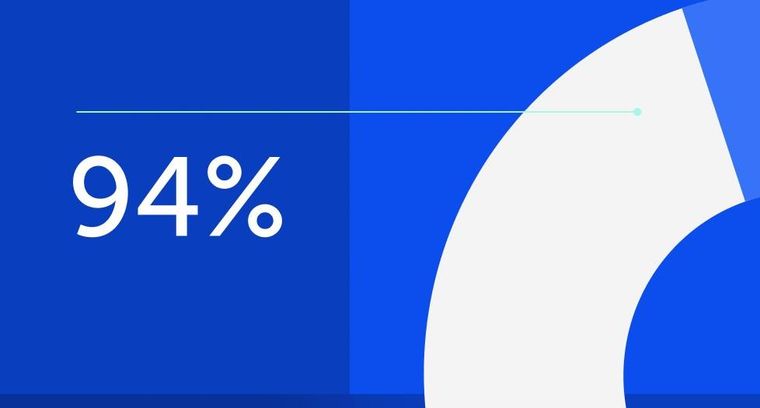
94% of researchers rate our articles as excellent or good
Learn more about the work of our research integrity team to safeguard the quality of each article we publish.
Find out more
ORIGINAL RESEARCH article
Front. Mar. Sci., 10 July 2024
Sec. Aquatic Microbiology
Volume 11 - 2024 | https://doi.org/10.3389/fmars.2024.1388725
This article is part of the Research TopicMarine Microalgae: from Biodiversity to BiotechnologyView all 5 articles
In oligotrophic oceans, primary productivity is widely limited by nitrogen bioavailability. The broadly distributed and abundant nitrogen-fixing cyanobacterium Trichodesmium plays an important role in the oceanic nitrogen and carbon cycles by providing a “new” source of nitrogen to many non-diazotrophic microbes, thereby driving new primary production in the ocean. However, the underlying process and mechanism of nitrogen supply from Trichodesmium to other phytoplankton remain unclear. Here, our results demonstrated that the fixed nitrogen released by Trichodesmium could sustain the growth of a non-nitrogen-fixing cyanobacterium Synechococcus sp. PCC 7002, including a mutant strain (Mut-ureA) that cannot use urea. However, the growth rate of Mut-ureA was approximately 20% lower than that of the wild strain when Trichodesmium filtrate was used for nitrogen supply. This result was consistent with the composition of the Trichodesmium exudate, in which urea comprised more than 20% of the total fixed nitrogen that was released. It is evident from the experiments that a fraction of the Trichodesmium-derived nitrogen was not available to Mut-ureA. Our results suggested that Trichodesmium produces dissolved organic nitrogen, especially a certain amount of urea as a “new” nitrogen source, benefiting in particular populations of surrounding phytoplankton species.
The diazotrophic marine cyanobacterium Trichodesmium is ubiquitous in tropical and subtropical seas, where it fixes both CO2 and N2, making it among the most biogeochemically significant microorganisms in the ocean (Moisander et al., 2010; Zehr, 2011; Walworth et al., 2018). It has been estimated that Trichodesmium may release approximately 30 to 50% of its newly fixed nitrogen (N), providing an important source of bioavailable N to other non-diazotrophic phytoplankton species in the N-limited subtropical marine ecosystems (Glibert and Bronk, 1994; Walworth et al., 2018). The global N input via N2 fixation by Trichodesmium is estimated around 60–80 Tg N annually (Capone et al., 1997; Mahaffey, 2005; Carpenter and Capone, 2008), which contributes substantially to the annual global marine N2 fixation estimated to be around 223 ± 30 Tg N (Shao et al., 2023).
Although Trichodesmium is not the sole diazotrophic cyanobacterium in the open ocean (Sohm et al., 2011; Gradoville et al., 2020; Hutchins and Capone, 2022), it is the most intensively explored cyanobacterium. Since it has been in culture from the early 1990s (Prufert-Bebout et al., 1993), numerous studies have focused on the eco-physiological responses of Trichodesmium to different environmental factors. Despite many studies emphasizing the physiology and biogeographical distribution of Trichodesmium, fewer have examined the fate of the N2 fixed by Trichodesmium (diazotroph-derived N, DDN) in the ocean (Capone et al., 1997). Some of these studies have reported that Trichodesmium blooms may encourage the growth of other non-N-fixing organisms (Padmakumar et al., 2010; Basu et al., 2011). For instance, the abundance of bacteria and the growth of diatoms and dinoflagellates were found to be significantly increased during Trichodesmium blooms in the Eastern Arabian Sea (Padmakumar et al., 2010; Basu et al., 2011). Both field and laboratory data demonstrated that the co-occurrence of Trichodesmium spp. and diatoms might be driven by the transfer of N fixed by Trichodesmium spp. in the N-limited oligotrophic ocean (Chen et al., 2011). Similarly, a previous study showed that a unicellular dinoflagellate, Karenia brevis, could grow well in the culture medium supplemented with Trichodesmium exudates as the sole source of nitrogen (Mulholland, 2007).
In general, Trichodesmium helps sustain marine life via both the active release of key nutrients such as carbon and nitrogen, and passive release processes like cell death, virus-induced lysis, and grazing by heterotrophs. Hence, it plays a crucial role in the biogeochemical cycling of bioactive elements in contemporary oceans (Capone and Carpenter, 1982; Capone et al., 1997). However, it is unknown how the specific forms of nitrogen are transferred by Trichodesmium to non-N-fixing organisms. Moreover, there is a lack of quantitative information regarding the concentrations, composition, and fate of DDN. With the development of techniques such as nanometer scale secondary ion mass spectrometry (nanoSIMS) coupled with 15N2 isotopic labelling and flow cytometry cell sorting, researchers have observed the transfer of DDN to the dissolved N pool and to non-diazotrophic plankton such as diatoms, dinoflagellates, and bacteria (Mulholland et al., 2004; Dore et al., 2008; Lenes and Heil, 2010; Chen et al., 2011). Previous reports have shown that the DDN transfer efficiency of Trichodesmium is higher compared to other diazotrophic cyanobacteria, indicating that Trichodesmium blooms are more efficient in promoting non-diazotrophic production in N-depleted areas (Konno et al., 2010; Benavides et al., 2013; Berthelot et al., 2016; Bonnet et al., 2016). However, this method based on isotopic conversion does not allow for accurate discrimination of nitrogen sources, as well as the assessment of the nitrogen utilization efficiency of non-nitrogen-fixing marine phytoplankton.
In addition to nitrogen-fixing cyanobacteria, many non-nitrogen-fixing cyanobacteria exist in nitrogen-deficient oceans, such as Synechococcus and Prochlorococcus, which are among the most abundant phytoplankton in the ocean (Flombaum et al., 2013). However, it is unclear whether a substantial fraction of nitrogen required for phytoplankton growth comes from DDN released by Trichodesmium. Previous research has concluded that the transfer of DDN to nearby plankton is mediated through the dissolved N pool, as diazotrophs release a significant fraction of their fixed N (10–50%) in the form of ammonium and dissolved organic nitrogen (DON) (Glibert and Bronk, 1994; Mulholland and Bernhardt, 2005; Konno et al., 2010; Benavides et al., 2013; Berthelot et al., 2015; Klawonn et al., 2020; Benavides et al., 2022). These studies suggest that although more than 50% of recently fixed N2 is released by diazotrophs as DON, NH4+ is the main pathway of DDN transfer from diazotrophs to non-diazotrophs. However, NH4+ may not accumulate in the surrounding environment as it could be utilized by the diazotrophs themselves and the surrounding organisms as soon as it is produced (Klawonn et al., 2020). Instead, DON concentrations of 5 to 10 μM are common, making it the most abundant form of dissolved nitrogen (Mulholland et al., 2004; Benavides et al., 2013). This suggests that organic compounds exuded by diazotrophs can fuel primary production, and may have considerable effect on the composition of the plankton community in the oligotrophic ocean. The dominant non-nitrogen-fixing cyanobacteria in these ecosystems, including Synechococcus and Prochlorococcus, possess pathways to take advantage of DON sources, such as urea or dissolved free amino acids (Ludwig and Bryant, 2012; Veaudor et al., 2019; Muñoz-Marín et al., 2020). However, the underlying potential mechanisms have rarely been explored.
Trichodesmium often forms large macroscopic colonies and acts as relatively nutrient-rich substrate for a diverse microbial community through physical attachment and direct colonization in tropical and subtropical oligotrophic oceans (Eichner et al., 2023). A common feature of these associations is the passive transfer of fixed nitrogen (N), such as ammonia, urea, and other DON, from Trichodesmium to epibionts (Mulholland et al., 2004). On the other hand, epibionts assist the host Trichodesmium in iron and phosphorus acquisition, vitamin B12 exchange, small carbon compound catabolism, and detoxification of reactive oxygen species (Lee et al., 2017). Thus, it is difficult to maintain Trichodesmium in culture and unsuccessful to establish stable axenic cultures, due to its obligate dependency on associated organisms. In this study, Trichodesmium erythraeum IMS 101 has been maintained in the laboratory for decades and shown to harbor microbial communities similar to natural microbial populations (Lee et al., 2017). Previous meta-transcriptomics research has detected the community transcripts involved in the reduction of nitrate, nitrite, and nitrous oxide, and suggested that the associated organisms may play an important role in colony-level nitrogen cycling (Lee et al., 2018). To understand the significance of fixed nitrogen released by Trichodesmium to other non-diazotrophic microorganisms, it is prudent to consider the entire Trichodesmium-epibiont assemblage for the stable cohabitation of a diverse community within Trichodesmium consortia.
Although previous studies have shown that Trichodesmium can input nascent nitrogen sources into the habitat, there still lacks direct experimental evidence on whether Trichodesmium can drive phytoplankton growth (Glibert and Bronk, 1994; Mulholland et al., 2004). To investigate under what conditions and in what form Trichodesmium provides nitrogen sources for other cyanobacteria, we studied the growth and nitrogen-fixing gene expression of Trichodesmium under different nitrogen sources as well as nitrogen concentration conditions. The types and amounts of nitrogen sources secreted by the Trichodesmium symbiotic system under nitrogen-deficient conditions were analyzed, and marine Synechococcus sp. strain PCC 7002 was chosen to study the specific forms of nitrogen sources provided by Trichodesmium to other phytoplankton. We also validated this result using a mutant strain that lacked some of the nitrogen source utilization functions. Our results provide experimental evidence that the nitrogen-fixing cyanobacterium Trichodesmium can provide a certain amount of urea to the different species of marine phytoplankton around it, a nitrogen source that has been previously overlooked.
Trichodesmium erythraeum IMS 101 (hereafter Trichodesmium IMS 101) was originally isolated from the Atlantic Ocean (Prufert-Bebout et al., 1993; Chen et al., 1998). Trichodesmium IMS 101 was cultured semi-continuously in YBC-II medium at 28°C, 50 μmol photons·m-2·s-1, and 12:12 L:D photoperiod. The axenic Synechococcus sp. strain PCC 7002 (hereafter Synechococcus 7002) originated from Jindong Zhao’s laboratory (Peking University, China) and the Synechococcus 7002 ureA mutant strain was constructed in our lab. The detailed methods have been described in the previous study of Li et al. (2023). Synechococcus 7002 wild-type and Mut-ureA were cultured in A+ medium under continuous light of 100 μmol photons m-2 s-1, at 30°C, with a rotatory shaker at 110 rpm. All growth media, buffers and solutions used in the experiments were autoclaved or filter sterilized.
Five experiments were conducted in this study:
Experiment 1. Co-culture of Trichodesmium IMS 101 and Synechococcus 7002 were conducted under nitrogen deficient condition. Synechococcus 7002 cells at the logarithmic growth stage were collected, centrifuged, washed and resuspended with nitrogen-free A+ medium to ensure the complete removal of artificially added nitrogen. Then 125 mL of Synechococcus 7002 (cell number about 1.6×107 cells/mL) and 125 mL of Trichodesmium IMS 101 (cell number about 8×103 cells/mL) cultured in N-free YBC II medium were added to 1 L conical flasks. Synechococcus 7002 of the same cell number mixed with fresh YBC-II medium without Trichodesmium IMS 101 served as a control. To determine the nitrogen deficiency status of the cells during long-term co-culture in an incubator with photoperiod (12:12 L:D), the color change of Synechococcus 7002 was observed every 2 days.
Experiment 2. Biomass growth and nifH gene expression were determined during Trichodesmium IMS 101 cultivation in media containing different nitrogen resources in varying concentrations. The stock culture of Trichodesmium IMS 101 was prepared by growing the cells in nitrogen-free YBC-II medium for 7 days. The Trichodesmium cells were then transferred separately into 500 mL flasks containing 300 mL of medium composed of NaNO3, NH4Cl, or urea (with concentrations of 0, 20, 40 to 80 μM, respectively). Culture samples (5 mL) were collected every 3 days for 12 days to measure the biomass of Trichodesmium IMS 101. 100 mL sample of each culture was collected onto a 10 micron polycarbonate (PC) membrane after 24 hours of incubation, and quickly frozen in liquid nitrogen for further analysis of nifH gene expression.
Experiment 3. A complete growth curve of Trichodesmium IMS 101 was drawed and the secreted nitrogen sources and concentrations were measured. Cells were cultivated in nitrogen free YBC-II medium, and the in vivo fluorescence was measured in every three days for 24 days to measure changes in biomass. The culture filtrate was collected on day 12th (late exponential growth phase) to measure the concentrations of the secreted nitrogen sources.
Experiment 4. A nitrogen-deficient Synechococcus 7002 strain was cultured for 10 days, and then its recovery was monitored in the presence of various nitrogen sources. To do this, Synechococcus 7002 stock culture cells were collected during exponential phase and centrifuged. Then the cells were resuspended and washed twice with nitrogen-free A+ medium to ensure the removal of any dissolved nitrogen sources outside of the Synechococcus 7002 cells. The cells were then transferred to fresh nitrogen free A+ medium, and maintained for 10 days to induce the nitrogen-deficient condition. Finally, the nitrogen-deficient cells of Synechococcus 7002 were collected by centrifugation. These cells were then transferred and resuspended into A+ medium containing 150 μM of different types of nitrogen source (NaNO3, NH4Cl, urea or glutamic acid). The recovery rate of Synechococcus 7002 was monitored by determining the biomass of Synechococcus 7002 in the culture suspensions in every 2 days through turbidity measurement (OD730).
Experiment 5. Trichodesmium IMS 101 culture filtrate was used as a sole nitrogen source for wild-type and Mut-ureA Synechococcus 7002 strains. The mutant strain could not utilize urea. The filtrate of Trichodesmium IMS 101 culture was collected on day 12th using a sterile PC membrane (0.22 μm) to remove the filamentous Trichodesmium cells and the associated bacteria. Then a mixture of filtrate and N-free medium (1:1) was used to cultivate wild-type and Mut-ureA nitrogen-deficient Synechococcus 7002 strains at the same time. The biomass contents of wild-type and Mut-ureA Synechococcus 7002 were measured at 0 th, 24 th, 36 th, 48 th, and 72th hours. At the 72nd hour, 5 mL culture of each sample was collected for Chl a measurement.
The growth curve of Trichodesmium IMS 101 was estimated by measuring minimum yields of chlorophyll-a fluorescence (F0) of the culture solution using a FIRe (Fluorescence Induction and Relaxation) Chlorophyll Fluorescence Analyzer (Satlantic, Canada). The biomass of Synechococcus 7002 wild type and mutant strains were determined both by measuring the turbidity (OD730) of individual culture using an ultraviolet-visible spectrophotometer TU1810 (PERSEE, China) and by measuring the cell counts using a CytoFLEX flow cytometer (Beckman Coulter, America). F0 for Trichodesmium IMS 101 and turbidity (OD730) for Synechococcus 7002 were highly correlated with cell counts, of R2 were 0.9931 and 0.9984, respectively (Supplementary Figures S1A, B).
To determine chlorophyll a (Chl a) content, the absorption peaks at 648.6 and 664.1 nm of 95% ethanol extracts were measured using an ultraviolet-visible spectrophotometer TU1810 (PERSEE, China) and calculated according to the formula: Chl a =13.36×A664.1−5.19×A648.6, and the final results were normalized based on the absorbance value of the algal solution at 730 nm (OD730), for details of the method refer by Li et al. (2023).
The total nitrogen content was determined using the alkaline potassium persulfate method (Hagedorn and Schleppi, 2000). Briefly, an alkaline potassium persulfate solution was added to the sample and digested at 120–124°C. The mixed solution was decomposed to potassium bisulfate and atomic nitrogen under high temperatures. The atomic nitrogen not only converts the nitrogenous compounds to nitrate under high temperatures but also oxidizes or decomposes other organic matter in the sample, thus eliminating the interference caused by other nutrient sources. Finally, the absorbance of nitrate was measured by UV-Vis spectrophotometer (210 PLUS) at 220 nm and 275 nm, and the corresponding total nitrogen content was calculated using a standard curve (Supplementary Figure S2A).
The ammonia nitrogen content was measured by using salicylate spectrophotometry (Le and Boyd, 2012). In an alkaline environment (pH 11.7), NH4+ will react chromogenically with salicylate and hypochlorite to produce a blue compound under sodium nitrosoferricyanide as catalyst. The absorbance of this compound was measured at 697 nm, and the ammonia nitrogen content was calculated using a standard curve (Supplementary Figure S2B).
Diacetylmonoxime spectrophotometric method was used to determine the urea content (Chen et al., 2015). Diacetylmonoxime was hydrolyzed under acidic conditions to form diacetyl, which condensed the urea under acidic conditions to produce a red diazine compound. The concentration of urea in the sample corresponded to the color shade of the solution. The maximum absorbance at 525 nm was measured and the urea content in the sample was calculated by using the standard curve (Supplementary Figure S2C).
Trichodesmium IMS 101 cells grown in YBC-II media containing different nitrogen sources for 24 h were collected and quickly frozen in liquid nitrogen. Each sample had three replicates collected from three independent cultures. Total RNA was extracted using the TRIzol reagent kit (Invitrogen, USA). Reverse transcription (RT) was performed using the PrimeScript RT reagent kit (TaKaRa, Japan). RNA extraction and RT-PCR were performed as described previously (Jiang et al., 2012). To detect the gene expression level of nifH, the real-time quantitative PCR was conducted. The primers of nifH gene were as follows: 5’- CAATACGCTCCAGAAGATAACCAA -3’ and 5’- GTAGGAATAGTTAGTTTTTCGTTGTTGATT-3’, which was designed using Primer Premier 5.
Biogeography of Trichodesmium IMS 101 16S gene sequence was explored in a pan-oceanic collection of plankton metagenomes using Tara ocean’s Microbiome Reference Gene Catalog metagenomes (https://tara-oceans.mio.osupytheas.fr/ocean-gene-atlas/). The 16S nucleic acid sequence of Trichodesmium IMS 101 was obtained from NCBI database (https://www.ncbi.nlm.nih.gov/). The expected threshold was set at 1e-100. The abundance of Synechococcus 7002 in each Trichodesmium IMS 101 distribution site was also obtained from Tara ocean’s Microbiome Reference Gene Catalog Metagenomes. Specific details have been previously reported (Vernette et al., 2022; Villar et al., 2018).
Using the DNA of wild-type Synechococcus 7002 as a template, PCR amplification was carried out with ureA-up-F/ureA-up-R and ureA-DN-F/ureA-DN-R primers, respectively, to obtain upstream homologous fragment (ureA-UP) and downstream homologous fragment (ureA-DN) of ureA gene. The recombinant plasmid NBU-KAN-Mut-ureA was obtained by inserting the ureA-UP and ureA-DN fragments into the upstream and downstream segments of kanamycin resistance gene (KanR) of a pUC-MCS-KAN-MCS plasmid vector, respectively, maintaining the orientation of gene sequences. Then, the recombinant plasmid NBU-KAN-Mut-ureA was transformed into the wild-type Synechococcus 7002 to obtain the Mut-ureA strain.
All data were tested for homogeneity of variance and normal distribution, and statistical analyses including Student’s t-test, ANOVA, and Duncan’s multiple range test were performed using the open-source statistical software SPSS 26.0. Values of p < 0.05 were considered statistically significant. Data with significant differences are marked with different lowercase letters or ∗.
To explore the potential co-existing phytoplankton with Trichodesmium, we used the macro-genome database of Tara Ocean to investigate the global distribution of Trichodesmium in the sea and the composition of co-existing planktonic communities. The results showed that Trichodesmium is distributed in low to mid-latitude waters worldwide (Figure 1A), with its highest abundance in several sites of southern Atlantic Ocean (e.g., T_68, T_76, T_78).
Figure 1 (A) Global distribution of Trichodesmium spp. from Tara Ocean datasets; (B) Abundance of Synechococcus 7002 at all stations where Trichodesmium spp. were present; (C) The co-existing phytoplankton at all stations; (D) Different species of cyanobacteria co-existing with Trichodesmium at all sites; (E) The photo of a co-culture of nitrogen-deficient Synechococcus 7002 and Trichodesmium IMS 101. Tara Ocean sites were analyzed using the partial 16S rRNA gene sequence. T_XXX (e.g., T_128) in the figure represents the station number, and the color of the circles represents the abundance at a specific site.
Organisms co-existing with Trichodesmium belonged to various phyla including Proteobacteria, Bacteroidetes, Firmicutes, Cyanobacteria, Actinobacteria, Thermotogae, Planctomycetes, etc, among Cyanobacteria, Synechococcus occupied the dominant co-occurrence with Trichodesmium (Figures 1B–D), the abundance of Synechococcus and Trichodesmium was very strongly correlated at each station (R2 = 0.8343) (Supplementary Figure S3).
The ability of other phytoplankton to directly use the nitrogen source fixed and released by Trichodesmium needed to be confirmed through physiological experiments. As shown in Figure 1E, when Synechococcus 7002 was monocultured with N-free medium, the cells gradually turned yellow and eventually stopped growing, indicating that Synechococcus cells underwent chlorosis. Such distinctive color changes were considered typical of nitrogen deficiency in cyanobacteria (Klotz et al., 2016). However, when Synechococcus 7002 was co-cultured with Trichodesmium in the N-free medium, it is obvious that the addition of Trichodesmium could help sustain the growth of Synechococcus 7002 in N-free medium (Figure 1E). Due to the mixed culture of the two cyanobacteria and the adhere of Synechococcus to Trichodesmium filaments, we were unable to make a specific count of cell numbers for both. But we observed a greater density of Synechococcus 7002 cells as well as a greener cell color (Figure 1E). Thus, we hypothesized that Trichodesmium might produce some nitrogen sources and support the growth of Synechococcus 7002. In this context, we conducted a series of experiments focused on what forms of nitrogen sources in Trichodesmium N-free culture system could support the growth of Synechococcus 7002.
Trichodesmium can not only obtain nitrogen source through biological nitrogen fixation, but also directly uptake and assimilate combined nitrogen. To understand the preference of Trichodesmium for nitrogen sources and the influence of different nitrogen sources on nitrogen fixation genes, we carried out the following research. As shown in Figure 2, low concentrations of nitrogen (5 or 10 μM) did not affect the cell growth of Trichodesmium IMS 101. However, high concentrations of inorganic nitrogen (40 and 80 μM NaNO3 or NH4Cl) significantly inhibited the growth of Trichodesmium IMS 101 (p < 0.05), especially in presence of NH4Cl in high concentration (Figures 2A, B). In contrast, the addition of urea did not significantly affect the cell growth compared with the control without any nitrogen. The cell growth increased significantly at the high urea concentration of 80 μM (p < 0.05) (Figure 2C).
Figure 2 The growth curves of Trichodesmium IMS 101 in presence of different nitrogen sources (n=3). (A–C) are the growth curves of Trichodesmium IMS 101 under different concentrations of NaNO3, NH4Cl, and Urea, respectively. The pictures of culture plates were taken on the 9th day of cultivation. The error bar represents the standard deviation between the three replicates, (p < 0.05).
When the nitrogen sources were added into the Trichodesmium IMS 101 culture at lower concentrations (< 20 μM), no significant difference in the growth of Trichodesmium was observed. However, significant down-regulation of nifH gene expression in Trichodesmium IMS 101 was observed with increasing nitrogen concentration, indicating the relatively lower nitrogen fixation rate of Trichodesmium IMS 101 under high nitrogen supply (Figure 3). Although all the three added nitrogen sources inhibited the expression of nifH gene, more inhibitory effects were observed after the supply of inorganic nitrogen (NaNO3 and NH4Cl), as compared with the organic nitrogen (urea) supply. NH4Cl exhibited the most significant inhibiting effect on nifH gene expression, especially at high concentrations, which was consistent with its inhibitory effect on the growth of Trichodesmium IMS 101.
Figure 3 nifH gene expression in Trichodesium IMS 101 grown for 24 h in YBC-II media supplemented with different nitrogen sources. The error bar represents the standard deviation of the three replicates. Significance analysis was based on Duncan’s multiple range test and marked with lowercase letters, with different letters representing significant differences in nifH gene expression between different nitrogen source treatments, (p < 0.05).
The growth curve of Trichodesmium IMS 101 cultured in YBC medium (no N supply) included three growth phases: exponential growth phase, plateau phase, and decline phase (Figure 4A). The exponential growth phase lasted for 12 days with a specific growth rate at ~0.1 d-1 (Figure 4A). The plateau phase lasted for 6 days and sharply dropped into the decline phase (Figure 4A). In addition, the results showed that the main form of nitrogen source present in the culture filtrate at day 12th was DON, with urea accounting for a relatively considerable proportion of DON (more than 25%) and total nitrogen (around 20%). Other forms such as amino acids and proteins accounted for the majority of DON. Inorganic nitrogen forms, such as NH4+, accounted for only a small proportion of the total nitrogen, which was far less than urea (Figure 4B).
Figure 4 The complete growth curve of Trichodesmium IMS 101 including exponential phase, plateaus and decline phase (A); and the types and concentrations of different nitrogen forms secreted by Trichodesmium IMS 101 during late exponential phase (B). The error bar represents the standard deviation of three replicates. Significance analysis was based on Duncan’s multiple range test and marked with lowercase letters, with different letters representing significant differences between the contents of different nitrogen type, (p < 0.05).
As shown in Figure 5A, Synechococcus 7002 cells cultured without nitrogen showed chlorosis after day 10th. When nitrogen deprivation was further prolonged, the cells of Synechococcus 7002 entered a dormant state and stopped growing and dividing. However, upon encountering an external nitrogen source, the cells immediately initiated a recovery program (Figures 5A, B). When nitrogen sources such as NO3-, NH4+, urea and glutamate were provided to the nitrogen-deficient Synechococcus 7002, both inorganic and organic nitrogen sources were absorbed and utilized by Synechococcus 7002 for cell division and reproduction (Figure 5C). Synechococcus 7002 preferred to use urea and NH4+ as the nitrogen source rather than NO3- and glutamate (Figure 5B). These results indicate that Synechococcus 7002 may serve as an ideal model to study the utilization of nitrogen secreted by Trichodesmium.
Figure 5 Growth rates and photographs of nitrogen-deficient Synechococcus 7002 after addition of different nitrogen sources: (A) growth curves and photographs of Synechococcus 7002 recovered by the addition of nitrogen after 10 days of nitrogen deficiency (added 12 mM NaNO3 on day 10); (B) growth rates after supply of different nitrogen sources to nitrogen-deficient Synechococcus 7002; (C) photos of Synechococcus 7002 taken at different days after supply of different nitrogen sources. Error bars represent the standard deviation of three replicates. Significance analysis was based on Duncan’s multiple range test and marked with lowercase letters, with different letters representing significant differences between growth rates obtained from different nitrogen sources supplied, (p < 0.05).
After the addition of Trichodesmium IMS 101 filtrate to the nitrogen-deficient Synechococcus 7002 culture, the growth curves and growth rates of Synechococcus 7002 showed a significant increase compared to the control treatment with the addition of nitrogen-free YBC-II medium (Figures 6A, C). Moreover, the cellular Chl a content of Synechococcus 7002 increased significantly (p < 0.01) after 72 h of the addition of Trichodesmium IMS 101 filtrate, whereas the Chl a content of Synechococcus 7002 added with nitrogen-free YBC-II medium decreased further, even though the cells could still grow slowly (Figure 6E). These results suggest that the filtrate of Trichodesmium IMS 101 can restore the growth of nitrogen-starved Synechococcus 7002 in N-free medium.
Figure 6 (A, C, E) show the effects of Trichodesmium IMS 101 filtrate addition on biomass accumulation, growth rate, and Chl a content of nitrogen-deficient Synechococcus 7002, respectively. (B, D) show the effects of Trichodesmium IMS 101 filtrate addition on nitrogen-deficient Synechococcus 7002 urease mutant strain Mut-ureA on biomass accumulation and growth rate, respectively. Error bars represent the standard deviation of three replicates. Significance analysis was based on Duncan’s multiple range test and marked with ∗ (∗ P < 0.05, ∗∗ P < 0.01, ∗∗∗ P < 0.001, n.s., not significant).
To investigate whether the abundant urea in the Trichodesmium filtrate can be utilized by Synechococcus 7002, a Synechococcus 7002 urease mutant strain (Mut-ureA) was obtained using a constructed homologous double-exchange plasmid (Supplementary Figures S4A–C). By using different types of nitrogen sources, it was confirmed that Mut-ureA completely lost its urea utilization ability but still had a normal ability to take up and utilize other forms of nitrogen sources (Supplementary Figures S4D–F)
The growth curves and growth rates of chronic nitrogen-deficient Synechococcus 7002 Mut-ureA strain were also measured after the addition of the same Trichodesmium IMS 101 filtrate and the N free YBC-II medium respectively. The result showed that the cells growth and the pigment content of the Synechococcus 7002 Mut-ureA strain (p < 0.01) were also increased significantly with the addition of Trichodesmium filtrate. However, its total biomass was lower than that of the wild-type strain (Figures 6B, D). Together, the results indicated that there were multiple nitrogen sources from the Trichodesmium filtrate could be used by Synechococcus 7002, but urea contributed at least part of the total bioavaible nitrogen. Since the Mut-ureA strain lost the urea utilization ability, its growth restoration was slower compared to the wild type strain when they using the N sources released by Trichodesmium IMS 101 filtrate. The growth difference of 20% was consistent with the urea content accounting for 20% of the total released nitrogen in Trichodesmium IMS 101 filtrate (Figure 4B). Overall, these results indicate that the nitrogen secreted by Trichodesmium plays a crucial role in supporting the growth recovery of chronically nitrogen-deficient Synechococcus 7002, and urea might contribute a similar fraction of nitrogen source.
Trichodesmium may fix dinitrogen (N2) in the oligotrophic near-surface regions of ocean, alleviating N limitation and encouraging a more diverse plankton community. The nitrogen fixed by Trichodesmium has been predicted to provide up to 50% of the new nitrogen-supporting open-ocean food webs in the subtropical North Pacific Gyre (Dore et al., 2002). Many reports have demonstrated that Trichodesmium fixes nitrogen under nitrogen deficient conditions and high concentrations of exogenous inorganic nitrogen in the environment may significantly decrease the N2 fixation rate of Trichodesmium (Holl and Montoya, 2005; Sandh et al., 2011; Eichner et al., 2014; Walworth et al., 2018). To date, most diazotrophic nitrogen assimilation studies have focused on the relationship between N2 fixation and uptake of nitrate, ammonia, and amino acid (Ohki et al., 1991; Mulholland et al., 1999, Mulholland et al., 2001). The absorption and utilization characteristics of Trichodesmium for various nitrogen compounds are largely unknown. In this study, the growth and expression of nifH gene (encodes nitrogen-fixing enzymes of Trichodesmium) were analyzed in the presence of different types of nitrogen sources in varying concentrations. The results demonstrated that urea (common DON in the water) supply at a certain concentration had little effect on nifH gene expression in Trichodesmium, as compared with the similar concentration of NH4+ and NO3- (mainly dissolved inorganic nitrogen (DIN) in the water) supply. Moreover, urea supply even could promote the growth of Trichodesmium. Similarly, recent research has demonstrated that carbon-, nitrogen- and phosphorus-rich dissolved organic matters (DOMs) enhance N2 fixation rates and nifH gene expression in natural Trichodesmium colonies (Stolte et al., 2006; Letscher and Moore, 2015; Benavides et al., 2018). It was speculated that in the presence of exogenous DON, Trichodesmium could continue to fix nitrogen and release new nitrogen sources to improve primary productivity in marine ecosystems.
In this study, the results demonstrated that DON accounted for the majority, while NH4+ contributed to only a small proportion of Trichodesmium derived N left in the filtrate in the late exponential growth phase. This result was consistent with previous studies reporting low DIN and high DON in the culture filtrate of Trichodesmium (Mulholland et al., 2001; Glibert et al., 2008). It must be noticed that whether in the lab culture or the field, we should consider the entire Trichodesmium-epibiont assemblage as a whole for the stable cohabitation of a diverse community within Trichodesmium consortia. Thus, the main form of nitrogen source present in the filtrate was DON after equilibrium between nitrogen fixation by Trichodesmium and consumption of nitrogen source by the Trichodesmium consortia. However, compared to the released DON, the remaining NH4+ concentration in the surrounding environment of Trichodesmium was extremely low (Berthelot et al., 2016). This phenomenon may be explained by the rapid release and uptake of NH4+ by the Trichodesmium community as a potential major recycling intermediate of Trichodesmium-fixed N2 in the culture system, which facilitates the rapid turnover of the nitrogen pool and leaves little residue in the culture medium (Mulholland et al., 1999, 2001). Whereas DON was the main nitrogen resource accumulated in the medium, contributing a large fraction of the N pool that could be utilized by other microorganisms in the surrounding environment.
Few studies evaluated the bioavailable proportion of Trichodesmium-derived DON and the compounds within the uncharacterized DON pool that were utilized by other phytoplankton (Mulholland et al., 2004). DON has been largely understood by extrapolating the data obtained from the studies of dissolved organic carbon flow or through studies of individual compounds, such as urea or dissolved free amino acids (Glibert and Bronk, 1994; Mulholland et al., 2004; Finzi-Hart et al., 2009). In the present study, the nitrogen forms released by Trichodesmium were evaluated in the culture medium without exogenous N and the proportion of urea in the total nitrogen was found to be more than 20%. These results indicate that urea may play an important role in marine nitrogen cycle and serve as nitrogen resource for transferring nitrogen to auto- and heterotrophic plankton communities in the surrounding environment.
Many non-nitrogen-fixing cyanobacteria, such as Synechococcus and Prochlorococcus, co-exist with nitrogen-fixing cyanobacteria in nitrogen-deficient oceans (Flombaum et al., 2013; Masuda et al., 2022). Synechococcus 7002, originally isolated from the mud of the Atlantic coast, can be genetically manipulated and grown in axenic cultures. Recent studies have shown that the distribution of Synechococcus in coastal regions is far more extensive than previously thought (Lee et al., 2017; Yong et al., 2023). By using the macro-genome database of Tara Ocean, the presence of Synechococcus 7002 has been detected in the sights where Trichodesmium exists. This shows that Trichodesmium and Synechococcus 7002 (at least strains similar to Synechococcus 7002 due to the inaccuracy of bioinformatics prediction) possibly have a close association in the oceans (Figure 1; Supplementary Figure S3). To further reveal the potential interaction between Trichodesmium and the “consumer” of its secreted nitrogen, Synechococcus 7002 was co-cultured with Trichodesmium and its secreted filtrate was used as the sole nitrogen resource. When Trichodesmium reached a dynamic equilibrium with Synechococcus cells in the culture system, both in terms of biomass and nitrogen turnover, chronically nitrogen-deficient Synechococcus 7002 recovered from chlorosis to green cells (Figure 1E). This implied that nitrogen fixation by Trichodesmium provided an important source of nitrogen for the recovery of Synechococcus 7002. In mono-cultures, both Synechococcus 7002 and Trichodesmium preferred urea as a nitrogen source than other inorganic nitrogen sources (e.g., ammonium and nitrate). Inhibition of Trichodesmium growth was observed at high dose of exogenous inorganic nitrogen (NO3-, NH4+), but the growth increased at a higher concentration of urea. Synechococcus 7002 showed good recovery after a prolonged period of nitrogen deficiency, when exogenous nitrogen sources were supplied. Among the added exogenous nitrogen sources, ammonium and urea were preferred by Synechococcus 7002, which was consistent with the previous study reporting ammonia as the preferred microbial nitrogen source for most, if not all, cyanobacteria (Walworth et al., 2018). After the addition of Trichodesmium IMS 101 filtrate to Synechococcus 7002 culture, the biomass of nitrogen-deficient dormant Synechococcus 7002 also increased significantly (Figure 6). These results suggest that the nitrogen source released by Trichodesmium can be directly utilized by Synechococcus 7002 for its growth.
Some studies investigated the transfer of diazotroph-derived nitrogen to non-diazotrophic planktonic communities (Chen et al., 2011; Sipler et al., 2013). For instance, Chen et al. (2011) conducted 15N tracing experiments and revealed the transfer of newly fixed N from Trichodesmium to diatoms. Sipler et al. (2013) used the FT-ICR MS method to detect the changes in DON concentration and the composition of N compound showed that Trichodesmium sp. provided a sufficient source of nitrogen to directly or indirectly support Karenia brevis blooms. However, the potential mechanism involved in the transfer of Trichodesmium-derived DON such as urea and amino acids to non-diazotrophic plankton need to be further studied at physiological and molecular level. In the present study, the biomass of Mut-ureA strain was around 20% lower than the biomass of the wild type Synechococcus. This result indicated that urea provided a fraction of nitrogen source that Synechococcus could obtain from Trichodesmium filtrate. The physiological study of genetic mutants provided direct evidence that chronically nitrogen-starved marine Synechococcus was able to access urea (produced during nitrogen fixation by Trichodesmium) as the nitrogen source, although other forms of nitrogen released by Trichodesmium also had positive effects on the growth of Synechococcus, which account for ~80% of the biomass accumulation. A previous study examined the transcriptional profile of cyanobacteria co-existing in Trichodesmium populations, which was primarily dominated by Synechococcus (Lee et al., 2018). The study reported that the cyanobacteria allocated more of their transcriptional pool to ammonium (amtB) and urea (urtA) transport, with protein products also detected during urea transport, indicating the potential utilization of Trichodesmium-derived urea by Synechococcus (Lee et al., 2018). In the present study, wild-type and Mut-ureA strains of Synechococcus 7002 were used in this study to understand the relationship between Trichodesmium and non-nitrogen-fixing Synechococcus linked by the fixed and released nitrogen of Trichodesmium. The results showed that the Trichodesmium-derived urea contributed at least part of the nitrogen source for the growth of phytoplankton populations. This suggests that urea exuded by diazotrophs can fuel the primary production and may have a considerable impact on the composition of the plankton community in the oligo-trophic oceans.
Long-term evolution has led to the development of different nitrogen uptake and utilization mechanisms as well as the nitrogen source preferences of various marine phytoplankton. This may challenge the potential of Trichodesmium as an important supplier of nitrogen sources to promote marine primary productivity (Moore et al., 2002; Zubkov et al., 2003; Esteves-Ferreira et al., 2018; Li et al., 2023). This study emphasized the crucial role of Trichodesmium-derived DON, especially urea, as new nitrogen to support the growth of phytoplankton in the surrounding environment, represents an important pathway of N and C export to the deeper parts of tropical and subtropical oceans. Further studies are needed to investigate the fate of other dominant Trichodesmium-derived N forms and to highlight the complex interactions of diazotrophs with their environment.
The datasets presented in this study can be found in online repositories. The names of the repository/repositories and accession number(s) can be found in the article/Supplementary Material.
S-QL: Conceptualization, Data curation, Formal analysis, Investigation, Methodology, Writing – original draft, Writing – review & editing. YX: Data curation, Formal analysis, Investigation, Methodology, Writing – review & editing. H-LH: Data curation, Investigation, Methodology, Validation, Visualization, Writing – review & editing. ZL: Formal analysis, Investigation, Methodology, Software, Writing – review & editing. T-RS: Formal analysis, Investigation, Software, Writing – review & editing. H-YG: Data curation, Investigation, Methodology, Software, Writing – review & editing. X-WW: Conceptualization, Funding acquisition, Resources, Supervision, Validation, Visualization, Writing – original draft, Writing – review & editing. H-BJ: Conceptualization, Funding acquisition, Supervision, Writing – original draft, Writing – review & editing.
The author(s) declare financial support was received for the research, authorship, and/or publication of this article. This study was funded by the Science and Technology Innovation 2025 Major Project of Ningbo City (Grant No. 2022Z189), National Natural Science Foundation of China (Grant No. 32170108, No. 42188102, No.91951111 and No. 42206083) and the National Natural Science Foundation of Zhejiang Province (Grant No. LQ21D060004).
We are very thankful to Baosheng Qiu (Central China Normal University), David A. Hutchins and Feixue Fu (University of Southern California) for their help and advices for this study.
The authors declare that the research was conducted in the absence of any commercial or financial relationships that could be construed as a potential conflict of interest
All claims expressed in this article are solely those of the authors and do not necessarily represent those of their affiliated organizations, or those of the publisher, the editors and the reviewers. Any product that may be evaluated in this article, or claim that may be made by its manufacturer, is not guaranteed or endorsed by the publisher.
The Supplementary Material for this article can be found online at: https://www.frontiersin.org/articles/10.3389/fmars.2024.1388725/full#supplementary-material
Basu S., Matondkar S. G. P., Furtado I. (2011). Enumeration of bacteria from a Trichodesmium spp. bloom of the Eastern Arabian Sea: elucidation of their possible role in biogeochemistry. J. Appl. Phycol 23, 309–319. doi: 10.1007/s10811-010-9589-4
Benavides M., Agawin N., Arístegui J., Peene J., Stal L. (2013). Dissolved organic nitrogen and carbon release by a marine unicellular diazotrophic cyanobacterium. Aquat. Microb. Ecol. 69, 69–80. doi: 10.3354/ame01621
Benavides M., Bonnet S., Le Moigne F. A. C., Armin G., Inomura K., Hallstrøm S., et al. (2022). Sinking Trichodesmium fixes nitrogen in the dark ocean. ISME J. 16, 2398–2405. doi: 10.1038/s41396-022-01289-6
Benavides M., Martias C., Elifantz H., Berman-Frank I., Dupouy C., Bonnet S. (2018). Dissolved organic matter influences n2 fixation in the New Caledonian lagoon (Western Tropical South Pacific). Front. Mar. Sci. 5. doi: 10.3389/fmars.2018.00089
Berthelot H., Bonnet S., Camps M., Grosso O., Moutin T. (2015). Assessment of the dinitrogen released as ammonium and dissolved organic nitrogen by unicellular and filamentous marine diazotrophic cyanobacteria grown in culture. Front. Mar. Sci. 2. doi: 10.3389/fmars.2015.00080
Berthelot H., Bonnet S., Grosso O., Cornet V., Aude B. (2016). Transfer of diazotroph-derived nitrogen towards non-diazotrophic planktonic communities: a comparative study between Trichodesmium erythraeum, Crocosphaera watsonii and Cyanothece sp. Biogeosciences 13, 4005–4021. doi: 10.5194/bg-13-4005-2016
Bonnet S., Berthelot H., Turk-Kubo K., Cornet-Barthaux V., Fawcett S., Berman-Frank I., et al. (2016). Diazotroph derived nitrogen supports diatom growth in the South West Pacific: A quantitative study using nanoSIMS: Transfer of diazotrophic N into plankton. Limnol Oceanogr 61, 1549–1562. doi: 10.1002/lno.10300
Capone D. G., Carpenter E. (1982). Nitrogen fixation in the marine environment. J. Science 217, 1140–1142. doi: 10.1126/science.217.4565.1140
Capone D. G., Zehr J. P., Paerl H. W., Bergman B., Edward J. (1997). Trichodesmium, a globally significant marine cyanobacterium. Science 276, 1221–1229. doi: 10.1126/science.276.5316.1221
Carpenter E. J., Capone D. G. (2008)Chapter 4 - nitrogen fixation in the marine environment in Nitrogen in the marine environment, 2nd ed.Eds. Capone D. G., Bronk D. A., Mulholland M. R., Carpenter E. J. (Academic Press, San Diego), 141–198.
Chen L., Ma J., Huang Y., Dai M., Li X. (2015). Optimization of a colorimetric method to determine trace urea in seawater: Trace urea analysis in seawater. Limnol Oceanogr-meth 13, 303–311. doi: 10.1002/lom3.10026
Chen Y.-B., Dominic B., Mellon M. T., Zehr J. P. (1998). Circadian rhythm of nitrogenase gene expression in the diazotrophic filamentous nonheterocystous cyanobacterium Trichodesmium sp. Strain IMS 101. J. Bacteriol 180, 3598–3605. doi: 10.1128/JB.180.14.3598-3605.1998
Chen Y. L. L., Tuo S., Chen H. (2011). Co-occurrence and transfer of fixed nitrogen from Trichodesmium spp. to diatoms in the low-latitude kuroshio current in the NW Pacific. Mar. Ecol. Prog. Ser. 421, 25–38. doi: 10.3354/meps08908
Dore J. E., Brum J. R., Tupas L. M., Karl D. M. (2002). Seasonal and interannual variability in sources of nitrogen supporting export in the oligotrophic subtropical North Pacific Ocean. Limnol. Oceanogr. 47, 1595–1607. doi: 10.4319/lo.2002.47.6.1595
Dore J. E., Letelier R. M., Church M. J., Lukas R., Karl D. M. (2008). Summer phytoplankton blooms in the oligotrophic North Pacific Subtropical Gyre: Historical perspective and recent observations. Prog. Oceanogr 76, 2–38. doi: 10.1016/j.pocean.2007.10.002
Eichner M., Inomura K., Pierella Karlusich J. J., Shaked Y. (2023). Better together? Lessons on sociality from Trichodesmium. Trends Microbiol. 31, 1072–1084. doi: 10.1016/j.tim.2023.05.001
Eichner M., Kranz S. A., Rost B. (2014). Combined effects of different CO2 levels and N sources on the diazotrophic cyanobacterium Trichodesmium. Physiol. Plantarum 152, 316–330. doi: 10.1111/ppl.12172
Esteves-Ferreira A. A., Inaba M., Fort A., Araújo W. L., Sulpice R. (2018). Nitrogen metabolism in cyanobacteria: metabolic and molecular control, growth consequences and biotechnological applications. Crit. Rev. Microbiol. 44, 541–560. doi: 10.1080/1040841X.2018.1446902
Finzi-Hart J. A., Pett-Ridge J., Weber P. K., Popa R., Fallon S. J., Gunderson T., et al. (2009). Fixation and fate of C and N in the cyanobacterium Trichodesmium using nanometer-scale secondary ion mass spectrometry. Proc. Natl. Acad. Sci. U.S.A. 106, 6345–6350. doi: 10.1073/pnas.0810547106
Flombaum P., Gallegos J. L., Gordillo R. A., Rincón J., Zabala L. L., Jiao N., et al. (2013). Present and future global distributions of the marine Cyanobacteria Prochlorococcus and Synechococcus. Proc. Natl. Acad. Sci. U.S.A. 110, 9824–9829. doi: 10.1073/pnas.1307701110
Glibert P. M., Bronk D. (1994). Release of dissolved organic nitrogen by marine diazotrophic cyanobacteria. A. Trichodesmium Appl. Environ. Microbiol. 60, 3996–4000. doi: 10.1128/aem.60.11.3996-4000.1994
Glibert P. M., Azanza R., Burford M., Furuya K., Abal E., Al-Azri A., et al. (2008). Ocean urea fertilization for carbon credits poses high ecological risks. Mar. pollut. Bull. 56, 1049–1056. doi: 10.1016/j.marpolbul.2008.03.010
Gradoville M. R., Farnelid H., White A. E., Turk-Kubo K. A., Stewart B., Ribalet F., et al. (2020). Latitudinal constraints on the abundance and activity of the cyanobacterium UCYN-A and other marine diazotrophs in the North Pacific. Limnol Oceanogr 65, 1858–1875. doi: 10.1002/lno.11423
Hagedorn F., Schleppi P. (2000). Determination of total dissolved nitrogen by persulfate oxidation. J. Plant Nutr. Soil Sci. 163, 81–82. doi: 10.1002/(sici)1522-2624(200002)163:1<81::aid-jpln81>3.0.co;2-1
Holl C. M., Montoya J. P. (2005). Interactions between nitrate uptake and nitrogen fixation in continuous cultures of the marine diazotroph Trichodesmium (cyanobacteria). J. Phycol 41, 1178–1183. doi: 10.1111/j.1529-8817.2005.00146.x
Hutchins D. A., Capone D. (2022). The marine nitrogen cycle: new developments and global change. G. Nat. Rev. Microbiol. 20, 401–414. doi: 10.1038/s41579-022-00687-z
Jiang H.-B., Lou W.-J., Du H.-Y., Price N. M., Qiu B.-S. (2012). Sll1263, a unique cation diffusion facilitator protein that promotes iron uptake in the cyanobacterium Synechocystis sp. strain PCC 6803. Plant Cell Physiol. 53, 1404–1417. doi: 10.1093/pcp/pcs086
Klawonn I., Eichner M. J., Wilson S. T., Moradi N., Thamdrup B., Kümmel S., et al. (2020). Distinct nitrogen cycling and steep chemical gradients in Trichodesmium colonies. ISME J. 14, 399–412. doi: 10.1038/s41396-019-0514-9
Klotz A., Georg J., Bučinská L., Watanabe S., Reimann V., Januszewski W., et al. (2016). Awakening of a dormant cyanobacterium from nitrogen chlorosis reveals a genetically determined program. Curr. Biol. 26, 2862–2872. doi: 10.1016/j.cub.2016.08.054
Konno U., Tsunogai U., Komatsu D. D., Daita S., Nakagawa F., Tsuda A., et al. (2010). Determination of total N2 fixation rates in the ocean taking into account both the particulate and filtrate fractions. Biogeosciences 7, 2369–2377. doi: 10.5194/bg-7-2369-2010
Le P. T. T., Boyd C. E. (2012). Comparison of phenate and salicylate methods for determination of total ammonia nitrogen in freshwater and saline water. J. World Aquaculture Soc. 43, 885–889. doi: 10.1111/j.1749-7345.2012.00616.x
Lee M. D., Walworth N. G., McParland E. L., Fu F.-X., Mincer T. J., Levine N. M., et al. (2017). The Trichodesmium consortium: conserved heterotrophic co-occurrence and genomic signatures of potential interactions. ISME J. 11, 1813–1824. doi: 10.1038/ismej.2017.49
Lee M. D., Webb E. A., Walworth N. G., Fu F.-X., Held N. A., Saito M. A., et al. (2018). Transcriptional activities of the microbial consortium living with the marine nitrogen-fixing cyanobacterium Trichodesmium reveal potential roles in community-level nitrogen cycling. Appl. Environ. Microb. 84, e02026–e02017. doi: 10.1128/AEM.02026-17
Lenes J. M., Heil C. A. (2010). A historical analysis of the potential nutrient supply from the N2 fixing marine cyanobacterium Trichodesmium spp. to Karenia brevis blooms in the eastern gulf of Mexico. J. Plankton Res. 32, 1421–1431. doi: 10.1093/plankt/fbq061
Letscher R. T., Moore J. K. (2015). Preferential remineralization of dissolved organic phosphorus and non-Redfield DOM dynamics in the global ocean: Impacts on marine productivity, nitrogen fixation, and carbon export. Global Biogeochem Cy 29, 325–340. doi: 10.1002/2014GB004904
Li S.-Q., Huang H.-L., Sun T.-R., Gao H.-Y., Wang X.-W., Fu F.-X., et al. (2023). Cyanobacteria using urea as a nitrogen source can overcome acid stress. bioRxiv 2023, 3.29.534730. doi: 10.1101/2023.03.29.534730
Ludwig M., Bryant D. A. (2012). Acclimation of the global transcriptome of the Cyanobacterium Synechococcus sp. Strain PCC 7002 to nutrient limitations and different nitrogen sources. Front. Microbio. 3. doi: 10.3389/fmicb.2012.00145
Mahaffey C. (2005). The conundrum of marine N2 fixation. Am. J. Sci. 305, 546–595. doi: 10.2475/ajs.305.6-8.546
Masuda T., Inomura K., Kodama T., Shiozaki T., Kitajima S., Armin G., et al. (2022). Crocosphaera as a major consumer of fixed nitrogen. Microbiol. Spectr. 10, e02177–e02121. doi: 10.1128/spectrum.02177-21
Moisander P. H., Beinart R. A., Hewson I., White A. E., Johnson K. S., Carlson C. A., et al. (2010). Unicellular cyanobacterial distributions broaden the oceanic N2 fixation domain. Science 327, 1512–1514. doi: 10.1126/science.1185468
Moore L. R., Post A. F., Rocap G., Chisholm S. W. (2002). Utilization of different nitrogen sources by the marine cyanobacteria Prochlorococcus and Synechococcus. Limnol. Oceanogr. 47, 989–996. doi: 10.4319/lo.2002.47.4.0989
Mulholland M. R. (2007). The fate of nitrogen fixed by diazotrophs in the ocean. Biogeosciences 4, 37–51. doi: 10.5194/bg-4-37-2007
Mulholland M. R., Bernhardt P. W. (2005). The effect of growth rate, phosphorus concentration, and temperature on N2 fixation, carbon fixation, and nitrogen release in continuous cultures of Trichodesmium IMS 101. Limnol Oceanogr 50, 839–849. doi: 10.4319/lo.2005.50.3.0839
Mulholland M., Bronk D., Capone D. (2004). Dinitrogen fixation and release of ammonium and dissolved organic nitrogen by Trichodesmium IMS 101. Aquat. Microb. Ecol. 37, 85–94. doi: 10.3354/ame037085
Mulholland M. R., Ohki K., Capone D. G. (1999). Nitrogen utilization and metabolism relative to patterns of N2 fixation in cultures of Trichodesmium Nibb1067. J. Phycology 35, 977–988. doi: 10.1046/j.1529-8817.1999.3550977.x
Mulholland M. R., Ohki K., Capone D. G. (2001). Nutrient controls on nitrogen uptake and metabolism by natural populations and cultures of Trichodesmium (cyanobacteria). J. Phycol 37, 1001–1009. doi: 10.1046/j.1529-8817.2001.00080.x
Muñoz-Marín M. C., Gómez-Baena G., López-Lozano A., Moreno-Cabezuelo J. A., Díez J., García-Fernández J. M. (2020). Mixotrophy in marine picocyanobacteria: use of organic compounds by Prochlorococcus and Synechococcus. ISME J. 14, 1065–1073. doi: 10.1038/s41396-020-0603-9
Ohki K., Zehr J. P., Falkowski P. G., Fujita Y. (1991). Regulation of nitrogen-fixation by different nitrogen sources in the marine non-heterocystous cyanobacterium Trichodesmium sp. NIBB1067. Arch. Microbiol. 156, 335–337. doi: 10.1007/BF00248706
Padmakumar K. B., Smitha B. R., Thomas L. C., Fanimol C. L., SreeRenjima G., Menon N. R., et al. (2010). Blooms of Trichodesmium erythraeum in the south eastern Arabian Sea during the onset of 2009 summer monsoon. Ocean Sci. J. 45, 151–157. doi: 10.1007/s12601-010-0013-4
Prufert-Bebout L., Paerl H. W., Lassen C. (1993). Growth, Nitrogen fixation, and spectral attenuation in cultivated Trichodesmium species. Appl. Environ. Microb. 59, 1367–1375. doi: 10.1128/aem.59.5.1367-1375.1993
Sandh G., Ran L., Xu L., Sundqvist G., Bulone V., Bergman B. (2011). Comparative proteomic profiles of the marine cyanobacterium Trichodesmium erythraeum IMS 101 under different nitrogen regimes. Proteomics 11, 406–419. doi: 10.1002/pmic.201000382
Shao Z., Xu Y., Wang H., Luo W., Wang L., Huang Y., et al. (2023). Global oceanic diazotroph database version 2 and elevated estimate of global oceanic N2 fixation. Earth Syst. Sci. Data 15, 3673–3709. doi: 10.5194/essd-15-3673-2023
Sipler R. E., Bronk D. A., Seitzinger S. P., Lauck R. J., McGuinness L. R., Kirkpatrick G. J., et al. (2013). Trichodesmium-derived dissolved organic matter is a source of nitrogen capable of supporting the growth of toxic red tide Karenia brevis. Mar. Ecol. Prog. Ser. 483, 31–45. doi: 10.3354/meps10258
Sohm J. A., Webb E. A., Capone D. G. (2011). Emerging patterns of marine nitrogen fixation. Nat. Rev. Microbiol. 9, 499–508. doi: 10.1038/nrmicro2594
Stolte W., Balode M., Carlsson P., Grzebyk D., Janson S., Lips I., et al. (2006). Stimulation of nitrogen-fixing cyanobacteria in a Baltic Sea plankton community by land-derived organic matter or iron addition. Mar. Ecol. Prog. Ser. 327, 71–82. doi: 10.3354/meps327071
Veaudor T., Cassier-Chauvat C., Chauvat F. (2019). Genomics of urea transport and catabolism in cyanobacteria: biotechnological implications. Front. Microbiol. 10. doi: 10.3389/fmicb.2019.02052
Vernette C., Lecubin J., Sánchez P., Coordinators T. O., Acinas S. G., Babin M., et al. (2022). The Ocean Gene Atlas v2.0: online exploration of the biogeography and phylogeny of plankton genes. Nucleic Acids Res. 50, W516–W526. doi: 10.1093/nar/gkac420
Villar E., Vannier T., Vernette C., Lescot M., Cuenca M., Alexandre A., et al. (2018). The Ocean Gene Atlas: exploring the biogeography of plankton genes online. Nucleic Acids Res. 46, W289–W295. doi: 10.1093/nar/gky376
Walworth N. G., Fu F.-X., Lee M. D., Cai X., Saito M. A., Webb E. A., et al. (2018). Nutrient-colimited Trichodesmium as a nitrogen source or sink in a future ocean. Appl. Environ. Microb. 84, e02137–e02117. doi: 10.1128/AEM.02137-17
Yong C.-W., Deng B., Liu L.-M., Wang X.-W., Jiang H.-B. (2023). Diversity and evolution of iron uptake pathways in marine cyanobacteria from the perspective of the coastal strain Synechococcus sp. strain PCC 7002. Appl. Environ. Microb. 89, e01732–e01722. doi: 10.1128/aem.01732-22
Zehr J. P. (2011). Nitrogen fixation by marine cyanobacteria. Trends Microbiol. 19, 162–173. doi: 10.1016/j.tim.2010.12.004
Keywords: Trichodesmium, fixed nitrogen, Synechococcus, nitrogen supply, urea
Citation: Li S-Q, Xiao Y, Huang H-L, Luo Z, Sun T-R, Gao H-Y, Wang X-W and Jiang H-B (2024) Experimental evidence that nitrogen-fixing cyanobacterium Trichodesmium spp. supplies new nitrogen source to marine phytoplankton. Front. Mar. Sci. 11:1388725. doi: 10.3389/fmars.2024.1388725
Received: 20 February 2024; Accepted: 13 June 2024;
Published: 10 July 2024.
Edited by:
Yenny Risjani, University of Brawijaya, IndonesiaReviewed by:
Keisuke Inomura, University of Rhode Island, United StatesCopyright © 2024 Li, Xiao, Huang, Luo, Sun, Gao, Wang and Jiang. This is an open-access article distributed under the terms of the Creative Commons Attribution License (CC BY). The use, distribution or reproduction in other forums is permitted, provided the original author(s) and the copyright owner(s) are credited and that the original publication in this journal is cited, in accordance with accepted academic practice. No use, distribution or reproduction is permitted which does not comply with these terms.
*Correspondence: Xin-Wei Wang, d2FuZ3hpbndlaUBuYnUuZWR1LmNu; Hai-Bo Jiang, amlhbmdoYWlib0BuYnUuZWR1LmNu
Disclaimer: All claims expressed in this article are solely those of the authors and do not necessarily represent those of their affiliated organizations, or those of the publisher, the editors and the reviewers. Any product that may be evaluated in this article or claim that may be made by its manufacturer is not guaranteed or endorsed by the publisher.
Research integrity at Frontiers
Learn more about the work of our research integrity team to safeguard the quality of each article we publish.