- 1Department of Botany and Zoology, Stellenbosch University, Stellenbosch, South Africa
- 2Department for Biodiversity and Conservation Biology, University of the Western Cape, Bellville, South Africa
Mangroves are expanding polewards due to global change, often encroaching into adjacent temperate saltmarshes. In both vegetated ecosystems, brachyurans are responsible for ecological processes and functions such as nutrient cycling and sediment bioturbation. South African mangroves occur at a latitudinal limit and are establishing further south due to past planting events and global change, making these ideal study systems for the effects of mangrove expansion and encroachment. Here, we investigated the effect of mangrove encroachment on brachyuran community composition at two saltmarsh sites with planted mangrove stands of different ages. Transects were laid perpendicular to each estuary where three habitat types were demarcated (mangrove, ecotone, saltmarsh). Sediment samples were collected for analyses and quadrats were used to measure pneumatophore density, saltmarsh cover, and brachyuran abundance and diversity. We found that brachyuran community structure at each site has significantly changed over seven years, with two mangrove-associated fiddler crab species, Tubuca urvillei and Paraleptuca chlorophthalmus, now recorded at the younger planted site, indicating a new southern distributional limit. Community structure was also significantly different amongst habitat types (p < 0.05) with Parasesarma catenatum dominating saltmarshes while Danielella edwardsii was more prominent in mangroves. However, community composition did not differ significantly between the two (differently aged) sites (p > 0.05). Pneumatophore density had a proportional relationship with crab abundance, diversity and richness, while saltmarsh cover had an inversely proportional relationship with crab abundance, diversity and richness. It is likely that as mangroves continue to expand into saltmarshes, more mangrove-associated species will move into saltmarshes, potentially altering ecosystem processes in this unique habitat.
1 Introduction
Globally, many faunal species are expanding or shifting their geographic range in response to climate change (Perry et al., 2005; Chen et al., 2011; Cannizzo et al., 2018, Loveless and Smee, 2019, Peer et al., 2018a; Pinsky et al., 2020; Adams et al., 2024). These faunal shifts are usually associated with shifts in foundation species, such as coral reefs, kelp forests, and terrestrial forests, responsible for structuring and shaping ecosystems (Walther, 2010; Cannizzo and Griffen, 2019). Species are expected to move polewards at a rate of 13 km per decade due to rising temperatures (Poloczanska et al., 2013). However, some faunal species move and expand into novel habitats at a faster rate than foundation species (Schweiger et al., 2008). This difference in the shifting rate between foundation species and their associated fauna may result in a species inhabiting a new ecosystem that it has not previously occupied (Schweiger et al., 2008) and could lead to cascading effects on both community structure and ecosystem function (Cannizzo et al., 2018; Muthukrishnan et al., 2020; Hensel et al., 2023). This range shift of both foundation and associated faunal species is expected to increase with ongoing climate change (Schweiger et al., 2008; Cannizzo et al., 2019). For example, mangrove encroachment into saltmarsh habitats can cause changes in both ecosystem-level processes and functions (Kelleway et al., 2017; Cavanaugh et al., 2019). This change in function is likely to affect the community structure of fish, invertebrates and other species inhabiting coastal vegetated ecosystems (Kelleway et al., 2017; Smee et al., 2017; Cavanaugh et al., 2019).
Mangrove-saltmarsh ecotones exist near mangrove latitudinal range limits across the world, in countries such as China (Liu et al., 2020), New Zealand (Lovelock et al., 2007), Australia (Eslami-Andargoli et al., 2009), Mexico (Comeaux et al., 2012), the United States (Osland et al., 2013) and South Africa (Adams et al., 2004; Raw et al., 2019). In South Africa, saltmarshes occur along the entire coastline (Adams, 2020), while mangroves occur along the east coast extending from Kosi Bay to Tyolomnqa (Raw et al., 2022). Mangroves are expanding their distribution to higher latitudes, due to the warming of estuarine and coastal waters and rising sea levels assisting propagule dispersion (Godoy and Lacerda, 2015; Raw et al., 2022). Where mangroves and saltmarsh habitats co-occur, mangroves have been encroaching into saltmarsh habitats, creating a mangrove-saltmarsh ecotone (Saintilan et al., 2014; Geldenhuys et al., 2016; Cavanaugh et al., 2019). Many mangrove-associated species reach their southernmost distributional range limit in South Africa (Peer et al., 2015). Ecotones occurring at these species range limits are sensitive to changes, making South African mangrove-saltmarsh ecotones ideal to study and predict future responses of macrofauna to global climate change (Saintilan et al., 2014; Raw et al., 2019).
Brachyurans (true crabs) tend to be the most dominant macrofauna, in terms of abundance and diversity, in mangroves and saltmarshes (Emmerson, 1994; Gutierrez, 2008; Vorsatz, 2009). The most abundant brachyuran superfamilies occurring in these two ecosystems are Ocypodoidea (mainly fiddler crabs) and Grapsoidea which are burrowing species (Nobbs, 2003). In mangroves, sesarmids and other Grapsoidae families can alter mangrove forest structure (Dahdouh-Guebas et al., 1997), facilitate nutrient recycling (Gao et al., 2024) and energy flow (Lee, 1998), while fiddler crabs (family: Ocypodidae) can alter both soil dynamics (Warren and Underwood, 1986) and primary productivity (Ólafsson and Ndaro, 1997). The herbivorous and detritivorous diets of these species are essential for mangrove ecosystem functioning, as these crabs consume mangrove leaf litter and organic materials on the mangrove floor (Dahdouh-Guebas et al., 1997). Both these groups consist of burrowing species, which aerate and oxygenate mangrove soil (Steinke et al., 1993), allowing movement of nutrients and water through soil influencing mangrove seedling development (Steinke et al., 1993) and altering physical structures in saltmarshes (Bertness, 1985; Taylor and Allanson, 1993).
Since the foundation species themselves (mangrove and saltmarsh vegetation) can influence crab assemblages, as mangroves move into saltmarshes, it is likely that their associated macrofauna, will also shift into this novel habitat (Cannizzo et al., 2018). While mangroves are characterized as woody shrubs and trees that grow in saline coastal habitats (Giri et al., 2011; Adams & Rajkaran, 2021), saltmarshes are characterized by a variety of halophytic shrubs, grasses and herbs (Adams, 2020). The mangroves provide a shaded habitat through canopy cover which allows crabs to maintain moderate internal temperatures and experience less evaporative losses compared to crabs in open clearings such as saltmarshes (Edney, 1961; Hogarth, 2015; Nobbs and Blamires, 2017). Mangroves also have dense pneumatophore mats which may influence the crab presence and abundance either positively through food availability, shelter or soil composition (Demopoulos and Smith, 2010) or negatively by acting as an obstruction for both feeding and burrowing (Sweetman et al., 2010; Leung, 2015; Freitas and Pagliosa, 2020). While in the saltmarshes, certain saltmarsh species are affiliated with mangrove-associated crabs (Luk and Zajac, 2013). For example, Bertness and Miller (1984) found that the fiddler crab Minuca pugnax uses the saltmarsh Spartina alterniflora (L.) in Barrington Rhode Island saltmarsh habitats to gain structural support for their burrows. However, a subsequent study found no significant evidence to support the idea that saltmarsh species support burrowing crabs (Nomann and Pennings, 1998). In South Africa, two species of fiddler crabs Tubuca urvillei and Austruca occidentalis were found in saltmarshes in the warm temperate Kariega Estuary, well beyond the mangrove latitudinal distribution limit, with the former being a dominant species at this habitat (Hodgson, 1987; Peer et al., 2016). Therefore, it is highly likely that the presence or absence of certain vegetation types (mangroves, saltmarshes or both) would influence the distribution of these burrowing species (Nobbs, 2003). Understanding the interaction between macrofauna and vegetation types is essential to understanding the ecological implications, especially with the expansion of mangroves into saltmarsh habitats amidst global climate change.
Although several studies have focused on mangrove encroachment into saltmarshes in South Africa (Adams et al., 2004; Saintilan et al., 2014; Hoppe-Speer et al., 2015; Adams et al., 2016; Geldenhuys et al., 2016; Whitfield et al., 2016, Raw et al., 2019; Adams and Rajkaran, 2021; Raw et al., 2023), very few studies focused on the impact of mangrove encroachment on associated macrofauna (Vorsatz, 2009; Whitfield et al., 2016). South African mangroves provide insight into the climatic effect of mangrove expansion into saltmarshes (Adams and Rajkaran, 2021). The aim of this study is to understand the impact of mangrove encroachment into saltmarsh habitats on associated macrofauna. The objectives are therefore to, (i) record brachyuran abundance and diversity across the mangrove-saltmarsh gradient, (ii) compare brachyuran community structure over time and across two planted mangrove sites of different ages, and (iii) investigate the relationship between crab diversity, abundance and richness and vegetation structures. It was hypothesized that:
(i) Brachyuran community composition in mangroves would have changed over the last seven years when comparing data collected in 2016 (past data from Peer et al., 2018a) and 2023 (present).
(ii) There are more mangrove-associated species occurring at the older, more established mangrove site (Nahoon) compared to the younger mangrove site (Tyolomnqa).
(iii) Brachyuran community composition are different amongst all habitat types (mangrove, ecotone, saltmarsh).
(iv) Brachyuran abundance and diversity decreases with increasing saltmarsh cover and increases with increasing pneumatophore density.
2 Methods and materials
2.1 Study sites
The range expansion of mangrove forests further south along the South African east coast has been confounded by the planting of mangroves at two sites i.e. Nahoon and Tyolomnqa (Figures 1, 2A, B). Although some speculation exists as to the motivation for planting, it is known that the mangroves were planted by botanists to determine whether or not they would establish at higher latitudes, similar to areas around the world (Hoppe-Speer et al., 2015). The two planted sites were predominantly saltmarsh habitats prior to mangrove planting (Adams and Rajkaran, 2021). In Nahoon Estuary, A. marina was first planted in 1969 by Prof. Steinke (Steinke, 1972), followed by Bruguiera gymnorrhiza (L.) Lam. and Rhizophora mucronata Lam. planted by Carl Vernon a few years after (Hoppe-Speer et al., 2015). All three mangrove species are now well established at this site. In Tyolomnqa, A. marina was first planted in 1991 and has established in pockets along the estuary. The mangrove forest in Nahoon is relatively small, occupying less than 2 ha (Hoppe-Speer et al., 2015), while Tyolomnqa is less than 0.12 ha (Bolosha, 2016). Nahoon is a predominantly open estuary, while Tyolomnqa is a large temporarily closed estuary (Adams and Rajkaran, 2021), in which the warm Agulhas Current flows through. The rise in sea level and the flow of the Agulhas Current move mangrove propagules in a poleward direction, resulting in propagules being dispersed further south from these two planted sites, expanding latitudinally (Steinke and Ward, 2003; Raw et al., 2023). These two Eastern Cape sites were chosen as mangroves and saltmarshes co-exist here (Geldenhuys et al., 2016, Figure 2A, B) and to represent mangrove stands of different ages. Sites were sampled in summer (February) and winter (August) of 2023.
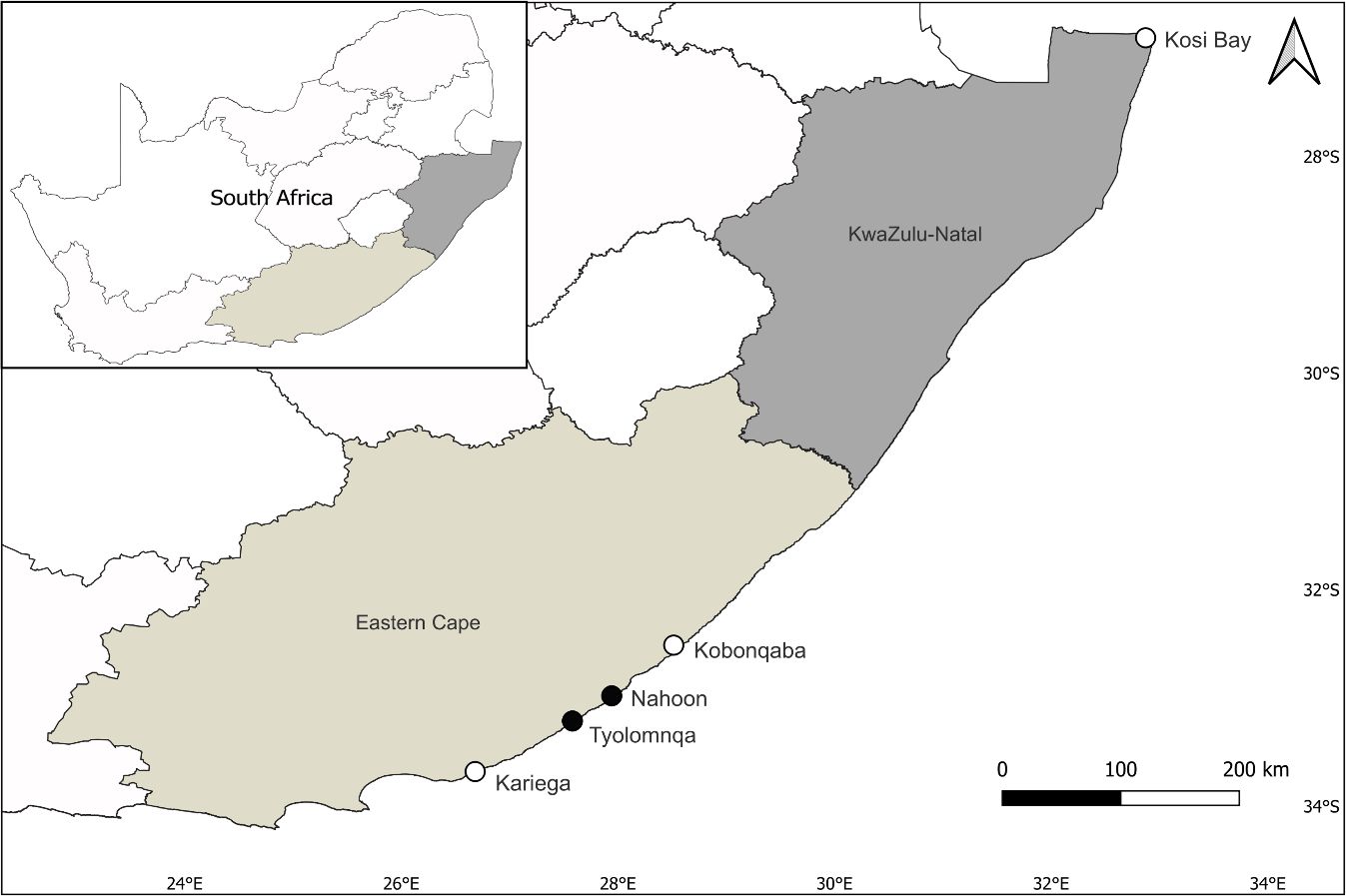
Figure 1 Map of South Africa, showing the two planted sites, Nahoon and Tyolomnqa, sampled in the Eastern Cape Province (represented by black dots) and other sites mentioned in this study (represented by white dots).
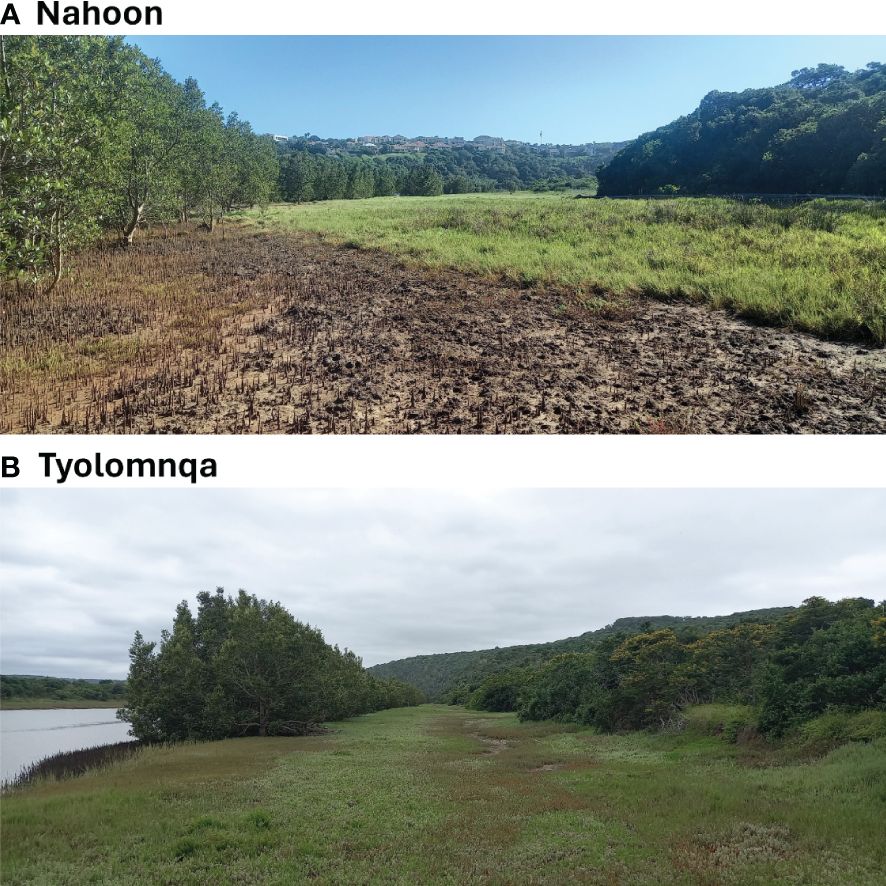
Figure 2 The two planted mangrove sites (A) Nahoon and (B) Tyolomnqa, showing each habitat type (mangrove, ecotone and saltmarsh). Mangroves occur on the left of the image, the ecotone in the middle and saltmarsh on the right. Images credited to Anusha Rajkaran.
2.2 Field work and laboratory analysis
Two 20 m transects were placed perpendicular to the flow of the estuary. Along each transect, three 4 x 4 m quadrats were laid at each habitat type (mangrove, ecotone, saltmarsh) at 2 m, 10 m, and 16 m (Figure 3). Within each 4 x 4 m quadrat, four 50 x 50 cm sub-quadrats were randomly placed where the density of pneumatophores, abundance and diversity of brachyurans, and percentage cover of each saltmarsh species was recorded. Individual trees and saplings were counted and height was measured using a tape measurer at each of the three 4 x 4 m quadrats at each habitat type. To determine sediment grain size (SGS), sediment moisture content (SMC %) and sediment organic matter (SOM %), three sediment samples (approximately 100 g each) were collected from each 4 x 4 m quadrat from each habitat type at both sites. Analyses of SGS, SMC and SOM were conducted in the laboratory following the protocol outlined in Adams and Human (2016). All data were recorded during low tide.
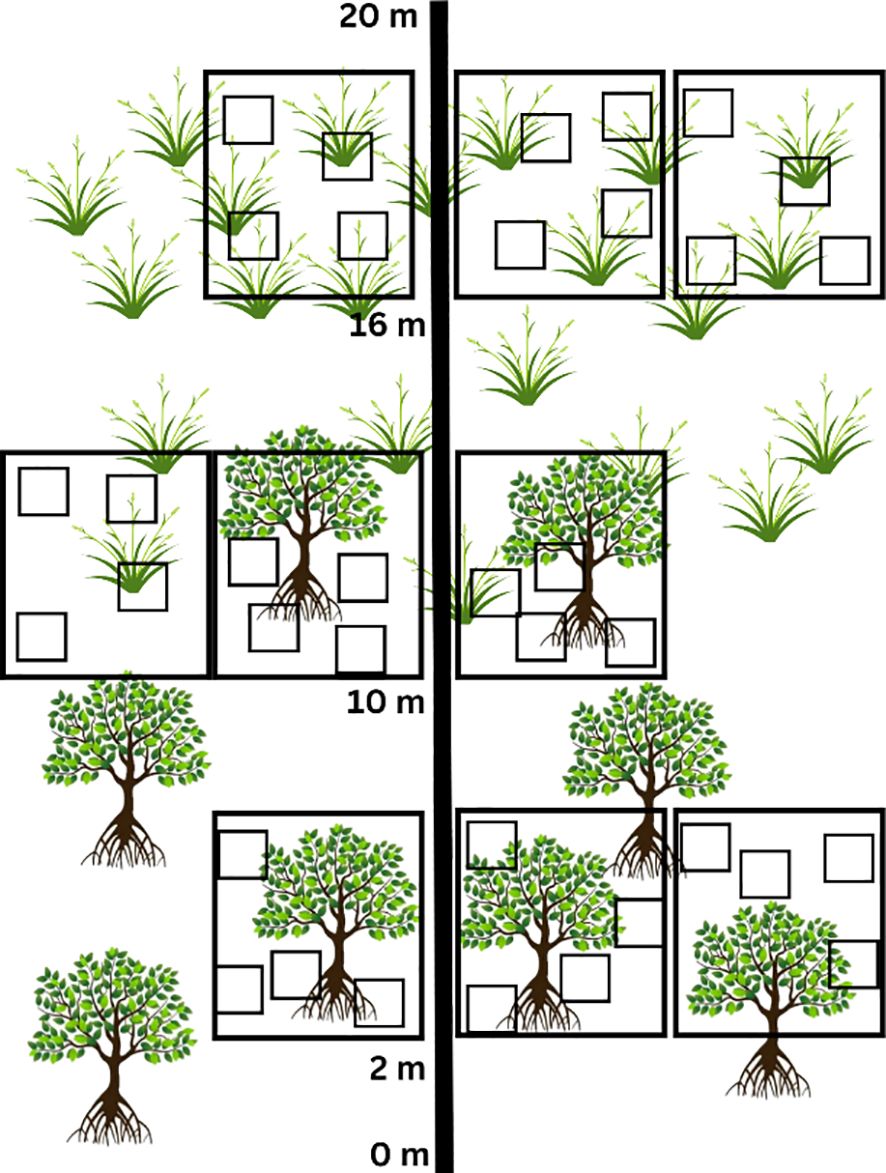
Figure 3 Depiction of sample design (Mitchell, 2010; Theron et al., 2022) for understanding mangrove encroachment into saltmarsh habitat. Two 20 m transects cut across saltmarsh habitats into mangrove habitats, where four 50 x 50 cm sub-quadrats were randomly placed within each of three 4 x 4 m quadrats.
2.3 Statistical analysis
All statistical analyses were conducted using the statistical package R v 4.3.0 (R Core Team 2024) and PRIMER (Clarke and Gorley, 2006). Multivariate abundance data were square root transformed using the Bray-Curtis similarity index. A resemblance matrix was generated from the transformed data. The effects of site (Nahoon, Tyolomnqa), habitat type (mangrove, ecotone, saltmarsh), year (2016, 2023) and season (Feb, Aug) on brachyuran community composition were analyzed using a Permutational multivariate analysis of variance (PERMANOVA). Where significant differences were found amongst habitat types and years, a pairwise analysis was performed.
To determine if saltmarsh cover, pneumatophore density and mean burrow abundance were significantly different amongst habitat types, an analysis of variance (ANOVA) was performed for each with habitat and site as factors. To determine SGS, a ranking system was used according to the percentage composition with 1 = clay, 2 = clay/silt, 3 = silt, 4 = silt/sand and 5 = sand following protocol outlined in Peer et al. (2018b). Thereafter, an ANOVA was performed to determine if SGS, SMC and SOM significantly differed between site and habitat type nested within site. Where significant differences were found, a posthoc Tukey’s HSD was performed. To determine the species contributions (similarities and dissimilarities) amongst sites and habitat types, a SIMPER analysis was performed on PRIMER using transformed data with a Bray Curtis similarity and a cut off low of 90%.
The species diversity (Shannon-Wiener diversity index), richness (total number of species) and evenness (Pielou’s evenness) of brachyurans at each habitat type was calculated. To determine if pneumatophore density and saltmarsh cover drive brachyuran abundance, diversity and richness among sites and habitat types a ‘manyglm’ was performed. Thereafter, an ‘anova’ (p.uni = ‘adjusted’, nBoot = 1000) was used to determine the significance of predictors for overall abundance, diversity and richness of crabs, which included univariate tests nested within the multivariate model for each response variable (Wang et al., 2012; Peer et al., 2018b; Theron et al., 2022). For all analyses, the significance value was determined by α =0.05.
3 Results
3.1 Brachyuran community structure over time
There was a significant change in brachyuran community structure in mangroves over the time (2016 vs 2023 data), at both Nahoon (p = 0.001, t = 2.83) and Tyolomnqa (p = 0.002, t = 2.34). Overall, 11 species of brachyurans were identified in both the planted mangroves (Table 1). The species richness decreased at Nahoon over the seven years from nine (2016) to seven (2023), while at Tyolomnqa species richness increased from four (2016) to five (2023). The crabs Austruca occidentalis, Tubuca urvillei, Paraleptuca chlorophthalmus and Metograpsus messor were observed in Nahoon (2016), however absent from this site in 2023.In Tyolomnqa the fiddler crabs T. urvillei and P. chlorophthalmus were absent in 2016 but were recorded for the first time at this site in 2023, explaining the change in species richness over time at both sites. The most abundant species at both sites (2016 and 2023) was Danielella edwardsii (Table 1) and the most common was also Cyclograpsus punctatus and Parasersarma catenatum. Four species, Dotilla fenestrata, Neosarmatium africanum, C. eulimene and P. capensis were absent from Tyolomnqa (2023) and only present in Nahoon, with three of these species (D. fenestrata, N. africanum, and P. capensis) all considered as mangrove-associated species. Cristarma eulimene and Parasesarma capensis occurred only at Nahoon (2023) at low average densities of one and four individuals per square meter, respectively.
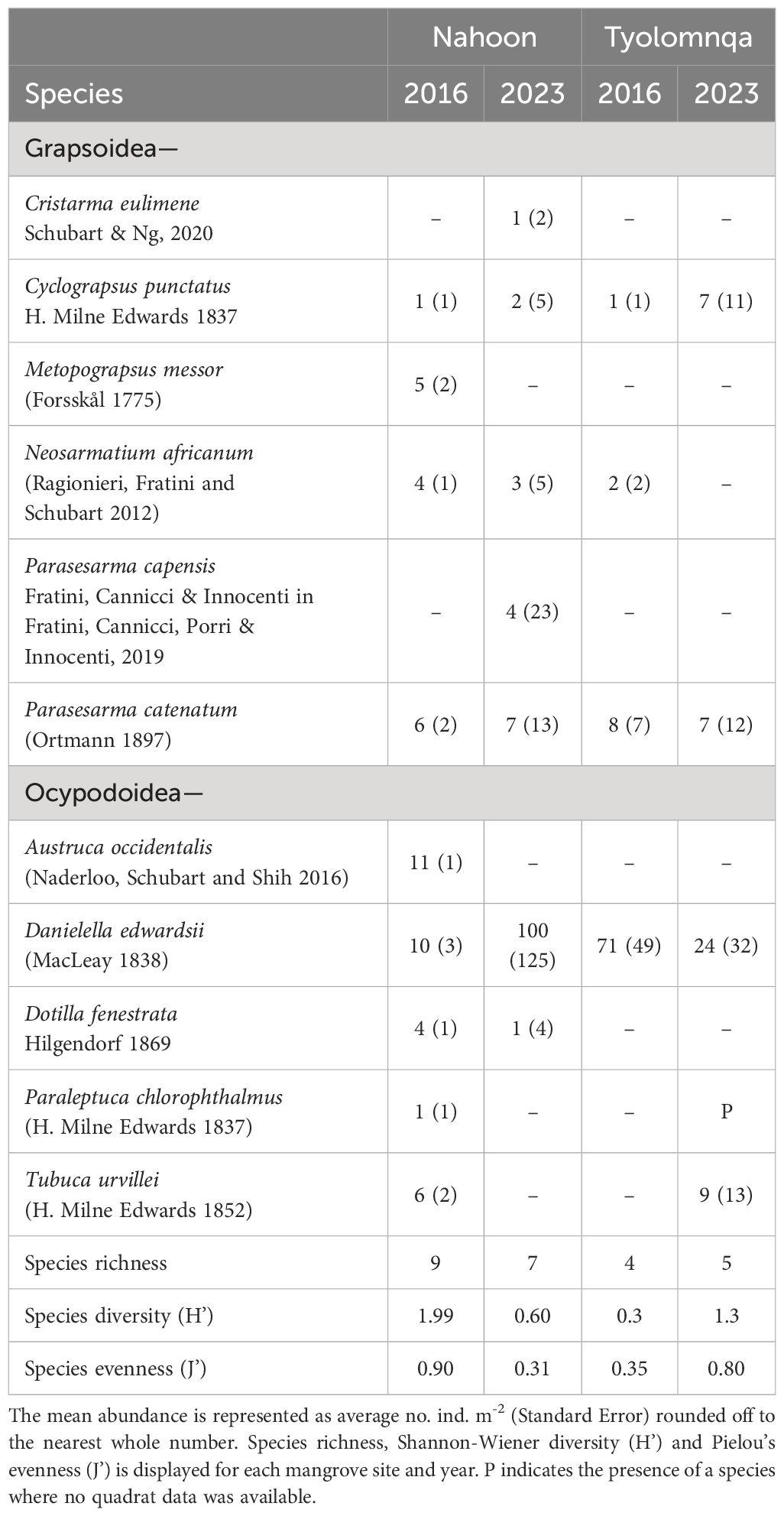
Table 1 The mean abundance of brachyuran species in two planted mangrove estuaries, Nahoon and Tyolomnqa, in Eastern Cape, South Africa, comparing past (year 2016 - Peer et al., 2018a) and present (2023) data.
3.2 Brachyuran community structure between older and newer aged mangrove forests
Community structure was similar between older (Nahoon) and newer (Tyolomnqa) aged planted mangroves, with no significant difference found (Table 2). However, the effect of habitat type (nested within site) and the interaction between habitat type and season had a significant influence on brachyuran community structure (Table 2). Other factors that had a significant influence on brachyuran assemblages were season within habitat types.
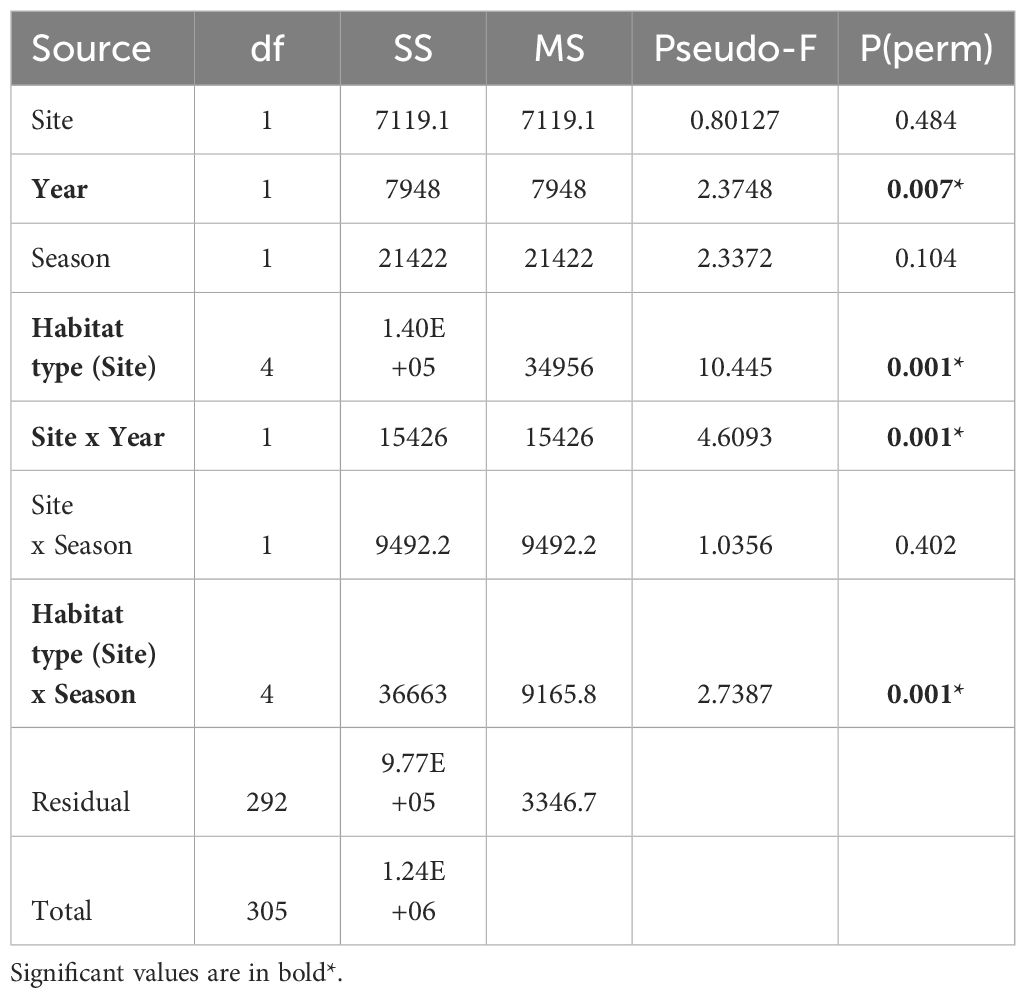
Table 2 Results of PERMANOVA test for differences in brachyuran community structure between sites (fixed), habitat types (nested in site, fixed), season (fixed) and year (random).
3.3 Brachyuran community structure amongst habitat types
Species richness generally decreased from the mangroves to the saltmarsh habitats (Table 3). A few key crabs are shown in Figures 4A–F). Parasesarma catenatum was the only species to occur at all three habitat types at both sites. Whereas Dotilla fenestrata only occurred at the ecotone, and Cristarma eulimene and Parasesarma capensis only occurred at the mangroves in Nahoon. The species Cyclograpsus punctatus, Daniellela edwardssi, P. catenatum and Tubuca urvillei occurred at both the mangrove and ecotone habitats in Tyolomnqa. The only species that occurred in saltmarsh habitats were Neosarmatium africanum (Nahoon), D. edwardsii (Nahoon) and P. catenatum (both sites).
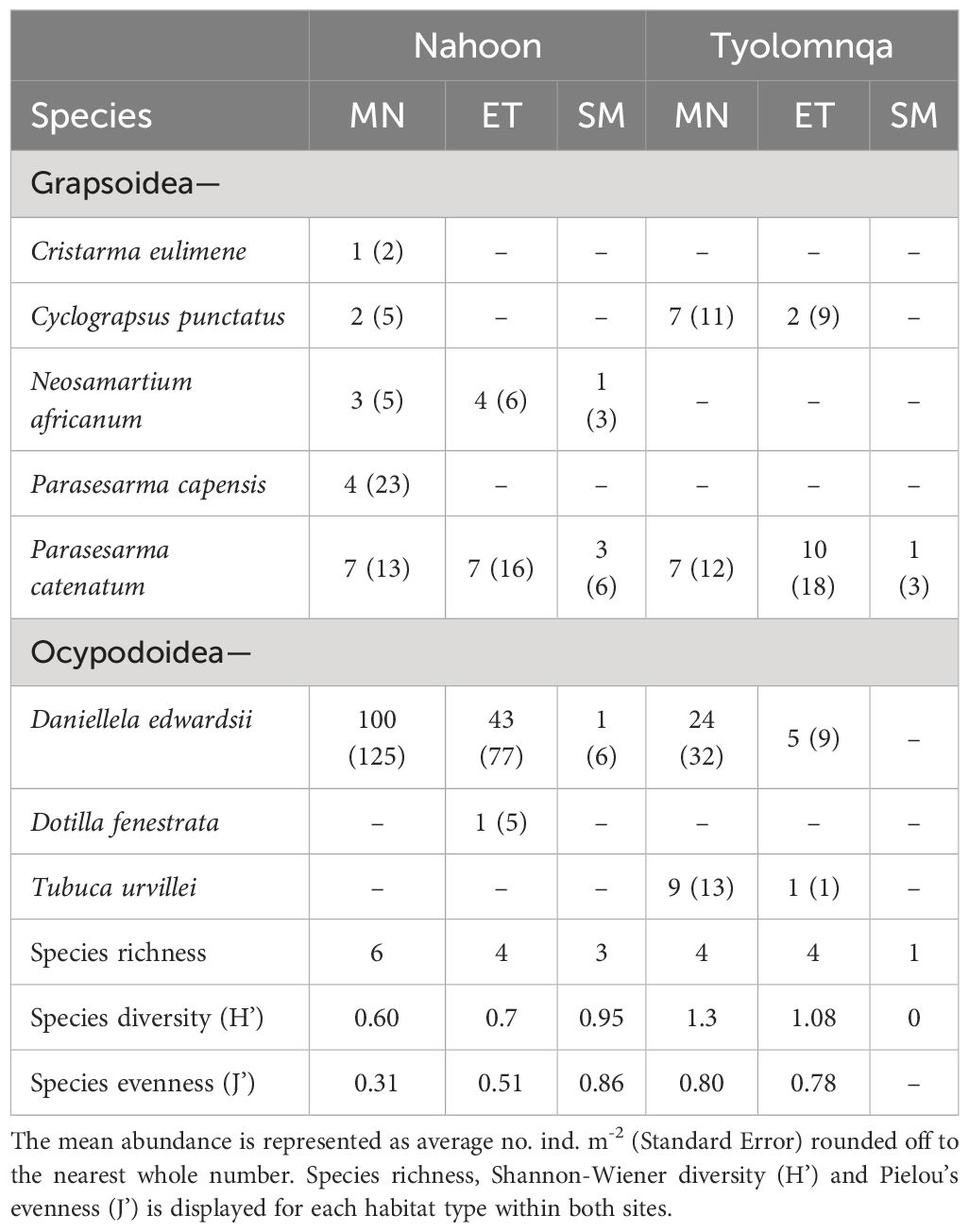
Table 3 Mean abundance of brachyuran species occurring at each of three habitat types (mangrove - MN, ecotone - ET, saltmarsh - SM) at both Nahoon and Tyolomnqa (for 2023), over a summer and winter sampling period.
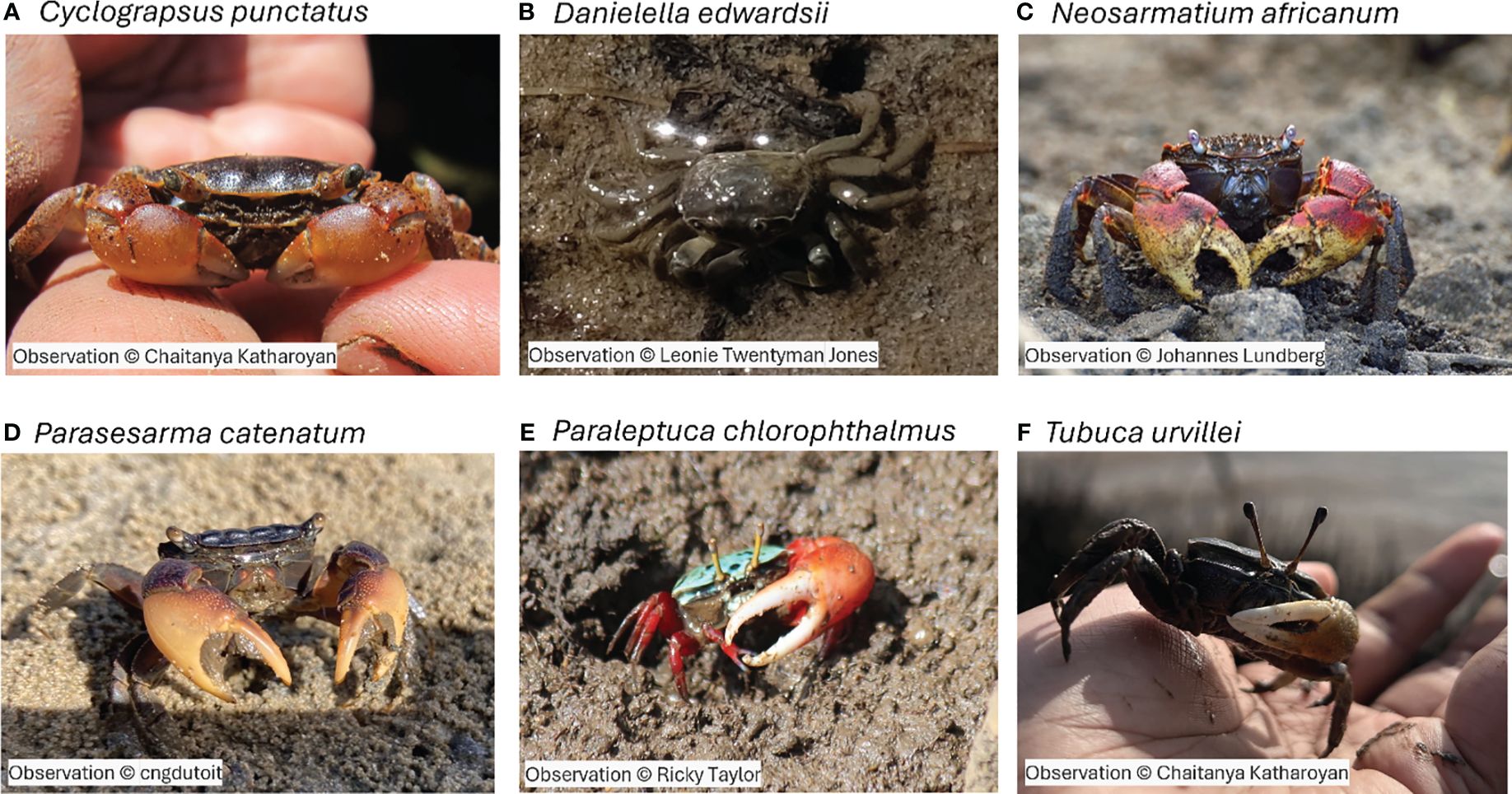
Figure 4 A few key crabs (A–F) that can be found at one or both planted sites (Nahoon and Tyolomnqa) amongst habitat types (mangrove, ecotone, saltmarsh). All photos are listed on iNaturalist.
A pairwise comparison among three habitat types revealed that there is a significant difference in brachyuran assemblages between all habitat groups (Saltmarsh vs Ecotone, Saltmarsh vs Mangrove and Ecotone vs Mangrove) at both Nahoon and Tyolomnqa (Table 4). Habitat types also differed in sediment and vegetation characteristics that are presented in this section and Section 3.4.
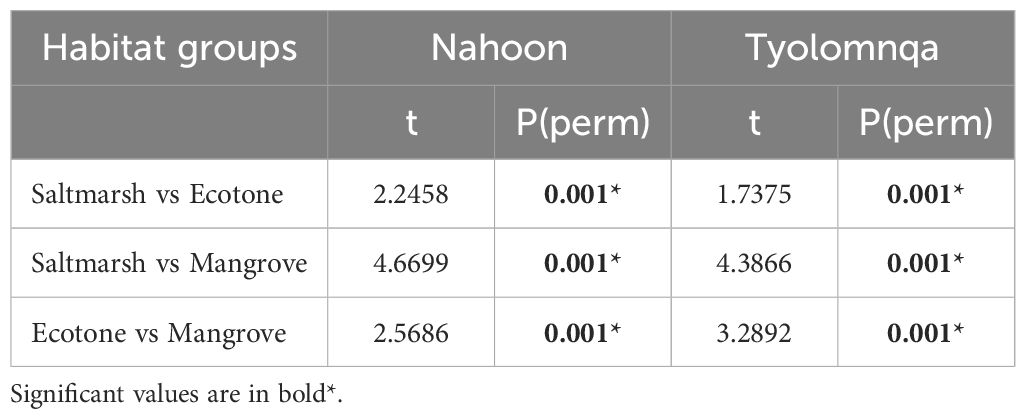
Table 4 Pairwise comparison of brachyuran community structure among three habitat types (mangrove, ecotone, saltmarsh) at both study sites, Nahoon and Tyolomnqa, South Africa.
There was an average dissimilarity in the abundance of crabs of 93.29% between saltmarshes and ecotones, 94.06% between saltmarshes and mangroves and 78.6% between mangroves and ecotones (Table 5). The crabs Daniella edwardsii and Parasesarma catenatum contributed the most towards the dissimilarities seen between all habitat types, Saltmarsh vs Ecotone, Saltmarsh vs Mangrove and Mangrove vs Ecotone (Table 5). Daniellela edwardsii is more common and abundant in mangrove habitats compared to other habitat types (Table 4). Whereas Parasesarma catenatum occured at all habitat types, generally having the highest abundance in mangroves and lowest in saltmarshes, except for in Tyolomnqa where the ecotone has the highest abundance per square meter (Tables 4, 5). Mangroves had a higher average abundance of crab species followed by ecotone and saltmarsh groups.
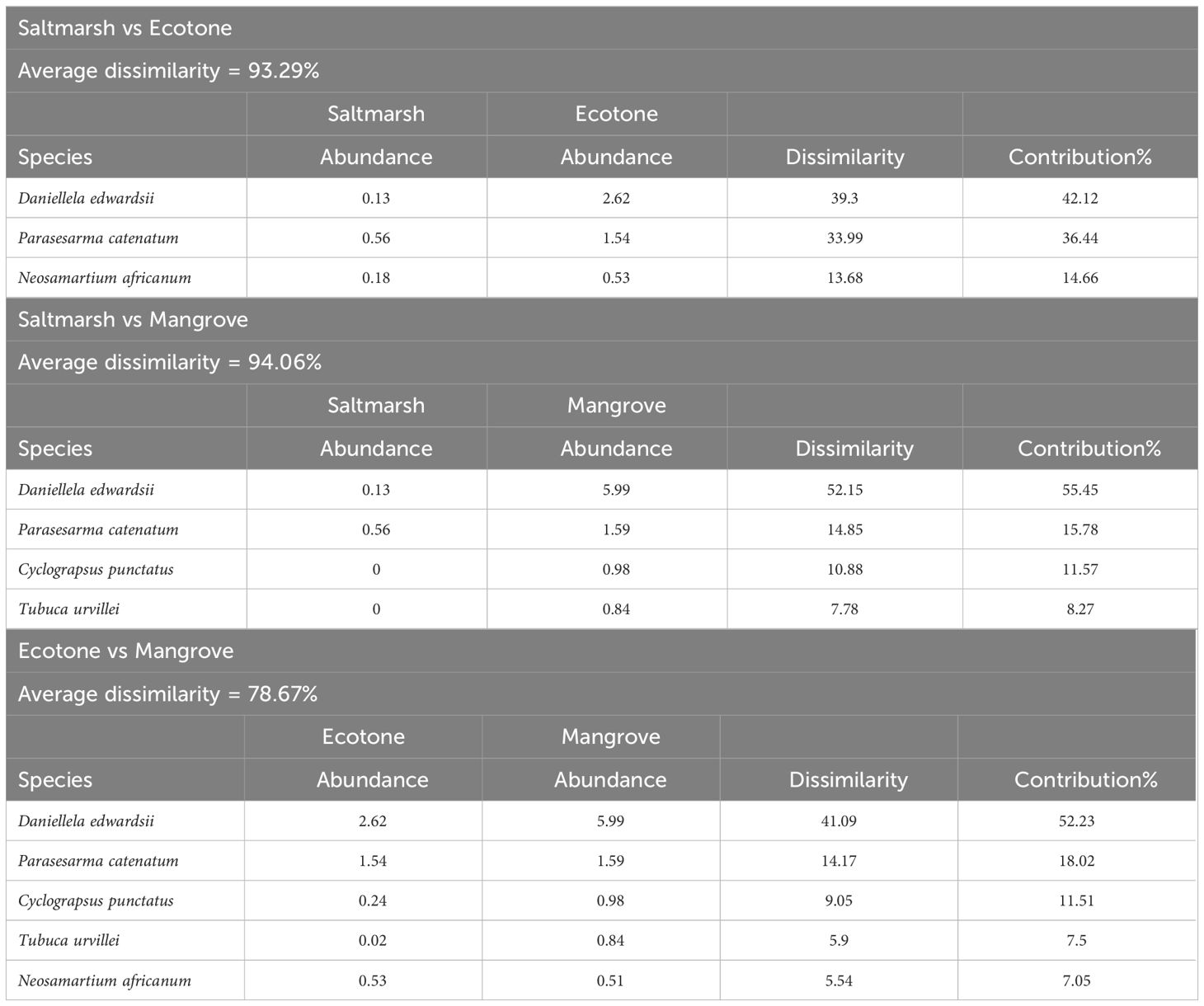
Table 5 The average abundance and dissimilarities of crab species amongst habitat types (across both sites), with a cut of low of 90.00%.
Avicennia marina was the only mangrove species present along the 20 m transects at both sites and was confined to the mangrove and ecotone habitats (Table 6). The differences occurred between the ecotone and mangrove habitats at both sites in terms of mangrove tree density, height and adult:seedling ratios. Nahoon had the highest tree density in the mangrove habitats, with trees ranging between 4 – 530 cm in height and having an adult:seedling ratio of 1:1. While in Tyolomnqa, mangrove tree density was highest in mangrove habitats, with tree heights ranging from 3 – 661 cm, with an adult:seedling ratio of 1:30.
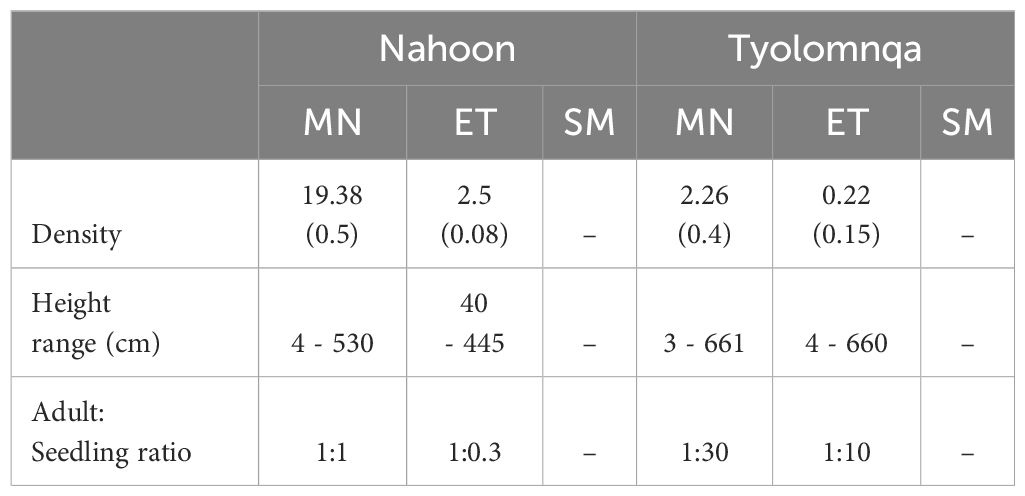
Table 6 The mean mangrove tree density (no. ind. m-2 (Standard Error)), range of tree height (cm) and the adult to seedling ratios of Avicennia marina counted within three habitat types (Mangrove – MN, Ecotone – ET, Saltmarsh – SM) in both sampling sites.
Regarding sediment characteristics, mangrove habitats had the highest sediment moisture content (%) and sediment organic matter (%) at both sites (Table 7). In Tyolomnqa, the ecotone habitat had the lowest SMC (%) and SOM (%), while in Nahoon, the ecotone had the lowest SMC (%) and the saltmarsh habitat had the lowest SOM (%). Mangroves generally consisted of clay and clay/silt, while the ecotone was generally clay/silt at Nahoon and silt/sand at Tyolomnqa. The sediment in the saltmarsh at Nahoon was silt while at Tyolomnqa, it was drier and sandier.
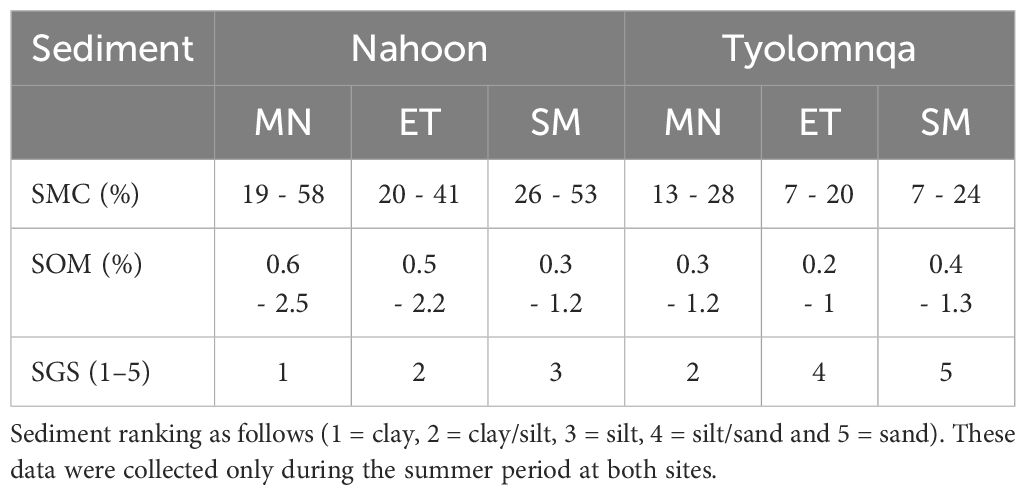
Table 7 The range of sediment moisture content (%) and sediment organic matter (%) and sediment grain size ranking from each of three habitat types (Mangrove – MN, Ecotone – ET, Saltmarsh – SM) at both sampling sites.
Sediment characteristics, such as sediment grain size, sediment organic moisture (%) and sediment moisture content (%) were significantly different amongst habitat types and site (ANOVA, p < 0.01), therefore the Tukey’s HSD post-hoc test was conducted and the results for habitat type are presented in the Table 8. When comparing Mangroves vs Ecotone, only SMC and SOM differed significantly, however when comparing Saltmarsh vs Ecotone, only SGS was significantly different. When comparing the Mangrove vs Saltmarsh habitats, SGS and SOM differed significantly.
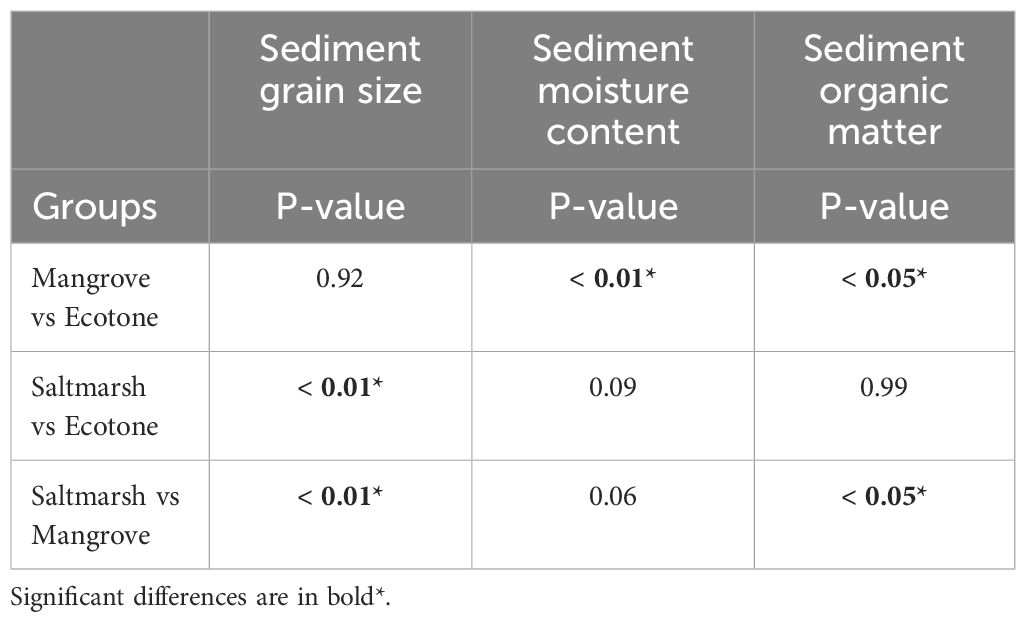
Table 8 Results from Tukey’s HSD post-hoc test, showing the significant differences in sediment grain size, sediment moisture content (%) and sediment organic matter (%) amongst habitat types across sites.
3.4 Drivers of brachyuran abundance, diversity, and richness
Both Nahoon and Tyolomnqa were previously dominated by saltmarsh species. The three habitat types (mangrove, ecotone and saltmarsh) were chosen to make comparisons across established and transitional habitats. From the ANOVA, saltmarsh species composition was significantly different amongst habitat types (p < 0.05), however, a TukeyHSD posthoc test revealed that there was only a significant difference between mangrove and saltmarsh habitats at both sites (p = 0.03). In Nahoon, saltmarsh species composition differed between the saltmarsh and ecotone habitat types (Figure 5A), as Juncus kraussii Hochst. (< 2%) only occurred in the saltmarsh habitat and the buffalo grass, Stenotaphrum secundatum (H. Walter) Kuntze, had a higher coverage in the saltmarsh habitat (41.5%) than the ecotone habitat (0.02%). The Nahoon mangrove habitat had < 1% of Sarcocornia spp. present. In Tyolomnqa, saltmarsh species were similar between all three habitat types, with Bassia diffusa (Thunb.) Kuntze, dominating in saltmarsh and ecotone habitats. Sarcocornia spp. occurred in all three habitat types in both sites (Figure 5A). In Nahoon, Stenotaphrum secundatum (H. Walter) Kuntze was more abundant in the saltmarsh habitat while Limonium latifolium (L.f.) Kuntze was more abundant in the ecotone.
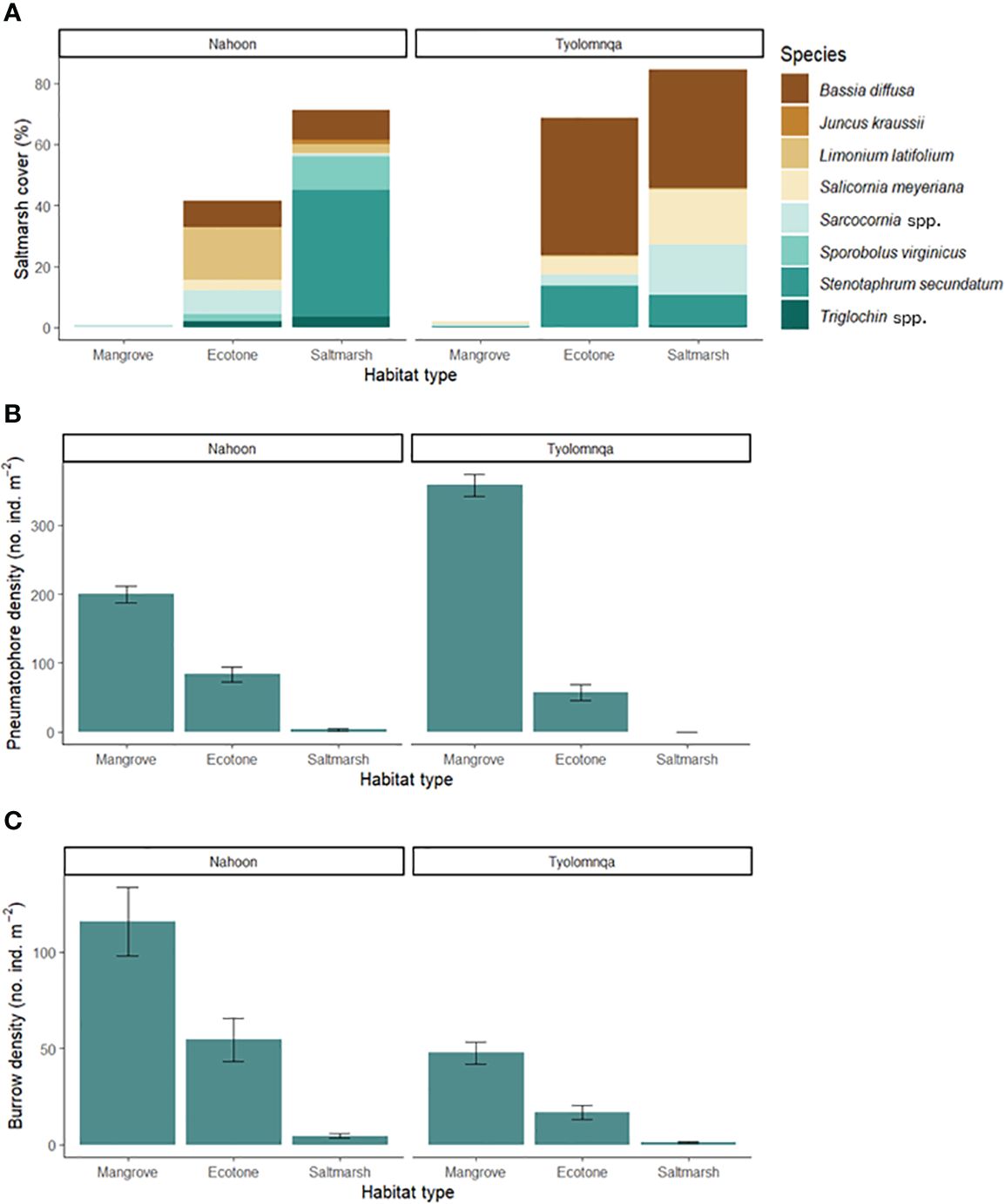
Figure 5 The composition and structure of the vegetation and crab burrows was characterised in 50 x 50 cm quadrats at each habitat type in both estuaries (Nahoon and Tyolomnqa) with (A) showing species composition of saltmarsh cover (%), (B) showing average (Standard Error) pneumatophore density (no. ind. m-2) and (C) showing average (Standard Error) burrow density (no. ind. m-2).
Inversely, as expected, pneumatophore density (no. ind. m-2) significantly decreased from mangroves to saltmarsh habitats at both estuaries (Figure 5B). In Tyolomnqa, pneumatophore density was highest in mangroves (358 (± 15 SE) ind. m-2) and absent in saltmarsh habitats. In Nahoon, mangroves had the highest pneumatophore density of 199 (± 12 SE) ind. m-2 with the saltmarsh habitats having the lowest of 3 (± 2 SE) ind. m-2.
There was a significant difference in mean burrow abundance amongst habitat types (p = 0.003). The mean burrow abundance generally decreased from mangroves into saltmarsh habitats (Figure 5C). As saltmarsh species cover increases, we see that burrow abundance decreases (Figures 5A, C) and as pneumatophore density increased, burrow abundance increased (Figures 5B, C).
Saltmarsh percentage cover and pneumatophore density appear to be significant drivers of brachyuran abundance (no. ind. m-2), diversity (Shannon-Wiener index) and richness (Table 9). Saltmarsh cover was negatively correlated with brachyuran abundance (r = - 0.43) and diversity (r = - 0.37) while pneumatophore density was positively correlated with abundance (r = 0.39) and diversity (r = 0.46).
4 Discussion
Global climate change is resulting in coastal vegetation shifts, as mangroves expand polewards and into temperate saltmarsh habitats (Cavanaugh et al., 2014; Saintilan et al., 2014; Raw et al., 2022). In both mangroves and saltmarshes, brachyurans fulfil important functional roles, although geographic and vegetated structural differences can be a driver of macrofauna community structure variation. These shifts in vegetation types can have an impact on associated macrofaunal species by altering their behavior (Cannizzo and Griffen, 2016), morphology (Weiskopf et al., 2020) or causing range shifts (Lenoir and Svenning, 2015). In turn, a shift in fauna will influence the existing habitat type and ecosystem function. The effects of mangrove expansion in South Africa have rarely been studied in faunal assemblages (Vorsatz, 2009; Whitfield et al., 2016) and this paper is among the first to present insight into this phenomenon.
4.1 Differences in past and present brachyuran assemblages
There was a significant change in brachyuran community composition over the past seven years, supporting hypothesis 1. Our results are similar to Freitas et al. (2021) who also found that brachyuran community composition has changed over an eight-year monitoring period, in the Babitonga Bay mangrove ecosystem located along the southern Brazilian coast due to anthropogenic impacts. Other studies have found that brachyuran communities are also influenced by habitat modifications (Skilleter and Warren, 2000), environmental shifts in foundation species including poleward expansions (Kelleway et al., 2017; Peer et al., 2018a; Walker et al., 2021; Theron et al., 2022), biotic interactions such as competition for resources and predation (Cannicci et al., 2018; Freitas et al., 2021) or natural environmental fluctuations such as tides and salinity fluctuations (Lee, 1999; Macintosh et al., 2002; Peer et al., 2014). Over the past seven years, species richness decreased at Nahoon and increased years in Tyolomnqa. More notably, this includes the absence of the fiddler crabs from the Nahoon Estuary and the southward range shift of the fiddler crab Tubuca urvillei and Paraleptuca chlorophthlamus from its previous known distribution limit in Nahoon (Peer et al., 2018b; Theron et al., 2022) to Tyolomnqa. It is likely that more mangrove-associated species will move further south towards Tyolomnqa as climate change continues to influence poleward distribution.
4.2 Differences between mangrove sites of different ages
Given that Nahoon was an older and more established planted mangrove site compared to Tyolomnqa (Hoppe-Speer et al., 2015), we expected community structure to differ between sites with more mangrove-associated species at Nahoon. However, brachyuran community structure was similar between these two sites supporting hypothesis 2. Ashton et al. (2003) observed that grapsoid crabs (mainly sesarmids) dominated in both high abundances and diversity at mature mangrove forests, whereas ocypodid crabs (such as fiddler crabs) were found at high abundances in younger mangrove forests. We found similar patterns to Ashton et al. (2003) as the fiddler crabs T. urvillei and Paraleptuca chlorophtalmus inhabited the younger mangrove site (Tyolomnqa), while only grapsoidcrabs (mainly sesarmids) inhabited the older site (Nahoon) (Table 1). Other studies also found this trend in both Malaysia and Thailand (Berry, 1972; Sasekumar, 1974; Macintosh, 1984).
4.3 Differences amongst habitat types
Brachyuran community structure differed between all habitat types at both sites supporting hypothesis 3. Factors such as vegetation and sediment characteristics could have resulted in the differences seen in community structure between habitat types. For instance, both grapsoids (mainly sesarmids) and ocypodids (fiddler crabs) are burrowing taxa and are thus influenced by both above- and below-ground vegetation structures (Nobbs, 2003; Walker et al., 2021). In terms of above-ground vegetation, mangrove adult trees provide shade through canopy cover which can drive high abundances of crabs (Peer et al., 2018b; Theron et al., 2022). Whereas the saltmarsh habitat is an open clearing with direct sunlight, which could be unfavourable for most mangrove crabs that have a physiological limitation to both high temperatures and high evaporation rates (Nobbs, 2003 and references therein). Our results show that brachyuran abundance and diversity significantly decrease with an increase in saltmarsh cover, which is supported by several other studies (Raposa et al., 2018; Wasson et al., 2019; Tyrrell et al., 2023), although van der Wal and Herman (2012) in the Netherlands, found that Aster tripolium and Salicornia procumbens saltmarsh species cover had no significant effect on macrofaunal diversity, structure or biomass; and Chen et al. (2022) found that crab burrows were more abundant in saltmarsh vegetation, with higher organic matter and water content, compared to unvegetated areas in the Doulong harbor of Yancheng intertidal flat in China.
In terms of pneumatophores, which form both above- and below-ground structures, mangrove quadrats had the highest pneumatophore density (Figure 5B) which drives the abundance, diversity and richness of brachyuran crabs. Our results correspond to other studies which find that macrofauna were more abundant and diverse with higher pneumatophore density (Virnstein, 1977; Walker et al., 2019; Freitas et al., 2021). On the other hand, saltmarsh vegetation has different root complexities (Bertness et al., 2014), that could inhibit and obstruct crabs from burrowing into the sediment (Wang et al., 2008). Roots of the buffalo grass Stenotaphrum secundatum are dense and deep (Busey, 2003) and had a high coverage in saltmarshes at Nahoon, likely reducing the available below-ground space for burrowing crabs. Whereas Bassia diffusa is a herb with fibrous taproots (Turki et al., 2008) occurring in saltmarshes and ecotones in Tyolomnqa, that likely obstruct burrowing crabs. The highly dense roots of saltmarshes usually stabilize and obstruct the sediment, making it difficult for brachyurans to burrow, as supported by the decrease in mean burrow abundance from mangroves to saltmarshes (Figure 5).
Studies have shown that crabs prefer soft substrates where sediment is easy to create connected tunnels in burrows (Flores et al., 2005; Bertness et al., 2014). The sediment grain size in saltmarshes was significantly different to mangroves and ecotones. Sediment grain size has been found to drive fiddler crab abundance (Peer et al., 2018b) and sesarmid crabs prefer soft clay substrates that occur in the mangroves (Nobbs, 2003). Contrary to Min et al. (2023) who found that crab burrow density decreases under canopy cover, we found that mean burrow abundance increases in mangrove areas where canopy cover is higher than in saltmarshes. Canopy cover can likely reduce predation risk (Nomann and Pennings, 1998) or increase soil moisture content (Nobbs, 2003; Kon et al., 2010). This is supported by our results which show that mangroves have a higher sediment moisture content (%) compared to the ecotones and saltmarshes which were much drier.
Lastly, the situation of mangroves and saltmarshes in relation to the adjacent estuary could also play a role in determining community structure, as mangroves in Nahoon and Tyolomnqa are situated adjacent to the estuarine water bodies and saltmarshes are situated further away. This results in more sesarmids occupying mangroves as they depend on water for respiration (Nobbs, 2003) and would thus require higher immersion rates. If mangrove associated species move into saltmarshes, it is likely that they can alter the ecosystem by either clearing saltmarsh species or changing the sediment composition. It is worth noting that many seedlings were recorded in both ecotones of Nahoon and Tyolomnqa, indicative of potential mangrove expansion into saltmarsh habitats. However, mangroves in Nahoon expanded in an open sandflat area rather than outcompeting saltmarsh species (Hoppe-Speer et al., 2015). Changes in the intertidal zone can favour saltmarshes over mangroves or vice versa (Whitfield et al., 2016).
4.4 Brachyuran ecology
The marsh crab, Parasesarma catanatum, was the only crab species that was common across all three habitats and both sites. This sesarmid crab is found at high densities in southern Cape estuaries within Spartina saltmarshes and is associated with mangrove forests in Eastern Cape (Paula et al., 2003) as they consume both decomposed leaves of saltmarsh plants (Paterson & Whitfield, 1997; Bergamino and Richoux, 2015) and mangrove leaf litter (Lee, 1998). In Nahoon, aside from P. catenatum, Neosarmatium africanum and Daniellela edwardsii also occurred at all three habitat types. The red mangrove crab, N. africanum occurs in saltmarshes possibly because it can survive in drier areas (Dahdouh-Guebas et al., 1997) due to its semi-terrestrial air breathing trait (Lee, 1998). Danielella edwardsii is common in both mangroves and marshes, preferring wet, soft sand (Macnae, 1963). In Tyolomnqa, D. edwardsii was the dominant species. Although mangrove stands in Tyolomnqa are established, and despite the newly recorded presence of two fiddler crab species, the mangrove-associated macrofauna are still predominantly estuarine species that are known to occupy a range of different habitats (Peer et al., 2018b).
Fiddler crabs and grapsoids such as sesarmids differ in their burrowing and feeding activities (Warren and Underwood, 1986; Szura et al., 2017). Fiddler crabs create complex vertical burrows (Min et al., 2023) and are considered deposit feeders, consuming mainly microphytobenthos and plant and animal detritus (Dye and Lasiak, 1986), while sesarmids create simple and horizontal burrows (Min et al., 2023) and are considered herbivorous as they consume mangrove leaf litter (Micheli et al., 1991). The excessive burrowing and bioturbation from mangrove-associated fauna in saltmarshes can cause increased soil erosion and lower productivity of plants (Smith et al., 2013). Studies have shown that crabs such as Parasesarma catenatum and Cyclograpsus punctatus feed on the saltmarsh species in the genus Spartina (Alexander and Ewer, 1969; Smith and Tyrrell, 2012; Smith et al., 2013). If these species occur at high densities, they have the potential to weaken both above and below ground structures of saltmarsh causing a loss in plants and root decay (Coverdale et al., 2012). Whereas fiddler crabs could have positive effects on saltmarsh vegetation by oxygenating soil through bioturbation and enhancing sediment organic matter (Hughes et al., 2014).
5 Conclusion
Nahoon, the more established mangrove site, was dominated by mangrove-associated brachyuran species, while Tyolomnqa, the younger mangrove site, was still dominated by estuarine-associated crabs. Despite this, we present a new record of two fiddler crab species in Tyolomnqa, with one species occurring at a new southernmost limit. At both sites, mangrove-associated macrofauna do not occupy the saltmarsh habitat, however, with the expansion of mangroves into saltmarshes, it is possible that these species will start to move into saltmarsh habitats as reported in other studies (Whitfield et al., 2016; Cannizzo et al., 2018, 2019). Future studies should continue to monitor changes in brachyuran assemblages as mangroves continue to expand into saltmarsh habitats and investigate relationships between various saltmarsh species and mangrove-associated crabs.
Data availability statement
The raw data supporting the conclusions of this article will be made available by the authors, without undue reservation.
Author contributions
CK: Writing – original draft, Investigation, Formal analysis, Data curation, Conceptualization. AR: Writing – review & editing, Supervision, Resources, Methodology, Investigation, Funding acquisition, Conceptualization. NP: Writing – review & editing, Supervision, Resources, Project administration, Methodology, Investigation, Funding acquisition, Conceptualization.
Funding
The author(s) declare financial support was received for the research, authorship, and/or publication of this article. This work is based on the research supported by the National Research Foundation of South Africa through a Thuthuka Grant (Grant Reference TTK2204052094) and a Marine and Coastal Research Grant (Grant no. 136490). The authors acknowledge that opinions, findings and conclusions or recommendations expressed in this publication generated by the NRF supported research is that of the authors, and that the NRF accepts no liability whatsoever in this regard.
Acknowledgments
We are grateful to Emma Rossouw and Jake Mulvaney who assisted with data collection during field trips for this study. We would also like to thank Susana Fietz and Megan Mathese for use of their equipment during laboratory processing. A sampling permit was obtained from the Department of Forestry, Fisheries and the Environment of the Republic of South Africa (permit number Res2023–79).
Conflict of interest
The authors declare that the research was conducted in the absence of any commercial or financial relationships that could be construed as a potential conflict of interest.
Publisher’s note
All claims expressed in this article are solely those of the authors and do not necessarily represent those of their affiliated organizations, or those of the publisher, the editors and the reviewers. Any product that may be evaluated in this article, or claim that may be made by its manufacturer, is not guaranteed or endorsed by the publisher.
References
Adams J. B. (2020). Salt marsh at the tip of Africa: Patterns, processes and changes in response to climate change. Estuarine Coast. Shelf Sci. 237, 106650. doi: 10.1016/j.ecss.2020.106650
Adams J. B., Colloty B. M., Bate G. C. (2004). The distribution and state of mangroves along the coast of Transkei, Eastern Cape Province, South Africa. Wetlands Ecol. Manage. 12, 531–541. doi: 10.1007/s11273-005-5165-0
Adams D. H., Edwards D. D., Schneider J. E., Searles A. R. (2024). Range expansion and population shifts of estuarine fishes in a changing subtropical estuary. Mar. Ecol. Prog. Ser. 728, 221–238. doi: 10.3354/meps14314
Adams J. B., Human L. R. D. (2016). Investigation into the mortality of mangroves at St. Lucia Estuary. South Afr. J. Bot. 107, 121–128. doi: 10.1016/j.sajb.2016.03.018
Adams J. B., Rajkaran A. (2021). Changes in mangroves at their southernmost African distribution limit. Estuarine Coast. Shelf Sci. 248, 107158. doi: 10.1016/j.ecss.2020.107158
Adams J. B., Veldkornet D., Tabot P. (2016). Distribution of macrophyte species and habitats in South African estuaries. South Afr. J. Bot. 107, 5–11. doi: 10.1016/j.sajb.2016.08.001
Alexander S. J., Ewer D. W. (1969). A comparative study of some aspects of the biology and ecology of Sesarma catenata and Cyclograpsus punctatus M.Edw., with additional observation on Sesarma meinerti De Man. Zoologica Africana 4, 1–35. doi: 10.1080/00445096.1969.11447364
Ashton E. C., Hogarth P. J., Macintosh D. J. (2003). A comparison of brachyuran crab community structure at four mangrove locations under different management systems along the Melaka Straits-Andaman Sea Coast of Malaysia and Thailand. Estuaries 26, 1461–1471. doi: 10.1007/BF02803654
Bergamino L., Richoux N. B. (2015). Spatial and temporal changes in estuarine food web structure: differential contributions of marsh grass detritus. Estuaries Coasts 38, 367–382. doi: 10.1007/s12237-014-9814-5
Berry A. J. (1972). The natural history of West Malaysian mangrove faunas. Malayan Nat. J. 25, 135–162. doi: 10.1136/jmg.9.1.135
Bertness M. D. (1985). Fiddler crab regulation of Spartina alterniflora production on a New England salt marsh. Ecology 66, 1042–1055. doi: 10.2307/1940564
Bertness M. D., Brisson C. P., Bevil M. C., Crotty S. M. (2014). Herbivory drives the spread of salt marsh die-off. PloS One 9, e92916. doi: 10.1371/journal.pone.0092916
Bertness M. D., Miller T. (1984). The distribution and dynamics of Uca pugnax (Smith) burrows in a New England salt marsh. J. Exp. Mar. Biol. Ecol. 83, 211–237. doi: 10.1016/S0022-0981(84)80002-7
Bolosha U. (2016). Revising the distribution of mangrove forests in South Africa and changes in growth of mangrove species along a latitudinal gradient (Masters dissertation, M. Sc. Thesis. Rhodes University, South Africa, 139.
Busey P. (2003). St. Augustine grass, Stenotaphrum secundatum (Walt.) Kuntze. Turfgrass biology, genetics, and breeding (Hoboken, NJ: John Wiley & Sons), 309–329.
Cannicci S., Fusi M., Cimó F., Dahdouh-Guebas F., Fratini S. (2018). Interference competition as a key determinant for spatial distribution of mangrove crabs. BMC Ecol. 18, 1–12. doi: 10.1186/s12898-018-0164-1
Cannizzo Z. J., Dixon S. R., Griffen B. D. (2018). An anthropogenic habitat within a suboptimal colonized ecosystem provides improved conditions for a range-shifting species. Ecol. Evol. 8, 1521–1533. doi: 10.1002/ece3.3739
Cannizzo Z. J., Griffen B. D. (2016). Changes in spatial behavior patterns by mangrove tree crabs following climate-induced range shift into novel habitat. Anim. Behav. 121, 79–86. doi: 10.1016/j.anbehav.2016.08.025
Cannizzo Z. J., Griffen B. D. (2019). An artificial habitat facilitates a climate-mediated range expansion into a suboptimal novel ecosystem. PloS One 14, e0211638. doi: 10.1371/journal.pone.0211638
Cannizzo Z. J., Nix S. K., Whaling I. C., Griffen. B. D. (2019). Individual morphology and habitat structure alter social interactions in a range-shifting species. Diversity 11, no. doi: 10.3390/d11010006
Cavanaugh K. C., Dangremond E. M., Doughty C. L., Williams A. P., Parker J. D., Hayes M. A., et al. (2019). Climate-driven regime shifts in a mangrove–salt marsh ecotone over the past 250 years. Proc. Natl. Acad. Sci. 116, 21602–21608. doi: 10.1073/pnas.1902181116
Cavanaugh K. C., Kellner J. R., Forde A. J., Gruner D. S., Parker J. D., Rodriguez W., et al. (2014). Poleward expansion of mangroves is a threshold response to decreased frequency of extreme cold events. Proc. Natl. Acad. Sci. 111, 723–727. doi: 10.1073/pnas.1315800111
Chen I. C., Hill J. K., Ohlemüller R., Roy D. B., Thomas C. D. (2011). Rapid range shifts of species associated with high levels of climate warming. Science 333, 1024–1026. doi: 10.1126/science.1206432
Chen X., Zhou Z., He Q., Zhang H., Bouma T., Gong Z., et al. (2022). Role of abiotic drivers on crab burrow distribution in a saltmarsh wetland. Front. Mar. Sci. 9, 1040308. doi: 10.3389/fmars.2022.1040308
Clarke K. R., Gorley R. N. (2009). PRIMER: Getting started with v6. PRIMER-E Ltd. Plymouth, United Kingdom.
Comeaux R. S., Allison M. A., Bianchi T. S. (2012). Mangrove expansion in the Gulf of Mexico with climate change: Implications for wetland health and resistance to rising sea levels. Estuarine Coast. Shelf Sci. 96, 81–95. doi: 10.1016/j.ecss.2011.10.003
Coverdale T. C., Altieri A. H., Bertness M. D. (2012). Belowground herbivory increases vulnerability of New England salt marshes to die-off. Ecology 93, 2085–2094. doi: 10.1890/12-0010.1
Dahdouh-Guebas F., Verneirt M., Tack J. F., Koedam N. (1997). Food preferences of Neosarmatium meinerti de Man (Decapoda: Sesarminae) and its possible effect on the regeneration of mangroves. Hydrobiologia 347, 83–89. doi: 10.1023/A:1003015201186
Demopoulos A. W., Smith C. R. (2010). Invasive mangroves alter macrofaunal community structure and facilitate opportunistic exotics. Mar. Ecol. Prog. Ser. 404, 51–67. doi: 10.3354/meps08483
Dye A. H., Lasiak T. A. (1986). Microbenthos, meiobenthos and fiddler crabs: trophic interactions in a tropical mangrove sediment. Mar. Ecol. Prog. Ser. 32, 259–264. doi: 10.3354/meps032259
Edney E. B. (1961). The water and heat relationships of fiddler crabs (Uca spp.). Trans. R. Soc. South Afr. 36, 71–91. doi: 10.1080/00359196109519035
Emmerson W. D. (1994). Seasonal breeding cycles and sex ratios of eight species of crabs from Mgazana, a mangrove estuary in Transkei, southern Africa. J. Crustacean Biol. 14, 568–578. doi: 10.2307/1549002
Eslami-Andargoli L., Dale P. E. R., Sipe N., Chaseling J. (2009). Mangrove expansion and rainfall patterns in Moreton Bay, southeast Queensland, Australia. Estuarine Coast. Shelf Sci. 85, 292–298. doi: 10.1016/j.ecss.2009.08.011
Flores A. A., Abrantes K. G., Paula J. (2005). Estimating abundance and spatial distribution patterns of the bubble crab Dotilla fenestrata (Crustacea: Brachyura). Austral Ecol. 30, 14–23. doi: 10.1111/j.1442-9993.2005.01409.x
Freitas R. F., Pagliosa P. R. (2020). Mangrove benthic macrofauna: drivers of community structure and functional traits at multiple spatial scales. Mar. Ecol. Prog. Ser. 638, 25–38. doi: 10.3354/meps13260
Freitas F. Jr., Pescinelli R. A., Costa R. C., Hilesheim J. C., Dieh F. L., Branco J. O. (2021). Brachyuran crab diversity across spatial and temporal scales in a mangrove ecosystem from the western Atlantic. Regional Stud. Mar. Sci. 43, 101703. doi: 10.1016/j.rsma.2021.101703
Gao X., Gaitan-Espitia J. D., Lee S. Y. (2024). Regulatory role of sesarmid crabs in nutrient dynamics and implications for the productivity of mangroves. Estuarine Coast. Shelf Sci. 298, 108655. doi: 10.1016/j.ecss.2024.108655
Geldenhuys C., Cotiyane P., Rajkaran A. (2016). Understanding the creek dynamics and environmental characteristics that determine the distribution of mangrove and salt marsh communities at Nahoon Estuary. South Afr. J. Bot. 107, 137–147. doi: 10.1016/j.sajb.2016.04.013
Giri C., Ochieng E., Tieszen L. L., Zhu Z., Singh A., Loveland T., et al. (2011). Status and distribution of mangrove forests of the world using earth observation satellite data. Global Ecol. Biogeography 20, 154–159. doi: 10.1111/j.1466-8238.2010.00584.x
Godoy M. D., Lacerda L. D. D. (2015). Mangroves response to climate change: a review of recent findings on mangrove extension and distribution. Anais da Academia Bras. Ciências 87, 651–667. doi: 10.1590/0001-3765201520150055
Gutierrez J. L. (2008). Crab influences on the export of plant detritus from salt marshes and mangroves: A review. In Chen J., Guo C. (eds.), Ecosystem Ecol. Res. Trends, 327–344.
Hensel M. J., Patrick C. J., Orth R. J., Wilcox D. J., Dennison W. C., Gurbisz C., et al. (2023). Rise of Ruppia in Chesapeake Bay: Climate change–driven turnover of foundation species creates new threats and management opportunities. Proc. Natl. Acad. Sci. 120, e2220678120. doi: 10.1073/pnas.2220678120
Hodgson A. N. (1987). Distribution and abundance of the macrobenthic fauna of the Kariega Estuary. Afr. Zoology 22, 153–162.
Hogarth P. J. (2015). The biology of mangroves and seagrasses (Oxford, UK: Oxford University Press). doi: 10.1093/acprof:oso/9780198716549.001.0001
Hoppe-Speer S. C., Adams J. B., Rajkaran A. (2015). Mangrove expansion and population structure at a planted site, East London, South Africa. South. Forests: J. For. Sci. 77, 131–139. doi: 10.2989/20702620.2014.1001622
Hughes A. R., Moore A. F., Piehler M. F. (2014). Independent and interactive effects of two facilitators on their habitat-providing host plant, Spartina alterniflora. Oikos 123, 488–499. doi: 10.1111/j.1600-0706.2013.01035.x
Kelleway J. J., Cavanaugh K., Rogers K., Feller I. C., Ens E., Doughty C., et al. (2017). Review of the ecosystem service implications of mangrove encroachment into salt marshes. Global Change Biol. 23, 3967–3983. doi: 10.1111/gcb.13727
Kon K., Kurokura H., Tongnunui P. (2010). Effects of the physical structure of mangrove vegetation on a benthic faunal community. J. Exp. Mar. Biol. Ecol. 383, 171–180. doi: 10.1016/j.jembe.2009.11.015
Lee S. Y. (1998). Ecological role of grapsid crabs in mangrove ecosystems: a review. Mar. Freshw. Res. 49, 335–343. doi: 10.1071/MF97179
Lee S. Y. (1999). Tropical mangrove ecology: physical and biotic factors influencing ecosystem structure and function. Aust. J. Ecol. 24, 355–366. doi: 10.1046/j.1442-9993.1999.00984.x
Lenoir J., Svenning J. C. (2015). Climate-related range shifts–a global multidimensional synthesis and new research directions. Ecography 38, 15–28. doi: 10.1111/ecog.00967
Leung J. Y. (2015). Habitat heterogeneity determining the macrobenthic infaunal community in a mangrove swamp in South China: implication for plantation and plant invasion. J. Coast. Res. 31, 624–633. doi: 10.2112/JCOASTRES-D-13-00091.1
Liu T., Liu S., Wu B., Xu H., Zhang H. (2020). Increase of organic carbon burial response to mangrove expansion in the Nanliu River estuary, South China Sea. Prog. Earth Planetary Sci. 7, 1–9. doi: 10.1186/s40645-020-00387-3
Loveless J. B., Smee D. L. (2019). Changes in arthropod communities as black mangroves Avicennia germinans expand into Gulf of Mexico salt marshes. Arthropod-Plant Interact. 13, 465–475. doi: 10.1007/s11829-018-9643-8
Lovelock C. E., Feller I. C., Ellis J., Schwarz A. M., Hancock N., Nichols P., et al. (2007). Mangrove growth in New Zealand estuaries: the role of nutrient enrichment at sites with contrasting rates of sedimentation. Oecologia 153, 633–641. doi: 10.1007/s00442-007-0750-y
Luk Y. C., Zajac R. N. (2013). Spatial ecology of fiddler crabs, Uca pugnax, in southern New England salt marsh landscapes: potential habitat expansion in relation to salt marsh change. Northeastern Nat. 20, 255–274. doi: 10.1656/045.020.0213
Macintosh D. J. (1984). Ecology and productivity of Malaysian mangrove crab populations (Decapoda: Brachyura). Eds. Soepadmo E., Rao A. N. & Macintosh D. J. (University of Malaya and UNESCO), 354–377. Proceedings of the Asean Symposium on Mangrove Environment – Research & Management. Chopmen Publishers: Singapore.
Macintosh D. J., Ashton E. C., Havanon S. (2002). Mangrove rehabilitation and intertidal biodiversity: a study in the Ranong mangrove ecosystem, Thailand. Estuarine Coast. Shelf Sci. 55, pp.331–pp.345. doi: 10.1006/ecss.2001.0896
Micheli F., Gherardi F., Vannini M. (1991). Feeding and burrowing ecology of two East African mangrove crabs. Mar. Biol. 111, 247–254. doi: 10.1007/BF01319706
Min W. W., Kandasamy K., Balakrishnan B. (2023). Crab species-specific excavation and architecture of burrows in restored mangrove habitat. J. Mar. Sci. Eng. 11, 310. doi: 10.3390/jmse11020310
Mitchell K. (2010). Quantitative analysis by the point-centered quarter method. arXiv preprint:1010.3303.
Muthukrishnan R., Chiquillo K. L., Cross C., Fong P., Kelley T., Toline C. A., et al. (2020). Little giants: a rapidly invading seagrass alters ecosystem functioning relative to native foundation species. Mar. Biol. 167, 1–15. doi: 10.1007/s00227-020-03689-8
Nobbs M. (2003). Effects of vegetation differ among three species of fiddler crabs (Uca spp.). J. Exp. Mar. Biol. Ecol. 284, pp.41–pp.50. doi: 10.1016/S0022-0981(02)00488-4
Nobbs M., Blamires S. J. (2017). Fiddler crab spatial distributions are influenced by physiological stressors independent of sympatric interactions. J. Exp. Mar. Biol. Ecol. 491, 19–26. doi: 10.1016/j.jembe.2017.03.007
Nomann B. E., Pennings S. C. (1998). Fiddler crab–vegetation interactions in hypersaline habitats. J. Exp. Mar. Biol. Ecol. 225, 53–68. doi: 10.1016/S0022-0981(97)00209-8
Ólafsson E., Ndaro S. G. (1997). Impact of the mangrove crabs Uca annulipes and Dotilla fenestrata on meiobenthos. Mar. Ecol. Prog. Ser. 158, 225–231. doi: 10.3354/meps158225
Osland M. J., Enwright N., Day R. H., Doyle T. W. (2013). Winter climate change and coastal wetland foundation species: salt marshes vs. Mangrove forests in the southeastern United States. Global Change Biol. 19, 1482–1494. doi: 10.1111/gcb.12126
Paterson A. W., Whitfield A. K. (1997). A stable carbon isotope study of the food-web in a freshwater-deprived South African estuary, with particular emphasis on the ichthyofauna. Estuarine Coast. Shelf Sci. 45, 705–715. doi: 10.1006/ecss.1997.0243
Paula J., Dornelas M., Flores A. A. V. (2003). Stratified settlement and moulting competency of brachyuran megalopae in Ponta Rasa mangrove swamp, Inhaca Island (Mozambique). Estuarine Coast. Shelf Sci. 56, 325–337. doi: 10.1016/S0272-7714(02)00165-8
Peer N., Miranda N. A. F., Perissinotto R. (2016). Suspended silt and salinity tolerances of the first zoeal stage of the fiddler crab Uca annulipes (Decapoda: Brachyura) and why marine connectivity is essential to the survival of the species. African Journal of Marine Science 38 (2), 161–169. doi: 10.2989/1814232X.2016.1169217
Peer N., Miranda N. A., Perissinotto R. (2015). A review of fiddler crabs (genus Uca Leach 1814) in South Africa. Afr. Zoology 50, 187–204. doi: 10.1080/15627020.2015.1055700
Peer N., Perissinotto R., Miranda N. A. F., Taylor R. H. (2014). Temporal variations in the diversity of true crabs (Crustacea: Brachyura) in the St Lucia Estuary, South Africa. Afr. Invertebrates 55, 39–65. doi: 10.5733/afin.055.0103
Peer N., Rajkaran A., Miranda N. A. F., Taylor R. H., Newman B., Porri F., et al. (2018a). Latitudinal gradients and poleward expansion of mangrove ecosystems in South Africa: 50 years after Macnae’s first assessment. Afr. J. Mar. Sci. 40, 101–120. doi: 10.2989/1814232X.2018.1466728
Peer N., Rishworth G. M., Miranda N. A., Perissinotto R. (2018b). Biophysical drivers of fiddler crab species distribution at a latitudinal limit. Estuarine Coast. Shelf Sci. 208, 131–139. doi: 10.1016/j.ecss.2018.05.001
Perry A. L., Low P. J., Ellis J. R., Reynolds J. D. (2005). Climate change and distribution shifts in marine fishes. Science 308, 1912–1915. doi: 10.1126/science.1111322
Pinsky M. L., Selden R. L., Kitchel Z. J. (2020). Climate-driven shifts in marine species ranges: Scaling from organisms to communities. Annu. Rev. Mar. Sci. 12, 153–179. doi: 10.1146/annurev-marine-010419-010916
Poloczanska E. S., Brown C. J., Sydeman W. J., Kiessling W., Schoeman D. S., Moore P. J., et al. (2013). Global imprint of climate change on marine life. Nat. Climate Change 3, 919–925. doi: 10.1038/nclimate1958
Raposa K. B., McKinney R. A., Wigand C., Hollister J. W., Lovall C., Szura K., et al. (2018). Top-down and bottom-up controls on southern New England salt marsh crab populations. PeerJ 6, e4876. doi: 10.7717/peerj.4876
Raw J. L., Godbold J. A., Van Niekerk L., Adams J. B. (2019). Drivers of mangrove distribution at the high-energy, wave-dominated, southern African range limit. Estuarine Coast. Shelf Sci. 226, 106296. doi: 10.1016/j.ecss.2019.106296
Raw J. L., van der Stocken T., Carroll D., Harris L. R., Rajkaran A., Van Niekerk L., et al. (2022). Dispersal and coastal geomorphology limit potential for mangrove range expansion under climate change. J. Ecol. 111, 139–155. doi: 10.1111/1365-2745.14020
Raw J. L., Van Niekerk L., Chauke O., Mbatha H., Riddin T., Adams J. B. (2023). Blue carbon sinks in South Africa and the need for restoration to enhance carbon sequestration. Sci. Total Environ. 859, 160142. doi: 10.1016/j.scitotenv.2022.160142
R Core Team. (2024). R: A Language and Environment for Statistical Computing. Vienna, Austria: R Foundation for Statistical Computing.
Saintilan N., Wilson N. C., Rogers K., Rajkaran A., Krauss K. W. (2014). Mangrove expansion and salt marsh decline at mangrove poleward limits. Global Change Biol. 20, 147–157. doi: 10.1111/gcb.12341
Sasekumar A. (1974). The distribution of macrofauna on a Malayan mangrove shore. J. Anim. Ecol. 43, 51–69. doi: 10.2307/3157
Schweiger O., Settele J., Kudrna O., Klotz S., Kühn I. (2008). Climate change can cause spatial mismatch of trophically interacting species. Ecology 89, 3472–3479. doi: 10.1890/07-1748.1
Skilleter G. A., Warren S. (2000). Effects of habitat modification in mangroves on the structure of mollusc and crab assemblages. J. Exp. Mar. Biol. Ecol. 244, 107–129. doi: 10.1016/S0022-0981(99)00133-1
Smee D. L., Sanchez J. A., Diskin M., Trettin C. (2017). Mangrove expansion into salt marshes alters associated faunal communities. Estuarine Coast. Shelf Sci. 187, 306–313. doi: 10.1016/j.ecss.2017.02.005
Smith S. M., Tyrrell M. C. (2012). Effects of mud fiddler crabs (Uca pugnax) on the recruitment of halophyte seedlings in salt marsh dieback areas of Cape Cod (Massachusetts, USA). Ecol. Res. 27, 233–237. doi: 10.1007/s11284-011-0886-4
Smith S. M., Tyrrell M. C., Congretel M. (2013). Palatability of salt marsh forbs and grasses to the purple marsh crab (Sesarma reticulatum) and the potential for re-vegetation of herbivory-induced salt marsh dieback areas in cape cod (Massachusetts, USA). Wetlands Ecol. Manage. 21, 263–275. doi: 10.1007/s11273-013-9298-2
Steinke T. D. (1972). Further observations on the distribution of mangroves in the Eastern Cape Province. Journal of South African Botany 38, 165–178.
Steinke T. D., Rajh A., Holland A. J. (1993). The feeding behavior of the red mangrove crab Sesarma meinerti De Man 1887 (Crustacea: Decapoda: Grapsidae) and its effect on the degradation of mangrove leaf litter. South Afr. J. Mar. Sci. 13, 151–160. doi: 10.2989/025776193784287455
Steinke T. D., Ward C. J. (2003). Use of plastic drift cards as indicators of possible dispersal of propagules of the mangrove Avicennia marina by ocean currents. Afr. J. Mar. Sci. 25, 169–176. doi: 10.2989/18142320309504007
Sweetman A. K., Middelburg J. J., Berle A. M., Bernardino A. F., Schander C., Demopoulos A. W. J., et al. (2010). Impacts of exotic mangrove forests and mangrove deforestation on carbon remineralization and ecosystem functioning in marine sediments. Biogeosciences 7, 2129–2145. doi: 10.5194/bg-7-2129-2010
Szura K., Mckinney R. A., Wigand C., Oczkowski A., Hanson A., Gurak J., et al. (2017). Burrowing and foraging activity of marsh crabs under different inundation regimes. J. Exp. Mar. Biol. Ecol. 486, 282–289. doi: 10.1016/j.jembe.2016.10.029
Taylor D. I., Allanson B. R. (1993). Impacts of dense crab populations on carbon exchanges across the surface of a salt marsh. Mar. Ecology-Progress Ser. 101, 119–119. doi: 10.3354/meps101119
Theron W., Peer N., Rajkaran A. (2022). Effects of the mangrove forest environment and tree species characteristics on fiddler crab communities. Mar. Freshw. Res. 73, 1283–1296. doi: 10.1071/MF21309
Turki Z., El-Shayeb F., Shehata F. (2008). Taxonomic studies in the camphorosmeae (Chenopodiaceae) 1. Subtribe: kochiinae (Genera: bassia all., kochia roth and chenolea thunb.). Acta Botanica Hungarica 50, 181–201. doi: 10.1556/ABot.50.2008.1-2.14
Tyrrell M. C., Courtney S., Brown E., von Sperber C. (2023). Burrowing crabs’ influence on tidal marsh vegetation species composition and abundance in a temperate back-barrier marsh, Cape Cod, Massachusetts, USA. J. Exp. Mar. Biol. Ecol. 567, 151931. doi: 10.1016/j.jembe.2023.151931
van der Wal D., Herman P. M. (2012). Ecosystem engineering effects of Aster tripolium and Salicornia procumbens salt marsh on macrofaunal community structure. Estuaries Coasts 35, 714–726. doi: 10.1007/s12237-011-9465-8
Virnstein R. W. (1977). The importance of predation by crabs and fishes on benthic infauna in Chesapeake Bay. Ecology 6), 1199–1217. doi: 10.2307/1935076
Vorsatz J. P. (2009). Ecological role of estuarine brachyuran crabs in mangrove and salt marsh estuaries, Eastern Cape, South Africa (Doctoral dissertation, PhD thesis. University of Port Elizabeth, South Africa.
Walker J. E., Angelini C., Safak I., Altieri A. H., Osborne T. Z. (2019). Effects of changing vegetation composition on community structure, ecosystem functioning, and predator–prey interactions at the saltmarsh-mangrove ecotone. Diversity 11, 208. doi: 10.3390/d11110208
Walker J. B., Grosholz E. D., Long J. D. (2021). Predicting burrowing crab impacts on salt marsh plants. Ecosphere 12, e03803. doi: 10.1002/ecs2.3803
Walther G. R. (2010). Community and ecosystem responses to recent climate change. Philos. Trans. R. Soc. B: Biol. Sci. 365, pp.2019–2024. doi: 10.1098/rstb.2010.0021
Wang Y. I., Naumann U., Wright S. T., Warton D. I. (2012). Mvabund–an R package for model-based analysis of multivariate abundance data. Methods Ecol. Evol. 3, pp.471–pp.474. doi: 10.1111/j.2041-210X.2012.00190.x
Wang J. Q., Zhang X. D., Nie M., Fu C. Z., Chen J. K., Li B. (2008). Exotic Spartina alterniflora provides compatible habitats for native estuarine crab Sesarma dehaani in the Yangtze River estuary. Ecol. Eng. 34, pp.57–pp.64. doi: 10.1016/j.ecoleng.2008.05.015
Warren J. H., Underwood A. J. (1986). Effects of burrowing crabs on the topography of mangrove swamps in New South Wales. J. Exp. Mar. Biol. Ecol. 102, pp.223–pp.235. doi: 10.1016/0022-0981(86)90178-4
Wasson K., Raposa K., Almeida M., Beheshti K., Crooks J. A., Deck A., et al. (2019). Pattern and scale: evaluating generalities in crab distributions and marsh dynamics from small plots to a national scale. Ecology 100, e02813. doi: 10.1002/ecy.2813
Weiskopf S. R., Rubenstein M. A., Crozier L. G., Gaichas S., Griffis R., Halofsky J. E., et al. (2020). Climate change effects on biodiversity, ecosystems, ecosystem services, and natural resource management in the United States. Sci. Total Environ. 733, 137782. doi: 10.1016/j.scitotenv.2020.137782
Whitfield A. K., James N. C., Lamberth S. J., Adams J. B., Perissinotto R., Rajkaran A., et al. (2016). The role of pioneers as indicators of biogeographic range expansion caused by global change in southern African coastal waters. Estuarine Coast. Shelf Sci. 172, 138–153. doi: 10.1016/j.ecss.2016.02.008
Keywords: species distribution, ecotone, poleward expansion, brachyuran diversity, southern limit
Citation: Katharoyan C, Rajkaran A and Peer N (2024) Caught in transition: changes in brachyuran diversity following mangrove encroachment into saltmarshes at a southern distribution limit. Front. Mar. Sci. 11:1389428. doi: 10.3389/fmars.2024.1389428
Received: 22 February 2024; Accepted: 24 May 2024;
Published: 10 June 2024.
Edited by:
Kareen E. Schnabel, National Institute of Water and Atmospheric Research (NIWA), New ZealandReviewed by:
Luzhen Chen, Xiamen University, ChinaCharles Griffiths, University of Cape Town, South Africa
Copyright © 2024 Katharoyan, Rajkaran and Peer. This is an open-access article distributed under the terms of the Creative Commons Attribution License (CC BY). The use, distribution or reproduction in other forums is permitted, provided the original author(s) and the copyright owner(s) are credited and that the original publication in this journal is cited, in accordance with accepted academic practice. No use, distribution or reproduction is permitted which does not comply with these terms.
*Correspondence: Nasreen Peer, cGVlci5uYXNyZWVuQGdtYWlsLmNvbQ==