- 1Zhejiang Ocean University, Zhoushan, China
- 2Key Laboratory of Mariculture and Enhancement, Zhejiang Marine Fisheries Research Institute, Zhoushan, China
- 3Aquaculture Genetics and Breeding Laboratory, Zhoushan Fisheries Research Institute, Zhoushan, China
In Zhejiang province, large yellow croakers are primarily cultured in net cages, facing significant challenges during the overwintering period such as susceptibility to cold and starvation stress. Notably, the observable sexual dimorphism in the large yellow croaker hints at the likelihood of gender differences in their responses to these environmental stresses. However, the potential sex-specific adaptive changes during overwintering remain unexplored. To gain deeper insights, we investigated the expression of intestinal barrier-related genes, immune responses, and changes in intestinal microbiota during the overwintering period in males and females separately. The results revealed a more pronounced loss of body weight in females than that in males. In male intestines, there was a significant decrease in the expression of intestinal barrier-related genes (arp2/3, occludin, and zo1), contrasting with a significant increase in females. The expression of TLR1, TLR3, TLR7, TLR9, MyD88, and NF-κB genes in the intestines of female fish decreased significantly in March compared to November, while the opposite trend was observed in male fish. However, in the liver, TLR1, TLR3, TLR7, TLR9, MyD88, and NF-κB genes expression were both decreased significantly in males and females. In the male intestines, there was a significant increase in the expression of inflammatory cytokine genes (IL-1β and IL-6). In the females, IL-1β gene expression significantly decreased, while IL-6 expression increased significantly. The expression of IL-10 genes decreased in both males and females. In the liver, both the males and females exhibited a significant increase in the expression of IL-1β and IL-6 genes. Further analysis revealed greater susceptibility of male intestinal microbiota diversity during the overwintering period. Firmicutes’ relative abundance exhibited opposing changes between the males and females, and Proteobacteria abundance, driven by a significant increase in Vibrio bacteria, significantly increased in the males. In conclusion, the overwintering period may compromise the structural integrity of male fish intestines, reducing their immune function. Additionally, the response strategy of the intestinal microbiota differs between sexes. The findings provide crucial insights for crafting effective strategies and management decisions in cage-cultured large yellow croaker during the overwintering period, as well as offering theoretical references for monosex aquaculture.
1 Introduction
Winter poses a substantial challenge to fish, especially those cultured in outdoor environments. The prevalence of low temperatures in winter significantly impacts the health and production of farmed fish. Periodically, fish may experience mass mortality attributed to conditions commonly referred to as “Winter Disease” or “Winter Syndrome” (Ibarz et al., 2010). The onset of these conditions is closely associated with low temperatures, serving as the primary precipitating factor. Fish affected by Winter Syndrome exhibit a distinctive pattern of multi-organ dysfunction, resulting in tissue damage, immune suppression, and metabolic depression. Additionally, the low winter temperature can trigger cold-induced fasting in fish, leading to specific physiological responses. Previous studies have demonstrated that starvation leads to a profound disruption of the digestive system (Ibarz et al., 2010). The intestine, serving as the primary organ for food digestion and absorption, also functions as a vital immune organ. The intestinal epithelial tissue, a pivotal component of the intestinal physical barrier, constitutes the first line of defense against infections and plays a crucial role in maintaining fish health. Moreover, exposure to low temperatures can trigger the activation of Toll-like receptors (TLRs), such as TLR3 and TLR5. This activation is concomitant with the initiation of the NF-κB signaling pathway, leading to the overexpression of pro-inflammatory cytokines, including TNF-α, IL-6, and IL-1β (Chen et al., 2021). For instance, the pro-inflammatory cytokines interleukin-1β (IL-1β) and interferon-γ (IFN-γ) exhibited a substantial up-regulation in the spleens of rainbow trout exposed to low temperatures (Raida and Buchmann, 2007).
The intestinal microbiota is essential for the development and regulation of the immune system of the host (Tremaroli and Bäckhed, 2012; Sommer and Bäckhed, 2013). In their natural environment, fish experience complex physiological changes during overwintering, affecting various aspects including metabolism, immunity, and reproduction (Myers, 1949; Cunjak, 1988; Song et al., 2019). The intestinal microbiota is closely linked to fish physiology, with its fluctuations directly impacting the fish’s health (Liu et al., 2021). The large yellow croaker (Larimichthys crocea), widely cultured in China, is predominantly farmed using inshore cage culture methods (Luo, 2020). Zhejiang Province in China stands is the second-largest production area for large yellow croaker aquaculture, achieving a production of 35,000 tons in 2022. In recent years, rapid global climate change has resulted in extremely low winter temperatures in the southern coastal regions of China. In the northern region of Zhejiang Province, specifically Zhou Shan, sea temperatures can drop below 10°C in winter. Large yellow croakers are particularly sensitive to cold conditions, with reports indicating reduced activity and decreased food intake when the water temperature drops below 10°C (Yi et al., 1998).Previous studies have shown that the morphological structures of the digestive tract and liver in large yellow croaker change after overwintering (Wang et al., 2016). Our previous study found that low temperatures impact the antioxidant capability and heat shock protein expression in large yellow croaker (Lv et al., 2021). However, this experiment was conducted in a laboratory setting with temperature regulated by an automatic control system, but there is still a lack of studies in fish living in natural conditions.
Numerous studies have highlighted gender disparities in immune responses. Klein and Flanagan’s research demonstrated that in humans, women with acute HIV infections have lower viral RNA levels in their blood compared to men, alongside heightened phagocytic activity and antibody responses (Klein and Flanagan, 2016). Dou et al. revealed that the abundance of immune-related genes on the X chromosome plays a pivotal role in immune regulation, with these genes exhibiting higher expression levels in females (Dou et al., 2024). In murine models, studies by Dubois et al. using the NZB/NZW mice illustrated the impact of gender differences on autoimmune diseases like systemic lupus erythematosus, where female mice exhibited higher disease incidence and severity (Dubois et al., 1966). These findings not only shed light on gender differences in immune responses among humans and rodents but also underscore the necessity for further research. Notably, investigations into similar phenomena in fish remain unreported, indicating an unexplored area worthy of investigation.
In this study, we collected large yellow croakers from the cage during overwintering to investigate various aspects of their physiology. First, we analyzed the expression levels of genes associated with tight junction barriers in the intestines of both male and female individuals. Second, we examined the expression levels of genes related to Toll-like receptor (TLR) signaling and inflammation-related cytokines in the intestines and livers of both sexes throughout the overwintering period. Third, we investigated the dynamic changes in intestinal microbiota between males and females. By elucidating these aspects, our study aims to comprehensively elucidate how both male and female individuals adapt to low temperatures in winter, providing insights into the sexual dimorphism of large yellow croakers and establishing a theoretical foundation for their monosexual aquaculture.
2 Materials and methods
2.1 Experimental animal
This study obtained juvenile large yellow croakers, bred from fertilized eggs through natural spawning of the Dai-qu broodstock after the injection of luteinizing hormone-releasing hormone (LHRH)-A3 (Ningbo Second Hormone Factory, Cixi, China), from the Zhoushan Fisheries Research Institute in Zhoushan, China. Prior to commencing the experiment, the fish underwent a one-month acclimation period in a holding cage measuring 4 × 5 × 2 m, strategically positioned near Dengbu Island in Zhoushan to facilitate adaptation to the prevailing culture conditions. Subsequently, 1,200 juvenile large yellow croakers, 180 days post-hatch (dph) and averaging 72.35 ± 4.41 g in weight, were selected randomly and allocated evenly to six experimental cages (2 × 2 × 2 m), with 200 individuals per cage. The fish were fed three times daily with commercial feed. The components of this feed included 49.0% crude protein, 8.0% crude fat, 6.0% crude fiber, 16% ash, 3.2% lysine, 11% moisture, and 1.2% total phosphorus. Detailed environmental parameters can be found on Supplementary Table S1. The study was conducted from November 2021 to April 2022.
2.2 Sample collection
The experiment commenced on November 1st, and fish was sampled on November 1st, January 1st, March 1st, and April 1st. Prior to sampling, the fish underwent a 24-hour fasting period. In each cage, we randomly selected twenty individuals and measured their body weight and length. The fish were euthanized with MS-222 (tricaine methane sulphonate) before the dissection. Subsequently, a dissection was performed to observe the gonads and determine the sex of each individual. We sampled the liver, mid-intestine, and mid-intestinal contents following the methods described by Li et al. (2022). We cleaned the surface of each fish using 75% ethanol and then rinsed with sterile water. The mid-intestine was excised, and its contents were transferred into a 2 mL sterile tube. Initially, the mid-intestine was carefully transferred into a sterile culture dish, following which it underwent a meticulous dissection utilizing sterilized scissors. Thereafter, sterile phosphate-buffered saline was employed to gently lavage the mid-intestine, with the resultant contents being cautiously transferred into a sterile 2 mL tube. Similarly, liver and mid-intestinal samples were separately enclosed in 2 mL sterile tubes. All samples were immediately frozen in liquid nitrogen and stored at -80°C. The collected livers and mid-intestines were used for gene expression analysis, and the contents were analyzed for intestinal microbiota. In this study, fish samples were systematically categorized into eight groups based on the sampling month and gender. These groups included ‘Nov-M’ and ‘Nov-F’ for male and female fish sampled on November 1st, ‘Jan-M’ and ‘Jan-F’ for those sampled on January 1st, ‘Mar-M’ and ‘Mar-F’ for the samples taken on March 1st, and ‘Apr-M’ and ‘Apr-F’ for the samples taken on April 1st.
2.3 Growth indices
In the sampling process, twenty individuals were randomly selected from each experimental cage for the measurement of weight, body length, and total length. The calculation of Fulton’s K condition factor was performed using the following equation, which is in accordance with previous reports (Faggion et al., 2021):
where BW= body weight (g), and BL= body length (cm).
2.4 RNA preparation, cDNA synthesis, and quantitative real-time PCR
Total RNA from the liver and mid-intestine was extracted using the Total RNA Extraction Kit (Solarbio, China), with three replicates conducted for each sample during the reaction. The quality and concentration of total RNA were assessed with a NanoDrop-2000 (Thermo, USA). cDNA synthesis for each sample was performed using the PrimeScript™ RT Reagent Kit (Takara, Japan). Inflammation-related genes, such as TLR1, TLR3, TLR7, TLR9, MyD88, NF-κB, Arg1, IL-6, IL-10, IL-1β, arp2/3, occludin, and zo1, were analyzed. Primers were listed in Supplementary Table S2. PCR amplification was conducted as follows: 95°C for 10 min, then 40 cycles of 95°C for 5 s and 60°C for 30 s. The relative expression levels of the target genes were quantified using the 2-ΔΔCt method (Livak and Schmittgen, 2001).
2.5 DNA extraction of intestinal contents and 16S rRNA gene sequencing
Total DNA from each intestinal content sample was extracted using the QIAGEN DNeasy PowerSoil Pro Kit (Hilden, Germany). DNA concentration and integrity were detected using NanoDrop-2000 (Thermo, USA). The total DNA samples were sent to Shanghai Oe Biotech Co., Ltd. (Shanghai, China) for Illumina sequencing on the MiSeq platform. The barcoded fusion primers of 343F (5′-TACGGRAGGCAGCAG-3′) and 798R (5′-AGGGTATCTAATCCT-3′) were employed to amplify the V3+V4 region of 16S rRNA.
2.6 Bioinformatic analysis
Raw sequencing data were preprocessed with cutadapt software for adapter detection and removal. Paired-end reads were merged and chimeric sequences removed using DADA2 (Callahan et al., 2016). ASVs were annotated using Silva database Version 138. The bacterial diversity metrics, i.e. Chao1 and Shannon indices, were assessed using QIIME software. The differences of α-diversity metrics were analyzed using a two-way ANOVA test with SPSS software (v. 25.0). Principal coordinate analysis (PCoA) based on weighted Unifrac distance was executed using QIIME software. Adonis analysis was applied to assess the dissimilarity of the bacterial community composition between the female and male based on Bray-Curtis distance using the vegan package in R (v. 4.1.0). The categories of bacterial taxa were assessed according to the report of Dai et al. (2016).
2.7 Statistical analysis
Relative gene expression levels were analyzed using one-way analysis of variance (ANOVA). For multiple comparisons, Tukey’s test was employed. Variations in bacterial composition between groups were assessed using Kruskal-Wallis rank-sum tests. Significant differences were considered at a P value of less than 0.05. All data were presented as means ± standard errors of the mean (SEM).
3 Results
3.1 Growth indices
The body weight, body length, total length and Fulton’s K condition factor were analyzed to evaluate the growth performance of fish which were cultured in the cages (Figure 1). The body length and total length of Jan-M, Mar-M and Apr-M group were significantly larger than those of the Nov-M group (P < 0.05). Similarly, the body length of Jan-F, Mar-F and Apr-F group were significantly larger than those of the Nov-F group (P < 0.05). The body weight of the Jan-M and Mar-M groups were significantly higher than those of the Nov-M and Apr-M groups (P < 0.05). The body weight in the Jan-F group was significantly higher than that of the Nov-F, Mar-F and Apr-F groups (P < 0.05). There was no significant difference in body weight among the Nov-F, Mar-F, and Apr-F groups (P > 0.05). Fulton’s K condition factor significantly decreased during the overwintering period, regardless of males or females. The Jan-F group exhibited a significantly higher Fulton’s K condition factor than the Jan-M group (P < 0.05), and similarly, the Mar-F group’s factor was notably higher than that of the Mar-M group (P < 0.05).
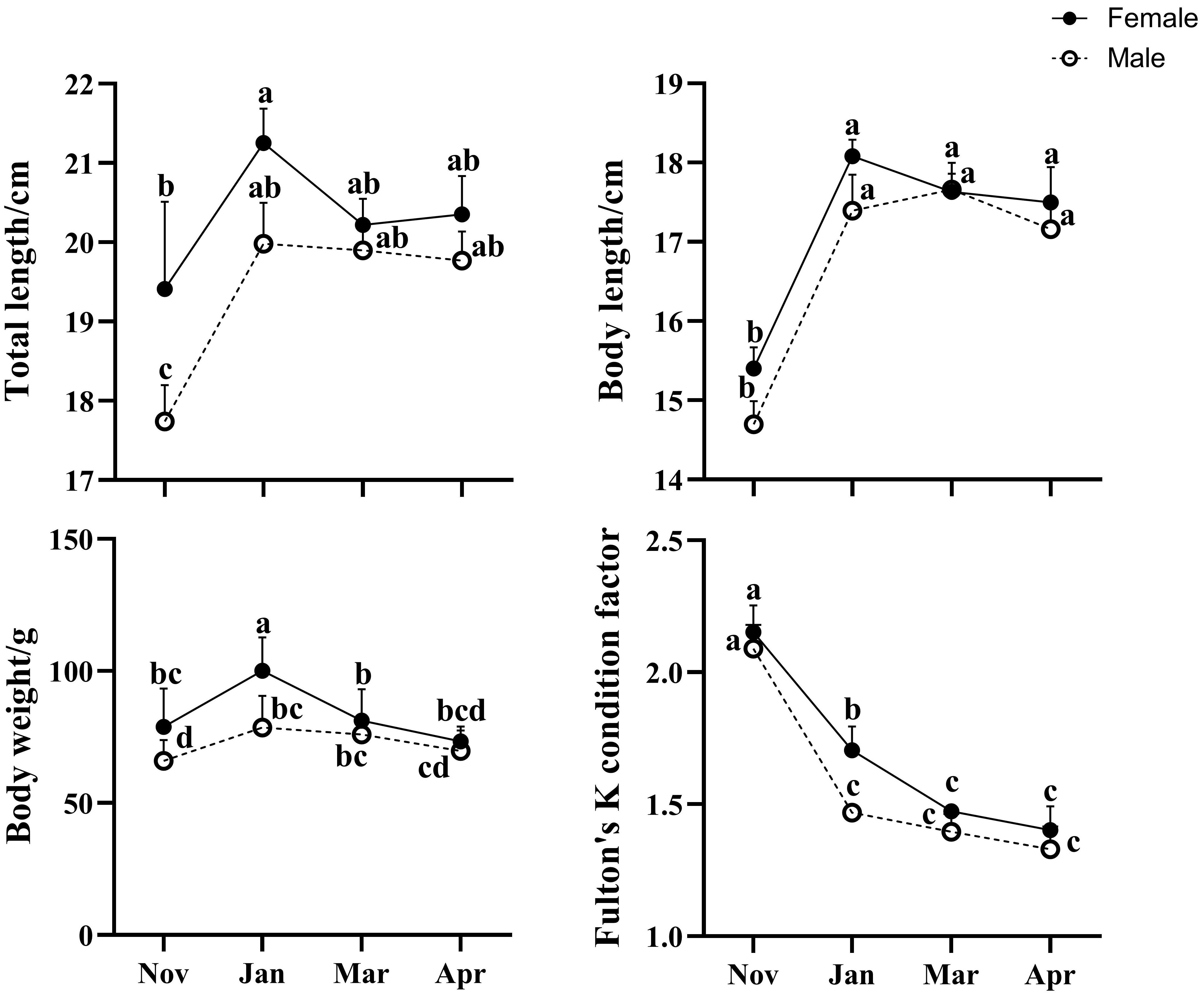
Figure 1 The changes of the total length, body length, body weight, and Fulton’s K condition factor of large yellow croaker during the overwintering period. Data were expressed as mean ± S. E. M. Data with different letters at the column indicated significant differences (P < 0.05). Nov, Jan, Mar and Apr represented November, January, March and April, respectively. M, males; F, females.
3.2 Expression levels of arp2/3, occludin and zo1 genes in the intestine
The expression levels of arp2/3, occludin and zo1 genes were detected to assess the intestinal structural integrity. Figure 2 showed significantly lower expression of arp2/3 and zo1 genes in the Jan-M and Mar-M groups compared to the Nov-M and Apr-M groups (P < 0.05). The occludin gene expression in the Jan-M and Mar-M groups was noticeably lower than in the Nov-M and Apr-M groups. In contrast, arp2/3, occludin, and zo1 gene expression levels increased significantly in the Jan-F and Mar-F groups compared to the Nov-F group. Specifically, the expression level of arp2/3 gene in the Jan-F and Mar-F groups was significantly higher than those in the Nov-F and Apr-F groups (P < 0.05).
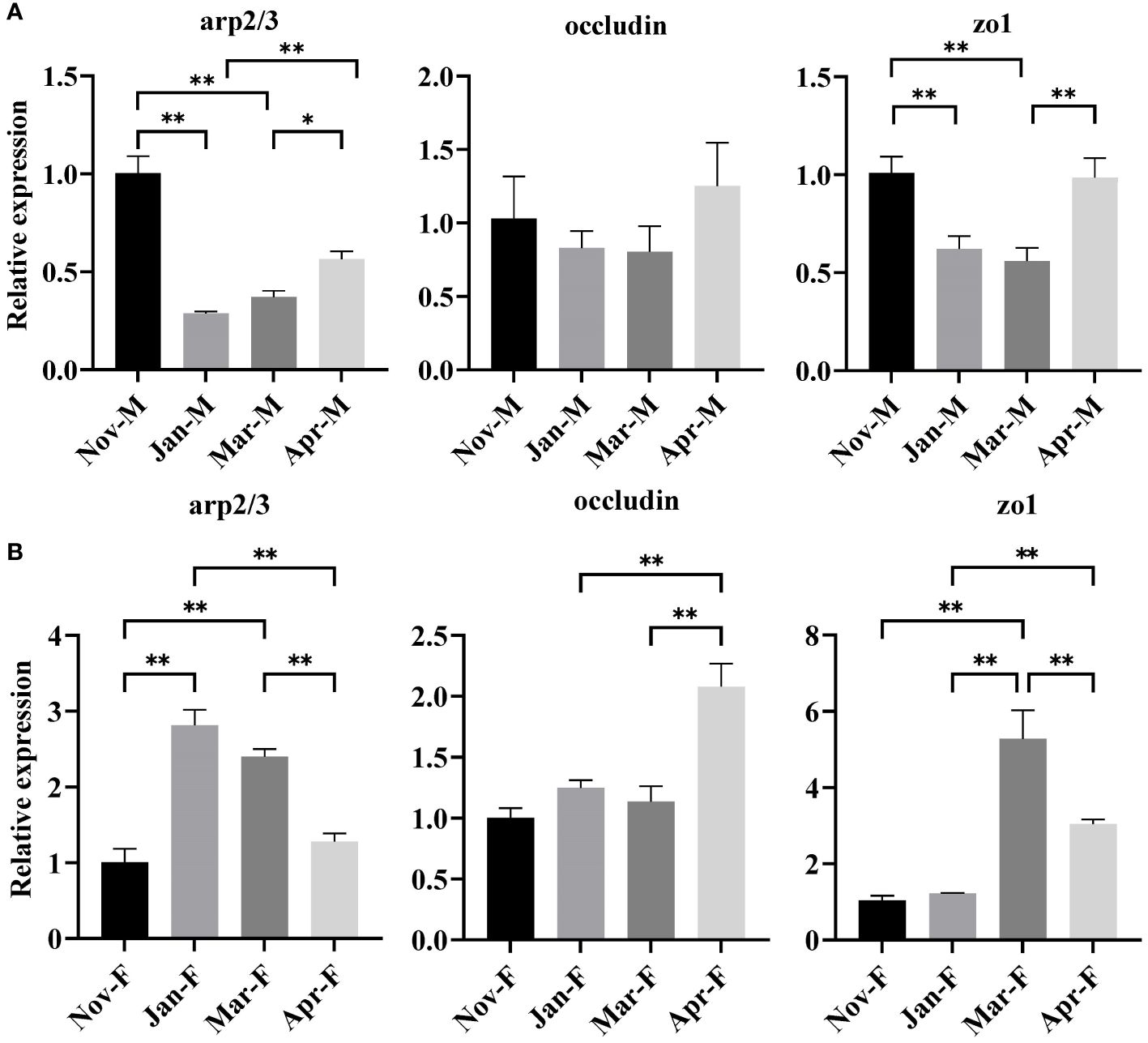
Figure 2 Expression levels of barrier-related genes in the intestine of males (A) and females (B). Data were expressed as mean ± S. (E) M. * represented P < 0.05 between two groups; ** represented P < 0.01 between two groups. Nov, Jan, Mar and Apr represented November, January, March and April, respectively. M, males; F, females.
3.3 Expression levels of TLR-related genes and inflammation-related cytokine genes in the intestine
The expression levels of TLR-related genes and inflammation-related cytokine genes in mid-intestine of the male and female fish were analyzed during overwintering period (Figure 3). The expression levels of TLR1, TLR7 and TLR9 genes in the Jan-M and Mar-M groups decreased significantly compared to the Nov-M group (P < 0.05). The Jan-M group showed a significant increase in TLR3 gene expression compared to the Nov-M and Mar-M groups (P < 0.05). The expression level of MyD88 and NF-κB genes in the Jan-M group was notably lower than in the Nov-M group. Compared to the Nov-M and Jan-M groups, expression level of MyD88 gene in the Mar-M group significantly decreased (P < 0.05). In female fish, the expression levels of TLR1 and TLR3 genes in the Jan-F and Mar-F groups were significantly higher than those in the Nov-F group (P < 0.05). The expression level of TLR7 gene increased significantly in the Mar-F group compared to the Nov-F and Apr-F groups (P < 0.05). The expression level of TLR9 gene in the Jan-F group was significantly lower than that in the Nov-F group (P < 0.05). The expression level of MyD88 gene in the Jan-F and Mar-F groups significantly increased compared to the Nov-F group (P < 0.05). The expression level of NF-κB gene in the Mar-F group was significantly higher than that in the Nov-F group (P < 0.05). For inflammation-related cytokine genes, the expression levels of IL-6 and IL-1β genes in the Jan-M and Mar-M groups were significantly higher than those in the Nov-M group (P < 0.05). Similarly, the expression levels of IL-6 gene in the Jan-F and Mar-F groups were significantly higher than those in the Nov-F group (P < 0.05). The expression level of IL-1β gene significantly decreased in comparison to the Nov-F and Apr-F groups (P < 0.05). Arg1 and IL-10, as anti-inflammatory genes, showed significantly lower expression in the Jan-M, Mar-M, and Apr-M groups compared to the Nov-M group (P < 0.05). No significant difference in the expression level of Arg1 gene was observed among Nov-M, Jan-M and Mar-M groups (P > 0.05). The expression level of IL-10 gene in the Jan-F, Mar-F and Apr-F groups was significantly lower than that in the Nov-F group (P < 0.05). The expression level of Arg1 gene in the Jan-F group was significantly higher than that in the Nov-F, Mar-F and Apr-F groups (P < 0.05).
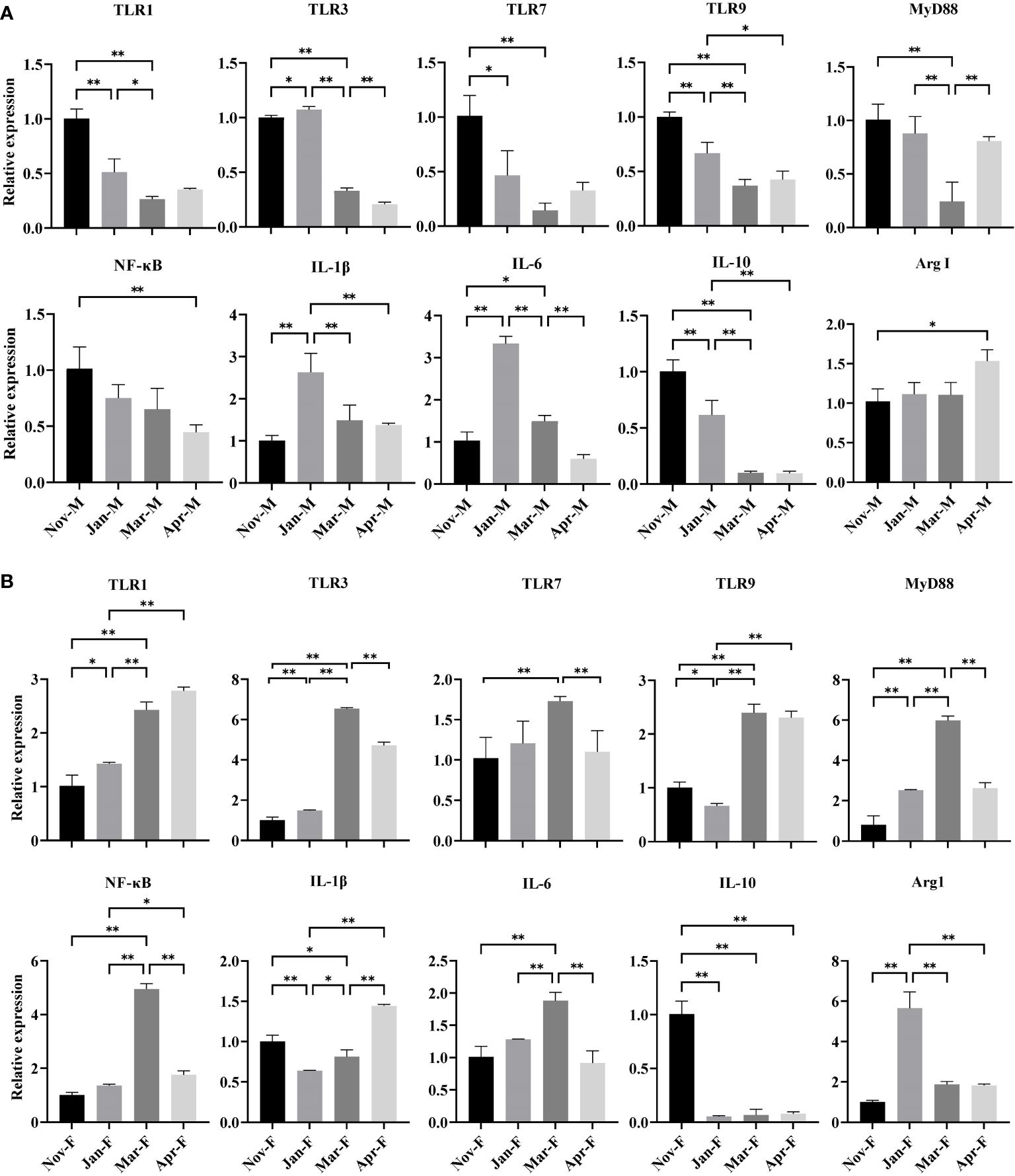
Figure 3 Expression levels of TLR-related genes and inflammation-related cytokine genes in the intestine of males (A) and females (B). Data were expressed as mean ± S. (E) M. * represented P < 0.05 between two groups; ** represented P < 0.01 between two groups. Nov, Jan, Mar and Apr represented November, January, March and April, respectively. M, males; F, females.
3.4 Expression levels of TLR-related genes and inflammation-related cytokine genes in the liver
The expression levels of TLR1, TLR3, TLR7, TLR9, MyD88 and NF-κB genes in the Jan-M and Mar-M groups were significantly lower than those in the Nov-M and Apr-M groups (P < 0.05, Supplementary Figure 1). Similarly, the expression levels of TLR1, TLR3, TLR7 and TLR9 genes in the Jan-F and Mar-F groups were significantly lower than those in the Nov-F and Apr-F groups (P < 0.05). The expression levels of MyD88 and NF-κB genes in the Jan-F group was significantly lower than that in the Nov-F group (P < 0.05). The expression levels of IL-6, IL-1β and IL-10 genes in the Jan-M and Mar-M groups were significantly higher than those in the Nov-M group (P < 0.05). Conversely, the expression level of Arg1 gene in the Jan-M and Mar-M was significantly lower than that in the Nov-M group (P < 0.05). The expression levels of IL-6, IL-1β and Arg1 genes in the Jan-F and Mar-F groups were significantly higher than those in the Nov-F group (P < 0.05). The expression level of IL-10 in the Jan-F, Mar-F and Apr-F groups were significantly lower than that in the Nov-F group (P < 0.05).
3.5 Richness, diversity, and structure of the intestinal microbiota
We obtained 2,940,565 valid sequences (average: 61,261 ± 1,061; range: 35,346–71,388) from 48 samples using Illumina MiSeq sequencing. No significant difference was observed in the species count of intestinal microbiota between males and females at any time point (P > 0.05) (Supplementary Figure 2). The Mar-M group exhibited the lowest number of ASVs in male intestinal microbiota (Figure 4A). Fifty-nine ASVs were shared among Nov-F, Jan-F, Mar-F, and Apr-F groups, and most of them belonged to Proteobacteria (42.39%), Bacteroidota (33.68%), Firmicutes (17.54%) and Desulfobacterota (6.39%) (Figure 4B). The Mar-F group had the lowest number of ASVs in female intestinal microbiota (Figure 4C). Among them, 66 shared ASVs were predominantly from Proteobacteria (40.35%), Bacteroidota (39.93%), Firmicutes (18.50%), and Campilobacterota (1.22%) (Figure 4D). The results of the Kruskal-Wallis test show that there is a significant difference in the number of ASVs among all groups (P < 0.05), with a total of 127 ASVs differing.
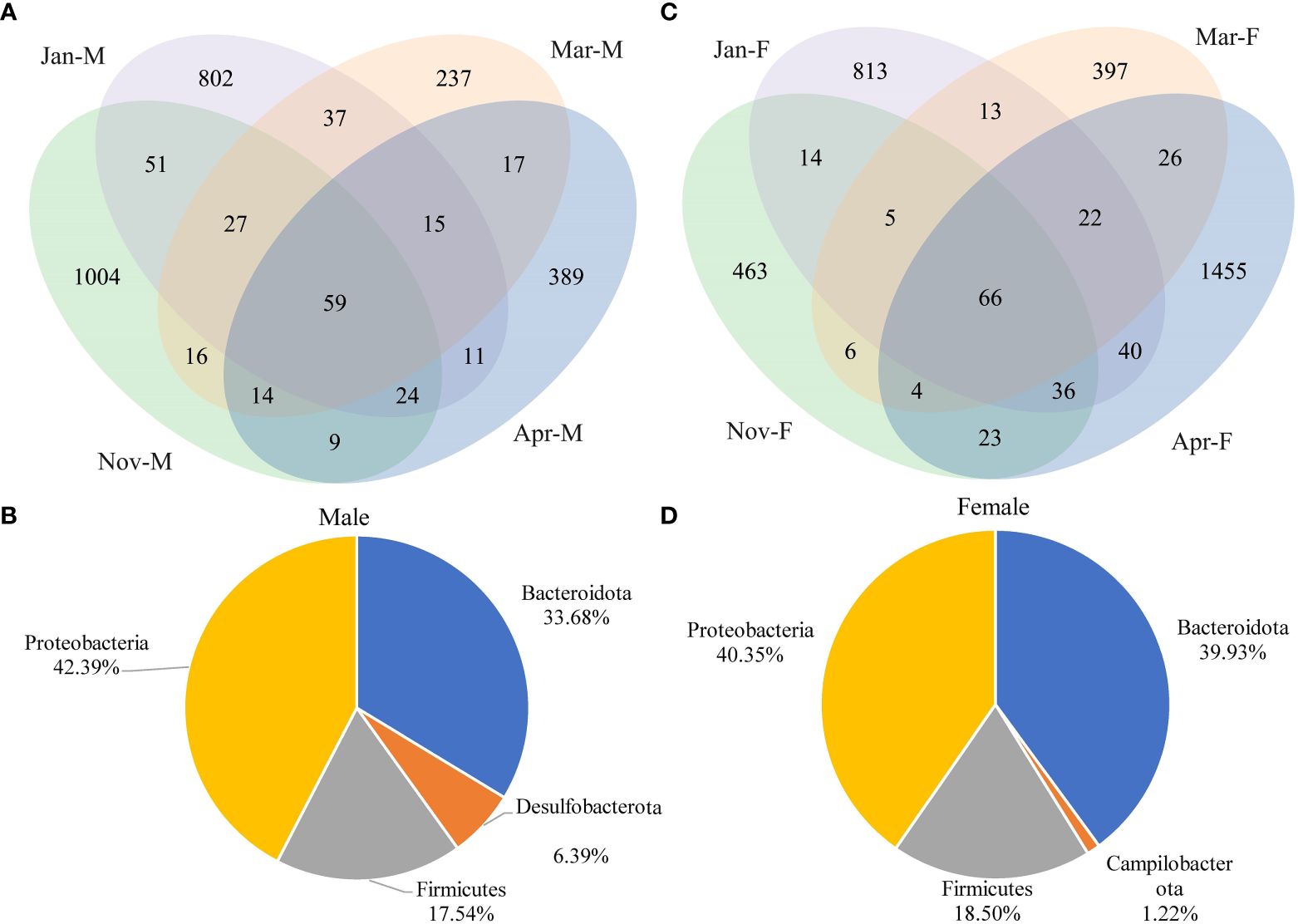
Figure 4 Venn diagram analysis of intestinal microbiota in all groups. Venn diagram analysis of intestinal microbiota in males (A) and females (C). Dominant composition of shared ASVs at phylum level in males (B) and females (D). Nov, Jan, Mar and Apr represented November, January, March and April, respectively. M, males; F, females.
The richness and diversity of intestinal microbiota were assessed using the Chao1 and Shannon indices, respectively (Figure 5). No significant differences in the Chao1 and Shannon indices were observed between the male and female fish (Chao1: F = 1.142, P = 0.291; Shannon: F = 0.191, P = 0.664) (Supplementary Table 3). There were no significant differences in the Chao1 and Shannon indices among Nov-F, Jan-F, Mar-F and Apr-F groups (P > 0.05). Significant differences in the Chao1 index were detected between Nov-M and Mar-M, Nov-M and Apr-M, and Jan-M and Mar-M groups (P < 0.05). Shannon index in the Mar-M group decreased significantly compared to the Nov-M and Jan-M groups (P < 0.05). These results indicated a significant decrease in richness and diversity of male intestinal microbiota during the overwintering period. In contrast, richness and diversity in female intestinal microbiota remained relatively stable during the overwintering period.
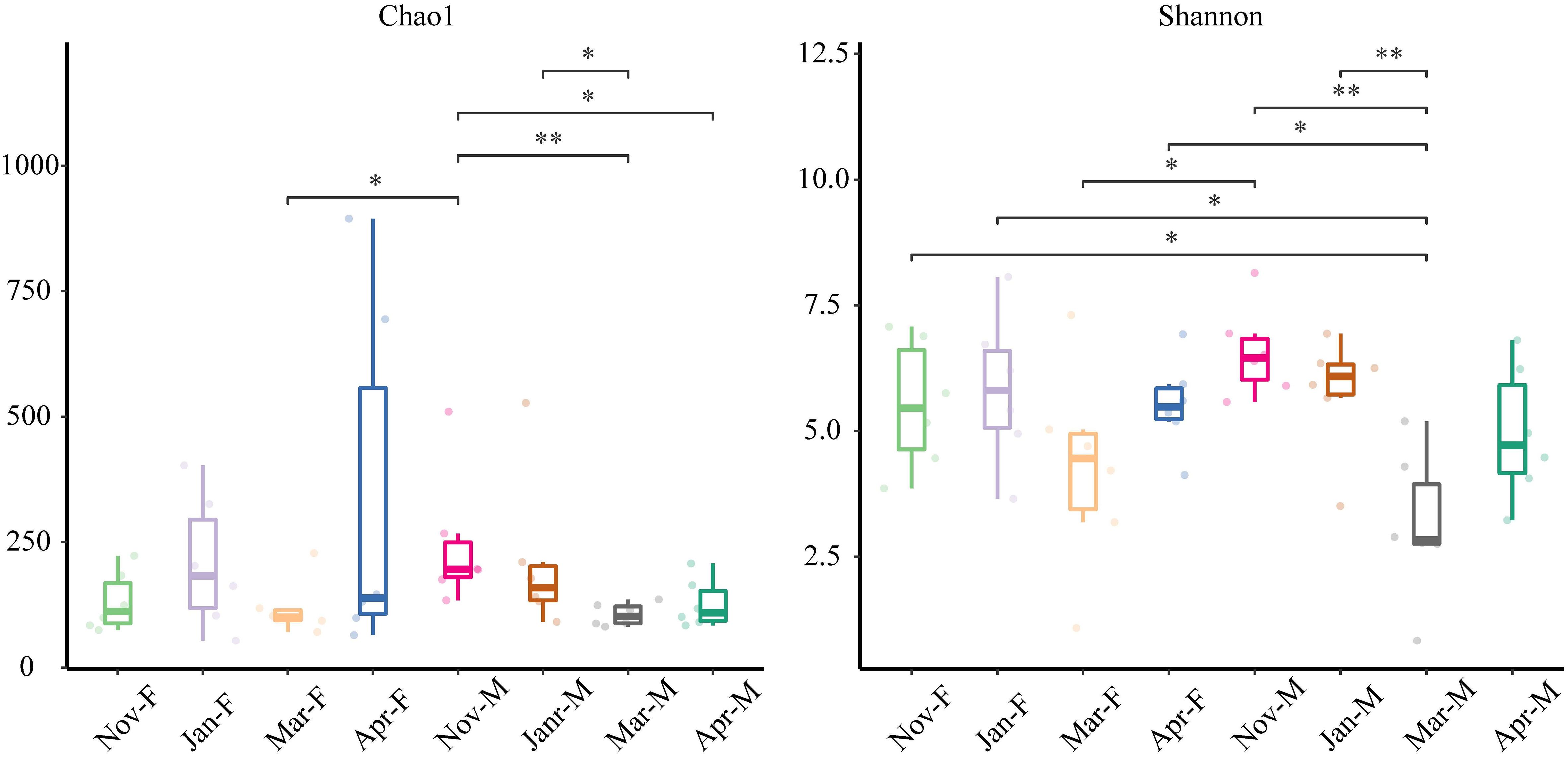
Figure 5 Chao1 and Shannon indices analysis in all groups. Data were expressed as mean ± S. E. M. * represented P < 0.05 between two groups; ** represented P < 0.01 between two groups. Nov, Jan, Mar and Apr represented November, January, March and April, respectively. M, males; F, females.
The β-diversity analysis was implemented by using PCoA based on Bray-Curtis distance (Supplementary Figure 3). The PCoA plot showed that most samples clustered closely together. Adonis analysis revealed no significant differences in β-diversity between Nov-M and Jan-M groups (F = 0.960, P = 0.688), and Jan-M and Mar-M groups (F = 1.158, P = 0.093). Similarly, no significant differences were observed between Nov-F and Jan-F groups (F = 1.005, P = 0.335), and Jan-F and Mar-F groups (F = 1.158, P = 0.092). A similar pattern of intestinal microbiota structure change was found in both male and female fish during the overwintering period. However, significant differences were observed in intestinal microbiota structure between males and females at the same time points. Notably, differences were evident in Nov-F vs Nov-M (F = 1.526, P = 0.002), Jan-F vs Jan-M (F = 1.252, P = 0.042), Mar-F vs Mar-M (F = 1.933, P = 0.004), and Apr-F vs Apr-M (F = 2.056, P = 0.004) (Supplementary Table 4).
3.6 Bacterial community composition and classification
Sequences from all groups, irrespective of gender, predominantly belonged to Bacteroidota, Proteobacteria, and Firmicutes (Figure 6A). Temporal changes in these three phyla were illustrated in Figure 6B. In the males, Bacteroidota and Firmicutes displayed similar trends in relative abundance, while Proteobacteria showed an opposite trend. In the females, the relative abundance of Bacteroidota decreased in Jan-F and Mar-F groups, but increased in Apr-F group. The relative abundance of Firmicutes and Proteobacteria increased in Jan-F and Mar-F groups but decreased in Apr-F group. Kruskal-Wallis rank-sum tests showed that there was no significant difference in the relative abundance of Firmicutes among Nov-M, Jan-M, Mar-M and Apr-M groups (P > 0.05). The relative abundance of Bacteroidota in the Mar-M group decreased significantly compared to the Jan-M group (P < 0.05). As compared to the Mar-M group, the relative abundance of Bacteroidota showed a significant increase in the Apr-M group (P < 0.05). No significant differences in the relative abundances of Bacteroidota and Proteobacteria were observed among the Nov-F, Jan-F, Mar-F and Apr-F groups (P > 0.05). The relative abundance of Bacteroidota in the Mar-F group was significantly higher than that in the Nov-F groups (P < 0.05). In addition, there were no significant differences in the relative abundances of Bacteroidota, Firmicutes and Proteobacteria between the male and female fish at the same time point (P > 0.05).
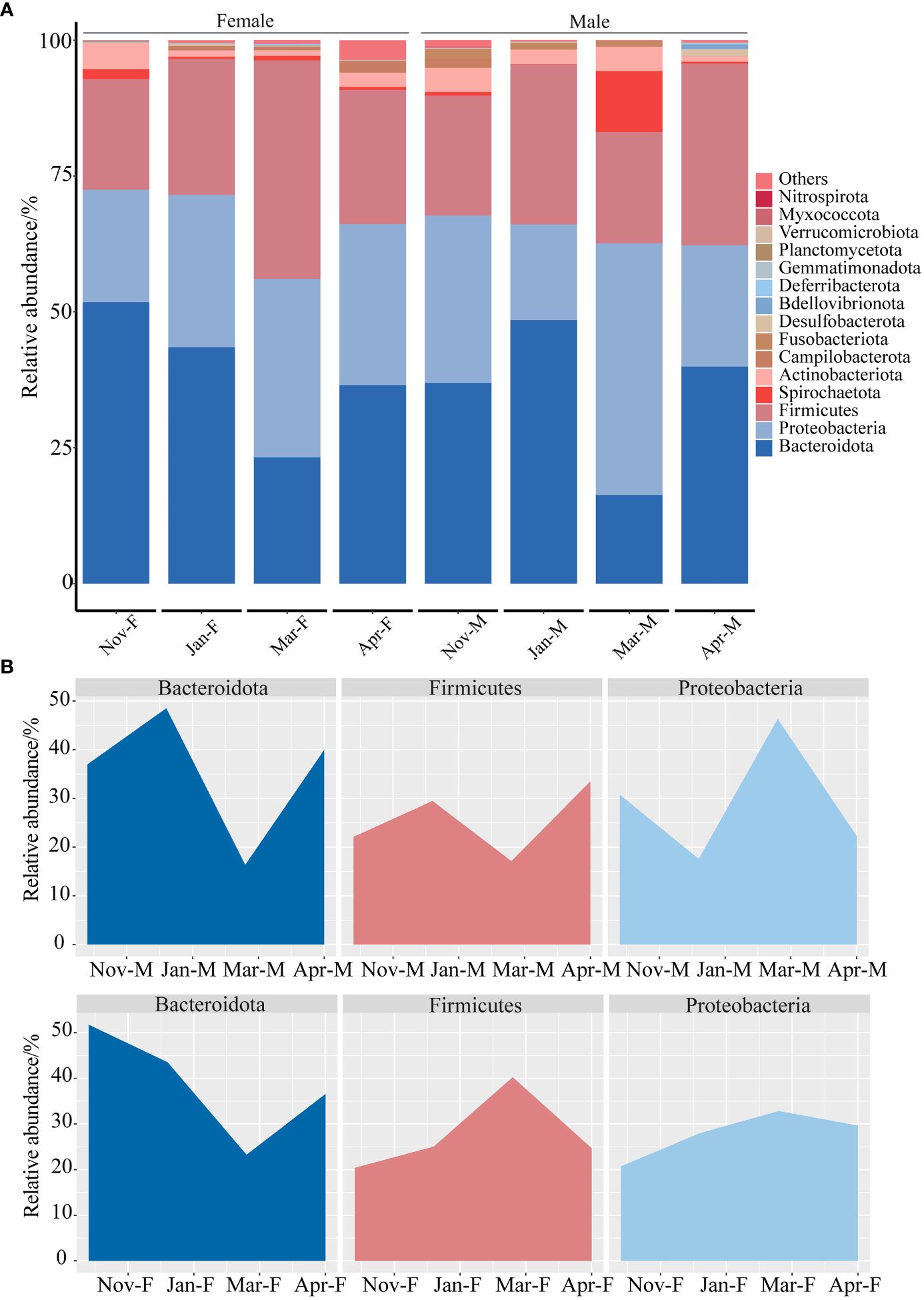
Figure 6 The composition of the intestinal microbiota. (A) Relative abundance of different phyla. (B) The abundance changes of dominant phyla in different groups. Nov, Jan, Mar and Apr represented November, January, March and April, respectively. M, males; F, females.
At genus level, as shown in the Supplementary Figure S4, the intestinal microbiota was dominated by norank_f_Muribaculaceae in the Nov-F, Jan-F, Mar-F, Apr-F, Jan-M and Apr-M groups. Acetobacter and Brevinema were the predominant genera in the Nov-M and Mar-M groups, respectively. In females, the relative abundance of norank_f_Muribaculaceae presented increase trend during overwintering period. However, there was no statistically significant difference in the relative abundance of norank_f_Muribaculaceae among the Nov-F, Jan-F, Mar-F, and Apr-F groups (P > 0.05). In the males, compared to the Nov-M, Jan-M and Apr-M groups, the relative abundance of Brevinema increased significantly (P < 0.05). There was no significant difference in the relative abundance of norank_f_Muribaculaceae among Nov-M, Jan-M, Mar-M and Apr-M groups (P > 0.05).
Most ASVs in all samples, ranging from 74.16% to 93.62%, were categorized as rare taxa (Supplementary Tables 5 and 6). Bacteroidota, Firmicutes, and Proteobacteria dominated the rare taxa in Nov-F, Jan-F, Mar-F, Apr-F, Nov-M, Jan-M, Mar-M, and Apr-M groups. Additionally, there was no significant difference in the rare taxa among Nov-F, Jan-F, Mar-F and Apr-F groups (P > 0.05). Similarly, there was no statistically significant differencein the proportion of rare taxa among the Nov-M, Jan-M, Mar-M, and Apr-M groups (P > 0.05).
3.7 Functional prediction of the intestinal microbiota
Functional prediction found that a total of 5532 functional genes were shared among the Nov-M, Jan-M, Mar-M and Apr-M groups (Figure 7A). The NMDS plot revealed clustering of functional genes from Nov-M, Jan-M, and Apr-M groups (Figure 7B). ANOSIM analysis showed significant differences between Nov-M and Mar-M groups (r = 0.664, P = 0.004), and between Mar-M and Apr-M groups (r = 0.267, P = 0.04). There was no significant difference between the Nov-M and Jan-M groups (r= 0.130, P = 0.135), between the Jan-M and Mar-M groups (r = 0.122, P = 0.092). A total of 5,807 shared ASVs were identified among Nov-F, Jan-F, Mar-F, and Apr-F groups (Figure 7C).
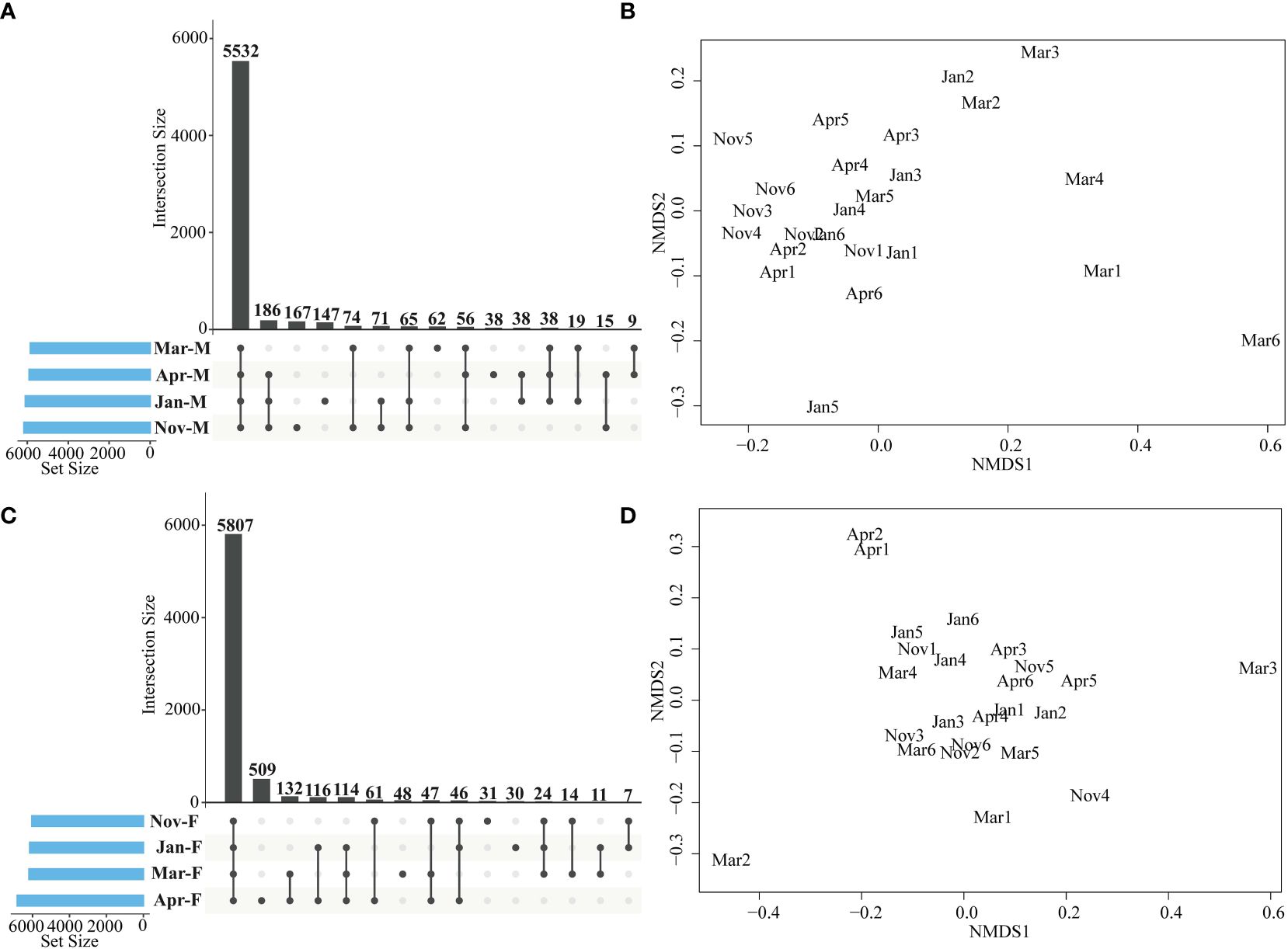
Figure 7 Functional genes analysis in the large yellow croaker. UpSet plot analysis of functional genes of intestinal microbiota in males (A) and females (C). Non-metric multi-dimensional scaling analysis of functional genes in males (B) and females (D). Nov, Jan, Mar and Apr represented November, January, March and April, respectively. M, males; F, females.
Functional genes from the intestinal microbiota of the Nov-F, Jan-F, Mar-F and Apr-F groups clustered together (Figure 7D). ANOSIM analysis revealed no significant differences between Nov-F and Jan-F groups (r = 0.065, P = 0.273), Jan-F and Mar-F groups (r = 0.021, P = 0.393), Mar-F and Apr-F groups (r = 0.556, P = 0.309), Nov-F and Mar-F groups (r = 0.219, P = 0.117), Nov-F and Apr-F groups (r = 0.032, P = 0.373). Most functional genes were clustered into categories like Cellular Processes, Environmental Information Processing, Genetic Information Processing, Human Diseases, Metabolism, and Organismal Systems (Supplementary Figure 5). Kruskal-Wallis tests revealed no significant differences in these six functional pathways among Nov-F, Jan-F, Mar-F, and Apr-F groups (P > 0.05). Significant differences were observed in Cellular Processes, Genetic Information Processing, and Organismal Systems among Nov-M, Jan-M, Mar-M, and Apr-M groups (P < 0.05). These results showed that the functional pathways of the intestinal microbiota in the males were more affected than those in the females during overwintering period.
4 Discussion
Winter, as a natural fasting period, incurred significant economic losses in fish aquaculture. Previous studies have demonstrated that fish activity and growth were minimized at temperatures below 13°C (Ibarz et al., 2010). Large yellow croaker was particularly sensitive to low temperatures. These fish refused food at temperatures below 10 °C (Yi et al., 1998). In this study, the body weight of the fish increased significantly in January, both in the male and female fish. The body weight of the male and female fish showed a downward trend from March until April. The surface of average temperatures in the seawater were 17.73°C in November, 15.90 °C in December, and 13.02 °C in January in the present study. Yi et al. revealed that large yellow croakers feed normally at temperatures above 14 °C (Yi et al., 1998). This likely explained the weight gain observed in the fish in January. In this study, we observed female fish experienced a significant decrease in body weight in March, while male fish showed no significant weight loss between January and March. This suggests that females experienced a more significant decrease in body weight compared to the males.
The preservation of the tight junction barrier in intestinal epithelium is paramount to the well-being of fish. Occludin serves as an indispensable functional and structural constituent of the tight junction (Feldman et al., 2005; Saito et al., 2021). The zo1, serving as the predominant constituent of the tight junction, possesses the capacity to modulate the permeability of this crucial cellular barrier (Fanning and Anderson, 2009; Kuo et al., 2021). The arp2/3 complex, a highly conserved protein, occupies a pivotal position in the regulation of the actin cytoskeleton. Its indispensable function lies in overseeing the morphology and mobility of cells (Liman et al., 2020). In the present study, the expression levels of zo1 and arp2/3 genes exhibited significant decrease in the Jan-M and Mar-M groups as compared to the Nov-M and Apr-M groups. Concurrently, the occludin gene expression level showed a conspicuous decrease in the Jan-M and Mar-M groups when compared to the Nov-M and Apr-M groups. Conversely, the expression levels of arp2/3, occludin, and zo1 genes demonstrated a discernible trend towards augmentation in the Jan-F and Mar-F groups as compared to the Nov-F group. This finding suggested potential structural damage to the tight junctions in the intestines of male fish during the overwintering period, while the tight junction barrier in the intestines of female fish appeared to have been better preserved.
The TLR-NF-κB signaling pathway serves as a crucial mediator of the host’s immune and inflammatory responses. Toll-like receptors (TLRs), as one of most important pattern recognition receptor families, could be activated by low temperature (Chen et al., 2021). Activation of this pathway by low temperature can lead to the generation of inflammatory cytokines (Raida and Buchmann, 2007; Wei et al., 2023a). In this study, TLR3 gene was only significantly up-regulated in the intestine of the male fish during overwintering period. However, in the intestine of the female fish, TLR1, TLR3, TLR7 and TLR9 genes were all significantly up-regulated. In the liver, these genes were significantly down-regulated in both males and females. Meanwhile, pro-inflammatory cytokines interleukin (IL)-1β and IL-6 were both significantly up-regulated. Raida and Buchmann (2007) also found IL-1β significantly up-regulated under low temperature conditions. In the intestines, IL-6 was significantly up-regulated while IL-10 was down-regulated in both males and females during the overwintering period. These findings suggested that immune-related genes exhibited different temperature dependencies in various organs. Additionally, immune-related genes in large yellow croaker displayed sexual dimorphism. These finding were similarly with previous studies that sexual dimorphism in immune function was observed in vertebrates, with females generally exhibiting stronger immune responses than males (Klein and Flanagan, 2016; Chakraborty et al., 2023).
Intestinal microbiota balance is crucial for maintaining host health. Cold exposure led to significant changes in intestinal microbiota composition (Chevalier et al., 2015; Wei et al., 2023b). In this study, ASVs showed significant changes during the overwintering period, irrespective of gender. During the overwintering period, the richness of intestinal microbiota in males was found to be more susceptible to change. Bacteroidota, Firmicutes, and Proteobacteria, being the predominant phyla, were widely present in teleosts (Kumari, 2020). Our study also verified that the majority of ASVs belonged to Bacteroidota, Firmicutes, and Proteobacteria, regardless of gender. In male fish, fluctuations in the relative abundances of Bacteroidota and Firmicutes were opposite to those of Proteobacteria. However, in female fish, the change in the relative abundance of Bacteroidota opposed that of Firmicutes and Proteobacteria. This indicated a differing response strategy between male and female fish. This finding was consistent with observations in a rat model, wherein the relative abundance of Proteobacteria increased under cold exposure, accompanied by a decrease in the relative abundance of Firmicutes (Chevalier et al., 2015).
Accumulating evidence indicated that Bacteroidota and Firmicutes, as the predominant phyla, were pivotal in maintaining the equilibrium of the intestinal microbiota (Sun et al., 2022). In female fish, the balance of Bacteroidota and Firmicutes in the intestinal microbiota remained dynamic. However, in male fish, the percentage of Bacteroidota and Firmicutes in the intestinal microbiota decreased significantly in March, signifying an imbalance in the male fish’s intestinal microbiota during this period.
Upon further analysis at the genus level, we found that the significant increase in Proteobacteria was attributed to a sharp rise in the number of Vibrio bacteria. Vibrio bacteria, as a significant pathogen of marine vertebrates, could potentially lead to mass mortality of fish (Austin and Zhang, 2006). Therefore, this might be a contributing factor to the widespread mortality of large yellow croaker during overwintering period.
In this study, there was a limited number of shared ASVs observed during the overwintering period in both males and females. Intriguingly, the majority of functional genes were shared during the overwintering period in both males and females. Additional KEGG analysis unveiled significant differences in Cellular Processes, Genetic Information Processing, and Organismal Systems in male fish during the overwintering period. Conversely, no statistical difference was observed in the functional pathways in female fish during the overwintering period. This observation suggested that the functional pathways of the intestinal microbiota in male fish were more readily influenced by environmental factors compared to those of female fish.
5 Conclusion
In the present study, we collected samples from the large yellow croaker during the overwintering period from Zhoushan coast, and analyzed the expression of intestinal barrier-related genes, immune responses, and changes in intestinal microbiota in the males and females, respectively. The findings indicated that females experienced a more significant decrease in body weight compared to the males. Analysis of intestinal barrier-related genes showed that the tight junction barrier in the male intestine was more susceptible than in females. Analysis of genes associated with TLR signaling pathway showed distinct expression patterns between males and females, with females exhibiting a higher level of immune competence. Further differences between males and females were revealed through the composition, structure, and function of their intestinal microbiota. Overall, large yellow croakers exhibit sex-specific differences in growth, immune responses, and intestinal microbiota during the overwintering period. These research findings contribute to a better understanding of the sexual dimorphism in large yellow croakers and provide important insights for monosex aquaculture management.
Data availability statement
The datasets presented in this study can be found in online repositories. The names of the repository/repositories and accession number(s) can be found below: https://www.ncbi.nlm.nih.gov/genbank/, PRJNA1053681.
Ethics statement
The animal study was approved by Zhejiang Ocean University’s Guidelines for the Care and Use of Laboratory Animals and received approval from the Animal Care and Use Ethics Committee of Zhejiang Marine Fisheries Institute. The study was conducted in accordance with the local legislation and institutional requirements.
Author contributions
HC: Conceptualization, Formal Analysis, Investigation, Methodology, Writing – original draft, Writing – review & editing. HL: Conceptualization, Formal Analysis, Methodology, Validation, Writing – original draft, Writing – review & editing. RC: Data curation, Investigation, Visualization, Writing – review & editing. WH: Conceptualization, Data curation, Visualization, Writing – review & editing. YY: Data curation, Methodology, Visualization, Writing – review & editing. WL: Data curation, Investigation, Visualization, Writing – review & editing. XY: Data curation, Investigation, Visualization, Writing – review & editing. BL: Data curation, Investigation, Visualization, Writing – review & editing. DX: Conceptualization, Formal Analysis, Funding acquisition, Methodology, Project administration, Supervision, Validation, Writing – review & editing.
Funding
The author(s) declare that financial support was received for the research, authorship, and/or publication of this article. This study was supported by the National Key Research and Development Program of China (No. 2022YFD2401003), General scientific research project of Education Department of Zhejiang Province (No. Y202147681), National Natural Science Foundation of China (No. 32202925), Grants from Zhoushan Fishery Breeding and Hatching Innovation Center (No. 2023001-1-1), Special Project of Zhoushan Fishery Breeding and Hatching Innovation Center (No. 2023Y001-1-2).
Conflict of interest
The authors declare that the research was conducted in the absence of any commercial or financial relationships that could be construed as a potential conflict of interest.
Publisher’s note
All claims expressed in this article are solely those of the authors and do not necessarily represent those of their affiliated organizations, or those of the publisher, the editors and the reviewers. Any product that may be evaluated in this article, or claim that may be made by its manufacturer, is not guaranteed or endorsed by the publisher.
Supplementary material
The Supplementary Material for this article can be found online at: https://www.frontiersin.org/articles/10.3389/fmars.2024.1391035/full#supplementary-material
References
Austin B., Zhang X. H. (2006). Vibrio harveyi: a significant pathogen of marine vertebrates and invertebrates. Lett. Appl. Microbiol. 43, 119–124. doi: 10.1111/j.1472-765X.2006.01989.x
Callahan B. J., Mcmurdie P. J., Rosen M. J., Han A. W., Holmes S. P. (2016). DADA2: High-resolution sample inference from Illumina amplicon data. Nat. Methods 13, 581–583. doi: 10.1038/nmeth.3869
Chakraborty B., Byemerwa J., Krebs T., Lim F., Chang C. Y., McDonnell D. P. (2023). Estrogen receptor signaling in the immune system. Endocrine Rev. 44, 117–141. doi: 10.1210/endrev/bnac017
Chen H., Liang Y., Han Y., Liu T., Chen S. (2021). Genome-wide analysis of Toll-like receptors in zebrafish and the effect of rearing temperature on the receptors in response to stimulated pathogen infection. J. Fish Dis. 44, 337–349. doi: 10.1111/jfd.13287
Chevalier C., Stojanović O., Colin D. J., Suarez-Zamorano N., Trajkovski M. (2015). Gut microbiota orchestrates energy homeostasis during cold. Cell 163, 1360–1374. doi: 10.1016/j.cell.2015.11.004
Cunjak R. A. (1988). Physiological consequences of overwintering in streams: the cost of acclimitization? Can. J. Fish. Aquat. Sci. 45, 443–452. doi: 10.1139/f88-053
Dai T., Zhang Y., Tang Y., Bai Y., Wen D. (2016). Identifying the key taxonomic categories that characterize microbial community diversity using full-scale classification: a case study of microbial communities in the sediments of Hangzhou Bay. FEMS Microbiol. Ecol. 92, fiw150. doi: 10.1093/femsec/fiw150
Dou D. R., Zhao Y., Belk J. A., Zhao Y., Casey K. M., Chen D. C., et al. (2024). Xist ribonucleoproteins promote female sex-biased autoimmunity. Cell 187, 733–749. doi: 10.1016/j.cell.2023.12.037
Dubois E. L., Horowitz R. E., Demopoulos H. B., Teplitz R. (1966). NZB/NZW mice as a model of systemic lupus erythematosus. JAMA 195, 285–289. doi: 10.1001/jama.1966.03100040091025
Faggion S., Vandeputte M., Vergnet A., Clota F., Blanc M. O., Sanchez P., et al. (2021). Sex dimorphism in European sea bass (Dicentrarchus labrax L.): New insights into sex-related growth patterns during very early life stages. PloS One 16, e0239791. doi: 10.1371/journal.pone.0239791
Fanning A. S., Anderson J. M. (2009). Zonula occludens-1 and-2 are cytosolic scaffolds that regulate the assembly of cellular junctions. Ann. N. Y. Acad. Sci. 1165, 113–120. doi: 10.1111/j.1749-6632.2009.04440.x
Feldman G. J., Mullin J. M., Ryan M. P. (2005). Occludin: structure, function and regulation. Adv. Drug Deliv. Rev. 57, 883–917. doi: 10.1016/j.addr.2005.01.009
Ibarz A., Padrós F., Gallardo M.Á., Fernández-Borràs J., Blasco J. (2010). Low-temperature challenges to gilthead sea bream culture: review of cold-induced alterations and ‘Winter Syndrome’. Rev. Fish Biol. Fish. 20, 539–556. doi: 10.1007/s11160-010-9159-5
Klein S. L., Flanagan K. L. (2016). Sex differences in immune responses. Nat. Rev. Immunol. 16, 626–638. doi: 10.1038/nri.2016.90
Kumari K. (2020). The fish gut microbiota, current approaches and perspectives: A review. J. Adv. Microbiol. Res. 1, 19–22. doi: 10.22271/micro
Kuo W. T., Zuo L., Odenwald M. A., Madha S., Singh G., Gurniak C. B., et al. (2021). The tight junction protein ZO-1 is dispensable for barrier function but critical for effective mucosal repair. Gastroenterology 161, 1924–1939. doi: 10.1053/j.gastro.2021.08.047
Li H., Lu L., Chen R., Li S., Xu D. (2022). Exploring sexual dimorphism in the intestinal microbiota of the yellow drum (Nibea albiflora, Sciaenidae). Front. Microbiol. 12. doi: 10.3389/fmicb.2021.808285
Liman J., Bueno C., Eliaz Y., Schafer N. P., Waxham M. N., Wolynes P. G., et al. (2020). The role of the Arp2/3 complex in shaping the dynamics and structures of branched actomyosin networks. PNAS 117, 10825–10831. doi: 10.1073/pnas.1922494117
Liu C., Zhao L. P., Shen Y. Q. (2021). A systematic review of advances in intestinal microflora of fish. Fish Physiol. Biochem. 1, 1–13. doi: 10.1007/s10695-021-01027-3
Livak K. J., Schmittgen T. D. (2001). Analysis of relative gene expression data using Real-Time Quantitative PCR and the 2–ΔΔCT method. Methods 25, 402–408. doi: 10.1006/meth.2001.1262
Luo Y. (2020). China fishery statistics yearbook published Guangdong’s total aquatic product output ranked first in China for the first time. Ocean Fishery 6, 12–13. Available at: https://www.cqvip.com/k/88802x/202006/7102533376.html.
Lv H., Liu Y., Li H., Yin X., Wang P., Qu X., et al. (2021). Modulation of antioxidant enzymes, heat shock protein, and intestinal microbiota of large yellow croaker (Larimichthys crocea) under acute cold stress. Front. Mar. Sci. 8. doi: 10.3389/fmars.2021.725899
Myers G. S. (1949). Usage of anadromous, catadromous and allied terms for migratory fishes. Copeia 1949, 89–97. doi: 10.2307/1438482
Raida M. K., Buchmann K. (2007). Temperature-dependent expression of immune-relevant genes in rainbow trout following Yersinia ruckeri vaccination. Dis. Aquat. Org. 77, 41–52. doi: 10.3354/dao01808
Saito A. C., Higashi T., Fukazawa Y., Otani T., Tauchi M., Higashi A. Y., et al. (2021). Occludin and tricellulin facilitate formation of anastomosing tight-junction strand network to improve barrier function. Mol. Biol. Cell 32, 722–738. doi: 10.1091/mbc.E20-07-0464
Sommer F., Bäckhed F. (2013). The gut microbiota—masters of host development and physiology. Nat. Rev. Microbiol. 11, 227–238. doi: 10.1038/nrmicro2974
Song H., Xu D., Tian L., Chen R., Wang L., Tan P., et al. (2019). Overwinter mortality in yellow drum (Nibea albiflora): Insights from growth and immune responses to cold and starvation stress. Fish Shellfish Immunol. 92, 341–347. doi: 10.1016/j.fsi.2019.06.030
Sun Y., Zhang S., Nie Q., He H., Tan H., Geng F., et al. (2022). Gut firmicutes: Relationship with dietary fiber and role in host homeostasis. Crit. Rev. Food Sci. Nutr. 1, 1–16. doi: 10.1080/10408398.2022.2131728
Tremaroli V., Bäckhed F. (2012). Functional interactions between the gut microbiota and host metabolism. Nature 489, 242–249. doi: 10.1038/nature11552
Wang Y. F., Zhang D. D., Shen W. L. (2016). Morphological changes of digestive tract and liver of Larimichthys crocea before and after over-wintering. J. Mar. Sci. Eng. 34, 63–71. doi: 10.3969/j.issn.1001-909X.2016.04.008
Wei H., Li T., Zhang Y., Liu X., Gong R., Bao J., et al. (2023a). Cold stimulation causes oxidative stress, inflammatory response and apoptosis in broiler heart via regulating Nrf2/HO-1 and NF-κB pathway. J. Thermal Biol. 116, 103658. doi: 10.1016/j.jtherbio.2023.103658
Wei W., Zhang G., Zhang Y., Zhang L., Wu S., Li X., et al. (2023b). Research progress on adaptive modifications of the gut microflora and regulation of host glucose and lipid metabolism by cold stimulation. Frigid Zone Med. 3, 13–21. doi: 10.2478/fzm-2023-0003
Keywords: overwintering period, immune responses, intestinal microbiota, sex difference, large yellow croaker
Citation: Cen H, Li H, Chen R, Hu W, Yang Y, Li W, Yin X, Liu B and Xu D (2024) Exploring the sex dimorphism in the expression of intestinal barrier and immune-related genes and intestinal microbiota in cage-cultured large yellow croaker (Larimichthys crocea) during the overwintering period along the Zhoushan coast. Front. Mar. Sci. 11:1391035. doi: 10.3389/fmars.2024.1391035
Received: 24 February 2024; Accepted: 22 March 2024;
Published: 04 April 2024.
Edited by:
Yafei Duan, South China Sea Fisheries Research Institute, ChinaReviewed by:
Guodong Wang, Qingdao Agricultural University, ChinaAiguo Zhou, South China Agricultural University, China
Copyright © 2024 Cen, Li, Chen, Hu, Yang, Li, Yin, Liu and Xu. This is an open-access article distributed under the terms of the Creative Commons Attribution License (CC BY). The use, distribution or reproduction in other forums is permitted, provided the original author(s) and the copyright owner(s) are credited and that the original publication in this journal is cited, in accordance with accepted academic practice. No use, distribution or reproduction is permitted which does not comply with these terms.
*Correspondence: Dongdong Xu, xudong0580@163.com
†These authors have contributed equally to this work