, and such costs can include mobility restriction, growth retardation, immune depression, and physical condition deterioration (Marconato et al., 1993; Sargent, 1997; Robison et al., 2014). However, in syngnathid fishes, the immunity of the caregivers is not reduced, as expected, but is instead boosted (Whittington and Friesen, 2020). Most syngnathid fishes show internal fertilization. The female deposits mature and hydrated eggs directly into the male’s brood pouch where the eggs are fertilized. Then, the male alone provides parental care; specifically, he broods the embryos, and parental care behavior includes respiratory gas exchange, osmoregulation, metabolic waste removal, and nutrition provisioning (Stölting and Wilson, 2007). This care form is commonly referred to as male pregnancy. Additionally, the number of embryos in some syngnathid fishes often reduces during pregnancy (Ahnesjö, 1992, 1996; Ripley and Foran, 2006). Based on the observations of internal fertilization and brood reduction, some researchers have speculated that some eggs in the pouch serve as nurse eggs, which are eggs that are absorbed by the brooding male (Ripley and Foran, 2006). Such embryo absorption is a form of filial cannibalism, which is a behavior found in many other families of fishes with paternal care (Manica, 2002).
Nurse eggs are widely suggested to be a possible reason for brood reduction and a possible reason for immune increase during the care period, in syngnathid fishes. However, there are few studies that verify nurse eggs in syngnathid fishes. To our knowledge, nurse eggs have only been verified in one species of syngnathid fishes, i.e., the broad-nosed pipefish (Syngnathus typhle). Ahnesjö (1996) demonstrated that there is intra-brood competition in the broad-nosed pipefish, and within the same brood, eggs from low-quality females commonly serve as nurse eggs. Sagebakken et al. (2010) verified through isotope labeling that nutrients in the eggs of the broad-nosed pipefish can indeed be transferred to the parent, and thus, they concluded that the brooding father does absorb nutrients from his embryos. These findings provide important evidence for nurse eggs and parent–embryo conflict in syngnathid fishes (Crespi and Semeniuk, 2004).
Despite this previous work, it remains unknown if nurse eggs and embryo reabsorption occur in other syngnathid fishes, especially in monogamous syngnathid fishes. Pipefishes mostly have a polygamous mating system, such as observed in the broad-nosed pipefish (Vincent et al., 1992). In such species, male pipefishes mate with and receive eggs from several females in one breeding event, which means that the eggs in the same brood can vary in quality across female mates, and those with poor quality can serve as nurse eggs (Ahnesjö, 1996). In monogamous syngnathid fishes, the male only mates with and receives eggs from one female in a given breeding event, which means that the eggs in the same brood come from the same female and there is expected to be limited or no variation in egg quality. Thus, the question remains: do some eggs of monogamous syngnathid fishes serve as nurse eggs? In addition, considering that some eggs of some syngnathid fishes are spontaneously impaired during the fertilization process (Ripley and Foran, 2006), another question remains: do nurse eggs originate from these impaired and inviable eggs (i.e., are impaired eggs the predecessor to nurse eggs) or from the intact and viable eggs? If it is the former, it indicates that the brooding male simply uses nutrients from inviable eggs, while if it is the latter, it indicates that the brooding male actively consumes viable eggs. To enhance our understanding of embryo reabsorption in syngnathid fishes, we used the monogamous lined seahorse (Hippocampus erectus) (Lin et al., 2021) and asked whether nurse eggs exist in this species. We also explored whether nurse eggs potentially originate from viable versus inviable eggs.
2 Materials and methods
2.1 Ethical approval
Ethical approval for this study was obtained from the Committee on the Ethics of Animal Experiments of Chinese Academy of Fishery Sciences (Permit Number: 20200225). All procedures were conducted in compliance with the recommendations in the Guide for the Management and Use of the Experimental Animals of China Science and Technology Commission. The study was conducted in accordance with the local legislation and institutional requirements.
2.2 The study species
The seahorse used in the present study was, as mentioned above, the lined seahorse (Hippocampus erectus). It is mainly distributed along the western Atlantic coast and has been artificially bred in China since its introduction in 2008 (Lin et al., 2008). It takes 4–5 months from birth to sexual maturity (with a body height of approximately 11 cm) and then begins to mate and breed. The optimal breeding temperature is 24°C–26°C. Within this temperature range, the gestation period of male seahorses is 12–15 days. After giving birth, pair-bonded male and female seahorses can mate again in a short time. Moreover, the monogamy of the lined seahorse and male’s immune improvement during the care period (i.e., pregnancy) have been demonstrated in our previous work (Lin et al., 2016, 2021). Therefore, the lined seahorse is a suitable species for studying possible nurse eggs in the syngnathid fishes.
The lined seahorse used in the present study was artificially cultivated and provided by the Seahorse Research Center of the East China Sea Fisheries Research Institute, Qionghai City, Hainan Province, China. The center housed various sizes of artificially cultivated lined seahorse. Males with body height of 13–14 cm and well-developed pouch, and females with body height of 12–13 cm and well-developed gonad were picked out as the experimental seahorses. The experimental seahorses were stored in holding tanks, separated by gender, and fed live Mysis twice a day. The feces in the tanks were siphoned out 4 h after each feeding. The tanks were continuously flowed with fresh seawater for 12 h a day at a flowrate of 1.2–1.5 L/min. The parameters of seawater were temperature of 25°C–26°C, salinity of 30‰–31‰, dissolved oxygen content of 6.0–6.5 mg/L and pH of 8.0–8.2, respectively.
2.3 Experimental design
The present study consisted of three experiments. The first experiment aimed to test whether nutrients can be transferred from the embryos to the father during pregnancy. In this experiment, eggs of a female seahorse were labeled with a stable isotope; then, she was allowed to mate with a male seahorse. After receiving the isotope-labeled eggs and undergoing pregnancy and giving birth, the male seahorse was sampled for isotope detection in his body. If the isotope ratio was enhanced, it indicated that the isotope-labeled nutrients had indeed transferred from the eggs to the male seahorse, implying that nurse eggs occur in the lined seahorse. The second experiment aimed to test whether there are impaired embryos in the pouch of the lined seahorse. In this experiment, a recently mated male seahorse was sampled to observe the viability of embryos in his pouch. The third experiment aimed to test whether the brooding male actively consumes his viable embryos. In this experiment, we assumed that harsh environments (e.g., limited food) would exacerbate the absorption of nutrients or energy from embryos by the brooding male. Here, we employed the unfavorable condition of limited food. The male seahorse who successfully received the isotope-labeled eggs was limited in feeding. After giving birth, his brood size and the isotope ratio in his body were quantified. If the brood size was reduced and the isotope ratio was enhanced, this indicated that a considerable portion of viable embryos had been consumed by the brooding male, implying that the brooding male actively kills the embryos to absorb nutrients in response to limited food.
2.4 Experimental protocols
2.4.1 Experiment 1: testing whether nutrients can be transferred from the embryos to the parent
The stable isotope used in the present study was 15N-labeled leucine (L-Leucine-15N, 98 atom%). It was purchased from Shanghai Research Institute of Chemical Industry Co., Ltd., Shanghai, China. In the pre-experiment, we bathed the live Mysis in a 0.2% L-Leucine-15N seawater solution for 1 h and then filtered the Mysis with a 60-mesh screen to feed female seahorses. Each seahorse was fed approximately 50 Mysis at a time. We fed the female seahorses twice a day for 14 days and found a great enrichment of 15N in their ovaries. Therefore, in this experiment, we also used this method to isotopically label the eggs of female seahorses.
A total of 48 female seahorses from the holding tanks were divided into two groups. They were the isotope group and the control group, with 24 seahorses in each group. The female seahorses in each group were placed in a rearing tank. The isotope group was fed isotopically labeled live Mysis, which had been bathed with 0.2% L-Leucine-15N solution for 1 h, while the control group was fed live Mysis without isotope labeling. Each group was fed twice a day, and each seahorse was fed approximately 50 Mysis at a time. Fourteen days later, eight females of each group were anesthetized with a MS-222 (50 mg/L) seawater solution and dissected to collect their ovaries for the analysis of 15N ratio. The remaining 16 females in each group were assigned to 16 breeding tanks (0.7 m in diameter and 0.8 m in depth), where each breeding tank had one female. Afterwards, each breeding tank was assigned a male from the holding tank to pair with the female. Starting from the meeting of the male and the female seahorse, they were both fed live Mysis without isotope labeling twice a day. The mating status of the male and the female seahorse in each breeding tank was checked daily. Checking operation included gently picking up the male and the female seahorse with a fishing net and observing the male’s pouch and the female’s abdomen, respectively. This operation was completed within 20 s. If an obvious expansion of the male’s pouch (due to the reception of eggs) and an obvious contraction of the female’s abdomen (due to the expulsion of eggs) were observed at the same time, we concluded that mating occurred. Once mating occurred, the breeding tank ID and mating date were recorded. The successfully mated male seahorse began giving birth after 12–15 days of pregnancy. After giving birth, the male was anesthetized for sampling. Considering that there might be some fragments or mucus from the embryos still remaining in the male’s pouch, thereby interfering with the experimental results, the male was cut along the last trunk ring, and the upper body (including the head and trunk but not including the pouch) was collected for the analysis of 15N ratio.
Samples of female ovary and male upper body were dried to constant weight at 60°C. Dried samples were ground to a fine powder and sieved with an 80-mesh sieve. Totally, 2–4 mg of each sample powder was weighed and wrapped into a tin capsule. Samples were analyzed for stable nitrogen isotope and percent nitrogen by the Shanghai Research Institute of Chemical Industry (Shanghai, China). An elemental analyzer (EA IsoLink CN/OH system, Thermo Scientific™, USA) liberated nitrogen from solid samples by flash combustion and subsequent oxidation (at 1,120°C) and reduction (at 850°C) reactions. Nitrogen was vented to an isotope ratio mass spectrometer (IRMS) (Delta V advantage, Thermo Scientific™, USA) via a helium carrier gas flow with a rate of 180 ml/min for isotope ratio analysis. Isotope ratio was expressed in a unit of atom percent (atom%). The following atom percent calculation determines the absolute number of atoms of a given isotope in 100 atoms of total element:
where AR is the absolute ratio, and δ is the δ15N value. The absolute ratio is a constant, which is 0.0036764 for 15N (Ripley and Foran, 2009).
2.4.2 Experiment 2: testing whether there are impaired embryos in the pouch
One male and one female seahorse from the holding tanks were paired in a breeding tank, with a total of 24 pairs. The mating status of each pair was checked every 2 h from 6 a.m. to 6 p.m. (seahorses do not mate at night). Once mating success was observed, the breeding tank ID and mating time were recorded. The successfully mated males were divided into four parts. Part I was to collect the eggs/embryos from the pouch immediately when mating success was observed; Part II, III, and IV were to collect the eggs/embryos from the pouch 4, 12, and 24 h after mating success was observed, respectively. These newly mated or recently mated males were first anesthetized with a MS-222 solution, and then, we gently inserted a pair of anatomical scissors into the opening of their pouches, making an incision along the last trunk ring. After that, the incision was facing downwards, and the eggs/embryos within the pouch spontaneously flowed out. A portion of the eggs/embryos was collected for microscopic (BX41, Olympus, Japan) observation.
2.4.3 Experiment 3: testing whether the brooding male actively consumes his viable embryos
A total of 40 female seahorses from the holding tanks were placed in a rearing tank and fed isotopically labeled live Mysis twice a day for 14 days. Each seahorse was fed approximately 50 Mysis at a time. Afterwards, these females were each paired with a male in a breeding tank. Starting from the meeting of the male and the female seahorse, they were both fed live Mysis without isotope labeling twice a day. The mating status of each pair was checked daily. The successfully mated male seahorses were picked out to divide evenly into two groups: one group continued with the original feeding level (i.e., feeding live Mysis without isotope labeling twice a day, with 50 Mysis for each male per feeding), and the other group experienced a reduced the feeding level (i.e., feeding live Mysis without isotope labeling only once a day, with only 25 Mysis for each male per feeding). For the convenience of counting the number of newborn offspring, each male in each group was placed in an independent breeding tank. After giving birth, newborn size (i.e., body height of 25 randomly selected individuals in each brood) and brood size (i.e., the total number of newborns born in a brood) were measured for each male, and the 15N ratios in their upper bodies were also quantified.
2.5 Statistical analysis
All data were expressed as the mean ± SD and were analyzed using SPSS statistical software (version 17.0, Chicago, Illinois). Prior to analysis, the data of isotope ratio (atom%) were first divided by 100 and then were −Log10 transformed. The normality of all data was evaluated using the Shapiro–Wilk’s W-test, and the homogeneity of variances was assessed using Levene’s test. The significance of the difference between the isotope group and the control group in Experiment 1, and between the food-sufficient group and food-limited group in Experiment 3, was analyzed using one-way ANOVA at a significance level of p<0.05.
3 Results
3.1 Experiment 1: testing whether nutrients can be transferred from the embryos to the parent
As expected, after 14 days of feeding the female seahorses (N=8) with 15N labeled Mysis, their ovaries were enriched with a large amount of 15N. The 15N ratio significantly increased from 0.371 ± 0.001 atom% in the control group to 0.423 ± 0.002 atom% (F1, 15 = 4961.21, p<0.001) (Figure 1).
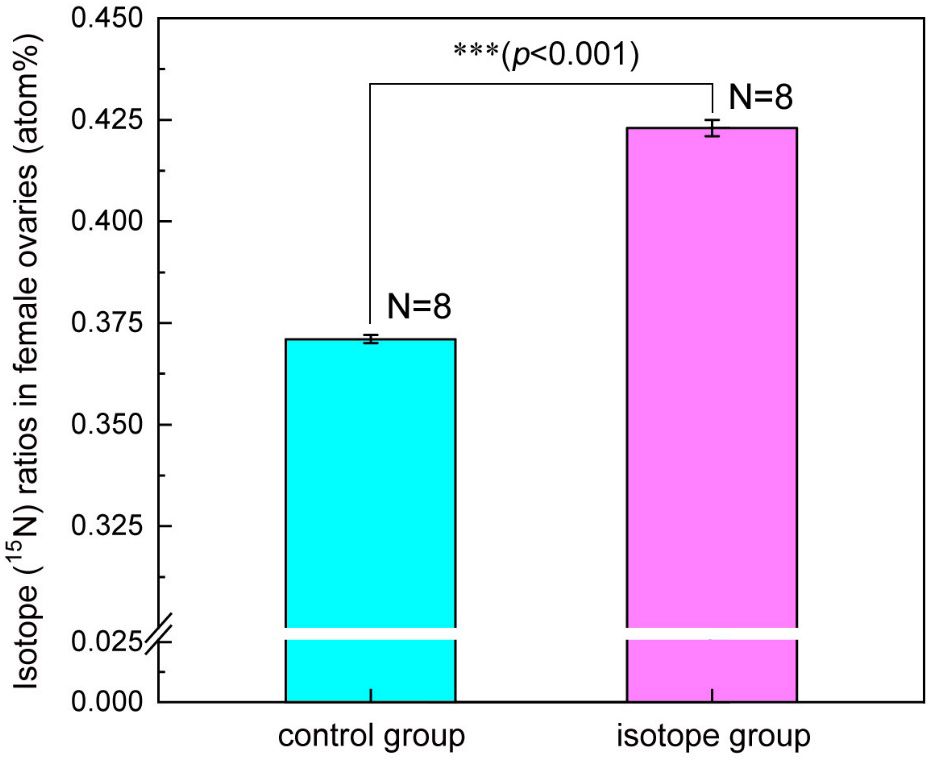
Figure 1 Variation of isotope (15N) ratio in the ovaries of the female lined seahorses (H. erectus) after feeding isotope-labeled Mysis for 14 days. Three asterisks (***) represent p-value<0.001.
A total of 16 males were paired one-on-one with the females that had been fed with 15N labeled Mysis. A total of 12 of them (N=12) successfully mated and received the eggs rich in 15N from the females. After experiencing pregnancy and giving birth, the 15N ratio of these males increased to 0.375 ± 0.002 atom%, which was significantly higher than that of control males (N=11, 0.371 ± 0.001 atom%) who had mated the females that had not fed with 15N labeled Mysis (F1, 22 = 51.47, p<0.001) (Figure 2).
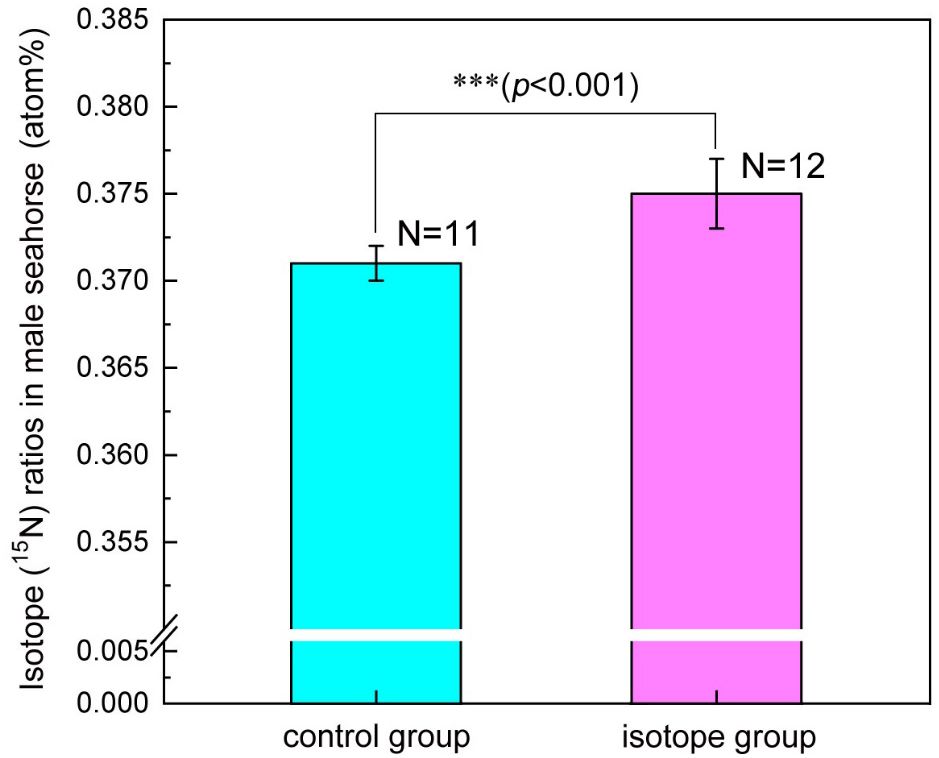
Figure 2 Variation of isotope (15N) ratio in the upper body of the male lined seahorses (H. erectus) after mating with the females whose ovaries were rich in isotope and giving birth. Three asterisks (***) represent p-value<0.001.
3.2 Experiment 2: testing whether there are impaired embryos in the pouch
Part I males (i.e., males examined within 2 h after mating) had pouch openings that were not yet fully closed and could be easily opened. The eggs in their pouches were intact (Figures 3A, B), and there was no seminal fluid in their pouches yet. For Part II males (i.e., males examined within 4–6 h after mating), their pouch openings were already fully closed and difficult to open. There was already seminal fluid in the pouches, and the embryos in their pouches were still intact (Figure 3C). For Part III males (i.e., males examined within 12–14 h after mating), some free yolk droplets (Figure 3D) and some impaired embryos (Figure 3E) began to appear in their pouches. The impaired embryos were characterized by a dull and tarnished egg cytoplasm, an edge-blurred yolk membrane, and a shrunken and color-deepened (from orange to reddish brown) yolk. While for Part IV males (i.e., males examined within 24–26 h after mating), the number of free yolk droplets was increased compared to Part III, and some impaired embryos even had the entire nucleus and cytoplasm flowing out from the cracks in the egg membrane (Figure 3F).
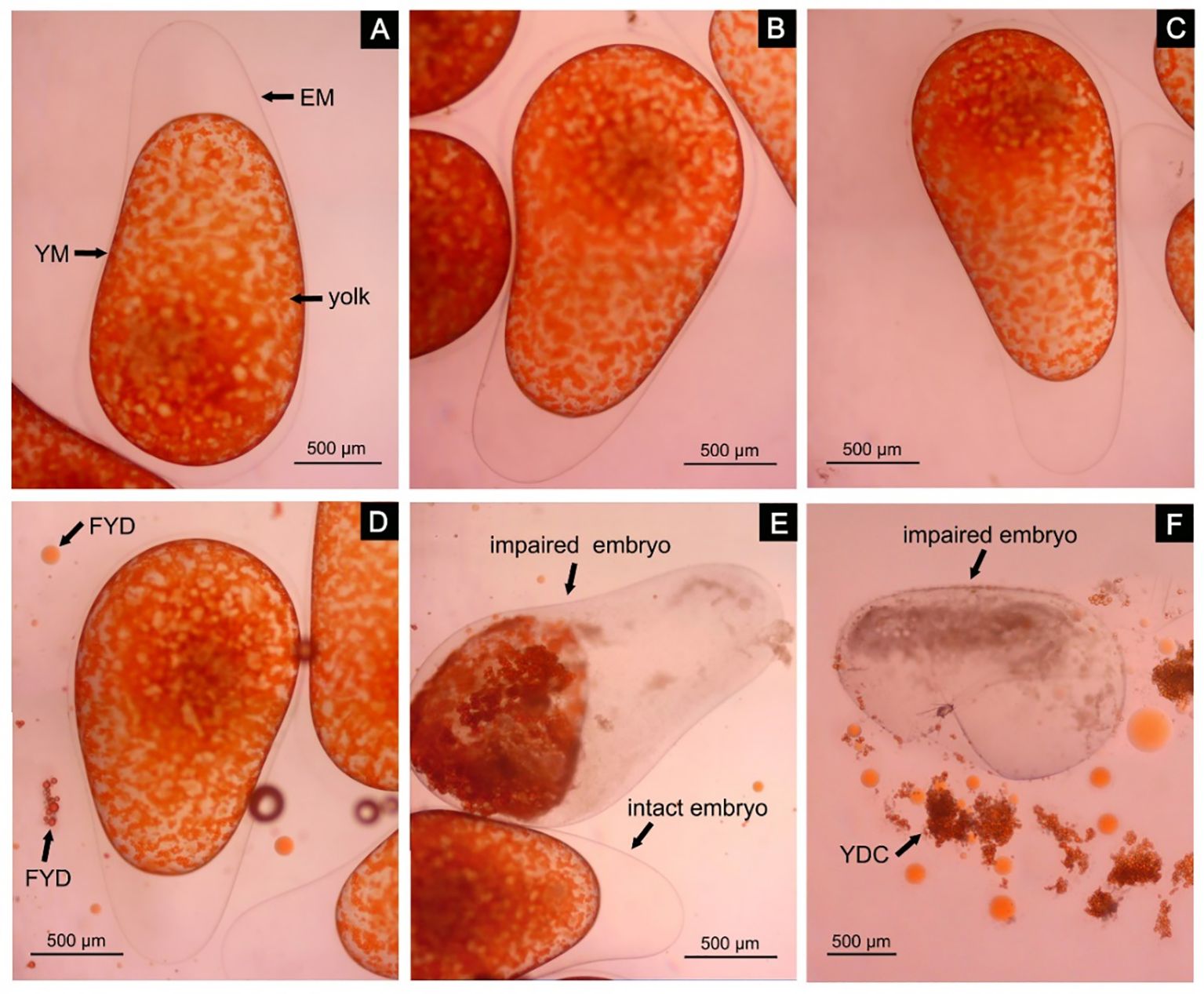
Figure 3 The morphology of eggs/embryos in the brood pouch of newly or recently mated male lined seahorses (H. erectus). (A, B) Eggs within 2 h after mating; (C) embryos within 4–6 h after mating; (D, E) embryos within 12–14 h after mating; (F) embryos within 24–26 h after mating. EM, egg membrane; YM, yolk membrane; FYD, free yolk droplet; YDC, yolk droplet cluster.
3.3 Experiment 3: testing whether the brooding male actively consumes his viable embryos
A total of 32 males successfully mated and received the 15N labeled eggs from females. They were divided into two groups (i.e., food-sufficient group and food-limited group), with 16 males (N=16) in each group.
The body height of newborns in the food-limited group was 1.152 ± 0.070 cm, which was significantly taller than that in food-sufficient group (1.008 ± 0.069 cm) (F1, 31 = 34.90, p<0.001) (Figure 4). The brood size of the food-limited group was 176.6 ± 38.1 individuals per brood, which was significantly lower than the brood size (277.4 ± 51.7 individuals per brood) of the food-sufficient group (F1, 31 = 39.40, p<0.001) (Figure 5). The 15N ratio of the food-limited group was 0.383 ± 0.004 atom%, which was significantly higher than the 15N ratio level (0.375 ± 0.001 atom%) of the food-sufficient group (F1, 31 = 59.96, p<0.001) (Figure 6). In addition, the pregnancy duration of males in the food-limited group (17.1 ± 1.1 days) was significantly longer than that of males in the food-sufficient group (14.4 ± 1.2 days) (F1, 31 = 44.85, p<0.001).
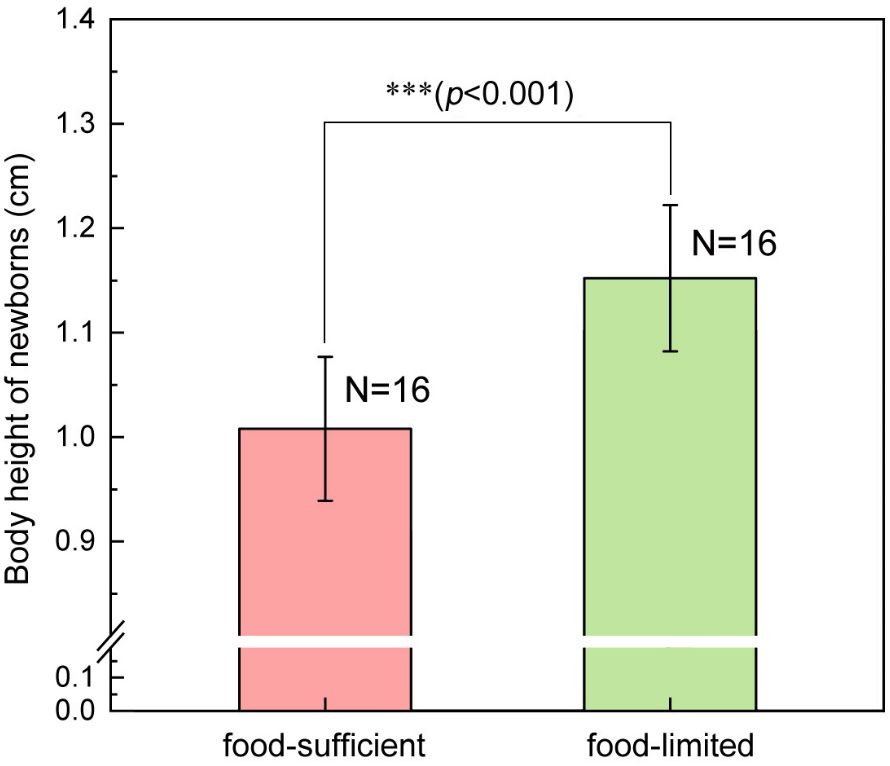
Figure 4 The difference in body height of newborns born to the male lined seahorses (H. erectus) across conditions of sufficient and limited food. Three asterisks (***) represent p-value<0.001.
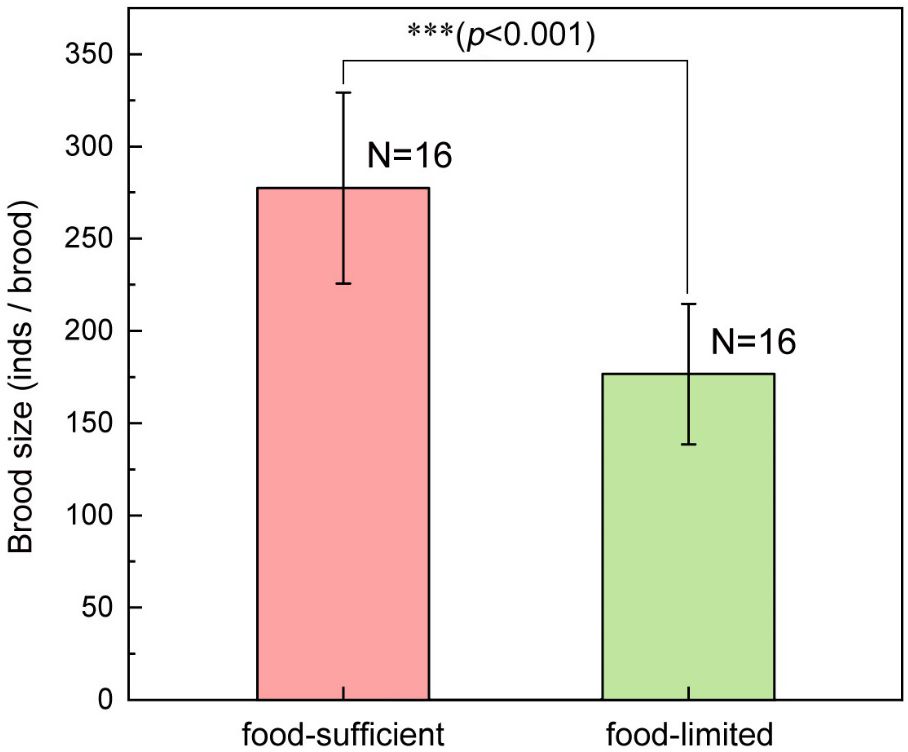
Figure 5 The difference in brood size of the male lined seahorses (H. erectus) between conditions of sufficient and limited food. Three asterisks (***) represent p-value<0.001.
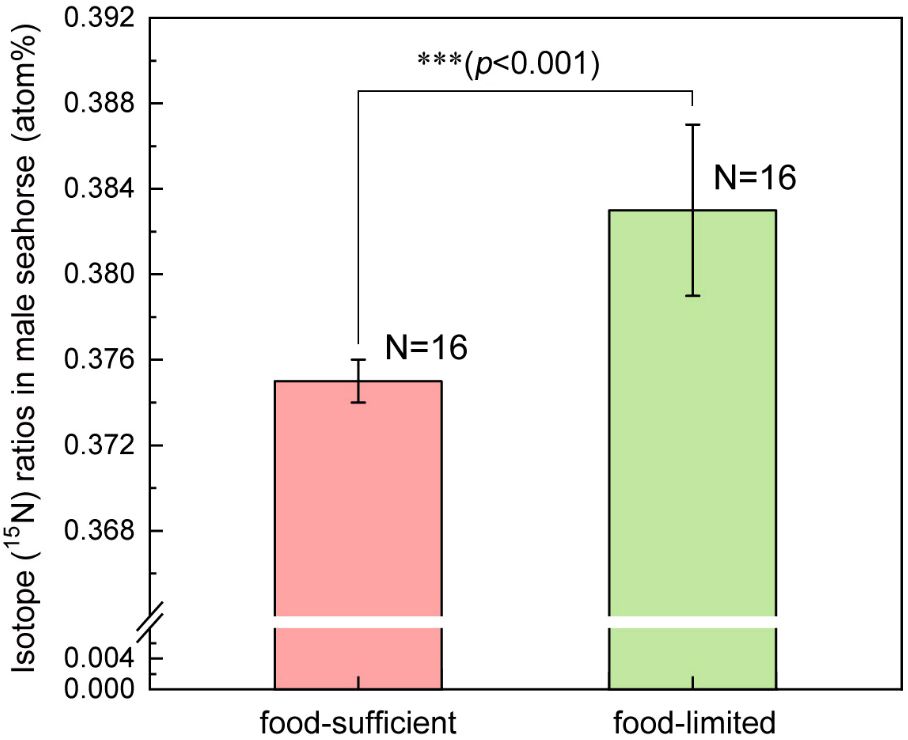
Figure 6 The difference in isotope (15N) ratio in the upper body of the male lined seahorses (H. erectus) between conditions of sufficient and limited food. Three asterisks (***) represent p-value<0.001.
4 Discussion
In the present study, we have demonstrated that brooding male seahorses take up nutrients that originate from embryos in their pouch, and their absorption of embryonic nutrients is actively adjusted according to environmental conditions.
In Experiment 1, the results showed that the 15N ratio in male seahorses significantly increased after brooding 15N-labeled embryos. The increased isotope in male seahorses could only come from the embryos developing in the pouch, as the 15N-labeled leucine was originally incorporated into the eggs of the females that were mated to the males. That is, the increased isotope was entirely attributed to the transfer of isotope-labeled leucine or its metabolites from embryos to the males. The fact that nutrients were transferred from embryos to the brooding father implies that the father is able to take up nutrients from his embryos. This nutrient uptake resembles filial cannibalism found in many other families of fishes (Manica, 2002).
In Experiment 1, the results demonstrated that nurse eggs occur in the lined seahorse, which has been previously documented in the broad-nosed pipefish (Sagebakken et al., 2010). The presence of nurse eggs both in the lined seahorse and the broad-nosed pipefish may indicate that some embryos of the syngnathid fishes are destined to serve as a nutrient source to nourish the parent that provides parental care. Although these two fishes both have nurse eggs, their selectivity for nurse eggs may be different. In the lined seahorse, the eggs in the male’s pouch all come from the same female. In contrast, in the broad-nosed pipefish, the eggs in the male’s pouch come from several different females, which may mean that the male has a specificity in their selection of nurse eggs with respect to the female mate. Ahnesjö (1996) previously demonstrated that the eggs from low-quality females were commonly selected as nurse eggs. This difference in the selection of nurse eggs between these two fishes is clearly attributed to their different mating systems.
The mechanism of nutrient transfer from embryos to the brooding parent may rely on vascular transport. The brood pouch of seahorse is a placenta-like structure that primarily provides protection, nutrients, and oxygen for the developing embryos (Kvarnemo et al., 2011; Whittington and Friesen, 2020). During pregnancy, especially in the early stage of pregnancy, the inner epithelium of the pouch will become highly vascularized, and the inner layer of the pouch will be rich in blood vessels (Carcupino et al., 2002; Laksanawimol et al., 2006; Ripley et al., 2010; Lin et al., 2017). Therefore, researchers believe that the paternally derived nutrients and oxygen are likely transported to the embryos through these vascularized connective tissues and blood vessels (Ripley and Foran, 2009; Whittington and Friesen, 2020). Likewise, the transfer of nutrients from embryos to the father may rely on the opposite direction of this transportation way. Namely, the embryonically derived nutrients enter the paternal blood stream and are transported to the whole body of the father.
In Experiment 2, impaired embryos and free yolk droplets were observed in the pouch of early brooding males. This result provides evidence of the existence of nurse eggs, and these impaired embryos are likely to be broken-down nurse eggs (Ripley and Foran, 2006). As for the cause of embryo breakage, it may be related to the release of some substances (e.g., prolactin and growth hormone) by the male into the pouch after mating, which causes enzymatic breakdown of a small portion of embryos (Carcupino et al., 2002; Patron et al., 2008; Wilson et al., 2023). In addition, in Experiment 2, we also found that the deposition of sperm and eggs was not synchronous, but rather, the sperm lagged behind the eggs. This finding breaks a widespread but poorly founded belief that sperm and eggs are deposited synchronously or that sperm are deposited immediately after the eggs are deposited or inserted (Van Look et al., 2007; Whittington and Friesen, 2020). The fact that sperm deposition lagged behind that of the eggs in the seahorse indicates that the pattern of male fishes being the last to release gametes not only occurs in fishes showing external fertilization (Gross and Shine, 1981) but also in fishes showing internal fertilization. In seahorses, it is possible that the lagged deposition of sperm occurs because the males need some time to adjust the distribution of the eggs in their pouch after the successful reception of the eggs. We have found multiple times (personal observation) that newly mated males frequently shake their bodies, and the purpose of this shaking may be to distribute the eggs as evenly as possible in the pouch in order to achieve the best possible fertilization.
In Experiment 3, the results showed that compared with the males fed sufficient food, the males fed limited food had a significantly reduced brood size and a significantly increased 15N ratio in their bodies after giving birth. The increased isotope indicates that a considerable portion of embryos had been consumed and absorbed by the food-limited males, and the reduced brood size indicates that these consumed embryos are actually viable embryos that could develop into newborns if their father did not kill them. To put it briefly, the results of Experiment 3 demonstrated that the brooding males could actively consume their viable embryos in response to poor environmental conditions (i.e., limited food availability). Previous work in other fishes has found that fathers will increase the consumption of embryos to obtain more nutrients or energy in response to harsh conditions (Hoelzer, 1992; Manica, 2004).
Caring males adjust their consumption of offspring based on food availability, which may be an adaptive strategy to increase own fitness (Klug and Bonsall, 2007). In some cases, caring males consume some or all of their offspring, thus seemingly reducing their own fitness. Yet, since caring males may lose weight and fat reserves while caring, obtaining energy and nutrients from consuming their offspring actually may improve their parental condition, thus allowing them to better care for the remaining offspring and/or increasing future reproductive success. Therefore, brood reduction may increase parental fitness if males can use some of their own offspring as a nutritional resource (Lindström, 2000; Klug and Bonsall, 2007; Sagebakken et al., 2010).
In addition, the results of Experiment 3 showed that the newborn size of the food-limited males was significantly larger than that of food-sufficient males. There are two possible explanations. The first one is that a considerable portion of embryos are consumed, resulting in a larger pouch space for the remaining embryos to develop. Based on the pouch size, brood size, and newborn size of different species of seahorses, there is evidence suggesting that the size of newborns is related to the space of the pouch. For example, in the pot-bellied seahorse (H. abdominalis) (Martinez-Cardenas and Purser, 2011), males have an abnormally large pouch, but they give birth to very few newborns in each brood (50–200 inds), and the size of newborns is large, approximately 2.0 cm in body height. In the three-spot seahorse (H. trimaculatus) (Lipton and Thangaraj, 2014) and hedgehog seahorse (H. spinosissimus) (Kang et al., 2005), males have a moderately sized pouch, but they give birth to a large number of newborns in each brood (300–500 inds), and the size of newborns is small, approximately 0.7 cm in body height. These examples indicate that the size of newborns is related to pouch space. The second potential explanation is that the pregnancy duration of males with limited food is longer than that of males with sufficient food, which means that the newborns of the former males develop in the pouch for a longer time than those of the latter males, resulting in a larger size.
5 Conclusions
In the present study, we demonstrated the presence of impaired embryos in the pouch of the brooding male, and we demonstrated that nutrients could be transferred from embryos to the brooding male. These results imply that the brooding male can indeed take up the nutrients from embryos, which answers the first question raised in the introduction that is nurse eggs also exist in a syngnathid fish showing monogamy. In addition, we demonstrated that the brooding male exacerbated his consumption and absorption of embryos under the limited food conditions. This result indicates that the brooding male is able to actively adjust the absorption of embryonic nutrients based on environmental conditions, which answers the second question raised in the introduction regarding whether the brooding male actively consumes his viable embryos to absorb the nutrients. Proactively regulating the absorption of embryonic nutrients may be a result of natural selection that is beneficial for increasing one’s own fitness.
Data availability statement
The raw data supporting the conclusions of this article will be made available by the authors, without undue reservation.
Author contributions
TL: Data curation, Formal analysis, Funding acquisition, Methodology, Writing – original draft, Investigation, Project administration, Writing – review & editing. XL: Formal analysis, Investigation, Methodology, Writing – original draft. SL: Methodology, Validation, Visualization, Writing – original draft. DZ: Conceptualization, Data curation, Funding acquisition, Resources, Writing – review & editing. FS: Project administration, Software, Writing – original draft.
Funding
The author(s) declare financial support was received for the research, authorship, and/or publication of this article. The present study was supported by the National Natural Science Foundation of China (Grant No. 32172950), the Natural Science Foundation of Shanghai (Grant No. 21ZR1479800), the Shanghai Sailing Program (21YF1459800), and the Central Public-interest Scientific Institution Basal Research Fund of CAFS (Grant No. 2023TD56).
Acknowledgments
We are very grateful to Bingcong Ye (Seahorse Research Center) for the assistance in the collection and microscopic photography of seahorse embryos.
Conflict of interest
The authors declare that the research was conducted in the absence of any commercial or financial relationships that could be construed as a potential conflict of interest.
Publisher’s note
All claims expressed in this article are solely those of the authors and do not necessarily represent those of their affiliated organizations, or those of the publisher, the editors and the reviewers. Any product that may be evaluated in this article, or claim that may be made by its manufacturer, is not guaranteed or endorsed by the publisher.
References
Ahnesjö I. (1992). Fewer newborn result in superior juveniles in the paternally brooding pipefish Syngnathus typhle L. J. Fish Biol. 41, 53–63. doi: 10.1111/j.1095-8649.1992.tb03868.x
Ahnesjö I. (1996). Apparent resource competition among embryos in the brood pouch of a male pipefish. Behav. Ecol. Sociobiol. 38, 167–172. doi: 10.1007/s002650050229
Carcupino M., Baldacci A., Mazzini M., Franzoi P. (2002). Functional significance of the male brood pouch in the reproductive strategies of pipefishes and seahorses: a morphological and ultrastructural comparative study on three anatomically different pouches. J. Fish Biol. 61, 1465–1480. doi: 10.1111/j.1095-8649.2002.tb02490.x
Crespi B., Semeniuk C. (2004). Parent-offspring conflict in the evolution of vertebrate reproductive mode. Am. Nat. 163, 635–653. doi: 10.1086/382734
Dawkins R., Carlisle T. R. (1976). Parental investment, mate desertion and a fallacy. Nature 262, 131–133. doi: 10.1038/262131a0
Forsgren E. (1997). Female sand gobies prefer good fathers over dominant males. P. R. Soc B-Biol. Sci. 264, 1283–1286. doi: 10.1098/rspb.1997.0177
Goldberg R. L., Downing P. A., Griffin A. S., Green J. P. (2020). The costs and benefits of paternal care in fish: A meta analysis. P. R. Soc B-Biol. Sci. 287, 20201759. doi: 10.1098/rspb.2020.1759
Gross M. R., Sargent R. C. (1985). The evolution of male and female parental care in fishes. Am. Zool. 25, 807–822. doi: 10.1093/icb/25.3.807
Gross M. R., Shine R. (1981). Parental care and mode of fertilization in ectothermic vertebrates. Evolution 35, 775–793. doi: 10.2307/2408247
Hoelzer G. A. (1992). The ecology and evolution of partial-clutch cannibalism by parental Cortez damselfish. Oikos 65, 113–120. doi: 10.2307/3544893
Kang S. Y., Koh J. R., Jeong S. B., Kim S. (2005). Morphological development and reproductive behavior of hedgehog seahorse Hippocampus spinosissimus (Teleostei: Syngnathidae). Kor. J. Fish. Aquat. Sci. 38, 298–303. doi: 10.5657/kfas.2005.38.5.298
Klug H., Bonsall M. B. (2007). When to care for, abandon, or eat your offspring: the evolution of parental care and filial cannibalism. Am. Nat. 170, 886–901. doi: 10.1086/522936
Kvarnemo C. (2006). Evolution and maintenance of male care: is increased paternity a neglected benefit of care? Behav. Ecol. 17, 144–148. doi: 10.1093/beheco/ari097
Kvarnemo C., Mobley K. B., Partridge C., Jones A. G., Ahnesjö I. (2011). Evidence of paternal nutrient provisioning to embryos in broad-nosed pipefish Syngnathus typhle. J. Fish Biol. 78, 1725–1737. doi: 10.1111/j.1095-8649.2011.02989.x
Laksanawimol P., Damrongphol P., Kruatrachue M. (2006). Alteration of the brood pouch morphology during gestation of male seahorses, Hippocampus kuda. Mar. Freshw. Res. 57, 497–502. doi: 10.1071/MF05112
Lin Q., Lin J., Zhang D. (2008). Breeding and juvenile culture of the lined seahorse, Hippocampus erectus Perr. Aquaculture 277, 287–292. doi: 10.1016/j.aquaculture.2008.02.030
Lin T., Liu X., Xiao D., Zhang D. (2017). Plasma levels of immune factors and sex steroids in the male seahorse Hippocampus erectus during a breeding cycle. Fish Physiol. Biochem. 43, 889–899. doi: 10.1007/s10695-017-0343-6
Lin T., Liu X., Zhang D. (2021). Does the female seahorse still prefer her mating partner after a period of separation? J. Fish Biol. 99, 1613–1621. doi: 10.1111/jfb.14867
Lin T., Liu X., Zhang D., Li S. (2023). Extensive parental care experience of male seahorses increases their future mating attractiveness. Curr. Zool. 69, 106–108. doi: 10.1093/cz/zoac017
Lin T., Zhang D., Liu X., Xiao D. (2016). Parental care improves immunity in the seahorse (Hippocampus erectus). Fish Shellfish Immun. 58, 554–562. doi: 10.1016/j.fsi.2016.09.065
Lindström K. (2000). The evolution of filial cannibalism and female mate choice strategies as resolutions to sexual conflict in fishes. Evolution 54, 617–627. doi: 10.1111/j.0014–3820.2000.tb00063.x
Lindström K., St. Mary C. M., Pampoulie C. (2006). Sexual selection for male parental care in the sand goby Pomatoschistus minutus. Behav. Ecol. Sociobiol. 60, 46–51. doi: 10.1007/s00265–005-0138–0
Lipton A., Thangaraj M. (2014). Courtship behaviour, brood Characteristics and embryo development in three spotted seahorse, Hippocampus trimaculatus (Leacn 1814). Int. Res. J. Biol. Sci. 3, 6–10.
Manica A. (2002). Filial cannibalism in teleost fish. Biol. Rev. 77, 261–277. doi: 10.1017/S1464793101005905
Manica A. (2004). Parental fish change their cannibalistic behaviour in response to the cost-to-benefit ratio of parental care. Anim. Behav. 67, 1015–1021. doi: 10.1016/j.anbehav.2003.09.011
Marconato A., Bisazza A., Fabris M. (1993). The cost of parental care and egg cannibalism in the river bullhead, Cottus gobio L. (Pisces, Cottidae). Behav. Ecol. Sociobiol. 32, 229–237. doi: 10.1007/BF00166512
Martinez-Cardenas L., Purser G. J. (2011). Effect of temperature on growth and survival in cultured early juvenile pot-bellied seahorses, Hippocampus abdominalis. J. World Aquacult. Soc 42, 854–862. doi: 10.1111/j.1749–7345.2011.00526.x
Östlund S., Ahnesjö I. (1998). Female fifteen-spined sticklebacks prefer better fathers. Anim. Behav. 56, 1177–1183. doi: 10.1006/anbe.1998.0878
Patron J. J., Herrera A. A., Oconer E. P. (2008). Prolactin and growth hormone levels in the pouch fluid of gravid male seahorse, Hipocampus barbouri Jordan and Richardson 1908. Asia Life Sci. 17, 261–269.
Reynolds J. D., Goodwin N. B., Freckleton R. P. (2002). Evolutionary transitions in parental care and live bearing in vertebrates. Philos. T. R. Soc B-Biol. Sci. 357, 269–281. doi: 10.1098/rstb.2001.0930
Ripley J. L., Foran C. M. (2006). Differential parental nutrient allocation in two congeneric pipefish species (Syngnathidae: Syngnathus spp.). J. Exp. Biol. 209, 1112–1121. doi: 10.1242/jeb.02119
Ripley J. L., Foran C. M. (2009). Direct evidence for embryonic uptake of paternally-derived nutrients in two pipefishes (Syngnathidae: Syngnathus spp.). J. Comp. Physiol. B 179, 325–333. doi: 10.1007/s00360-008-0316-2
Ripley J. L., Williams P. S., Foran C. M. (2010). Morphological and quantitative changes in paternal brood-pouch vasculature during embryonic development in two Syngnathus pipefishes. J. Fish Biol. 77, 67–79. doi: 10.1111/j.1095-8649.2010.02659.x
Robison B., Seibel B., Drazen J. (2014). Deep-sea octopus (Graneledone boreopacifica) conducts the longest-known egg-brooding period of any animal. PloS One 9, e103437. doi: 10.1371/journal.pone.0103437
Roth O., Scharsack J. P., Keller I., Reusch T. B. H. (2011). Bateman’s principle and immunity in a sex-role reversed pipefish. J. Evol. Biol. 24, 1410–1420. doi: 10.1111/j.1420-9101.2011.02273.x
Sagebakken G., Ahnesjö I., Mobley K. B., Goncalves I. B., Kvarnemo C. (2010). Brooding fathers, not siblings, take up nutrients from embryos. P. R. Soc B-Biol. Sci. 277, 971–977. doi: 10.1098/rspb.2009.1767
Sargent R. C. (1997). “Parental care,” in Behavioural ecology of teleost fishes. Ed. Godin J. J. (Oxford University Press, New York, NY), 292–311.
Stölting K. N., Wilson A. B. (2007). Male pregnancy in seahorses and pipefish: Beyond the mammalian model. BioEssays 29, 884–896. doi: 10.1002/bies.20626
Sutton F. B., Wilson A. B. (2019). Where are all the moms? External fertilization predicts the rise of male parental care in bony fishes. Evolution 73, 2451–2460. doi: 10.1111/evo.13846
Trivers R. L. (1972). “Parental investment and sexual selection,” in Sexual selection and the descent of ma. Ed. Campbell B. (Aldine Publishing Company, Chicago), 136–179. doi: 10.4324/9781315129266–7
Van Look K. J. W., Dzyuba B., Cliffe A., Koldewey H. J., Holt W. V. (2007). Dimorphic sperm and the unlikely route to fertilisation in the yellow seahorse. J. Exp. Biol. 210, 432–437. doi: 10.1242/jeb.02673
Vincent A. C. J., Ahnesjö I., Berglund A., Rosenqvist G. (1992). Pipefishes and seahorses: Are they all sex role reversed? Trends Ecol. Evol. 7, 237–241. doi: 10.1016/0169–5347(92)90052-D
Whittington C. M., Friesen C. R. (2020). The evolution and physiology of male pregnancy in syngnathid fishes. Biol. Rev. 95, 1252–1272. doi: 10.1111/brv.12607
Whittington C. M., Griffith O. W., Qi W., Thompson M. B., Wilson A. B. (2015). Seahorse brood pouch transcriptome reveals common genes associated with vertebrate pregnancy. Mol. Biol. Evol. 32, 3114–3131. doi: 10.1093/molbev/msv177
Keywords: filial cannibalism, infanticide, monogamous syngnathid fishes, nurse eggs, seahorse Hippocampus erectus
Citation: Lin T, Liu X, Li S, Zhang D and Shen F (2024) Brooding fathers actively consume viable embryos to take up nutrients in a monogamous syngnathid fish. Front. Mar. Sci. 11:1399526. doi: 10.3389/fmars.2024.1399526
Received: 12 March 2024; Accepted: 31 May 2024;
Published: 21 June 2024.
Edited by:
Hope Klug, University of Tennessee at Chattanooga, United StatesReviewed by:
Geng Qin, Chinese Academy of Sciences (CAS), ChinaEwa Sokołowska, Polish Academy of Sciences, Poland
Copyright © 2024 Lin, Liu, Li, Zhang and Shen. This is an open-access article distributed under the terms of the Creative Commons Attribution License (CC BY). The use, distribution or reproduction in other forums is permitted, provided the original author(s) and the copyright owner(s) are credited and that the original publication in this journal is cited, in accordance with accepted academic practice. No use, distribution or reproduction is permitted which does not comply with these terms.
*Correspondence: Dong Zhang, zhangdong@ecsf.ac.cn