- 1Jiangsu Key Laboratory of Marine Bioresources and Environment, Jiangsu Ocean University, Lianyungang, China
- 2Co-Innovation Center of Jiangsu MarineBio-industry Technology, Jiangsu Institute of Marine Resources Development, Jiangsu Ocean University, Lianyungang, China
- 3Affair Center of Animal Husbandry and Aquaculture in XiangXi Autonomous Prefecture, Jishou, China
- 4State Ministry of Education Key Laboratory of Protein Chemistry and Developmental Biology, College of Life Sciences, Hunan Normal University, Changsha, China
- 5Jiangsu Key Laboratory of Marine Biotechnology, Jiangsu Ocean University, Lianyungang, China
The clam Cyclina sinensis is an economically important marine species in China. However, overfishing, habitat destruction, and inbreeding have led to the destruction of genetic resources. In this study, five natural populations [Dandong (DD), Dongying (DY), Tianjin (TJ), Wenzhou (WZ), and Yancheng (YC)] from different geographical locations were relocated and cultured homogeneously to study their potential for translocation and environmental adaptation by observing growth performance and nutrient content. There were significant differences in the growth rates of shell length (SL), shell height (SH), and shell width (SW) among the five populations (P<0.05). The DD, TJ, and YC populations exhibited the highest SL and SH growth rates, whereas these were lowest in the WZ population. The survival rate was highest in the YC population and lowest in the WZ population, which was significantly lower than the other four populations (P<0.05). The DD population exhibited superior plumpness and glycogen content, and the overall glycogen content in male C. sinensis was higher compared with that in females (P<0.05). All populations displayed a high total amino acid content and the essential amino acid/Total Amino Acid (EAA/TAA) and Non-essential amino acid/Total Amino Acid (NEAA/TAA) ratios in the soft tissue of all five populations aligned with the FAO/WHO ideal protein evaluation standards. Despite the same aquaculture environment, however, the nutrient composition of C. sinensis sampled from different populations varied significantly (P<0.05).Therefore, ex situ conservation did not eliminate nutritional differences between the different populations of C. sinensis. These findings highlight the importance of considering environmental and ecological factors in the nutritional assessment and cultivation of shellfish. In addition, C. sinensis from sampling sites closer to the transplantation sites had higher survival rates and growth rates, and the gonadal development of all populations showed adaption to the local environment, resulting in synchronization of the reproductive period.
1 Introduction
Owing to habitat and genetic differences, different geographical groups of the clam Cyclina sinensis show significant differences in growth performance (Liu et al., 2002), reproductive indicators (Dong et al., 2011), stress tolerance (Li et al., 2006; Liang et al., 2016), and sex ratio (Dong et al., 2021). The timing of gonadal development and differences in the energy balance of adult C. sinensis vary in response to habitat conditions (Liu et al., 2006). Different geographical populations of C. sinensis also have a high level of genetic diversity (Shao et al., 2018), with genetic differences between different C. sinensis populations being reflected in culture growth, behavioral traits, and reproduction characteristics, as well as significant differences in growth and performance and survival of juvenile clams at different temperatures (Zhao et al., 2016). In addition, the nutrient content of shellfish is affected by a variety of factors—such as shellfish species, the environment in which they grow, the stage of development, and the freshness of the raw material—resulting in large differences in the nutrient value of different groups of shellfish of the same species (Chen et al., 2006; Zhang et al., 2006; Wu and Yan, 2011; Watanabe and Katayama, 2014). However, there are few data available comparing the nutritional differences between groups of C. sinensis.
The intricate marine circulation system in China, encompassing warm currents, coastal flows, and localized circulations, combined with salinity and temperature variations between the northern and southern coasts, and the presence of rivers of varying sizes flowing into the sea, has led to geographical isolation and genetic differentiation within the eurythermal, euryhaline C. sinensis. Such high genetic diversity is indicative of the environmental adaptability, reproductive capacity, and evolutionary potential of this species and different geographical populations of C. sinensis have been reported to have high levels of genetic diversity (Shao et al., 2018).
However, in recent years, factors such as environmental degradation, overfishing, and the increasing scale of aquaculture have severely compromised this genetic diversity, leading to issues including resource degeneration, inbreeding, and suboptimal cultivation outcomes. Consequently, research into the relocation protection of C. sinensis has become vital. Given the differences in habitat and genetics, significant variation occurs among geographical populations of C. sinensis in terms of growth performance (Liu et al., 2002), reproductive indices (Dong et al., 2011), stress tolerance capabilities (Li et al., 2006; Liang et al., 2016), and gender ratios (Dong et al., 2021). Influenced by their habitats, the timing of gonadal development and energy balance of mature C. sinensis vary (Liu et al., 2006).
Thus in the current study, to understand the growth performance and body composition of C. sinensis from different geographic locations following ex situ conservation, five wild groups of C. sinensis of the same size and different geographic locations were intensively reared in the Ganyu Sea area of Lianyungang Port for 1 year. Growth parameters, environmental adaptive ability, nutritional indices, and changes in nutrient composition of the groups were assessed to provide insights into ways to preserve the genetic diversity associated with the growth of this species in the wild.
2 Materials and methods
2.1 Animals and sample processing
Five wild C. sinensis populations were sampled from Dandong (DD)(40.1243°N, 124.3947°E), Dongying (DY) (37.4645°N, 118.4914°E), Tianjin (TJ) (39.3434°N, 117.3616°E), Wenzhou (WZ) (27.9938°N, 120.6994°E), and Yancheng (YC) (33.3474°N, 120.1635°E). After disinfection and temporary rearing at the Jiangsu Provincial Shellfish Test Station, individuals of a uniform size and similar specifications were screened within each population.There are 465 clams in each group. Thirty C. sinensis were randomly sampled from each population and their body mass, shell length (SL), shell width (SW), and shell height (SH) were recorded (Table 1). They were then temporarily cultured in separate ponds for 7 days at the Jiangsu Province Shellfish System Comprehensive Experimental Station. During this period, food was withheld while oxygen was continuously supplied to facilitate sand expulsion and emptying of internal organs (food residues and feces) to minimize experimental errors caused by impurities within their bodies. Each C. sinensis was then thoroughly cleaned using pure water and a brush to remove any attachments from the surface of their body before being wiped dry with a cloth. After being weighed using an electronic balance (accurate to 0.01 g), each C. sinensis was dissected with a scalpel and their soft tissues were extracted. Absorbent paper was used to remove any excess moisture from the soft tissues before they were then weighed. The soft tissue from each C. sinensis from the same geographical population was then crushed and mixed together. A portion of this mixture was stored at –80°C, while the remaining samples underwent drying in a constant temperature drying oven at 105°C to determine the soft tissue moisture content. Once completely dried, these samples were ground into powder for subsequent nutritional analysis.
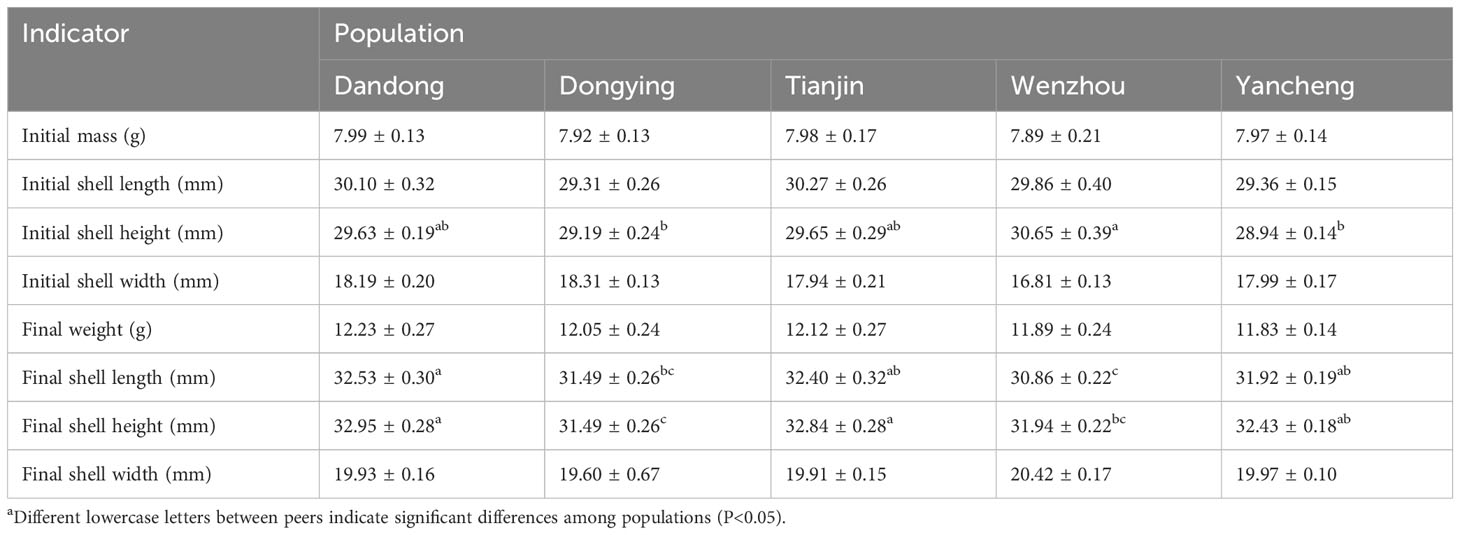
Table 1 Initial body mass, shell length, shell height, shell width; Final body weight, shell length, shell height, and shell width in different wild populations of C. sinensisa.
2.2 Experimental design
The breeding site used for the study was a natural seawater pond located in the Ganyu Sea Area of Lianyungang City in China. On August 1, 2020, five population zones were established evenly across the pond and intensively stocked with C. sinensis. The experiment ran for 366 days and concluded on August 1, 2021.
The seine net (3 m long × 3 m wide, 2 m in height, aperture diameter of 0.5 cm) was deployed in the experimental area to ensure the preservation of distinct germplasm among the different C. sinensis groups and prevent predation. All net contained a uniform distribution of 150–160 C. sinensis. Every day maintenance was conducted to address any potential gaps in the nets. The test site comprised sandy and muddy substrates, with water levels was 1.5 m. Regular water quality assessments by measuring the pond’s ammonia, nitrite, dissolved oxygen, and pH on a weekly basis, ensuring timely adjustments to maintain all environmental parameters within normal ranges.
To maximize the restoration of a natural growth environment for C. sinensis, no artificial feeding was conducted during the cultivation period, and phytoplankton, sediment, and nutrient salts in natural seawater were utilized as feed sources. During periods of high temperature, an aerator was employed at strategic intervals to ensure adequate oxygenation, keeping oxygen levels above 4 mg/L.
2.3 Data and sample collection
The growth of C. sinensis was monitored during the study, with the initial weight (W), SL, SH, and SW of the test clams measured on August 1, 2020 and then again on August 1, 2021. To ensure accurate assessment of reproductive indicators, From April 2021 onwards, 30 random samples were taken each month and subsequently dissected to observe the level of gonadal development. Through visual inspection, microscopy observations, and examination of tissue sections, it was determined that all five groups had entered their reproductive period by August 2021.
Individuals with intact and unspent gonads were selected for dissection. After sex determination, the gonads, hepatopancreas, and other soft tissues were removed using scissors and dissecting forceps. The samples were placed in labeled centrifuge tubes and measured using electronic vernier calipers and an electronic balance to determine SL (to the nearest 0.01 mm) and mass (to the nearest 0.01 g). Results of these measurements were recorded to calculate growth and reproduction indexes for each group of C. sinensis.
These results were used to calculate the growth and reproduction indexes of each group. The growth indexes included terminal body mass, terminal SL, terminal SW, and terminal SH, and were used to calculate the weight gain rate (RW), specific weight gain rate (SRW), SL growth rate (RSL), SL-specific growth rate (SRSL), SH growth rate (RSH), SH-specific growth rate (SRSH), SW growth rate (RSW), and SW-specific growth rate (SSW). Reproductive indicators included the gonadosomatic index (GSI), the hepatopancreas index (HSI), and the condition factor (CF). To ascertain the survival rate, one must conduct a meticulous exploration of a square meter area of clams, meticulously tallying the survival rates through three random excavations. The survival status was determined by counting the number of survivors and the number of empty shells in each group, and the number of parallel groups was three.
The equations used to determine each index were as follows:
where N1 and N0 represent the number of survivors and the overall number, respectively; W1 and W2 represent the average body weight (g) of C. sinensis at the start and end of the study, respectively; SL1, SW1, and SH1 represent the average SL, SW, and SH (all mm) of C. sinensis at the start of the study, respectively; SL2, SW2 and SH2 represent the average SL, SW, and SH (all mm) of C. sinensis at the end of the study, respectively; and D is the stocking level.
2.4 Biochemical analysis
2.4.1 Determination of glycogen content
The glycogen content in the mantle,adductor muscle, and gonad-visceral mass of C. sinensis was determined using a Glycogen Assay Kit (A043-1-1, Nanjing Jiancheng, China) following the manufacturer’s instructions. Five mature male and female C. sinensis were selected from each group for dissection, and their tissues were rinsed with saline before being homogenized according to sex and tissue type. For each group, 50 mg of each sample was weighed into a test tube and mixed with 150 μL of alkaline solution before being heated for 20 min at 100°C in a water bath followed by cooling under running water. The samples were then diluted based on weight (mg) with double-distilled water (μL), using a 1:16 ratio of the dilution of the original test solution. Chromogenic powder was added to each tube along with a corresponding volume of chromogenic solution made by adding 25 mL concentrated H2SO4 to a final concentration of 98%. After thorough mixing, samples were heated for an additional 5 min at 100°C before measuring OD values using a spectrophotometer; glycogen content was calculated as follows:
where 20 reflects the number of times the sample was diluted before carrying out the measurements; and 100 reflects the number of times they were diluted during the measurement.
2.4.2 Nutrient content analysis
Thirty individuals from each population were randomly selected for nutrient content analysis.The various determinants of the nutrient content of the soft tissue of C. sinensis were analyzed as follows: moisture content, using the direct drying method according to GB5009.3-2016;Weighing bottles were dried and weighed within 2 mg, and a mixed sample of the mollusc parts of C. sinensis (3.0-5.0 g, weighed to 0.0001 g) was added to each bottle, which was then uncapped and placed in a 105°C oven to dry for 16 h. At the end of the drying period, the bottles were placed in a desiccator to dry for 0.5 h, and then weighed and recorded; after that the bottles were placed again in the oven to dry for 6 h, and then placed in a desiccator to dry for 0.5 h, and then weighed for a second time. After that, the weighing bottle with the sample was put into the drying oven again for 6 h, and then put into the desiccator for 0.5 h for the second weighing. If the weighing error is >0.002 g, the drying process is repeated until the two weighing errors are within the specified range. crude protein content, using the Kjeldahl method, following GB5009.5-2016; crude fat content, using the cable extraction method following GB/T 14772-2016; the ash content, determined following GB5009.4-2016; fatty acid content, using the area normalization method, following GB5009.168-2016; and the amino acid content, using oxidative acid hydrolysis GB/T 18246-2019.
2.4.3 Nutritional evaluation of amino acids
Muscle Amino Acid Score (ASS), Muscle Amino Acid Chemistry Score (CS), and Essential Amino Acid Index (EAAI) were used to evaluate the amino acid nutrition of the different groups of C. sinensis. ASS was calculated by adopting the model of amino acid scoring criteria (%, dry) jointly recommended by the Food and Agriculture Organization of the United Nations and World Health Organization, using the following equation:
where aa is the corresponding amino acid content (%) in the sample and AA[FAO/WHO] is the corresponding amino acid content (%) in the scoring standard model.
CS was calculated using the amino acid standard model of whole egg protein (%, dry) with the following equation:
where aa is the amino acid content of the protein in the test sample (mg/gN) and AA (egg) is the content of the same amino acid in the egg protein (mg/gN).
EAAI was determined using the following equation:
where n denotes the number of essential amino acids for which comparisons were made, A represents the essential amino acid content (%) of the protein of the mollusk portion of C. sinensis, and B represents the corresponding essential amino acid content (%) in the protein of the whole egg.
2.5 Statistical analysis
The results were analyzed using SPSS 19.0 (IBM, USA), and one-way ANOVA was used to analyze the differences between groups for data with more than two groups and satisfying the chi-square test for variance. When the variance did not satisfy the chi-square test, significance was analyzed using the Games–Howell test. Differences between two groups were analyzed using the independent samples t-test, with the level of significance set at P<0.05. Results are expressed as mean ± standard error (SE).
3 Results
3.1 Annual changes in water temperature and water quality in the study area
The average monthly water temperature in the study area ranged from 4.20°C to 27.91°C (Figure 1), reflecting seasonal changes.Ponds are adjacent to the sea and exchange water with the ocean through tides, and the ambient water temperature is essentially the same as the pond water temperature. The salinity of the water body ranged from 21.2 to 24.3, the pH ranged from 7.63 to 8.03, and the dissolved oxygen ranged from 4.9 mg/L to 6.21 mg/L. The salinity, pH, and dissolved oxygen were relatively stable, which met the conditions required for the normal growth of C. sinensis.
3.2 Comparison of C. sinensis population survival rates
The survival rates of the five different wild populations of C. sinensis after 1-year ex situ stocking were as follows: YC (65.86 ± 0.57)%>TJ (64.63 ± 2.72)%>DY (55.50 ± 4.88)%>DD (47.66 ± 3.29)%>WZ (29.90 ± 2.40)% (Table 2). There were significant differences in the survival of some groups after ex situ stocking. For example, the survival rate of the WZ group was significantly lower than that of the other four groups (P<0.05), whereas that of the DD group was significantly lower than that of the TJ and YC groups (P<0.05), but the difference with the DY group was not significant (P>0.05).
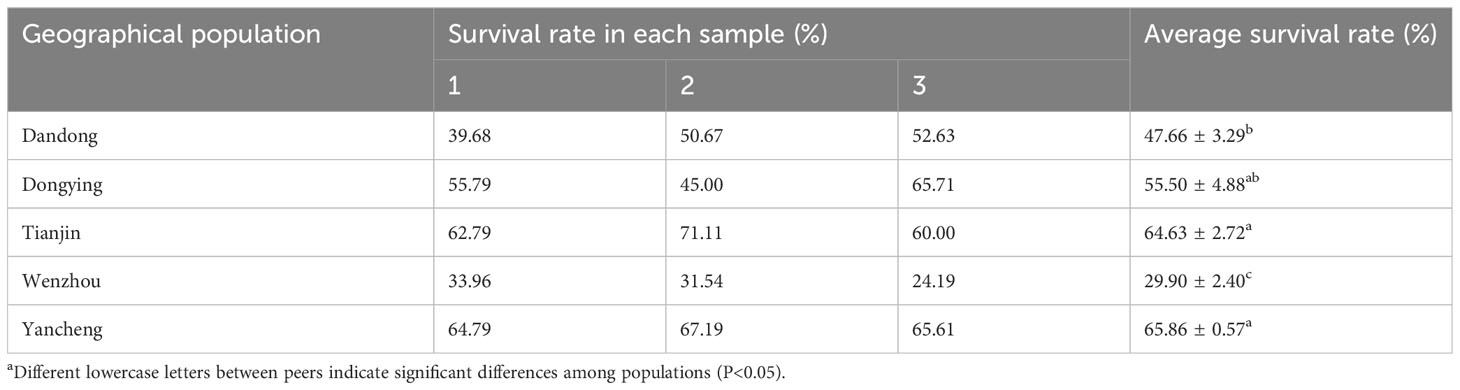
Table 2 Percentage survival rate of the five populations of C. sinensisa.
3.3 Comparison of C. sinensis population growth performance
The growth measurements at the start and end of the experimental period are detailed in Table 1, respectively. The average mass of C. sinensis among the five groups at the end of the experiment ranged from 11.83g to 12.23g, with no significant difference observed among the groups (P>0.05). Significant variations were observed among all five groups in terms of final SL and final SH (P<0.05). There was no significant difference in mean initial SL and SW across all five groups. However, by the end of experimental period, the terminal SLs in the DD and TJ groups were similar. These groups demonstrated significantly longer terminal SL compared with the DD and WZ groups (P<0.05).
The terminal SH of the five groups of C. sinensis ranged from 31.49 mm to 32.95 mm, with the highest being in the DD group (32.95 ± 0.28 mm) and the lowest in the DY group (31.49 ± 0.26 mm). The terminal SH of the DY group were significantly lower than those of the other groups, whereas those of the DD and TJ groups were significantly higher than those of the WZ group (P<0.05).
3.3.1 Growth rates of growth indicators
Table 3 details the growth rates calculated using the initial and final values of each growth metric. The WZ group had a significantly slower SL growth rate compared with the other four groups (P<0.05), but a significantly faster growth rate of SH (P<0.05). In terms of specific growth rate, the specific weight gain rate of all five groups exceeded 0.10%, and there was no significant difference between the groups (P>0.05). The highest specific growth rate in SL was observed in the YC group (0.0227 ± 0.0016)%-d–1, whereas the lowest was in the WZ group (0.0088 ± 0.0019)%-d–1, which was significantly lower compared with the other groups (P<0.05).
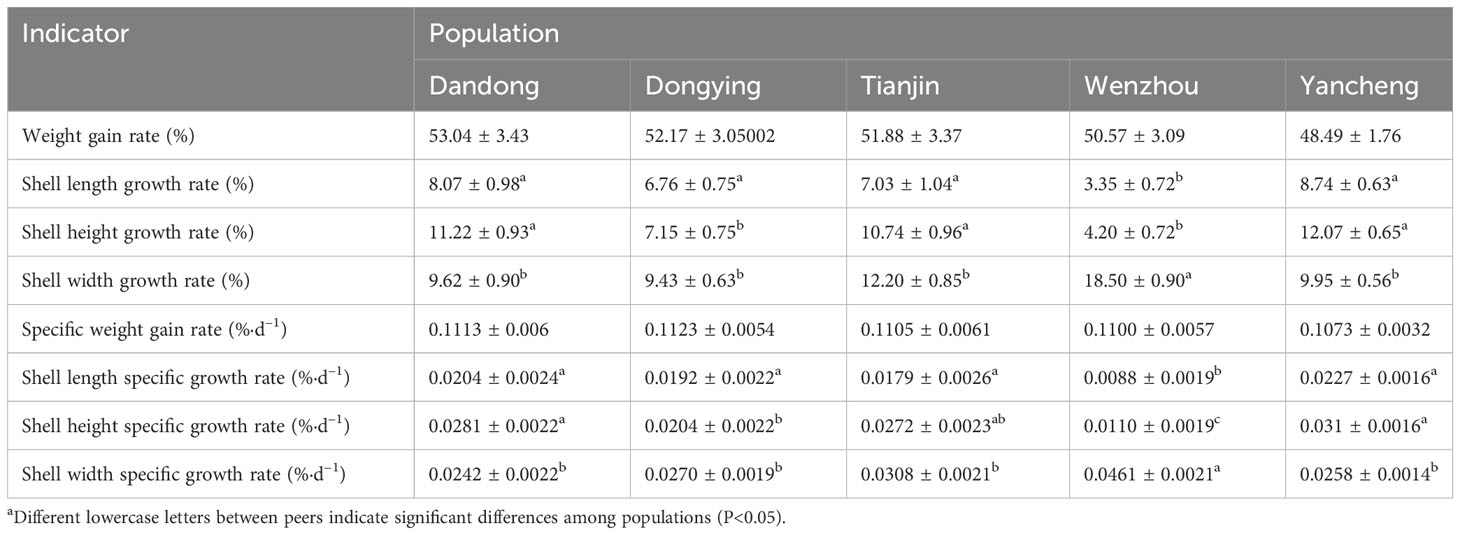
Table 3 Percentage growth rates of various indicators of five populations of C. sinensisa.
The SH-specific growth rates of the five groups decreased in order as follows: YC > DD > TJ > DY > WZ. Notably, the WZ group displayed significantly lower growth rates compared with the other four groups, whereas the DY group showed significantly lower growth rates compared with both the DD and YC groups (P<0.05).
3.4 Reproductive indicators of C. sinensis
After June 2021, the average monthly water temperature in the study area approached 20°C. The gonads of C. sinensis gradually matured, allowing for initial determination of their sex through dissection. However, gamete emission had not yet occurred. From late July to early August, samples were collected from each group of C. sinensis and inspected visually. Both male and female C. sinensis in each group exhibited fully developed gonads that were visible to the naked eye, with the entire visceral mass filled with mature gametes. Histological examination confirmed that spermatozoa in all groups of clams had reached maturity.
3.4.1 Reproductive indicators of female C. sinensis
Reproductive indicators (GSI, HSI), were compared among the five groups of C. sinensis (Figure 2). The GIS of female C. sinensis ranged from 6.99% to 9.11%, but with no significant difference among the groups from (P>0.05). The plumpness of female C. sinensis ranged from 16.78% to 22.03%, being highest in the DD group. One-way ANOVA revealed that females from the DD group were significantly more plump than females from the DY group, and that the environmental adaptations of the wild groups were higher than other group (P<0.05). In terms of HSI, the ratios ranged from 0.58% to 0.69%, but did not differ significantly among the groups (P>0.05).
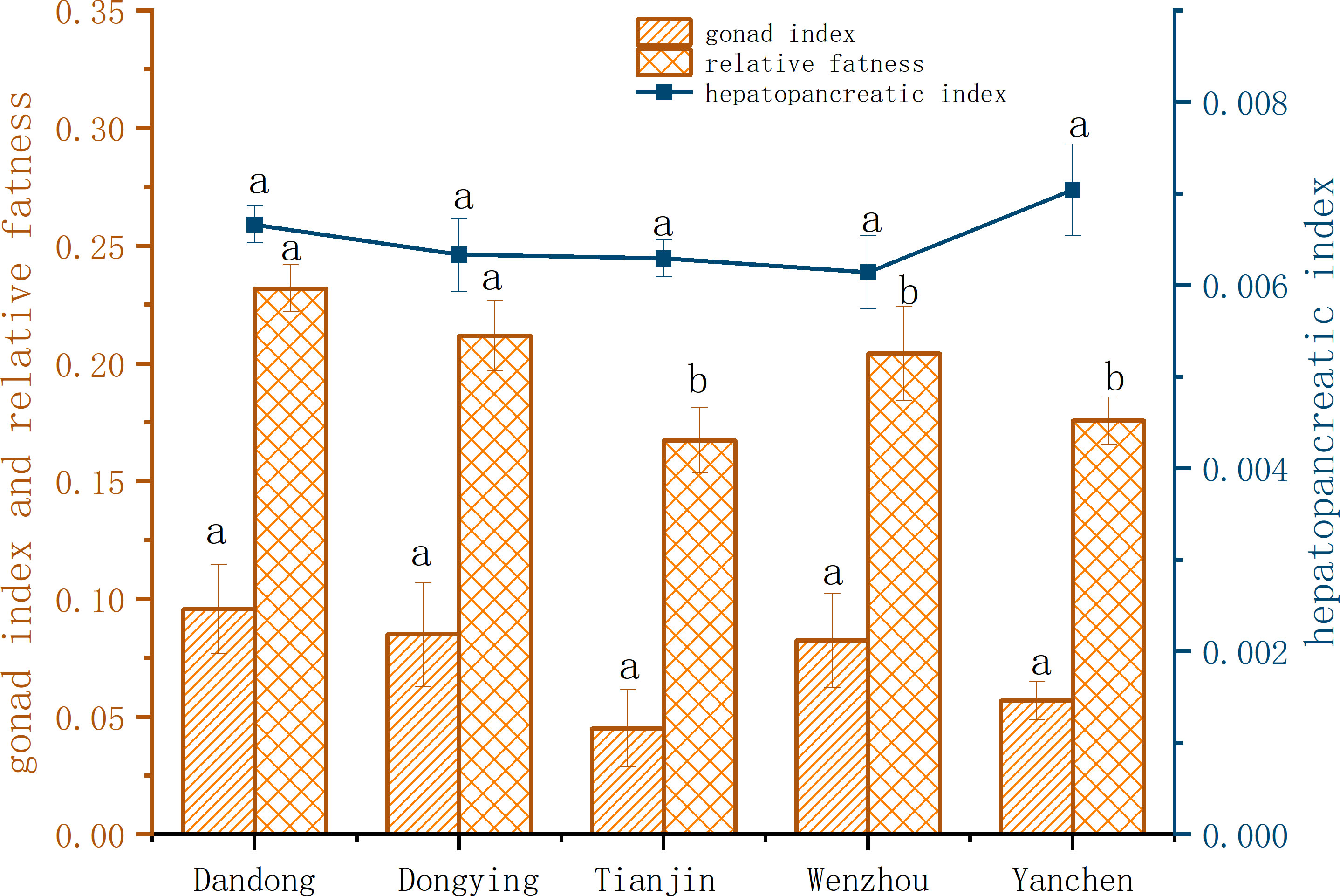
Figure 2 Comparison of reproductive indexes of female C. sinensis from different populations. Different lowercase letters on the same index indicate significant differences among different populations (P<0.05).
3.4.2 Reproductive indicators of male C. sinensis
There were no statistically significant differences in HSI or GSI among males from the five groups (P>0.05) (Figure 3). In terms of plumpness indices, males in the DD group were most plump, followed by the DG group, whereas YC group were the least plump, with the results being significantly different (P<0.05).
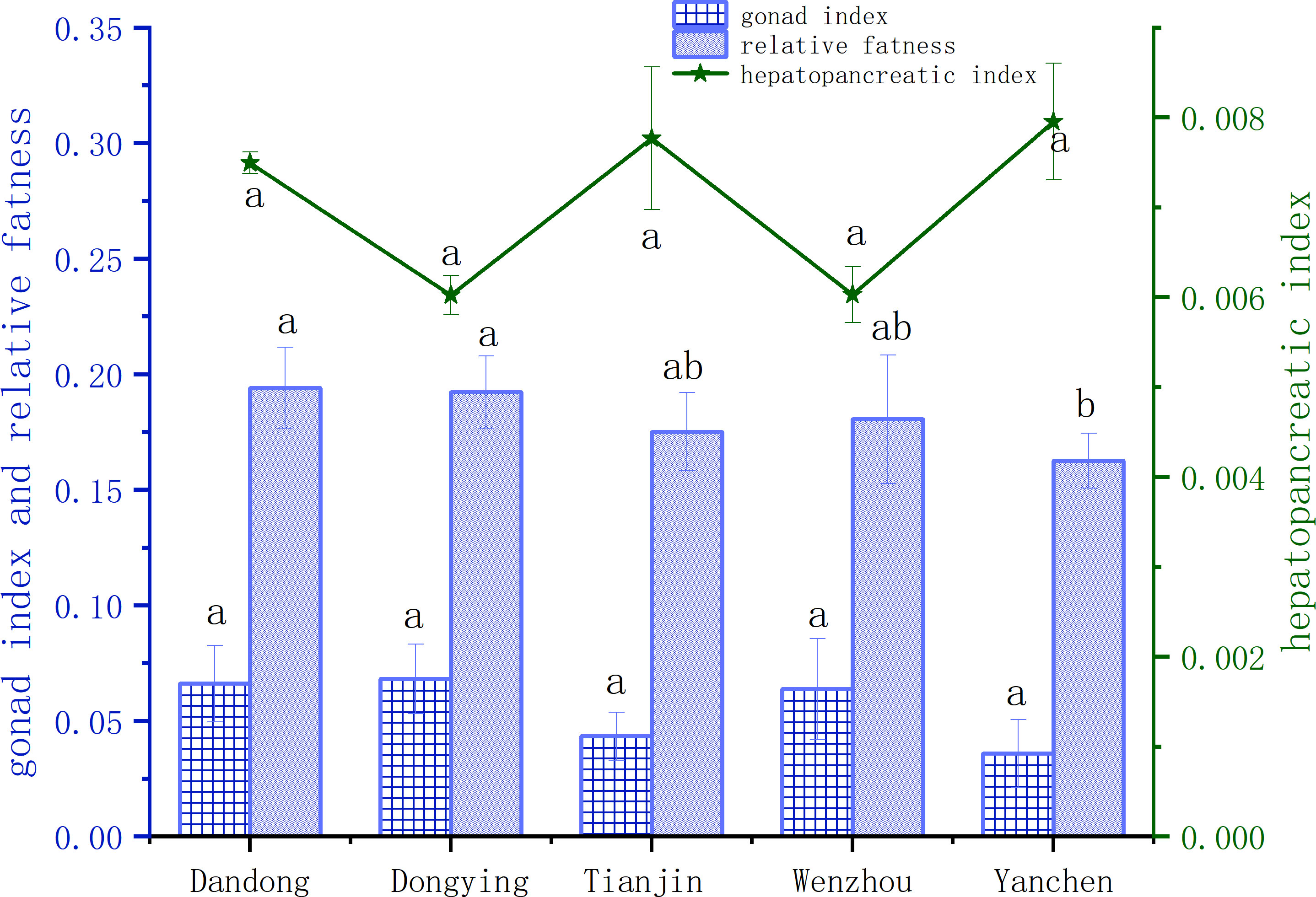
Figure 3 Comparison of reproductive indexes of male C. sinensis from different populations. Different lowercase letters on the same index indicate significant differences among different populations (P<0.05).
3.5 Glycogen content of C. sinensis tissues
3.5.1 Glycogen content of tissues of female C. sinensis
The glycogen contents of the outer coat membrane, closed-shell muscle, and gonad-visceral mass of female C. sinensis from different groups are shown in Figure 4. The glycogen content in the coat membrane of female C. sinensis from the YC group was significantly lower than that in the other four groups (P<0.05), whereas that of the closed-shell muscle of female C. sinensis from different groups did not differ significantly (P>0.05). By contrast, the glycogen content of the gonad-visceral mass of female C. sinensis from different groups varied considerably, being significantly higher in the DD group compared with all other groups (P<0.05) and lowest in the TJ group,.
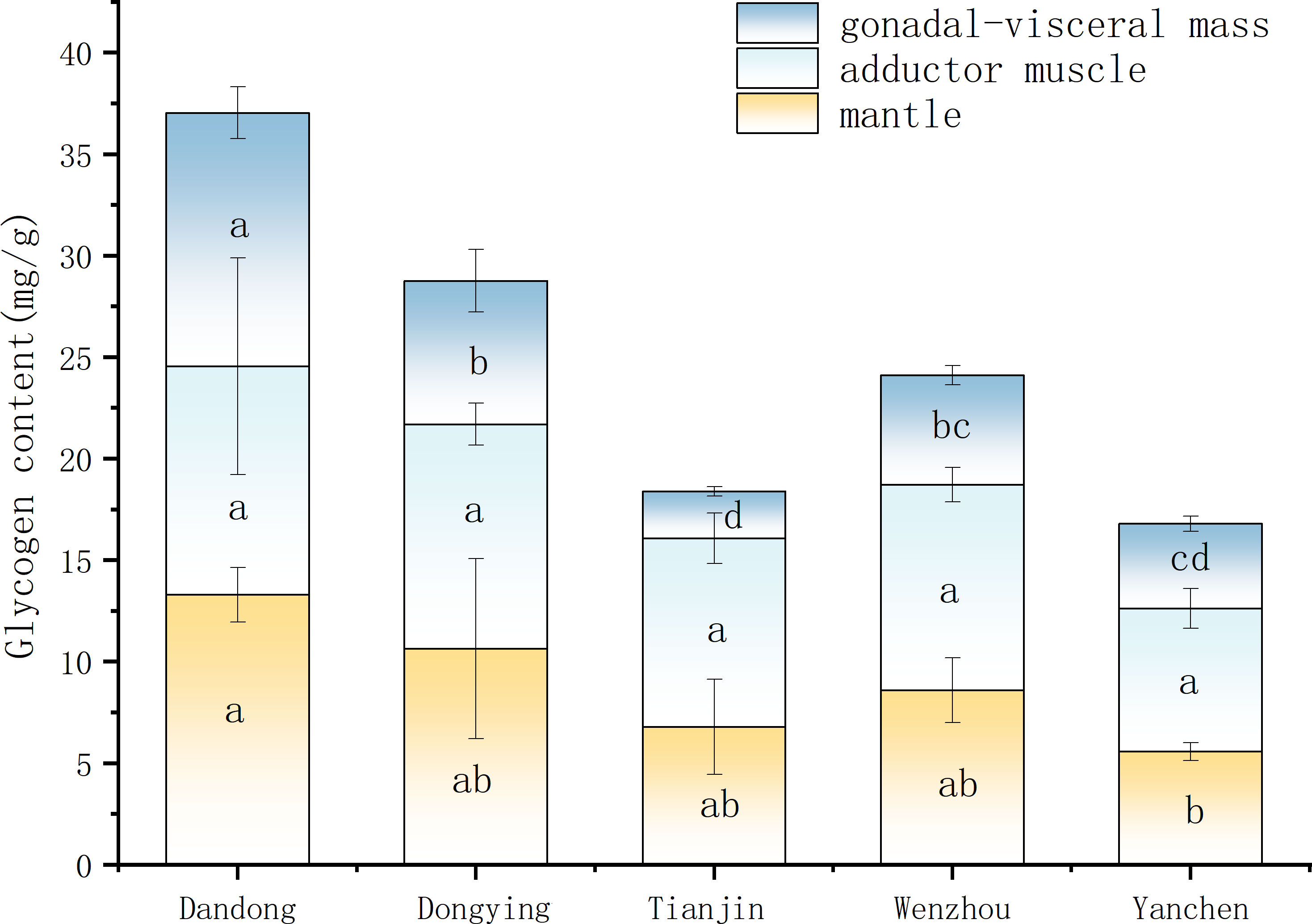
Figure 4 Comparison of glycogen content of female C. sinensis from different populations. Different lowercase letters on the same index indicate significant differences among different populations (P<0.05).
3.5.2 Glycogen content of tissues of male C. sinensis
The glycogen contents of three tissues of male C. sinensis are shown in Figure 5. The glycogen content of the coat membrane of male C. sinensis from the DD group was significantly higher than that of the other groups (P<0.05), with no significant differences found among the other groups (P>0.05). In addition, the glycogen content of the closed-shell muscle of male C. sinensis was significantly higher in the DD group compared with all other groups (P<0.05), and lowest in the DY group. The glycogen content of the gonads of male C. sinensis from different groups ranged from 4.96 to 10.68 mg/g, being highest in the DY group and lowest in the WZ group, the latter being significantly lower compared with all other groups (P<0.05).
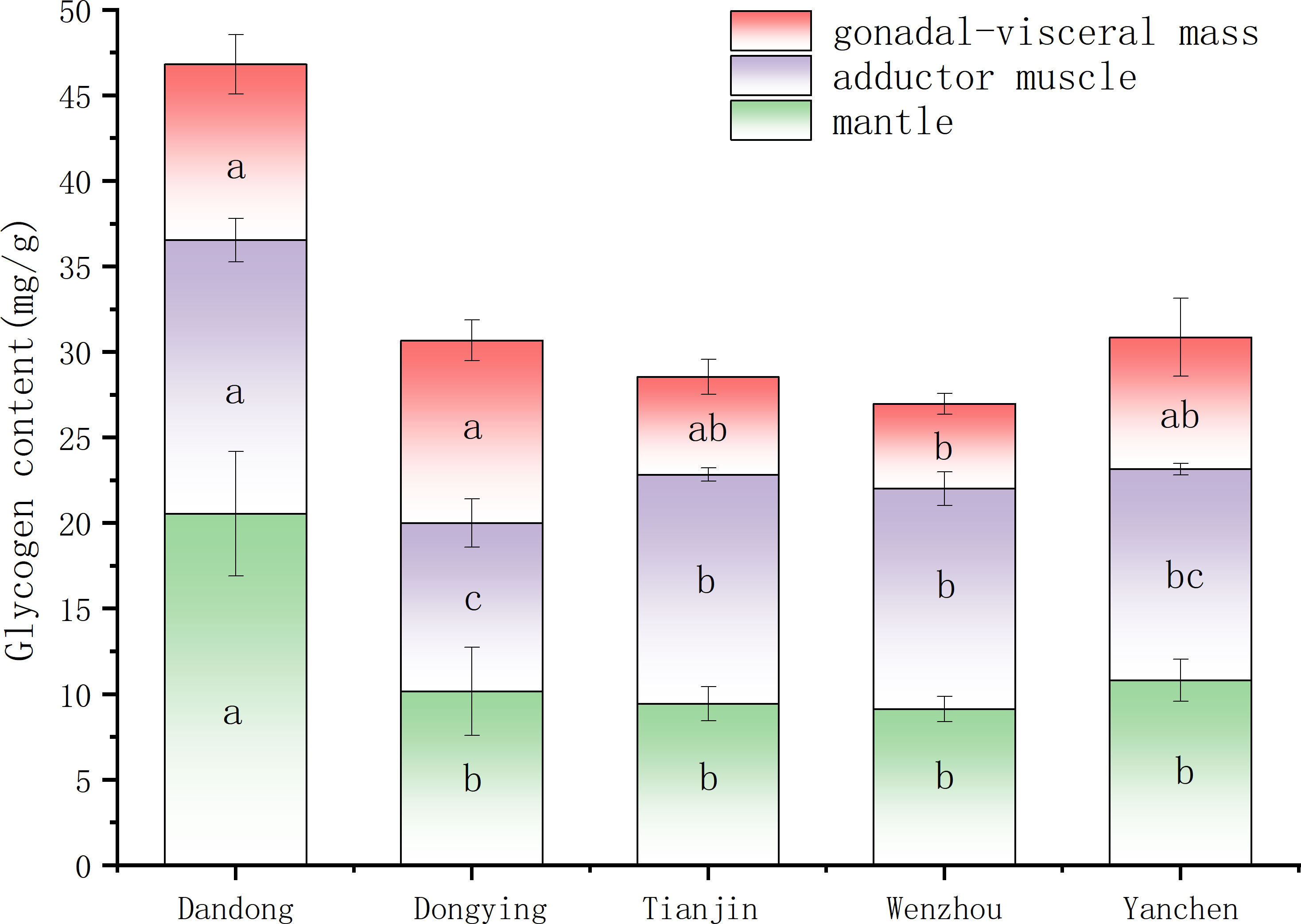
Figure 5 Comparison of glycogen content of male C. sinensis from different populations. Different lowercase letters on the same index indicate significant differences among different populations (P<0.05).
3.6 Comparison of the nutritional value of C. sinensis
The general nutritional composition of the soft tissues of five wild populations of C. sinensis were determined at the start of the study (Group A from each location) and then 1 year after ex situ conservation in the same environment (Group B from each original location) (Table 4). The moisture content was highest in the DY A and TJ A groups (82.21 ± 0.29% and 82.04 ± 0.05%, respectively) and lowest in DD A, which was significantly lower than that of the other four groups (P<0.05). The crude protein contents of DD A, WZ A, and DY A were significantly different from each other, with that of DY A being significantly lower than that of the other four groups (P<0.05).
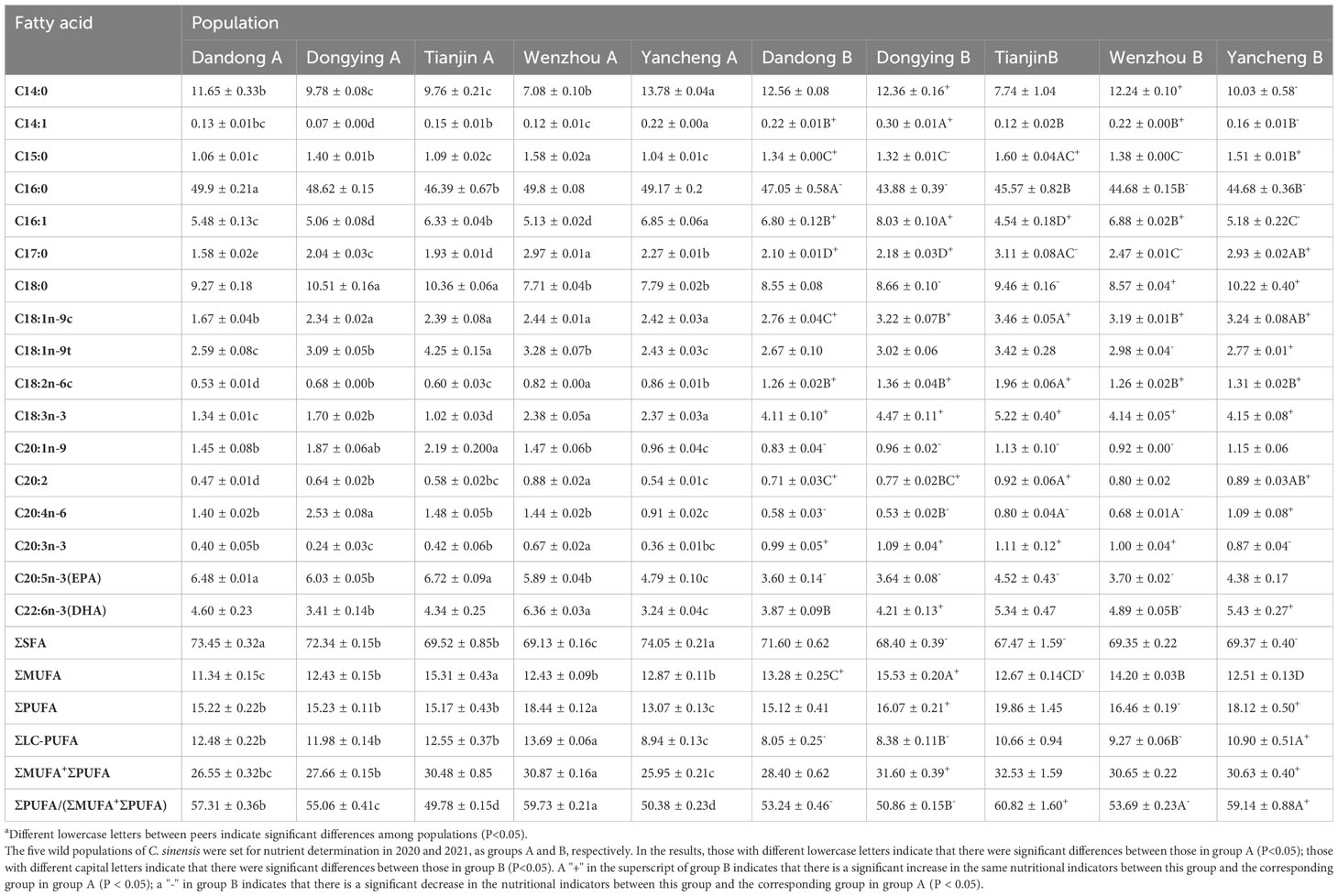
Table 4 Percentage fatty acid composition of five populations of C. sinensis before (A) and after (B) transplantationa.
The crude fat content ranged from 3.44% to 8.60%, being highest in WZ A and YC A (8.60 ± 0.57% and 8.27 ± 0.13%, respectively), which were significantly higher than in DD A, DY A, and TJ A (P<0.05). There was no significant difference in the ash content among the five groups (P>0.05).
The moisture content of the five populations of C. sinensis after 1 year in the same environment ranged from 80.62% to 82.62%, being highest in DY B and lowest in WZ B; there was a significant difference in moisture content only between these two populations (P<0.05). The crude protein content ranged from 55.88% to 62.03%, being highest in YC B, and lowest in WZ B, with a significant difference between these two groups (P<0.05). There was a significant difference in average crude fat content between TJ B and WZ B (P<0.05) and between WZ B and DY B in terms of ash content (P<0.05).
The results also showed that, except for the ash content, the biochemical composition of the different populations of C. sinensis changed significantly after ex situ conservation in terms of comparisons between the same geographic groups. Among the five populations, the moisture content of DD B was significantly higher than that of DD A, whereas that of the TJ B population showed a significant decrease (P<0.05). There was a significant increase in crude protein in DY B compared with DY A, but a significant decrease in TJ B compared with TJ A, and WZ B compared with WZ A (P<0.05). The crude fat content of the soft tissues of two groups, DD B and DY B, were significantly increased (P<0.05) compared with that of DD A and DY A, respectively.
3.7 Comparison of fatty acid composition
Five saturated fatty acids (∑SFA), five monounsaturated fatty acids (∑PUFA), and seven polyunsaturated fatty acids (∑PUFA) were detected in the soft tissues of the five populations of C. sinensis (Table 4). The EPA content of TJ A was significantly higher than that of DY A, WZ A, and YC A, whereas the DHA content of WZ A was significantly higher than that of DY A and YCA (P<0.05).
After transplantation, the fatty acid species measured in the soft parts of the C. sinensis of the five groups were the same as those 1 year previously. The saturated fatty acid content of DY B was significantly higher than that of the other four groups (P<0.05), whereas there were no significant differences in the PUFA content among the five groups (P>0.05). However, the long-chain PUFA content was highest in YC B and lowest in DD B, with a significant difference between the two groups (P<0.05). Moreover, there were no significant differences among the five populations after transplantation in terms of the EPA, ΣSFA, ΣPUFA, and ΣMUFA + PUFA content (P>0.05).
By contrast, the total amount of saturated fatty acids in the transplanted DY, TJ, and YC populations was significantly lower than at the start of the study (P<0.05). The total amount of monounsaturated fatty acids in the DD and DY populations was significantly higher than before transplantation, whereas that in the TJ population was significantly lower (P<0.05). The PUFA content in the DY and YC populations was significantly higher, whereas that in the WZ population was significantly lower (P<0.05). However, there were no significant differences in the long-chain PUFA content before and after transplantation, although it increased significantly in the YC group compared with the other groups, but decreased significantly in the DD, DY, and WZ groups (P<0.05). The total amount of unsaturated fatty acids in the DY and YC groups was significantly increased, and ΣPUFA/(ΣMUFA + ΣPUFA) was elevated significantly in the TJ and YC groups, and significantly elevated in the DD, DY and WZ groups significantly decreased (P<0.05).
3.8 Comparison of amino acid composition
In total, 17 amino acids were detected in the soft tissues of the five populations of C. sinensis, including seven essential amino acids, two semi-essential amino acids, and eight non-essential amino acids (Table 5).
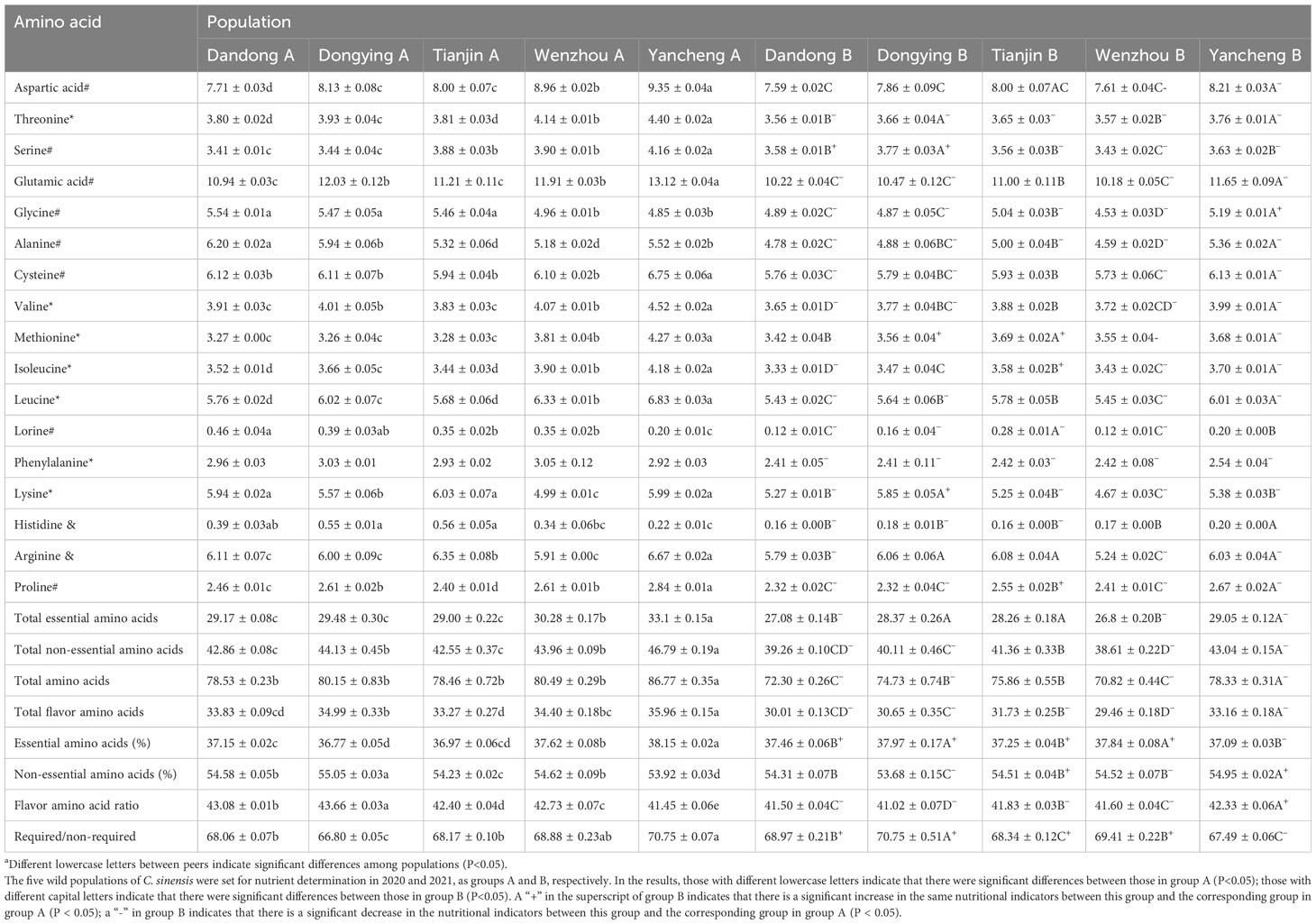
Table 5 Percentage amino acid composition of five populations of C. sinensis before (A) and after (B) transplantationa.
The proportion of essential amino acids in YC A was significantly higher than that in the other four groups (P<0.05), whereas that of non-essential amino acids in DY A was significantly higher than that in the other four groups (P<0.05). There was no significant difference in the proportion of non-essential amino acids between DD A and WZ A (P>0.05), but there was a significant difference in the proportion of fresh flavor (Glutamic acid,Glycine,Alanine and Aspartic acid) amino acids between the five groups (P<0.05).
After 1 year of ex situ conservation, there was no change in the amino acid profile of the groups, although the specific contents differed significantly. The essential amino acid contents of DD B and WZ B were significantly lower than those of the other three groups (P<0.05), whereas the difference between YC B, TJ B, and DY B was not significant (P>0.05). The non-essential amino acid content of YC B was significantly higher than that of the other four groups (P<0.05). The total amount of amino acids of DD B and WZ B was significantly lower than that of the other three groups (P<0.05), and the fresh flavor amino acid content of YC B was significantly higher than that of the other four groups (P<0.05). The proportion of essential amino acids in DY B was significantly higher than that in DD B, TJ B, and YC B (P<0.05). The proportion of essential amino acids to non-essential amino acids in DY B was significantly higher than the other four groups (P<0.05). The proportion of non-essential amino acids and of fresh flavor amino acids was highest in YC B. The proportion of essential amino acids compared with non-essential amino acids and fresh flavor amino acids of DD B and WZ B was significantly higher than that of TJ B and WZ B (P>0.05).
3.9 Amino acid scores for soft tissues
The crude protein content of each group (Table 6) was converted into milligrams of amino acids per gram of protein, and the essential amino acid content, AAS, CS, and EAAI of the soft-bodied tissues of each group were calculated according to the FAO/WHO standard model and the amino acid standard model of the protein of the whole egg. Whether the limiting amino acids in the soft-bodied fraction changed or not, the first limiting amino acid of the populations of C. sinensis in the AAS model was phenylamino acid + complex amino acid, and the second limiting amino acid was valine. There was no change in the first and second limiting amino acids of the five populations after 1 year of ex situ conservation (Table 7). After transplantation of the wild populations of C. sinensis, the EAAI of all populations decreased, apart from that of TJ B, which was slightly higher than that of TJ A (Table 8).
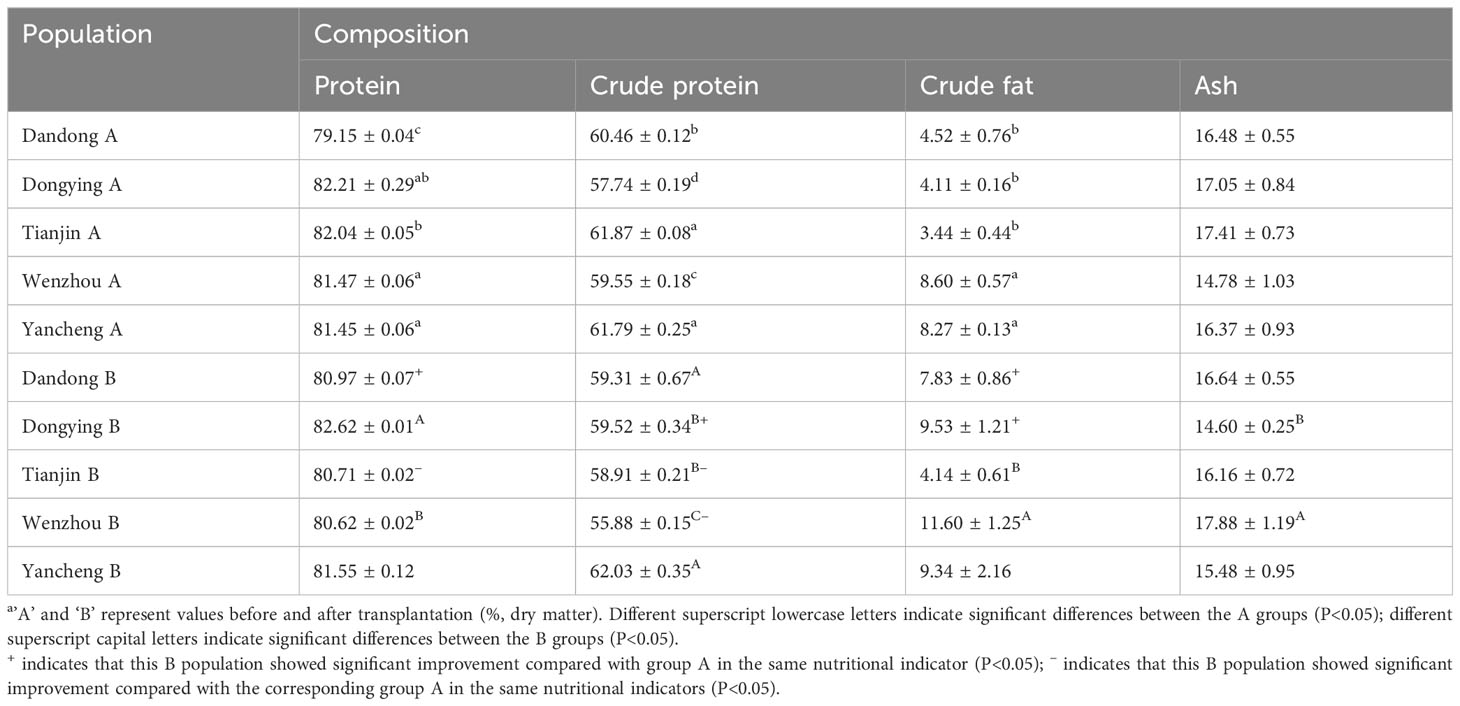
Table 6 Proximate composition of soft tissues of five populations of C. sinensisa.

Table 7 AAS scores of essential amino acids in the soft tissue of five wild populations of C. sinensis before (A) and after (B) transplantation.
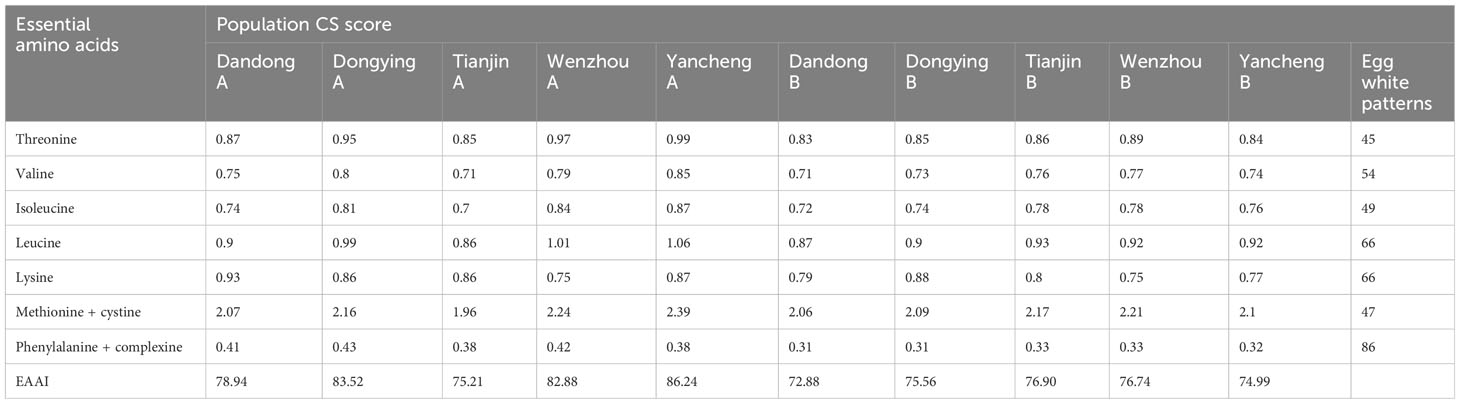
Table 8 CS scores and essential amino acids index EAAI in the soft tissue of C. sinensis before (A) and after (B) transplantation.
4 Discussion
4.1 Differences in survival rates of different populations after ex situ conservation
Bivalve shellfish are susceptible to pathogens and environmental fluctuations and, in response to such threats, C. sinensis activates resistance genes (Yao et al., 2018; Hou et al., 2019). The survival or mortality of transplanted individuals not only reflects natural selection, but also indicates the adaptability of different populations to their habitats. In terms of the survival rates during the study, both the YC and TJ groups had a survival rate exceeding 60%, whereas the WZ group had the lowest survival rate, which did not even reach 30%. Geographically, the origin of the YC group was closest to the study area and exhibited the most similar environmental conditions. Conversely, the origins of the TJ and DY groups were relatively close to each other, and no significant difference was observed in their post-transplantation survival rates. Different populations of C. sinensis display varying capacities for temperature adaptation because of genetic differentiation (Jin, 2005; Chen et al., 2016). The collection sites of the DD and WZ populations were farthest from the study area; DD was from the northernmost location, whereas WZ was from the southernmost location. Variations in the annual seawater temperature at the collection sites of the DD and WZ populations differed significantly from those observed at the experimental area, which could explain the lower survival rates of these groups at the study site. Therefore, temperature could be one of the primary factors contributing to lower survival rates in these two groups compared with the other wild populations.
As a benthic shellfish, C. sinensis is highly susceptible to humus and bacterial infections in their environment, which could have a detrimental impact on their survival rate (Chen et al., 2016). To facilitate daily management and environmental monitoring, this study used offshore ponds and simulated natural environments for stocking different groups of C. sinensis. However, smaller water bodies have limited self-purification capacity, leading to the production of harmful substances through organic matter decomposition and subsequent substrate deterioration (Zhou, 2018). Therefore, sowing different groups in the self-purifying environment of Lianyungang beach or using alternative stocking methods, such as net-pen or hanging-bag culture, could enhance the survival rate of C. sinensis in aquaculture systems (Wang et al., 2003).
4.2 Variability in growth performance and reproductive indicators
Growth-related traits and disease resistance of shellfish can be influenced by parental inheritance, and the growth performance of different populations has a crucial role in determining optimal shellfish selection. The study results revealed that the DG C. sinensis population exhibited superior overall performance in terms of body mass, SL, and SH increases. Conversely, the WZ population displayed lower increments in SL and SH after transplantation, but exceptional growth in terms of SW increases.
The findings also suggest that establishment of hybrid populations of C. sinensis should be based on the degree to which morphological traits influence body mass. Different populations of C. sinensis are influenced by local water temperature and environmental factors, and their reproductive periods have a specific duration, thus imposing limitations on the hybrid breeding of C. sinensis (Li et al., 2005a). Common methods for catalysis include shade drying, running water stimulation, and temperature regulation, among others (Li et al., 2005b). It was observed that 1 year of environmental transplantation enabled different wild populations of C. sinensis to achieve overall synchronization in their reproductive period.
Higher reproductive indexes can, to a certain extent, reflect the adaptability of the population to the local environment. Conversely, higher index coefficients for good growth and development indicate limitations imposed by environmental factors on development. Dong et al. observed significant differences in three reproductive indicators between two wild populations of C. sinensis, whereas this study found no significant differences in GSI and HSI 1 year after transplantation, suggesting that the environment has a crucial role in influencing the reproductive indicators of C. sinensis (Dong et al., 2011).
The groups with higher GSI exhibited superior fecundity, indicating a strong correlation between fecundity and GSI of C. sinensis. Previous studies also highlighted the close relationship between fecundity and gonadal development in shellfish (Kim et al., 2005; Ke et al., 2010). Zhao et al. observed variations in predevelopmental rates among females of different Chinese mitten crab species under identical culture conditions, whereas, by the end of October, there were no significant differences in GSI between the populations (Zhao et al., 2017). These studies demonstrated that samples originating from different locations gradually acclimatize to local environmental conditions after 1 year or more of cultivation, leading to a convergence in growth patterns and reproductive indices over time (Ahn et al., 2016).
4.3 Glycogen differences
Glycogen phosphorylase is the rate-limiting enzyme for glycogen degradation, which directly replenishes blood glucose through glycogenolysis. Additionally, glycogen has a crucial role in germ cell synthesis. In shellfish, the synthesis of yolk proteins heavily relies on lipid production from glycogen (Bayne et al., 1982). The stored glycogen in shellfish also serves as an energy source to defend against external stimuli and is vital for germ cell development. Consequently, measurement of tissue-specific glycogen content is used as an indicator of environmental stress tolerance (Ansell, 1974). Yan revealed that the lowest levels of glycogen are observed during gonad development and maturation in male and female C. sinensis. However, after spawning, glycogen content increased. Furthermore, Yan suggested that the higher body glycogen content in C. sinensis represents energy accumulation to support gamete development and overwintering post discharge (Yan, 2009).
The current results revealed that male C. sinensis had a higher overall glycogen content at maturity compared with females, suggesting their superior energy storage capacity and enhanced adaptability to the environment. Additionally, the DD group displayed significantly advantageous glycogen levels in the outer coat membrane, closed shell muscle, and gonad-visceral mass compared with the other four groups. This observation led to the hypothesis that this particular group relies on elevated glycogen reserves during autumn and winter for improved metabolic functionality.
4.4 Comparison of nutritional quality
4.4.1 Factors affecting nutritional levels
The nutritional composition of shellfish is influenced by the growth environment, developmental cycle, and meat freshness (Yang et al., 2016). Results relating to the environmental conditions of the areas where each C. sinensis group originated revealed a significant variation in their geographical locations, with obvious differences in air temperature, air pressure, and soil.
Previous studies have reported significant variations in the species composition and biomass of phytoplankton across the sampling sites. Diatoms were dominant in terms of cell abundance and species richness within the phytoplankton communities at all five sampling sites. Specifically, the shallow waters at DY contained 51 diatom species, with linear cirripedes and radiolarian algae being the dominant species (Fan et al., 2020). Yueqing Bay in WZ revealed 57 diatom species, with Sphaerophora predominantly represented by round sieve algae followed by horned Trichoderma (Song et al., 2010). The nearshore area of TJ contained 49 diatom species, primarily comprising horned Trichoderma and Sphaerophora, but the latter were dominant in terms of number of species (Yin et al., 2015). Offshore YC exhibited middle ribbed stripe algae and short-horned curved horn algae (Mao et al., 2018). Given its proximity to the Yalu River estuary and freshwater influences, the DD sampling site demonstrated lower diversity and evenness among phytoplankton communities in its marine area. This resulted in an absolute dominance in terms of individual species abundance and led to reduced diversity of edible shellfish species compared with other marine areas (Liu et al., 2013). Thus, various factors contribute significantly toward variations in nutritional quality among different groups of C. sinensis.
4.4.2 Comparison of nutritional quality
The results of the present analysis demonstrated significant variations in conventional nutrient content among different populations of C. sinensis. Compared with Gu et al.’s results (41.02% and 38.18%) (Gu et al., 2006) for cultured populations from Shooting Yang in June and December respectively, the five wild populations examined in this study demonstrated a substantial higher crude protein content before and after transplantation. However, the crude fat content was much lower than that observed for cultured populations from Sheyang in Jiangsu Province during June and December (24.08% and 22.34%, respectively). This suggests that wild populations of C. sinensis have a significantly higher value of crude protein compared with their cultured counterparts, resulting in enhanced nutritional value.
The results of nutritional studies on farmed and wild turbot demonstrated a significantly higher crude protein content in wild populations compared with farmed populations, whereas the crude fat content was significantly lower in the wild population (Han et al., 2015). Similarly, for Masu salmon, the crude protein content was significantly higher in the wild population than in the farmed population, with a corresponding significant decrease in crude fat content (Wei et al., 2020). These findings align with, and support, the results presented in this study.
4.4.3 Comparison of the nutritional quality of fatty acids
Comparison of the fatty acid composition of different groups of C. sinensis showed similar profiles in terms of fatty acid content. Fatty acid composition can serve as an indicator of nutritional quality and, although saturated fatty acids are a source of energy, excessive intake can contribute to cardiovascular diseases, such as hypertension and hyperlipidemia (Zhang et al., 2007).
The results indicated that WZ A exhibited the highest nutritional value, with long-chain PUFAs, including ARA (C20:4n-6), EPA (C20:5n-3), and DHA (C22:6n-3). Adequate intake of these fatty acids can reduce the risk of cardiovascular and neurological diseases in humans (Fernandes and Venkatraman, 1993; Sun, 1993; You et al., 2019). In the current study, the five C. sinensis groups exhibited varying fatty acid profiles because of differences in their original growth environments and food sources. YC A and DD A had higher levels of saturated fatty acids, whereas WZ A had the highest content of PUFAs and long-chain PFUAs. Therefore, we recommend using these three groups as parents for improving the fatty acid nutritional quality of C. sinensis.
4.4.4 Comparison of amino acid nutritional quality
Amino acids are fundamental building blocks of proteins. Thus, assessments of the nutritional quality of the soft tissues of C. sinensis populations should consider protein content, amino acid composition, and abundance (Zheng, 2013). Experimental results revealed that glutamic acid was the most abundant amino acid in both pre- and post-measurements across all five populations, which aligns with other nutritional research on C. sinensis (Wei et al., 2022). According to the FAO/WHO ideal protein model, a food source can be deemed high quality if it has an essential amino acid content-to-total amino acid content ratio of ~40%, along with an essential-to-non-essential amino acid ratio exceeding 60%.
According to the current results, the essential amino acid ratio of the five populations of C. sinensis before and after intensive stocking ranged from 36.77% to 38.15%. Additionally, the ratio of essential amino acids to non-essential amino acids ranged from 66.80% to 70.75%, which aligns with the ideal protein model, indicating that both before and after transplantation, all five populations of C. sinensis exhibited a well-balanced profile of amino acids and would be excellent sources of high-quality proteins. In terms of the amino acid scores, two measurements revealed consistent findings: phenylamino acid + complex amino acid were identified as the first limiting amino acid in the populations of C. sinensis according to AAS model analysis; valine emerged as the second limiting amino acid across these populations. Gu et al. also reported methionine + cystine as the first limiting amino acid in C. sinensis based on ASS scoring, while valine remained as the second limiting amino acid (Gu et al., 2006). By contrast, Li et al. identified valine as the first limiting amino acid in C. sinensis whereas phenylamino acid + complexine ranked second (Li et al., 2010).
The EAAI is a protein evaluation method, a higher ratio of which indicates closer proximity to the standard of egg white, thereby indicating superior nutritional value (Bing and Zhang, 2006; Shi et al., 2020). Based on the results obtained, the initial EAAI values for the five groups ranged from 75.21 to 86.24 (YC A > DY A > WZ A > DD A > TJ A), suggesting that proteins from the YC group could be more efficiently absorbed and utilized by the human body.
4.5 Nutritional changes in different populations after transplantation
The results of the study revealed that, even after 1 year of stock cultivation in the same environment, the nutritional variability among the five populations of C. sinensis could not be eliminated, as evidenced by substantial differences in conventional nutrients, fatty acid content, and amino acid content. Basic indicators, such as crude protein, crude fat, moisture, and ash content, are commonly used to assess nutritional quality but often vary with environmental conditions (Yang et al., 2018; Lü et al., 2022). Comparing the pre- and post-transplantation differences in conventional nutrients among populations, the YC population maintained its advantage in terms of crude protein content, while DY B exhibited a significant increase compared with DY A. Moreover, there were notable differences in crude protein content between the DY B and DY A populations. No significant variations were found in saturated fatty acids and PFUAs among all five groups following transplantation.
Meanwhile, the total essential amino acid levels were significantly lower in the DD, WZ, and YC groups 1 year after transplantation. Additionally, the total non-essential amino acid and overall amino acid contents were significantly reduced in the DD, DY, WZ, and YC groups compared with pre-transplantation. Despite this decrease, the YC group still exhibited the highest concentration of flavor-related amino acids among all five groups.
In summary, the transplantation of various wild populations of C. sinensis to local sea areas had a positive impact on the content of a limited number of amino acid species in their soft tissues, while adversely affecting the flavor profiles of geographically distinct wild populations. In terms of the amino acid index, changes were observed in the indices (72.88–76.90) and alterations in their order from highest to lowest were TJ B > WZ B > DY B > YC B > DD B. The essential amino acid index increased for TJ B (76.90) compared with TJ A (75.21), whereas protein quality significantly decreased in the other four populations. These findings suggest that most C. sinensis populations experienced reduced protein quality following transplantation into a Lianyungang Sea habitat. After 1 year of transplantation, several nutrient indices showed significant decreases compared with pre-transplantation levels, indicating that bait conditions in this region were less favorable compared with the original environments.
Furthermore, the persistence of significant differences in nutrient content among groups post transplantation suggests that 1 year of cohabitation in the same environment was insufficient to eliminate inherent variations in nutrient composition between C. sinensis populations.
5 Conclusion
Our comparisons of the environmental adaptability of five populations of C. sinensis showed that the survival rates of different populations varied greatly, with those from sampling sites closer to the transplantation sites having higher survival rates after transplantation. After 1 year of transplantation, the gonadal development of all populations had adapted to the local environment, resulting in overall synchronization of the reproduction period, which would facilitate cross-breeding. The results showed that the gonadal development of all groups also adapted to the local environment after 1 year of transplantation. Meanwhile, the GSI and HSI of C. sinensis in the reproductive stage after transplantation were no longer significantly different from each other. There was a strong correlation between the plumpness and GSI of different groups of C. sinensis, and the changes in the two tended to be consistent. Combined with the plumpness and the glycogen content of different parts of C. sinensis, the DD group increased energy storage, suggesting that this group might be more able to survive the following autumn and winter because of its superior metabolism. Meanwhile, the overall glycogen content of male C. sinensis was higher than that of female C. sinensis, suggesting that male C. sinensis have a better energy storage capacity and are more adaptable to the environment.
In the nutrient analysis and quality evaluation of the five groups of C. sinensis, the levels of fatty acids and amino acids were consistent across groups, but differed in terms of their composition. The soft body parts of different groups of C. sinensis were rich in saturated fatty acids, mainly C16:0. The contents of EPA and DHA in PUFAs were higher, indicating that C. sinensis from different sources were all of high nutritional value. The large proportion of fresh flavor amino acids in the soft tissue of C. sinensis and the ratio of EAA/TAA and NEAA/TAA in accordance with the FAO/WHO evaluation criteria for the ideal protein pattern also indicate that C. sinensis have good nutritional flavor and are a high-quality protein source.
AAS evaluation showed that the first limiting amino acid of the populations of C. sinensis was phenylamino acid + complex amino acid, and the second was valine, whereas the CS scoring pattern was slightly different, showing that the second limiting amino acid was isoleucine in the DD population and the TJ population before transplantation. The essential amino acids index was highest in the YC group and lowest in the TJ group before transplantation, but highest in the TJ group after transplantation.
Comparison of the nutritional indices before and after transplantation showed that the nutritional complexity and variability of different populations remained, suggesting that 1 year of stocking in the same environment did not eliminate the nutritional differences between different populations of C. sinensis. Despite transplantation into a common environment, significant nutritional differences persist among populations, indicating that inherent variations in nutrient composition are not easily homogenized. These findings highlight the importance of considering environmental and ecological factors in the nutritional assessment and cultivation of shellfish.
The complexity and diversity observed in the nutritional quality of C. sinensis from various groups after transplantation indicate that a singular centralized transplantation approach failed to maintain the distinct nutrient advantages for each group. Consequently, the successful ex situ conservation of C. sinensis in the Lianyungang Sea requires not only meticulous selection of superior breeding stock, but also an emphasis on enhancing bait quality and diversifying bait species to improve the overall nutritional profile.
Data availability statement
The raw data supporting the conclusions of this article will be made available by the authors, without undue reservation.
Ethics statement
The manuscript presents research on animals that do not require ethical approval for their study.
Author contributions
SY: Data curation, Formal analysis, Investigation, Project administration, Visualization, Writing – original draft. QX: Investigation, Writing – review & editing. ZC: Investigation, Writing – review & editing. GR: Investigation, Writing – review & editing. XL: Investigation, Writing – review & editing. HG: Investigation, Writing – review & editing. ML: Investigation, Writing – review & editing. ZD: Conceptualization, Funding acquisition, Writing – review & editing.
Funding
The author(s) declare financial support was received for the research, authorship, and/or publication of this article. This work was supported by the Jiangsu Natural Resources Development Special-Marine Science and Technology Innovation Project (JSZRHYKJ202008), the Jiangsu Modern Agriculture Independent Innovation Project (CX(20)3150), the ‘JBGS’ Project of Seed Industry Revitalization in Jiangsu Province [JBGS(2021)034]; Modern Agro-industry Technology Research System (CARS-49), and the Priority Academic Program Development of Jiangsu Higher Education Institutions and Jiangsu Graduate Research and Practice Innovation Program (KYCX2021-035).
Conflict of interest
The authors declare that the research was conducted in the absence of any commercial or financial relationships that could be construed as a potential conflict of interest.
Publisher’s note
All claims expressed in this article are solely those of the authors and do not necessarily represent those of their affiliated organizations, or those of the publisher, the editors and the reviewers. Any product that may be evaluated in this article, or claim that may be made by its manufacturer, is not guaranteed or endorsed by the publisher.
References
Ahn H. M., Ki H. J., Jeong H. D., Lee H. J., Han H. K., Park K. J., et al. (2016). Comparison of growth, condition index, and mortality of Manila clam (Ruditapes philippinarum) between originated from China (Liaoning Dandong) and Chungnam (Taean) in Gochang tidal flats. Korean J. Malacol. 32 (3), 175–184. doi: 10.9710/kjm.2016.32.3.175
Ansell A. D. (1974). Seasonal changes in biochemical composition of the bivalve Chlamys septemradiata from the Clyde Sea Area. Mar. Biol. 25, 85–99. doi: 10.1007/BF00389258
Bayne B. L., Bubel A., Gabbott P. A., Livingstone D. R., Moore M. N. (1982). Glycogen utilization and gametogenesis in Mytilus edulis L. Mar. Biol. Letters 3, 89–105.
Bing X., Zhang X. (2006). Evaluation of nutritional composition and quality of Siniperca scherzeri muscle. Periodical Ocean Univ. China 2006, 107–111. doi: 10.16441/j.cnki.hdxb.2006.01.021
Chen J., Yang X., Ke A., Huang B. (2006). Selection of attachment bases in artificial seed breeding of hard clams (Cyclina Sinensis). J. Shanghai Ocean University 01), 114–117.
Chen L., Zhang S., Guo L., Qin Y., Guan T., Cui F., et al. (2016). Influence of temperature on the burrowing ability of Mytilus coruscus, Meretrix meretrix, and Ruditapes philippinarum. Chin. J. Fisheries 29, 35–38.
Dong Z., Duan H., Zheng H., Ge H., Wei M., Liu M., et al. (2021). Research progress on germplasm, aquaculture, and development utilization of Cyclina Sinensis. J. Fisheries China 45, 2083–2098.
Dong Z., Li X., Cheng H., Meng X., Yan B., Shen H., et al. (2011). The influence of seasonal factors, geographical distribution, and habitat on sex ratio and reproductive parameters of Cyclina Sinensis. Trans. Oceanol. Limnol. 03, 21–26. doi: 10.13984/j.cnki.cn37-1141.2011.03.001
Fan Z., Zhao H., Song N., Gao T. (2020). Ecological investigation of shallow-sea bivalves in the Dongying Estuary National Marine Special Protection Area in autumn. Periodical Ocean Univ. China 50, 82–90. doi: 10.16441/j.cnki.hdxb.20190427
Fernandes G., Venkatraman J. T. (1993). Role of omega-3 fatty acids in health and disease. Nutr. Res. 13, S19–S45. doi: 10.1016/S0271-5317(05)80282-9
Gu R., Yu Y., Cai Y. (2006). Analysis and evaluation of nutritive composition of hard clams. Chin. J. Zoolo. 41, 70–74.
Han X., Jia L., Wang Q., Wang X., Chen C., Shang X. (2015). Comparison of nutritional compositions between wild and cultured flounder muscle. J. Guangdong Ocean University 2015, 94–99.
Hou Z., Gao S., Pan B., Yan C. (2019). Cloning of IKK gene in hard clam (Cyclina Sinensis) and its expression analysis under Vibrio Anguillarum stimulation. Oceanologia Limnologia Sinica 50, 437–442.
Jin C. (2005). Effects of temperature and salinity on the oxygen consumption rate and ammonia excretion rate of hard clams (Cyclina Sinensis). J. Lishui University 02), 46–51.
Ke Q., Li Q., Chen C., Liu H., Liu X., Yan H., et al. (2010). Reproductive biology of Manila clam in the Yellow River Delta. Periodical Ocean Univ. China 40, 99–104. doi: 10.16441/j.cnki.hdxb.2010.s1.015
Kim S., Rosenthal H., Clemmesen C., Park K., Kim D., Choi D., et al. (2005). Various methods to determine the gonadal development and spawning season of the purplish Washington clam, Saxidomus purpuratus (Sowerby). J. Appl. Ichthyol. 21.2, 101-106. doi: 10.1111/j.1439-0426.2004.00636.x
Li C., Fang B., Wang H., Zhuang P., Zhou K., Yao Z., et al. (2006). Study on the correlation of sulfides in sediment. J. Fisheries China 06, 796–800.
Li X., Dong Z., Yan B., Cheng H., Meng X., Shen H., et al. (2010). Nutritional composition analysis and evaluation of hard clam and Manila clam. Food Sci. 31, 366–370.
Liang J., Li Y., Guo Y., Gao S., Dong H., Xing K., et al. (2016). Breeding techniques for hard clam seedlings and the effects of different salinities on various developmental stages of. Cyclina Sinensis.Hebei Fisheries 12, 1–4.
Liu S., Fan J., Wang Z. (2013). Seasonal variations of phytoplankton community in the northern Yellow Sea. Acta Ecologica Sin. 22, 1173–1181.
Liu W., Ma Y., Hu S., Miao G., Li J. (2002). Rearing Venusclam seeds, Cyclina Sinensis (Gmelin), on a commercial scale. Aquaculture 211, 109–114. doi: 10.1016/S0044-8486(01)00859-6
Liu Y., Ma S., Tian X., Wang Y. (2006). Study on energy budget of hard clams (Cyclina Sinensis) under different temperatures. Periodical Ocean Univ. China 36, 89–92.
Lü M., Gan H., Chen T., Lu X., Ruan Z., Zhu J., et al. (2022). Comparison of growth performance and muscle quality between rice-field-cultured and pond-cultured Wuchang bream. Chin. J. Fisheries 35, 75–81.
Mao C., Hua W., Jiao X., Zhang X., Zhou C., Yuan G., et al. (2018). Species composition and distribution of phytoplankton in the summer of Jiangsu tidal flat. Mar. Environ. Sci. 37, 753–759+765. doi: 10.13634/j.cnki.mes.2018.05.019
Shao Y., Chai X., Zhang L., Fang J. (2018). “SSR analysis of genetic structure in six hard clam (Cyclina Sinensis) populations in China,” in Proceedings Abstracts of the 13th Zhejiang Fisheries Science and Technology Forum, Zhejiang Fisheries Society (Wenzhou, Zhejiang, China: (The Thirteenth) Zhejiang Fishery Science & Technology Forum). 1, 11. Available at: https://kns.cnki.net/kcms2/article/abstract?v=MuKJieOJLT1kUyCfw6MRCAmh_sxhz2TxITu3Fc3tRDro7umKZyAY-GUs2eJTna2rl0XF3hzFy1LIGyxcIAiDFNiJkb5PuuLaPVEoQS8ZPJhD3OHTP6x3T6ARFWzQVprNjXq5PF8PbiwwSZpEjpdtag==&uniplatform=NZKPT&language=CHS.
Shi Y., Xu J., Xie Y., Liu Y., Shui C., Lu G., et al. (2020). Analysis and evaluation of nutritional composition of roe of American shad fish. Fisheries Sci. 39, 407–413. doi: 10.16378/j.cnki.1003-1111.2020.03.014
Song L., Zhao C., Long H., Chen L., Yu J., Lv B., et al. (2010). Characteristics of phytoplankton community structure in Leqing Bay during summer 2009. J. Mar. Sci. 28, 34–42.
Wang G., Chang Y., Fu Q., Wang Z., Liu Q., Sun M., et al. (2003). Preliminary study on attachment bases and multi-layer seedling attachment technology of three intertidal bivalves. J. Dalian Ocean University 02, 104–108. doi: 10.16535/j.cnki.dlhyxb.2003.02.006
Watanabe S., Katayama S. (2014). Relationships among shell shape, shell growth rate, and nutritional condition in the Manila clam (Ruditapes philippinarum) in Japan. J. Shellfish Res. 29, 353–359. doi: 10.2983/035.029.0210
Wei M., Wu Y., Chen D., Zhang M., Wang Y., Zhang J., et al. (2022). Analysis of growth and nutritional differences among individuals with different shell colors in hard clam. J. Fisheries China 46 (08), 1458-1465. Available at: http://kns.cnki.net/kcms/detail/31.1283.S.20210318.1520.010.html.
Wei K., Zheng W., Geng Y., Ma L., Chen C. (2020). Comparison of nutritional compositions between wild and cultured large-scale mandarin fish. Chin. J. Fisheries 33, 1–6.
Wu Y., Yan X. (2011). “Analysis and evaluation of nutritive composition of six different organizations of Manila clam Ruditapes philippinarum,” in Abstracts of the 9th Member Representative Assembly of the Shellfish Branch of China Society of Oceanography and the 15th Academic Symposium, Guangzhou. 230.
Yan H. (2009). Study on the reproductive physiology of Sinonovacula constricta and Cyclina Sinensis. (Yushan Campus) Gate 4 SHANDONG Qingdao Shinan: Ocean University of China.
Yang C., Du X., Wang Q., Deng Y. (2016). Research progress on nutrient requirements and artificial feed for bivalves. Chin. J. Anim. Nutr. 28, 3422–3428.
Yang H., He J., Lv M., Huang G., Wen L., Bi X., et al. (2018). Preliminary effects of dietary protein levels on muscle quality and digestive enzyme activities in GIFT-Oreochromis niloticus. Aquaculture Stud. 18, 1–10. doi: 10.4194/2618-6381-v18_1_01
Yao Y., Wang Y., Hou Z., Wang B., Shi Y., Pan B., et al. (2018). Cloning of MAPK/p38 gene in hard clam (Cyclina Sinensis) and expression analysis under Poly:Ic stress. J. Anhui Agric. Sci. 46, 100–102+118.DOI:10.13989/j.cnki.0517-6611.2018.04.029.
Yin C., Zhang Q., Niu F., Shi H., Xu Y., Tu J., et al. (2015). Primary productivity and species composition of net-collected phytoplankton in the coastal waters off Tianjin in Bohai Bay. Mar. Sci. 33, 82–92.
You C., Lu F., Wang S., Chen C., Li Y. (2019). Comparison of growth performance and long-chain PUFA biosynthetic ability of genetically improved farmed tilapia (Oreochromis niloticus) reared in different salinities. Br. J. Nutr. 121.4, 374–383. doi: 10.1017/S0007114518003471
Zhang D., Chen Y., Zhou G., Li J. (2007). A discussion on DHA, EPA, and their applications. China Condiment 10), 30–33.
Zhang A., Li T., Su X., Liu B. (2006). Study on nutritional components of Meretrix meretrix from different geographical populations. Fisheries Sci. 25, 79–81. doi: 10.16378/j.cnki.1003-1111.2006.02.008
Zhao Y., Wang C., Liu B. (2016). A comparison of thermal adaptability among different geographical populations of hard clams (Cyclina Sinensis). J. Anhui Agric. Sci. 2016 (04), 151-153. doi: 10.13989/j.cnki.0517-6611.2016.04.049
Zhao H., Wu X., Jiang X., Long X., He J., Liu N., et al. (2017). Comparative study on ovarian development and nutritional composition of female crabs of the Chinese mitten crab (Eriocheir Sinensis) populations from the Yangtze River, Yellow River, and Liao River under pond culture conditions. J. Fisheries China 41, 109–122.
Zheng Y. (2013). Analysis of morphological differences and amino acids of different regions in wild Procambarus clarkii. District8 Hongdao Rd No. 999 Xuefu Avenue, Honggutan New District, Nanchang, Jiangxi Province: Nanchang University.
Keywords: environmental adaptation, wild populations, growth performance, nutrient analysis, Cyclina sinensis
Citation: Yan S, Xia Q, Cui Z, Ren G, Li X, Ge H, Liu M and Dong Z (2024) Environmental adaptation, growth performance and nutrient content of the clam Cyclina sinensis from different geographic locations. Front. Mar. Sci. 11:1397324. doi: 10.3389/fmars.2024.1397324
Received: 08 March 2024; Accepted: 12 April 2024;
Published: 17 May 2024.
Edited by:
Youji Wang, Shanghai Ocean University, ChinaReviewed by:
Yafei Duan, South China Sea Fisheries Research Institute, ChinaZhi Hu, Chinese Academy of Sciences (CAS), China
Copyright © 2024 Yan, Xia, Cui, Ren, Li, Ge, Liu and Dong. This is an open-access article distributed under the terms of the Creative Commons Attribution License (CC BY). The use, distribution or reproduction in other forums is permitted, provided the original author(s) and the copyright owner(s) are credited and that the original publication in this journal is cited, in accordance with accepted academic practice. No use, distribution or reproduction is permitted which does not comply with these terms.
*Correspondence: Zhiguo Dong, ZHpnNzcxMkAxNjMuY29t
†These authors have contributed equally to this work