- 1GEOMAR Helmholtz Centre for Ocean Research Kiel, Kiel, Germany
- 2European Global Ocean Observing System (EuroGOOS) AISBL, Brussels, Belgium
- 3Intergovernmental Oceanographic Commission of UNESCO, Paris, France
- 4Institute of Oceanography, Hellenic Centre for Marine Research, Crete, Greece
The interdisciplinary community of ocean and coastal observers and modelers in Europe is driven by national observing needs for prediction and management of intricate processes shaping Europe’s Seas. Not all observing needs can be addressed by nations alone and various coordination activities exist to overcome fragmentation and create cross benefit within the European Ocean Observing Community (EOOC). This way critical insights into impacts of climate change on European Waters and cross border marine resources management can be achieved. Based on a large number of published material, this article is identifying and addressing the current state of activities of the EOOC and states gaps that potentially prevent efficacy. Key challenges include spatial and temporal coverage in observations, data integration, accessibility, uncertainties in projections, technological hurdles, and engagement and communication gaps. Detailed recommendations are provided for identified gaps, offering valuable insights for stakeholders, funders, and supporters of the EOOC. These recommendations, extending beyond academic interest, carry significant implications for climate change mitigation, marine resource management efficiency, ecosystem resilience, disaster preparedness, economic benefits, and the broader scientific advancements in European marine science, thereby benefiting society at large. As the world undergoes transformative changes impacting all facets of European life, substantial investment and support for the EOOC are crucial for precise information, accurate predictions, supporting sustained services that contribute to business growth and community resilience, and a sustainable ocean.
1 Introduction
The European Ocean Observing Community, abbreviated here as EOOC to avoid repeating the long term throughout the paper, refers to the community of observers and modelers relying on an extensive array of infrastructure and predictions from various European institutes, organizations, networks, and data portals. This community contributes to observing, analyzing, and forecasting marine phenomena across coastal and open-ocean settings, spanning shallow to deep ocean layers. Covering a broad spectrum of oceanic aspects from physics to biology, the EOOC not only focuses on European waters but also extends its reach to large parts of the world’s ocean, making it crucial for understanding the large number of physical, chemical, biological, and geological phenomena that define the dynamic marine environment (Tintoré et al., 2019; Pérez-Gómez et al., 2021b).
Despite efforts towards homogenization by participating countries in ocean observation networks, there are disparities in infrastructure, expertise, and data policies within the EOOC, affecting its uniformity and effectiveness. As identified in a recent detailed report (Hassoun et al., 2023), key gaps include spatial and temporal data coverage, data integration and accessibility, uncertainties in projections, technological challenges, and stakeholder engagement. These issues arise from variations in methodologies, platforms, and the extent of coverage across different habitats and ecosystems, further complicated by diverse climate and ocean dynamics within European Seas (Heymans et al., 2018; Guidi et al., 2020; Hassoun et al., 2023). The EOOC faces challenges rooted in its diverse origins and disparate purposes, hindering coordinated efforts at national, regional, and EU scales. This complexity contributes to gaps in the system, impeding its readiness and responsiveness to emerging challenges and societal needs not only on national levels but also on contributing to a collective understanding and management of global ocean challenges.
This paper aims to highlight the gaps within the European Ocean Observing Community (EOOC), relying on the findings of Hassoun et al. (2023), while widening and updating the literature review to capture more recent challenges as well. Recommendations are also provided to address these gaps, offering insights for key actors within the EOOC. The implementation of these recommendations could enhance the EU’s capabilities in observing and forecasting ocean phenomena, aligning resources and efforts to improve overall system effectiveness and resilience.
2 Methodology
The methodology adopted can be divided into three steps as illustrated in Figure 1:
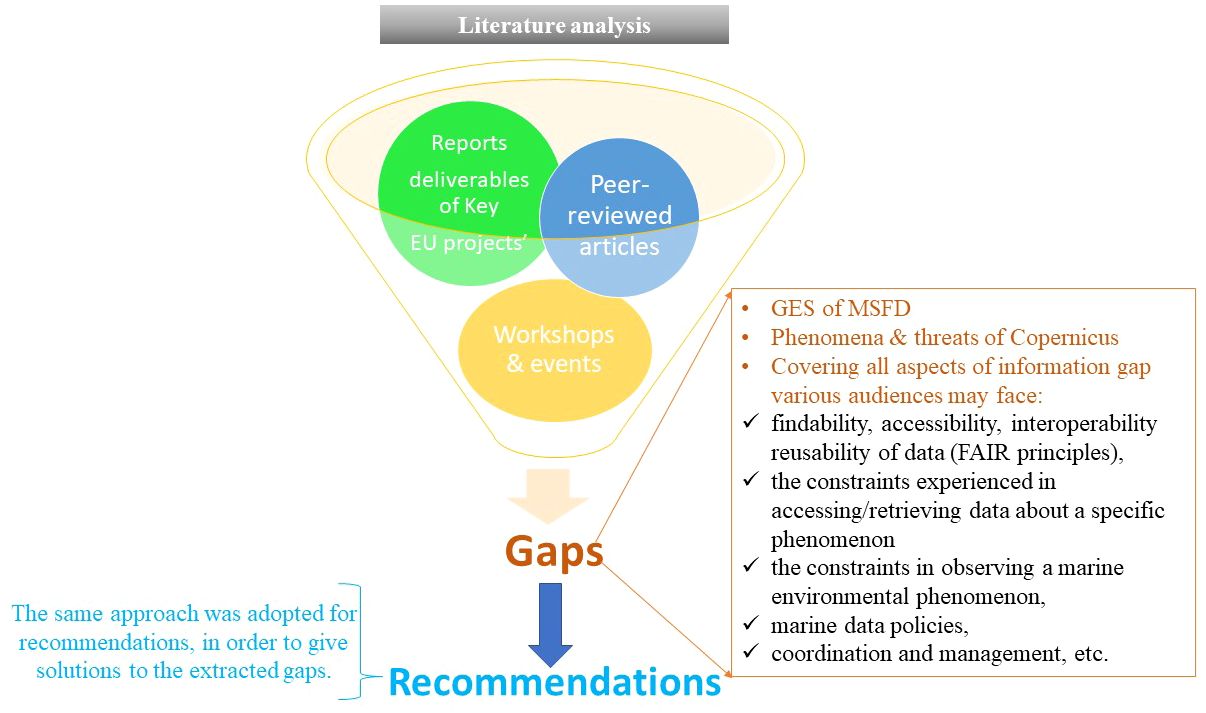
Figure 1 Infographic summarizing the methodology used to extract the gaps and provide recommendations.
2.1 Documents selection
An extensive examination of EU project reports as well as key peer-reviewed publications were conducted. Deliverables and reports from these projects were scrutinized to identify gaps associated with various aspects of the ocean observing and forecasting related to key ocean phenomena, marine applications/services and components of the ocean value chain.
The review included connections to physical, chemical, and biological observations, data availability, system readiness and adherence to FAIR principles. Moreover, a focus was directed towards gaps tied to specific societal needs within the “blue economy” sector (e.g., wind farms, oil leakage). Our assessment considered the needs of three distinct audiences: 1) the scientific community keen on closing observational and forecasting gaps for research purposes; 2) businesses and industries relying on EOOC for operations and opportunities; and 3) the general public relying on data produced by EOOC for insights into ocean health, ecosystems, and resources.
2.2 Literature review process
After selecting the key documents to be inspected, a systematic literature review was conducted with a particular emphasis on addressing the needs of the identified audiences (step 2.1). The review process unfolded in two stages. First, key EU projects and initiatives related to EOOC were identified. Subsequently, all documents and articles originating from these projects were meticulously examined to categorize gaps and recommendations. Peer-reviewed articles addressing gaps within EOOC were thoroughly assessed, alongside insights from specialized workshops dedicated to ocean observations and forecasting updates, technological advancements, and climate change monitoring. The initial assessment included 47 documents, as detailed in Hassoun et al. (2023). Additional references are used here to keep the literature review updated. The majority of the documents assessed (62%) have been published since 2020, signifying the ongoing validity of the identified gaps, emphasizing their persisting significance and the need for comprehensive solutions.
2.3 Extracted gaps and recommendations
The identified gaps align with key directives and frameworks, such as the Good Environmental Status (GES) of the EU Marine Strategy Framework Directive (MS FD)1, phenomena and threats2 covered by the Copernicus program, and components of the ocean value chain (Pearlman et al., 2019). These gaps encompass challenges in observing and forecasting physical, chemical and biological ocean phenomena, and gaps related to data, observing networks, the sustainability of observations, technological, research and development aspects, marine policies and legislations, coordination, management and reporting, marine services and applications (namely wind farms and oil spills response), and challenges related to users’ satisfaction regarding the EOOC products, and the ocean literacy efforts conducted by the EOOC (Figure 2). Recommendations are thoughtfully provided to offer targeted solutions.
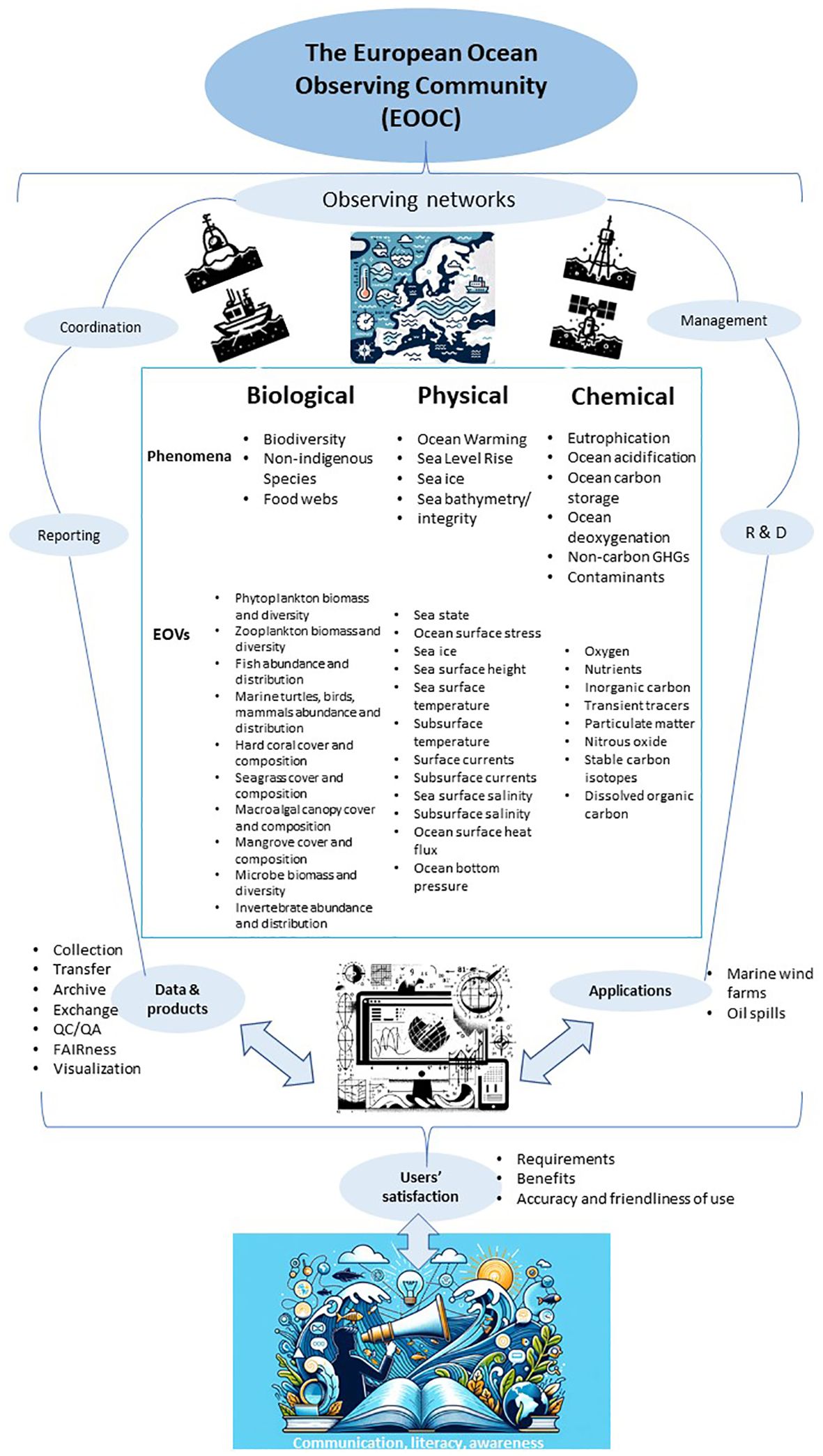
Figure 2 A summary illustrating our understanding of the EOOC, highlighting the ocean phenomena and ocean value chain components assessed in this study as well as the GOOS EOVs needed to observe each phenomena.
3 Results and discussion
In this section, all identified gaps are briefly elaborated, shedding light on the most urgent ones. A report describing all these gaps and the recommendations in detail was newly published as a deliverable of the EuroSea project (D1.9; Hassoun et al., 2023). An overview of the identified gaps are summarized in Figure 3, and main provided recommendations are illustrated in Figure 4.
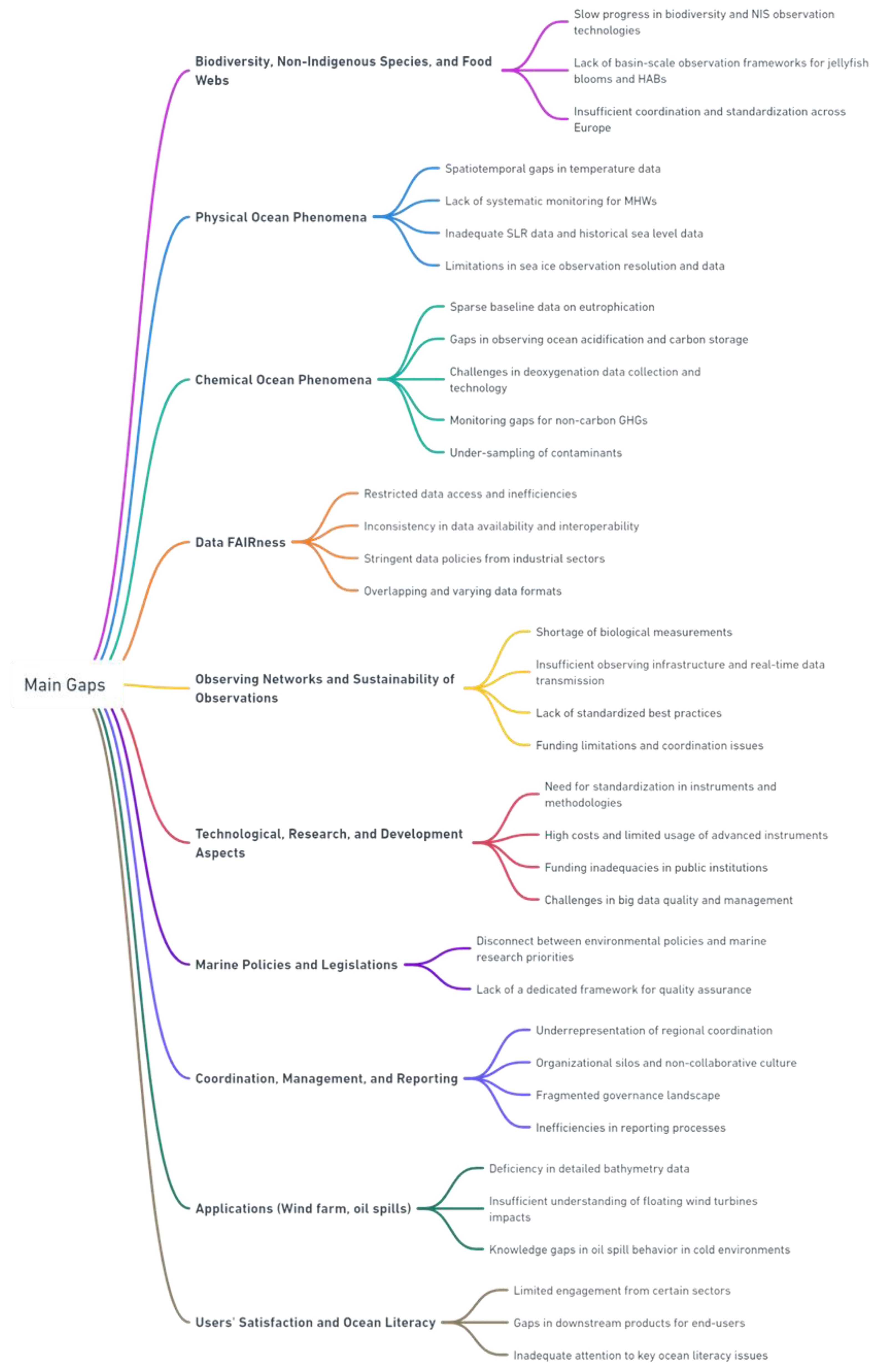
Figure 3 An overview of the gaps detailed in our assessment. Each color refers to one of the topics covered in our assessment.
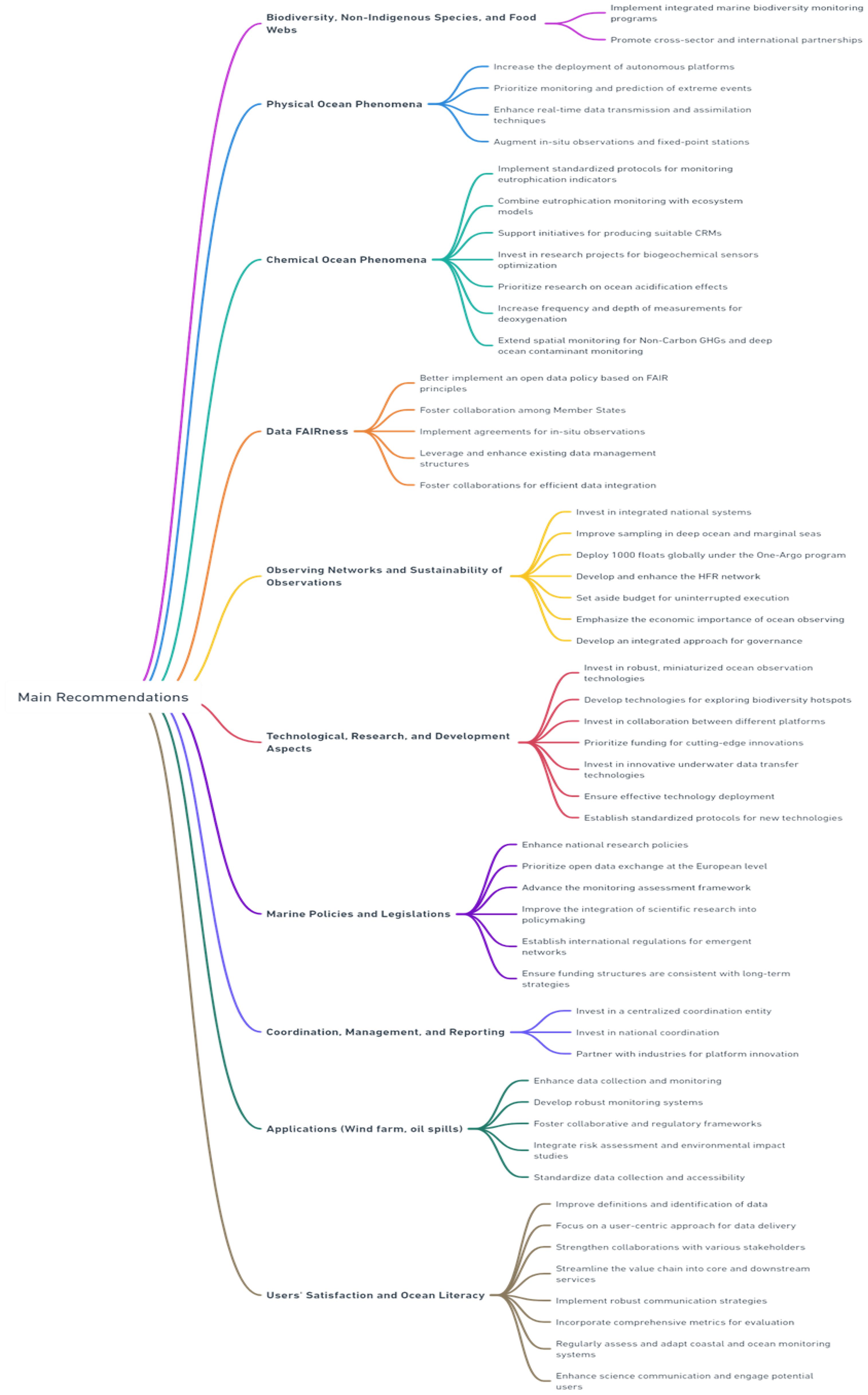
Figure 4 An overview of the recommendations detailed in our assessment. Each color refers to one of the topics covered in our assessment.
3.1 Biodiversity, non-indigenous species and food webs (biological phenomena)
The EOOC has made remarkable progress in the past decade in monitoring Biodiversity and Non-Indigenous Species (NIS), providing invaluable data on key biological Essential Ocean Variables (EOVs). This progress represents a positive step towards comprehensively understanding biological patterns, highlighting the system’s pivotal role in marine ecosystem monitoring. Moreover, the EOOC has demonstrated notable advancements in monitoring Food Webs by instituting more extensive and standardized observing networks. These endeavors have led to enhanced data availability and quality pertaining to plankton, fish, and other crucial components of marine food webs. These improvements significantly contribute to our understanding of ecosystem dynamics, support effective fisheries management, and inform policies aimed at preserving marine resources and safeguarding the health of European Seas. However, while recognizing these positive developments, significant challenges persist within the EOOC, primarily in the processes and outputs’ pillars.
Recent technological advancements in biodiversity monitoring offer promising avenues for addressing the existing deficiency in the domain of biodiversity and NIS observation. These advancements include the use of environmental DNA (eDNA) and metabarcoding techniques (Valentini et al., 2016) supported by supervised machine learning (Cordier et al., 2017) and new high-tech flexible networks for deep-sea ecosystems (Aguzzi et al., 2019). Nevertheless, this progress is slow compared to automated advancements in physico-chemical observations. This gap is particularly pronounced in offshore oceanic waters, where time-consuming traditional sampling methods persistently prevail. For instance, the remoteness and sparse population densities of the Atlantic and Arctic Oceans contribute to the scarcity of comprehensive data on deep-sea biodiversity and microbial communities (Glöckner et al., 2012). The absence of systematic, science-based monitoring systems exacerbates these challenges, particularly with phenomena like jellyfish blooms and Harmful Algal Blooms (HABs) conspicuously lacking basin-scale observation frameworks (EMODnet checkpoints, 2016-2020). The insufficiency in coordination and standardization of observational methods across European countries further hampers progress (Tintoré et al., 2019). This lack of uniformity impedes the development of a harmonious and comprehensive dataset. Additionally, taxonomic analyses are primarily conducted at the genus level, which adds complexity to understanding species-specific distribution and abundance (Tintoré et al., 2019). For instance, a comprehensive “Catalogue of Marine Biodiversity Indicators” has been developed by Teixeira et al. (2016), which comprises over 600 marine biodiversity indicators, including information on NIS, food webs, and ecosystem health. Nevertheless, this tool, accessible through the DEVOTool software application, needs continuous updates to better provide a broad coverage of the most relevant indicators for marine biodiversity and ecosystem integrity in European Seas.
The observation of Food webs within the EOOC witnesses significant gaps, particularly in the realm of plankton data. Phytoplankton and zooplankton, crucial components of the marine food web, face challenges in data visibility, policy, delivery readiness, and cost-effectiveness. These issues are notably pronounced in the Black and Mediterranean Seas (Ratnarajah et al., 2022). In terms of fisheries data, there are considerable challenges related to the abundance and distribution of fish species. Issues with data quality, particularly concerning discards and the reporting of bycatch, are prevalent (EMODnet checkpoints, 2016-2020; EuroStat, 2020). Also, fisheries data presentation and accessibility on national portals vary significantly, often requiring extensive processing and incurring costs that impede their widespread use and interoperability (EuroStat, 2020). The International Council for the Exploration of the Sea (ICES)3 plays a pivotal role in providing expert fisheries advice based on diverse data sets. However, there is an absence of coverage for the Mediterranean and Black Seas within the ICES framework. Although the General Fisheries Commission for the Mediterranean (GFCM)4 does offer advisory services, the availability of detailed data is limited, with only summary reports that are challenging to utilize effectively for detailed analyses. These data gaps and challenges complicate efforts in forecasting and mitigating the impacts of fisheries techniques on fish stocks, habitat loss, and overall ecosystem health. In particular, the lack of long-term data availability, especially in the Mediterranean and Black Seas, hinders the accuracy of predictions regarding future trends and impacts (SWD, 2020). The reliability of data on catch components, notably discards, is compromised by uncertainties concerning data quality and the influence of illegal fishing activities (EMODnet checkpoints, 2016-2020). Forecasting the repercussions of fisheries techniques on fish stocks, habitat loss, and ecosystem damages is further impeded by the dearth of observational data. This shortfall in data poses a significant challenge in accurately predicting and addressing the broader environmental impacts of fisheries practices (SWD, 2020).
3.2 Recommendations for biodiversity, non-indigenous species and food webs (biological phenomena)
• Implement integrated marine biodiversity monitoring programs, emphasizing biological Essential Ocean Variables, invasive species tracking (mainly in regions conducive to their occurrence), and the use of both traditional and innovative observation technologies (e.g., eDNA), while fostering data sharing and enhancing databases (e.g., developing a regularly updated central information system for alien species) for comprehensive ecosystem assessment and protection.
• Promote cross-sector and international partnerships to align marine biodiversity monitoring strategies, ensuring interoperability among observation systems, enhancing global data sharing, and fostering interdisciplinary efforts for ecosystem-based management and conservation.
3.3 Physical ocean phenomena
The EOOC plays a pivotal role in monitoring crucial physical ocean phenomena, encompassing ocean warming, sea level, sea ice, and seafloor bathymetry and integrity, among others. While substantial progress has been made in these domains, noteworthy gaps persist. In the realm of ocean warming, despite the maturity of existing monitoring efforts and the wealth of data from remote sensing, critical spatiotemporal gaps persist in temperature data across several European Seas. For example, addressing gaps in temperature observations, particularly in the upper 10 meters, is crucial for validating satellite data, as highlighted in the Atlantic Ocean (Buch et al., 2017). Furthermore, the anticipated increase in the risk of extreme heat waves necessitates a more comprehensive understanding of their future patterns, frequency, and intensity. Remarkably, there is an absence of a systematic, scientifically based monitoring system dedicated to tracking Marine Heat Waves (MHWs) (Prieto et al., 2015; Tintoré et al., 2019). The availability of temperature data, while generally accessible, presents challenges in terms of data quality, metadata verification, and technological constraints, especially in challenging oceanic areas such as the North Atlantic and Arctic Oceans, where harsh winter conditions and sea ice formation limit observation resolutions (Kwok and Maksym, 2014; Buch et al., 2017; EMODnet, 2018a). Regarding sea level rise (SLR) observation, despite its significant environmental, social, and economic implications, it is not recognized as an indicator by EuroStat5 (the statistical office of the European Union, responsible for publishing high-quality Europe-wide statistics and indicators) for monitoring climate impacts in Europe, as the focus remains primarily on average global and European temperature, ocean acidity, and economic costs related to weather and climate-related disasters (EuroStat, 2020). Additionally, there are no network Key Performance Indicators (KPIs) dedicated to SLR (Karstensen et al., 2020). A substantial gap lies in the availability of historical sea level data from certain regions, including Denmark, Estonia, Poland, Lithuania, and others, which are incomplete in the EMODnet Physics database (EMODnet checkpoints, 2016-2020). These gaps extend to coastal regions, where sea level and sediment mass balance data for the past 10, 50, and 100 years are insufficient, hindering assessments of long-term trends and SLR-related impacts. The lack of density in long-term time-series data from tidal gauges further complicates the assessment of sea level changes at the coast, with co-location challenges remaining (EMODnet checkpoints, 2016-2020). For example, sea level reconstructions are associated with large uncertainties (up to 0.3–0.6 mm·yr−1) due to the choice of glacial isostatic adjustment (GIA) corrections, with difference up to 8 mm·yr−1 in rate of sea level rise in the Arctic, Baltic and Antarctic regions, suggesting the need for further constraining GIA models, especially in the Arctic and Antarctic where rapid mass change is occurring (Jevrejeva et al., 2014). Also, inadequate SLR data exchange, particularly in the Mediterranean Sea region, hampers accurate assessments and monitoring efforts (Bonaduce et al., 2016). End-user challenges, including inconsistent content on data portals and issues with geographical coordinates and naming conventions, further hinder SLR monitoring (EMODnet checkpoints, 2016-2020). It is noteworthy that in the framework of the EuroSea project, significant progress was made with the Permanent Service for Mean Sea Level (PSMSL) launching a portal of sea level data obtained using the novel measurement technique of Global Navigation Satellite System-Interferometric Reflectometry (GNSS-IR) (Pérez-Gómez et al., 2021a). Regarding the observation of sea ice, gaps are related to limitations in resolution and long-term data availability. While satellite-based remote sensing provides valuable information on sea ice extent, it often lacks the necessary resolution to capture fine-scale processes and changes (Boulze et al., 2020; De Gelis et al., 2021). Furthermore, consistent, long-term monitoring efforts spanning multiple decades are lacking, resulting in gaps in sea ice cover data over the past 100 years, particularly in regions like the Baltic Sea (EMODnet, 2018b) where although historical data exist they were designed for storage on punch cards making them difficult to use (Löptien and Dietze, 2014). Although these data have been recently digitized and commonly known as BASIS-ice data set, they are still to be included in the EMODnet data portal. This initiative is a good example that can be implemented in the Arctic, for instance, where there should be integration of historical hydrographic data (Jakobsson et al., 2020), as sea ice declines and waterways such as the Northwest Passage become more navigable, and the need for sea ice nowcasts and forecasts and the need for detailed digital elevation models (DEMs) is increasing. Further, sea ice observations still lack ridged ice and better understanding of ice dynamics. Ice ridges are difficult to break and thus form substantial obstacles for ships (Löptien and Dietze, 2014). While realistic ice dynamics are needed in seasonal modeling for the ice transport and, particularly, for opening and closing of leads (Leppäranta, 2023). Whereas observing sea ice thickness presents another challenge, especially in remote and challenging regions like the Arctic, where technological advancements are needed to overcome limitations in traditional methods (Lüpkes et al., 2012). For instance, to better assess the snow radar retrievals and for informing methodologies for conversion of freeboard to thickness, there is a need for better sampling of the Antarctic sea ice cover as the ice areas with much of the snow depths and freeboards would be largely impenetrable to most icebreakers, and the accessibility issues remain a challenge to provide adequate sampling of the ice cover (Kwok and Maksym, 2014). Integrating various remote sensing technologies and in-situ observations remains a significant gap in understanding sea ice changes and interactions with the ocean. For seafloor bathymetry and integrity, the system faces substantial gaps that need attention, such as limited spatial coverage and resolution, insufficient biodiversity data of the seafloor, and a lack of real-time capabilities (Buch et al., 2017). Current data sources often provide coarse-scale measurements, which are insufficient for capturing intricate seafloor details (EMODnet, 2018). Moreover, the absence of consistent and comprehensive data on seafloor biodiversity and ecological status impedes the evaluation of anthropogenic impacts (Teixeira et al., 2019). Continuous observations are essential for detecting dynamic changes, yet real-time capabilities are underutilized, impacting the ability to respond to rapid alterations (Ardhuin, 2018). Technical metadata gaps, data format issues, and the lack of data policy standardization further complicate the utilization of bathymetric data (EMODnet, 2018). These technical challenges contribute to the inadequacy of certain descriptors related to seafloor integrity and hydrographical conditions (SWD, 2020).
3.4 Recommendations for physical ocean phenomena
• Increase the deployment of autonomous platforms, including Argo floats, drifters, and sensors, within the top 10 meters for validating satellite data and to enhance deep ocean and high-latitude observations.
• Prioritize the monitoring and prediction of extreme events such as MHWs and droughts. Develop advanced predictive models and mitigation strategies using state-of-the-art scientific methodologies.
• Enhance real-time data transmission and assimilation techniques to improve operational forecasting and emergency response capabilities.
• Address climate impacts by designating Sea Level Rise (SLR) as a crucial indicator, digitizing historical data for comprehensive monitoring, and refining Glacial Isostatic Adjustment (GIA) models to improve sea level predictions, especially in polar regions.
• Improve seafloor monitoring by enhancing bathymetry data coverage, standardizing data policies for easier use, and leveraging real-time technologies to assess ecological status and anthropogenic impacts on seafloor integrity.
• Enhance Arctic monitoring by deploying autonomous platforms for satellite data validation, developing models for extreme event prediction, expanding satellite networks for comprehensive sea ice and ocean observation, digitizing historical data for sea level and sea ice monitoring, utilizing technological advances for sea ice thickness assessment, and establishing sustained monitoring of seafloor integrity and bathymetry to better understand and respond to climate impacts in polar regions.
• Augment the number of accurate in-situ observations and fixed-point stations, encouraging coincident measurements of various variables with a special attention to the under-observed ones such as sediment transport, important to improve sediment modeling and better understand sediment dynamics and their impact on seafloor integrity.
3.5 Chemical ocean phenomena
The EOOC has significantly advanced in observing key ocean chemical phenomena, employing sophisticated techniques to meticulously study eutrophication, ocean carbon, dissolved oxygen, non-carbon Greenhouse Gases, and contaminants in European Seas. These developments have been instrumental in providing detailed insights into the chemical makeup of open and coastal ecosystems and facilitating effective management against eutrophication. The system’s ability to gather real-time data on ocean chemistry is pivotal in tackling ocean acidification, aiding policymakers to be informed about shifts in ocean carbon storage. Additionally, the EOOC’s observation of dissolved oxygen levels sheds light on the scope and implications of deoxygenation, crucial for understanding its impact on marine life, fisheries, and coastal communities. Moreover, the EOOC’s enhanced capability to accurately track various pollutants plays a critical role in protecting marine ecosystems and human health. Overall, these advancements by the EOOC are essential in informing and guiding policy and environmental management strategies, leading for example to the recognition of the “inorganic carbon” as a GOOS EOV6 and ocean acidification as one of the phenomena, and initiatives like the one led by the IOC-UNESCO to encourage observers to report data under SDG 14.3.17 made small advancements, highlighting ongoing challenges (Tilbrook et al., 2019). However, the EOOC still encounters several significant challenges that, if solved, would optimize the observation and forecasting of these phenomena. Observing eutrophication, a significant marine environmental concern particularly in coastal areas, still witness sparse baseline data. In the Atlantic Ocean, only around 10% of observing networks gather essential biogeochemical parameters such as nutrients, contributing to significant gaps in eutrophication data (Buch et al., 2017). The complexity of coastal systems requires not only higher spatial coverage but also more frequent temporal coverage (e.g., the MSFD-related observations are mainly seasonal) compared to the open sea. Key parameters like nitrate, phosphate, chlorophyll pigments, and dissolved oxygen exhibit gaps due to low spatial and temporal sampling density (EMODnet checkpoints, 2016-2020; EuroStat, 2020). A comprehensive observation strategy that integrates physical, biogeochemical, and biological parameters is still missing in many European Seas, hindering a thorough interpretation of the causes and intensity of eutrophication, as well as to understand organic matter and inorganic nutrient cycling (EMODnet checkpoints, 2016-2020). The EOOC also faces challenges in observing ocean acidification and carbon storage. Science-driven research, often constrained by short-term funding cycles, limits the ability to capture long-term patterns and responses of ocean ecosystems (GOA-ON, 2019). The absence of fixed-point observatories in deep water formation regions impedes monitoring sub-decadal variability, as there are several spatial gaps and seasonal biases in underway ship-based observations, especially in key areas like the South Atlantic and North Atlantic deep-water formation zones (Buch et al., 2017; GOA-ON data portal), as well as in many Mediterranean Sea sub-basins (Hassoun et al., 2022; Álvarez et al., 2023). Additionally, significant uncertainties observed in several datasets, along with the different methods adopted to measure the carbonate system across European institutions, hinder a comprehensive understanding of ocean acidification patterns and the role of European Seas as carbon sources or sinks (Hoppe et al., 2012; Hassoun et al., 2022; Álvarez et al., 2023). An additional quality gap is emerging recently but jeopardizing ocean carbon research in the future is the limited-availability of Certified Reference Materials (CRMs) (Hassoun et al., 2022). Further, observing ocean deoxygenation within the EOOC is challenged by gaps in data collection and technological limitations. In the Atlantic Ocean, for instance, a mere 10% of observing networks collect crucial biogeochemical parameters like dissolved oxygen (Buch et al., 2017). These limitations, stemming from the limited number of monitoring stations, historical monitoring designs, limitations of the available automated sensors, and inadequate spatial coverage, particularly in areas with significant variations in dissolved oxygen, impede the comprehensive assessment of deoxygenation and hypoxia. Current monitoring efforts are primarily focused on coastal areas, leading to limited spatial coverage across European waters and inhibiting a thorough understanding of deoxygenation processes and their impacts on marine ecosystems (Pereiro et al., 2022). For non-carbon GHGs, the EOOC encounters monitoring gaps, primarily attributed to insufficient atmospheric condition data, especially in European basins like the Mediterranean, Black Seas, Atlantic, and Arctic Oceans (Buch et al., 2017; EMODnet, 2017; EMODnet, 2018a; EMODnet, 2018c). While some non-CO2 greenhouse gases data, like for Nitrous Oxide (N2O), are regularly collected, accessibility might be restricted, impacting open access efforts. For instance, the MarinE MethanE and NiTrous Oxide (MEMENTO)8 database for N2O requires email requests and registration and needs significant updates, introducing a gap in real-time data findability and accessibility (Lange et al., 2023). Moreover, observations with limited temporal resolution are not covering critical events, from diurnal to seasonal and interannual variations, failing to accurately capture long-term trends, particularly for N2O and methane in European Seas (Sutton et al., 2014). These observations are primarily concentrated in coastal regions, resulting in limited spatial coverage of non-carbon GHGs measurements across European Seas, including the Arctic Ocean (Rees et al., 2022). Also, these observations lack the necessary vertical resolution to capture the vertical variations of non-carbon GHGs throughout the water column (Lennartz et al., 2017), and the spatial coverage as there are still underrepresented regions, such as the Arctic which is a significant gap, given its crucial role in global non-carbon GHGs dynamics and its vulnerability to climate change (Rödenbeck et al., 2015). Lastly, observing contaminants within the EOOC witnesses several gaps across different marine regions, spanning from coastal and shelf areas to the open ocean and deep layers. These gaps are characterized by under-sampling of the deep ocean and an absence of comprehensive measurements encompassing the related EOVs (namely nutrients and inorganic carbon), which ultimately hinder our ability to accurately interpret changes related to key environmental parameters and contaminants (Buch et al., 2017). One significant issue is that the current list of biogeochemical EOVs9 does not extend beyond nutrients. Notably, contaminants such as Persistent Organic Pollutants (POPs), which encompass organochlorine pesticides and industrial chemicals, as well as biotoxins produced by toxic marine species, have not been officially recognized as EOVs. Nevertheless, these contaminants have been detected in various European Seas, including the Mediterranean Sea, Arctic Ocean, and among deep-sea species (Wenning and Martello, 2014; Castro-Jimenez et al., 2017; Klinčić et al., 2020; Hassoun et al., 2021; Ikuta et al., 2021; Romanić et al., 2021; Hung et al., 2022). Furthermore, while existing monitoring programs are considered adequate for specific contaminants, they encounter challenges when attempting to address the broader spectrum of marine pollutants. This challenge arises because certain contaminants may be more relevant to observe in specific European Seas compared to others, highlighting the need for a tailored approach (SWD, 2020; EuroStat, 2020). Additionally, a noteworthy gap exists in the limited number of accredited laboratories dedicated to routinely assessing concentrations of emergent contaminants, such as biotoxins, in marine and coastal environments. This gap impacts the timely provision of essential information, which is crucial for mitigating impacts, particularly those stemming from Harmful Algal Blooms (HABs) and their associated biotoxins, on industries like aquaculture (Roose et al., 2011; Buch et al., 2017).
3.6 Recommendations for chemical ocean phenomena
• Implement standardized protocols for monitoring key eutrophication indicators such as nutrient concentrations (nitrogen and phosphorus), chlorophyll-a, dissolved oxygen, and primary production. This aligns with the need to minimize human-induced eutrophication, contaminants’ concentrations, and marine litter as part of the 11 elements required for marine and coastal waters to achieve Good Environmental Status (GES) of the Marine Strategy Framework Directive (MSFD). A newly established JPI10 Knowledge Hub for Science for Good Environmental Status (S4GES11) will address this point as it aims to contribute to a better understanding and achievement of GES in support of the MSFD.
• Enhance the coverage of nutrients’ measurements, including the monitoring of non-point source pollution (e.g., agricultural runoff and urban wastewater, rivers’ inputs), while improving the connection between marine and terrestrial networks doing these types of observations independently.
• Develop and deploy autonomous sensor networks, underwater observatories, and fixed-point observatories, especially in eutrophic areas and regions of deep-water formation. These systems should enable real-time, continuous monitoring of nutrient dynamics, algal blooms, oxygen depletion, ocean carbon storage, and acidification, addressing spatial and seasonal data biases through collaboration with maritime initiatives.
• Combine eutrophication monitoring with ecosystem models and socio-economic indicators to understand the full impacts of eutrophication, guiding informed policy and management decisions. This approach should include the establishment of additional time-series stations across under-sampled European Seas.
• Support initiatives like the Integrated Carbon Observation System (ICOS) to produce suitable and affordable Certified Reference Materials (CRMs) for biogeochemical measurements, ensuring their accuracy and reliability.
• Invest in research projects aimed at improving the accuracy, portability, and cost-effectiveness of biogeochemical sensors on autonomous platforms, including the optimization of the global Biogeochemical-Argo array.
• Prioritize research on the effects of ocean acidification on marine ecosystems and biodiversity, focusing on sensitive and ecologically important species to mitigate potential adverse outcomes.
• Increase the frequency and depth of measurements to monitor the progression of deoxygenation, particularly in critical areas like the Mediterranean Sea, while standardizing observing protocols and utilizing advanced platforms.
• Extend spatial monitoring and atmospheric data collection for Non-Carbon GHGs and deep ocean contaminant monitoring.
• Standardize measurement protocols and support policy development for the rapid identification and monitoring of emerging pollutants, ensuring data comparability across different platforms.
3.7 Data FAIRness
Despite the remarkable advancements in implementing data FAIR principles, numerous challenges related to data availability, quality, accessibility, and management can still be identified in the EOOC, affecting its operational efficiency in observing various marine phenomena (Figure 5). For example, restricted data access due to inefficient search functions and prolonged timelines for public data access is still common. This particularly hinders the utilization of vital data such as ship measurements for timely forecasting and environmental assessment. The delayed availability of such data, for instance, ship data accessible years after collection, impedes the system’s real-time operational capabilities, thus reducing the efficacy of forecasts and assessments (EMODnet, 2018). Data accessibility issues, such as restrictions on access duration and prerequisites for registration, further complicate the open access to essential data used to understand various marine environmental phenomena such as eutrophication and non-carbon GHGs. Another gap within the EOOC is the inconsistency in data availability and interoperability, especially concerning national-level datasets that are essential for comprehending phenomena like offshore wind profiles and historical sea levels. However, the accessibility of these datasets varies across different platforms, leading to challenges in data integration and analysis. Additionally, there are significant gaps in certain datasets, such as those related to climate and river inputs, biological monitoring programs, and zooplankton community data, which limits large-scale research capabilities (EMODnet, 2018; Ratnarajah, 2021). Additionally, stringent data policies, primarily from industrial, naval, and military sectors, further exacerbate data accessibility problems. The reluctance to share data, driven by concerns over business impacts or the potential for “incorrect” data interpretation, results in significant data gaps. This situation is evident in the Black and the Mediterranean Seas, where a considerable proportion of datasets are inadequately aligned with policy visibility and data policy requirements (EMODnet, 2017; EMODnet, 2018a; SWD, 2020). Furthermore, the EOOC faces challenges in data quality and format. Overlapping and varying data formats across different portals hinder user convenience and interoperability. The lack of standardized metadata formats and sources, as highlighted by the EU Commission (SWD, 2020), leads to difficulties in identifying useful datasets and understanding their overlap or duplication with other portals. Insufficient data coverage and resolution, coupled with inadequate metadata, contribute to these data gaps. For instance, there are gaps in wind profiles, sedimentation rate, grain size, and historical ice thickness data, among others. These issues are further compounded by the non-digital and non-standard nature of some data formats, which lack essential elements like standard identifiers or harmonized Digital Object Identifiers (DOIs), critical for data traceability and accessibility (EMODnet, 2017; EMODnet, 2018c; SWD, 2020; Tsontos, 2021). Also, the landscape of data management within the EOOC is complex and fragmented, lacking standardized practices and structures. This results in delayed data receipts, missing data, and undocumented data processing procedures, which hampers the retrieval of specific data types or data from certain areas under national jurisdiction. For instance, there are issues related to the management of marine image data and linking these with global data infrastructures (de Young et al., 2019; Guidi et al., 2020). The fragmented nature of data management within the EOOC underscores the need for engagement of data and user communities to improve data utility and applicability (Tanhua et al., 2019a). Nevertheless, recent developments signal a positive shift in this trend. Initiatives like SeaDataNet12 and EMODnet have demonstrated successful models for improving data management, showcasing the benefits of standardized practices and structures in enhancing data availability and interoperability. These efforts are complemented by the endorsement of the IOC data policy13 by EuroGOOS members14, reflecting a growing consensus on the importance of open and accessible data policies. Additionally, the MINKE project15 is making significant strides in addressing data quality, focusing on metrology and the estimation of uncertainties, further enriching the data’s value for scientific research and policy-making. These advancements underscore a growing commitment within the EOOC, marking a promising step towards overcoming the previously fragmented nature of data management.
3.8 Recommendations for data FAIRness
• Better implement an open data policy based on FAIR principles to ensure easy, fast, and open access to data across European data systems. This includes promoting Digital Object Identifiers (DOIs) for data citation and incentivizing data sharing among various stakeholders, including Member States, to make data available on platforms like EMODnet. Encouraging European countries to endorse an open data policy for EOVs defined by the Global Ocean Observing System (GOOS) is crucial for enhancing data accessibility and interoperability (Tanhua et al., 2019).
• Foster collaboration among Member States through directives like the MSFD or MSPD, and encourage sharing data with EMODnet.
• Implement agreements for in-situ observations to expand spatial and temporal resolution, particularly in national Exclusive Economic Zones (EEZs). This includes educating data originators and funding agencies about the importance of existing marine data infrastructures and fostering official partnerships between marine scientists and industries to facilitate high-quality data collections (Bakker et al., 2016; Lauvset et al., 2016).
• Promote interdisciplinary collaborations among various scientific disciplines to integrate diverse data into broader applications and ensure public availability of crucial long-term monitoring data (Ratnarajah, 2021).
• Leverage and enhance existing structures, such as SeaDataNet or EMODnet, to provide a viable solution to standardize data usage terms and conditions, addressing challenges related to data link maintenance and metadata. By building upon these established networks, we can ensure professional data management and enhance data accessibility, focusing on the continuous development of marine data management facilities and open access data portals. This approach would capitalize on the strengths and existing capacities of these structures to improve data availability and quality, avoiding the complexities and resources required to establish a new, integrated, multidisciplinary center.
• Foster collaborations between marine data management infrastructures to provide tools for efficient data integration, addressing data heterogeneity and fragmentation. This includes adopting systems like a Spatial Relational Database Management System based on INSPIRE guidelines, improving data discoverability and access, and ensuring comprehensive data access. Collaborations should also focus on ensuring metadata standardized vocabularies, metadata completeness, and availability to facilitate effective use of datasets (Pérez-Gómez et al., 2021b; EMODnet, 2018).
3.9 Observing networks and sustainability of observations
The EOOC has played a pivotal role in expanding the coverage and capacity of observing networks across the European Seas, resulting in improved spatial and temporal resolution of critical ocean variables. These efforts have facilitated a more detailed understanding of ocean dynamics, biodiversity, and various environmental parameters, thereby enhancing the quality and accuracy of the collected data. Nevertheless, the EOOC is still facing substantial challenges regarding its observing networks and the sustainability of its observations. Firstly, the EOOC is marked by a notable shortage of biological measurements compared to physical and biogeochemical parameters. This disparity is particularly pronounced in the Atlantic Ocean, leading to gaps in the comprehensive understanding of marine phenomena (Buch et al., 2017). A critical issue in this regard is the insufficient observing infrastructure needed to maintain real-time data transmission, for example in the Atlantic and Arctic regions (Buch et al., 2017) and the Mediterranean Sea (Tintoré et al., 2019). Further, due to the size of the relevant dynamical and mesoscale structures in the Mediterranean Sea, the limited number of platforms is insufficient to properly contribute to data assimilation in numerical models, reflecting the insufficient amount of observing platforms crucial to collect parameters such as currents, salinity and water temperature (Tintoré et al., 2019). This limitation hampers effective real-time data transmission and validation for satellite sensors, which are vital for accurate and timely marine observations. Further compounding the problem is the disproportionate focus on coastal waters, which constitute 68% of all monitoring programs. Although this spatial coverage is very needed in the highly variable coastal areas, it certainly reflects the imbalance in sampling the deep ocean (SWD, 2020). This skewed emphasis raises concerns about the quality and availability of data needed for societal benefit products (Tanhua et al., 2019b; SWD, 2020), impacting sectors such as aquaculture, especially along Europe’s coasts. Additionally, the lack of standardized best practices across various networks and variables impedes effective data collection and interpretation, thereby affecting the progress assessment in different marine areas over the past 15 years (Buch et al., 2017; EuroStat, 2020). Furthermore, the diversity of observing networks within EOOC represents a spectrum of readiness levels and initially posed challenges in creating systematic linkages, leading to interoperability issues (de Young et al., 2019; Révelard et al., 2022). However, this diversity is increasingly seen as an asset, not a limitation. The adoption of a multiplatform approach leverages the strengths of various platforms, such as GO-SHIPS, gliders, Argos, and high-frequency radar systems, to address gaps in spatial and seasonal data collection. This collaborative, synthetic approach enhances the accuracy and comprehensiveness of marine data collection. The ongoing development of more marine autonomous systems (MAS) is expected to further improve this integration, showcasing the positive evolution of technology in facilitating holistic marine observations. As examples of the gaps that still need to be addressed to enhance the data collection, HFR systems do not yet cover the Baltic and the Black Seas, while there are several gaps of coverage in the North and the Mediterranean Seas where they are very few, mainly located in a couple of EU countries’ waters (Tintoré et al., 2019; Rubio et al., 2021). Among the 107 HFRs included in the current EuroGOOS task team inventory (July 2021), only 45 HFRs (~42%) are connected to the HFR node and sending data in near real-time (Rubio et al., 2021). For ASVs, true autonomy is still unavailable as they still require a significant deal of human interaction and support. These platforms are still quite expensive and complex. Conversely, systems of numerous, small, and inexpensive observing platforms can increase spatio-temporal coverage, but only for a limited number of ocean variables because small size and limited power implies a limited scientific payload (Cianca et al., 2023) (see detailed gaps of other networks in Hassoun et al., 2023). In terms of governance, EuroGOOS offers a structured mechanism where its Task Teams serve as a pivotal platform for fostering cooperation, harmonization, and coordination across marine observational efforts. However, several networks still lack defined Terms of Reference (ToR), as is the case with ASV (Karstensen et al., 2020). ToR documents are vital as they outline a network’s scope, purpose, structure, goals, and operational guidelines. While network Key Performance Indicators (KPIs) are still being developed for Gliders and are in progress for Vessels (Ferrybox and MetOcean) and Eulerian methods, additional KPIs are expected to be established within JERICO-S3 (Karstensen et al., 2020). These governance-related gaps, including the absence of ToRs for specific networks and the need for improved inclusivity and collaboration, pose challenges to the effectiveness of ocean monitoring initiatives (Karstensen et al., 2020; JERICO-S3 D4.1, 2021; JERICO-S3-D8.1, 2021).
Moreover, the sustainability of observations within the EOOC faces significant challenges stemming from funding limitations, coordination issues, and infrastructure vulnerabilities. Currently, a lack of sustained funding for ocean observations is a pressing concern, with approximately 70% of data reliant on short-term research projects (i.e., 3-5-year funding cycles), leading to uncertainties and hampering network coordination and management efforts (Buch et al., 2017; Tanhua et al., 2019a; EMB, 2021; Révelard et al., 2022). Declining numbers of observational sites and platforms exacerbate this problem due to aging instruments, changing scientific priorities, and reduced funding opportunities (Buch et al., 2017). For insurance, the closure of the Carbon Dioxide Information Analysis Center ocean trace gases section (CDIAC-Oceans) in the U.S. Department of Energy’s Oak Ridge National Laboratory exposed vulnerabilities in data management and highlighted the need for a more sustainable approach (Álvarez et al., 2023). Gaps in centralized data management persist, and some networks, like Argo, face challenges in the data flow process, risking sustainability in the near future (Buch et al., 2017). Additionally, achieving a balance between incorporating new marine observational technology and maintaining legacy components presents a significant challenge, requiring careful investment decisions and calibration processes (Tanhua et al., 2019a). These sustainability gaps extend to local and regional scales, such as the Central-Eastern Mediterranean and Northern African coasts, where in-situ observations are lacking, and data policies may be inadequate, jeopardizing long-term sustainability and hindering coordination efforts (Tintoré et al., 2019).
3.10 Recommendations for observing networks and sustainability of observations
• Invest in integrated national systems to maximize resource use, coordination, and harmonization across Member States, and ensure diverse marine ecosystem coverage through coordination with regional conventions and organizations.
• Create a body involving national and EU funding entities to ensure sustained funding and balanced strategy development, formulating a long-term plan covering all aspects of the Ocean Observing System, including instrument purchase and operational costs while also integrating with centralized infrastructures like ERICs to secure consistent funding.
• Improve sampling in deep ocean and marginal seas to better understand heat flux, thermohaline circulation, oxygen distribution, and biological components.
• Aim for the deployment of 1000 floats globally, each equipped with six biogeochemical sensors, under the One-Argo program.
• Create extensive databases to address spatial gaps in networks and increase contributions to programs like OceanGliders.
• Develop and enhance the HFR network for coastal services and establish multidisciplinary fixed-point time-series networks globally.
• Promote the use of EDMO Catalogue for identifying institutions and create an Environmental Sensitivity Index (ESI) map.
• Set aside a significant percentage of each network’s budget for uninterrupted execution, data quality control, and accessibility.
• Emphasize the economic importance of ocean observing for societal benefits and explore innovative funding models.
• Develop an integrated approach that encompasses enhancing governance frameworks for data interoperability and openness, aligning R&D with societal and environmental priorities in line with EU policies, ensuring system inclusivity, and fostering robust national and international collaboration among research, meteorological, and environmental stakeholders.
3.11 Technological, research and development aspects
Technological advancements have enabled the concurrent deployment of diverse platforms, each equipped with a comprehensive suite of sensors, facilitating simultaneous observations of various environmental variables across multiple spatial and temporal scales. This integration enhances our capacity for detailed analysis of critical oceanographic processes. Significant progress in the development of autonomous unmanned vehicles now allows for extensive exploration over vast distances and depths previously inaccessible, overcoming substantial logistical challenges. However, this technological evolution introduces new complexities. For example, there is a pressing need for standardization of instruments and methodologies to maintain consistency across various platforms and regions. This challenge is particularly acute in the development and testing of new sensors for biogeochemical and biological observations. These sensors, essential for comprehensive marine understanding, often have issues of accuracy, portability, and affordability (Buch et al., 2017). Furthermore, high costs associated with advanced instruments and sensors restrict their usage, especially in regions and periods where manned platforms are not accessible. The geographical distribution of automated observation systems reveals significant imbalances across European coastal systems. For example, many regions remain under-sampled for EOVs, particularly in the Mediterranean Sea, where there is a significant lack of sensors for critical parameters like wave data, sea level rise, surface currents, and sea surface temperature (Tintoré et al., 2019; Karstensen et al., 2020). This under-sampling results in inadequate data for effective assimilation in numerical models, even in areas where sensors are available. Moreover, Autonomous Surface Vehicles (ASVs) and other similar systems face difficulties in achieving true autonomy, as they continue to require significant human support. Despite their potential, these systems are often costly and complex, limiting their widespread adoption and application (Cianca et al., 2023). In the realm of research and development, funding inadequacies present a major obstacle, particularly in public institutions. There is a lack of investment directed towards the development of innovative tools and technologies that would be accessible and usable by the European ocean observing community. Although there are remarkable advancements including innovations in chemical sensor arrays for monitoring deep ocean floor disposal sites and novel sensing systems for detecting contaminant content in the ocean (Jeremic and Nehorai, 1998; Kedar and Arnon, 2006), these technologies are not widely used within the EOOC and not integrated in operational platforms. The majority of research and development expenditure is concentrated in the business enterprise sector, with minimal growth observed in higher education, government, and private non-profit sectors over the past 15 years (EuroStat, 2020). According to EuroStat (2020), the EU gross domestic expenditure on R&D in relation to GDP (R&D intensity) has shown only modest growth over the past 15 years. Although the EU economy is facing increasing global competition, only 2.19% of GDP was spent on R&D in the EU in 2018. Furthermore, the surge in data from autonomous observation systems has introduced new challenges in big data quality, transfer, exchanges, mining and visualization. This highlights the urgency for enhanced tools and methodologies to manage, document, standardize, and use the increasing quantity and quality of ocean data (Buch et al., 2017; EuroStat, 2020; Cianca et al., 2023). Moreover, there is still a coordination, dialogue and partnership gap between technology developers (industry) and the observational community (scientific users), an obstacle in efficiently addressing specific needs of ocean observation.
3.12 Recommendations for technological, research and development aspects
• Invest in robust, miniaturized, and cost-effective ocean observation technologies like ROVs and AUVs, and develop low-pollution, energy-efficient, and minimally invasive technologies to ensure sustainable ocean observing practices. Focus on autonomous platforms for efficient biodiversity and fisheries assessment and contaminants’ monitoring in regions under anthropogenic pressures.
• Develop technologies for exploring biodiversity hotspots and sustainable harvesting of marine bioresources powered by AI and advanced image-based techniques. This includes innovations in aquaculture, biological indicators, and synthetic biology applications while supporting technologies that combine abiotic and biotic information, such as -omic tools, imaging techniques, and hydro-acoustic approaches, to enable real-time, high-resolution ecosystem monitoring.
• Invest in the collaboration between different platforms under a single system to improve communication between platforms, exchange of data, maintain data accuracy and common data formats and protocols, and integrate these data in assimilation systems for more refined forecasts.
• Prioritize funding for research and development programs that focus on cutting-edge intersectional innovations, such as the Horizon project, to address key societal and environmental challenges, and maintain consistent investment in innovation to keep the observing system updated with new variables, improved quality, and minimal environmental impact.
• Invest in innovative underwater data transfer and autonomous deep-sea sampling technologies to improve the efficiency of ocean observations. Support the upgrade and expansion of existing monitoring infrastructure and collaborate with initiatives like CMEMS, EMODnet, and SeaDataNet for improved data management and archival.
• Ensure effective technology deployment by offering extensive training in the use of new sensors and technologies and enhance regulatory frameworks to facilitate the sharing of marine biotechnology data and resources, and establish effective public-private partnerships.
• Establish standardized protocols and quality assurance measures for new technologies (sensors and instruments) to guarantee data accuracy and reliability.
3.13 Marine policies and legislations
The EOOC has been instrumental in shaping marine policies and legislation, aiding the European Union in fulfilling its sustainable ocean governance goals. Its crucial contributions include supporting the implementation of key directives and policies, such as the MSFD, the WFD and the Common Fisheries Policy (CFP). The EOOC provision of vital data and insights has enabled policymakers to set and monitor ambitious environmental targets, crucial for achieving Good Environmental Status (GES) in marine ecosystems. Additionally, the EOOC has bolstered international cooperation in ocean governance, aligning EU efforts with global initiatives like the United Nations Sustainable Development Goals and the Paris Agreement. These efforts have enhanced the EU’s role as a global leader in responsible ocean stewardship and have been vital in developing effective marine policies for the protection and sustainable management of European Seas. However, the EOOC faces significant challenges in upgrading its ocean observation capabilities due to gaps in marine policies and legislations. A major hindrance is the complexity in data sharing, particularly in exclusive economic zones (EEZs). There is an absence of unified approaches to data collection, leading to discrepancies in data formats, which affects the synthesis and availability of marine data (Guidi et al., 2020). For example, high-resolution Vessel Monitoring System (VMS) and Electronic Recording System (ERS) data are restricted due to policy constraints, limiting the assessment of fisheries impact (EMODnet, 2018). Non-sharing of crucial data, such as tide gauge data by certain countries surrounding European Seas, obstructs a comprehensive understanding of phenomena like sea level changes, especially in politically sensitive regions like the Mediterranean Sea (Karstensen et al., 2020). Additionally, there is a disconnect between environmental policies and marine research priorities, leading to insufficient funding and resources for ocean observing activities (AtlantOS, 2017). This is particularly related to the gap of direct and relevant consultations on marine observations’ matters, for instance between the observing community and DG MARE16. This gap extends to the consideration of marine geohazards in policies at local, national, and EU levels, resulting in inadequate risk mitigation strategies (Kopp et al., 2021). Also, the underdevelopment of regulatory frameworks for technologies such as ASVs adversely affects the safe deployment of these innovations in the marine sector (Cianca et al., 2023). Moreover, the lack of a dedicated framework for quality assurance in marine environmental monitoring underscores the need for standardized protocols and guidelines. Additionally, the absence of consistent pan-European or regional legal frameworks addressing oil and gas activities at sea challenges preventive measures and incident management. In regions with increased oil and gas activity, such as the Mediterranean Sea, this gap is particularly problematic for managing oil-related incidents (Alves et al., 2016; Tintoré et al., 2019). Another example of incoherence is reflected by the presence of over 300 existing environmental policies that often lack coherence with marine research policies, resulting in them not being a priority in national agendas (AtlantOS, 2017). Certain measures under the MSFD, like marine litter and underwater noise, lack broader integration into other EU legislations and international agreements (SWD, 2020). In general, policy support, crucial for the development of the marine sector including agreements on access to marine bioresources and the establishment of common licensing for the use of these resources, is still missing (EMB, 2017). Also, there is a gap in establishing public-private partnerships, necessary to address funding challenges in the bio-economy (EMB, 2017).
3.14 Recommendations for marine policies and legislations
• Enhance national research policies to embed ocean observation as a crucial component, ensuring alignment with the objectives of the UN Decade for Ocean Science. This can be addressed through creating a more efficient consultation with DG MARE on ocean observations to better shape related EU regulations and future funds’ priorities.
• Implement a sustainable, comprehensive system that aligns with European and international policy requirements. This should involve regular consultations and mapping of policy needs to ensure the system delivers relevant and accurate ocean data.
• Prioritize open data exchange at the European level, especially from countries currently restricting data sharing, to improve data management, accessibility, and long-term retention of ocean observations.
• Advance the monitoring assessment framework and the Checkpoint network for periodic evaluation of monitoring systems at European marine basin scales.
• Incorporate marine geohazards in relevant policies at all levels for effective risk mitigation and sustainable blue economy development.
• Improve the integration of scientific research into policymaking, bridging gaps in timescales, objectives, methods, and language for better science-based decision-making.
• Establish international regulations for emergent networks and technologies, such as ASVs, to ensure safe operations across waters, accounting for the unique characteristics of unmanned vessels.
• Ensure funding structures are consistent with long-term strategies, promoting collaboration, data sharing, and capacity building.
3.15 Coordination, management and reporting
Coordination within the EOOC has notably improved, incorporating effective management and reporting mechanisms that streamline operations. This evolution is highlighted by the establishment of EuroGOOS within the GOOS structure, and the development of Regional Operational Oceanographic Systems (ROOSs)17, which exemplifies Europe’s proactive approach to enhancing maritime coordination. These initiatives, acknowledging the need for robust coordination mechanisms, have significantly contributed to the development and joint service production across European maritime regions. By integrating efforts with OceanOPS18, EMODnet, and EOOSõ19, and feeding marine data into pan-European portals, these structures have added substantial value to European cooperation. Furthermore, the adoption of enhanced reporting practices, including tracking metrics and key performance indicators, supports the continuous evaluation and improvement of these governance structures and ocean observing activities. This approach, a successful bottom-up initiative complemented by the GOOS Regional Alliances (GRAs)20, EuroGOOS and MONGOOS21, addresses the fragmentation and complexity of the broader European marine observation landscape, marking a significant step forward in the region’s oceanographic coordination efforts. Despite this significant progress, EOOC is still witnessing many gaps that, if well addressed, will transform it into a more efficient and transparent system that is better equipped to fulfill its vital functions. A stark underrepresentation of regional coordination is evident within the EOOC. For example, only a meager 18 out of 363 marine biological monitoring programs in Europe demonstrate regional coordination (Ratnarajah, 2021). This glaring shortfall points to a widespread fragmentation due to the largely isolated operations of national and international efforts. EMODnet exemplifies this disjunction, suffering from a pronounced lack of collaboration between thematic centers, critically undermining the delivery of unified in-situ observations (EMODnet, 2018). The EOOC is still having entrenched organizational silos, a byproduct of the prevalent non-collaborative and competitive ethos among scientists and institutions. This entrenched culture not only erodes research quality but also significantly impairs the well-being of researchers (Révelard et al., 2022). Moreover, the governance landscape of European ocean observation is characterized by fragmentation, with a conspicuous absence of clear leadership and coordination among myriad institutions at national and European levels (Davidson et al., 2019). More specifically, the national landscape is characterized by a diverse array of entities such as research institutions, universities, and NGOs conducting observations independently, leading to uncoordinated efforts and resource inefficiencies. This fragmentation results in competition for limited funding, often causing overlap and resource wastage. At the EU level, this disjointed national representation impacts cohesive participation in structures like EuroGOOS, as multiple individual research centers with varying priorities and strategies represent countries instead of a unified national voice. Also, the reporting processes from EU Member States are mired in inefficiencies, notably marked by delays and an outdated reliance on paper-based methods. The variation in reporting quality across Member States is a testament to the inadequate coordination and communication, and a lack of a unified understanding of objectives (SWD, 2020). Further, a significant disconnect is apparent in the integration of ocean observing networks, predominantly due to their independent operations and lack of basin-wide and global coordination. The fragmented national institutional landscape further exacerbates the fragility in the ocean observation value chain (EMB, 2021). The governance of the EOOC witnesses duplications and a lack of clarity, accountability, and effective data sharing, pointing to a dire need for a comprehensive governance architecture (Tanhua et al., 2019a). Besides, the prevailing research culture, overly fixated on bibliometric indicators for evaluation, undermines holistic integration and data sharing. This hypercompetitive environment, coupled with inadequate management and leadership, is linked to detrimental research practices, adversely impacting researcher well-being and the quality of scientific output (Van Noorden, 2018; Bleasdale, 2019).
3.16 Recommendations for coordination, management and reporting
• Invest in a centralized coordination entity that would have a centralized governance structure with competent teams for strategic, executive, and operational management, such as EOOS for instance. This structure should streamline processes, ensure transparency, and facilitate collaboration across disciplines and countries and establish key performance indicators for observing platforms and data usage, conduct regular reviews (e.g., every 5 years) to assess the effectiveness of the governance structure and the ocean observing activities.
• Invest in national coordination to enhance management and reporting nationally and at the EU scale of the various ocean observing and forecasting activities.
• Partner with industries specializing in cutting-edge technologies like AI and big data mining to enhance capabilities. Incorporate ethical principles in governance, emphasizing free access to research, environmental respect, and cultural reciprocity.
• Promote a unified environment with shared vision and common priorities among all stakeholders, encouraging collaboration and integration between European and non-EU countries for a global ocean observing system.
• Develop a new recognition system that values diverse contributions, including societal impact and data management. Implement effective outreach to highlight the societal importance of ocean observing and its role in achieving sustainable development goals.
3.17 Applications (wind farm, oil spills)
The EOOC supports various marine vital applications, including aquaculture, the blue economy sector, oil spill response, and wind farm development. It made remarkable progress in monitoring wind farms installations and oil spill detection and response, offering crucial insights into their environmental impacts, enhancing the efficiency of renewable energy production, and oil spill management. However, to maximize its effectiveness in these vital marine sectors, several significant gaps in the EOOC capabilities require attention. Regarding wind farm siting and energy production, a deficiency lies in the availability of detailed bathymetry data, which are essential for offshore wind farm design, coastal protection, shipping, and coastal/offshore engineering. This limitation hampers accurate assessments and planning for wind farm installations (EMODnet, 2018). Additionally, the scarcity of publicly accessible wind profiler observations further complicates wind farm siting, as available data often do not reach the necessary heights (EMODnet checkpoints 2016-2020). Wind farm monitoring efforts tend to lack comprehensive spatial coverage, primarily focusing on individual sites and neglecting the broader assessment of cumulative impacts and interactions between multiple wind farms (EMODnet checkpoints 2016-2020). Moreover, there is an insufficient understanding of the potential impacts of floating wind turbines on seabeds across various regions and a lack of wind strength data at appropriate spatial and temporal resolutions (EMODnet, 2016; 2018; 2020). Insufficient data also hinder our understanding of environmental impacts on marine ecosystems, biodiversity, and migratory species related to wind farm development (EMODnet, 2016; 2018; 2020). Key parameters such as those related to wind farm siting, aggregate extraction, offshore installations, dumped munitions, and dredging lack the needed spatial coverage. Additionally, data accessibility, usability, referencing, and format variations challenge access and data sharing from wind farm monitoring efforts (EMODnet, 2018; 2017). The absence of standardized monitoring protocols further hampers data comparability and integration across different wind farm sites (EMODnet, 2018). Further gaps in monitoring encompass noise, electromagnetic fields, physical seabed alterations, and potential changes in hydrodynamics (Halpern et al., 2015). Limited integration of wind farm monitoring data with other ocean observing systems and data, such as those related to biology, ecology, fisheries, and migration routes, do not help having accurate holistic projections (Willsteed et al., 2017). Another critical area requiring attention is the observation and response to oil spills, particularly in cold environments like the Arctic and sub-Arctic regions. There exists a significant knowledge gap concerning oil spill behavior and fate in these sensitive ecosystems (EMODnet, 2020). The integration and accessibility of data related to oil spills often suffer from fragmentation, affecting horizontal and vertical resolutions (EMODnet, 2016). Challenges in locating freely accessible wind and current data at a sufficient spatial scale, for example in the North Sea, contribute to this issue (EMODnet, 2016), reflecting the lack of robust real-time monitoring and early warning systems for oil leakage and spills, impacting the ability to respond rapidly and minimize environmental impacts.
3.18 Recommendations for applications (wind farm, oil spills)
• Enhance data collection and monitoring: Invest in comprehensive biodiversity and distribution data for marine species, focusing on cold regions and sea-ice areas to inform ecological impact assessments and oil spill response strategies. Improve the resolution of bathymetry data to support detailed offshore wind farm siting and coastal engineering projects.
• Develop robust monitoring systems: Implement real-time monitoring systems for oil spills, emphasizing areas at high risk for catastrophic ecological impacts. Upgrade oil spill monitoring techniques by enhancing horizontal and vertical data resolutions, particularly near coastlines, and ensure high-quality data availability for wind patterns and currents to facilitate accurate spill trajectory modeling.
• Foster collaborative and regulatory frameworks: Establish collaborative frameworks among EU Member States and stakeholders for requirement settings, shared data, expertise, and best practices in monitoring and responding to oil spills. Develop consistent legal frameworks for regulating offshore oil and gas activities.
• Integrate risk assessment and environmental impact studies: Create comprehensive risk assessment models that incorporate environmental vulnerability and species sensitivity for oil spills. Conduct research on the ecological impacts of wind turbines and wind farm installations on the seabed to guide future design and installation practices.
• Standardize data collection and accessibility: Enhance data quality and standardization, particularly for wind and wave conditions relevant to wind farm siting. Utilize advanced technologies and cloud facilities for efficient data processing and accessibility.
• Ensure EOOC’s activities align with EU energy policies, focusing on renewable energy development, particularly marine wind farms, to reduce energy import dependency and promote sustainable energy practices.
3.19 Users’ satisfaction and ocean literacy
The EOOC prioritizes user satisfaction through ongoing engagement, feedback, and adaptation to meet user needs. Open data policies ensure transparency and accessibility for research and decision-making. In parallel, ocean literacy and public engagement efforts have expanded through education programs, citizen science, and strategic collaborations, fostering broader awareness and understanding of ocean science. Nevertheless, the EOOC is still witnessing important gaps in addressing users’ needs, particularly in delivering products and services effectively. These gaps also extend to ocean literacy and awareness, impacting the overall utility and perception of services provided by the EOOC by various audiences. A primary concern is the limited engagement of actors/users from certain sectors such as oil and gas, offshore wind, and aquaculture with the EOOC services (i.e., data portals). This issue points to a need for improved accessibility and communication of the system’s benefits to a broader range of users (JERICO-S3 D9.1, 2021; Kopp et al., 2021). The geographical concentration of users in certain countries and the disparities among regions reflect the need for more tailored approaches to meet diverse users’ needs. This includes addressing the challenges in integrating biodiversity into models and linking ecosystems to socio-economic models, as well as difficulties in sharing business-critical data in commercial sectors, such as in aquaculture, although innovative initiatives like AquaCloud have shown promise (Heymans et al., 2018; Guidi et al., 2020). Moreover, gaps in downstream products for end-users are evident, stemming from data adequacy issues and inconsistencies across data portals. This results in challenges in policy visibility and responsiveness. For example, in an assessment conducted by the EMODnet Mediterranean checkpoint (EMODnet, 2017), it is demonstrated that over 60% of the core products contributing to the monitoring of the Mediterranean Sea are totally or partly inadequate for their non-compliance with INSPIRE Catalog principles, and above 40% of the input data-sets contributing to the monitoring of the Mediterranean Sea are partly or totally inadequate for policy visibility, delivery mechanism, data policy and responsiveness (Tintoré et al., 2019). Furthermore, the varied contents in data portals, including station details and metadata information, hinder accurate site-by-site comparisons due to the absence of unique identifiers. Thus, it can be difficult to monitor how many stations (e.g., tide gauge stations) are actively contributing to a network or to perform a gap analysis (Pérez-Gómez et al., 2021b). In terms of ocean awareness and literacy, the EOOC struggles to adequately address key issues like ocean acidification, carbon sinks, oil spills, harmful algal blooms, biotoxins, and non-indigenous species. These topics are often lost within broader subjects, thereby hindering specific attention and understanding (Seys et al., 2022). The absence of well-trained communication teams within EOOC organizations limits the system’s ability to effectively disseminate research findings. For instance, less than 5% of the workforce in European Marine Board Member organizations is typically engaged in marine science communication, underscoring the need for more professionals skilled in this area (Seys et al., 2022). Furthermore, current communication channels fall short in effectively engaging and collaborating with regional policy stakeholders (Tintoré et al., 2019).
3.20 Recommendations for users’ satisfaction and ocean literacy
• Improve definitions and identification of data, products, and services (e.g., including establishing unique identifiers, example for tide gauge stations) and enhancing data visualization techniques tailored to diverse user needs.
• Focus on a user-centric approach for data delivery, continuously aligning EOOC services with changing societal, technological, and scientific demands. This includes developing innovative and integrated metrics for evaluating data services effectiveness.
• Strengthen collaborations with various stakeholders, including industries, public authorities, and manufacturers, to enhance platform innovation. Establish forums or User Committees for guiding data portal governance and addressing societal needs.
• Streamline the value chain into core and downstream services, ensuring smooth operation and compliance with FAIR data policies. Develop measurable Key Performance Indicators focusing on scientific excellence and operational sustainability.
• Implement robust communication strategies to maximize visibility and use of products and services. This includes improving educational and outreach initiatives to foster public understanding of ocean influences and decision-making in ocean matters.
• Incorporate comprehensive metrics for evaluating physical, biogeochemical, biological/ecological, and socio-economic aspects, including user access and feedback. Fund data and technology training to equip researchers with advanced skills in data science, effective data management and communication.
• Regularly assess and adapt coastal and ocean monitoring systems to evolving user needs, focusing on developing Use Case Products to cover a broad range of requirements.
• Enhance science communication to balance the presentation of marine science issues, fostering a culture that integrates science communication with research. Engage potential users from the Blue Economy and marine communities, ensuring data portals cater to their specific needs.
4 Conclusion
The European Ocean Observing Community (EOOC) has made significant progress, yet significant gaps persist. These gaps encompass the observation and forecasting of critical ocean phenomena, regional coordination, governance fragmentation, communication gaps, and the need to better serve users and engage the public. Addressing these gaps is imperative to protect Europe’s seas, coastal communities, economies, and ecosystems. Recommendations include improving spatio-temporal coverage, increasing research and development investment, enhancing coordination mechanisms, addressing reporting disparities, refining governance structures, and harmonizing data policies. Additionally, strengthening communication efforts through dedicated marine science teams, engaging regional policy stakeholders, promoting citizen science, and fostering global collaboration is crucial for public awareness and support. Capacity building, specialized training, open access, and data sharing are essential priorities. Implementing these actions, to achieve the UN Ocean Decade’s goals, will fortify the EOOC, raise public awareness, and enable sustainable ocean management, securing a healthier future for European Seas.
Data availability statement
The original contributions presented in the study are included in the article/supplementary material. Further inquiries can be directed to the corresponding author.
Author contributions
AH: Conceptualization, Investigation, Methodology, Visualization, Writing – original draft, Writing – review & editing. TT: Conceptualization, Funding acquisition, Project administration, Supervision, Writing – review & editing. IL: Conceptualization, Writing – review & editing. EH: Conceptualization, Writing – review & editing. GP: Writing – review & editing. JK: Writing – review & editing.
Funding
The author(s) declare financial support was received for the research, authorship, and/or publication of this article. This work has been supported by the European Union, through the EuroSea project, in the context of the Horizon 2020 research and innovation programme under grant agreement No 862626.
Conflict of interest
The authors declare that the research was conducted in the absence of any commercial or financial relationships that could be construed as a potential conflict of interest.
The author(s) declared that they were an editorial board member of Frontiers, at the time of submission. This had no impact on the peer review process and the final decision.
Publisher’s note
All claims expressed in this article are solely those of the authors and do not necessarily represent those of their affiliated organizations, or those of the publisher, the editors and the reviewers. Any product that may be evaluated in this article, or claim that may be made by its manufacturer, is not guaranteed or endorsed by the publisher.
Footnotes
- ^ Descriptors under the Marine Strategy Framework Directive - European Commission (europa.eu).
- ^ Phenomena & Threats | CMEMS (copernicus.eu).
- ^ ICES.
- ^ General Fisheries Commission for the Mediterranean - GFCM | Food and Agriculture Organization of the United Nations (fao.org).
- ^ Home - Eurostat (europa.eu).
- ^ Document – Global Ocean Observing System (goosocean.org).
- ^ SDG 14.3.1 data portal (iode.org).
- ^ Introduction - MEMENTO (geomar.de).
- ^ Essential Ocean Variables – Global Ocean Observing System (goosocean.org).
- ^ JPI Oceans (jpi-oceans.eu).
- ^ Science for Good Environmental Status | JPI Oceans (jpi-oceans.eu).
- ^ SeaDataNet - SeaDataNet.
- ^ International Oceanographic Data and Information Exchange (IODE).
- ^ EuroGOOS member organisations and contact persons - EuroGOOS.
- ^ Minke – Metrology for Integrated marine maNagement and Knowledge-transfer nEtwork.
- ^ Maritime Affairs and Fisheries - European Commission (europa.eu).
- ^ Regional Operational Oceanographic Systems (ROOS) - EuroGOOS.
- ^ OceanOPS – Global Ocean Observing System (goosocean.org).
- ^ European Ocean Observing System (eoos-ocean.eu).
- ^ GOOS Regional Alliances – Global Ocean Observing System (goosocean.org).
- ^ Mongoos | The Mediterranean Oceanographic Network for the Global Ocean Observing System (eurogoos.eu).
References
Aguzzi J., Chatzievangelou D., Fanelli E., Danovaro R., Flögel S., et al. (2019). New high-tech flexible networks for the monitoring of deep-sea ecosystems. Environ. Sci. Technol. 53, 6616–6631. doi: 10.1021/acs.est.9b00409?casa_token=NMMW2XSX670AAAAA%3AR6PIBO9BYzgwqKKkBzpEjhNmI8QVMlCt38dpvXiFp_y2E4RBB4GBdI-H-rBaCjJEYNBK236KisQROg
Álvarez M., Catalá T. S., Civitarese G., Coppola L., Hassoun A. E., Ibello V., et al. (2023). “Mediterranean Sea general biogeochemistry,” in Oceanography of the Mediterranean Sea (Elsevier), 387–451. Available at: https://www.sciencedirect.com/science/article/abs/pii/B9780128236925000042.
Alves T. M., Kokinou E., Zodiatis G., Radhakrishnan H., Panagiotakis C., Lardner R. (2016). Multidisciplinary oil spill modeling to protect coastal communities and the environment of the Eastern Mediterranean Sea. Sci. Rep. 6, 36882. doi: 10.1038/srep36882
Ardhuin F. (2018). Large-scale forces under surface gravity waves at a wavy bottom: A mechanism for the generation of primary microseisms. Geophysical Res. Lett. 45, 8173–8181. doi: 10.1029/2018GL078855
AtlantOS (2017). Available online at: https://atlantos-h2020.eu/download/Full-document-European-Strategy-for-All-Atlantic-Ocean-Observing-System.pdf.
Bakker D. C., Pfeil B., Landa C. S., Metzl N., O'brien K. M., Olsen A., et al. (2016). A multi-decade record of high-quality fCO2 data in version 3 of the Surface Ocean CO 2 Atlas (SOCAT). Earth System Sci. Data 8, 383–413. doi: 10.5194/essd-8-383-2016
Bleasdale B. (2019). Researchers pay the cost of research. Nat. Mater. 18, 772–772. doi: 10.1038/s41563-019-0443-z
Bonaduce A., Pinardi N., Oddo P., Spada G., Larnicol G. (2016). Sea-level variability in the Mediterranean Sea from altimetry and tide gauges. Climate Dynam. 47, 2851–2866. doi: 10.1007/s00382-016-3001-2
Boulze H., Korosov A., Brajard J. (2020). Classification of sea ice types in Sentinel-1 SAR data using convolutional neural networks. Remote Sens. 12, 2165. doi: 10.3390/rs12132165
Buch E., Palacz A., Karstensen J., Fernandez V., Dickey-Collas M., Borges D., et al. (2017). AtlantOS – 633211, Deliverable number D.1.3. Capacities and Gap analysis. Available online at: https://atlantos-h2020.eu/download/deliverables/1.3%20Capacities%20and%20Gap%20analysis.pdf.
Castro-Jimenez J., Barhoumi B., Paluselli A., Tedetti M., Jiménez B., Muñoz-Arnanz J., et al. (2017). Occurrence, loading, and exposure of atmospheric particle-bound POPs at the African and European edges of the Western Mediterranean Sea. Environ. Sci. Technol. 51, 13180–13189. doi: 10.1021/acs.est.7b04614
Cianca A., Barrera C., de Sousa J. B., Burris J., Waldmann C. (2023). D3.5. ASV-Network structure and roadmap (Revised version). Available online at: https://oceanrep.geomar.de/id/eprint/59165/13/D3.5_ASV-Network_structure_and_roadmap_revised_resubmitted.pdf.
Cordier T., Esling P., Lejzerowicz F., Visco J., Ouadahi A., Martins C., et al. (2017). Predicting the ecological quality status of marine environments from eDNA metabarcoding data using supervised machine learning. Environ. Sci. Technol. 51, 9118–9126. doi: 10.1021/acs.est.7b01518?casa_token=jdeAJXf8YYcAAAAA%3AnREVpEepVZczyqoLDNDO9osAA9qmHwMnE3QFIhPr3ClCWXSMKKBjAAcSz0xSerCygSDmh2Koa4KxYA
Davidson F., Alvera-Azcarate A., Barth A., Brassington G. B., Chassignet E. P., Clementi E., et al. (2019). Synergies in operational oceanography: the intrinsic need for sustained ocean observations. Front. Mar. Sci. 6. doi: 10.3389/fmars.2019.00450/full
De Gelis I., Colin A., Longépé N. (2021). “Prediction of categorized sea ice concentration from Sentinel-1 SAR images based on a fully convolutional network,” in IEEE Journal of Selected Topics in Applied Earth Observations and Remote Sensing, Vol. 14. 5831–5841.
de Young B., Visbeck M., de Araujo Filho M. C., Baringer M. O., Black C. A., Buch E., et al. (2019). An integrated all-atlantic ocean observing system in 2030. Front. Mar. Sci. 6. doi: 10.3389/fmars.2019.00428/full
EMB (2021). Sustaining in situ ocean observations in the age of the digital ocean: EMB policy brief no. 9 (Oostende: European Marine Board). doi: 10.5281/zenodo.4836060
EMB. (2017). European Marine Board and Marine Biotechnology ERA-NET. Marine Biotechnology: Advancing Innovation in Europe’s Bioeconomy. EMB Policy Brief No. 4, September, 2017.
EMODnet (2016). Growth and Innovation in the Ocean Economy: North Sea Checkpoint. Available online at: https://emodnet.ec.europa.eu/sites/emodnet.ec.europa.eu/files/public/Final%20report_NorthSea.pdf.
EMODnet (2017). Growth and innovation in ocean economy Gaps and priorities in sea basin observation and data D12.5 Version: V3. Available online at: https://emodnet.ec.europa.eu/sites/emodnet.ec.europa.eu/files/public/D11.4-draft18.pdf.
EMODnet (2018a). Atlantic EMODnet Sea-basin checkpoints Sea Basin Checkpoint Lot 2 : Atlantic, D 14.2.1, Version: 2.0, Date: 17/07/2018.
EMODnet (2018b). Baltic Checkpoint Final Report/EMODnet Sea-basin checkpoints Lot n° 3 – Baltic , EASME/EMFF/2014/1.3.1.4. Available online at: https://emodnet.ec.europa.eu/sites/emodnet.ec.europa.eu/files/public/BSCP_%20FinalReport_revised.pdf.
EMODnet (2018c). Black Sea Checkpoint Second Data Adequacy Report, D 15.4 Version: V7 Date: 23/04/2018. Available online at: https://emodnet.ec.europa.eu/sites/emodnet.ec.europa.eu/files/public/D15.4_DAR2_v7_FINAL.pdf.
EMODnet (2020). Arctic Ocean EMODnet Sea-basin checkpoints Lot n° 1 Arctic , Final Report. Available online at: https://emodnet.ec.europa.eu/sites/emodnet.ec.europa.eu/files/public/FinalReportArctic%20new%20style.pdf.
EuroStat (2020). Sustainable development in the European Union 2020. Available online at: https://ec.europa.eu/eurostat/documents/3217494/11011074/KS-02-20-202-EN-N.pdf/334a8cfe-636a-bb8a-294a-73a052882f7f?t=1592994779000.
Glöckner F. O., Gasol J. M., McDonough N., Calewaert J. B. (2012). Marine microbial diversity and its role in ecosystem functioning and environmental change. European Science Foundation. Available at: http://hdl.handle.net/10261/65386
GOA-ON (2019). Global Ocean Acidification Observing Network (GOA-ON) Implementation Strategy 2019. Available online at: www.goa-on.org.
Guidi L., Fernandez Guerra A., Canchaya C., Curry E., Foglini F., Irisson J.-O., et al. (2020). Big Data in Marine Science. Eds. Alexander B., Heymans J. J., Muñiz Piniella A., Kellett P., Coopman J. (Ostend, Belgium: Future Science Brief 6 of the European Marine Board). Available at: https://www.marineboard.eu/sites/marineboard.eu/files/public/publication/EMB_FSB6_BigData_Web_v4_0.pdf, ISBN: ISBN: 9789492043931. ISSN: 2593-5232.
Halpern B. S., Frazier M., Potapenko J., Casey K. S., Koenig K., Longo C., et al. (2015). Spatial and temporal changes in cumulative human impacts on the world’s ocean. Nat. Commun. 6, 1–7. doi: 10.1038/ncomms8615
Hassoun A. E. R., Bantelman A., Canu D., Comeau S., Galdies C., Gattuso J. P., et al. (2022). Ocean acidification research in the Mediterranean Sea: Status, trends and next steps. Front. Mar. Sci. 9. doi: 10.3389/fmars.2022.892670/full
Hassoun A. E. R., Tanhua T., Petihakis G., Lips I., Heslop E. (2023). Report on gaps in the European ocean observing and forecasting system. EuroSea Project. doi: 10.3289/eurosea_d1.9
Hassoun A. E. R., Ujević I., Mahfouz C., Fakhri M., Roje-Busatto R., Jemaa S., et al. (2021). Occurrence of domoic acid and cyclic imines in marine biota from Lebanon-Eastern Mediterranean Sea. Sci. Total Environ. 755, 142542. doi: 10.1016/j.scitotenv.2020.142542
Heymans J. J., Skogen M., Schrum C., Solidoro C. (2018). Enhancing Europe’s capability in marine ecosystem modelling for societal benefit. Eds. Larkin K. E., Coopman J., Muñiz Piniella A., Kellett P., Simon C., Rundt C., Viegas C., Heymans J. J. (Ostend, Belgium: Future Science Brief 4 of the European Marine Board), 32, ISBN: ISBN: 9789492043580. ISSN: 2593-5232.
Hoppe C. J. M., Langer G., Rokitta S. D., Wolf-Gladrow D. A., Rost B. (2012). Implications of observed inconsistencies in carbonate chemistry measurements for ocean acidification studies. Biogeosciences 9, 2401–2405. doi: 10.5194/bg-9-2401-2012
Hung H., Halsall C., Ball H., Bidleman T., Dachs J., De Silva A., et al. (2022). Climate change influence on the levels and trends of persistent organic pollutants (POPs) and chemicals of emerging Arctic concern (CEACs) in the Arctic physical environment–a review. Environ. Sci.: Processes Impacts 24, 1577–1615. doi: 10.1039/D1EM00485A
Ikuta T., Nakajima R., Tsuchiya M., Chiba S., Fujikura K. (2021). Interdecadal distribution of persistent organic pollutants in deep-sea chemosynthetic bivalves. Front. Mar. Sci. 8, 751848. doi: 10.3389/fmars.2021.751848
Jakobsson M., Mayer L. A., Bringensparr C., Castro C. F., Mohammad R., Johnson P., et al. (2020). The international bathymetric chart of the Arctic Ocean version 4.0. Sci. Data 7, 176. doi: 10.1038/s41597-020-0520-9
Jeremic A., Nehorai A. (1998). Design of chemical sensor arrays for monitoring disposal sites on the deep ocean floor. IEEE J. Oceanic Eng. 23, 101–111. doi: 10.1109/48.725229
JERICO-S3 D4.1 (2021). WP4 Pilot Supersite monitoring strategies. Available online at: https://www.jerico-ri.eu/download/jerico-s3_deliverables/JERICO-S3_DELIVERABLE_4.1_V2-FINAL.pdf.
JERICO-S3-D8.1 (2021). WP8-D8.1-311020-V2.3. Available online at: https://www.jerico-ri.eu/download/jerico-s3_deliverables/JERICO-S3-D8.1-TA-Provision.pdf.
JERICO-S3 D9.1 (2021). User requirement and classification. Available online at: https://www.jerico-ri.eu/download/jerico-s3_deliverables/JERICO-S3_D9.1_User-requirement-and-classification_Final.pdf.
Jevrejeva S., Moore J. C., Grinsted A., Matthews A. P., Spada G. (2014). “Trends and acceleration in global and regional sea levels since 1807,” in Global and Planetary Change, vol. 113. , 11–22. Available at: https://www.sciencedirect.com/science/article/pii/S0921818113002750?casa_token=Z4gBCRfKHaAAAAAA:wxg7KFPXmxSmMVcHrraLQ94CqO_yQg0CYZ2TOFX_RL4JT6fUbuIXItAFL8dPAIKFioGpXxZshn8#s0050.
Karstensen J., Petihakis G., Fernandez V. (2020). D3.2. Observing Networks initial Assessment. Available online at: https://eurosea.eu/download/eurosea-d3-2-observing-networks-initial-assessment/?wpdmdl=3570&refresh=63b6f6ea8a6c31672935146.
Kedar S., Arnon S. (2006). A distributed sensor system for the detection of contaminants in the ocean. IEEE J. Selected Topics Quantum Electron. 12, 713–722. doi: 10.1109/JSTQE.2005.862944
Klinčić D., Romanić S. H., Kljaković-Gašpić Z., Tičina V. (2020). Legacy persistent organic pollutants (POPs) in archive samples of wild Bluefin tuna from the Mediterranean Sea. Mar. pollut. Bull. 155, 111086. doi: 10.1016/j.marpolbul.2020.111086
Kopp H., Chiocci F. L., Berndt C., Çağatay M. N., Ferreira T., Fortes C. J. E. M., et al. (2021). Marine geohazards: Safeguarding society and the Blue Economy from a hidden threat. Eds. Muñiz Piniella A., Kellett P., van den Brand R., Alexander B., Rodríguez Perez A., Van Elslander J., Heymans J. J. (Ostend, Belgium: Position Paper 26 of the European Marine Board), 100, ISBN: ISBN: 9789464206111. ISSN: 2593-5232. doi: 10.5281/zenodo.5591938
Kwok R., Maksym T. (2014). Snow depth of the Weddell and Bellingshausen sea ice covers from IceBridge surveys in 2010 and 2011: An examination. J. Geophys. Res. Oceans 119, 4141–4167. doi: 10.1002/2014JC009943
Lange N., Tanhua T., Pfeil B., Bange H. W., Lauvset S. K., Grégoire M., et al. (2023). A status assessment of selected data synthesis products for ocean biogeochemistry. Front. Mar. Sci. 10. doi: 10.3389/fmars.2023.1078908
Lauvset S. K., Key R. M., Olsen A., Van Heuven S., Velo A., Lin X., et al. (2016). “A new global interior ocean mapped climatology: The 1× 1 GLODAP version 2,” in Earth System Science Data, vol. 8. , 325–340. Available at: https://essd.copernicus.org/articles/8/325/2016/.
Lennartz S. T., Marandino C. A., von Hobe M., Cortes P., Quack B., Simo R., et al. (2017). Direct oceanic emissions unlikely to account for the missing source of atmospheric carbonyl sulfide. Atmospheric Chem. Phys. 17, 385–402. doi: 10.5194/acp-17-385-2017
Leppäranta M. (2023). History and Future of Snow and Sea Ice in the Baltic Sea (Oxford Research Encyclopedia of Climate Science). doi: 10.1093/acrefore/9780190228620.013.891
Löptien U., Dietze H. (2014). Sea ice in the Baltic Sea–revisiting BASIS ice, a historical data set covering the period 1960/1961–1978/1979. Earth System Sci. Data 6, 367–374. ESSD - Sea ice in the Baltic Sea – revisiting BASIS ice, a historical data set covering the period 1960/1961–1978/1979 (copernicus.org). doi: 10.5194/essd-6-367-2014
Lüpkes C., Vihma T., Birnbaum G., Dierer S., Garbrecht T., Gryanik V. M., et al. (2012). “Mesoscale modelling of the Arctic atmospheric boundary layer and its interaction with sea ice,” in Arctic Climate Change: The ACSYS Decade and Beyond, 279–324. doi: 10.1007/978-94-007-2027-5_7
Pearlman J., Bushnell M., Coppola L., Karstensen J., Buttigieg P. L., Pearlman F., et al. (2019). Evolving and sustaining ocean best practices and standards for the next decade. Front. Mar. Sci. 6. doi: 10.3389/fmars.2019.00277/full
Pereiro D., Cusack C., Dunbar M., Navarro G., Álvarez-Berastegui D., O’Carroll J., et al. (2022). EuroSea, D6.1. Connections between “Extreme Marine Events” and Biological EOVs Report. Available online at: https://eurosea.eu/download/eurosea_d6-1_connections_between_extreme-marine-events_and_biological_eovs/?wpdmdl=4928&refresh=63b6f6ec47c231672935148.
Pérez-Gómez B., García-León M., García-Valdecasas J., Clementi E., Mösso Aranda C., Pérez-Rubio S., et al. (2021a). Understanding sea level processes during Western Mediterranean storm Gloria. Front. Mar. Sci. 8. doi: 10.3389/fmars.2021.647437/full
Pérez-Gómez B., Garcı́a-León M., Garcı́a-Valdecasas J., Clementi E., Mösso Aranda C., Pérez-Rubio S., et al. (2021b). Understanding sea level processes during Western Mediterranean storm Gloria. Front. Mar. Sci. 8. doi: 10.3389/fmars.2021.647437/full
Prieto L., Macías D., Peliz A., Ruiz J. (2015). Portuguese Man-of-War (Physalia physalis) in the Mediterranean: A permanent invasion or a casual appearance? Sci. Rep. 5, 11545. doi: 10.1038/srep11545
Ratnarajah L. (2021). Map of BioEco Observing networks/capability. EuroSea D1.2. Available online at: https://eurosea.eu/download/eurosea-d1-2-bioeco-observing-networks/?wpdmdl=3580&refresh=63762dec99ea41668689388.
Ratnarajah L., Heslop E., Lips I., Simpson P., Mtwana Nordlund L., Unsworth R., et al. (2022). D1.4. Report on the European BioEco observing system. Available online at: https://eurosea.eu/download/eurosea_d1-4_report_european_bioeco_observing_system_reformatted/?wpdmdl=4969&refresh=63762decce3981668689388.
Rees A. P., Bange H. W., Arévalo-Martínez D. L., Artioli Y., Ashby D. M., Brown I., et al. (2022). Nitrous oxide and methane in a changing Arctic Ocean. Ambio 51, 398–410. doi: 10.1007/s13280-021-01633-8
Révelard A., Tintoré J., Verron J., Bahurel P., Barth J. A., Belbéoch M., et al. (2022). Ocean integration: the needs and challenges of effective coordination within the ocean observing system. Front. Mar. Sci. 8. doi: 10.3389/fmars.2021.737671
Rödenbeck C., Bakker D. C., Gruber N., Iida Y., Jacobson A. R., Jones S., et al. (2015). Data-based estimates of the ocean carbon sink variability–first results of the Surface Ocean pCO2 Mapping intercomparison (SOCOM). Biogeosciences 12, 7251–7278. doi: 10.5194/bg-12-7251-2015
Romanić S. H., Jovanović G., Mustać B., Stojanović-Đinović J., Stojić A., T. and Popović A. (2021). Fatty acids, persistent organic pollutants, and trace elements in small pelagic fish from the eastern Mediterranean Sea. Mar. pollut. Bull. 170, 112654. doi: 10.1016/j.marpolbul.2021.112654
Roose P., Albaigés J., Bebianno M. J., Cronin M., de Leeuw J., Gabrielsen G., et al. (2011). Chemical Pollution in Europe’s Seas: Programmes, Practices and Priorities for Research, Marine Board Position Paper 16. Eds. Calewaert J. B., McDonough N. (Ostend, Belgium: Marine Board-ESF). Available at: https://www.marineboard.eu/sites/marineboard.eu/files/public/publication/Monitoring%20Chemical%20Pollution-71.pdf.
Rubio A., Reyes E., Mantovani C., Corgnati L., Lorente P., Solabarrieta L., et al. (2021). D3.4. European High Frequency Radar network governance. Available online at: https://eurosea.eu/download/eurosea-d3-4-hfr-governance/?wpdmdl=3585&refresh=63b6f6eab7c701672935146.
Seys J., Cox L., Şahin Yücel E., Ezgeta-Balić D., Faimali M., Garaventa F., et al. (2022). Marine Science Communication in Europe – A way forward. Eds. Muñiz Piniella A., Kellett P., van den Brand R., Alexander B., Rodriguez Perez A., Van Elslander J., Heymans J. J. (Ostend, Belgium: Future Science Brief 8 of the European Marine Board), 48, ISBN: ISBN: 9789464206166. ppISSN: 2593-5232. doi: 10.5281/zenodo.6444143
Sutton A. J., Sabine C. L., Maenner-Jones S., Lawrence-Slavas N., Meinig C., Feely R. A., et al. (2014). A high-frequency atmospheric and seawater pCO 2 data set from 14 open-ocean sites using a moored autonomous system. Earth System Sci. Data 6, 353–366. doi: 10.5194/essd-6-353-2014
SWD (2020). Available online at: https://ec.europa.eu/info/sites/default/files/swd202060final.pdf.
Tanhua T., McCurdy A., Fischer A., Appeltans W., Bax N., Currie K., et al. (2019a). What we have learned from the framework for ocean observing: Evolution of the global ocean observing system. Front. Mar. Sci. 6. doi: 10.3389/fmars.2019.00471/full
Tanhua T., Pouliquen S., Hausman J., O’brien K., Bricher P., De Bruin T., et al. (2019b). Ocean FAIR data services. Front. Mar. Sci. 6. doi: 10.3389/fmars.2019.00440/full
Teixeira H., Berg T., Uusitalo L., Fürhaupter K., Heiskanen A. S., Mazik K., et al. (2016). A catalogue of marine biodiversity indicators. Front. Mar. Sci. 3. doi: 10.3389/fmars.2016.00207/full
Teixeira H., Lillebø A.I., Culhane F., Robinson L., Trauner D., Borgwardt F., et al. (2019). Linking biodiversity to ecosystem services supply: Patterns across aquatic ecosystems. Sci. Total Environ. 657, 517–534. doi: 10.1016/j.scitotenv.2018.11.440
Tilbrook B., Jewett E. B., DeGrandpre M. D., Hernandez-Ayon J. M., Feely R. A., Gledhill D. K., et al. (2019). An enhanced ocean acidification observing network: from people to technology to data synthesis and information exchange. Front. Mar. Sci. 6, 337. doi: 10.3389/fmars.2019.00337
Tintoré J., Pinardi N., Álvarez-Fanjul E., Aguiar E., Álvarez-Berastegui D., Bajo M., et al. (2019). Challenges for sustained observing and forecasting systems in the Mediterranean Sea. Front. Mar. Sci. 6. doi: 10.3389/fmars.2019.00568
Tsontos V. (2021). COVERAGE: next generation data service infrastructure for a digitally integrated ocean observing system in support of marine science and ecosystem-based management. Mar. Technol. Soc. J. doi: 10.4031/MTSJ.55.3.45
Valentini A., Taberlet P., Miaud C., Civade R., Herder J., Thomsen P. F., et al. (2016). Next‐generation monitoring of aquatic biodiversity using environmental DNA metabarcoding. Mol. Ecol. 25 (4), 929–942. doi: 10.1111/mec.13428
Van Noorden R. (2018). Leadership problems in the lab. Nature 557, 294–296. doi: 10.1038/d41586-018-05143-8
Wenning R. J., Martello L. (2014). “POPs in marine and freshwater environments,” in Environmental forensics for persistent organic pollutants (Elsevier), 357–390. Available at: https://www.sciencedirect.com/science/article/abs/pii/B9780444594242000086.
Keywords: ocean observing, gaps, recommendations, EU, UN Ocean Decade
Citation: Hassoun AER, Tanhua T, Lips I, Heslop E, Petihakis G and Karstensen J (2024) The European Ocean Observing Community: urgent gaps and recommendations to implement during the UN Ocean Decade. Front. Mar. Sci. 11:1394984. doi: 10.3389/fmars.2024.1394984
Received: 02 March 2024; Accepted: 09 July 2024;
Published: 29 July 2024.
Edited by:
Oliver Zielinski, Leibniz Institute for Baltic Sea Research (LG), GermanyReviewed by:
Lars Arneborg, Swedish Meteorological and Hydrological Institute, SwedenMarcello Gatimu Magaldi, Consiglio Nazionale delle Ricerche, Italy
Copyright © 2024 Hassoun, Tanhua, Lips, Heslop, Petihakis and Karstensen. This is an open-access article distributed under the terms of the Creative Commons Attribution License (CC BY). The use, distribution or reproduction in other forums is permitted, provided the original author(s) and the copyright owner(s) are credited and that the original publication in this journal is cited, in accordance with accepted academic practice. No use, distribution or reproduction is permitted which does not comply with these terms.
*Correspondence: Abed El Rahman Hassoun, YWhhc3NvdW5AZ2VvbWFyLmRl