- Department of Invertebrate Zoology and Hydrobiology, Faculty of Biology and Environmental Protection, University of Lodz, Łódź, Poland
Here we analyze available recorded occurrences for species of Neotanaoidea, a deep-sea peracarid superfamily, in the frame of biogeographic bathyal, abyssal, and hadal provinces. In addition, we provide the first phylogeny based on molecular data for this group. Despite the existence of large knowledge gaps, the observed patterns reveal levels of connectivity across biogeographic provinces, oceans, and depths higher than initially expected for a superfamily consisting of relatively small deep-sea benthic invertebrates without a pelagic larval stage, and therefore hypothetically low mobility capabilities. We have detected neotanaid species with closely related populations across the Pacific Ocean or able to overpass a geographical barrier as significant as the Mid-Atlantic Ridge. Additionally, the molecular analyses expose the need for a taxonomic review of the four genera within Neotanaoidea. A search for better suited morphological and possibly ecological characters as diagnostic traits for genera and species should be undertaken, aiming at a better definition of the existing taxa and the description of new ones.
1 Introduction
Neotanaidae Lang, 1956 is a deep-water family within Tanaidacea, represented by species with most charismatic males. They grow up larger than most tanaidaceans [although with some exceptions (e.g. Kudinova-Pasternak, 1975)], and are therefore considered the giants of this order (Gardiner, 1975; Larsen, 2005; Błażewicz-Paszkowycz et al., 2012), even when they are relatively small if compared to other invertebrates. They are distinguished by an exceedingly marked sexual dimorphism (Wolff, 1956; Gardiner, 1975) in addition to a set of unique morphological characteristics revealed by the females (Wi et al., 2015). Neotanaids lack eyes and the thoracic or spinning glands found in other tanaidaceans, they have antennules with seven or eight articles and antennae with nine, a mandible with strong and heavily calcified molars, well-developed maxillae with multiple specialized setae, an ischium is present in the chelipeds (although small and incomplete), and they count five pairs of pereopods, all of similar unspecialized “walking” type with a coxa present in all of them (e.g., Kakui et al., 2011 or Larsen et al., 2015, Figure 1A).
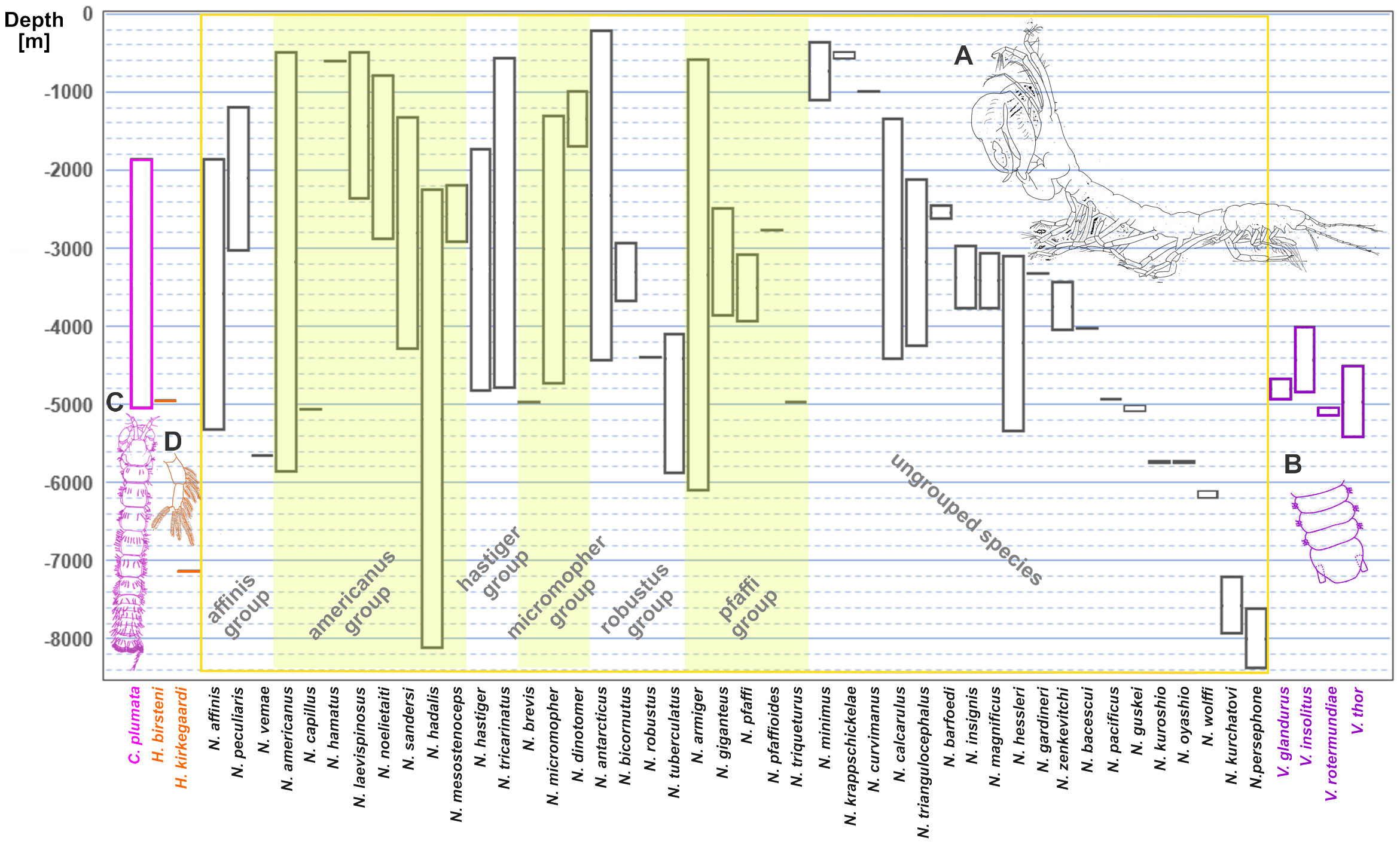
Figure 1 Depth ranges for the currently accepted 51 species and 4 genera of the family Neotanaidae. The text colour of the species names groups them into the four genera: Carololangia, Herpotanais, Neotanais, and Venusticrus. Golden shades separate the genus Neotanais into the six “Gardiner groups” (Gardiner, 1975). (A) Male of a species of Neotatanis from the Sea of Okhotsk. (B) Part of the pleotelson of V. thor, redrawn from [Araújo-Silva et al. (2015), (fig. 5K)], to show the ventral attachment of the uropods, characteristic for the genus. (C) Carololangia plumata, only species of its genus, redrawn from [Gardiner (1975): (fig. 74, as C. mirabunda)], and (D) pleopod of Herpotanais kirkegaardi, with one ramus only, characteristic of the genus, redrawn from [Gardiner (1975): (fig. 88)].
Despite them being found in all oceans at depths starting at 223 m (Neotanais antarcticus Kussakin 1967, www.obis.org, last visited 02 Feb 2024), neotanaids are not as often collected from the oceanic floor as other tanaidaceans and much is still unknown about their distribution patterns, life history, or behavior. They form a relatively diverse family represented currently by a total of 51 species, distributed among four genera: Neotanais Beddard, 1886, Herpotanais Wolff, 1956, Carololangia Gardiner, 1975, and Venusticrus Gardiner, 1975. That means that, out of the 36 extant tanaidacean families currently recognized, neotanaids hold place 16 regarding the number of species. Of the four genera, Neotanais, which was described as one of the first tanaidaceans ever, is the genus with the largest number of species. It covers 44 of the 51 species in the family. Herpotanais and Venusticrus include two and four species respectively, whereas Carololangia remains to this date a monotypic genus (Figure 1). In addition to the number of species and genera, the taxonomical classification of the entire family has been updated as well. It has transitioned along history from been originally grouped together with all other tanaidaceans in one family within the isopods, to being currently placed as a single family within Neotanaoidea, one of three superfamilies in the suborder Tanaidomorpha (Kakui et al., 2011).
The genus Neotanais, originally placed in the family Tanaidae, at that time part of the order Isopoda, was erected by Beddard (1886a). The same year, Norman and Stebbing (1886) described three species, assigned to the genus Alaotanais (Norman and Stebbing, 1886), and placed them within Tanaidae as well. They considered Isopoda to be a subclass rather than an order though, indicating a lack of consensus for higher taxonomic ranks in the group back then. Genus Alaotanais was later synonymized with Neotanais, since Neotanais serratispinosus, one of the three species described by Norman and Stebbing (1886), was declared a junior synonym of N. americanus, the first species introduced by Beddard (Gardiner, 1975). In 1895, Tanaidacea appeared on a publication for the first time as a name with the rank of order (Hansen, 1895). Later, Hansen (1913) reported on the Danish Ingolf Expedition, which explored Greenland, Iceland, and the Faroe Islands waters, and recorded the collection of two neotanaid species, one of them previously undescribed. Nevertheless, Hansen did not yet deem necessary to establish a new family for the genus. That happened only after Lang studied details in antennules, antennae, and mouth parts of a few Neotanais species (Lang, 1956). He recognized the similarities and differences to other tanaidaceans and proposed a new system for the order, dividing it into five families (two of them new) separated in two suborders, based on the morphology of cephalothorax appendages, the number of oostegites in the marsupium, and the genital cones in males. Neotanaidae was accepted as a distinct group, at an intermediary position between what were at the time the families Apseudidae, Tanaidae and Paratanaidae. The particular placement of neotanaids among them, however, varied depending on the weight attributed by the different authors to the chosen set of morphological characters (Lauterbach, 1970; Gardiner, 1975; Sieg, 1980a; Sieg, 1984). Eventually, Sieg (1980b) found the differences between families larger than previously considered and replaced Lang’s two-suborder system with a classification consisting of four suborders, one of them being Neotanaidomorpha, elevating thus the rank for neotanaids. A revision of fossil tanaidaceans led to the addition of a fifth, extinct, superfamily (Schram et al., 1986). Suborder Neotanaidomorpha, however, suffered no change, until the taxonomic relationships within the order Tanaidacea were analyzed using molecular methods (Kakui et al., 2011). These phylogenetic analyses revealed that the relationships between some of the previously established suborders are closer than suggested by their ranks. These associations were supported by morphological characters as well, which warranted an amendment of the previous five-suborder classification. The classification established by Kakui et al. (2011) for extant tanaidaceans divides the group into two suborders, Apseudomorpha and Tanaidomorpha, which encompass apseudids, tanaids, paratanaids and neotanaids, accepted currently as superfamilies. Neotanaids are placed within suborder Tanaidomorpha as a superfamily composed of a single family. Some claim the suborder rank is still under debate (Larsen et al., 2015), nevertheless the two-suborder classification system for extant species is accepted as the current valid system at the World Register of Marine Species (WoRMS, http://www.marinespecies.org, last visited 02 Feb 2024). According to the most updated classification, Neotanaoidea is the only of the four superfamilies within Tanaidacea composed solely of deep-sea species. This makes it an important key for resolving the puzzle of colonization, diversity, and evolution of invertebrates in the ocean depths.
The genera within Neotanaidae can be distinguished by morphological characters, including the shape and size of pereopods and uropods, together with their setation or spines, the number of pleopod rami, the geometry of the pleoteson, or the number of articles for the antennae (Figure 1).
Gardiner (1975) established a key for the genera, although Araújo-Silva et al. (2015) provided a re-diagnosis for Venusticrus when describing V. thor and reassigning Neotanais rotermundiae Gardiner, 1975 to this genus as well. On the other hand, Larsen (1999) produced a key for the 30 species for which the female was known, out of the 37 species described at the time. The differences between species can be subtle and elusive. Rather than by a particular character, most species are identified by a combination, or a set of morphological features. Furthermore, identification can be challenging due to marked sexual dimorphism, differences among developmental (ontogenetic) stages, and the fact that deep-sea collections are usually low in numbers and in many cases one of the sexes or some stages remain unknown. Neotanaid species, similar to other deep-sea tanaidacenas, are usually described based on the female, since they are more common, and for more than a dozen the males are unknown. However, the opposite is true for N. magnificus Kudinova-Pasternak, 1972 and N. mesostenoceps Gardiner, 1975. They were described on the basis of a male specimen and the females remain unknown. In some cases, not all developmental stages are known either. The most notable example is N. hessleri Gardiner, 1975, known only from two mancas. These facts deliver additional compelling arguments for an integrative approach when tackling the study of the biodiversity, taxonomy, and ecology of the group, for which molecular data will be necessary. However, DNA fragments are available so far only for two of the 51 neotanaid species (GenBank, www.ncbi.nlm.nih.gov/genbank/, last visited 2 Feb 2024). Furthermore, only one of them, Venusticrus thor Araújo-Silva and Larsen, 2015, has been identified to specific level. The second taxon for which DNA sequences are available was simply identified as Neotanais sp. Additionally, one transcriptome is available for a taxon identified as Neotanais cf. kuroshio, included in a study on the silk proteins of tanaidaceans (Kakui et al., 2021).
Here we initiate an integrative-approach study of the singular deep-sea superfamily Neotanaoidea. Initially we analyze available records for occurrences of neotanaids worldwide to compare their distribution among bathyal and hadal oceanic biogeographic provinces. In addition, we are building the first molecular phylogeny for neotanaids, using material collected relatively recently and preserved in a way that allows for nucleic acids extraction and sequencing, in order to scope the magnitude of genetic diversity and connectivity across oceanic regions and ocean depths.
2 Materials and methods
In future mentions the following abbreviations will be used for DNA fragments: COI and H3 for the mitochondrial cytochrome c oxidase subunit I and the nuclear histone subunit 3 genes respectively, and 16s, 18s, and 28s for the mitochondrial 16s rRNA, and the nuclear 18s and 28s rRNA genes.
For the sampling campaigns from which the sequenceable material was obtained we will use in figures and tables the following abbreviations:
AleB: AleutBio, in 2022, aboard the RV Sonne along the Aleutian Trench, led by the Senckenberg Gesellschaft für Naturforschung, Germany.
Div3: Diva3, in 2009, latitudinal gradients of deep-sea biodiversity in the Atlantic, aboard the RV Meteor, German Centre of Marine Biodiversity (DZMB).
IceA: IceAge, Icelandic marine animals: genetics and ecology, in 2011, aboard the RV Meteor, German Centre of Marine Biodiversity (DZMB).
JPIO: JPI Oceans Ecological Aspects of Deep-Sea Mining, in 2015, aboard the RV Sonne at the Mn-nodule belt in the Clarion-Clipperton Zone (CCZ), Joint Programming Initiative Healthy and Productive Seas and Oceans.
KaD: KanaDeep, in 2019 aboard L’Atalante, off New Caledonia, French National Museum of Natural History (MNHN) and Research Institute for Development (IRD).
KuB: KuramBio, Kuril-Kamchatka Biodiversity studies, in 2012 and 2016, aboard the RV Sonne, German Ministry for Science and Education (BMBF) and Russian Scientific Foundation.
Saya: MEIO1-Saya, Monaco Explorations Indian Ocean Expedition, Saya de Malha ecosystem, in 2022 aboard the SA Agulhas.
SoB: SokhoBio, Sea of Okhost expedition, in 2015, aboard the Akademik M.A. Lavrentyev, German Ministry for Science and Education (BMBF) and Russian Scientific Foundation.
VT: Vema-TRANSIT, in Dec 2014 – Jan 2015, aboard the RV Sonne, in the Vema Fracture are and the Puerto Rican Trench, GEOMAR Helmholtz-Zentrum für Ozeanforschung, Germany.
The abbreviations used for sampling gear are:
AGT: Agassiz Trawl.
BC: box corer.
EBS: epibenthic sledge.
2.1 Analyses of the geographical and depth distributions for neotanaids
Data on occurrences for geographical distribution and depth for neotanaids were downloaded from OBIS (Ocean Biodiversity Information System, obis.org, March 2023). We checked for duplicates, updated the list with the most recently described species, and completed the information, when possible, with records from the literature. Most records correspond to the original species descriptions, and nearly half of the species have been reported only for the type location. Other entries can be traced back to digitally available museum collections, such as those for the natural history museums in London or the Smithsonian Institution in Washington DC. The information shown reflects only the occurrences for taxa identified to species level (Figures 2, 3). Out of the 635 records, about 70 occurrences correspond to unidentified neotanaids, simply identified as “Neotanais sp.” or “Neotanaidae”. Although only records from digitalized collections and published occurrences were available to us, the available data shed some light on the known geographical and depth distribution for members of the family.
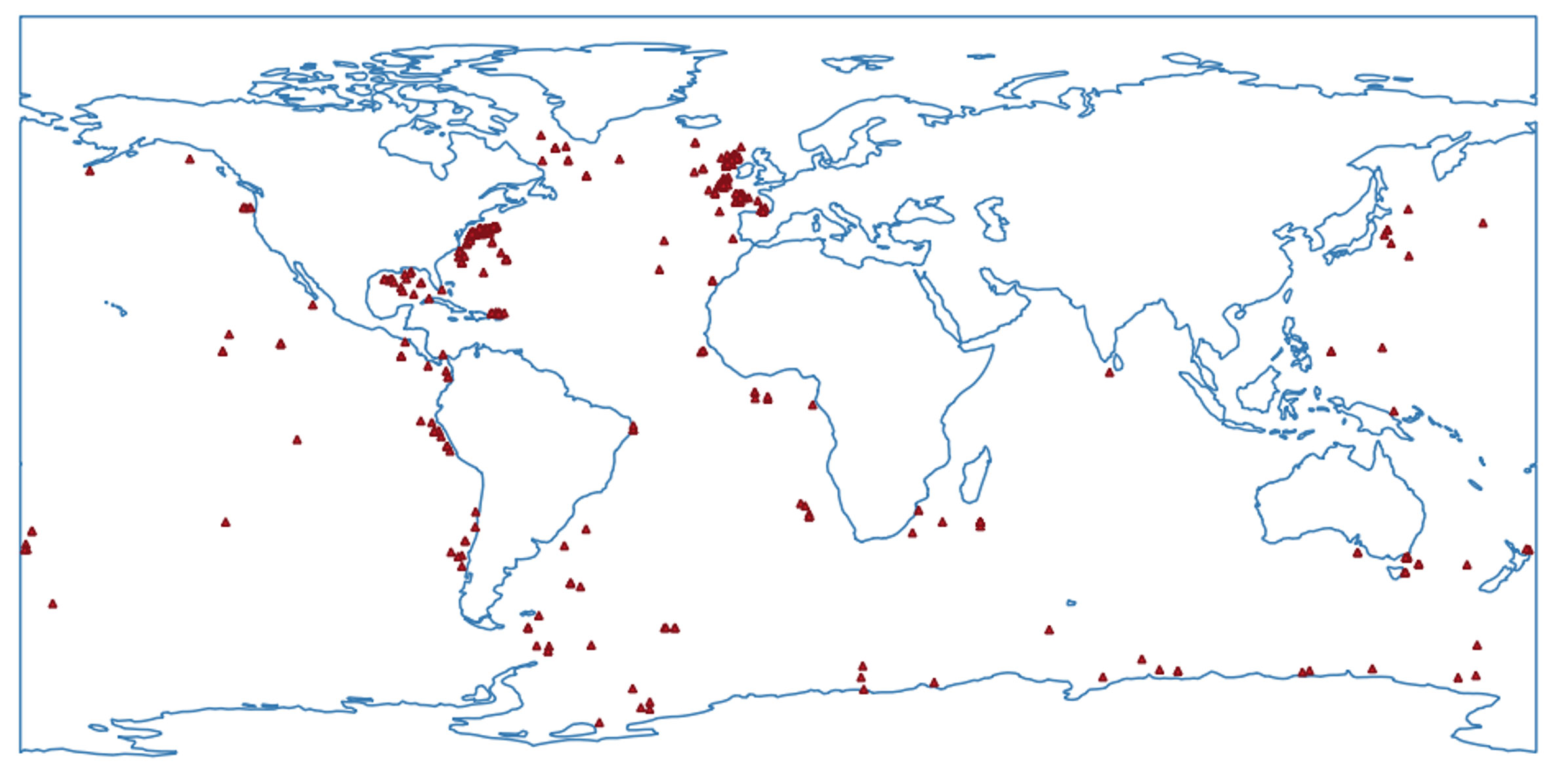
Figure 2 Map of the available occurrences for samples of Neotanaidae identified to the level of species, obtained from the Ocean Biodiversity Information System (www.obis.org) and curated to eliminate duplicates, and to add data from recent species descriptions and digitally available museum collections.
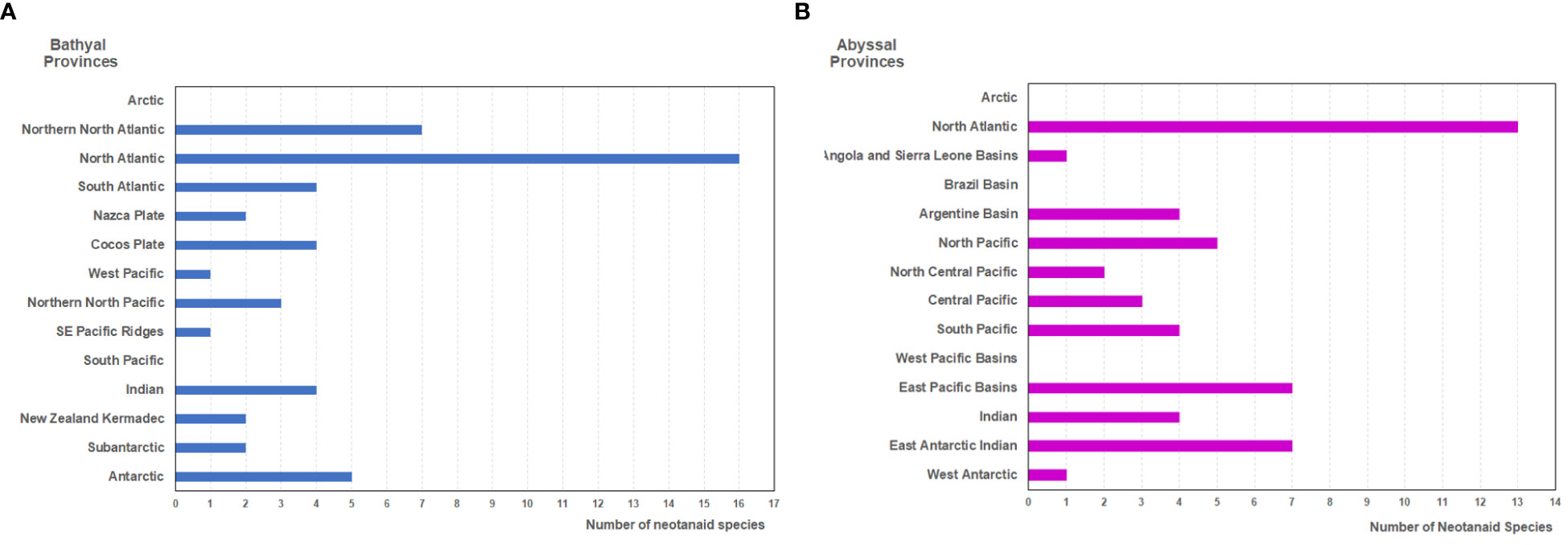
Figure 3 Number of neotanaid species in the biogeographic provinces of the deep ocean floor (as proposed by Watling et al., 2013) for (A) bathyal, and (B) hadal provinces.
The distribution of neotanaid records was evaluated in the frame of the biogeographic provinces proposed by Watling et al. (2013) for the ocean floor. In order to do that, two layers with biogeographic provinces, kindly provided by Les Watling, were mapped together with the layer containing the occurrences for neotanaids and analyzed using QGIS 3.22.14 (newer versions available at http://qgis.org, last checked 31 Jan 2024). When defining these biogeographic provinces, Watling et al. (2013) excluded from their analyses regions within economic exclusive zones (EEZs) by discarding bathymetric data from layers above 800 m depth. For the evaluation of the neotanaid occurrences studied here it means that only 15 points could not be assigned to one of the provinces as described by Watling. Nevertheless, most of them belong to locations within the Gulf of Mexico or off Antarctica, and all of them could be manually assigned to a region. The layers provided did not include hadal provinces, since for these Watling et al. (2013) adopted the ones proposed by Belyaev (1989). For our case in particular, this had very little impact on the analyses of species among provinces again, since only 18 of the records were located in hadal zones, most of which could be easily attributed to well-known deep-sea trenches.
2.2 Molecular data
A collection of more than 320 neotanaid samples from all over the world were available to us at the University of Lodz, gathered from sampling campaigns carried out in the last 15 years, or from museum loans. The samples were visually examined and for every project they were roughly divided into morphogroups, for which sequencing attempts were made, targeting both mitochondrial (16s, COI) and nuclear markers (18s, 28s, H3). Tentative identifications of the specimens to species were made, however, are not included here. Additional morphological studies, beyond the scope of the current work, are ongoing, aiming at refining valid diagnostic characters and apomorphies to characterize potential new genera, and in some cases, when the available number of specimens allows, including morphometrics and species delimitation analyses, in order to distinguish members of species complexes. For the purposes of the present work, specimens used for molecular studies are identified to the level of genus, and given numeric identifications in combination with a code assigning them to the corresponding sampling campaign. Two of the analyzed specimens were loaned from the Victoria Museum in Melbourne, Australia, and are labelled “aus”. When necessary, also haplotypes are properly identified. This will make it possible to track the specimens used in this study, if future investigations should so require (Table 1; Figure 4; Supplementary Figures S1–S4). Genomic DNA was extracted using the Sherlock AX kit for DNA purification (A&A Biotechnology, Gdynia, Poland), following the instructions indicated for fresh tissue, although adding a step to re-hydrate the tissue in sterile water, before the lysis step. If the specimens were small (up to 1-2 mm), the whole animal was used in the extractions, but if the specimen was large enough and both chelipeds were available, one of them was dissected and used in the extraction. In some cases, if parts of the specimen were already damaged or detached, they were used for the DNA extraction instead. When possible, the specimens or the parts used in the extractions were recovered after the lysis step for future potential morphological analyses. The isolation protocol was slightly modified to increase the yield of extracted DNA: after the washing steps, when mixing the filtrates with DNA with the isopropanol solution, instead of continuing right away with the next step, the mix was incubated at -20°C for a few hours (usually overnight). The DNA was resuspended in the TE buffer provided with the kit.
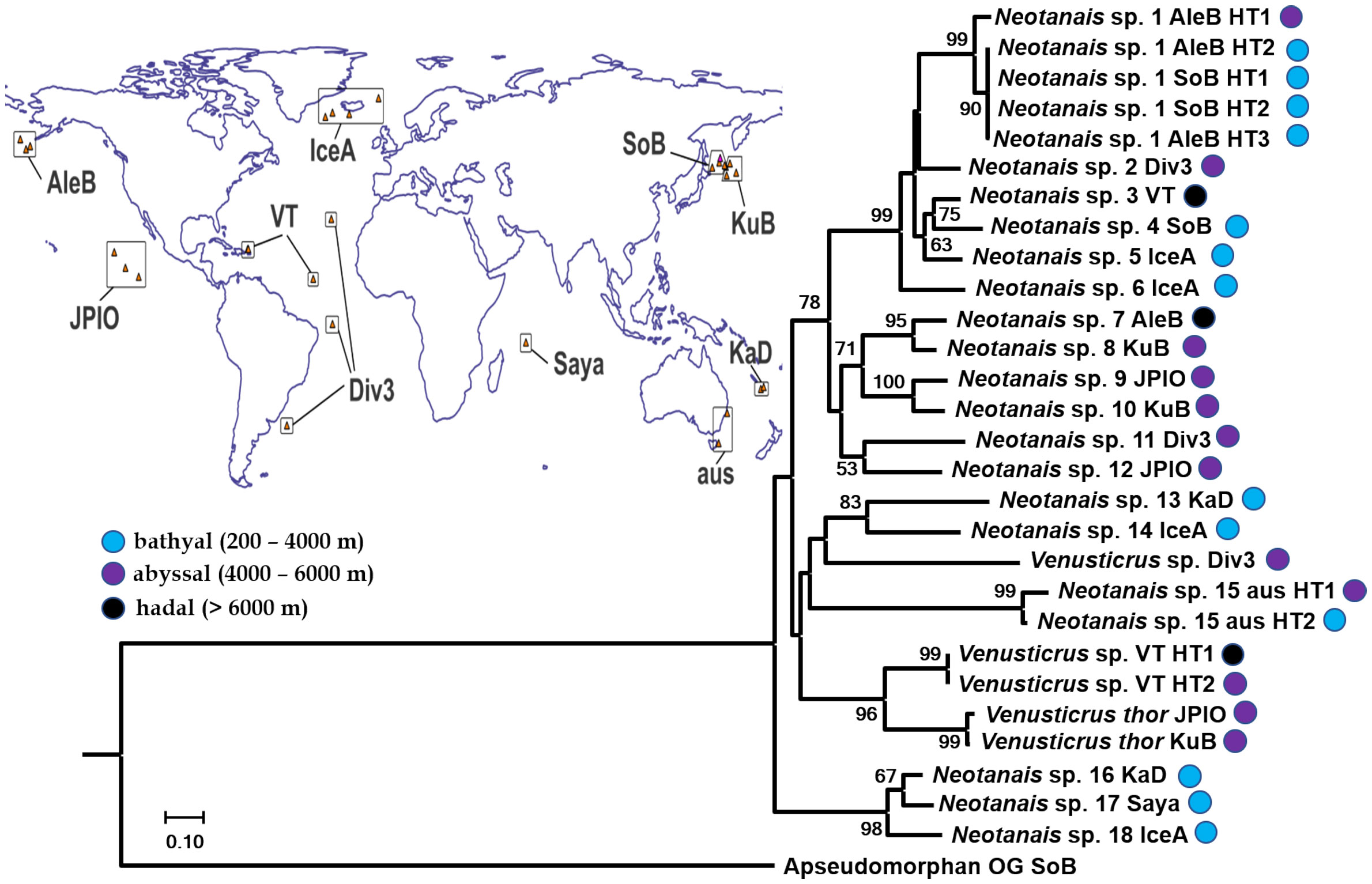
Figure 4 Phylogeny of Neotanaoidea (best tree) inferred from Maximum Likelihood analysis (RAxML) of concatenated 1360 bp long sequences including fragments of the mitochondrial COI (656 bp) and 16S (375 bp), and the nuclear H3 (329 bp) genes. Bootstrap values (5000 bp with bootstopping) are shown when higher than 50%. Taxa are identified to the level of genus, to species for Venusticrus thor, the only neotanaid species with genetic data previously available on GenBank. For tracking down of the corresponding specimens, the abbreviation of the corresponding sampling campaign or museum lending the material (see Material and Methods) is added. When necessary, the haplotypes are identified with the abbreviation HT and assigning them a number. Locations for the sampling campaigns are indicated on the included world map.
Polymerase chain reactions (PCRs) were performed following the recommendations of the polymerase’s manufacturer (DreamTaqTM Green DNA polymerase, Thermo-Fisher Scientific, Waltham, MA, USA, and AccuStart II PCR SuperMix, QuantaBio, Beverly, MA, USA). The fragments targeted were the mitochondrial COI and 16s, as well as the nuclear 18s, 28s and H3 genes. For COI we used primers LCOI-1490 and HCOI-2198 (Folmer et al., 1994), and their degenerated forms designed by Astrin and Stüben (2008) (LCOI1490-JJ and HCOI2198-JJ), and by Lobo et al. (2013) (LoboF1 and LoboR1). For 16s we used primers Ft_amp and Rt2_amp, designed for amphipods (Lörz et al., 2018), but that have proven successful also for their use with neotanaids. Some fragments for 18s, 28s, and H3 could be obtained using primers 18s 4F and 18s 2R (Pitz and Sierwald, 2010) or SSU_F04 and SSU_R22 (Blaxter et al., 1998), 28sA and 28sB (Taylor et al., 1999), and Hex3AF and Hex3AR (Svenson and Whiting, 2004) respectively. The PCR conditions for amplifying COI and 28s sequences followed the protocol indicated by Hou et al. (2007) for COI. The settings for 16s and H3 fragments included an initial 5 min activation at 95°C, followed by 36 cycles of 30 s at 95°C, 60 s at 48°C, 60 s at 72°C, and a final 5 min extension at 72°C. The PCR conditions for 18s were similar to those for 16s, but with an annealing temperature of 54°C and an extension time of 120 s. A 2-μl aliquot of the PCR product was visualized in a Midori Green-stained (Nippon Genetics) 1.5% -agarose gel to verify its quality and length and consequently 5 μl of PCR products were cleaned using a combination of 0.1 μl Exonuclease I (0.1 μl) and 1 μl Fast Polar-BAP Thermosensitive Alkaline Phosphatase (EURx Sp.z o.o., Gdansk, Poland), diluted with 1.9 μl water for reactions following a standard protocol including 15 min incubation at 37°C and 15 min inactivation at 85°C. The cleaned PCR products with the appropriate primers were sent for sequencing at Macrogen Europe (Amsterdam, Netherlands). The obtained sequences were assembled and manually edited, when necessary, with Geneious Prime® 2023.2.1 (Biomatters Ltd., Boston, MA, USA). To reduce the chances of including pseudogenes or contaminated fragments in the analyses, the obtained sequences were compared with those available on GenBank using the BLASTn suite (Basic Local Alignment Search Tool, Altschul et al. 1990). Preliminary alignments for the 16s, COI and H3 sequences were assembled, including the apseudomorphan Carpoapseudes varindex Bamber, 2007 as an outgroup, and subsequently concatenated into one final alignment with BioEdit 7.1.3.0 (Hall, 1999). An additional concatenated alignment was built with the two mitochondrial genes. The number of sequences successfully obtained for the other two nuclear genes, 18s and 28s, was very low, and were therefore not included in the concatenated alignment. Nevertheless, the combinations of 16s, COI and H3, and of 16s and COI, have shown enough resolution for the identification of neotanaids to specific level, with the possibility for further studies on the relationships among neotanaids in the future, when more sequences will be available for comparison and additional taxa can be included in the analyses.
Prior to the concatenation, the 16s alignment was tested for accuracy with MUSCLE (Multiple Sequence Comparison by Log-Expectation; Edgar, 2004) using the online tool made available by the European Bioinformatics Institute (www.ebi.ac.uk, last visited 29th Jan 2024). Poorly aligned positions were identified with Gblocks v. 0.91b (Castresana, 2000) using the online tool on http://www.phylogeny.fr/index.cgi (Dereeper et al., 2008, last visited 29th Jan 2024) allowing all three categories for choosing a less stringent selection. This was not necessary for the COI or the H3 alignments, as the alignments had no gaps.
The resulting concatenated alignment had a total sequence length of 1357 bp, for which 656 bp corresponded to COI, 375 bp to 16s and 329 bp to H3. This alignment, the concatenated alignment of both mitochondrial genes, as well as the alignments for the individual genes were submitted to the Cyberinfrastructure for Phylogenetic Research (CIPRES) web portal (www.phylo.org, last visited 19th Nov 2023) for Model test of the individual gene alignments (jModelTest2 on XSEDE, Darriba et al., 2012) and Randomized Accelerated Maximum Likelihood (RAxML) analyses (Stamatakis et al., 2008) using the RAxML-NG tool with 5000 bootstraps, although using autoMRE with a cutoff of 0.3 for bootstopping, choosing the “All-in-One” option, indicating the outgroup and the partition. The best models resulting from the model tests, and subsequently indicated in the partition, were HKY+I+G for COI, TIM2+G for 16s and K80+I+G for H3. The resulting trees from the RAxML analyses were visualized and analyzed with Mega 11.0.11 (Tamura et al., 2021). The obtained tree files were edited with Microsoft PowerPoint (Microsoft Office 2019) and Corel Photo-Paint (Home & Student 2018, v. 20.0.0.633). The fragments resulting from these Sanger sequencing efforts have been accessioned to GenBank (Table 1). The corresponding sequences will be updated in a near future in order to add the identification of the specimens to species, once associated morphological and morphometric studies, discussed in upcoming papers, make it possible. Furthermore, the studied specimens will be deposited at a museum, the Senckenberg Museum in Germany for samples associated with German projects or the National Museum of Natural History in France for samples from projects funded by French and Monegasque agencies, where they will be properly catalogued and linked accordingly to their GenBank accession numbers.
In addition to the phylogenetic analyses, genetic distances were calculated for the COI sequences. The fragments were trimmed to the length of the shortest sequence (507 bp) and the genetic distances were obtained using DNADIST version 3.5c (copyright 1986-1993 by Joseph Felsenstein and the University of Washington) on BioEdit 7.1.3.0 (Hall, 1999).
3 Results
3.1 Biogeographic patterns
The map (Figure 2) shows the world distribution of the current records for neotanaid samples. We have counted a total of 565 individual occurrences for neotanaid samples identified to species. Of these occurrences, 410 are located in the North Atlantic.
Mapping the occurrences for neotanaid species together with the layers provided by Watling et al. (2013) with the bathyal and abyssal biogeographic provinces for the deep-sea floor allowed us to assign a province to a large majority of our records. Of the 565 individual records, only 36 did not match any of these bathyal or abyssal biogeographic provinces. These 36 records correspond for the most part to locations where neotanaids were collected from waters 6156 m deep or more (18 occurrences) and from waters 676 m deep or less (15 occurrences). The records from the deepest waters represent collections resulting from the exploration of deep-sea trenches (Puerto Rican trench 11 records, Kermadec Trench three records, South Sandwich Trench two records, Mariana Trench one record, all deeper than 7150 m) and from the North Pacific, off Honshu, Japan (one record). The occurrences from shallower waters were recorded in the Gulf of Mexico (six records), the Antarctic (four records), off the coast of Pernambuco, Recife (three records), the straits of Florida (one record) and off Valentia, southwest of Ireland (one record). In addition, three occurrences did not fall into any of biogeographic provinces as described by Watling et al. (2013), although their depths were within the bathyal or the abyssal range: one location off the Falkland Islands at -1105 m, one off Antarctica at -2920 m, and one location west of Nicaragua at -3777 – -3950 m.
Neotanaids have been recorded in all but in two of the 14 bathyal biogeographic provinces (Figure 3A). Figure 3 shows the number of neotanaid species present in each of these provinces. The North Atlantic is, with 16 species, the province that hosts the most species, followed by far by the Northern North Atlantic, where seven neotanaid species are present. In a bathyal province usually two to five species can be found. In the West Pacific province and the south east Pacific ridges, on the other hand, only one neotanaid taxon is present, whereas in the Arctic and the South Pacific provinces, no neotanaid has been recorded.
The distribution of neotanaid species among the 14 abyssal biogeographic provinces (Figure 3B) shows to some extent a similar picture. The North Atlantic province displays again the highest diversity in terms of number of neotanaid species. Whereas the highest number for neotanaid species present in an abyssal province is four or five, the North Atlantic hosts 13 species. It is followed in numbers by the East Pacific Basins with seven. For the abyssal provinces, the Antarctic is divided into East Antarctic Indian and West Antarctic. While the East Antarctic Indian province shows also a high diversity with seven neotanaid species, only one can be found in the West Antarctic. Similar as for bathyal provinces, in the case of the abyssal biogeographic provinces, no neotanaid is reported for the Arctic. Neither for the Brazil or the West Pacific Basins could any collection occurrences be found.
When considering the distribution of neotanaids among the biogeographic provinces, we observed that most species have been found in only one province, no matter if deep-bathyal, abyssal, hadal, or shallower than 800 m (Figure 5). Of the 51 known species, 24 have been reported in only one province, while only six species have been reported for five or more provinces. The most widespread neotanaid species in terms of their presence in a variety of biogeographic provinces are Neotanais americanus and Neotanais armiger Wolff, 1956. They are present in eight provinces, when adding together their distribution considering depth as well as coordinates.
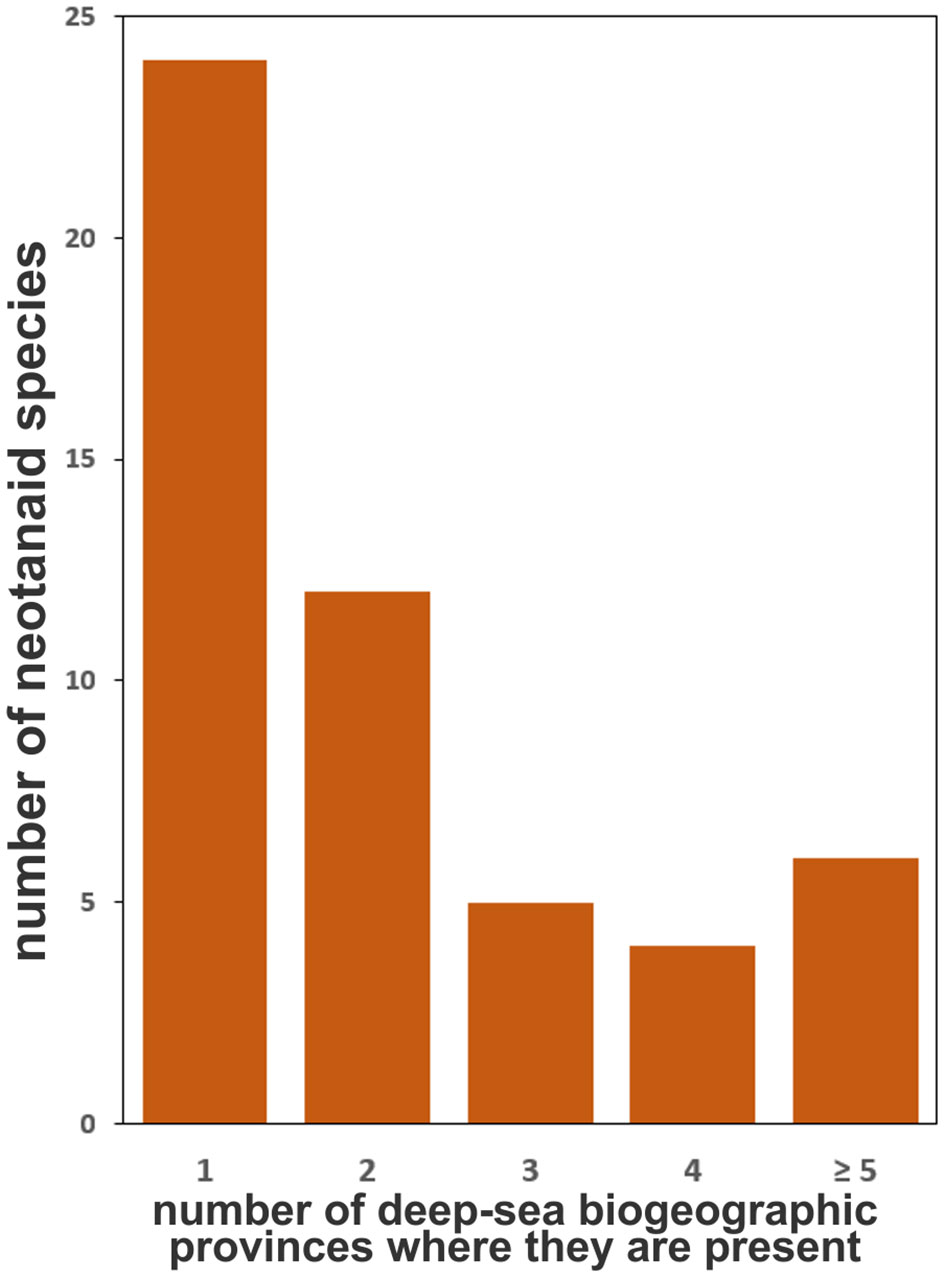
Figure 5 Number of the 51 current neotanaid species that are present in one or two biogeographic provinces of the deep ocean floor (as proposed by Watling et al., 2003) and number of species that are more widespread (present in 3, 4, or more provinces).
3.2 Phylogenetics
A total of 69 neotanaid specimens were considered for DNA extraction. When available, adults were used, although in some cases juvenile mancas were subjected to molecular analyses for the purpose of confirming identification, or to extend the geographical coverage of the study. In 13 cases out of these 69 DNA extractions no DNA fragment could be successfully obtained. The most successful were PCRs targeting 16s, for which high-quality fragments were obtained for 44 specimens, followed by COI with 39 fragments, and H3 with 30 fragments. For 18s and 28s only 13 and 19 high-quality sequences respectively were obtained. In the final alignments, only sequences representing unique haplotypes were included. In addition, only those sequences were taken into account for which at least two of the three genes were available, so that robust comparisons between the different alignments were possible. That resulted in a final number of 28 sequences included in the alignments for COI, 27 sequences for 16s, and 16 sequences for H3 (Table 1).
Of these 28 studied specimens, five were identified as belonging to the genus Venusticrus and all others to Neotanais. No representatives of Herpotanais were available to us, and, although we had access to catalogued samples of Carololangia plumata (Kudinova-Pasternak, 1975) from the Natural History Museum in London and the National Museum of Natural History in Paris, several PCR attempts failed and no quality molecular data could be produced for this species. After comparison of the obtained sequences with the publicly available fragments on GenBank, two of the specimens could be identified as Venusticrus thor, which was in agreement with the tentative morphological identification.
None of the five phylogenies built, based on the analyses with the two different combinations of genes (concatenation of COI, 16s and H3, Figure 4, and concatenation of COI and 16s, Supplementary Figure S1) or on the alignments of the individual genes (Supplementary Figures S2–S4 for COI, 16s, and H3 in that order), resulted in either of the two represented genera showing as monophyletic. In the case of Venusticrus, of the three included species only two, Venusticrus thor and Venusticrus species from the North Atlantic, grouped together as a highly supported clade (no support in the COI phylogeny, Supplementary Figure S2), whereas the Venusticrus sp. from the Atlantic tropical area, just south of the Equator, is included in clades with Neotanais species, although never with any relevant support values. Regarding the genus Neotanais, the included specimens grouped into up to five well supported clades, the largest one including Neotanais sp. 1–6, always with very high support values (94% or higher), followed in size by a clade formed by Neotanais sp. 7 – 10, although this one with lower support values (no higher than 72%). Neotanais sp. 11 and Neotanais sp. 12 always grouped together, but with low support (no higher than 58%), same for Neotanais sp. 13 and Neotanais sp. 14, in this case with relatively high support values (as high as 86%). Additionally, Neotanais sp. 16–18 built also a highly supported clade. On the other hand, Neotanais sp. 15, represented by two specimens loaned from the Museum Victoria in Melbourne, Australia, was never included in a highly supported clade grouping it to any other neotanaid species included in the analyses.
The two phylogenies based on the two concatenated alignments, one including only the mitochondrial genes and the other one analyzing additionally the available H3 fragments, were very similar, showing the same major clade formation, and differing mainly in the support values and the genetic distances. In general, the built clades showed slightly better support in the phylogeny based on all three genes than when dropping H3. The same tendency is observed when comparing to the phylogeny based on COI only: similar clades were built, although with, once again, slightly lower support values. In some cases, the internal clade formation of the major six clades changed to a small extent. However, for most instances, those internal clades showed low support values. Likewise, the phylogeny based on 16s only, showed a similar main clade formation. In this case, however, some more obvious differences were observed. Unlike in the previous tree, in the 16s phylogeny Neotanais sp. 7-8 and Neotanais sp. 9-10 did not group together into a well-supported clade, but split instead into the two internal clades, both with high support values. Additionally, and since no 16s fragment was available for Neotanais sp. 12, the corresponding clade formation with Neotanais sp. 11 resolved in the other phylogenies could not be analyzed here. The phylogeny built based on H3 only revealed a low resolution power for this gene. Only three clades grouping different species together showed support values higher than 50%, the highest been for a clade formed by the two north Atlantic species of Venusticrus.
3.3 Phylogenetics within biogeographic and bathymetric frames
When examining the phylogenies built within a biogeographic frame, of the six well-supported clades observed, only one brings together species from one single ocean, namely the clade composed of Neotanais sp. 7–10, all from the Pacific, but that fit into three different biogeographic provinces according to Watling et al. (2013) distribution. Neotanais sp. 7 was collected at the Aleutian Trench from more than 6500 m deep, Neotanais sp. 8 and 10 in the abyssal North Pacific, and Neotanais sp. 9 in the abyssal Central Pacific provinces. All other five highly supported clades combining different neotanaid species are grouping together specimens from distant regions, including species from different oceans.
Regarding the depths from where the specimens were collected, of the six highly supported main clades only two are formed of species from only one depth zone: the clade with only two species, Neotanais sp. 11 and 12, encompasses only abyssal specimens, and the clade formed of the three bathyal species Neotanais sp. 16–18. On the other hand, in the clade assembling species Neotanais sp. 1–6, we find the two extremes of Neotanais sp. 6, collected during an epibenthic sled tow starting at a depth of 1588 m and finishing at 1626 m, grouped together with Neotanais sp. 3, collected from waters deeper than 8300 m at the Puerto Rican Trench.
Table 2 shows the genetic distances for the 507 bp-long COI fragments between all 29 specimens included in the phylogenetic analyses (28 neotanaids and one apseudomorphan outgroup). The largest genetic distance between neotanaid specimens corresponds to that between Neotanais sp. 14 from Icelandic waters (IceA) and Neotanais sp. 1 haplotypes collected in the North Pacific, off Alaska (AleB), and in the Sea of Okhotsk (SoB) with 0.347, whereas the smallest genetic distances shown correspond to those between haplotypes of what are here considered specimens of the same species, Venustricus thor collected in the Kuril-Kamchatka Trench (KuB) and the Clarion-Clipperton Zone (JPIO) with 0.047, Neotanais sp. 1 haplotypes (AleutB and SoB), values between 0.00 and 0.087, Venusticrus sp. collected in the North Atlantic (VT), from the Puerto Rican Trench and the Vema Fracture with 0.002, and the two specimens from the Museum Victoria in Melbourne, with 0.0806. The smallest genetic distance observed for neotanaid specimens considered here as belonging to different species is 0.117, the distance between Neotanais sp. 9 (JPIO) and Neotanais sp. 10 (KuB).
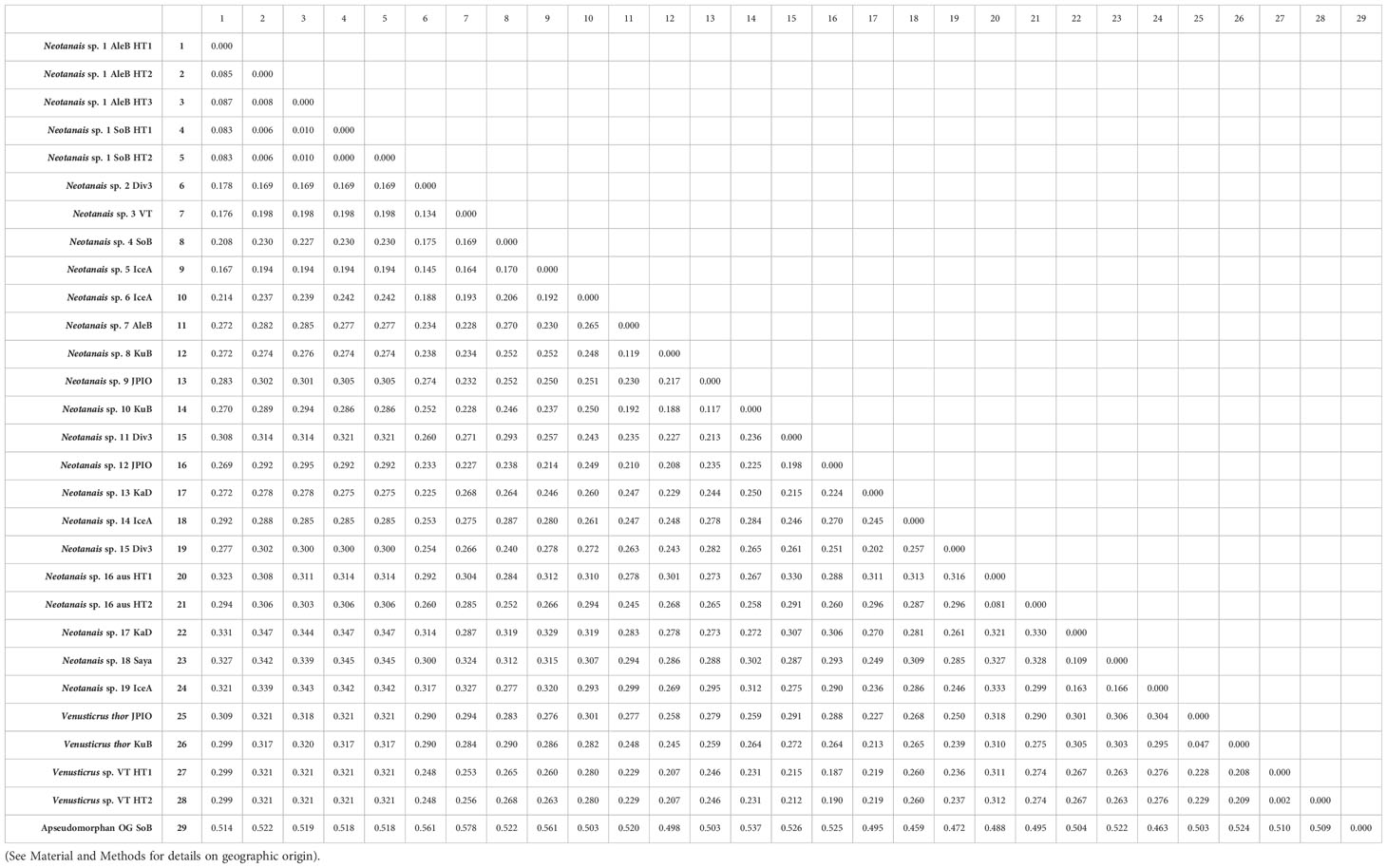
Table 2 Genetic distances matrix for COI sequences (507 bp) for the specimens included in this study.
4 Discussion and conclusions
4.1 Discovering neotanaid species
The family Neotanaidae Lang, 1956 presents a great opportunity to illustrate the exploration of the deep-sea and the study of its biodiversity. The first species of the group, Neotanais americanus Beddard, 1886, was collected in 1873 and 1876 during the historical HSM Challenger expeditions off American Atlantic coasts (Beddard, 1886a, b). Its description was based on only two specimens, two 6-mm long males, one of which was collected SE of New York, and the other off Río de la Plata. That publication was followed shortly after by the report of two more species, N. hastiger Norman and Stebbing, 1886 and N. laevispinosus Norman and Stebbing, 1886, originally described as members of the genus Alaotanais, now a junior synonym for Neotanais Beddard, 1886. Both species were collected 1869 and 1875 from Northeast Atlantic waters during British Expeditions aboard the HSM Valorous and the HSM Porcupine (Norman and Stebbing, 1886). After this promising start, however, for the next seven decades only one more neotanaid, Neotanais giganteus Hansen, 1913, was described (Hansen, 1913). Six more species were reported after a 43-years gap from the previous description (Wolff, 1956), as a result of the study of the collections from the Danish Galathea Expedition (1950–1952). Since then, however, a slow but steady trickling income of new species are being added to the family, found not only in the Atlantic Ocean but around the world, due to the increasing number of oceanic expeditions and the exploration of the deep-sea accelerating in the second half of the last century (e.g. Koslow, 2007; Thaler and Amon, 2019). The steepest increase in the number of neotanaid species up to this date took place in the 1970s, when Kudinova-Pasternak studied material collected mostly, although not exclusively, during Soviet expeditions in a variety of oceanic regions, including North Pacific bathyal provinces, the Pacific and South Atlantic abyssal zones, and specimens from the newly discovered deepest trenches and Antarctic waters (Kudinova-Pasternak, 1970; 1972; Kudinova-Pasternak 1973a; b; 1975; 1978). In addition, Gardiner published in 1975 a major revision of the family Neotanaidae, as part of his studying material collected in the 1960s aboard ships of the Woods Hole Oceanographic Institution. Gardiner (1975) erected two new neotanaid genera as well, bringing it to the current number of four. Despite the amount of information contributed up to this point, many questions on the taxonomy, systematics and ecology of the group remained open.
It was not until the beginning of this century that a new update in the study of neotanaids and their diversity was tackled. Larsen studied the deep-sea tanaids from the Gulf of Mexico, what led, among other results, to the publication of an extensive monograph on the biology, ecology and taxonomy of deep-sea tanaids (Larsen, 2005). These four authors together, Wolff, Gardiner, Kudinova-Pasternak and Larsen, are responsible for the description of two-thirds of the known 51 neotanaid species to date (Figure 1).
Here we have been able to detect at least 21 species, based mainly on molecular data, combined with morphological examinations for a limited number of specimens. The majority of them belong to already known species. Nevertheless, a few probably represent complexes of species that are similar morphologically, but separated due to ecological or biogeographic factors, such as dispersal abilities, currents, or other physical barriers. Establishing the extent of such separations will require studies including more specimens, to allow for species delimitation analyses, morphometrics, or ecological modelling. In some cases, the number of required specimens are readily available to us, whereas for other species, further sampling would be necessary. (See below, under 4.4. Genetic diversity and connectivity for neotanaids, for additional discussion points on the matter).
4.2 Ecology of neotanaids
Similarly to many deep-sea taxa, ecological information on individual neotanaid species is scarce, as many of them are collected from benthic and epibenthic dredge and sled or box core samples, mostly in low numbers, and therefore with little possibility to record information on habitat, lifestyle, feeding habits or social and reproductive behavior. Their small size limits the number of collecting devices that can be deployed. It also imposes a very restrictive approach when it comes to material handling and preservation. Therefore, most ecological data had to be inferred from morphological characteristics (e.g. the development of walking or swimming appendages, the absence of glands for the building of tubes, or the shape, size, or setation of mouth appendages) and from indirect observations, such as gut contents. Their lack of eyes clears any doubt of this family radiating in the deep-sea. So far, no neotanaid has been reported from depths above 200 m (Gardiner, 1975; Figure 1), not even in polar regions, possibly ruling out a depth distribution associated with water temperature rather than with depth. Whether or not the distribution of neotanaids is subjected to polar emergence should be investigated once sufficient data on depth, salinity, and temperature for the collection stations can be assembled. On the other end of the depth range, Neotanais hadalis Wolff, 1956 was described based on four specimens collected from a depth of 8210 m at the Kermadec Trench, northeast of New Zealand, whereas N. persephone Messing, 1977 was collected from the Puerto Rican Trench from 8381 m deep waters. These are two of the deepest records for neotanaids up to date. A few other species have been collected from waters deeper than 7000 m, however, most commonly occurrences for neotanaids are found at depths 1000–5000 m (Figure 1). Nevertheless, it should be pointed out that this might not reflect the actual depth distribution for the group, but rather indicate the allocation of sampling efforts for deep-sea expeditions.
4.3 Biogeographic distribution of neotanaids
The distribution of the occurrences for neotanaids, with an overwhelming majority located in the North Atlantic, reveals the strong asymmetry in the historic exploration of deep-sea biodiversity (Figures 2, 3). Many of these North Atlantic samples can be traced back to the activity of the Woods Hole Oceanographic Institution and the British Natural History Museum, where some of the efforts toward the study of the deep-sea were promoted with periodical transects and repeated cruises for the collections and study of material in relatively close areas off their respective coasts. It is even possible to find some of these locations labelled as “permanent station”, quotation marks included, beautifully hand-written on the pages of the paper catalogue for the crustacean collection of the British Museum of Natural History (e.g. https://data.nhm.ac.uk/media/738536fd-9ec3-426a-b477-09ef60ed1a57) Natural History Museum (2014). The North Atlantic biogeographic provinces, both bathyal and abyssal, host much larger numbers of neotanaid species than any other province, even when some other regions are better known as biodiversity hotspots (Costello et al., 2022). One could almost say that some oceanic regions seem to have been forgotten. The complete lack of records for neotanaids from the Arctic Ocean, or the extremely low numbers of occurrences in the South Atlantic are puzzling, indicating how perhaps more remote or hard-to-access locations remain unexplored. Since scientific exploration and knowledge go hand in hand with technological advance and economic progress, we would expect that additional regions will gain more attention in the future, especially if accompanied by an increase in international collaboration in scientific projects. Most likely, when other oceanic areas are explored to the same extent as the North Atlantic has historically been studied, the levels of biodiversity for the deep-sea will increase exponentially, probably not only for neotanaids, but for marine fauna in general.
Therefore, looking into the known distribution of neotanaids from the perspective of the individual species and how widespread they are, needs to be done with care. The examined records show that of the 51 neotanaid species, 24 can be found in only one biogeographic province (Figure 5). It is necessary to remember, however, that many of them are still known only from the type collection. According to the available records, 27 have been so far reported only in the original species description and for 15 of them only a single collection station was indicated. The study of deep-sea fauna remains in the discovery phase (e.g. Webb et al., 2010; Paulus, 2021), with large gaps and scarce data for marine invertebrates (Tittensor et al., 2010), and many deep-sea species remain undescribed (Ramírez-Llodra et al., 2010). It could be suspected that at such a young stage in the study of deep-sea fauna there will be a correlation between the number of times a taxon has been reported and the amount of time for how long that species has been known. Nevertheless, the data do not offer an unequivocal answer regarding such hypothesis. For Neotanais americanus, the species described the longest ago, there are relatively many occurrences (50). However, for N. giganteus, described already in 1913, we have only 10 records. Whether the differences among the occurrences for neotanaid species can be explained through sample bias, or if they reflect to some extent the differences in their levels of rarity and endemism, will require a deeper knowledge on their ecology and life strategies, in addition to exploring a larger portion of the sea floor than what has been covered so far. Sampling effort, method of collections chosen in deep-sea expeditions, and definitely pure chance most likely play a part in the numbers and current known distribution for neotanaid species. The number of records for the species were also plotted against the number of biogeographic provinces in which they are present (Figure 6). The chances of a species being found in more than one region should increase if that species is recorded more often. The plot on Figure 6 shows that tendency, although there are some outliers. Neotanais giganteus and N. hadalis have been reported only 11 times each, but at locations that span over five and seven biogeographic provinces respectively. Moreover, their distributions extend across two oceans (Atlantic, Indian) for N. giganteus and three oceans (Atlantic, Antarctic, Indian) for N. hadalis. One of the other most widespread species, N. antarcticus, is, with only 12 records, present in six provinces. These are, however, all located within the Antarctic Ocean or adjacent regions (Subantartic, Falklands). The data for Carololangia plumata include 12 records distributed across six biogeographic provinces located in three oceans (Atlantic, Antarctic, Indian). However, cases in the opposite direction can also be observed, for which species with a relatively high number of occurrences show a more limited distribution: Neotanais micromopher, the neotanaid with the most occurrences reported (89), is present in three biogeographic provinces, all of them in the Atlantic Ocean. Similarly, N. peculiaris, has been reported 38 times, but always from the bathyal North Atlantic (37 records) and the bathyal Northern North Atlantic (one record only) provinces.
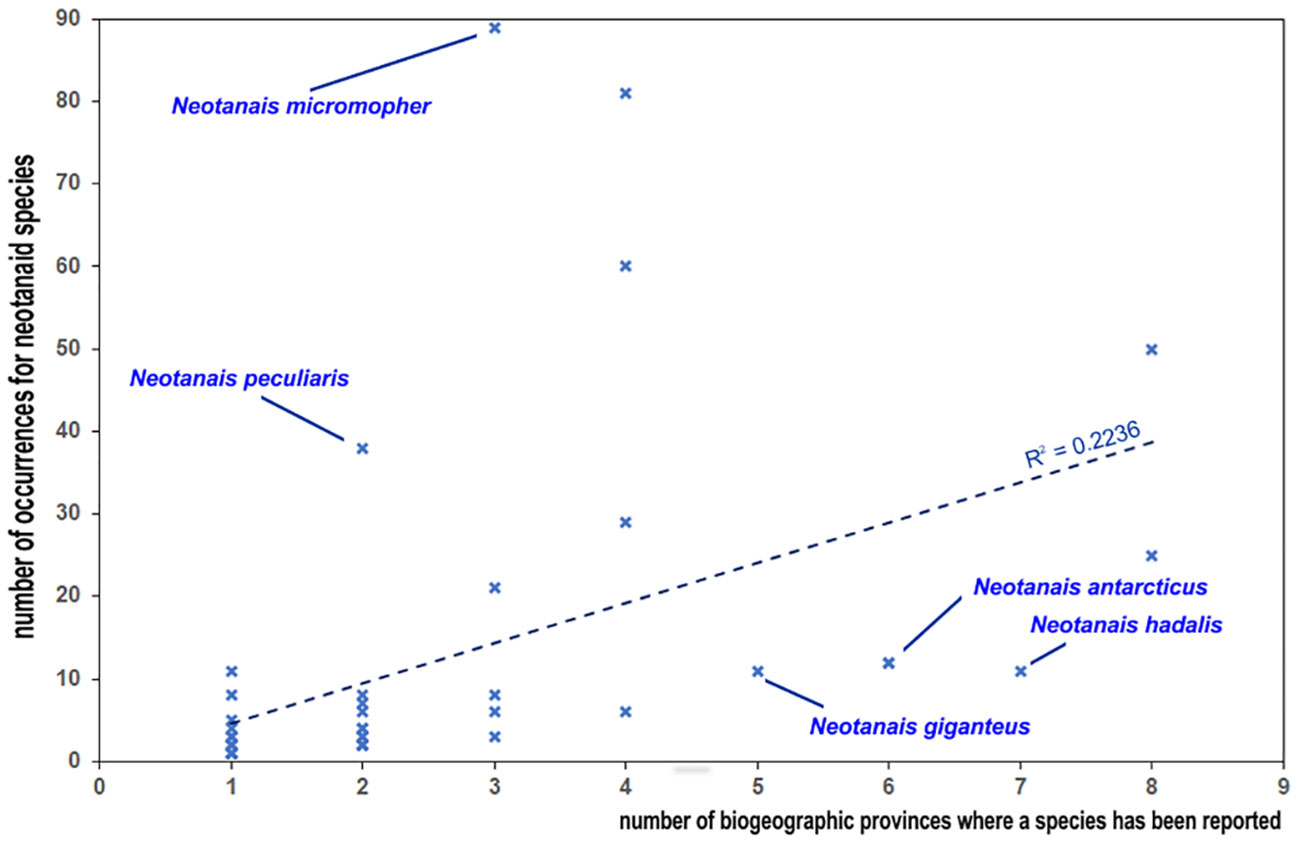
Figure 6 Plot of the relationship between the number of records for species and how widespread they are according to the number of biogeographic provinces where they have been recorded.
Additional data and studies are necessary to separate sampling artifacts from evolutionary and ecological factors that can help us explain neotanaid biodiversity and distribution. One of those factors will be certainly depth. The number of provinces in which a neotanaid species is present will depend to some extent on its depth range and whether or not it is capable of occupying habitats in different zones. The depth ranges for neotanaids (Figure 1) show a rather large variability, with some species displaying a range restricted to the bathyal zone, but others having a very wide range, reaching from the upper-bathyal into the abyssal zones, some even into hadal depths. The two species with a widest depth range are N. armiger, with depths records from 600 to 6100 m depth, and N. hadalis, with records between 2270 and 8120 m. Such wide depth ranges and widespread distributions are unusual for marine fauna with limited dispersal abilities, as is the case for neotanaids with their lifestyle of brooding and walking benthic organisms. Further studies of already existing as well as of new collections will most probably lead to the description of new species, as some of those wide ranges and distributions could be explained by the fact that they might not be a single species, but rather a species complex of cryptic species, morphologically similar in appearance but with different physiological and ecological characters. In order to reveal these potential cryptic species, specimens with similar morphologies, but from different depths need to be included in species delimitation analyses. Ideally, these should be complemented with morphometric studies. Of special interests are the species whose depth ranges span over both, bathyal and abyssal depths. A high species turnover characterizes the transition between bathyal and abyssal fauna, related to a physiological bottleneck that requires adaptation for species to adapt to higher hydrostatic pressure (Brown and Thatje, 2014). These adaptations have been associated with the ability of membrane-folding proteins to withstand the increasing loss of membrane fluidity with increasing hydrostatic pressure past that threshold (Yancey, 2020) and including specimens from both sides of that potential physiological barrier could help detect if these adaptations translate into the formation of complexes of different species separated by depth.
The discovery of such species complexes would lead to the establishment of yet-to-be described genera as well, due to both, the placement of known species into currently undescribed taxa and because of the description of genera for newly discovered species. Gardiner (1975) established already six subgroups for 23 species of Neotanais based on morphological similarities among the members of each group. Some of the species described since then show characters matching features corresponding to one of these groups (Larsen and Hansknecht, 2003; Wi et al., 2014; Wi et al., 2015). These groups are indicated on Figure 1 with an intercalated shade-light pattern superimposed on the species of genus Neotanais (yellow rectangle) labelled with their corresponding names, and to the right of these groups, the remaining ungrouped 26 species, in ordered of their depth range, from shallowest, N. minimus, to deepest, N. persephone. The features characterizing some of these groups have potential as diagnostic characters for possible undescribed genera. For example, the species in the micromopher group are distinguished by having rectangular wider-than-long pereonites 4-6, a very short pleotelson fused to pleonite 5, long, slender, ventral cheliped carpal setae, and, for the males, slender and not sharply bent chelipedal carpi (Gardiner, 1975). However, for other groups it could be problematic. For instance, the americanus group, is, in Gardiner’s own words “noted for a lack of distinctive morphologic features compared to other groups” (Gardiner, 1975; page 43). Something similar can be observed when looking into the depth ranges within the groups. In some groups, for example the americanus group, the depth ranges are quite similar for all the species, but in others, such as the hadalis group or the robustus group, there is little consistency. On the same note, the depth ranges for species within Herpotanais and Venusticrus, especially the latter, display rather similar values, indicating that this ecological character could represent further support for the morphological characters defining genera in Neotanaidae.
Additional study of these groups should reveal if such characteristics can support their elevation to genus rank. This task will be facilitated by the implementation of an integrative approach, taking advantage of morphology, physiology, ecology, and molecular data.
4.4 Genetic diversity and connectivity for neotanaids
The phylogenies built for Neotanaidae were somewhat limited in their coverage, since they only included two of the four current genera and 21 species, while there are 51 recognized species in the family. The specimens included in the phylogenetic analyses were divided into species based on a combination of criteria, including tentative morphological identifications, biogeographic distances, or the results of the molecular analyses themselves. Some of those divisions might change in the future, when more specimens are available for further research, such as species delimitation analyses, and when ongoing morphological studies can be completed. These will allow us to establish further taxonomic assignments, discern which ones are valid species, or which rather represent species complexes, in addition to detecting new species as well as potential synonymies. For example, the genetic distance between the two haplotypes of Neotanais sp. 15, from the southeast coasts of Australia (Table 2), indicates that despite their morphological and geographic similarities, they might belong into different species. Another interesting study case is offered by Neotanais sp. 1, collected in the North Pacific in both, eastern and western areas. This species belongs to the N. americanus-sandersi-laevispinosus complex, three taxa already pointed out as “sibling species” by Gardiner (1975) and whose further investigation could result in them been appointed as populations of the same species, segregated by geographic distance or possibly depth. Neotanaids are usually collected in very low numbers, which hinders population studies. In this case, however, we are fortunate to have access to material from several locations in the North Pacific, allowing for our upcoming study on the genetic connectivity of populations of this species across the region.
Despite the limited coverage of the phylogenetic analyses, we can conclude that the generic assignments within Neotanaidae is in need of a thorough revision. This was not unexpected for genus Neotanais, historically the first genus, including 44 species, a large majority of neotanaids. Nevertheless, this is striking for the second genus included in the analyses, genus Venusticrus. There are four recognized species within that genus and it has recently been the subject of a revision, including a re-diagnosis of its morphological characters, the main diagnostic trait being a uropod ventrally attached to the telson (Figure 1B), as opposed to laterally or dorsally, as in other neotanaids. We were able to include three species of Venusticrus in our alignments, and in none of the phylogenies these three species formed a monophyletic clade. One of the specimens, labelled here Venusticrus sp. Div3 and that probably belongs to V. glandurus Gardiner, 1975, the type species of the genus, was never part of a well-supported clade with any of the other species included. Morphological re-examinations will be necessary to re-evaluate which characters can represent true diagnostic features, and what weights should be attributed to them for the purpose of assigning taxonomic identities, at generic, as well at specific levels. Furthermore, additional molecular analyses should be undertaken, in an effort to increase the number of taxa included at all levels, population, species and genus, to achieve better resolution and robustness in the analyses.
The increase in the studied specimens should also aim at providing a better picture of the connectivity of neotanaids across biogeographic regions and oceans. Our molecular analyses indicate that the evolutionary processes shaping the diversity and biogeography of neotanaid species might not be as confined as initially assumed, considering the frame of a small deep-sea benthic invertebrate with, in theory, limited dispersal abilities. Our observations reveal that, at least some, neotanaid species are able to travel relatively long distances. As an example, specimens from the Aleutian Trench in the eastern East Pacific are closely related to what seem to be conspecific populations from the Sea of Okhotsk, in the western North Pacific (Figure 4, Neotanais sp. 1). A similar case can be made for samples found in the North Atlantic, where two specimens, one collected east of the Mid-Atlantic Ridge, east of the Vema fracture, and another one from the Puerto Rican Trench, display nearly identical haplotypes (Figure 4, Venusticrus VT). In the latter case, the high genetic connectivity of the populations takes place not only across large distances, overpassing a hypothetically substantial geographical barrier, but also along a wide depth gradient, as one of the samples was collected from as deep as 8340 m and the other one from about 5100 m. Such high connectivity across large distances is not observed in other deep-sea tanaidaceans. For instance, Jakiel et al. (2019) observed spatial structure of the species of family Pseudotanaidae collected in different regions of the Clarion-Cliperton Fracture Zone (CCFZ), in correlation with geographical barriers, such as fractures and seamount chains crossing the CCFZ and separating the sampled areas. Similarly, diversity studies with other peracarids also in the CCFZ, namely deep-sea isopods, showed that, although a large proportion of species have rather restricted distributions, some could be found in areas as far from each other as 5000 km (Kaiser et al., 2023 and references therein). Nevertheless, some deep-sea isopods are known to be good swimmers, and display different types of swimming behavior (see for ex. Marshall and Diebel, 1995). In fact, distribution patterns in abyssal isopods have been linked to the locomotion abilities of adult stages for four families (Brix et al., 2020). Whether or not the findings on neotanaid connectivity across biogeographic provinces and depths, can be linked as well to behavioral or physiological patterns can only be determined once enough information on their ecology is available.
From our survey of what is known so far about the distribution and biogeography of neotanaid species, together with a first phylogeny based on molecular data for the family, we have been able to gather new insights on the diversity and genetic connectivity patterns of the group. Nevertheless, we also have opened new questions that can only be adequately answered after assembling new data. Much of the sea-floor remains unexplored, with big gaps for some large and important regions. The search for scientific knowledge is motivated mostly by short- to mid-term commercial interests, and the deep-sea is no exception. The deep-sea floor is screened for economically valuable resources and new ways of gaining power, and the study of biodiversity is often simply the means to justify such enterprises or the result of a need to find an urgent solution for a problem, generated by our ways of exploiting natural resources in unsustainable ways. Many times the search for long-term effects of our actions on natural ecosystems or for a big-picture understanding of natural processes is neglected. The records or occurrences and the available samples for neotanaids are biased towards the northern hemisphere, in addition to areas that have been historically considered of economic or strategical interest. Only if we close the existing large knowledge gaps, will we be able to develop a clear picture of the processes that shape marine biodiversity and only then will we have the ability to properly protect marine ecosystems.
Data availability statement
The genetic data produced for this study have been made publicly available by accessioning them to GenBank, with the accession numbers indicated in Table 1.
Ethics statement
This study was conducted in accordance with local legislations and insitutional requirements, as part of a project approved by Poland National Science Centre. For the collection of specimens appropriate permits were issued.
Author contributions
EPT: Conceptualization, Data curation, Formal analysis, Investigation, Methodology, Software, Validation, Visualization, Writing – original draft, Writing – review & editing. MB: Funding acquisition, Project administration, Resources, Software, Supervision, Validation, Writing – review & editing.
Funding
The author(s) declare financial support was received for the research, authorship, and/or publication of this article. Major support was provided under Polish Nacional Science Centre grant BIOPASS: BIOdiversity PAtternS and Scale: the case of peracarid Crustacea from south−eastern Australia (OPUS 2018/31/B/NZ8/03198), in addition to financial support by the Dean's office of the Faculty of Biology and Environmental Protection of the University of Lodz. Frontiers has granted this paper a discount of 10% on the total invoice fee.
Acknowledgments
We would like to thank the organizing committee of the 10th International Crustacean Congress and the Crustacean Society for making such an interesting and successful meeting possible, as well as Rachael Peart and Kareen Schnabel for creating this opportunity for us to share our findings, offering their abilities, professionalism, and time as the editors of this special issue. We want to thank also the masters, nautical officers, and all crew members of the research vessels Sonne (AleutBio2022, JPIO2015, KuramBio2012 and 2016), Meteor (Diva3-2019, IceAge2011), L’Atalante (KanaDeep2019), SA Agulhas (MEIO1-Saya-2022), and Akademik M.A. Lavrentyev (SokhoBio2015), as well as the science parties involved in these expeditions for their support collecting samples. We would like to acknowledge as well the efforts of the curators of the invertebrate collections of the Natural History Museum in London, the National Museum of Natural History in Paris, the Museum Victoria in Melbourne, and the Senckenbeg Museum in Frankfurt, and their assistance with attaining further material. We would like to appreciate also the help of two rewires, whose comments and suggestions proved useful for improving the quality of the manuscript. Additionally, we need to thank our colleagues and friends in the Polarna lab at the University of Lodz for their help and support along the way, whether facilitating the sorting and preservation of material, the adjustment of PCR protocols, or the edition of text and figures, among others.
Conflict of interest
The authors declare that the research was conducted in the absence of any commercial or financial relationships that could be construed as a potential conflict of interest.
The authors declare that one of them (MB) was an editorial board member of Frontiers at the time of submission. This had no impact on the peer review process and the final decision.
Publisher’s note
All claims expressed in this article are solely those of the authors and do not necessarily represent those of their affiliated organizations, or those of the publisher, the editors and the reviewers. Any product that may be evaluated in this article, or claim that may be made by its manufacturer, is not guaranteed or endorsed by the publisher.
Supplementary material
The Supplementary Material for this article can be found online at: https://www.frontiersin.org/articles/10.3389/fmars.2024.1395000/full#supplementary-material
Supplementary Figure 1 | Phylogeny of Neotanaoidea (best tree) inferred from Maximum Likelihood analysis (RAxML) of concatenated 1031 bp long sequences including fragments of the mitochondrial COI (656 bp) and 16S (375 bp) genes. Bootstrap values (5000 bp with bootstopping) are shown when higher than 50%. Taxa are identified to the level of genus, to species for Venusticrus thor, the only neotanaid species with genetic data previously available on GenBank. For tracking down of the corresponding specimens, the abbreviation of the corresponding sampling campaign or museum lending the material (see Material and Methods) is added. When necessary, the haplotypes are identified with the abbreviation HT and assigning them a number.
Supplementary Figure 2 | Phylogeny of Neotanaoidea (best tree) inferred from Maximum Likelihood analysis (RAxML) of 656 bp long sequences of the mitochondrial COI gene. Bootstrap values (5000 bp with bootstopping) are shown when higher than 50%. Taxa are identified to the level of genus, to species for Venusticrus thor, the only neotanaid species with genetic data previously available on GenBank. For tracking down of the corresponding specimens, the abbreviation of the corresponding sampling campaign or museum lending the material (see Material and Methods) is added. When necessary, the haplotypes are identified with the abbreviation HT and assigning them a number.
Supplementary Figure 3 | Phylogeny of Neotanaoidea (best tree) inferred from Maximum Likelihood analysis (RAxML) of concatenated 375 bp long sequences of the mitochondrial 16S gene. Bootstrap values (5000 bp with bootstopping) are shown when higher than 50%. Taxa are identified to the level of genus, to species for Venusticrus thor, the only neotanaid species with genetic data previously available on GenBank. For tracking down of the corresponding specimens, the abbreviation of the corresponding sampling campaign or museum lending the material (see Material and Methods) is added. When necessary, the haplotypes are identified with the abbreviation HT and assigning them a number.
Supplementary Figure 4 | Phylogeny of Neotanaoidea (best tree) inferred from Maximum Likelihood analysis (RAxML) of concatenated 329 bp long sequences including fragments of the nuclear H3 gene. Bootstrap values (5000 bp with bootstopping) are shown when higher than 50%. Taxa are identified to the level of genus, to species for Venusticrus thor, the only neotanaid species with genetic data previously available on GenBank. For tracking down of the corresponding specimens, the abbreviation of the corresponding sampling campaign or museum lending the material (see Material and Methods) is added. When necessary, the haplotypes are identified with the abbreviation HT and assigning them a number.
References
Altschul S. F., Gish W., Miller W., Myers E. W., Lipman D. J. (1990). Basic local alignment search tool. J. Mol. Biol. 215, 403–410. doi: 10.1016/S0022-2836(05)80360-2
Araújo-Silva C. L., Froufe E., Larsen K. (2015). Two new species of family Neotanaidae (Peracarida: Tanaidacea) from the Antarctic and Mid-Pacific Oceans. Zootaxa 4018, 535–552. doi: 10.11646/zootaxa.4018.4.3
Astrin J. J., Stüben P. (2008). Phylogeny in cryptic weevils: molecules, morphology and new genera of western Palaearctic Cryotorhynchinae (Coleoptera: Curculionidae). Inv. Syst. 22, 503–522. doi: 10.1071/IS07057
Beddard F. E. (1886a). Preliminary notice of the Isopoda collected during the voyage of H.M.S. ‘Challenger.’ - Part III. Proc. Sci. Meetings Zoological Soc. London 1886, 97–122.
Beddard F. E. (1886b). “Report on the Isopoda collected by H.M.S. Challenger during the years 1873-76. Second part,” in Zoology. Report on the Scientific Results of the Voyage of H.M.S. Challenger During the Years 1873–76 Under the Command of Captain George S. Nares, R.N., F.R.S. and the Late Captain Frank Tourle Thomson, R.N. Wyville Thomson, C. and J. Murray (series eds.), vol. 17 . Ed. Murray J. (Neill and Company, Edinburgh), 1–178. Plates I–XXV, 1 chart.
Belyaev G. M. (1989). Deep Sea Ocean Trenches and their Fauna (Moscow: Nauka), 255. in Russian, translation courtesy of Scripps Institution of Oceanography Library.
Błażewicz-Paszkowycz M., Bamber R. N., Anderson G. (2012). Diversity of Tanaidacea (Crustacea: Peracarida) in the World’s Oceans – How far have we come? PloS One 7, e33068. doi: 10.1371/journal.pone.0033068
Blaxter M. L., De Ley. P., Garey J. R., Liu L. X., Scheldeman P., Vierstraete A., et al. (1998). A molecular evolutionary framework for the phylum Nematoda. Nature 392, 71–75. doi: 10.1038/32160
Brix S., Osborn K. J., Kaiser S., Truskey S. B., Schnurr S. M., Brenke N., et al. (2020). Adult life strategy affects distribution patterns in abyssal isopods – implications for conservation in Pacific nodule areas. Biogeosciences 17, 6163–6184. doi: 10.5194/bg-17-6163-2020
Brown A., Thatje S. (2014). Explaining bathymetric diversity patterns in marine benthic invertebrates and demersal fishes: physiological contributions to adaptation of life at depth. Biol. Rev. 89, 406–426. doi: 10.1111/brv.12061
Castresana J. (2000). Selection of conserved blocks from multiple alignments for their use in phylogenetic analysis. Mol. Biol. Evol. 17, 540-552. doi: 10.1093/oxfordjournals.molbev.a026334
Costello M. J., Vale M. M., Kiessling W., Maharaj S., Price J., Talukdar G. H. (2022). “Cross-chapter paper 1: biodiversity hotspots,” in Climate Change 2022: Impacts, Adaptation and Vulnerability. Contribution of Working Group II to the Sixth Assessment Report of the Intergovernmental Panel on Climate Change. Eds. Pörtner H.-O., Roberts D. C., Tignor M., Poloczanska E. S., Mintenbeck K., Alegría A., Craig M., Langsdorf S., Löschke S., Möller V., Okem A., Rama B. (Cambridge University Press, Cambridge, UK and New York, NY, USA), 2123–2161. doi: 10.1017/9781009325844.018
Darriba D., Taboada G. L., Doallo R., Posada D. (2012). jModelTest 2: more models, new heuristics and parallel computing. Nat. Methods 9, 772. doi: 10.1038/nmeth.2109
Dereeper A, Guignon V, Blanc G, Audic S, Buffet S, Chevenet F, et al. (2008). Phylogeny.fr: robust phylogenetic analysis for the non-specialist. Nucleic Acids Res. 36, W465-9. doi: 10.1093/nar/gkn180
Edgar R.C. (2004). MUSCLE: multiple sequence alignment with high accuracy and high throughput. Nucleic Acids Res. 32, 1792–1797. doi: 10.1093/nar/gkh340
Folmer O., Black M., Hoeh W., Lutz R., Vrijenhoek R. (1994). DNA primers for amplification of mitochondrial cytochrome c oxidase subunit I from diverse metazoan invertebrates. Mol Mar. Biol. Biotech. 3, 294–299.
Gardiner L. F. (1975). The systematics, postmarsupial development, and ecology of the deep-sea family Neotanaidae (Crustacea: Tanaidacea). Smithsonian Contributions to Zoology 170, 1–265. doi: 10.5479/si.00810282.170
Hall T. A. (1999). BioEdit: a user-friendly biological sequence alignment editor and analysis program for Windows 95/98/NT. Nucleic Acids Symp. Ser. 41, 95–98.
Hansen H. J. (1895). “Isopoden, Cumaceen u. Stomatopoden der Plankton-Expedition,” in Ergebnisse der Plankton-Expedition der Humboldt-Stiftung. Bd. II G.c (Kiel, Germany: Lipsius & Tischer). Hensen, V. doi: 10.5962/bhl.title.10413
Hansen H. J. (1913). Crustacea Malacostraca II. Danish Ingolf-Expedition. Available online at: https://www.biodiversitylibrary.org/item/40413 (Accessed 11th Jan 2024).
Hou Z., Fu J., Li S. (2007). A molecular phylogeny of the genus Gammarus (Crustacea: Amphipoda) based on mitochondrial and nuclear gene sequences. Mol. Phylogenet. Evol. 45, 596–611. doi: 10.1016/j.ympev.2007.06.006
Jakiel A., Palero F., Błażewicz M. (2019). Deep ocean seascape and Pseudotanaidae (Crustacea: Tanaidacea) diversity at the Clarion-Clipperton Fracture Zone. Sci. Rep. 9, 17305. doi: 10.1038/s41598-019-51434-z
Kaiser S., Christodoulou M., Janssen A., Kihara T. C., Mohrbeck I., Pasotti F., et al. (2023). Diversity, distribution and composition of abyssal benthic Isopoda in a region proposed for deep−seafloor mining of polymetallic nodules: a synthesis. Mar. Biodivers. 53, 30. doi: 10.1007/s12526-023-01335-2
Kakui K., Fleming J. F., Mori M., Fujiwara J., Arakawa K. (2021). Comprehensive transcriptome sequencing of Tanaidacea with proteomic evidences for their silk. Genome Bio. Evol. 13. doi: 10.1093/gbe/evab281
Kakui K., Katoh T., Hiruta S. F., Kobayashi N., Kajihara H. (2011). Molecular systematics of Tanaidacea (Crustacea: Peracarida) based on 18S sequence data, with an amendment of suborder/superfamily-level classification. Zoological Sci. 28, 749–757. doi: 10.2108/zsj.28.749
Koslow T. (2007). The Silent Deep: The Discovery, Ecology and Conservation of the Deep Sea (Sydney: UNSW Press), 270.
Kudinova-Pasternak R. K. (1970). Tanaidacea of the Kuril-Kamchatka trench. Trudy Instituta Okeanologii, Akademiya Nauk SSSR 86, 341–381.
Kudinova-Pasternak R. K. (1972). New species of the genus Neotanais (Crustacea, Tanaidacea). N. magnificus n. sp. from the Antarctic. Complex Res. Nat. Ocean (Moscow) 3, 259–263.
Kudinova-Pasternak R. K. (1973a). New species of the genus Herpotanais Wolff 1956 of the deep-sea family Neotanaidae (Crustacea: Tanaidacea) from the Pacific. Complex Res. Nat. Ocean (Moscow) 4, 189–193.
Kudinova-Pasternak R. K. (1973b). Tanaidacea (Crustacea, Malacostraca) collected on the R/V “Vitajz” in regions of the Aleutian Trench and Alaska. Trudy Instituta Okeanologii, Akademiya Nauk 91. 141–168.
Kudinova-Pasternak R. K. (1975). Tanaidacea (Crustacea, malacostraca) from the atlantic sector of antarctic and subantartic. Trudy Instituta Okeanologii, Akademiya Nauk SSSR 103. 194–229.
Kudinova-Pasternak R. K. (1978). Tanaidacea (Crustacea, Malacostraca) from the deep-sea trenches of the western part of the Pacific. Trudy Instituta Okeanologii, Akademiya Nauk SSSR 108. 115–135.
Lang K. (1956). Neotanaidae nov. fam., with some remarks on the phylogeny of the Tanaidacea. Arkiv för Zoologi Uppsala 2. 9, 469–475.
Larsen K. (1999). Deep-sea tanaidaceans (Crustacea: Peracarida) from the Albatross cruises 1885-86 with keys to the suborder Neotanaidomorpha. J. Natural History 33, 1107–1132. doi: 10.1080/002229399299969
Larsen K. (2005). “Deep-see tanaidacea (Peracarida) from the gulf of Mexico,” in Crustacean Monographs, vol. 5. (Brill, Leiden, Boston).
Larsen K., Guţu M., Sieg J. (2015). “Order Tanaidacea Dana, 184,” in Treatise on zoology – anatomy, taxonomy, biology. The Crustacea. Chapter 59. Eds. von Vaupel Klein J. C., Charmantier-Daures M., Schram F. R. (Brill, Leiden, Boston), 249–329.
Larsen K., Hansknecht T. (2003). Three new species of the deep-sea genus Neotanais Beddard (Crustacea: Peracarida). J. Natural History 37, 2787–2806. doi: 10.1080/0022293021000007390
Lauterbach K.-E. (1970). Der Cephalothorax von Tanais cavoliniid Milne Edwards (Crustacea—Malacostraca). Ein Beitrag zur vergleichenden Anatomie und Phylogenie der Tanaidacea. Zoologische Jahrbücher Abteilung Anatomie und Ontogenie der Tiere 87, 94–204.
Lobo J., Costa P. M., Teixeira M. A., Ferreira M. S. G., Costa M. H., Costa F. O. (2013). Enhanced primers for amplification of DNA barcodes from a broad range of marine metazoans. BMC Ecol. 13, 34. doi: 10.1186/1472-6785-13-34
Lörz A.-N., Tandberg A. H. S., Willassen E., Driskell A. (2018). Rhachotropis (Eusiroidea, Amphipoda) from the North East Atlantic. Zookeys 731, 75–101. doi: 10.3897/zookeys.731.19814
Marshall N., Diebel C. (1995). 'Deep-sea spiders' that walk through the water. J. Exp. Biol. 198, 1371–1379. doi: 10.1242/jeb.198.6.1371
Natural History Museum. (2014). Specimens (from Collection specimens) [Data set resource] (London, UK: Natural History Museum). Available at: https://data.nhm.ac.uk/dataset/collection-specimens/resource/05ff2255-c38a-40c9-b657-4ccb55ab2feb.
Norman A. M., Stebbing T. R. R. (1886). On the crustacea of the ‘Lighting’, ‘Porcupine’ and ‘Valorous’ Expeditions. Trans. Zoological Soc. London 12, 77–141. doi: 10.1111/j.1096-3642.1886.tb00008.x
Paulus E. (2021). Shedding light on deep-sea biodiversity – A highly vulnerable habitat in the face of anthropogenic change. Front. Mar. Sci. 8. doi: 10.3389/fmars.2021.667048
Pitz K. M., Sierwald P. (2010). Phylogeny of the millipede order Spirobolida (Arthropoda: Diplopoda: Helminthomorpha). Cladistics 26, 497–525. doi: 10.1111/j.1096-0031.2009.00303.x
Ramírez-Llodra E., Brandt A., Danovero R., De Mol B., Escobar E., German C. R., et al. (2010). Deep, diverse and definitely different: unique attributes of the world’s largest ecosystem. Biogeoscienes 7, 2851–2899. doi: 10.5194/bg-7-2851-2010
Schram F. R., Sieg J., Malzahn E. (1986). Fossil tanaidacea. Trans. San Diego Soc. Natural History 21, 127–144.
Sieg J. (1980a). Taxonomische monographie der tanaidae dana 1849 (Crustacea: tanaidacea). Abh Senckenb. Natforsch. Ges. 537, 1–267.
Sieg J. (1980b). Sind die Dikonophora eine polyphyletische Gruppe? Zoologischer Anzeiger 205, 401–416.
Sieg J. (1984). Neuere Erkenntnisse zum natürlichen System der Tanaidacea. Eine phylogenetische Studie. Zoologica 136, 1–132.
Stamatakis A., Hoover P., Rougemont J. (2008). A fast bootstraping algorithm for the RAxML web-servers. Syst. Biol. 57, 758–771. doi: 10.1080/10635150802429642
Svenson G. J., Whiting F. M. (2004). Phylogeny of Mantodea based on molecular data: evolution of a charismatic predator. Syst. Entomol. 29, 359–370. doi: 10.1111/j.0307-6970.2004.00240.x
Tamura K., Stecher G., Kumar S. (2021). MEGA11: Molecular Evolutionary Genetics Analysis versión 11. Mol. Biol. Evol. 38, 3022–3027. doi: 10.1093/molbev/msab120
Taylor D. J., Crease T. J., Brown W. M. (1999). Phylogenetic evidence for a single long-lived clade of crustacean cyclic parthenogens and its implications for the evolution of sex. Proc. R. Soc Lond. B (1999) 266, 791–797. doi: 10.1098/rspb.1999.0707
Thaler A. D., Amon D. (2019). 262 Voyages Beneath the Sea: A global assessment of macro- and megafaunal biodiversity and research effort at deep-sea hydrothermal vents. Peer J. 7, e7397. doi: 10.7717/peerj.7397
Tittensor D., Mora C., Jetz W., Lotze H. K., Ricard D., Vanden Berghe E., et al. (2010). Global patterns and predictors of marine biodiversity across taxa. Nature 466, 1098–1101. doi: 10.1038/nature09329
Watling L., Guinotte J., Clark M. R., Smith C. R. (2013). A proposed biogeography of the deep ocean floor. Prog. Oceanography 111, 91–112. doi: 10.1016/j.pocean.2012.11.003
Webb T. J., Vanden Berghe E., O’Dor R. (2010). Biodiversity’s big wet secret: The global distribution of marine biological records reveals chronic under-exploration of the deep pelagic ocean. PloS One 5, e10223. doi: 10.1371/journal.pone.0010223
Wi J. H., Suh H. L., Yu O. H. (2014). Two new species of the deep-sea genus Neotanais Beddard, 1886 (Tanaidacea: Neotanaididae) from the eastern central Pacific. J. Crustacean Biol. 34, 875–885. doi: 10.1163/1937240X-00002274
Wi J. H., Suh H. L., Yu O. H. (2015). Description of two new species of Neotanais Beddard, 1886 (Crustacea, Tanaidacea) from KODOS area. Zootaxa 39, 244–256. doi: 10.11646/zootaxa.3926.2.5
Keywords: abyssal, bathyal, benthic crustacean, direct development, hadal, marine biodiversity, sexual dimorphism, small invertebrate
Citation: Palacios Theil E and Błażewicz M (2024) Distribution of species in deep-sea biogeographic provinces and molecular phylogeny for the superfamily Neotanaoidea (Peracarida; Tanaidacea) indicate high levels of connectivity. Front. Mar. Sci. 11:1395000. doi: 10.3389/fmars.2024.1395000
Received: 02 March 2024; Accepted: 17 June 2024;
Published: 16 July 2024.
Edited by:
Kareen E. Schnabel, National Institute of Water and Atmospheric Research (NIWA), New ZealandReviewed by:
Ajit Kumar Mohanty, Indira Gandhi Centre for Atomic Research (IGCAR), IndiaHiroki Kise, University of the Ryukyus, Japan
Copyright © 2024 Palacios Theil and Błażewicz. This is an open-access article distributed under the terms of the Creative Commons Attribution License (CC BY). The use, distribution or reproduction in other forums is permitted, provided the original author(s) and the copyright owner(s) are credited and that the original publication in this journal is cited, in accordance with accepted academic practice. No use, distribution or reproduction is permitted which does not comply with these terms.
*Correspondence: Emma Palacios Theil, emma.palaciostheil@biol.uni.lodz.pl