- 1GEOMAR Helmholtz Centre for Ocean Research Kiel, Kiel, Germany
- 2Ecosystem Processes Department, Institute of Marine Research, Tromsø, Norway
- 3Department of Zoology, Kazan Federal University, Kazan, Russia
- 4Laboratory of Marine Research, Zoological Institute of Russian Academy of Sciences, Saint−Petersburg, Russia
Climate change is threatening marine ecosystems on a global scale but particularly so in the Arctic. As a result of warming, species are shifting their distributions, altering marine communities and predator-prey interactions. This is known as the Atlantification of the Arctic. Warming may favor short-lived, opportunistic species such as cephalopods, marine mollusks that previously have been hypothesized to be winners in an ocean of change. To detect temporal regional trends in biodiversity, long-term annual surveys in hotspots of climate change are an unparalleled source of data. Here, we use 18 years of annual bottom trawl data (2005–2022) to analyse cephalopods in the western Barents Sea. More specifically, our research goals are to assess temporal trends in cephalopod fauna composition, abundance and biomass, and to relate these trends to climate change in the western Barents Sea. Main changes in cephalopod diversity and distribution occurred in mid-2000s and early 2010s, which corresponds with a period of warming in the Arctic since the late 1990s/early 2000s. Repeated increased occurrence of the boreal-subtropical cephalopods was recorded from 2005–2013 to 2014–2022. Moreover, the abundance of cephalopods in the area (in general and for most taxa) increased from 2005–2013 to 2014–2022. These observations suggest that the cephalopod community of the Barents Sea is subjected to Atlantification since the 2005–2013 period. This corresponds with previously reported evidence of the Atlantification in fishes and benthic invertebrates in the Barents Sea and benthic invertebrates. ‘Typical’ Arctic cephalopod species such as Bathypolypus spp., Gonatus fabricii and Rossia spp., however, are still much more abundant in the western Barents Sea compared to the deep-sea and the boreal-subtropical species. We also found indirect indications for body-size reduction in Bathypolypus spp. from 2005–2013 to 2014–2022. Overall, the temporal trends in the Barents Sea cephalopod fauna provide evidence for changing marine communities in the Arctic.
1 Introduction
The Arctic is heavily affected by climate change (Lind et al., 2018; Praetorius et al., 2018; Rantanen et al., 2022) with increase of temperature, decrease of sea-ice extent, weakening of ocean stratification, and changes in physical ocean dynamics and hydrochemistry (Brandt et al., 2023; Gerland et al., 2023). The Barents Sea is one of the fastest warming areas in the Arctic (Overland et al., 2014; Lind et al., 2018; Gerland et al., 2023). This sea is of high commercial importance due to its biological and mineral resources (Moe and Jørgensen, 2013; Jørgensen et al., 2020; Mikkelsen et al., 2023). The Barents Sea is simultaneously impacted by anthropogenic activities and climate change (Moe and Jørgensen, 2013; Jørgensen et al., 2020; Mikkelsen et al., 2023) but with largely unknown consequences for the marine communities in the area.
Due to ocean warming, many warm-water species are entering the Arctic from the Atlantic, causing changes in biomass, distribution and ecology of local Arctic species and altering predator-prey interactions (Brandt et al., 2023; Gerland et al., 2023). The changes in the Arctic ecosystems, which make them more similar to the North Atlantic ecosystems over time, are known as the ‘Atlantification’ (Jørgensen et al., 2016). The Atlantification of the Barents Sea is best studied for pelagic and demersal fishes (Fossheim et al., 2015; Frainer et al., 2017), but less so for benthic invertebrate communities (Renaud et al., 2015; Jørgensen et al., 2019). One of the reasons for our poor understanding of the extent of the Atlantification of seafloor communities is the understudied benthic biodiversity and difficulty in obtaining temporal trends in Arctic benthos (CAFF, 2017; Jørgensen et al., 2022).
One abundant but particularly understudied group of invertebrates in the Arctic are cephalopods (Phylum Mollusca, Class Cephalopoda) (Nesis, 1987; Golikov et al., 2013; Xavier et al., 2018). Despite their relatively low biodiversity in the Arctic, cephalopods have a high ecological importance in the regional food webs (Nesis, 1987; Bjørke and Gjøsaeter, 1998; Gardiner and Dick, 2010; Golikov et al., 2013; Xavier et al., 2018). They attain high regional biomass, are important as prey and predators, and have high ecological diversity (Bjørke and Gjøsaeter, 1998; Gardiner and Dick, 2010; Golikov et al., 2013; Xavier et al., 2018). Cephalopods are challenging to identify morphologically (e.g., Nozères and Roy, 2021; this study, below), and genetic identification of certain species is hampered by limited availability of reference sequences in GenBank (e.g., Fernández-Álvarez et al., 2021; Katugin and Zolotova, 2023; Taite et al., 2023). Finally, cephalopods respond opportunistically to climate change, resulting in increasing regional biomass, changing size-at-maturity and expanding geographical ranges (e.g., Pecl and Jackson, 2008; Hoving et al., 2013a; Doubleday et al., 2016; Xavier et al., 2018; Golikov et al., 2019b; Oesterwind et al., 2022). In the mid-2000s and early 2010s, northward range expansions in the Barents Sea and adjacent areas of the Nordic Seas of 2000 km were documented for two boreal-subtropical squid species (Todaropsis eblanae and Teuthowenia megalops) and of 100 km for one sepiolid (Sepietta oweniana) (Sabirov et al., 2009, Sabirov et al., 2012; Golikov et al., 2013, Golikov et al., 2014). In the mid-2000s, the Arctic squid species (Gonatus fabricii) increased its range over the eastern Barents Sea and adjacent deep waters of the Kara Sea (Golikov et al., 2012, Golikov et al., 2013). These areas were previously too cold for this species (Nesis, 1987, Nesis, 2001).
To regulate human activities in important and geographically extensive systems, monitoring of marine ecosystems’ structure and functioning is needed. An example is the Norwegian-Russian Ecosystem Survey that has taken place annually since 2003 in the Barents Sea (Michalsen et al., 2011; Eriksen et al., 2018). The long term benthos data from this survey were recently used in management decisions. Management assigned specific seafloor grounds with vulnerable benthos species in the northern Barents Sea and adjacent areas of the Central Polar Basin to be closed for commercial fisheries as a response to northward migrating commercial fish stocks and fishing fleets (Jørgensen et al., 2020). Here, we use annual bottom trawl data from this survey spanning across 18 years (2005–2022) to study the cephalopods in the western Barents Sea and adjacent areas of the Nordic Seas and Central Polar Basin. We aim: 1) to describe the temporal dynamics of cephalopod community composition; and 2) to assess the temporal dynamics of cephalopod abundance and biomass.
2 Materials and methods
2.1 Study area, survey details, sampling and identification
The study area includes the western Barents Sea, the adjacent marginal areas of the Norwegian and Greenland Seas, and of the Central Polar Basin covered by the bottom trawl stations of the Norwegian-Russian Ecosystem Survey (eastward to 40° E) (Figure 1). Since 2004, the bottom trawling within this survey has been standardized to the use of a Campelen-1800 shrimp trawl by all the participating four research vessels (RVs) (Michalsen et al., 2011; Eriksen et al., 2018; Zakharov et al., 2018). All data from the 2005–2022 period were included in the analyses, except from three RVs for 2005, where cephalopods were not identified within benthos catch. The study period was separated into two equal periods for comparative purposes (2005–2013 and 2014–2022). Overall, 2720 and 1609 bottom trawl stations were performed in the studied area during the 2005–2013 and 2014–2022 period, respectively (Table 1; Supplementary Table 1).
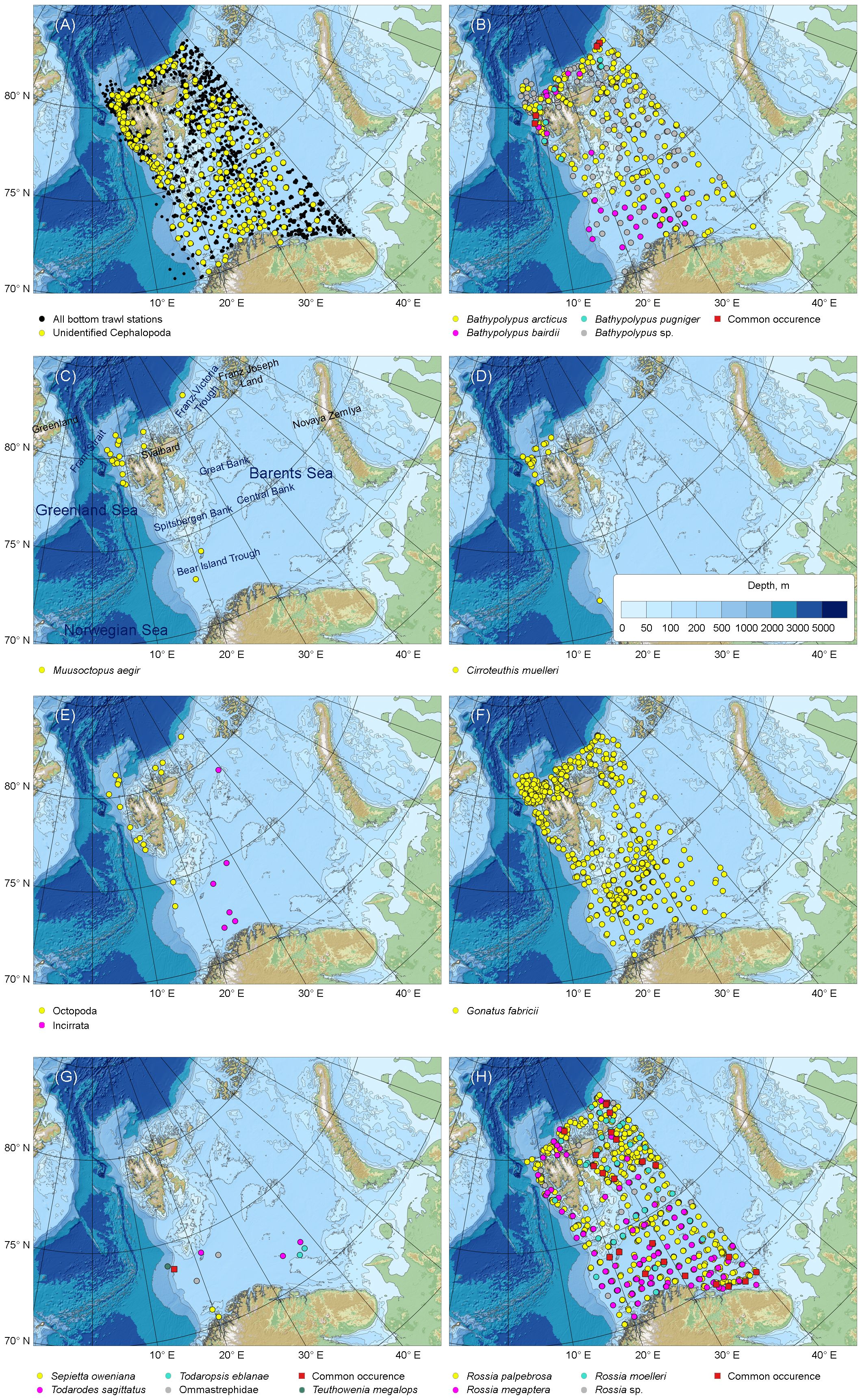
Figure 1 Bottom trawl station taken during 2005–2022 within the Norwegian-Russian Ecosystem Survey in the western Barents Sea, and distribution of cephalopods caught by bottom trawl stations. (A) All bottom trawl stations and unidentified Cephalopoda. (B) Bathypolypus spp. (C) Muusoctopus aegir. (D) Cirroteuthis muelleri. (E) Unidentified Incirrata and Octopoda. (F) Gonatus fabricii. (G) Boreal-subtropical species. (H) Rossia spp. Blank maps made with Natural Earth and IBCAO V. 4.2 (Jakobsson et al., 2020). Common occurrence in (B, G, H) indicated stations where more than one of the represented species were caught.
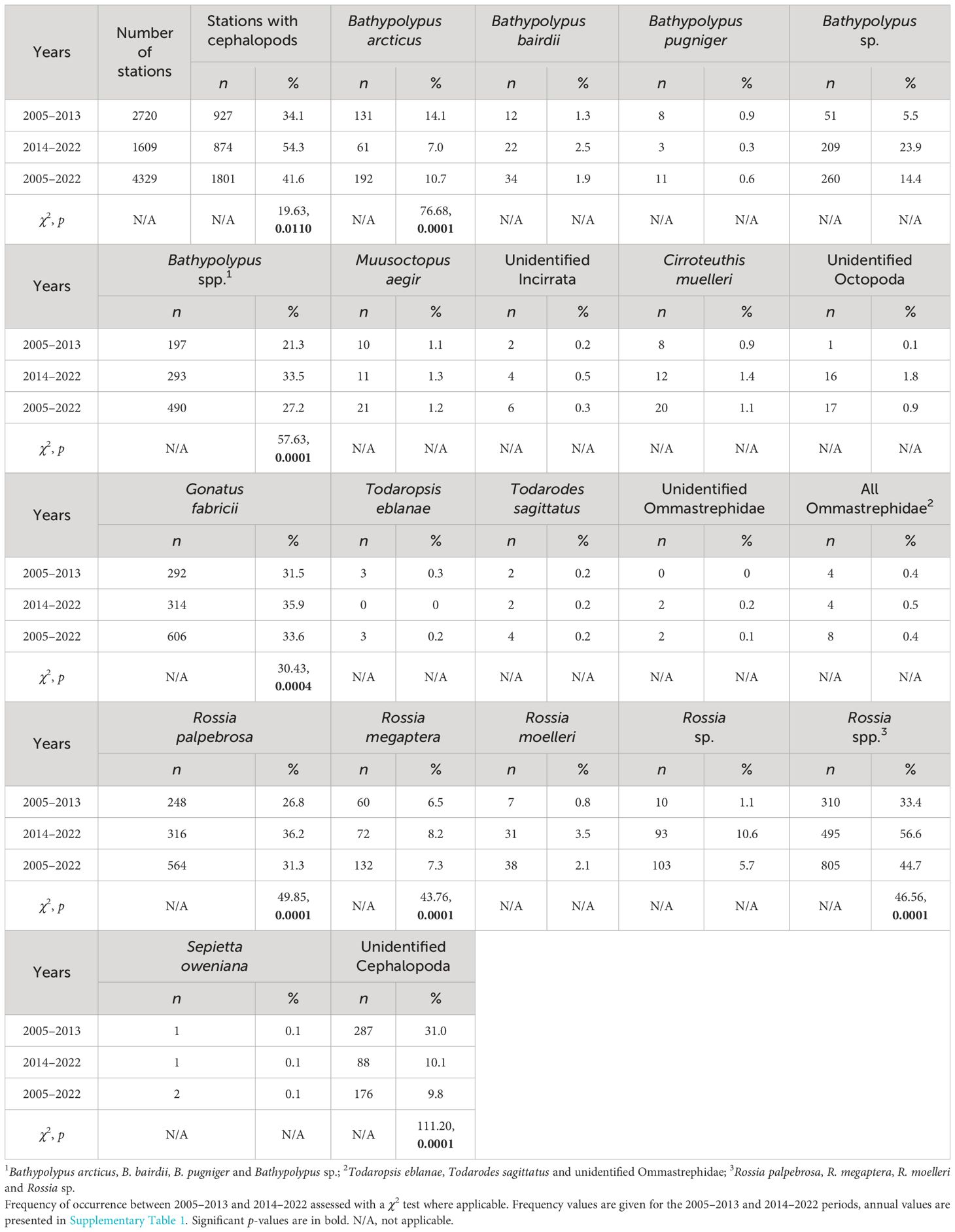
Table 1 Bottom trawl station number and frequency of cephalopods in their catches in 2005–2022 in the western Barents Sea.
All invertebrate benthos catch from the shrimp trawl surveys was identified onboard by benthic experts alongside fish experts (Zakharov et al., 2018; Jørgensen et al., 2019; Zakharov et al., 2020; Jørgensen et al., 2022). Cephalopods usually were treated as the other benthos bycatch taxa onboard (Zakharov et al., 2018; Jørgensen et al., 2019; Zakharov et al., 2020; Jørgensen et al., 2022). Since 2005, a varying number of cephalopods from the benthos catch was fixed or frozen and analysed ashore by Alexey V. Golikov (A.V.G.) and Rushan M. Sabirov (R.M.S.). Such re-identification of catches from the older surveys increased the number of species initially found. Moreover, in many cases old unfixed samples were photo-verified by A.V.G. and R.M.S. These data, when provided to onboard benthic experts, resulted in significant increase of onboard identification quality in the 2014–2022 period in comparison to the 2005–2013 period (see Results & Discussion). Outdated names such as Benthoctopus sp. and later Muusoctopus sp. were changed to Muusoctopus aegir after this species was formally described in 2023 (Golikov et al., 2023a). Rossia individuals that did not fit either R. palpebrosa or R. moelleri taxonomic descriptions were identified to Rossia megaptera after this species was found to present in the Barents Sea (Golikov et al., 2020).
In some years, a cephalopod expert was absent onboard during a particular cruise. It was never the case for all participating RVs simultaneously, if we account for ashore/photo-reidentification described above. During these years, Bathypolypus and Rossia were often not identified to the species level and labelled as “Bathypolypus sp.” and “Rossia sp.”, respectively. Also during these years, species were labelled as “Incirrata” if either Bathypolypus sp. or Muusoctopus aegir; “Octopoda” if either both of the latter or Cirroteuthis muelleri; “Ommastrephidae” if either Todaropsis eblanae, Todarodes sagittatus or unidentified Ommastrephidae; and “Cephalopoda” if nothing else was recorded/photographed to help the identification ashore. In this manuscript, the data were provided on group level as Bathypolypus spp. (= combined three species and Bathypolypus sp.), Rossia spp. (= combined three species and Rossia sp.) and Ommastrephidae (T. eblanae, To. sagittatus or unidentified Ommastrephidae), as well as on individual species level, to show both species’ patterns and patterns unbiased by identification quality.
2.2 Abundance and biomass
The ratio of trawl stations with cephalopod catch to standardized total catches was recorded annually and was used as a measure of cephalopod relative abundance. Frequency of occurrence of individual species/group of cephalopods in % of all stations with cephalopods was used as an abundance proxy of particular species/group. Statistical comparisons of frequencies between the 2005–2013 and 2014–2022 period were possible where we have enough data annually (= caught for more than four years within either one of the periods). These taxa were Bathypolypus arcticus, Bathypolypus spp., Gonatus fabricii, Rossia palpebrosa, R. megaptera, Rossia spp. and unidentified Cephalopoda. The latter was done to assess if the quality of onboard identification changed from the 2005–2013 to 2014–2022 period.
Biomass density, i.e. biomass in gram per nautical mile of towing (g/n.m.) was a proxy of absolute biomass. The quantitative measures were estimated using towing speed and distance by standard IMR/PINRO protocols (Jørgensen et al., 2019; Zakharov et al., 2020). We decided not to correct density values to absolute biomass, because the Campelen-1800 shrimp trawl’s catchability for cephalopods is unknown, as revealed by previous attempts to do so (Lubin and Sabirov, 2007; Golikov et al., 2017). Statistical comparisons of biomass density between the 2005–2013 and 2014–2022 period were possible where enough data was available: Bathypolypus arcticus, B. bairdii, Bathypolypus spp., Gonatus fabricii, Rossia palpebrosa, R. megaptera and Rossia spp. Deep-sea octopods (Muusoctopus aegir and Cirroteuthis muelleri) were not temporally compared, because deep-sea slopes were sampled infrequently and differently among years, unlike the shelf areas.
2.3 Data analyses
To compare the biomass of species/taxa (see above) between the two groups (i.e., the 2005–2013 and 2014–2022 period), a Mann–Whitney U-test was used; and to compare among three or more groups (i.e., within Bathypolypus spp., Rossia spp. and among other taxa) we used a Kruskal–Wallis H test with a post-hoc Dunn’s Z test (Zar, 2010). Frequencies of species/taxa were compared using a χ2 test (Zar, 2010). Statistical analysis, calculations, equations and plots were performed in PAST 4.12b (Hammer et al., 2001), Statistica 12.0 (Statsoft) and MS Excel 2010. The value of α = 0.05 was considered significant in this study.
3 Results
3.1 Cephalopod species and abundance
The Norwegian-Russian Ecosystem Survey recorded twelve species in the Barents Sea and adjacent areas during 2005–2022: Bathypolypus arcticus, B. bairdii, B. pugniger, Muusoctopus aegir (incirrate octopods; Octopoda Incirrata); Cirroteuthis muelleri (cirrate octopod; Octopoda Cirrata); Gonatus fabricii, Todaropsis eblanae, Todarodes sagittatus (squids; Oegopsida); and Rossia palpebrosa, R. megaptera, R. moelleri and Sepietta oweniana (bobtail squids; Sepiolida). Among those, T. eblanae, To. sagittatus and S. oweniana were boreal-subtropical migrants, and all others belonged to permanent Arctic residents. All records of the boreal-subtropical species occurred south of 75° N (Figure 1). All these species were recorded during both the 2005–2013 and 2014–2022 period, except for T. eblanae, which was absent during the 2014–2022 period (Table 1). The latter species, however, was found east of 40° E in the Barents Sea during this period (Golikov et al., in prep.). So, the fauna composition over the studied area seemed identical between the 2005–2013 and 2014–2022 period.
The trawl catch of cephalopods increased significantly over time (Figure 2, Table 1; Supplementary Table 1). Moreover, significant increase in frequency was recorded in every taxon it was checked for (i.e., Bathypolypus spp., G. fabricii, R. palpebrosa, R. megaptera, Rossia spp. and unidentified Cephalopoda), except for B. arcticus (Table 1). In the latter species, significant decrease in frequency was recorded (Table 1). Among the taxa where the significance of temporal frequency changes could not be checked, it was increase in B. bairdii, Bathypolypus sp., deep-sea and unidentified octopods, boreal-subtropical Ommastrephidae squids, R. moelleri and Rossia sp. and decrease in B. pugniger (Figure 2, Table 1; Supplementary Table 1). Boreal-subtropical S. oweniana was infrequently caught and no differences in frequency were observed over time. Aggregations of Bathypolypus sp. and B. bairdii were recorded in the south-western Barents Sea (Figure 1). Both deep-sea incirrate and cirrate octopods, M. aegir and C. muelleri, were much less frequent than Bathypolypus spp. (Figure 2, Table 1). Both these two deep-sea octopods were found on the slope and in the troughs, but not on the shelf (Figure 1). Unidentified Incirrata and Octopoda were also largely found over the deep-sea areas, but closer to the shelf than ‘identified’ deep-sea octopods (Figure 1).
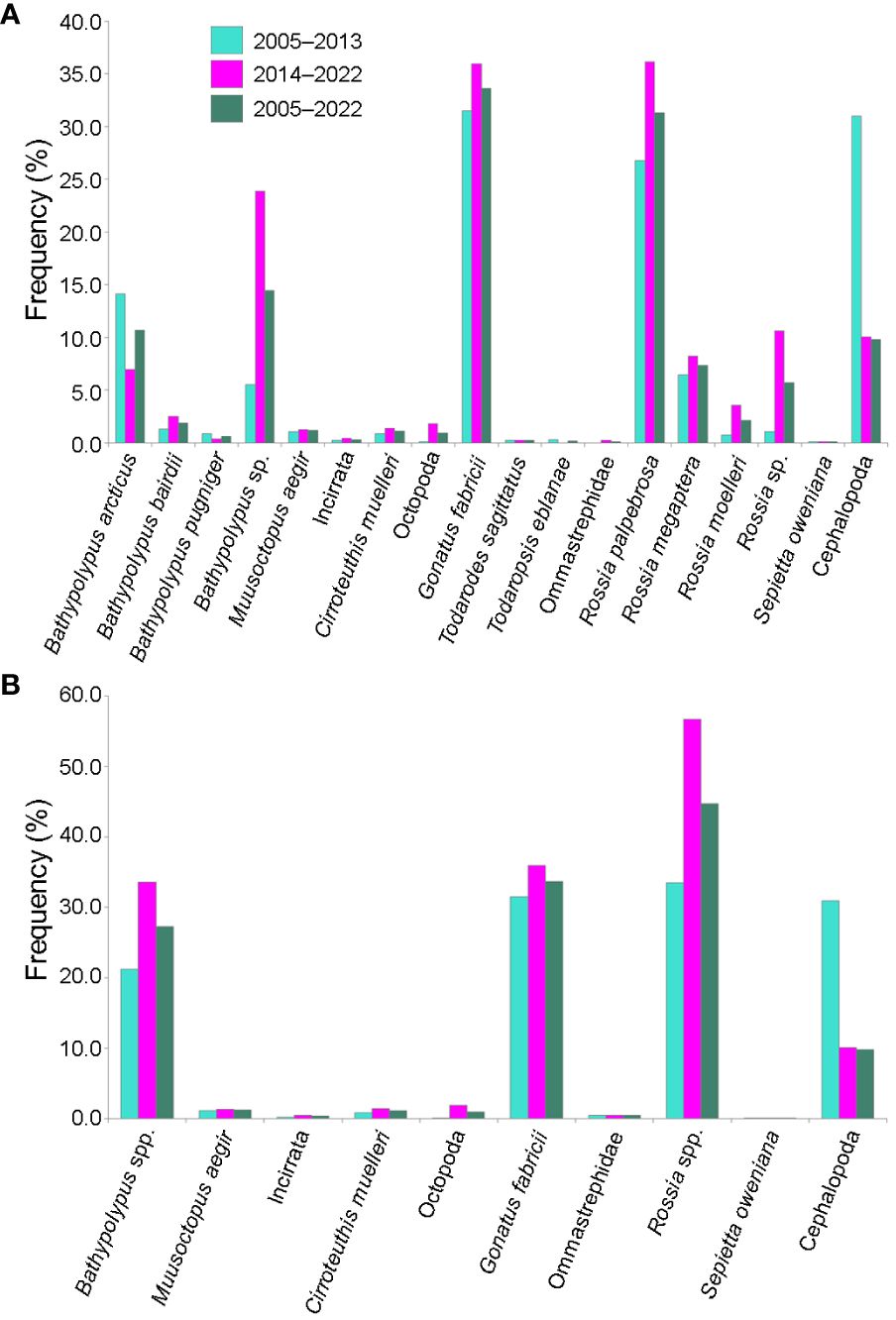
Figure 2 Frequency of occurrence of different species/taxa of cephalopods in % of all bottom trawl stations with cephalopod catch in 2005–2022 in the western Barents Sea. (A) All species/taxa. (B) Bahtypolypus taxa combined as Bathypolypus spp., Rossia taxa combined as Rossia spp. and Ommastrephidae taxa combined as Ommastrephidae.
Overall, Bathypolypus spp., G. fabricii and Rossia spp. were much more frequent than the deep-sea (M. aegir and C. muelleri) and the boreal-subtropical (Ommastrephidae and S. oweniana) species, and distributed all over the study area (Figures 1, 2, Table 1). Among the three most abundant cephalopod taxa, Rossia spp. was the most frequently recorded during both the 2005–2013 and 2014–2022 period, while Bathypolypus spp. was the least frequently recorded (Table 2). Within the Bathypolypus spp. and Rossia spp., widespread boreal-arctic species B. arcticus and R. palpebrosa were the most ubiquitous (Figures 1, 2, Table 1). While the high-boreal R. megaptera and the arctic R. moelleri preferred warmer and colder areas, respectively, both of the two high-boreal species B. bairdii and B. pugniger preferred warmer areas (Figure 1, Table 1).
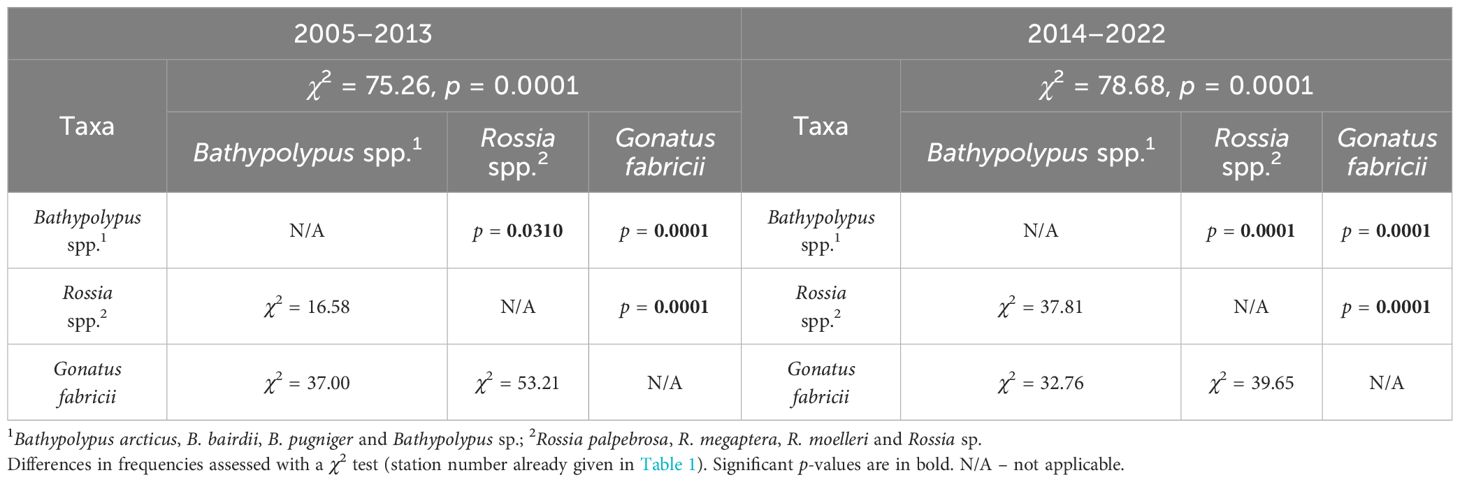
Table 2 Cross-taxa comparison of frequencies among the most abundant cephalopods in 2005–2013 and 2014–2022 in the western Barents Sea.
3.2 Biomass
While frequencies of occurrence showed significant differences over time, the biomass only showed significant decrease from the 2005–2013 and 2014–2022 period for Bathypolypus spp. (Table 3). A non-significant decrease was recorded for B. arcticus, B. bairdii and Gonatus fabricii, while a non-significant increase was found in Rossia palpebrosa, R. megaptera and Rossia spp. (Table 3). Among the three most abundant cephalopod taxa, the biomass density was the highest for Bathypolypus spp., medium for Rossia spp. and the lowest for G. fabricii (Tables 3, 4). Within Bathypolypus spp., no significant differences were found among the species (Tables 3, 4). Within Rossia spp., high-boreal R. megaptera had significantly lower biomass density than the two other species (Tables 3, 4).
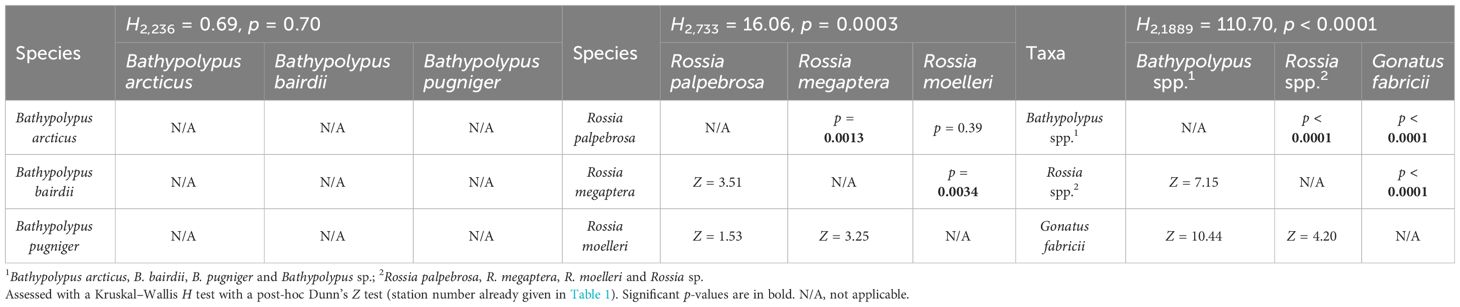
Table 4 Cross-species/taxa comparison of biomass density of cephalopods in 2005–2022 in the western Barents Sea.
4 Discussion
4.1 Summary of the main findings from the 18 years of annual trawl data
The main changes in cephalopod fauna occurred during the mid-2000s and early 2010s, when four boreal-subtropical species appeared in the area (three of them for the first time ever, and one for the first time since the early 1980s). The timing of their occurrence (i.e., the mid-2000s and early 2010s) followed the onset of increased climate-driven warming in the Barents Sea since late 1990s/early 2000s (Swart et al., 2015; Rantanen et al., 2022). The Atlantification of the cephalopod community in the Barents Sea was evidenced by increased abundance of boreal-subtropical Ommastrephidae squids, and repeated occurrence of Ommastrephidae squids and Sepietta oweniana during two periods (2005–2013 and 2014–2022). ‘Typical’ Arctic cephalopod species (Bathypolypus spp., Gonatus fabricii and Rossia spp.) are still much more abundant in the western Barents Sea compared to the deep-sea and the boreal-subtropical cephalopod species. Moreover, the abundance of these Arctic taxa increased from the 2005–2013 to 2014–2022 period, as did the abundance of other cephalopods across the studied area. The increased abundance but reduced biomass of Bathypolypus spp. from the 2005–2013to 2014–2022 indirectly suggests a body-size reduction. This would be the first evidence of size reduction in response to ocean warming in octopods, a phenomena known for other taxa elsewhere (e.g., Gardner et al., 2011; Sheridan and Bickford, 2011; Ikpewe et al., 2021). Lastly, a significant increase of onboard identification quality followed when onboard benthic experts were educated by cephalopod taxonomic experts. Such taxonomic training increases the value of the ecosystem surveys for the future monitoring.
4.2 Temporal dynamics of cephalopod community composition
Our study is the first to document temporal trends in the cephalopod community of the Barents Sea, which is one of the fastest warming regions in the Arctic (Overland et al., 2014; Lind et al., 2018; Gerland et al., 2023). Before 2005 (= start of the time series used here), cephalopod biodiversity of the Barents Sea was lower than it currently is (Nesis, 1987; Golikov et al., 2013; Xavier et al., 2018) (Table 5). Todarodes sagittatus has been the only boreal-subtropical cephalopod known from the Barents Sea before 2005 (Nesis, 1987; Golikov et al., 2013; Xavier et al., 2018) (Table 5). It was last recorded in the Barents Sea in 1983 (Nesis, 1987), while in our studies the first records of this species start from 2010 (Golikov et al., 2013, 2014; Xavier et al., 2018). Other boreal-subtropical cephalopods that were found in the Barents Sea in 2005–2013 are Todaropsis eblanae, Teuthowenia megalops and Sepietta oweniana (Golikov et al., 2013, 2014; Xavier et al., 2018) (Table 5). The deep-sea squid Te. megalops was only found once in 2009 (Golikov et al., 2013). This rare record was not from the Norwegian-Russian Ecosystem Survey area, but from the north-eastern slope of the Greenland Sea (Figure 1), and taken by the same Campelen-1800 trawl as used in the Norwegian-Russian Ecosystem Survey (Golikov et al., 2013). In 2014–2022, all boreal-subtropical cephalopods were recorded in the Barents Sea again, except for Te. megalops (Table 5). A gradual influx of Atlantic fauna in the Barents Sea is also well known for other invertebrates and fishes, providing a basis for ongoing Atlantification of the local ecosystems (Jørgensen et al., 2016; Frainer et al., 2017; Brandt et al., 2023). The records from our study show that the cephalopod community of the Barents Sea is subjected to the Atlantification since the 2005–2013 period. Mean annual temperatures in the Arctic, both modelled and observed, continuously increase since the late 1990s/early 2000s (Swart et al., 2015; Rantanen et al., 2022). This warming, which results from climate change, is often hypothesized as a main cause of the Atlantification (e.g., Jørgensen et al., 2016; Frainer et al., 2017; Brandt et al., 2023).
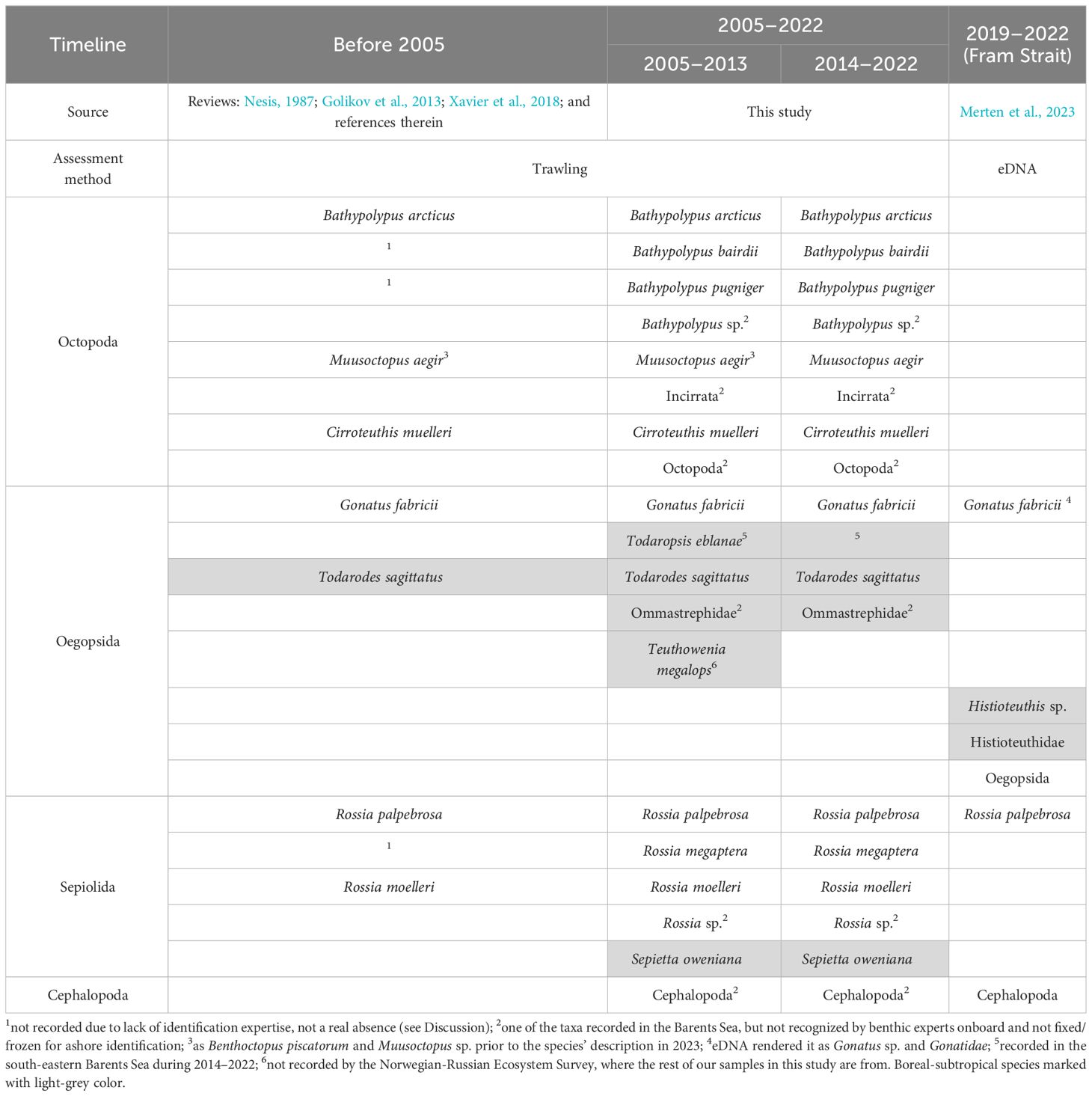
Table 5 Cephalopod fauna composition from the XIXth century to 2022 in the western Barents Sea and adjacent areas.
Of the twelve permanent Arctic resident cephalopod species (Xavier et al., 2018; Golikov et al., 2020; Golikov et al., 2023a), only Muusoctopus sibiricus, M. leioderma and Muusoctopus sp. were absent in the western Barents Sea and adjacent areas. These octopods live in the Siberian Seas, Beaufort and Chukchi Sea (M. sibiricus and M. leioderma), and in the northern Baffin Bay and Canadian Arctic Archipelago (Muusoctopus sp.) (Nesis, 1987; Xavier et al., 2018; Golikov et al., 2023a). The absence of published records of Rossia megaptera, Bathypolypus bairdii and B. pugniger before 2005 (Table 5) could rather be caused by the lack of taxonomic studies and identification expertise. Bathypolypus bairdii and B. pugniger were only taxonomically separated from B. arcticus in 2002 (Muus, 2002). And R. megaptera is often considered as living only in North-West Atlantic (Mercer, 1968; Reid and Jereb, 2005), despite its presence was later confirmed from Iceland (Golikov et al., 2018b) and the Barents Sea (Golikov et al., 2020). When reanalyzing samples from before 2005, both B. pugniger and R. megaptera have been found in the area, in 1967 and in 2003 respectively (Golikov et al., in prep.).
Alternative methods to assess biodiversity and community composition in marine ecosystems are underwater video imagery and environmental DNA (eDNA) analyses (e.g., Merten et al., 2021; Kopp et al., 2023). Environmental DNA analysis enables detection of species based on genetic material from marine animals that is released in the environment, such as shed skin cells, mucus, gametes and faeces (Taberlet et al., 2012a, Taberlet et al., 2012b). The advantages of eDNA analysis are that it gives a larger presence/absence time frame than trawl surveys (eDNA remains suspended in the water column for up to 60 days), and it allows simultaneous identification of different taxa within the same water sample (reviews: Thomsen and Willerslev, 2015; Rourke et al., 2022). Advances and successes have been made with the application of eDNA in fisheries surveys (Thomsen and Willerslev, 2015; Rourke et al., 2022) and also in cephalopod biodiversity studies (e.g., Merten et al., 2021; Visser et al., 2021; Merten et al., 2023). While the technique is being developed to be used for abundance estimates (Thomsen and Willerslev, 2015; Rourke et al., 2022), it is currently mostly used to obtain presence-absence for species or communities. Results of eDNA metabarcoding depend on the used primers, which may be biased towards certain taxonomic groups, and sequence availability in databases. For example, the 18S rRNA primer that is used in cephalopod eDNA studies does not detect all octopods (De Jonge et al., 2021). While, arctic and boreal-subtropical cephalopods recorded in the Barents Sea and adjacent areas are better represented in GenBank with COI, than with 18S. When applying, it is 18S which is better able to detect cephalopods during eDNA metabarcoding on water samples (detailed in De Jonge et al., 2021). The collection of underwater imagery enables analyses of biodiversity and community composition, color, behavior, habitat association, and estimation of abundance and biomass density in case of standardized surveys (e.g., Robison et al., 1998; Robison, 2004; Buhl-Mortensen et al., 2015; Stratmann et al., 2022). Underwater imagery analyses has been applied in biodiversity assessments of cephalopod communities (e.g., Merten et al., 2021; Pratt et al., 2021, Pratt et al., 2023). For example, G. fabricii was recorded in the central Arctic Ocean under ice by a mooring camera system (Snoeijs-Leijonmalm et al., 2022), and Arctic cirrate octopods were documented to perform benthopelagic migrations (Golikov et al., 2023b). While no studies have attempted to quantify squid biomass from underwater imaging surveys, this approach may be challenging since some squids may alter their behavior in response to lights (avoidance or attraction behavior) (e.g., Hoving et al., 2019; Snoeijs-Leijonmalm et al., 2022). On the other hand, presumably natural cephalopod behavior has been documented repeatedly via in situ observations of remotely operated vehicles (e.g., Hoving and Robison, 2012; Hoving et al., 2013b; Hoving and Haddock, 2017). Benthic cephalopods are often hard to identify to species level from images (e.g., Pratt et al., 2021; Robinson et al., 2021; Snoeijs-Leijonmalm et al., 2022; and many others). To summarise, currently trawling, eDNA and video imagery each have their strengths and weaknesses, and the best results are rendered by combining them (e.g., Thomsen et al., 2016; Merten et al., 2021; Kopp et al., 2023).
4.3 Temporal dynamics of cephalopod abundance and biomass
A clear increase in the ratio of cephalopod catches to standardized total catches, as well as an increase in the frequency of occurrence was recorded for most of the studied cephalopod species/taxa in the western Barents Sea from the 2005–2013 to 2014–2022 period. These observations align with ongoing continuous increase of mean annual temperatures in the Arctic during the same timeline (Swart et al., 2015; Rantanen et al., 2022). While the expansion of cephalopods’ ranges and habitats was previously indirectly implying to their increased abundance and biomass (Golikov et al., 2012, Golikov et al., 2013; Xavier et al., 2018; Golikov et al., 2019b; Oesterwind et al., 2022), our study is the first direct evidence that indeed the abundance of cephalopods in the Arctic is increasing. Our results are in line with a global trend that shows that cephalopods’ biomass is increasing in tropical and temperate areas (Doubleday et al., 2016). Our results are also in line with the trends observed for other Arctic nekton, such as increasing abundance of boreal pelagic fishes (Frainer et al., 2017; Brandt et al., 2023).
The biomass density used here can be a proxy of absolute biomass when coupled with abundance data and distribution maps. This makes comparisons of biomass among the studies possible (even though limited by the use of different gear). The biomass of deep-sea octopods is the largest in the troughs and on the slopes of the marginal areas of the Barents Sea. Cirroteuthis muelleri specifically reaches the highest biomass density in the studied area, even though it is still about two times lower compared to this species’ hotspots in the Baffin Bay (Golikov et al., 2022). At the same time widespread Arctic taxa (Bathypolypus spp., Gonatus fabricii and Rossia spp.) have much higher abundance and ubiquitous distribution in the studied area, while their biomass is lower than of deep-sea octopods. The biomass of Bathypolypus spp. and Rossia spp. in the Baffin Bay seems comparable to our values (Frandsen and Wieland, 2004; Treble, 2007), but the trawls are different and it may flaw a direct comparison. In the Porcupine Seabight, abundance data of Bathypolypus spp. and Rossia spp. suggest similar or slightly lower biomass than in the Barents Sea, but the used trawls are also different from those used in the Barents Sea (Collins et al., 2001). When correctly estimated, absolute biomass may be a good parameter to compare among areas. Because we do not know the trawl catchability of cephalopods, absolute biomass is currently rarely used. Previous conservative estimates of absolute biomass include 6.5 thousand tonnes of R. papebrosa and 24.8 thousand tonnes of G. fabricii in the Norwegian-Russian Ecosystem Survey area in 2007 and 2011, respectively by Golikov et al. (2017). Our current study exceeds these numbers in 8–10 times.
The standardized survey (gear, time and place) demonstrating the congruent significant decrease of biomass and significant increase in individual numbers (see Results and above) indirectly suggests a reduction in body-size of Bathypolypus spp. in the western Barents Sea. There are various examples of reduction in size in aquatic invertebrates, fishes, and some seabirds in response to climate change (e.g., Gardner et al., 2011; Sheridan and Bickford, 2011; Ikpewe et al., 2021). Among cephalopods, size reduction in response to climate change has only been recorded for squids (Jackson and Domeier, 2003; Hoving et al., 2013a; Arkhipkin et al., 2015; Takahara et al., 2017).
To date, cephalopod monitoring in the Barents Sea has been performed via analysis of benthos bycatch from the Norwegian-Russian Ecosystem Survey. This survey uses the Campelen-1800 bottom trawl which is designed for demersal shrimp and fish surveys (McCallum and Walsh, 1994). It is not typically used to assess pelagic squids, as was done in this study. Still, even with a gear that is suboptimal for nekton, G. fabricii is the second most abundant cephalopod in the study area. This suggests that biomass of G. fabricii and other squids in the Barents Sea may be even higher if sampled with a pelagic trawl (Golikov et al., 2012, Golikov et al., 2017; Golikov et al., 2019a).
4.4 Challenges to cephalopod identification
Cephalopod identification can be challenging for non-specialists, also because of wrong or insufficient species names in GenBank (e.g., Fernández-Álvarez et al., 2021; Katugin and Zolotova, 2023). Specifically, in Bathypolypus spp. only mature males can be reliably identified by non-specialists. Genetic barcodes from reliably identified B. bairdii and B. pugniger were only recently uploaded to GenBank (Taite et al., 2023). Muusoctopus aegir was first described in 2023 (Golikov et al., 2023a), and is in GenBank as ‘Muusoctopus sp.’ (Taite et al., 2023). Sequences of specimens that were morphologically identified as Gonatus fabricii and G. steenstrupi and originated from several studies in the North Atlantic cluster as a single species in GenBank (Lindgren et al., 2005; Lindgren, 2010; Vecchione et al., 2010; Taite et al., 2020). These Gonatus sequences were referred to as being different from ‘real’ G. fabricii from the Arctic (Taite et al., 2020; Katugin and Zolotova, 2023), which was cited as ‘Lindgren, unpublished’. To date, only G. fabricii has been recorded in the Barents Sea (Golikov et al., 2012; Xavier et al., 2018; Golikov et al., 2019a). The most northern distribution is still unknown for G. steenstrupi, and is supposed to be in the low Arctic areas, such as north off Iceland (Xavier et al., 2018; Golikov et al., 2018a). Rossia palpebrosa also has several misidentifications in GenBank (they do not even cluster as one species; A.V.G., pers. obs.), and there are no uploaded sequences for R. moelleri and R. megaptera.
In this study there was a significant increase in quality of onboard identification expertise by benthic experts over time. This is demonstrated by a three times-decrease of unidentified cephalopods from the trawl catches from the 2005–2013 period to the 2014–2022 period, and by changed ratios of taxa within Bathypolypus spp. Onboard experts recognized B. bairdii and B. pugniger more often. Still, 10% of cephalopod catches were unidentified in the 2014–2022 period, which imply necessary improvements of onboard identification.
Data availability statement
The data analysed in this study is subject to the following licenses/restrictions: The dataset is still in work under our team’s other projects; also the data from Russian part of the Norwegian-Russian Ecosystem Survey are in not in public access yet. Once they are, the whole dataset will be made available as soon as possible. Requests to access these datasets should be directed to golikov.ksu@gmail.com.
Ethics statement
Ethical approval was not required for the study involving animals in accordance with the local legislation and institutional requirements because samples were obtained as a bycatch from cruises; when they come as a bycatch, they are already dead when onboard. No animals were killed specifically for this project. The specimens are not vertebrates, neither are they non-human primates, genetically modified organisms, cloned farm animals or endangered species.
Author contributions
AG: Conceptualization, Formal analysis, Funding acquisition, Investigation, Resources, Visualization, Writing – original draft, Writing – review & editing. LJ: Formal analysis, Funding acquisition, Resources, Visualization, Writing – original draft, Writing – review & editing. RS: Conceptualization, Formal analysis, Investigation, Writing – review & editing. DZ: Formal analysis, Resources, Writing – review & editing. HJH: Conceptualization, Formal analysis, Funding acquisition, Resources, Writing – review & editing.
Funding
The author(s) declare financial support was received for the research, authorship, and/or publication of this article. This project has received funding from the European Union’s Horizon 2020 research and innovation program under Marie Skłodowska-Curie grant agreement № 101065960 (granted to AG); from Norwegian-Russian Ecosystem Survey (AG, LJ = PI on benthos and DZ); and HJH was supported by Helmholtz POF IV.
Acknowledgments
We are grateful to the scientific groups and crews of all RVs participating in the Norwegian-Russian Ecosystem Survey over these years, and especially to the benthic experts onboard (Pavel A. Lubin, Olga L. Zimina, Igor E. Manushin, Natalya A. Strelkova, Anne K. Sveistrup, Heidi Gabrielsen and many others); and to Julian B. Stauffer for his support with map design. We thank the editor, Dr. Paco Bustamante, two reviewers and Dr. Marek Lipinski whose comments helped us to improve the manuscript.
Conflict of interest
The authors declare that the research was conducted in the absence of any commercial or financial relationships that could be construed as a potential conflict of interest.
Publisher’s note
All claims expressed in this article are solely those of the authors and do not necessarily represent those of their affiliated organizations, or those of the publisher, the editors and the reviewers. Any product that may be evaluated in this article, or claim that may be made by its manufacturer, is not guaranteed or endorsed by the publisher.
Supplementary material
The Supplementary Material for this article can be found online at: https://www.frontiersin.org/articles/10.3389/fmars.2024.1392585/full#supplementary-material
References
Arkhipkin A., Argüelles J., Shcherbich Z., Yamashiro C. (2015). Ambient temperature influence adult size and life span in jumbo squid Dosidicus gigas. Can. J. Fish. Aquat. Sci. 72, 400–409. doi: 10.1139/cjfas-2014–0386
Bjørke H., Gjøsaeter H. (1998). Who eats the larger Gonatus fabricii (Lichtenstein) in the Norwegian Sea? ICES. CM. Pap. Rep. M10, 1–11.
Brandt S., Wassmann P., Piepenburg D. (2023). Revisiting the footprints of climate change in Arctic marine food webs: an assessment of knowledge gained since 2010. Front. Mar. Sci. 10. doi: 10.3389/fmars.2023.1096222
Buhl-Mortensen L., Buhl-Mortensen P., Dolan M. J. F., Gonzalez-Mirelis G. (2015). Habitat mapping as a tool for conservation and sustainable use of marine resources: some perspectives from the MAREANO Programme, Norway. J. Sea. Res. 100, 46–61. doi: 10.1016/j.seares.2014.10.014
CAFF (2017). State of the Arctic Marine Biodiversity Report (Akureyri: Conservation of Arctic Flora and Fauna International Secretariat).
Collins M. A., Yau C., Allcock L., Thurston M. H. (2001). Distribution of deep-water benthic and bentho-pelagic cephalopods from the north-east Atlantic. J. Mar. Biol. Assoc. U.K. 81, 105–117. doi: 10.1017/S0025315401003459
De Jonge D. S. W., Merten V., Bayer T., Puebla O., Reusch T. B. H., Hoving H.-J. T. (2021). A novel metabarcoding primer pair for environmental DNA analysis of Cephalopoda (Mollusca) targeting the nuclear 18S rRNA region. R. Soc Open Sci. 8, 201388. doi: 10.1098/rsos.201388
Doubleday Z. A., Prowse T. A. A., Arkhipkin A., Pierce G. J., Semmens J., Steer M., et al. (2016). Global proliferation of cephalopods. Curr. Biol. 26, R406–R407. doi: 10.1016/j.cub.2016.04.002
Eriksen E., Gjøsæter H., Prozorkevich D., Shamray E., Dolgov A., Skern-Mauritzen M., et al. (2018). From single species surveys towards monitoring of the Barents Sea ecosystem. Prog. Oceanogr. 166, 4–14. doi: 10.1016/j.pocean.2017.09.007
Fernández-Álvarez F.Á., Sánchez P., Villanueva R. (2021). Morphological and molecular assessments of bobtail squids (Cephalopoda: Sepiolidae) reveal a hidden history of biodiversity. Front. Mar. Sci. 7. doi: 10.3389/fmars.2020.632261
Fossheim M., Primicerio R., Johannesen E., Ingvaldsen R. B., Aschan M. M., Dolgov A. V. (2015). Recent warming leads to a rapid borealization of fish communities in the Arctic. Nat. Clim. Change 5, 673–677. doi: 10.1038/nclimate2647
Frainer A., Primicerio R., Kortsch S., Aune M., Dolgov A. V., Fossheim M., et al. (2017). Climate-driven changes in functional biogeography of Arctic marine fish communities. Proc. Natl. Acad. Sci. U.S.A. 114, 12202–12207. doi: 10.1073/pnas.1706080114
Frandsen R. P., Wieland K. (2004). Cephalopods in Greenland waters (Nuuk: Pinngortitaleriffik, Greenland Institute of Natural Resources).
Gardiner K., Dick T. A. (2010). Arctic cephalopod distributions and their associated predators. Polar. Res. 29, 209–227. doi: 10.1111/j.1751–8369.2010.00146.x
Gardner J. L., Peters A., Kearney M. R., Joseph L., Heinsohn R. (2011). Declining body size: a third universal response to warming? Trends Ecol. Evol. 26, 285–291. doi: 10.1016/j.tree.2011.03.005
Gerland S., Ingvaldsen R. B., Reigstad M., Sundfjord A., Bogstad B., Chierici M., et al. (2023). Still arctic? – the changing barents sea. Elem. Sci. Anth. 11, 88. doi: 10.1525/elementa.2022.00088
Golikov A. V., Artemev G. M., Blicher M. E., Gudmundsson G., Jørgensen L. L., Olafsdottir S. H., et al. (2022). Deep and cold: are Boreal and Arctic finned octopods, Stauroteuthis syrtensis and Cirroteuthis muelleri (Cephalopoda, Octopoda, Cirrata), ecological analogues? Deep-Sea Res. I. 181, 103706. doi: 10.1016/j.dsr.2022.103706
Golikov A. V., Blicher M. E., Jørgensen L. L., Walkusz W., Zakharov D. V., Zimina O. L., et al. (2019a). Reproductive biology and ecology of the boreoatlantic armhook squid Gonatus fabricii (Cephalopoda: Gonatidae). J. Moll. Stud. 85, 287–299. doi: 10.1093/mollus/eyz023
Golikov A. V., Ceia F. R., Sabirov R. M., Ablett J. D., Gleadall I. G., Gudmundsson G., et al. (2019b). The first global deep-sea stable isotope assessment reveals the unique trophic ecology of Vampire Squid Vampyroteuthis infernalis (Cephalopoda). Sci. Rep. 9, 19099. doi: 10.1038/s41598–019-55719–1
Golikov A. V., Ceia F. R., Sabirov R. M., Batalin G. A., Blicher M. E., Gareev B. I., et al. (2020). Diet and life history reduce interspecific and intraspecific competition among three sympatric Arctic cephalopods. Sci. Rep. 10, 21506. doi: 10.1038/s41598–020-78645-z
Golikov A. V., Gudmundsson G., Blicher M. E., Jørgensen L. L., Korneeva E. I., Olafsdottir S. H., et al. (2023a). A review of the genus Muusoctopus (Cephalopoda: Octopoda) from Arctic waters. Zool. Lett. 9, 21. doi: 10.1186/s40851–023-00220-x
Golikov A. V., Sabirov R. M., Gudmundsson G. (2018a) Cephalopoda (Smokkdýr), Gonatus steenstrupi Kristensen 1981. Available online at: http://www.ni.is/biota/animalia/mollusca/cephalopoda/gonatus-steenstrupi (Accessed February 3, 2022).
Golikov A. V., Sabirov R. M., Gudmundsson G. (2018b) Cephalopoda (Smokkdýr), Rossia megaptera Verrill 1881. Available online at: http://www.ni.is/biota/animalia/mollusca/cephalopoda/rossia-megaptera (Accessed February 3, 2022).
Golikov A. V., Sabirov R. M., Lubin P. A. (2012). New data on Gonatus fabricii (Cephalopoda, Teuthida) distribution and reproductive biology in the Western Sector of Russian Arctic. Proc. Kazan. Uni. Nat. Sci. Ser. 154, 118–128.
Golikov A. V., Sabirov R. M., Lubin P. A. (2017). First assessment of biomass and abundance of cephalopods Rossia palpebrosa and Gonatus fabricii in the Barents Sea. J. Mar. Biol. Assoc. U.K. 97, 1605–1616. doi: 10.1017/S0025315416001004
Golikov A. V., Sabirov R. M., Lubin P. A., Jørgensen L. L. (2013). Changes in distribution and range structure of Arctic cephalopods due to climatic changes of the last decades. Biodiversity 14, 28–35. doi: 10.1080/14888386.2012.702301
Golikov A. V., Sabirov R. M., Lubin P. A., Jørgensen L. L., Beck I.-M. (2014). The northernmost record of Sepietta oweniana (Cephalopoda: Sepiolidae) and comments on boreo-subtropical cephalopod species occurrence in the Arctic. Mar. Biodivers. Rec. 7, e58. doi: 10.1017/S1755267214000645
Golikov A. V., Stauffer J. B., Schindler S. V., Taylor J., Boehringer L., Purser A., et al. (2023b). Miles down for lunch: deep-sea in situ observations of Arctic finned octopods Cirroteuthis muelleri suggest pelagic–benthic feeding migration. Proc. R. Soc B. 290, 20230640. doi: 10.1098/rspb.2023.0640
Hammer Ø., Harper D. A. T., Ryan P. D. (2001). PAST: paleontological statistics software package for education and data analysis. Paleontol. Electron. 4, 1–9.
Hoving H. J. T., Christiansen S., Fabrizius E., Hauss H., Kiko R., Linke P., et al. (2019). The Pelagic In situ Observation System (PELAGIOS) to reveal biodiversity, behavior, and ecology of elusive oceanic fauna. Ocean. Sci. 15, 1327–1340. doi: 10.5194/os-15–1327-2019
Hoving H. J. T., Gilly W. F., Markaida U., Benoit-Bird K. J., Brown Z. W., Daniel P., et al. (2013a). Extreme plasticity in life-history strategy allows a migratory predator (jumbo squid) to cope with a changing climate. Glob. Change Biol. 19, 2089–2103. doi: 10.1111/gcb.12198
Hoving H. J. T., Haddock S. H. D. (2017). The giant deep-sea octopus Haliphron atlanticus forages on gelatinous fauna. Sci. Rep. 7, 44952. doi: 10.1038/srep44952
Hoving H. J. T., Robison B. H. (2012). Vampire squid: detritivores in the oxygen minimum zone. Proc. R. Soc B. 279, 4559–4567. doi: 10.1098/rspb.2012.1357
Hoving H. J. T., Zeidberg L. D., Benfield M. C., Bush S. L., Robison B. H., Vecchione M. (2013b). First in situ observations of the deep-sea squid Grimalditeuthis bonplandi reveal unique use of tentacles. Proc. R. Soc B. 280, 20131463. doi: 10.1098/rspb.2013.1463
Ikpewe I. E., Baudron A. R., Ponchon A., Fernandes P. G. (2021). Bigger juveniles and smaller adults: changes in fish size correlate with warming seas. J. Appl. Ecol. 58, 847–856. doi: 10.1111/1365–2664.13807
Jackson G. D., Domeier M. L. (2003). The effects of an extraordinary El Niño / La Niña event on the size and growth of the squid Loligo opalescens off Southern California. Mar. Biol. 142, 925–935. doi: 10.1007/s00227-002-1005-4
Jakobsson M., Mayer L. A., Bringensparr C., Castro C. F., Mohammad R., Johnson P., et al. (2020). The international bathymetric chart of the arctic ocean version 4.0. Sci. Data 7, 176. doi: 10.1038/s41597–020-0520–9
Jørgensen L. L., Archambault P., Armstrong C., Dolgov A., Edinger E., Gaston T., et al. (2016). “Arctic marine biodiversity. Chapter 36G,” in First Global Integrated Marine Assessment, World Ocean Assessment I. Eds. Inniss L., Simcock A. (United Nations, New York), 1–47.
Jørgensen L. L., Bakke G., Hoel A. H. (2020). Responding to global warming: new fisheries management measures in the Arctic. Prog. Oceanogr. 188, 102423. doi: 10.1016/j.pocean.2020.102423
Jørgensen L. L., Logerwell E. A., Strelkova N., Zakharov D., Roy V., Nozères C., et al. (2022). International megabenthic long-term monitoring of a changing arctic ecosystem: baseline results. Prog. Oceanogr. 200, 102712. doi: 10.1016/j.pocean.2021.102712
Jørgensen L., Primicerio R., Ingvaldsen R., Fossheim M., Strelkova N., Thangstad T., et al. (2019). Impact of multiple stressors on sea bed fauna in a warming Arctic. Mar. Ecol. Prog. Ser. 608, 1–12. doi: 10.3354/meps12803
Katugin O. N., Zolotova A. O. (2023). Species identification and genetic relationships in the squid family Gonatidae (Teuthida, Cephalopoda) based on partial sequencing of mitochondrial and nuclear genes. J. Mar. Biol. Assoc. U.K. 103, e88. doi: 10.1017/S0025315423000759
Kopp D., Faillettaz R., Le Joncour A., Simon J., Morandeau F., Le Bourdonnec P., et al. (2023). Assessing without harvesting: pros and cons of environmental DNA sampling and image analysis for marine biodiversity evaluation. Mar. Environ. Res. 188, 106004. doi: 10.1016/j.marenvres.2023.106004
Lind S., Ingvaldsen R. B., Furevik T. (2018). Arctic warming hotspot in the northern Barents Sea linked to declining sea-ice import. Nat. Clim. Change 8, 634–639. doi: 10.1038/s41558–018-0205-y
Lindgren A. R. (2010). Molecular inference of phylogenetic relationships among Decapodiformes (Mollusca: Cephalopoda) with special focus on the squid Order Oegopsida. Mol. Phylogenet. Evol. 56, 77–90. doi: 10.1016/j.ympev.2010.03.025
Lindgren A. R., Katugin O. N., Amezquita E., Nishiguchi M. K. (2005). Evolutionary relationships among squids of the family Gonatidae (Mollusca: Cephalopoda) inferred from three mitochondrial loci. Mol. Phylogenet. Evol. 36, 101–111. doi: 10.1016/j.ympev.2004.12.009
Lubin P. A., Sabirov R. M. (2007). “Fauna of cephalopods (Mollusca, Cephalopoda) of Spitsbergen Archipelago,” in Proceedings of VII International Scientific Conference ‘Complex Studies of Spitsbergen Archipelago. Ed. Matishov G. G. (Kola Scientific Centre of Russian Academy of Sciences, Apatity), 300–306.
McCallum B. R., Walsh S. J. (1994). Campelen 1800. Survey trawl reference manual (Newfoundland: Department of Fisheries and Oceans).
Mercer M. C. (1968). Systematics of the Sepiolid squid Rossia Owen 1835 in Canadian waters with a preliminary review of the genus and notes on biology (St. John’s: Memorial University of Newfoundland).
Merten V., Bayer T., Reusch T. B. H., Puebla O., Fuss J., Stefanschitz J., et al. (2021). An integrative assessment combining deep-sea net sampling, in situ observations and environmental DNA analysis identifies Cabo Verde as a cephalopod biodiversity hotspot in the Atlantic Ocean. Front. Mar. Sci. 8. doi: 10.3389/fmars.2021.760108
Merten V., Puebla O., Bayer T., Reusch T. B. H., Fuss J., Stefanschitz J., et al. (2023). Arctic nekton uncovered by e DNA metabarcoding: Diversity, potential range expansions, and pelagic-benthic coupling. Environ. DNA 5, 503–518. doi: 10.1002/edn3.403
Michalsen K., Dalpadado P., Eriksen E., Gjøsaeter H., Ingvaldsen R. B., Johannesen E., et al. (2011). “The joint Norwegian-Russian ecosystem survey: overview and lessons learned,” in Proceeding of the 15th Norwegian-Russian Symposium “Svalbard Climate Change and Effects on the Barents Sea Marine Living Resources. Eds. Haug T., Dolgov A. V., Drevetnyak K., Røttingen I., Sunnanå K., Titov O. (Institute of Marine Research, Bergen), 247–272.
Mikkelsen N., Planque B., Arneberg P., Skern-Mauritzen M., Hansen C., Fauchald P., et al. (2023). Multiple stakeholders’ perspectives of marine social ecological systems, a case study on the Barents Sea. Ocean. Coast. Manage. 242, 106724. doi: 10.1016/j.ocecoaman.2023.106724
Moe A., Jørgensen A.-K. (2013). Offshore mineral development in the Russian Barents Sea. Post-Sov. Geo. Eco. 41, 98–133. doi: 10.1080/10889388.2000.10641135
Muus B. J. (2002). The bathypolypus–benthoctopus problem of the north atlantic (Octopodidae, cephalopoda). Malacologia 44, 175–222.
Nesis K. N. (1987). “Cephalopod molluscs of the Arctic Ocean and its seas,” in Fauna and distribution of molluscs: North Pacifc and Arctic Basin. Ed. Kafanov A. I. (USSR Academy of Sciences, Vladivostok), 115–136.
Nesis K. N. (2001). West-Arctic and East-Arctic distributional ranges of cephalopods. Sarsia 86, 1–11. doi: 10.1080/00364827.2001.10420456
Nozères C., Roy V. (2021). Photo catalogue of coastal marine fauna on the Icelandic Scallop (Chlamys islandica) survey in the northern Gulf of St. Lawrence. Can. Manuscr. Rep. Fish. Aquat. Sci. 3207, 1–165.
Oesterwind D., Barrett C. J., Sell A. F., Nunez-Riboni I., Kloppmann M., Piatkowski U., et al. (2022). Climate change-related changes in cephalopod biodiversity on the North East Atlantic Shelf. Biodivers. Conserv. 31, 1491–1518. doi: 10.1007/s10531–022-02403-y
Overland J. E., Wang M., Walsh J. E., Stroeve J. C. (2014). Future Arctic climate changes: adaptation and mitigation time scales. Earth’s. Future 2, 68–74. doi: 10.1002/2013EF000162
Pecl G. T., Jackson G. D. (2008). The potential impacts of climate change on inshore squid: biology, ecology and fisheries. Rev. Fish. Biol. Fisheries. 18, 373–385. doi: 10.1007/s11160–007-9077–3
Praetorius S., Rugenstein M., Persad G., Caldeira K. (2018). Global and Arctic climate sensitivity enhanced by changes in North Pacific heat flux. Nat. Commun. 9, 3124. doi: 10.1038/s41467–018-05337–8
Pratt A., France S. C., Vecchione M. (2021). Survey of bathyal incirrate octopods in the western North Atlantic. Mar. Biodivers. 51, 49. doi: 10.1007/s12526–021-01191-y
Pratt A., France S. C., Vecchione M. (2023). Deep octopod habitat in the western North Atlantic characterized by Standard Ecological Classification from videos. Ecosphere 14, e4699. doi: 10.1002/ecs2.4699
Rantanen M., Karpechko A. Y., Lipponen A., Nordling K., Hyvarinen O., Ruosteenoja K., et al. (2022). The Arctic has warmed nearly four times faster than the globe since 1979. Commun. Earth Environ. 3, 168. doi: 10.1038/s43247–022-00498–3
Reid A., Jereb P. (2005). “Family Sepiolidae,” in Cephalopods of the world. An annotated and illustrated catalogue of species known to date. No.4, Vol. 1: Chambered nautiluses and sepioids (Nautilidae, Sepiidae, Sepiolidae, Sepiadariidae, Idiosepiidae and Spirulidae). Eds. Jereb P., Roper C. F. E. (FAO, Rome), 153–203.
Renaud P. E., Sejr M. K., Bluhm B. A., Sirenko B., Ellingsen I. H. (2015). The future of Arctic benthos: expansion, invasion, and biodiversity. Prog. Oceanogr. 139, 244–257. doi: 10.1016/j.pocean.2015.07.007
Robinson N. J., Johnsen S., Brooks A., Frey L., Judkins H., Vecchione M., et al. (2021). Studying the swift, smart, and shy: unobtrusive camera-platforms for observing large deep-sea squid. Deep-Sea Res. I. 172, 103538. doi: 10.1016/j.dsr.2021.103538
Robison B. H. (2004). Deep pelagic biology. J. Exp. Mar. Biol. Ecol. 300, 253–272. doi: 10.1016/j.jembe.2004.01.012
Robison B. H., Reisenbichler K. R., Sherlock R. E., Silguero J. M. B., Chavez F. P. (1998). Seasonal abundance of the siphonophore, Nanomia bijuga, in Monterey Bay. Deep-Sea Res. II. 45, 1741–1751. doi: 10.1016/S0967–0645(98)80015–5
Rourke M. L., Fowler A. M., Hughes J. M., Broadhurst M. K., Di Battista J. D., Fielder S., et al. (2022). Environmental DNA (eDNA) as a tool for assessing fish biomass: a review of approaches and future considerations for resource surveys. Environ. DNA 4, 9–33. doi: 10.1002/edn3.185
Sabirov R. M., Golikov A. V., Nigmatullin C., Lubin P. A. (2012). Structure of the reproductive system and hectocotylus in males of lesser flying squid Todaropsis eblanae (Cephalopoda: Ommastrephidae). J. Nat. Hist. 46, 1761–1778. doi: 10.1080/00222933.2012.700335
Sabirov R. M., Lubin P. A., Golikov A. V. (2009). Finding of the lesser flying squid Todaropsis eblanae (Oegopsida, Ommastrephidae) in the Barents Sea. Zool. J. 88, 1010–1012.
Sheridan J. A., Bickford D. (2011). Shrinking body size as an ecological response to climate change. Nat. Clim. Change 1, 401–406. doi: 10.1038/nclimate1259
Snoeijs-Leijonmalm P., Flores H., Sakinan S., Hildebrandt N., Svenson A., Castellani G., et al. (2022). Unexpected fish and squid in the central Arctic deep scattering layer. Sci. Adv. 8, 7536. doi: 10.1126/sciadv.abj7536
Stratmann T., Simon-Lledó E., Morganti T. M., de Kluijver A., Vedenin A., Purser A. (2022). Habitat types and megabenthos composition from three sponge-dominated high-Arctic seamounts. Sci. Rep. 12, 20610. doi: 10.1038/s41598–022-25240-z
Swart N. C., Fyfe J. C., Hawkins E., Kay J. E., Jahn A. (2015). Influence of internal variability on Arctic sea-ice trends. Nat. Clim. Change 5, 86–89. doi: 10.1038/nclimate2483
Taberlet P., Coissac E., Hajibabaei M., Rieseberg L. H. (2012a). Environmental DNA. Mol. Ecol. 21, 1789–1793. doi: 10.1111/j.1365–294X.2012.05542.x
Taberlet P., Coissac E., Pompanon F., Brochmann C., Willerslev E. (2012b). Towards next-generation biodiversity assessment using DNA metabarcoding. Mol. Ecol. 21, 2045–2050. doi: 10.1111/j.1365–294X.2012.05470.x
Taite M., Dillon L., Strugnell J. M., Drewery J., Allcock A. L. (2023). DNA barcoding reveals unexpected diversity of deep-sea octopuses in the North-East Atlantic. Biol. Environ. 123, 1–12. doi: 10.1353/bae.2023.0000
Taite M., Vecchione M., Fennell S., Allcock L. A. (2020). Paralarval and juvenile cephalopods within warm-core eddies in the North Atlantic. Bull. Mar. Sci. 96, 235–262. doi: 10.5343/bms.2019.0042
Takahara H., Kidokoro H., Sakurai Y. (2017). High temperatures may halve the lifespan of the Japanese flying squid, Todarodes pacificus. J. Nat. Hist. 51, 2607–2614. doi: 10.1080/00222933.2016.1244297
Thomsen P. F., Møller P. R., Sigsgaard E. E., Knudsen S. W., Jørgensen O. A., Willerslev E. (2016). Environmental DNA from seawater samples correlate with trawl catches of Subarctic, deepwater fishes. PloS One 11, e0165252. doi: 10.1371/journal.pone.0165252
Thomsen P. F., Willerslev E. (2015). Environmental DNA – an emerging tool in conservation for monitoring past and present biodiversity. Biol. Cons. 183, 4–18. doi: 10.1016/j.biocon.2014.11.019
Treble M. A. (2007). Analysis of data from the 2006 trawl surveys in NAFO Division 0A. NAFO SCR Doc. 07/41. Dartmouth: Northwest Atlantic Fisheries Organization. 1–25.
Vecchione M., Young R. E., Piatkowski U. (2010). Cephalopods of the northern mid-atlantic ridge. Mar. Biol. Res. 6, 25–52. doi: 10.1080/17451000902810751
Visser F., Merten V. J., Bayer T., Oudejans M. G., de Jonge D. S. W., Puebla O., et al. (2021). Deep-sea predator niche segregation revealed by combined cetacean biologging and eDNA analysis of cephalopod prey. Sci. Adv. 7, 5908. doi: 10.1126/sciadv.abf5908
Xavier J. C., Cherel Y., Allcock L., Rosa R., Sabirov R. M., Blicher M. E., et al. (2018). A review on the biodiversity, distribution and trophic role of cephalopods in the Arctic and Antarctic marine ecosystems under a changing ocean. Mar. Biol. 165, 93. doi: 10.1007/s00227–018-3352–9
Zakharov D. V., Jørgensen L. L., Manushin I. E., Strelkova N. A. (2020). Barents Sea megabenthos: spatial and temporal distribution and production. Mar. Biol. J. 5, 19–37. doi: 10.21072/mbj.2020.05.2.03
Zakharov D. V., Strelkova N. A., Manushin I. E., Zimina O. L., Jørgensen L. L., Lyubin P. A., et al. (2018). Atlas of the megabenthic organisms of the Barents Sea and adjacent waters (Murmansk: PINRO Press).
Keywords: arctic, climate change, atlantification, marine ecosystems, monitoring, Cephalopoda, shelf, deep-sea
Citation: Golikov AV, Jørgensen LL, Sabirov RM, Zakharov DV and Hoving HJ (2024) Long-term annual trawl data show shifts in cephalopod community in the western Barents sea during 18 years. Front. Mar. Sci. 11:1392585. doi: 10.3389/fmars.2024.1392585
Received: 27 February 2024; Accepted: 02 May 2024;
Published: 23 May 2024.
Edited by:
Paco Bustamante, Université de la Rochelle, FranceReviewed by:
Alexandra Lischka, Ecofish Research Ltd., CanadaTobias Büring, South Atlantic Environmental Research Institute, Falkland Islands
Marek Lipinski, Rhodes University, South Africa
Copyright © 2024 Golikov, Jørgensen, Sabirov, Zakharov and Hoving. This is an open-access article distributed under the terms of the Creative Commons Attribution License (CC BY). The use, distribution or reproduction in other forums is permitted, provided the original author(s) and the copyright owner(s) are credited and that the original publication in this journal is cited, in accordance with accepted academic practice. No use, distribution or reproduction is permitted which does not comply with these terms.
*Correspondence: Alexey V. Golikov, golikov.ksu@gmail.com