- 1GEOMAR Helmholtz Centre for Ocean Research Kiel, Kiel, Germany
- 2Mercator Ocean International, Toulouse, France
- 3European Global Ocean Observing System (EuroGOOS AISBL), Brussels, Belgium
- 4Institute of Marine Sciences of Andalusia (ICMAN-CSIC), Puerto Real, Spain
- 5Laboratoire de Météorologie Dynamique, Institut Pierre Simon Laplace (IPSL), École Normale Supérieure (ENS)-Université Paris Science Lettres (PSL), Paris, France
- 6Mediterranean Institute for Advanced Studies (IMEDEA), Spanish National Research Council (CSIC)-University of the Balearic Islands (UIB), Esporles, Spain
- 7Centro EuroMediterraneo sui Cambiamenti Climatici, Lecce, Italy
- 8Department of Biological, Geological, and Environmental Sciences, University of Bologna, Bologna, Italy
- 9Australian National Centre for Ocean Resources & Security, University of Wollongong, Wollongong, Australia
- 10Puertos del Estado, Madrid, Spain
- 11Marine Institute, Oranmore, County Galway, Ireland
- 12Intergovernmental Oceanographic Commission of UNESCO - Global Ocean Observing System, Paris, France
- 13Institute for Marine and Antarctic Studies, University of Tasmania, Hobart, Australia
- 14Hellenic Centre for Marine Research (HCMR), Institute of Oceanography, Heraklion, Greece
- 15International Ocean Carbon Coordination Project, Institute of Oceanology of Polish Academy of Sciences, Sopot, Poland
- 16UK Met Office, Exeter, United Kingdom
- 17Institute of Electrical and Electronics Engineers (IEEE) France Section, Paris, France
- 18European Marine Board, Ostend, Belgium
The EU funded project EuroSea brought together key actors of the European ocean observing and forecasting communities with key users of the ocean observing products and services in order to better integrate existing ocean observation systems and tools, and to improve the delivery of ocean information to users. EuroSea was constructed around the ocean observing value chain that connects observations to users of ocean information, and, just as intended, the value chain concept was a useful prism to improve the system. In this article, we summarize some of the main take-home messages from EuroSea on the needs for developing the European Ocean Observing System and its links with modeling and forecasting systems. During the project, the challenges and gaps in the design and coordination of the European ocean observing and forecasting system were identified and mapped. Many gaps and challenges related to the observations of physical, chemical and biological Essential Ocean Variables were identified. Some of these gaps are related to technological developments, while others are caused by insufficient and short-term funding leading to a not sustainable system, management, and cooperation between different entities, as well as limitations in foresight activities, policies and decisions. This article represents a compilation of the broader needs for advancing the observing and forecasting system, and is meant as a guide for the community, and to funders and investors to advance ocean observing and the delivery of ocean information in Europe. To enhance the sustainability of ocean observations, which is paramount for a reliable provision of quality oceanographic data and services, several recommendations were compiled for ocean observing networks, frameworks, initiatives, as well as the ocean observing funders within the European nations, and the European Commission.
1 Introduction
The ocean has a profound impact on humanity that transcends geographic boundaries and serves as the primary regulator of global climate. In addition, the ocean plays a central role in providing essential resources, including food, materials, energy, transportation, and recreation. However, the ocean also poses various potential hazards, stemming from both natural phenomena and human driven activities. These hazards encompass hurricanes, coastal flooding and erosion, tsunamis, storm surges, sea level rise, harmful algal blooms, deoxygenation and pollution. Moreover, the frequency and intensity of most extreme meteorological and marine events are expected to increase due to the effects of climate change (e.g. IPCC, 2021). In the face of these challenges, the ocean is hence exposed to numerous stressors that directly affect human populations and marine ecosystems. These stressors include, for instance, warming, heat waves, overexploitation, ecosystem degradation, changes in circulation patterns, ocean acidification, and pollution. To understand potential risks of these pressures and improve ocean’s resilience, there is a need for comprehensive information, knowledge and predictive capability on ocean dynamics, changes, risk, and vulnerability (e.g. Von Schuckmann et al., 2020; Visbeck, 2018). This information is essential to support and guide collaborative management of the ocean as efforts continue toward restoring and enhancing the resilience and adaptation of the multiple endangered marine ecosystems globally (e.g. IPBES, 2019) towards a sustainable future. The demand for reliable and timely ocean information and forecasts is steadily increasing, in line with the development of sustainable management solutions, blue economy and effective ocean and coastal governance.
However, several critical gaps continue to significantly limit Europe’s ability to observe and predict the ocean, which limits the effective and sustainable management of the marine environment and its precious resources (e.g. EMB, 2021). In this article we are primarily looking at the geographic region Europe, and try to distinguish this from the nations that are member in the EU. In order to establish a robust and comprehensive ocean observing and prediction system, it is essential to integrate and coordinate national initiatives and bring greater focus on users, ultimately leading to the development of an international/EU framework and observing infrastructures that can deliver the data and information society needs (e.g. IOC, 2019). With users we refer to both intermediate and end-users in this article. These users include government agencies that need to fulfil domestic, European and international obligations, industry partners that are active in blue economy and citizens. This envisioned system should seamlessly coordinate the deployment of ocean observing efforts and ensure adherence to standardized data management and sharing of protocols. By promoting appropriate acquisition, access and interoperability, this system aims to facilitate the efficient exchange of ocean data (e.g. Tanhua et al., 2019b). This system must be designed to improve the assessment of the current state of the ocean and provide more accurate predictions of its future trends to those who need this data for planning, policy and decisions, as well as to fuel operational weather and warning systems (Schuckmann et al., 2022; Le Traon et al., 2019). Such capabilities are also critical to facilitate a sustainable ecosystem-based management of ocean resources. It would enable society to find solutions for problems that result from increasing threats to the ocean, and adapt to changes as a consequence of these threats, and ultimately assess the impact of measures towards a sustainable ocean.
While the existing system harbors the potential for innovative technologies currently in development, its current state is marked by fragmentation, leading to inefficiencies and ineffectiveness. This fragmentation results in gaps in ocean information, ultimately impeding the formulation of decisions and the development of effective strategies. New ocean observing technologies, when proven, should be rolled out at the national level as recommended by the, yet to be sustainably funded, European ocean observing system coordination efforts.
In this article we shortly summarize the efforts of EuroSea1, an EU funded project that worked towards improving the ocean observing and forecasting. We then move on to discuss the ocean observing value chain, discuss the governance, and dive deeper into the components of the value chain and articulate key recommendations on the most urgent needs to improve the ocean observing and forecasting in Europe, and worldwide (Yang et al., 2022), as outcomes of the EuroSea project. For more details on the outcomes of EuroSea, we refer to the numerous deliverables and scientific publications from the project. To guide the reader through this article, we refer to Table 1 for a list of used acronyms.
2 EuroSea
The EU Horizon 2020 Innovation Action EuroSea (grant# 862626), funded from November 2019 to December 2023, brought together key European actors of ocean observing and forecasting with diverse users who depend on ocean observing products and services, to integrate existing ocean observing systems and tools, and to increase coordination at a higher level. The vision of EuroSea was: “Advancing research and innovation towards a user-focused, truly interdisciplinary, and responsive European ocean observing and forecasting system, that delivers the essential information needed for human wellbeing and safety, sustainable development and blue economy in a changing world”. The co-design approach of EuroSea is central to strengthening a community necessary to improve European ocean observation and forecasting services, and EuroSea worked closely with developers and potential users of products and services.
EuroSea worked towards two goals. First, to deliver ocean observations and forecasts to advance scientific knowledge about ocean climate, marine ecosystems and their vulnerability to human impacts. Second, to demonstrate how the ocean is an essential part of an economically viable and healthy society, not only today but also in the decades to come. The mission of EuroSea was: “Co-designing European ocean observing and forecasting services and products that deliver information and support decision-making in the areas of climate, coastal and maritime activities, and ocean health”. More specifically, EuroSea formulated seven objectives: 1) Strengthening European ocean observing and forecasting as an integrated entity within a global context; 2) Improving the design for an integrated European ocean observing and forecasting system for the European seas and the Atlantic, including the deep sea; 3) Improving and enhancing the readiness and integration of ocean observing networks; 4) Enabling FAIR (Findable, Accessible, Interoperable, Re-usable (Wilkinson et al., 2016), data, supporting integration of ocean data into Copernicus Marine Service (CMS), EMODnet and SeaDataNet portfolios; 5) Delivering improved forecasts and new synthesis products by better use of data in models; 6) Developing novel services, demonstrating the value of the ocean observing system to users; 7) Supporting integrated, sustainable and fit-for-purpose ocean observing system by engaging with a range of users and other stakeholders.
One important aspect of EuroSea was three “demonstrator” work-packages that use the whole value chain, see below for a description of the ocean observing value chain, from design, operations, data delivery to (information) products for end-users, see Figure 1. The three demonstrator work packages, i.e. WPs 5, 6 and 7, were focused on ocean health, operational services and climate. The demonstrators were thus able to exercise all the steps, and were supported from different partners in various work packages of EuroSea for implementation. Although it is out of the scope of this article to discuss all the information products that were developed in EuroSea in detail, we refer to reports and deliverables from EuroSea2 throughout this article.
3 The ocean observing value chain
The value chain of ocean observng was defined in the international Framework for Ocean Observing (FOO, Figure 2) and has been pivotal for improving the sustained ocean observing system through a system engineering view (Lindstrom et al., 2012). The value chain starts with requirements setting, i.e. determine what to measure and why, and then moves to the actual observations that can be made by a number of different platforms (pink area in Figure 2). The blue area describe the delivery of data and products to users, and has a feedback loop back to the issues statement that evaluates the efficiency of the value chain in delivering information on the issues of interest.
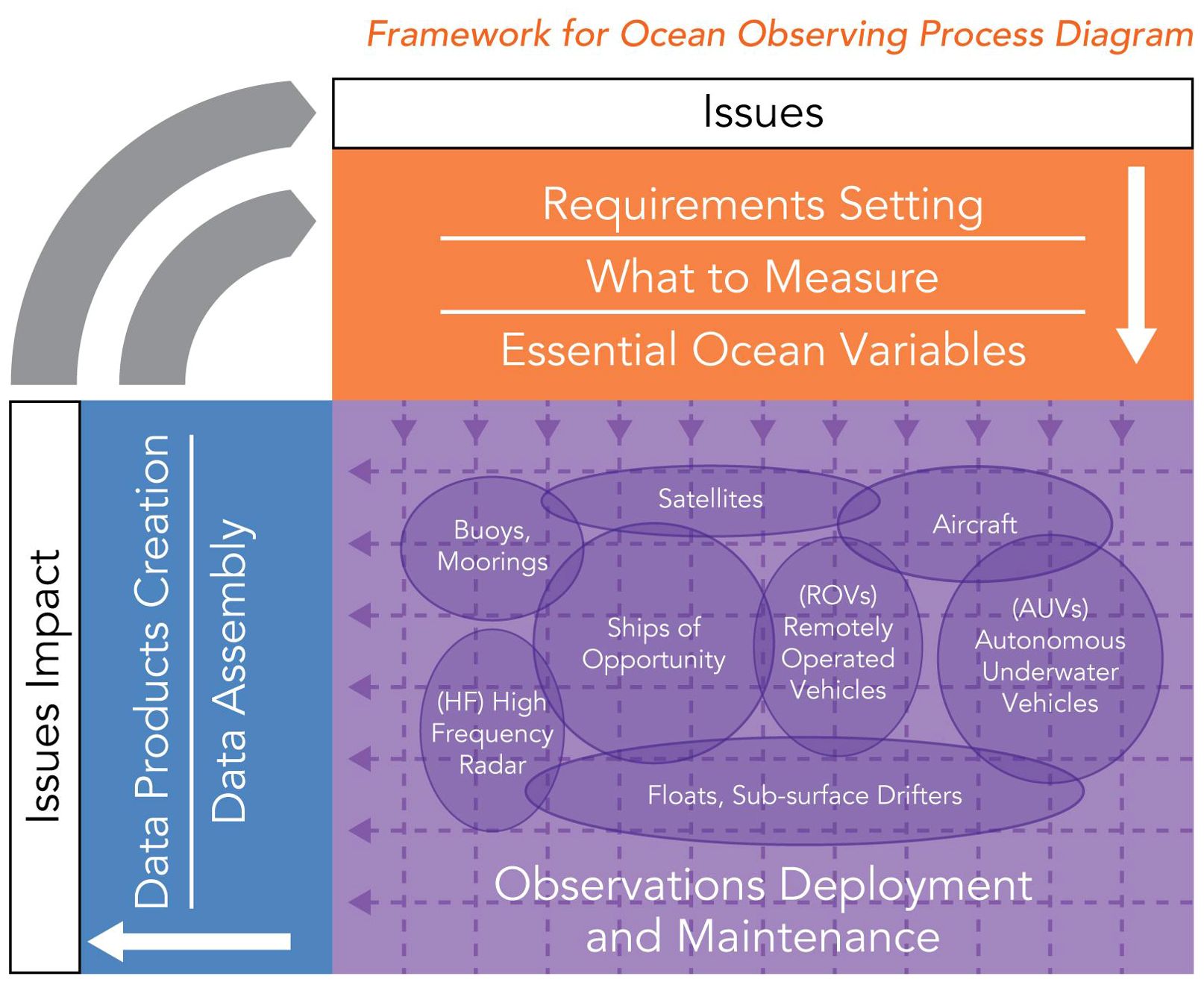
Figure 2. The ocean observing value chain as described in the Framework for Ocean Observing (Lindstrom et al., 2012).
The FOO also includes other aspects, such as the Readiness Level (RL) of the three steps in the value chain: requirement processes, coordination of observational elements and, data management and information products. Recently, the RL concept has been systematically applied to elements of the ocean observing system, for instance for ocean biogeochemical data products (Lange et al., 2023), and for rating the state of information delivery on various ocean phenomena (Hassoun et al., 2023), as well as for the assessment of the observation networks in Europe (Petihakis et al., 2023; Karstensen et al., 2020). This approach has proven a useful way to evaluate the current ability to operationally deliver adequate information to stakeholders and to understand where investments are needed to drive improvements.
Launched in 2019, the Global Ocean Observing System (GOOS) 2030 Strategy (IOC, 2019) is an ambitious call to advance our ocean observing system by expanding and integrating across the ocean observing value chain, to evolve a more fit-for-purpose and responsive system with users and stakeholders. The GOOS strategy offers a slightly different view of the ocean observing value chain (Figure 3), and while this visual depiction has less detail, the feedback loops are clearer and the impact is included as an equally important part of the value chain. The two figures are complimentary, with more details targeting the science/observing community provided in the FOO while a slightly higher-level view is provided in the GOOS Strategy.
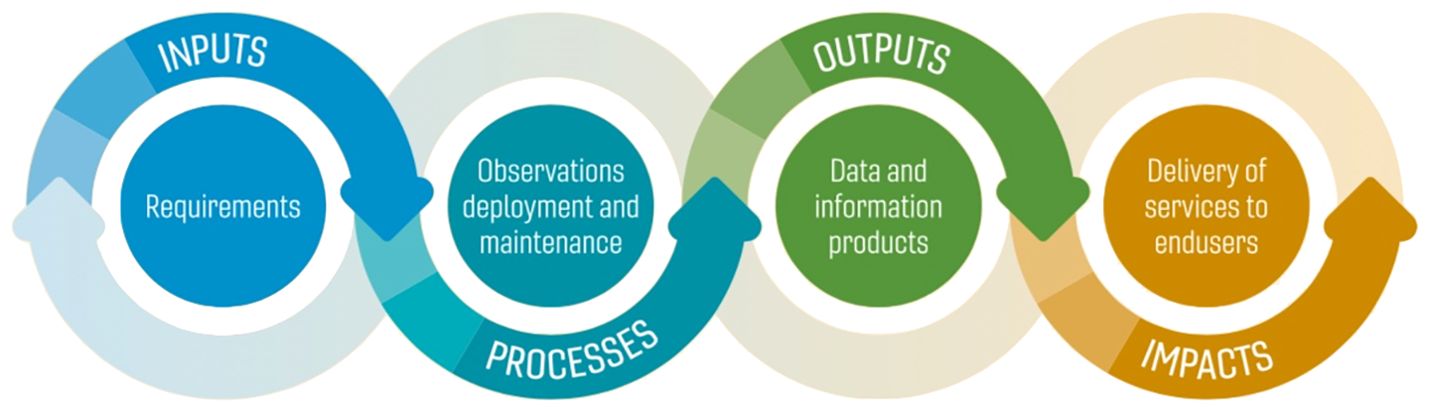
Figure 3. The ocean observing value chain as described in the GOOS 2030 strategy (IOC, 2019).
Several past projects and programs successfully used the value chain as a prism for designing the ocean observing and forecasting system. For example, the EU Horizon 2020 AtlantOS project (grant# 633211) and the TPOS 2020 project used the value chain approach for the Atlantic and Tropical Pacific Observing Systems respectively. The GOOS ocean observing value chain concept also guided the structure of the EuroSea project into work packages with targeted goals. Ocean observations and ocean prediction are components of the ocean observing value chain, which represent the full length of multi-platform observation networks, data management systems, data assimilative prediction systems, dissemination/accessibility systems, service delivery, as well as the end-users of ocean products (IOC, 2019; Lindstrom et al., 2012). These components must be well connected and include provisions for feedback loops and the exchange of impact information related to all value chain components.
The GOOS ocean observing value chain defines step one as the inputs, i.e. the requirement setting, which defines the reason for the investment in the observation, i.e. what are the questions or issues we would like to understand better and monitor over time. This include understanding of societal drivers, scientific application, readiness level needs, phenomena to capture, temporal and spatial scales of the phenomena, magnitude of the signal to capture, and the uncertainty relative to the signal. In here we define, in practical terms, what kind of observations are needed to get to this information. In this step, it is important to account for models that transform sparse in situ and surface satellite observations into 4D ocean analyses and forecasts. The Essential Ocean Variable (EOV) concept is a critically important link in the ocean observing value chain. EOVs are proposed by thematic expert groups and endorsed by the GOOS steering committee. EOVs are a fundamental element of the FOO and have proven in the past to be a useful mechanism to mobilize resources for observations. The systematic collection of variables from which EOVs are derived always includes traceable quality control of the underlying measurements. Deriving EOVs is closely linked to Standard Operating Procedures (SOPs) and best practices. The global availability of EOVs supports the provision of services to governments and civil society in the form of real-time decision support, assessments of the state of the oceans and long-term observations to inform policy on climate change in the ocean. Several, but not all, of the EOVs are also Essential Climate Variables (ECVs) that are recommended by the Global Climate Observing System (GCOS). The quality of the ECVs were recently independently evaluated by the Copernicus Climate Change Service (C3S, Yang et al., 2022), that provide information about the past, present and future climate.
The second step in the value chain is defined as the processes step where ocean observing assets are deployed and maintained. There are usually many options to measure an EOV, all with different attributes that should be matched to the requirements defined in the first step. One important task of an integrated ocean observing system is to weigh different priorities and goals to optimize implementation, serving different users and stakeholders. This requires a constant dialogue between the ocean observers, and a significant amount of coordination and negotiation between the beneficiaries and funders of the observing elements. Technical innovations leading to more accurate and precise sensors for use on novel platforms, i.e. increased feasibility to observe a variable, as well as changing requirements, i.e. re-assessment of impact, are considered in the evolution of EOVs, recalling that EOVs are selected based on impact vs. feasibility.
The third step of the value-chain is the outputs of data, data-products and information ready for uptake by downstream users. Effective data management systems are critical to ensure essential ocean variable data are collected, retained and accessible for analysis. The FAIR (Findable, Accessible, Interoperable, Re-usable) principles are the foundation for a functional ocean observing and forecasting system (e.g. Tanhua et al., 2019b; Wilkinson et al., 2016). The additional attributes of free, timely, and unrestricted delivery of ocean data is beneficial for both the data originators (recognition, citations, funding acknowledgement, etc.) and users (allows the data to be collected once and used many times (e.g. Martín Míguez et al., 2019), and ensures an uninhibited pathway to the development of novel information and forecasts products.
Ultimately, in step four, the aim of all ocean observing value chain activities is obviously the (positive) impacts through the delivery of services and information to users. This is where a constant feedback process should take place to assess the impact of the output vs. the initial issue identification. Impact is not an easy attribute to measure and to quantify, but it is an important aspect to consider. Additionally, it is important to regularly review that the value chain is working, exercising the value chain is and should be an iterative process. Just as the needs of the ocean data users and the technological possibilities are evolving in time, so should the observing system be flexible to this evolution.
In this article, we will discuss the steps in the value chain in more detail in sections 4-9, and articulate areas that need to be improved, in Europe and globally, towards a sustainable ocean observing and forecasting system. We end with a discussion of governance in section 10 and consider the sustainability of the system in section 11. In Table 2, we provide one brief example of exercising the ocean observing value chain for oxygen EOV.
4 Inputs: ocean observing system design
Because of its vastness, the wide range of scales of variability, processes, and properties to be measured, the level of information currently available is not enough for most end-user needs. This limits society’s ability to use ocean resources sustainably and to mitigate and adapt to ongoing changes. In addition, the societal demand for ocean information is growing rapidly, while users in different sectors require information at different levels of quality and latency. To date, numerous communities have developed global-scale observing plans, programs, and networks in response to these demands. Marine environmental information from these efforts is collected by an increasing number of experts in a variety of ways, but much of the resulting data is typically viewed in isolation and evaluated through the lens of individual programs and networks. The creation of individual observing systems focused on the needs of climate versus ocean health, marine transportation, etc. is clearly neither sustainable nor economical. Furthermore, there is a danger that the provision of ocean information will continue to become sector-specific, with increasing redundancy in effort and confusion in products, whereas the true value of these data can only be fully realized if they are brought together and fully integrated and developed across observing, modeling, product and service organizations. This can be achieved by changing current paradigms towards such integrated systems, driven by the need to be fit for purpose.
A properly designed ocean observing system, based on the requirements of the users and the prediction centers, is therefore essential to increase the efficiency and utility of the system and allow regular evaluations of its fit-for-purpose. Such a design is a great advantage when it comes to coordinating and implementing the system, which is usually operated by a large number of actors. While relatively simple in principle, it is difficult to design even a single observation network for a single phenomenon or EOV across different platforms and sensors. It is a very challenging task to design an observing system across multiple networks for all EOVs. Certainly, a bit of pragmatism is useful, recognizing that the “enemy of good is perfection”, and implementing an iterative process where the impact is measured against the requirements already established in the EOV definition. An example of a global network with a careful design is the Argo network, and the adaptation to technical progress articulated in the One-Argo design, which has added BGC-Argo and Deep-Argo, to a more comprehensive design responding to more requirements. The One-Argo design is also, to some extent, co-designed with the GO-SHIP repeat hydrography network.
One attempt to evaluate the availability of ocean data in order to guide the design on the ocean observing system is the Checkpoints3 conducted by EMODnet, which has been very useful to identify gaps and redundancies in the ocean observing system. A different approach was taken by (Hassoun et al., 2024) that used available reports and literature to assess gaps and issue recommendations for the ocean observing community. The use of the readiness level concept articulated in the FOO has also been efficiently been used to evaluate the ocean observing system (Hassoun et al., 2023; Lange et al., 2023), and this offers a way forward for regular assessments.
Different approaches to deliver an efficient ocean observing design have been adopted. For the evaluation of the global ocean observing system, in terms of climate monitoring or seasonal forecasts related requirements for physical variables, the Observing System Experiment (OSE) and the Observing System Simulation Experiment (OSSE) approaches have been further developed. They are based on Ocean Data Assimilation and Prediction (ODAP) systems, which include ocean reanalyses for seasonal forecasts and long-term estimates of ocean conditions. ODAP serves a wide range of oceanographic, meteorological, and climate forecasting applications.
Although these approaches work for physical (operational) oceanography, they have limited use for biogeochemistry, and almost none for biology and ecosystem observing system. However, the latter suffer from lack of user-driven design of the monitoring, which is a gap which ought to be addressed. One example is that EuroSea developed an aquaculture user demonstrator in order to obtain information from a targeted group of stakeholders to setup a dedicated observing system (Graves et al., 2023; Pereiro et al., 2023).
In the OSSE framework, synthetic observations designed to emulate observations from a proposed system are generated from a free-running simulation run of an ocean model, referred to as the “nature run”. This model aims to represent the true state of the ocean, with observational errors introduced based on community-sourced information (Hoffman and Atlas, 2016). The synthetic data are assimilated into a separate data-assimilated simulation run (OSSE), with different initial/forcing conditions and often a modified model with reduced resolution or altered physical parameterizations. The forecast improvement resulting from the assimilation of the new data is measured by the error reduction in the OSSEs. In this framework, errors across model space are estimated directly by contrasting variables between the nature run and OSSE fields. In particular, OSSEs facilitate the evaluation of both current and future observational data types (Fujii et al., 2019). For instance, Barceló-Llull and Pascual (2023) used OSSEs to optimize the design of in situ experiments aimed to reconstruct fine-scale ocean currents (~20 km) in preparation to the Surface Water and Ocean Topography (SWOT) mission.
Observing System Experiments, OSEs, are conducted to evaluate existing observations. OSEs examine the impact of a particular observing system or observations by either including or excluding them from a data assimilation system. An OSE involves a data assimilation run in which a particular type of observation is withheld or added to the regularly assimilated data. The effect of these manipulations is assessed by comparing the OSE with a control simulation that assimilates only regular data. While the methodology of OSE is straightforward, its widespread application is due to its adaptability to any ODAP system, including highly nonlinear or stochastic systems. The evaluation of error reduction in the OSE framework relies on reference data, preferably independent of the assimilation process, to ensure a fair evaluation (e.g. Fujii et al., 2019).
The assessment of OSEs and OSSEs is limited to those (few) variables that can be simulated and assimilated in state-of-the-art models. Developments in assimilation techniques, models and assimilation for biogeochemical variables and regional OSEs/OSSEs are still ongoing. EuroSea has demonstrated the high potential for biogeochemical OSSEs and for regional, high-resolution, multi-platform applications (Barceló-Llull and Pascual, 2023; Gasparin et al., 2023; Rosselló et al., 2023; Mignot et al., 2023).
To make further progress in the design of observing systems for the different variables and scales at which ocean observing system assessment is needed, EuroSea has demonstrated the relevance of a co-design approach with intermediate stakeholders and users. EuroSea has worked to identify requirements for existing observing networks in support of coastal resilience, operational services, ocean health and climate change (e.g. Ciliberti et al., 2023). The aim was to maximize the impact of the observing system by creating synergies between stakeholders and observing networks (e.g. Dunbar et al., 2023). The approach included a synergistic dialogue to understand the needs of different communities, co-develop indicators with stakeholders, and verify operational predictions. Key users and stakeholders across sectors were considered and a set of indicators. Indicators are easy to understand tools to describe, measure and monitor a complex ocean phenomenon such as, for instance, the extent of areas with suboxic conditions, or the length and duration of suboxic or anoxic events, see section 3. Another example would be the seven Global Climate Indicators4 that provides annual updates of the State of the Global Climate, of which five are ocean-related indicators, that was co-designed for efficient harmonization.
This enabled the identification of ocean variables to be measured, and their specific requirements and uncertainties in relation to the intended set of co-designed ocean indicators. The framework accommodated different scales and facilitated the transition from global to local indicators, thereby enhancing the relevance for different end-user sectors (e.g. Dayan et al., 2023, 2022). The result is a simple yet effective framework based on stakeholder-identified needs that promotes collaboration between users, data providers and services. This framework can be expanded and adapted to be used for other ocean indicator development. In addition to fostering collaboration between the observing and modeling communities, this work has shown that engagement with users of ocean observations in Europe and globally is essential to understand the requirements of observing systems (Box 1).
Box 1. Recommendations for system design.
❖ We recommend improving and advancing the integration between the observing and modeling communities (including using Observing System Experiments (OSEs) and Observing System Simulation Experiments (OSSEs).
❖ We recommend conducting targeted design experiments for an optimal integrated in-situ observing system considering complementarity/synergies with satellite observations and models. We also recommend that such experiments consider the feasibility of the system, e.g. the cost and precision of the measurements.
5 Processes: networks and systems
The second step in the ocean observing value chain is the processes step, i.e. the deployment and maintenance of observational platforms and sensors used to measure EOVs in the field. As mentioned before, EOVs are measured on a variety of different platforms and by different sensors. Ideally, these observations are coordinated for efficiency of operation, and there are agreements of best practices and standards. deYoung et al. (2019) defined two main coordination mechanisms, both of which would be part of the GOOS.
Firstly: Ocean observing networks are typically platform or theme-focused organizations, or groupings. Globally, many networks are overseen by the Ocean Coordination Group (OCG) of GOOS, that have set up a set of attributes5 needed to qualify as a network. The articulated attributes have been, and are, important to evolve the networks towards higher readiness levels, and are one of the guiding criteria for the evolution of the observing system. Similar structures are set up in Europe, for instance the EuroGOOS observing platformed-based Task Teams, also part of the EOOS framework, promote scientific and technological synergies among European ocean observing infrastructures, exchange best practices, and feed data to the regional and European data aggregators. Common attributes that need to be considered for observing networks are 1) the level of coordination within a network, 2) the availability of community agreed best practices, 3) the level of integration of the network in the observing system, and finally 4) the data delivery, including meta-data standards, FAIR conformity, etc. Secondly: Ocean observing systems that are large scale national, regional or international programs that build on observing networks within a region. EOOS would be an example of such a system, and the overarching goal of this article is to discuss its technical needs.
Few networks are implementing the use of Key Performance Indicators (KPIs) to monitor progress, and common KPIs across networks should be implemented to foster integration and comparability. Examples of KPIs could for instance be KPIs defined by Argo, such as “the number of operational floats for each of the three missions of OneArgo (Core, Biogeochemistry, and Deep)”.During the assessment carried out by Petihakis et al. (2023), the often-poor connection between platform-oriented networks and thematic networks, such as the Integrated Carbon Observation System (ICOS), was particularly noted as in need for increased efforts, ideally centrally coordinated. Another topic that needs further attention is the integration between networks towards coordination, design and observations of EOVs across networks.
We also note that there are a number of networks that are seen as aspirational, i.e. they are in very early stages of readiness, poorly organized, while the established networks reach readiness level 4, or higher. There is a need to increase the number of networks/monitoring programs for increased integration and efficiency to observe and report on all EOVs on a global scale. This need is in particular large for the biology and ecosystem networks and for the fisheries observing community, in addition to better communication and coordination between the ocean observing and environmental monitoring program communities.
Although progress has been made in biological, ecosystem and environmental monitoring, there is still work to be done to bring these observing communities up to the same level as the more operational observing networks (e.g. Ratnarajah et al., 2022). Enhancing open and public data availability, interoperability of data platforms, and the use and share of standard operating procedures (SOPs) and protocols are clear areas where improvements would strengthen and advance not only biodiversity but also pollution (e.g., marine litter, Palacz et al., 2022) monitoring in Europe. Community engagement and coordination among biological and ecosystem networks are essential to address these issues (Box 2).
Box 2. Recommendations for networks and systems.
❖ We recommend supporting the ocean observing community to form networks that comply to the attributes articulated by GOOS.
❖ We recommend integrating several more coastal monitoring programs in such networks; there is a larger potential for increased synergy and efficiency of the system in doing so.
❖ We recommend fostering, furthering and supporting the organization of European biological and chemical EOV observing communities to work towards FAIR data, standardization, harmonization and endorsement of protocols and best practices.
❖ We recommend promoting collaborative design of coastal biodiversity, biogeochemistry and marine litter monitoring on national and Regional Sea Conventions levels.
6 Processes: coordination and planning
There are numerous platforms or topic-based networks and RIs in Europe which provide information on the ocean observing activities and that facilitate open and often free access to the technology. In addition, past and ongoing projects have contributed significantly to these objectives, and four Regional Sea Conventions (the Helsinki Convention6, the OSPAR Convention7, the Mediterranean Action Plan – Barcelona Convention8, the Bucharest Convention9) facilitate cooperation amongst neighboring countries by providing a platform for regional collaboration, sharing of best practices, harmonizing monitoring and assessment methods, and data structures, among others. There is a need to foster collaboration and make the information on planned ocean observing activities better visible as the numerous developments during the last decade are leading to fragmented information sharing on multiple web pages (mainly about cruises/vessels schedules on national and regional scales, often in native languages). Information exchange and coordination can improve synergies, reduce overlaps, and efficiently address the existing gaps.
Open-access information on the spatial and temporal extent of existing marine ecosystem monitoring programs is crucial. Within GOOS, the operational tracking of observing networks, and meta-data collection, is performed by OceanOPS10 in a very efficient way. The EuroSea project helped establish the GOOS BioEco Portal11 for this purpose, offering an interactive map and spatial information on global ocean programs monitoring biological and ecosystem EOVs. To bolster its impact, connecting the BioEco Portal with OceanOPS would help visualize an integrated ocean observing system, provide metadata-based observing system status services across disciplines and help identify where observing efforts should be intensified and facilitate collaboration between nations/regions to improve spatial and temporal coverage.
Europe does not yet have a single central tool able to deliver a complete and accurate view of the European ocean observing capability and status (Turpin et al., 2022). Even though the collaboration between OceanOPS, EMODnet and Copernicus marine in situ exists, there is a need for an improved ability to monitor the European ocean observing capabilities and performances, especially for better planning of the EOOS implementation. For instance, the availability of open-access data and information on the spatial and temporal extent of existing biological and ecosystem monitoring and observing programs is a fundamental cornerstone to tracking biodiversity in European waters. EuroSea supported the improvement for several observing networks on an European and Global scale (Petihakis et al., 2023), and this framework is useful to build on for additional and enhanced information on the status of the ocean observing system (Box 3).
Box 3. Recommendations for coordination and planning.
❖ We recommend that the European Commission incentivizes Member States to use a common, pan-European ocean observation planning platform following OceanOPS, or similar efforts, to share information on cruise plans, deployments, observations (engage different observing communities, including the private sector), and collaboration opportunities. This will maximize the use of resources and lift up meaningful collaborations at the European scale, triggering meaningful collaborations also at the global scale.
❖ We recommend supporting the development of a central European ocean observation monitoring service, building upon OceanOPS, EMODnet and Copernicus Marine capabilities, to identify the temporal and spatial gaps in observations, facilitate cost-efficient operations and cross-networks cooperation, FAIR data sharing and ensure the fit-for-need data flow for the pan-European products and services.
❖ We recommend creating a development and resource plan to advance the GOOS BioEco Portal and connect it with OceanOPS, to provide an integrated perspective of the European biological ocean observing system that can serve as a tool for EOOS to enhance coordination and integration of the European ocean observing and as a blueprint for the global community.
7 Processes: standards and best practices
Best practices (BPs), standards, and metrology play fundamental roles in ensuring the interoperability of measuring instruments and enhancing the comparability of observed data. Despite the significant progress made in recent years, fragmented standards, formats and nomenclature, and lack of information on precision and accuracy are still barriers to the effective management and use of data.
The diversity within the ocean observing community and the continuous and asynchronous evolution of technology and capacity means that there can be several “best” approaches actively used that have not been universally evaluated across the observing community. Community-endorsed BPs increase opportunities for collaboration, improve interoperability between different systems, and optimize the efficient use of time and increase the reliability of obtained data. Important work is done by the Ocean Best Practices System (OBPS)12 working towards converging the many BPs into accepted and GOOS-endorsed BPs. Sharing and adopting methodologies that are considered BPs by one or more communities can ensure a superior product. Achieving global data sharing and interoperability requires agreed and broadly adopted methods across ocean research, operations and applications, which would foster collaboration, consensus building, and innovation. With the advancement of technologies used in ocean observing and data management, there is a growing need to secure ocean data quality and interoperability by promoting the use of standardized protocols and data management practices. Creating and using GOOS ‘community’ endorsed BPs for ocean observing are key to strengthening the system and delivering robust data (Box 4).
Box 4. Recommendations for standards and best practices.
❖ We recommend strategic investments into the European Committee for Standardization (CEN), National Standardization Bodies (NSBs), Ocean Best Practices System (OBPS) and other entities advancing the harmonization of comparative sampling and analysis methods. This should also include support for developing and producing reference materials and organizing regular interlaboratory comparison exercises for relevant EOVs.
8 Outputs: data integration, assimilation and forecasting
No matter how well the requirements are thought through, and how sophisticated the observations of an EOV is, the effort is useless unless that data is available. Today, governments and research funders are increasingly insisting that generated data funded by taxpayers must follow FAIR data principles and be free and open (Tanhua et al., 2019b; Wilkinson et al., 2016). Therefore, EuroSea supported the integration of ocean data into Copernicus Marine Service (Le Traon et al., 2019), EMODnet (Martín Míguez et al., 2019) and SeaDataNet (Pecci et al., 2020). It is important to develop a data management plan that evolves throughout the life cycle of the data, i.e. from the initial planning of sustained ocean observing activities and for scientific missions. The main issue is that researchers often don’t have the support (e.g. human capacity, expertise) needed to get the data submitted into the right databases in the correct format. Other important attributes include having access to free data, at least for data funded by public resources. Similarly, there is a discussion on how the observing system can engage external partners in taking ocean observations, and how that should be rewarded by either a grant for making the observations, or as an enterprise selling the data. The players are still finding the way around this topic and it is not likely to conclude anytime soon, and in reality, we will have different systems in place. We note that since the ocean is vast, and the need for more data from the ocean is large, this is something that the science community cannot do alone, and we need to build strong ties to the private sector and the public to support the observing efforts.
Another important attribute of data delivery is the latency. Traditionally, many data streams are delivered in delayed mode, sometimes years after the observations were taken – a practice in-line with the demands for scientific research and the demands from science funding agencies. However, this is less useful for a sustained observing system serving fit-for purpose information to operational systems where there is a clear need for real-time, near-real time data (nRT), or at least fit-for-purpose for the operational systems. High speed internet providers (e.g. Starlink) will help overcome the latency issue for some ocean observing networks, such as GO-SHIP, thus enhancing future efforts to disseminate data more quickly for model data assimilation. The observing community is generally getting accustomed to delivering data to repositories faster, and one of the reasons for this is the improved data management infrastructure in Europe, that facilitates the submission and curation of the data. However, the increasing variability of ocean data collected makes efficient curation and quality control more challenging. Very often though, there is no incentive for scientists to deliver data in nRT, and hence deliver only preliminary quality controlled, to operational data centers as this is most often not part of the science project that funded the mission. Nevertheless, the utility of nRT data delivery is being more recognized by the ocean science community, and data centers have increased the capacity to handle nRT data, leading to improved data delivery times. However, there is still large room for improvement when it comes to latency of data delivery.
Ocean forecasting systems are, on the very basic level, based on ocean modeling and assimilation of observations. Large progress has been made thanks to increased computer capacity and a wealth of satellite data that are assimilated into ocean forecast models of increasing resolution. However, links between modeling/data assimilation groups and groups designing and implementing the observing system need to be better established. Also, there is a need for an efficient mechanism in place to implement the suggestions proposed by the modeling/forecasting community into the European ocean observing system. Although being the key to assimilation, satellites provide very little information of structures and the state of the interior ocean. This is true for the physical state of the ocean, but maybe even more so for biogeochemistry and for biology. There is a distinct need to assimilate in-situ ocean data to the ocean model and forecasting system. EuroSea has progressed the assimilation of different in-situ data sets into the Copernicus Marine Service ocean analysis and forecasting system. But there are many observations that are still not assimilated. It is only via an integrated approach that different observations can efficiently constrain the different scales of variability of the ocean. Assimilation of in-situ data streams are dependent on timely delivery of data with well-known attributes and with some degree of sustainability to them. In addition to data delivery and assimilation, there is a need to improve the models, in particular for biogeochemistry and biology as these tend to be less well developed. In addition to traditional ocean forecasting, the concept of Digital Twin Oceans (DTOs) is gaining momentum and offers the users a framework for efficient management and understanding of the ocean, and what effect different (management) scenarios will have on the ocean system. The UN ocean decade program DITTO supports the development of digital twins of the ocean13. In Europe, several projects work on the DTO concept, with the EDITO14 programs are two important building blocks towards a digital twin of the Ocean, where EOOS will be a key component of provide the data needed for useful DTOs (Box 5).
Box 5. Recommendations for data integration, assimilation and forecasting.
❖ We recommend to improve the delivery chain of EOV data from the observers to data centers, and to increase the capacity to assimilate these data into operational ocean models.
❖ We recommend to integrate sustained ocean data to the digital ocean (DTO) concept.
9 Impacts: delivery of services
Ocean observing is complex due to the nature of the environment in which it operates. Ocean dynamics are variable and involve a wide range of spatial and temporal scales: from seconds to long-term climate scales and from local to global scales. It is reflected in the complexity of different actors providing observations at different local, national, regional, and international levels. This calls for agreements on common agendas and principles as well as a robust governance to avoid duplication and tap the full potential of observations. It is impossible, in the majority of cases, to determine the impact of a set of observations on a user’s satisfaction or a policy area. The impact of observations can be demonstrated through emphasizing the value of connectivity, partnerships, and synergies, which enable fit-for-purpose oceanographic information, products, and services (Eparkhina et al., 2022).
Besides national priorities, we need to set clear European priorities for ocean observations to enable the shaping and implementation of the European ocean observing system responding to the needs of ocean products and service providers to serve a broad European society. Solutions and incentives for long-term sustainable funding should be sought to support the sustained observations for ocean status assessments and pan-European ocean data products and services.
User and stakeholder interactive engagement is key to facilitating communication and understanding across disciplines and institutions and the joint exchange of perspectives, ideas, and knowledge to build future systems and foster collaborative engagement. We need to improve science-policy interface and communication promoting societal and economic value of ocean observations and forecasts and enhancing cross and trans-disciplinary cooperation among ocean observers, modelers, scientists, policymakers, industry, and the general public. The use of ocean indicators is a critical tool to facilitate this dialogue (e.g. Von Schuckmann et al., 2020). Besides advancing the observing and modeling communities’ collaboration, the engagement with users of ocean observations in Europe and globally is vital for understanding observing system requirements.
Effective communication and science-policy dialogue will be vital for sustaining the ocean observing system to make sure the role of the ocean and its impacts on the world and society are clearly highlighted and that the importance of the ocean observing system for ocean research, blue economy, green energy, and security is emphasized and understood by decision-makers and the broad public (e.g. Eparkhina et al., 2022; Ortiz et al., 2023a, 2023b; Straßer et al., 2023). To gain support for the ocean observing system, it is crucial to let the observation and service/product developing communities come together with the users and policy and decision makers to identify and agree on a series of top priorities for the observing system. Communication between all actors along the ocean observing value chain should be improved to secure support for the ocean observing and forecasting system in the long term and understand the direct and indirect impacts of not sustaining ocean observations on businesses and society (Box 6).
Box 6. Recommendations for delivery of services.
❖ We recommend clearly defining essential pan-European ocean products and services and providing incentives for the Member States to support the long-term/sustained provision of data flow for those, building on existing structures.
❖ We recommend analyzing the ocean information value-chain in its ability to deliver the relevant information to users and to update its requirements quickly and efficiently to address old and emergent societal needs and concerns.
❖ We recommend developing effective policy-supporting tools such as ocean indicators or DTOs, and communication tools to highlight the role of the ocean and its impacts on climate and society.
10 Governance of ocean observing and forecasting systems
It is imperative for an ocean observing system to extend beyond the capacities of individual nations since the “whole is greater than the sum of its parts”. Achieving a robust and sustained ocean observation system demands high-level integration, enabling coordinated observations that cater to long-term needs and furnish vital information for informed decisions and policies at local, national, regional, European, and global levels. In fact, one of the major opportunities to enhance the capacity to effectively manage the ocean observing and forecasting system is an improved coordination and governance at national, European and international levels. An effective governance system is required to ensure that a well-coordinated system is in place with guarantees of maintenance/continuity of existing/future ocean observing infrastructure, sufficient data management architecture and human resources across the value chain ready to deliver the necessary ocean information needed. Since there are a myriad of different actors conducting ocean observations, or are involved in other parts of the value chain, coordination of activities is essential and will have a major positive impact in improving the efficiency of the system. The need for coordination reaches across the entire value chain, and is particularly critical for the process step with a multitude of actors, both from governmental, academic and private sectors conducting ocean observations.
For the in-situ ocean observing system, GOOS is the leading body of global coordination (e.g. Tanhua et al., 2019a), see Figure 4. Since 1991, the Global Ocean Observing System (GOOS) has been leading the development of a global ocean observing system. GOOS leads a community of international, regional and national ocean observing programs, governments, UN agencies, research organizations and individual scientists, and creates the frameworks, standards and partnerships through which all these parts can deliver an integrated global ocean observing system that serves users as effectively as possible. GOOS work is delivered through the work of three Expert Panels: Physics and climate (OOPS), biogeochemistry (IOCCP), and biology and ecology (bio/eco). The coordination of the observations is done by the Observing Coordination Group (OCG) that support the observing networks, and the GOOS Regional Associations (GRAs) coordinate the observing and forecast system on a regional level. The OceanOPS group are responsible for the operational tracking of the observing system and meta data tracking. The Expert Team on Operational Ocean Forecast Systems (ETOOFS) enables the use of ocean forecasts, whereas the Ocean Biodiversity Information System (OBIS) is a clearing house for marine biodiversity (Figure 4). The GOOS Office is headquartered at UNESCO-IOC in Paris, which is the lead sponsor of GOOS, with the co-sponsors World Meteorological Organization (WMO), United Nations Environmental Programme (UNEP) and the International Science Council (ISC).
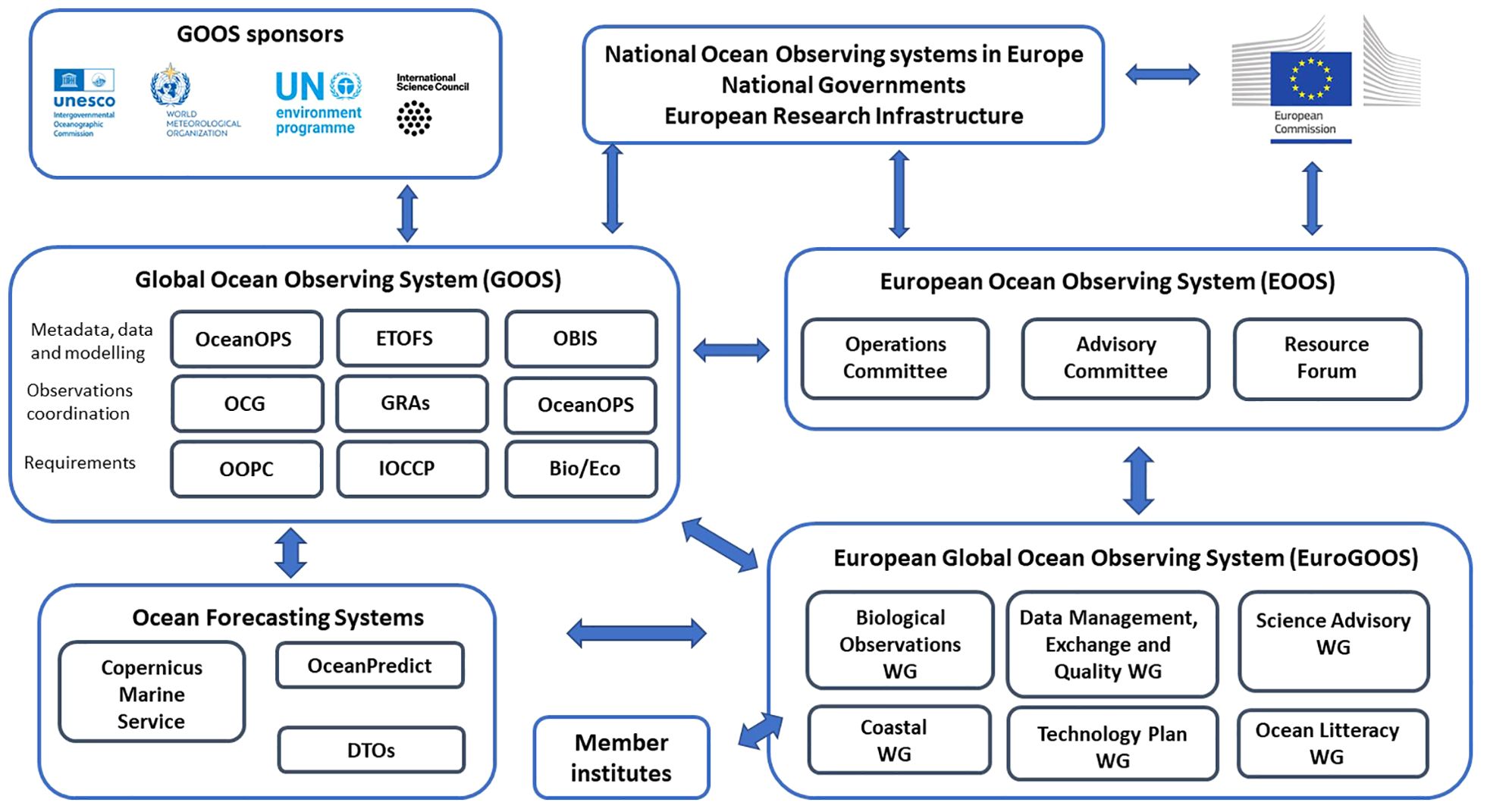
Figure 4. Sketch of the main elements of the governance of the Ocean Observing and Forecasting System in Europe and globally.
In Europe, the acknowledged GRAs15 are EuroGOOS as well as Black Sea GOOS and MonGOOS, although the latter is closely associated with EuroGOOS. EuroGOOS has been pivotal for the coordination of operational ocean observing in Europe, in particular through the system of Regional Operational Oceanographic Systems (ROOSs). EuroGOOS identifies priorities, enhances cooperation and promotes the benefits of operational oceanography to ensure sustained observations are made in Europe’s seas underpinning a suite of sustained fit-for-purpose products and services for marine and maritime users. Since its foundation in 1994, EuroGOOS has been pivotal in advancing pan-European cooperation in operational oceanographic research, technology development, capacity building, and data sharing. EuroGOOS working groups, networks of observing platforms (task teams), and regional systems (ROOSs), provide fora for cooperation, unlock quality marine data and deliver common strategies, priorities and standards.
In addition, projects such as EuroSea are very effective in advancing the ocean observing system in Europe, and globally, since advances in Europe are shared globally and vice versa. European countries play a leading role within the ocean observing community, many have active national and international ocean observing systems and programs and contribute to the global ocean observing networks (platforms) and EOV observing communities. Several principal investigators, scientists and technicians making long-term observations within national programs also play important roles in the coordination structure, supported by their nations.
The current ocean observing coordination efforts in Europe remain fragmented and need to be better integrated. Figure 4 shows one attempt to broadly show the current ocean observing governance situation in Europe, without going into a great deal of detail on different European and national systems. Note that several of the elements in the diagram are not funded, or severely under-funded, so that the efficiency of the system is not given. As the European Ocean Observing and Forecasting System evolves, the governance needs to evolve with it.
Although significant progress has been made during previous decades, Europe needs to implement the governance framework articulated in the European Ocean Observing System (EOOS)16 strategy to improve collaboration between ocean-observing networks and across the ocean observing value chain, connecting ocean-observing practitioners with marine data and information services and users. EOOS comprises the infrastructure, platforms, sensors and people that gather the essential data and information about Europe’s ocean, seas and coastal waters. EOOS’ vision is to achieve a European Ocean Observing System that is sustained and meets the specific needs of users through the EOOS Framework, a community-driven initiative. By aligning and integrating existing ocean observation initiatives, the EOOS Framework aims to ensure their quality, usefulness, efficiency and value for money. This includes avoiding duplication and finding synergies or complementarities between activities, leveraging the best possible value from Europe’s ocean observation capabilities and resources. The EOOS Framework focuses on ocean observation capability, including the infrastructure and activities engaged in coastal, open ocean, deep sea and seafloor observations in a multidisciplinary, Europe-wide transnational and cross-sectoral approach. Following EOOS’ inception in 2010, the EOOS Framework has grown from a concept to an established initiative with a clear governance structure, overseen by a Steering Group chaired by EuroGOOS and the EMB. Three further expert bodies contribute to specific aspects of EOOS’ development: An Advisory Committee, a Resource Forum, and an Operations Committee.
Through improved coordination, the significant ocean observing capacity in European can be optimized to meet a broad range of users’ needs most effectively, and new observing activities and services can be developed to meet emerging societal concerns and requirements. The EOOS is currently co-designed with a broad range of actors to build a European ocean observing system that is sustained and meets the specific needs of users, and provide a governance framework. Establishing an EOOS Coordination Office will consolidate the fragmented coordination efforts, optimize the use of existing resources, facilitate communication between different entities, and advance in implementing EOOS.
The majority of ocean observing activities are funded at the national level where ocean observations are conducted by a variety of organizations to fulfill national priorities (e.g. climate adaptation), European Member State regulations (national and EC Directives) and policy. Many nations have signed up to deliver on international commitments, e.g. networks such as Argo (Roemmich et al., 2019) and GO-SHIP (Sloyan et al., 2019). In addition, European Research Infrastructure Consortia (ERICs) play an increasingly important role in Europe. Most national ocean observing efforts are however still insufficiently coordinated which leads to inefficiencies. To advance the coordination and efficient use of resources at the Member State level, the formation of coordination structures for national ocean observing systems has to be facilitated. To maximize the use of resources and lift up meaningful collaborations at the European scale, a common, pan-European ocean observation planning platform to share information on plans and collaboration opportunities is needed together with an observing system performance monitoring tool and EOOS Technical Support Centre to strengthen coordination, interoperability, best practices drafting, reviewing and endorsement, and data harmonization. European policies and strategies are largely shaping the national marine observing plans, and even though these policies and strategies are meant to be integrated, there is still a lot of coordination that needs to be done at the national and regional level to avoid duplication and use resources more efficiently.
For national ocean forecasting systems international coordination is guided by the Expert Team on Operational Ocean Forecast Systems (ETOOFS, one of GOOS components), focusing on the operational aspects of ocean forecasting, and OceanPredict, the science programme for the coordination and improvement of global and regional ocean analysis and forecasting systems of the UN Decade for Ocean Science17. The UN Decade Collaborative Center for Ocean Prediction (DCC-OP18) is now also playing a leading role to coordinate ocean forecasting activities across the UN Decade activities. The European ocean forecasting systems are mainly organized and governed by the EU Copernicus program, e.g. Marine Service implemented by Mercator Ocean International through a contributing agreement with the European Commission. The Copernicus Marine Service (Le Traon et al., 2019) provides operational, regular, and systematic reanalyses, analyses and forecasts on the physical, biogeochemical and sea ice state, for the global ocean and European regional seas using both satellite and in-situ observations. Coastal forecasting services are organized downstream of Copernicus Marine by Member States as part of EuroGOOS (Box 7).
Box 7. Recommendations for governance of ocean observing and forecasting systems.
❖ We recommend the formation of coordination structures for national ocean observing activities to engage all national ocean observing funding/coordinating institutions and relevant sectors to better coordinate their activities to use available resources (financial, technological, and human) more efficiently and across sectors where possible and feasible.
❖ We recommend establishing and resourcing a European Ocean Observing System Coordination Office to facilitate regional and pan-European coordination and effectively manage and integrate European ocean observing operations.
❖ We recommend forming a decision-making body of high-level Member State national system representatives for targeted discussions on priorities and co-designing the observing system that supports societal needs in different countries and in Europe but also contributes to the Global Ocean Observing System (GOOS).
❖ We recommend long-term strategic investments in tools and entities that strengthen coordination, interoperability, best practices and data harmonization - leveraging international frameworks where feasible.
❖ We recommend to keep and increase the support for current ocean observing coordination structures such as the Global Ocean Observing System (GOOS) and the regional associations such as EuroGOOS.
11 Sustainability of operations
Even though the advantages of a fully functioning and sustainable ocean observing system are numerous, the necessary investments for making it fully operational and efficient are lagging. One possible reason is that the benefits are not clearly articulated, visible, easily measurable or comparable, in contrast to the capital and operational costs for deploying such a system, which are more readily available. This could lead to flawed business cases, leading to underinvestment in sustained ocean observations. Cost overview and estimation of the benefits is necessary to make an appropriate assessment (EMB, 2021).
One of the most significant identified overarching challenges in the European ocean observing and forecasting system is the sustainability of long-term routine observations relevant to monitoring the trends of key ocean phenomena and their variability through time. This would be needed in order to improve our knowledge about the ocean function, providing data essential for science-based decision making, and safeguarding the development and continuity of products and services critical for society (e.g., weather forecasts, tsunami warnings, biodiversity reporting). Besides requesting Member States’ long-term commitment to ocean observing, we need to improve the visibility of observing activities. While science and operational services are strong drivers for all observing networks, the wide mixture of funding sources, often short-term and project driven, makes long-term planning difficult. In fact, even the “sustained” ERICs rely heavily on short term funds from projects, for a significant part of their activity, and the membership fees only partially cover activities.
The systematic data collection and use of quality-assured data for assessing the marine environmental status and planning the mitigation and management actions require significant organization, commitment and hence economic investments at both national, regional and European levels. Long-term commitments are already made by the Member States under the Water Framework Directive (WFD) and Marine Strategy Framework Directive (MSFD), but additional investments made by national or local governments or government institutions, or at the European Union (EU) level, are mainly through short-term project funding. If we are to meet the innovation challenges of ocean observing, long-term sustainable commitments are required above and beyond the reliance on scientific research budgets (Révelard et al., 2022). We need partnerships and expertise from the private sector to develop ocean observing technologies and encompass field operations. Cooperation between the public and private sectors can evolve a thriving ocean observing enterprise and the blue economy, benefiting business, government and society (EMB, 2021).
Strategies and plans that are formulated at the regional level are often not fully implemented or findable/accessible for the broader community, nor are they tracked for implementation. Foresight and planning are crucial to support the development of a sustained, fit-for-purpose ocean observing system. Foresight initiatives are identified to help analyze how the governance of the observing systems can best respond to the relevant international and European legal frameworks and mechanisms focusing on sustained ocean observing. Further work identified the need for clear, regularly revised European priorities on what to observe and what the key European products and services supported by the Member States should be. Identifying priorities in European ocean observing and forecasting needs to consider the legal requirements at the national, European and international scales (e.g. Van Doorn and Veloso, 2023), but also respond to emerging societal needs.
Marine Research Infrastructures (RIs) offer an efficient coordination mechanism and, to some extent, counterbalance problems at the national level, providing the opportunity for individual observing systems to be integral parts of a harmonized integrated structure. Higher engagement by Member States will strengthen the RIs, enabling them to serve diverse users more efficiently by offering more and better products and services. It would also support the coordination, collaboration and harmonization in observations and data management, building a solid sustainable block of the EOOS. Considering grand challenges, such as climate change, EU’s marine RIs, and in particular the ERICs, can play a significant role as the European contribution to global efforts and as an efficient mechanism connecting national and regional systems with systems outside Europe (Box 8).
Box 8. Recommendations for the sustainability of operations.
❖ We recommend that the European Commission incentivizes Member States to join and support European-wide marine Research Infrastructures and data collection to support the central provision of sustained ocean information from all its sea basins.
❖ We recommend to improve the implementation of the international legal framework for ocean observing in order to enable a sustained ocean observing system for societal benefit.
❖ We recommend supporting innovation and industry engagement to advance ocean observing technologies development and integration. The EOOS Technology Forum can be a great venue for connecting the ocean observing community, including the private sector and funders.
❖ We recommend a broad cost-benefit analysis of the European ocean observing system.
❖ We recommend securing long-term funding schemes at the national and European levels for ocean observing to ensure the continuous delivery of ocean information. A co-funding mechanism between Member States and the European Commission should be set up for global and pan-European in-situ ocean observing systems.
12 Conclusions
EuroSea strengthened the European and Global Ocean Observing Systems (EOOS, EuroGOOS and GOOS) governance and implementation, and improved European and international coordination, working towards bringing individual observing elements into an integrated observing system. EuroSea connected users with the operators of the observing system and information providers. Moreover, during the project, existing gaps in the observing and forecasting of the Essential Ocean Variables (EOVs) were identified, and challenges in national, regional and international collaboration and coordination, management, reporting of the European ocean observing and forecasting system were described (e.g. Hassoun et al., 2023, 2024).
This article paints, in broad strokes, some of the most urgent needs along the European ocean observing value chain, as contribution to the global system. It is not a complete picture but represents a consorted view of the EuroSea community based on 4 years of work within the consortium, as reflected in some of the reports from EuroSea cited in this article, as well as the EuroSea Legacy Report (Eparkhina et al., 2023). An important outcome of EuroSea is the tangible results in form of services and products that the three demonstrator work packages produced for the societal benefit areas of ocean health, operational services and climate. The Eurosea Legacy report provides an overview of EuroSea results (Eparkhina et al., 2023). EuroSea has demonstrated the importance of addressing the ocean observing value chain as a whole, from data acquisition to processing, modeling, forecasting and services. The impacts of this work on stakeholders promote sustainability in the value chain, based on sustained and systematic ocean observing. The vision of EuroSea is to expand on those results and disseminate EuroSea innovations to other partners and geographical areas, and that these can serve as an inspiration for the ocean observing and forecasting community to keep engaging with both the stakeholders, users and implementers of the system to improve ocean information services and products, for the benefit of society, blue economy and science.
To achieve a European Ocean Observing System (EOOS) that is sustained and meets the specific needs of users, supported pan-European coordination is needed. This article has articulated some key recommendations from EuroSea on needs for the improving European ocean observing and forecasting towards delivering the information needed for a sustainable ocean.
Data availability statement
The original contributions presented in the study are included in the article/supplementary material. Further inquiries can be directed to the corresponding author.
Author contributions
TT: Writing – review & editing, Writing – original draft, Investigation, Conceptualization. P-YT: Writing – review & editing, Investigation. NK: Writing – review & editing, Investigation. DE: Writing – review & editing, Investigation. GN: Writing – review & editing, Investigation. MD: Writing – review & editing, Investigation. SS: Writing – review & editing, Investigation. AP: Writing – review & editing, Investigation. KV: Writing – review & editing, Investigation. GL: Writing – review & editing, Investigation. JK: Writing – review & editing, Investigation. AH: Writing – review & editing, Investigation. EV: Writing – review & editing, Investigation. BB-L: Writing – review & editing, Investigation. BP: Writing – review & editing, Investigation. CC: Writing – review & editing, Investigation. EH: Writing – review & editing, Investigation. AL-L: Writing – review & editing, Investigation. GP: Writing – review & editing, Investigation. MT: Writing – review & editing, Investigation. AP: Writing – review & editing, Investigation. KW-B: Writing – review & editing, Investigation. JP: Writing – review & editing, Investigation. ÁM: Writing – review & editing, Investigation. JH: Writing – review & editing, Investigation. IL: Writing – review & editing, Writing – original draft, Investigation.
Funding
The author(s) declare financial support was received for the research, authorship, and/or publication of this article. This study was fully funded by the European Union’s Horizon 2020 research and innovation programme under grant agreement No 862626, as part of the EuroSea project.
Acknowledgments
We acknowledge the H2020 EuroSea project which made this study possible. The EuroSea project aims to improve the European ocean observing system as an integrated entity within a global context, delivering ocean observations and forecasts to advance scientific knowledge about ocean climate, marine ecosystems, and their vulnerability to human impacts and to demonstrate the importance of the ocean to an economically viable and healthy society.
Conflict of interest
The authors declare that the research was conducted in the absence of any commercial or financial relationships that could be construed as a potential conflict of interest.
The author(s) declared that they were an editorial board member of Frontiers, at the time of submission. This had no impact on the peer review process and the final decision.
Publisher’s note
All claims expressed in this article are solely those of the authors and do not necessarily represent those of their affiliated organizations, or those of the publisher, the editors and the reviewers. Any product that may be evaluated in this article, or claim that may be made by its manufacturer, is not guaranteed or endorsed by the publisher.
Footnotes
- ^ https://eurosea.eu/deliverables/
- ^ https://eurosea.eu/deliverables/
- ^ https://emodnet.ec.europa.eu/en/checkpoints
- ^ https://gcos.wmo.int/en/global-climate-indicators
- ^ https://goosocean.org/document/33315
- ^ https://helcom.fi/about-us/convention/
- ^ https://www.ospar.org/
- ^ https://www.unep.org/unepmap/
- ^ https://www.unep.org/bucharest-convention
- ^ https://www.ocean-ops.org/board
- ^ https://bioeco.goosocean.org/
- ^ https://www.oceanbestpractices.org/
- ^ https://ditto-oceandecade.org/about/
- ^ https://www.edito.eu/
- ^ https://goosocean.org/who-we-are/goos-regional-alliances/
- ^ https://www.eoos-ocean.eu/
- ^ https://oceandecade.org/
- ^ https://oceandecade.org/actions/decade-collaborative-centre-for-ocean-prediction-dcc-op/
References
Barceló-Llull B., Pascual A. (2023). Recommendations for the design of in situ sampling strategies to reconstruct fine-scale ocean currents in the context of SWOT satellite mission. Front. Mar. Sci. 10. doi: 10.3389/fmars.2023.1082978
Ciliberti S. A., Alvarez Fanjul E., Pearlman J., Wilmer-Becker K., Bahurel P., Ardhuin F., et al. (2023). “Evaluation of operational ocean forecasting systems from the perspective of the users and the experts,” in 7th edition of the Copernicus Ocean State Report (OSR7), Vol. 2. 1–osr7. doi: 10.5194/sp-1-osr7-2-2023
Dayan H., Mcadam R., Juza M., Masina S., Speich S. (2023). Marine heat waves in the Mediterranean Sea: An assessment from the surface to the subsurface to meet national needs. Front. Mar. Sci. 10. doi: 10.3389/fmars.2023.1045138
Dayan H., Mcadam R., Masina S., Speich S. (2022). Diversity of marine heatwave trends across the Mediterranean Sea over the last decades. Copernicus Ocean State Report. J. Operational Oceanogr. (6), 57–643. doi: 10.1080/1755876X.2022.2095169
deYoung B., Visbeck M., De Araujo Filho M. C., Baringer M. O. N., Black C., Buch E., et al. (2019). An integrated all-atlantic ocean observing system in 2030. Front. Mar. Sci. 6. doi: 10.3389/fmars.2019.00428
Dunbar M., Alvarez D., Macias D., Ramirez E., Derhy G., Daly E., et al. (2023). Linking oceanographic products to fisheries advice. EuroSea Deliverable. GEOMAR D6.4, 18. doi: 10.3289/eurosea_d6.4
EMB (2021). “Sustaining in situ ocean observations in the age of the digital ocean,” in EMB policy brief. (Oostende, Belgium: European Marine Board). doi: 10.5281/zenodo.4836060
Eparkhina D., Hibbert A., León M. G., Köstner N., Tanhua T., Doorn E. V., et al. (2022). Lessons learnt on science-policy interfaces. EuroSea Deliverable D8.3, 34. doi: 10.3289/eurosea_d8.3
Eparkhina D., Keogh C., Tanhua T., Köstner N., Heslop E., Reilly K., et al. (2023). EuroSea legacy report, EuroSea deliverable D8.12, 23. doi: 10.3289/eurosea_d8.12
Fujii Y., Rémy E., Zuo H., Oke P., Halliwell G., Gasparin F., et al. (2019). Observing system evaluation based on ocean data assimilation and prediction systems: on-going challenges and a future vision for designing and supporting ocean observational networks. Front. Mar. Sci. 6. doi: 10.3389/fmars.2019.00417
Gasparin F., Lellouche J.-M., Cravatte S. E., Ruggiero G., Rohith B., Le Traon P. Y., et al. (2023). On the control of spatial and temporal oceanic scales by existing and future observing systems: An observing system simulation experiment approach. Front. Mar. Sci. 10. doi: 10.3389/fmars.2023.1021650
Graves I., Pereiro D., Dunbar M., Cusack C. (2023). Real-time data to central server with display to stakeholders. EuroSea Deliverable. GEOMAR D6.7, 13. doi: 10.3289/eurosea_d6.7
Grégoire M., Garçon V., Garcia H., Breitburg D., Isensee K., Oschlies A., et al. (2021). A global ocean oxygen database and atlas for assessing and predicting deoxygenation and ocean health in the open and coastal ocean. Front. Mar. Sci. 8. doi: 10.3389/fmars.2021.724913
Hassoun A. E. R., Tanhua T., Lips I., Heslop E., Petihakis G., Karstensen J. (2024). The European Ocean Observing Community: urgent gaps and recommendations to implement during the UN Ocean Decade. Front. Mar. Sci. 11. doi: 10.3389/fmars.2024.1394984
Hassoun A. E. R., Tanhua T., Petihakis G., Lips I., Heslop E. (2023). Report on gaps in the european ocean observing and forecasting system. EuroSea Deliverable D1.9, 185. doi: 10.3289/eurosea_d1.9
Hoffman R. N., Atlas R. (2016). Future observing system simulation experiments. Bull. Am. Meteorological Soc. 97, 1601–1616. doi: 10.1175/BAMS-D-15-00200.1
IPBES (2019). Global assessment report on biodiversity and ecosystem services of the Intergovernmental Science-Policy Platform on Biodiversity and Ecosystem Services. Eds. Brondizio E. S., Settele J., Díaz S., & Ngo H. T. (Bonn, Germany: IPBES secretariat). Available at: https://doi.org/10.5281/zenodo.3831673.
IPCC (2021). Climate change 2021: the physical science basis. Contribution of working group I to the sixth assessment report of the intergovernmental panel on climate change. Eds. Masson-Delmotte V., P. Z., Pirani A., Connors S. L., Péan C., Berger S., Caud N., Chen Y., Goldfarb L., Gomis M. I., Huang M., Leitzell K., Lonnoy E., Matthews J. B. R., Maycock T. K., Waterfield T., Yelekçi O., Yu R., & Zhou B. (Cambridge, United Kingdom and New York, NY, USA: Cambridge University Press). doi: 10.1017/9781009157896
Karstensen J., Petihakis G., Fernandez V. (2020). Observing networks initial assessment. EuroSea deliverable D3.2, 32. doi: 10.3289/eurosea_d3.2
Lange N., Tanhua T., Pfeil B., Bange H. W., Lauvset S. K., Grégoire M., et al. (2023). A status assessment of selected data synthesis products for ocean biogeochemistry. Front. Mar. Sci. 10. doi: 10.3389/fmars.2023.1078908
Le Traon P. Y., Reppucci A., Alvarez Fanjul E., Aouf L., Behrens A., Belmonte M., et al. (2019). From observation to information and users: the copernicus marine service perspective. Front. Mar. Sci. 6. doi: 10.3389/fmars.2019.00234
Lindstrom E., Gunn J., Fischer A., Mccurdy A., Glover L., Alverson K., et al. (2012). “. A framework for ocean observing,” in By the task team for an integrated framework for sustained ocean observing (Paris, France: UNESCO). doi: 10.5270/OceanObs09-FOO
Martín Míguez B., Novellino A., Vinci M., Claus S., Calewaert J.-B., Vallius H., et al. (2019). The european marine observation and data network (EMODnet): visions and roles of the gateway to marine data in europe. Front. Mar. Sci. 6. doi: 10.3389/fmars.2019.00313
Mignot A., Claustre H., Cossarini G., D'ortenzio F., Gutknecht E., Lamouroux J., et al. (2023). Using machine learning and Biogeochemical-Argo (BGC-Argo) floats to assess biogeochemical models and optimize observing system design. Biogeosciences 20, 1405–1422. doi: 10.5194/bg-20-1405-2023
Ortiz V., Rodriguez R., Tintoré J., Reilly K., Strasser F.-E., Koestner N., et al. (2023a). Lessons learnt from the EuroSea public engagement activities. EuroSea Deliverable. GEOMAR D8.5, 29. doi: 10.3289/eurosea_d8.5
Ortiz V., Tintoré J., Köstner N. (2023b). EuroSea Guide of communication and dissemination activities for enhanced visibility of innovation in ocean observing and forecasting for a sustainable ocea. EuroSea Deliverable. GEOMAR D8.10, 35. doi: 10.3289/eurosea_d8.10
Palacz A., Telszewski M., Tanhua T., Heslop E. (2022). Marine Plastics EOV and common sampling protocol. EuroSea deliverable D1.5, 34. doi: 10.3289/eurosea_d1.5
Pecci L., Fichaut M., Schaap D. (2020). SeaDataNet, an enhanced ocean data infrastructure giving services to scientists and society. IOP Conf. Series: Earth Environ. Sci. 509, 12042. doi: 10.1088/1755-1315/509/1/012042
Pereiro D., Korolev O. B., Dunbar M. B., Navarro G., Cusack C., Conway A., et al. (2023). Best practice on creating "Extreme Marine Events" Hazard maps & forecasts Report. EuroSea Deliverable. GEOMAR D6.3, 23. doi: 10.3289/eurosea_d6.3
Petihakis G., Karstensen J., Fernandez V. (2023). Observing networks final assessment. EuroSea deliverable D3.18, 95. doi: 10.3289/eurosea_d3.18
Ratnarajah L., Heslop E., Lips I., Simpson P., Nordlund L. M., Unsworth R., et al. (2022). Report on the European BioEco observing system. EuroSea Deliverable. GEOMAR D1.4, 23. doi: 10.3289/eurosea_d1.4
Révelard A., Tintoré J., Verron J., Bahurel P., Barth J. A., Belbéoch M., et al. (2022). Ocean integration: the needs and challenges of effective coordination within the ocean observing system. Front. Mar. Sci. 8. doi: 10.3389/fmars.2021.737671
Roemmich D., Alford M. H., Claustre H., Johnson K., King B., Moum J., et al. (2019). On the future of argo: A global, full-depth, multi-disciplinary array. Front. Mar. Sci. 6. doi: 10.3389/fmars.2019.00439
Rosselló P., Pascual A., Combes V. (2023). Assessing marine heat waves in the Mediterranean Sea: a comparison of fixed and moving baseline methods. Front. Mar. Sci. 10. doi: 10.3389/fmars.2023.1168368
Schuckmann K. V., Traon P.-Y. L., N. Smith A. P., Djavidnia S., Gattuso J.-P., Grégoire M. (2022). Copernicus ocean state report. J. Operational Oceanography 6, 1–220. doi: 10.1080/1755876X.2022.2095169
Sloyan B. M., Wilkin J., Hill K. L., Chidichimo M. P., Cronin M. F., Johannessen J. A., et al. (2019). Evolving the physical global ocean observing system for research and application services through international coordination. Front. Mar. Sci. 6. doi: 10.3389/fmars.2019.00449
Straßer F.-E., Köstner N., Eparkhina D., Noronha A., Ortiz V. (2023). Recommendations on engaging with the next generation of stakeholders. EuroSea Deliverable. GEOMAR D8.11, 65. doi: 10.3289/eurosea_d8.11
Tanhua T., Mccurdy A., Fischer A., Appeltans W., Bax N., Currie K., et al. (2019a). What we have learned from the framework for ocean observing: evolution of the global ocean observing system. Front. Mar. Sci. 6. doi: 10.3389/fmars.2019.00471
Tanhua T., Pouliquen S., Hausman J., O’brien K., Bricher P., De Bruin T., et al. (2019b). Ocean FAIR data services. Front. Mar. Sci. 6. doi: 10.3389/fmars.2019.00440
Turpin V., Heslop E., Tanhua T., Carval T., Pouliquen S. (2022). Maps and metrics on observing systems and metadata. EuroSea Deliverable. GEOMAR 1.6, 52. doi: 10.3289/eurosea_d1.6
Van Doorn R., Veloso P. F. (2023). Report on the use of legal frameworks for ocean observing systems. EuroSea Deliverable. GEOMAR D1.7, 23. doi: 10.3289/eurosea_d1.7
Visbeck M. (2018). Ocean science research is key for a sustainable future. Nat. Commun. 9, 690. doi: 10.1038/s41467-018-03158-3
Von Schuckmann K., Holland E., Haugan P., Thomson P. (2020). Ocean science, data, and services for the UN 2030 Sustainable Development Goals. Mar. Policy 121, 104154. doi: 10.1016/j.marpol.2020.104154
Wilkinson M. D., Dumontier M., Aalbersberg I. J., Appleton G., Axton M., Baak A., et al. (2016). The FAIR Guiding Principles for scientific data management and stewardship. Sci. Data 3, 160018. doi: 10.1038/sdata.2016.18
Keywords: ocean observing, governance, ocean science, forecasting, global ocean observing system
Citation: Tanhua T, Le Traon P-Y, Köstner N, Eparkhina D, Navarro G, Dunbar MB, Speich S, Pascual A, von Schuckmann K, Liguori G, Karstensen J, Hassoun AER, van Doorn E, Barceló-Llull B, Gómez BP, Cusack C, Heslop E, Lara-Lopez A, Petihakis G, Telszewski M, Palacz AP, Wilmer-Becker K, Pearlman J, Muñiz Piniella Á, Heymans JJ and Lips I (2024) Towards a sustained and fit-for-purpose European ocean observing and forecasting system. Front. Mar. Sci. 11:1394549. doi: 10.3389/fmars.2024.1394549
Received: 01 March 2024; Accepted: 16 September 2024;
Published: 16 October 2024.
Edited by:
Salvatore Marullo, Energy and Sustainable Economic Development (ENEA), ItalyReviewed by:
Rosalia Santoleri, National Research Council (CNR), ItalySimona Simoncelli, National Institute of Geophysics and Volcanology, Italy
Copyright © 2024 Tanhua, Le Traon, Köstner, Eparkhina, Navarro, Dunbar, Speich, Pascual, von Schuckmann, Liguori, Karstensen, Hassoun, van Doorn, Barceló-Llull, Gómez, Cusack, Heslop, Lara-Lopez, Petihakis, Telszewski, Palacz, Wilmer-Becker, Pearlman, Muñiz Piniella, Heymans and Lips. This is an open-access article distributed under the terms of the Creative Commons Attribution License (CC BY). The use, distribution or reproduction in other forums is permitted, provided the original author(s) and the copyright owner(s) are credited and that the original publication in this journal is cited, in accordance with accepted academic practice. No use, distribution or reproduction is permitted which does not comply with these terms.
*Correspondence: Toste Tanhua, ttanhua@geomar.de
†ORCID: Erik van Doorn, orcid.org/0009-0000-5951-7926