- 1State Key Laboratory of Freshwater Ecology and Biotechnology, Institute of Hydrobiology, Chinese Academy of Sciences, Wuhan, China
- 2College of Advanced Agricultural Sciences, University of Chinese Academy of Sciences, Beijing, China
- 3Hubei Hongshan Laboratory, Huazhong Agriculture University, Wuhan, China
- 4The Innovative Academy of Seed Design, Chinese Academy of Sciences, Wuhan, China
The implementation of a controllable sterility strategy is crucial for the commercialization of precise trait improvements in farmed fish using genome editing and sustainable development of fisheries. Our previous research has demonstrated that females deficient in pituitary gonadotropin luteinizing hormone β-subunit (lhβ) or gonadal steroidogenesis gene steroidogenic acute regulatory protein (star) exhibit sterility due to impaired oocyte maturation and ovulation. Nevertheless, the effective restoration of fertility in lhβ- or star-deficient females remains unsolved. This study has discovered that the administration of exogenous 17α,20β-dihydroxy-4-pregnen-3-one (DHP) at 100 and 300 μg/L for 6 h (from 02:00 to 08:00 a.m.) effectively restores the fertility of lhβ- or star-deficient females. Fertilized eggs from these mutant females can be raised without noticeable developmental defects for up to 3 weeks post-fertilization (wpf) compared to the wild-type (WT) control zebrafish. The increased expression levels of adamts9 and adam8b in lhβ- or star-deficient zebrafish females treated with DHP demonstrate a positive correlation with oocyte maturation and ovulation restoration. In contrast, exogenous DHP administration did not rescue the sterility phenotype observed in progesterone receptor (pgr)-deficient females. Building on our recent success in generating an all-female carp population through cytochrome P450, family 17, subfamily A, polypeptide 1 (cyp17a1)-depletion, our research presents a promising and effective strategy for an “off-on” switch for managing fertility in genome-edited cyprinids. The strategy would offer practical guidance and theoretical justification for developing “controllable fertility” in all-female fish, which would support the sustainable development of fisheries by promoting the use of novel biotechnologies in aquaculture in an eco-friendly manner.
Introduction
Advances in genome editing technologies, such as transcription activator-like effector nuclease (TALEN) and clustered regularly interspaced short palindromic repeats associated with Cas9 (CRISPR/Cas9), provide effective and precise tools for enhancing fish traits for aquaculture purposes. For example, improved growth and feed conversion efficiency have been observed in gibel carp (Carassius gibelio) and all-female common carp (Cyprinus carpio) populations by depleting the phosphoinositide-3-kinase, regulatory subunit 1 (alpha) (pik3r1), and cytochrome P450, family 17, subfamily A, polypeptide 1 (cyp17a1) loci, respectively (Huang et al., 2021; Zhai et al., 2022a). However, potential spread of edited alleles into wild-type (WT) stocks limits use of genome-edited fish in aquaculture. To circumvent this, we have dedicated ourselves to developing a strategy by creating an all-female fish population (Zhai et al., 2022a) and its sterilization (Gratacap et al., 2019; Okoli et al., 2022). In this study, we establish a “off-on” switch for fertility control of female fish to breed and maintain desirable traits of sex-controlled breeding. Building on our recent success with the all-female carp population generated via the cyp17a1-depletion strategy, a practicable “off-on” technique for sterility in female cyprinids will be exhilarating particularly.
In vertebrates, pituitary gonadotropins are critical for ovarian development throughout the reproductive cycle, a multifaceted biological process (McGee and Hsueh, 2000). The pituitary gonadotropin, Lhβ, a member of the glycoprotein hormone family, binds to its receptor Lhcgr on the granulosa or theca cells to regulate of oocyte growth, development and maturation (Gharib et al., 1990). Mutation of LH or Lhcgr in both humans and mice can result in ovarian hypogonadism and infertility due to defective gonadal steroidogenesis (Lei et al., 2001; Zhang et al., 2001; Ma et al., 2004; Huhtaniemi and Themmen, 2005; Huhtaniemi, 2006; Li and Ge, 2020). Ovarian development in zebrafish can be categorized into five stages: primary oocyte growth (stage I), accumulation of cortical alveoli (stage II), vitellogenesis (stage III), and maturation (stages IV and V). Stage IV includes stage IVa (onset of oocyte maturation and 3 h before lights on), and stage IVb (oocytes mature but before ovulation, 1 h before lights on) (Liu et al., 2018). Stimulation from Luteinizing hormone-releasing hormone (LHRH) prompts Lh secretion from the pituitary gland, resuming the meiotic cell cycle and inducing germinal vesicle breakdown (GVBD), an indicator of oocyte maturation. In stage V, the completely developed eggs are released and prepared for spawning (Nagahama et al., 1995; Nagahama and Yamashita, 2008).
In zebrafish, the crucial regulatory roles of Lh in ovarian steroidogenesis have been documented. Briefly, LH activates the intracellular signaling pathway (cAMP/PKA/CREB), the production of maturation-induced hormone and 17α,20β-dihydroxy-4-pregnen-3-one (DHP), and oocyte maturation by binding to Lhcgr, which is located on the theca cells of the ovary (Ascoli et al., 2002; Nagahama and Yamashita, 2008; Levavi-Sivan et al., 2010). In theca cells, cholesterol undergoes conversion to 17α-hydroxy-progesterone and testosterone. These hormones are then respectively converted to DHP and estradiol in the granulosa cells (Clelland and Peng, 2009). Lh binds to Lhcgr to increase insulin-like growth factor 3 (igf3) expression as well, which subsequently binds to the insulin-like growth factor 1 receptor (igf1r) and initiates oocyte maturation and ovulation (Li et al., 2018). Lh can also initiate follicle activation and promote follicle growth via the Fsh receptor (Fshr), which compensates for the absence of Fsh in folliculogenesis (Zhang et al., 2015). Steroidogenic acute regulatory protein (Star), an enzyme responsible for transporting cholesterol into the inner mitochondrial membrane for DHP production, has been identified as a downstream target of Lh for oocyte maturation in zebrafish (Shang et al., 2019) and some other teleost fishes (Nagahama and Yamashita, 2008). However, the specific functions and mechanisms of progestin signaling in initiating oocyte maturation and ovulation in zebrafish requires further elucidation.
Recently, we reported that the maturation-arrested oocyte phenotypes could be partially rescued by the administration of DHP precursors, pregnenolone or progesterone, including DHP itself, in lhβ- or star-deficient females (Shang et al., 2019). Progestin signals can be mediated through membrane progestin receptor α (mPRα) and nuclear progestin receptor (Pgr). Arrested oocyte maturation and mature oocytes trapped within the follicular cells were observed in mPRs-deficient and npr-deficient females, respectively (Zhu et al., 2015; Wu et al., 2020). These results indicate that the progestin signaling may be disrupted in lhβ- or star-deficient females due to impaired steroidogenesis in mutant fish. However, the exact pathological mechanisms responsible for impaired oocyte maturation and ovulation in lhβ- or star-deficient females remain elusive, hindering the development of effective restoration strategies for their fecundity.
A ovulation-impaired phenotype was also observed in the pgr-deficient females (Liu et al., 2018). In preovulatory follicular cells (stage IVb) of female zebrafish, the expression levels of four metalloproteinases, including ADAM metallopeptidase with thrombospondin type 1 motif, 9 (adamts9), ADAM metallopeptidase domain 8b (adam8b), ADAM metallopeptidase with thrombospondin type 1 motif, 1 (adamts1) and matrix metallopeptidase 9 (mmp9), increased dramatically in WT females, but reduced in pgr-/- zebrafish (Liu et al., 2018). In vitro experiments conducted on pre-ovulatory follicles have demonstrated that DHP upregulates adamts9 expression in a dose-, time-, and Pgr-indispensable manner (Liu et al., 2018). adamts9 expression in pre-ovulatory follicular cells is also regulated by human chorionic gonadotropin (hCG, a LH analog), which has been demonstrated to be Lhcgr-dependent, but not Pgr-dependent (Liu et al., 2020). These findings suggest that the regulation of adamts expression in pre-ovulatory follicular cells may involve multiple contributing factors.
In this study, we examined the effects on oocyte maturation/ovulation caused by deficiencies in lhβ or star in female zebrafish. The strategy for restoring fecundity in these mutants has been continuously refined through synchronized assessments of the gonadal anatomy, fertility capacity, and the efficacy of each chosen steroid compound, along with its dosage and duration (Chen et al., 2013; Zhu et al., 2015; Lau et al., 2016; Tang et al., 2016; Lu et al., 2017; Yin et al., 2017; Crowder et al., 2018; Tang et al., 2018; Yu et al., 2018; Zhai et al., 2018; Li et al., 2020; Shu et al., 2020). Our results indicate that treatment of exogenous DHP effectively restores the fecundity of lhβ- or star-deficient female zebrafish. The main findings of this study using genome editing models provide a valuable basis for developing an “off-on” switch to control female fertility in teleosts.
Materials and methods
Animals
Zebrafish were maintained under standard conditions at 28.5°C, in a circulated water system with a 14 h light and 10 h dark cycle as previously described (Westerfield, 2000). The lhβ, star and pgr heterozygous males and females were inbred to generate population that contained lhβ, star and pgr homozygotes, respectively (Tang et al., 2016; Shang et al., 2019). Mutant lhβ has an 8 bp deletion in the second exon, star has a 1 bp deletion in the second exon, and pgr has a deletion of the flanked genomic fragment (13.40 kb) between the first and the sixth exon.
Natural mating
Natural mating was conducted as previously described (Shu et al., 2020). Briefly, WT, lhβ- or star-deficient females were kept with WT males in a breeding tank with an insert and a divider in the middle. The divider was removed at 08:00 the following morning and the ratio of spawning, fertilization, and survival were recorded in every tank.
Administration with DHP
DHP (CAS No. 1662-06-2, P712080, Toronto Research Chemicals, Canada) was dissolved in Dimethylsulfoxide (DMSO), and the stock solutions of 10 g/L was sub-packed and stored in -80 °C. The lhβ- and star-deficient females were subjected to immersion treatments with 100 and 300 μg/L DHP from 02:00 to 08:00, respectively.
hCG intraperitoneal injection and administration with DHP
The hCG (hor-250, PROSPEC, Israel) was dissolved in water at 25 IU/μL as stock solution and the working solution was diluted to 1/5 with normal saline. Intraperitoneal hCG injection was conducted as described in a previous study, with minor modifications (Kinkel et al., 2010). After anesthetization, lhβ- or star-deficient females were quickly placed on a piece of wet gauze and carefully injected with 10 μL of the working solution into the pelvic midline fins at 00:00 using a microsyringe. lhβ- and star-deficient females were subsequently administrated with the immersion treatments with 100 and 300 μg/L DHP from 02:00 to 08:00, respectively.
Collection of follicular cells from stage IV follicles
The ovaries were dissected, and follicular cells of preovulatory follicles at stage IV were collected approximately 1 h prior to ovulation with lights on. Follicular cells, collected from 100 follicles per fish were separated from stage IV follicles using precision tweezers, according to a previous study with minor modifications (Liu et al., 2018).
RNA extraction and quantitative real-time polymerase chain reaction (qPCR)
Total RNA was extracted from the follicular cells of lhβ- or star-deficient females with Trizol reagent (15596026, Ambion, TX, USA). 500 ng of the RNA template was used for reverse transcription and cDNA synthesis using the EasyScript One-Step gDNA Removal and cDNA Synthesis SuperMix Kit (AE311-03, Transgen, China). The qPCR was conducted using PerfectStartTM Green qPCR SuperMix (AQ601-02, Transgen, China) according to a previous study (Shi et al., 2022). qPCR was conducted using a Bio-Rad Real-Time System (Bio-Rad Systems, USA). All the mRNA levels were calculated as the fold change relative to eukaryotic translation elongation factor 1 alpha 1 (ef1a). The primers of adamts9, adam8b, adamts1, and mmp9 used for qPCR are listed in Table 1.
Histological analysis
Hematoxylin and eosin staining was performed as previously described (Zhai et al., 2022b). Briefly, fish were euthanized with MS-222 and the ovary was isolated for fixation in Bouin’s solution. Fixed samples were dehydrated, infiltrated and embedded in paraffin for sectioning on a Leica microtome (RM2235, Leica Biosystems, German). Paraffin sections were stained with hematoxylin and eosin and examined microscopically using a Nikon Eclipse Ni-U microscope (Nikon, Tokyo, Japan). The scale bar is displayed in each image.
Statistical analysis
Detailed information regarding the number of zebrafish used per experiment is provided for each experiment and the corresponding figure. All analyses were conducted with the GraphPad Prism 6.0 software program and the differences were evaluated using the student’s t-test. The results were expressed as the mean ± SD. For all statistical comparisons, a P value 0.05 was used to indicate a statistically significant difference.
Results
WT females could be induced to spawn after DHP administration
For the regular WT zebrafish natural mating group, one male and one female were placed overnight in a tank separated by a transparent divider. The following morning, the divider was removed for natural fish mating (Figure 1A). Ovulation of mating females was induced by overnight housing with the mating males (Figures 1B–I, Table 2). If a female was not primed by an overnight housing with a male before the mating (no priming group) (Figure 1J), this naïve single female would not naturally engage in ovulation and mating behavior with a male (Figures 1K–R, Table 2). However, if the WT female was treated with 50 μg/L DHP for 6 h from 02:00 to 08:00, the effective ovulation could be seen in DHP treated female (Figures 1S-A’). When a pair of DHP-treated females and males was set up for mating after 6 h DHP treatment, the DHP treated WT females could naturally mate with the WT males and spawn (Table 2). Although the fertilization ratio of the offspring from DHP administrated WT females and WT males was significantly lower than that of the natural mating group, the ratios of membrane breakage and survival at 3 wpf were unaffected (Table 2). These results indicate that DHP treatment can effectively replace overnight housing with male fish to induce the ovulation and spawning in female fish. The viabilities of the naturally fertilized eggs from primed by overnight housing and DHP treatment were equivalent.
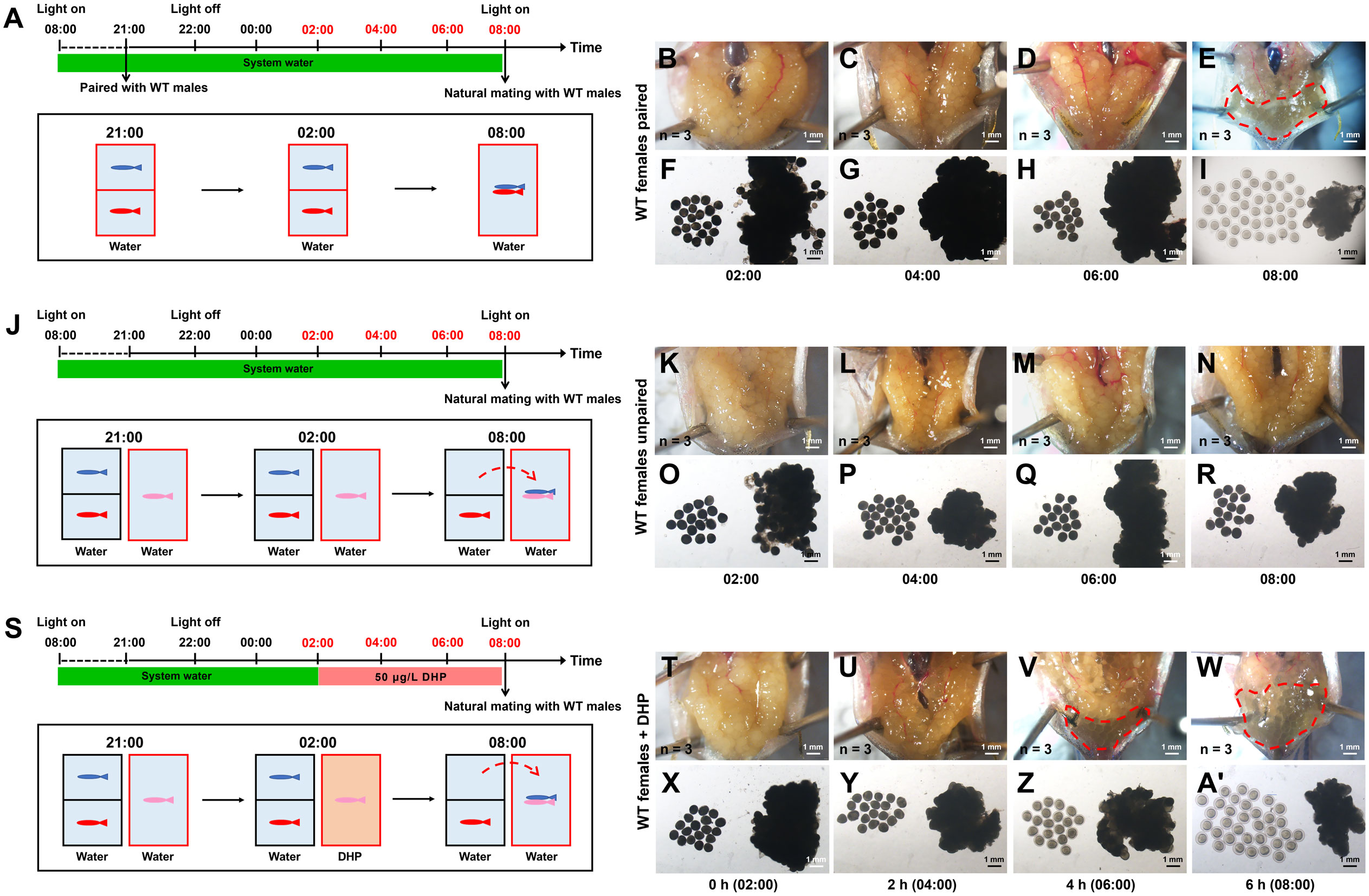
Figure 1 WT females could be induced to spawn after DHP administration. (A) Schematic illustration of the spawning test for paired WT females (depicted in red). One WT female (depicted in red) and one WT male (depicted in blue) are housed overnight in the tank, separated by a transparent divider. At 08:00 (light on), the divider is removed, then the spawning between the paired WT female and WT male succeeds. (B–I) The anatomical examination and microphotographs of follicles are sampled from paired WT females at 02:00, 04:00, 06:00, and 08:00 (n = 3 at each timepoint). (J) Schematic illustration of the spawning test for unpaired WT females (depicted in pink). WT females (depicted in red) are housed overnight in the tank with WT male (depicted in blue), then natural mating of the unpaired WT females (pink) with the paired males (blue) failed. (K–R) The anatomical examination and microphotographs of follicles sampled from unpaired WT females at 02:00, 04:00, 06:00, and 08:00 (n = 3 at each timepoint). (S) Schematic illustration of the spawning test for unpaired WT females (depicted in pink) treated with DHP (tank depicted in brown). WT females are exposed to 50 μg/L DHP from 2:00 to 8:00, then natural mating with males (depicted in blue) which are from another tank paired with WT females (depicted in red) overnight succeeds. (T–A’) The anatomical examination and microphotographs of follicles sampled from WT females treated with 50 μg/L DHP for 0 h (02:00), 2 h (04:00), 4 h (06:00), and 6 h (08:00) (n = 3 at each timepoint). The samples at the timepoints depicted in red are harvested for oocyte analysis. (B, F, K, O, T, X) 0 h (C, G, L, P, U, Y) 2 h (D, H, M, Q, V, Z) 4 h (E, I, N, R, W, A’) 6 h.
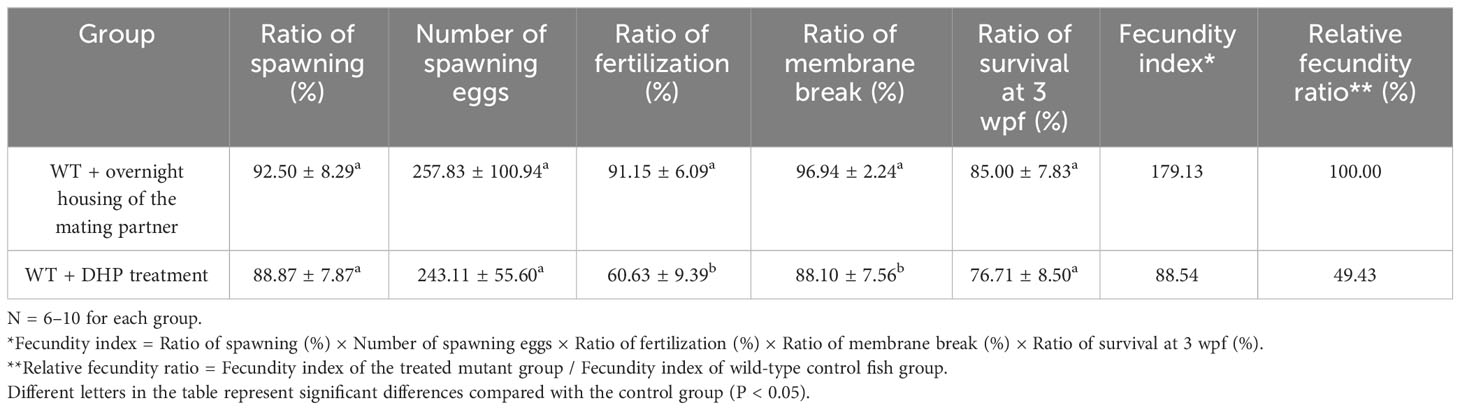
Table 2 Comparison of the fecundities of wild-type zebrafish female induced by overnight housing and DHP treatment.
Anovulation defects in lhβ- or star-deficient females rescued with the DHP administration
Previously, the phenotypes of oocyte maturation and ovulation were observed in lhβ- or star-deficient females. We found that the follicles in the lhβ mutants did not enter stage V as it normally occurred in the WT control females between 06:30 and 07:30 when mated with WT males (Shang et al., 2019). We first examined the restorative effects of DHP on ovulation in these females. The lhβ- and star-deficient females were exposed to 100 and 300 μg/L DHP, respectively, from 02:00 to 08:00 (Figure 2A). At 02:00, the follicles from the dissected ovaries of lhβ-deficient females were non-transparent (Figures 2B, F); however, after 2 h DHP administration (at 04:00), the follicles became semi-transparent (Figures 2C, G), marking the beginning of oocyte maturation. More apparently, the anatomical examination of lhβ-deficient females exposed to 100 μg/L DHP for 4 h (at 06:00) was sufficient to induce maturation of oocytes, as evidenced by the microphotographs of follicles, which had become transparent embedded in the dissected ovaries and few eggs with egg membrane formed (characterized as stage V) (Figures 2D, H). At 08:00, when the lhβ-deficient females were exposed to DHP for 6 h, more eggs with membrane formed, suggesting that the follicles reached to stage V, and released into the vicinity of the genital pore in lhβ-deficient females (Figures 2E, I). The successful induction of follicles at different time points after DHP administration was also supported by the observed oocyte maturation and ovulation in star-deficient females (Figures 2J-Q). These results suggest that the arrested oocyte maturation and ovulation in lhβ- or star-deficient females in vivo could be effectively rescued by DHP administration.
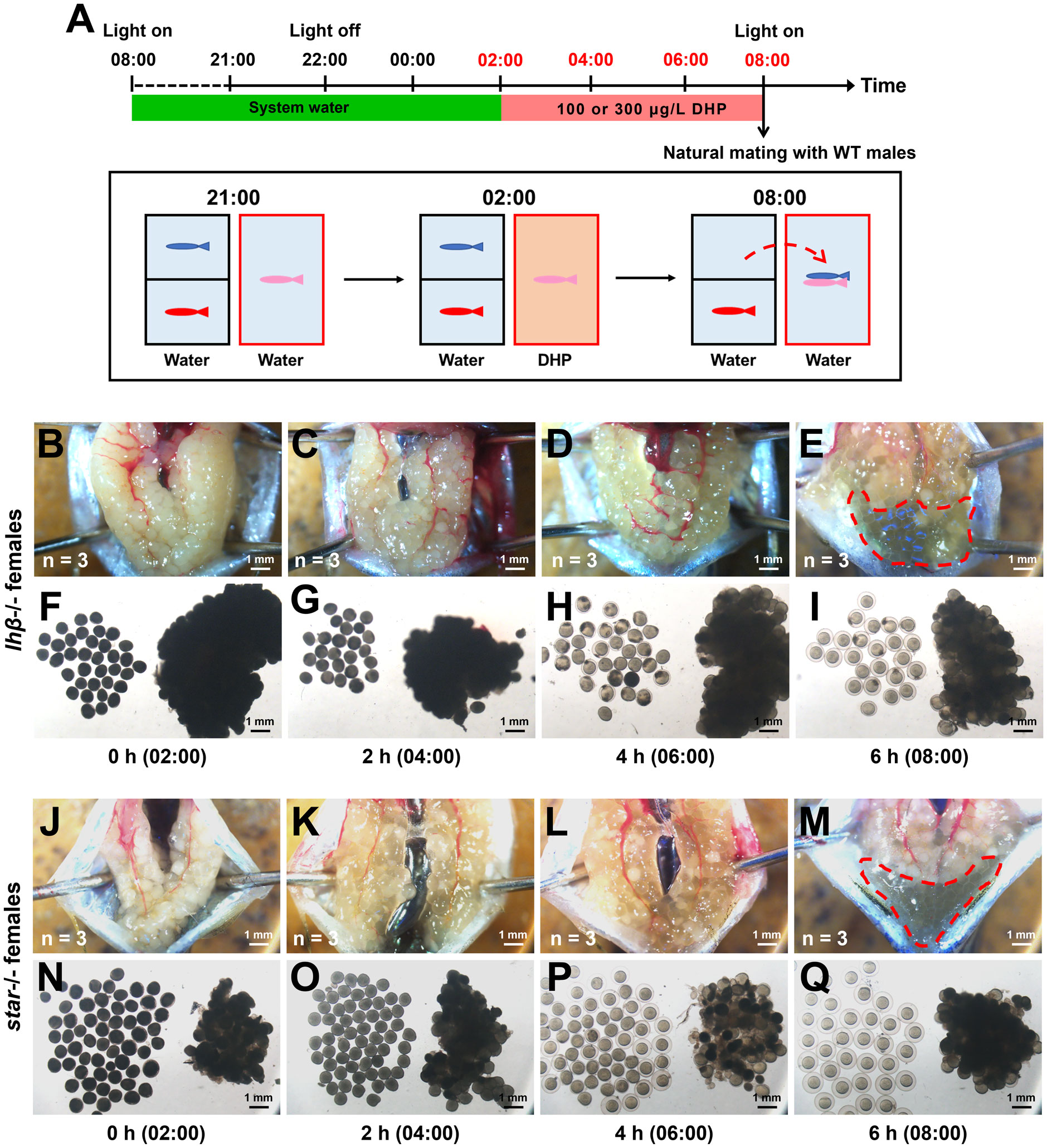
Figure 2 The arrested oocyte maturation of lhβ- or star-deficient females can be rescued by DHP administration. (A) Schematic illustration of the DHP administration for lhβ- or star-deficient females (depicted in pink) during the daily spawning cycle. lhβ- or star-deficient females are exposed to 100 or 300 μg/L DHP from 02:00 to 08:00, then natural mating with males (depicted in blue) which are from another tank and paired with WT females (depicted in red) overnight. (B–I) The anatomical examination and microphotographs of follicles sampled from lhβ-deficient females exposed to 100 μg/L DHP for 0 h (02:00), 2 h (04:00), 4 h (06:00), and 6 h (08:00) (n = 3 at each timepoint). (J–Q) The anatomical examination and microphotographs of follicles sampled from star-deficient females exposed to 300 μg/L DHP for 0 h, 2 h, 4 h, and 6 h (n = 3 at each timepoint). The samples at the timepoints depicted in red are harvested for oocyte analysis. (B, F, J, N) 0 h (C, G, K, O) 2 h (D, H, L, P) 4 h (E, I, M, Q) 6 h.
Infertility of lhβ- or star-deficient females was rescued with DHP administration
Following the sketches depicted in Figure 2A, the rescue of the infertility phenotypes in lhβ- or star-deficient females administrated diverse combinations of the hormones, including DHP and hCG respectively, were evaluated with natural mating with WT males (Table 3). For comparison of the rescue efficiency, the “fecundity index” was adopted for representing the capacity of each experimental mating group to produce healthy offspring. The “relative fecundity” indicates the rescue efficiency of each treatment, represented by the fecundity index of the treated mutant group/fecundity index of WT control group (Table 3). After a 6 h treatment (from 02:00 to 08:00), the lhβ-deficient females were placed in a new breeding tank with an insert at the bottom and a divider in the middle. WT males, which had been kept with WT females in another breeding tank contained system water that night, were transferred into the tank with the DHP-treated lhβ-deficient females. Natural mating was observed between WT males and DHP-treated lhβ-deficient females. Compared with the hCG treatment, the administration of DHP effectively rescued the defects of oocyte maturation and ovulation of the lhβ-deficient females, as the spawned and fertilized eggs with the genotype of lhβ+/- were observed (76.00 ± 23.32% lhβ-deficient females spawned, 25.90 ± 12.28% alive eggs were fertilized, 68.11 ± 31.45% fertilized eggs broke the egg membrane, and 59.80 ± 39.56% fertilized eggs survived to juvenile stage) (Table 3).
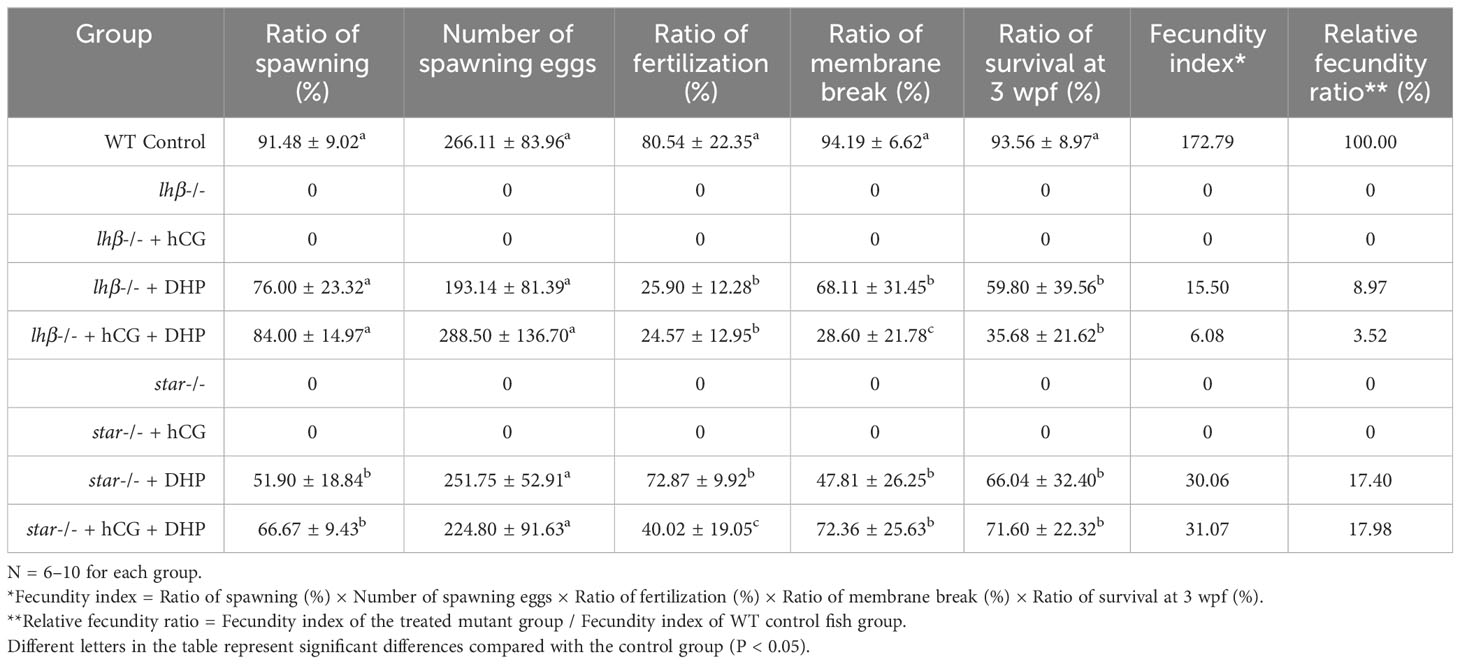
Table 3 Efficiencies of the fecundity rescue of lhβ- and star-deficient females with diverse steroids treatments.
Noteworthily, the administration of 100 μg/L DHP for 6 h, which was sufficient to rescue the defects of oocyte maturation and ovulation in the lhβ-deficient females, did not work well in the star-deficient females, as no spawned eggs were observed, even with artificial squeeze (Data not shown). The star-deficient females were subsequently exposed to 300 μg/L DHP from 02:00 to 08:00. Strikingly, 6 h DHP administration at 300 μg/L effectively rescued the defects of oocyte maturation and ovulation in the star-deficient females (Table 3), resulting in the observation of the spawned and fertilized eggs with the genotype of star+/- (51.90 ± 18.84% star-deficient females spawned, 72.87 ± 9.92% alive eggs were fertilized, 47.81 ± 26.25% fertilized eggs broke the egg membrane, and 66.04 ± 32.40% larvae of them survived to juvenile stage) (Table 3).
The developmental outcomes of the fish from the control females, the lhβ- or star-deficient females and WT males were also evaluated. The fertilized eggs at the early stage during embryonic development (12 h post-fertilization, hpf), larval stage (72 hpf and 5 days post-fertilization, dpf), and juvenile stage (3 weeks post-fertilization, wpf) from the control females and from the lhβ- or star-deficient females and WT males were comparable as evaluated by the gross appearance (Supplementary Figures 1A–L, Table 3). At 1-month post-fertilization (mpf), the body weight, body length and full length of the fish from control females were comparable with rescued fish from the lhβ- or star-deficient females mated with WT males (Supplementary Figures 1M–O). These results suggest that DHP at 100 or 300 μg/L from 02:00 to 08:00 is sufficient to rescue ovarian maturation and ovulation defects of lhβ- or star-deficient females as evaluated with natural mating with WT males, while the overall efficiency of star-deficient females administrated with 300 μg/L DHP for 6 h is better than those of DHP-treated lhβ-deficient females.
DHP administration upregulated adam8b and adamts9 expression in pre-ovulatory follicle cells of WT females mimicked the effect of natural mating with WT males
It is known that the metalloproteinases, including adamts9 and adam8b, are required for zebrafish ovulation, and their expression significantly increased at 1 h prior to ovulation (Liu et al., 2018; Liu et al., 2020). Both metalloproteinases, adamts9 and adam8b, but not adamts1 and mmp9 (Figures 3A–D, column 2 vs. 1), were significantly upregulated in the pre-ovulatory follicle cells of WT females paired with WT males 1 h before light on compared to those of unpaired WT females. Intriguingly, in the follicular cells of WT females exposed to DHP for 2 h (from 05:00 to 07:00) exhibited upregulated expression of adamts9 and adam8b compared to the unpaired WT females, mimicking the effect of natural mating with WT males (Figure 3D, column 3 vs. 2).
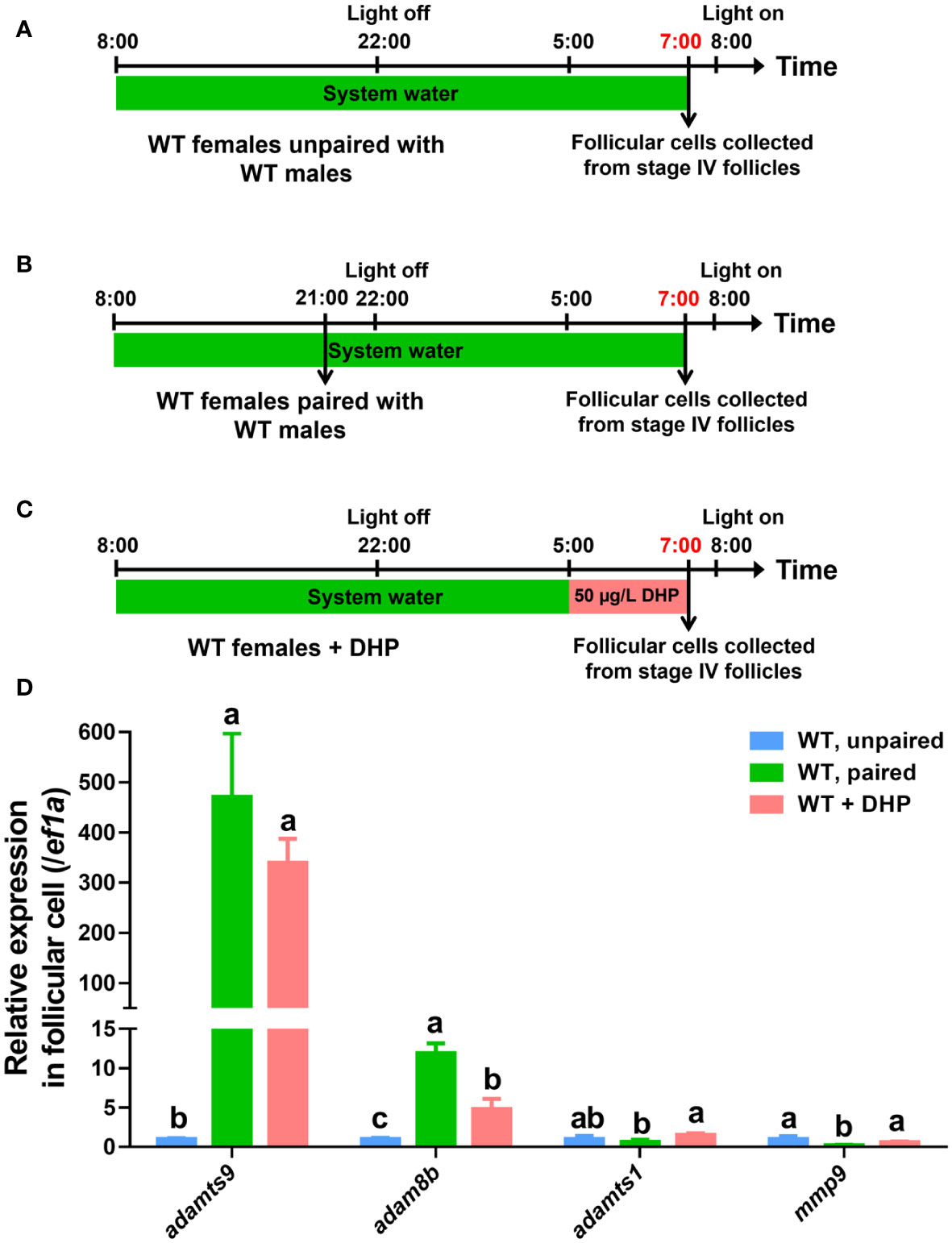
Figure 3 Expression of adamts9 and adam8b is upregulated after DHP administration. (A) Schematic illustration of unpaired WT females. (B) Schematic illustration of paired WT females. (C) Schematic illustration of the DHP administration on WT females from 05:00 to 07:00. The samples at the timepoint depicted in red are harvested for analysis. (D) The expression of adamts9, adam8b, adamts1, and mmp9 in pre-ovulatory follicular cells at 07:00 of paired WT females or WT females exposed to DHP are comparatively evaluated with that of control females unpaired with WT males. The letters in the bar charts represent significant differences.
DHP administration restores adam8b and adamts9 expression in pre-ovulatory follicle cells of lhβ- or star-deficient females
Both metalloproteinases, adamts9 and adam8b (Figures 4, 5A–E, column 2 vs. 1), but not adamts1 and mmp9 (Figures 4, 5F, G, column 2 vs. 1), were significantly upregulated in the pre-ovulatory follicle cells of control females paired with WT males 1 h before light on. Contrarily, the increased expression of adamts9 and adam8b in follicular cells was not observed in lhβ- or star-deficient females paired with WT males 1 h before light on (Figures 4, 5D, E, column 4 vs. 3). We hypothesized that this may be one of the major reasons for arrested oocyte maturation and ovulation. Intriguingly, the follicular cells of lhβ- or star-deficient females exposed to DHP for 2 h (from 05:00 to 07:00) exhibited upregulated adamts9 and adam8b expression, compared to the untreated fish (Figures 4, 5D, E, column 5 vs. 3). These results suggest that the upregulated adamts9 and adam8b expression may be induced by DHP to restore the final maturation of oocyte and ovulation in lhβ- or star-deficient females.
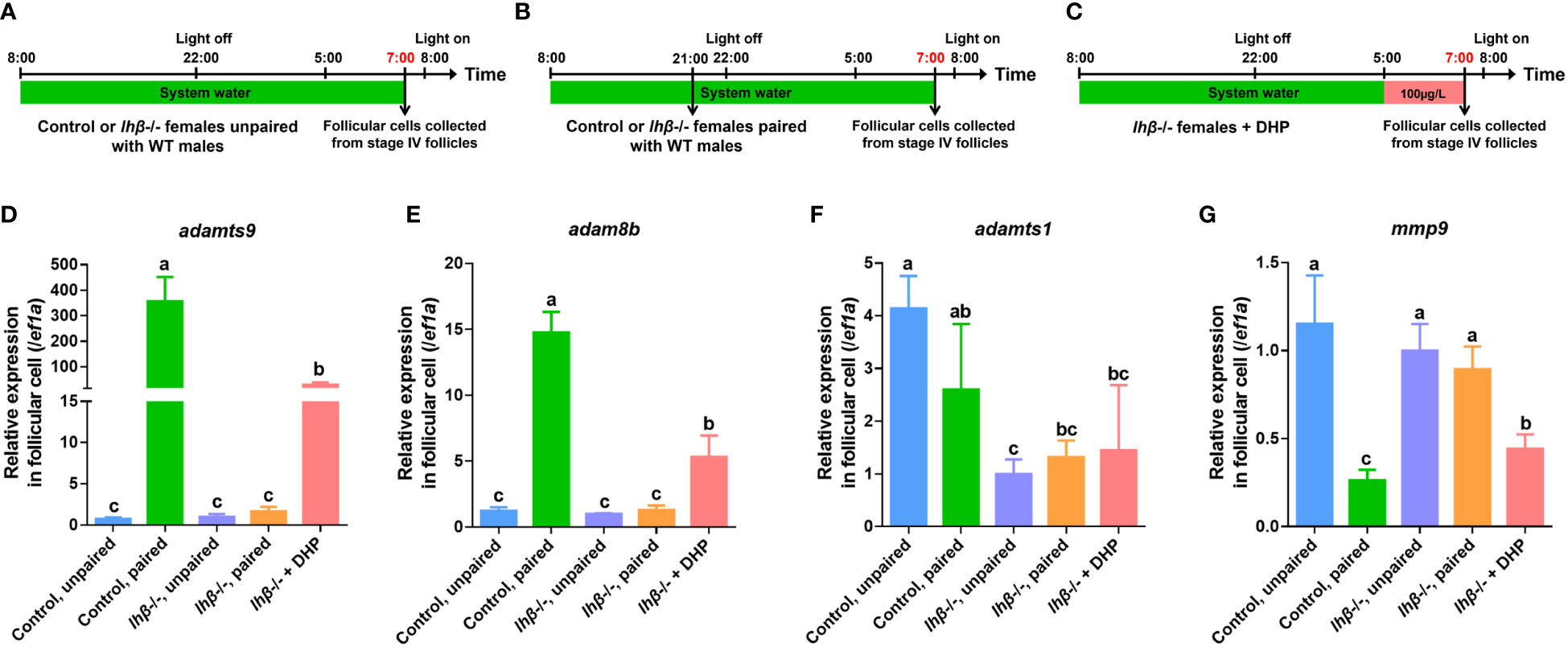
Figure 4 Expression of adamts9 and adam8b in follicular cells of lhβ-deficient females after pairing with WT males or DHP administration. (A) Schematic illustration of unpaired control or lhβ-deficient females. (B) Schematic illustration of paired control or lhβ-deficient females. (C) Schematic illustration of the DHP administration on lhβ-deficient females from 05:00 to 07:00. The samples at the timepoint depicted in red are harvested for analysis. (D–G) The expression of adamts9, adam8b, adamts1 and mmp9 in preovulatory follicular cells at 07:00 of control females unpaired with WT males, control females paired with WT males, lhβ-deficient females unpaired with WT males, lhβ-deficient females paired with WT males, and lhβ-deficient females treated with DHP. The letters in the bar charts represent significant differences.
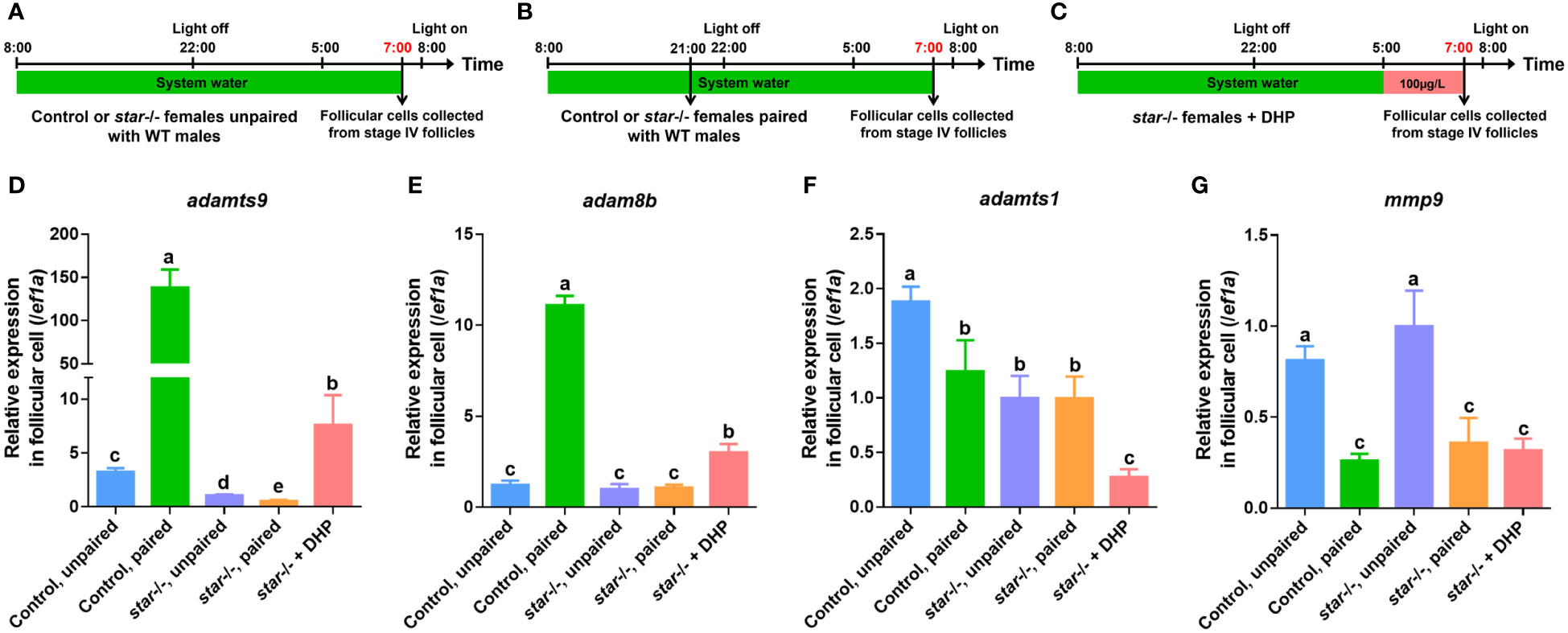
Figure 5 Expression of adamts9 and adam8b in follicular cells of star-deficient females after paired WT males or DHP administration. (A) Schematic illustration of unpaired control or star-deficient females. (B) Schematic illustration of paired control or star-deficient females. (C) Schematic illustration of the DHP administration on star-deficient females from 05:00 to 07:00. The samples at the timepoint depicted in red are harvested for analysis. (D–G) The expression of adamts9, adam8b, adamts1, and mmp9 in preovulatory follicular cells at 07:00 of control females unpaired with WT males, control females paired with WT males, star-deficient females unpaired with WT males, star-deficient females paired with WT males, and star-deficient females treated with DHP. The letters in the bar charts represent significant differences.
Pgr signaling is indispensable for DHP-induced ovulation in zebrafish
Genomic progestin signaling and ovulation require the participation of Pgr, and pgr depletion is known to cause ovulation failure but does not affect oocyte maturation in zebrafish (Zhu et al., 2015). Our observations in the pgr-deficient female zebrafish replicated the manifestations of impaired ovulation reported in previous studies (Zhu et al., 2015). In control females paired with WT males, normal mature stage V oocytes that were released into the vicinity of the genital pores were observed at 08:00 (Figure 6A). However, anovulation with mature stage V oocytes trapped within the ovary was observed in pgr-deficient females (Figure 6B). The results of histological analysis demonstrated that the outer layer cells of the mature follicles failed to breakdown in the pgr-deficient fish (Figure 6C, D). Moreover, we found that the anovulation defects observed in pgr-deficient female zebrafish could not be rescued by DHP administration at a range of concentrations (Figure 6E-H). These results suggest that Pgr signaling is indispensable for DHP-mediated ovulation in zebrafish.
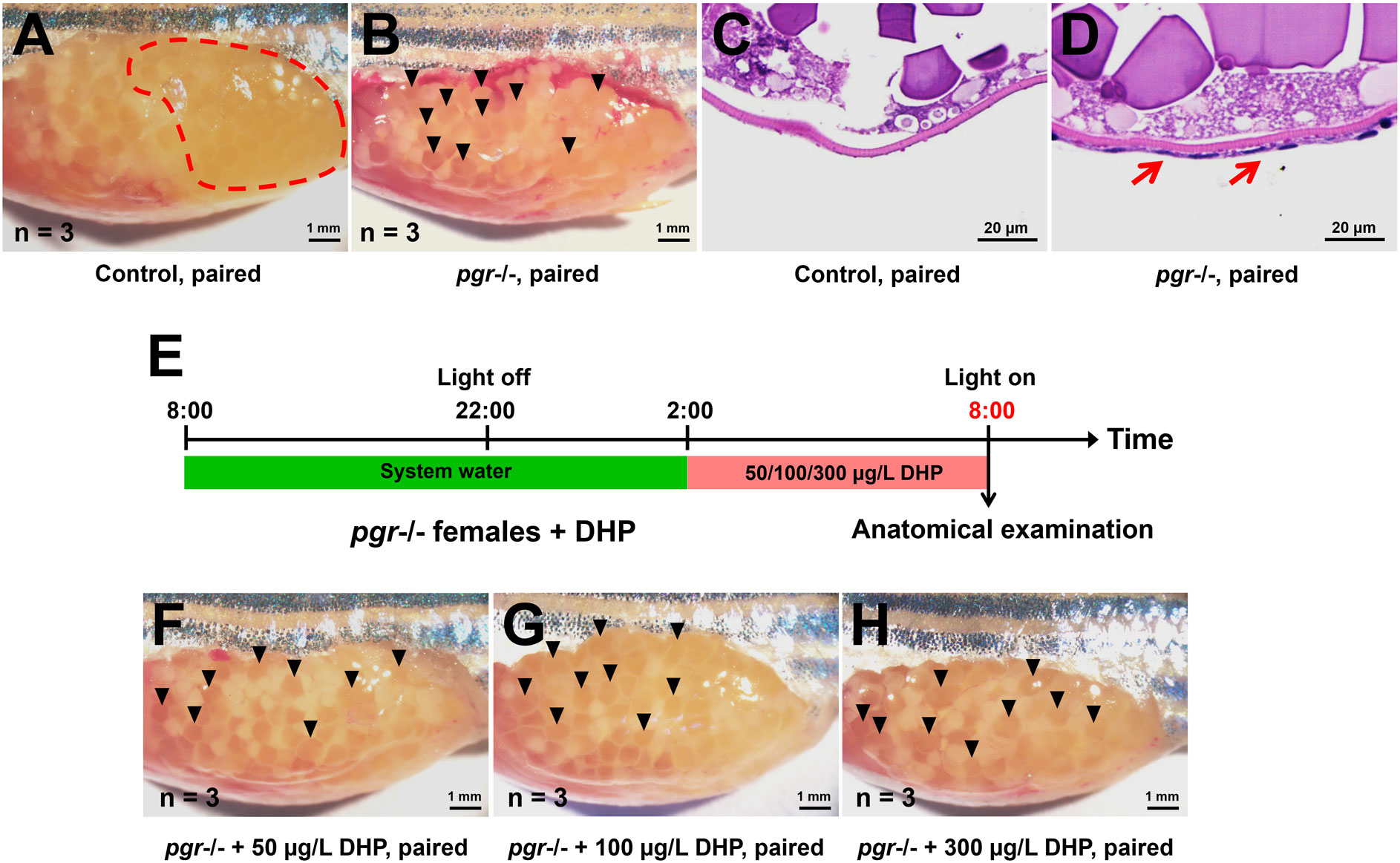
Figure 6 Comparison of ovaries from control and pgr-deficient female fish paired with WT males or treated with DHP after light onset. (A, B) Anatomical analysis of ovaries. Normal mature and ovulated oocytes were released into the vicinity of the genital pore in control females paired with WT males (A, dashed red circle), and anovulation with mature oocytes trapped within the ovary in pgr-deficient females paired with WT males (B, black arrow heads). (C, D) Histological analysis of mature follicles. Compared with control fish (C), the outer layer cells of the mature follicles from the pgr-deficient female fish failed to breakdown (D, red arrows). (E) Schematic illustration of the DHP administration on pgr-deficient females from 02:00 to 08:00. The samples at the timepoint depicted in red are harvested for analysis. (F–H) The anovulation with mature oocytes trapped within the ovary in pgr-deficient females (black arrow heads) cannot be rescued by administration of 50, 100 and 300 µg/L DHP.
Discussion
Although several studies have been conducted in zebrafish, the precise physiological processes and mechanisms involved in oocyte maturation and ovulation remain unclear. This study investigated the replacement of overnight mating partner housing with DHP treatment for oocyte maturation and ovulation induction in WT zebrafish. Based on these trails, DHP was selected to rescue sterility phenotype in lhβ- or star-deficient females. After multiple trials to determine the appropriate compound, dosage, and duration, it has been discovered that DHP and DHP + hCG administration can achieve consistently restore fertility in lhβ- or star-deficient females. The increased levels of adamts9 and adam8b present in follicular cells of lhβ- or star-deficient females, as well as WT females exposed to overnight housing pairing or DHP treatment, demonstrated a positive relationship with the induction of oocyte maturation and ovulation induction. Since DHP administration failed to rescue the ovulation failure observed in pgr-deficient female zebrafish despite the presence of mature stage V oocytes (Zhu et al., 2015), we concluded that Pgr is imperative for DHP-mediated ovulation.
The process of oocyte maturation and ovulation lasts for a few hours in fish within the spawning cycle. In the preovulatory follicles of WT female zebrafish, the relative expression of lhβ began to increase from 03:30, and reached the peak at 05:30, the beginning of oocyte maturation (follicles started to become semi-transparent) (Shang et al., 2019). Similarly, the transcript and protein levels of Pgr begin to increase at 05:00 (prior to maturation) and peak level at 06:00–07:00 (in the middle of maturation) in female zebrafish (Liu et al., 2018). DHP is the major maturation-inducing hormone in zebrafish (Nagahama and Yamashita, 2008; Tokumoto et al., 2011; El Mohajer et al., 2022). Previously, we found that Lhβ regulates DHP synthesis in zebrafish by targeting star, the first step of gonad steroidogenesis from cholesterol (Shang et al., 2019). This is supported by the observations in lhβ-, or star-, or mprs-deficient females, wherein the oocyte maturation was impaired (Wu et al., 2020). These findings suggest that Lhβ/Star/Progestin signaling is closely related to oocyte maturation. However, most of the conclusions that DHP promotes oocytes maturation were conducted on stage IV follicles in vitro. Here, the assessment of DHP in oocyte maturation and ovulation was firstly examined in vivo from 02:00 to 08:00 in lhβ- or star-deficient females with DHP administration at 100 and 300 μg/L, respectively. Oocytes become to be transparent following GVBD due to fusion of yolk proteins, allowing more lights to pass through. At 04:00, after 2 h DHP treatment, the follicles became semi-transparent, indicating the beginning of oocyte maturation. At 06:00, after 4 h DHP treatment, the follicles became transparent, and very few oocytes formed egg membranes, marking the completion of oocyte maturation (stage V) started. At 08:00, after 6 h DHP treatment, the transparent follicles were not trapped in the vicinity of the ejaculatory pore and were ready to spawn, forming egg membranes followed by dissection. These results provided direct in vivo evidence supporting that oocyte maturation and ovulation occurred effectively in lhβ- or star-deficient females after DHP administration.
The lhβ- or star-deficient females reared in control medium (1‱ DMSO system water) exhibited no egg-laying when naturally mated with WT males. Nevertheless, DHP treatment from 02:00 to 08:00 effectively rescued the defects in oocyte maturation and ovulation of lhβ- or star-deficient females when naturally mated with WT males, i.e. the restored spawned and fertilized eggs (Figure 2, Table 3). Noteworthily, the spawning ratio of the lhβ- or star-deficient females and the fertilization ratio of the offspring were decreased compared to the WT control group (Table 3). One possibility is that the spawned eggs may not be in their most appropriate status for fertilization with the above treatment method. Therefore, further efforts to optimize the process, including concentration of the chemical reagent, mode of the administration, or other factors, are needed to improve the quality of the eggs from the mutant females. This hypothesis could be partially supported by the observations that the ratio of membrane break and survival at 3 wpf of the offspring from the DHP + hCG treated star-deficient females mated with WT males were higher than with the females with DHP treatment only (Table 3). Intriguingly, the survived fish (lhβ+/- or star+/-) displayed normal growth performance (body weight, body length and full length) compared with the control fish (lhβ+/+ or star+/+) as observed at 1 mpf (Supplementary Figure 1).
Metalloproteinases are important for zebrafish ovulation. The relative expression of metalloproteinases, adamts9, adam8b, mmp9, and adamts1, was upregulated in the follicular cells of pre-ovulatory follicles at stage IV, approximately 1 h prior to ovulation and light on (Liu et al., 2018). Among the four metalloproteinases, adamts9 and adam8b, were significantly upregulated in follicular cells of control females paired with WT males, and WT females, lhβ- or star-deficient females exposed to DHP for 2 h (from 05:00 to 07:00), but not in lhβ- or star-deficient females paired with WT males. Thereby, it is reasonable to speculate that dysregulated expressions of adamts9 and adam8b in follicular cells of preovulatory follicles at stage IV of lhβ- or star-deficient females might be due to insufficient DHP synthesis. Thus the insufficient expressions of adamts9 and adam8b may be the cause for the impaired oocyte maturation and ovulation, which is in agreement with a previous report on the reduced expression of adam8b and adamts9 in the follicular cells of preovulatory follicles of pgr-/- females (Liu et al., 2018). We discovered that a concentration of 50 μg/L can induce oocyte maturation, ovulation, and spawning in WT females (Figure 1, Table 2). Upregulated adamts9 and adam8b expression in the follicular cells of the pre-ovulatory follicles of control females exposed to DHP from 05:00 to 07:00 mimicked the effect of natural mating with WT males (Figure 3D).
Anovulation, wherein mature oocytes were retained in the ovary, was observed in pgr-deficient females when mated with WT males. However, DHP administration was ineffective in restoring ovulation in pgr-deficient females when paired with WT males, indicating that the critical role of Pgr in ovulation. This is further demonstrated by the distinct phenotypes of pgr- and lhβ- or star-deficient females, which display impaired ovulation and oocyte maturation, respectively. Therefore, it is reasonable to hypothesize that Star plays a role in the downstream LH signaling pathway, leading to the direct synthesis of DHP to enhance oocyte maturation and ovulation, with ovulation being dependent on Pgr.
Previously, it has been reported that the intraperitoneally injection of hCG, DHP precursors (pregnenolone or progesterone), and DHP itself, partially rescued the maturation-arrested oocyte phenotypes in lhβ-deficient females (Shang et al., 2019; Chu et al., 2014). Among the chemicals, hCG administered via intraperitoneal injection successfully induced the maturation of a small number of follicles and ovulation in lhβ-deficient females. However, the report lacked clarity regarding the restorative effects of natural spawning and fertilization (Chu et al., 2014). Herein, we also evaluated the combined effect of hCG injection and DHP administration for 6 h (hCG injection at 00:00) and found no spawning and fertilized eggs from the hCG treated lhβ-deficient females mated with WT males. However, compared with the star-deficient females exposed to 300 μg/L DHP only, the improvement of the ratio of spawning, membrane broken and survival at 3 wpf were observed in star-deficient females with hCG injection and 300 μg/L DHP administration for 6 h (66.67 ± 9.43%, 72.36 ± 25.63%, and 71.60 ± 22.32%, respectively). The overall relative fecundity ratio, a novel concept evaluated in this study was that star-/- zebrafish treated with DHP and hCG exhibited a ratio at 17.98%. It is noteworthy that there was a limited improvement in these parameters in lhβ-deficient females after hCG injection and administration of 100 μg/L DHP for 6 h (Table 3). These observations suggest that although hCG is a LH analog, its function in regulating oocyte maturation and ovulation may differ in various animal species.
In channel catfish, sterilization was achieved through genetic editing of LH using a modified zinc finger nuclease technology with electroporation. This accomplishment not only enhances the comprehension of the roles of LH in farmed fish, but also represents progress towards fertility control in females of transgenic fish (Qin et al., 2016). CRISPR/Cas9 techniques have been suggested to improve economic traits. However, the application of sterilization technology can be utilized to establish fertility control strategies for genetically modified farm fish. Fertility-controlled fish, which are unable to breed naturally, offer natural biosecurity benefits by preventing escapee from mating with the WT stock and protecting intellectual property rights (Okoli et al., 2022). To the best of our knowledge, this study represents the first model with the manipulation of sex steroid-related genes, which opens up prospects for its application in the aquaculture industry, particularly in the genetic engineering of farmed fish with the aim of improving economic traits. Notably, our recent research involving zebrafish and common carp revealed that all homozygous cyp17a1-deficient fish gonads developed into testes with proper spermatogenesis; however, they lacked the typical male sexual characteristics and mating behaviors (Zhai et al., 2018; Zhai et al., 2022a). All offspring resulted from the artificial fertilization of the neomale common carp (cyp17a1-/-;XX genotype) sperm with the eggs from WT females (cyp17a1+/+;XX genotype) developed into all-female common carp. The genotype (cyp17a1+/-;XX genotype), all-ovarian differentiation (n>500) and significant growth advantage have been confirmed (Zhai et al., 2022a). The fertility of this genome-edited all-female population of common carp presents a potential eco-risk, thus hindering its application in aquaculture. This strategy offers the possibility of addressing the challenging situation. However, the “controllable fertility” strategy proposed in this study offers a solution by enabling the production and sterilization of the all-female population (cyp17a1+/-;lhβ (or star)-/-;XX). This can be achieved by crossing the cyp17a1-/-;lhβ (or star)-/-;XX males (neomales) with cyp17a1+/+;lhβ (or star)-/-;XX females, who are administered DHP. Fortunately, no obvious defect in fertility of lhβ-/- or star-/- male zebrafish was observed, suggesting that mutation of lhβ or star did not affect fertility in male fish (Shang et al., 2019). Based on the achieved relative fecundity ratio of star-/- zebrafish treated with the DHP and hCG procedure, a ratio of 17.98% was obtained (Table 3). This ratio suggests that more than 10,000 healthy fingerlings can be produced from each star-/- common carp with its egg-carrying capacity, providing an effective rescue strategy for farmed fish. However, further improvements will be required to enhance rescue efficiency. Overall, the genome-edited population of all-female carp rendered infertile via this procedure could impede the ecological risks by preventing the interbreeding of genome-edited escapees with WT carp stocks, as they are unable to reproduce naturally.
Data availability statement
The original contributions presented in the study are included in the article/Supplementary Material. Further inquiries can be directed to the corresponding author.
Ethics statement
The animal studies were approved by Guiding Principles for the Care and Use of Laboratory Animals, Institute of Hydrobiology, Chinese Academy of Sciences. The studies were conducted in accordance with the local legislation and institutional requirements. Written informed consent was obtained from the owners for the participation of their animals in this study.
Author contributions
SS: Data curation, Formal analysis, Investigation, Methodology, Writing – original draft. YZ: Data curation, Formal analysis, Investigation, Methodology, Writing – review & editing. JH: Investigation, Validation, Visualization, Writing – review & editing. QL: Investigation, Resources, Validation, Writing – review & editing, Methodology. XJ: Resources, Supervision, Visualization, Writing – review & editing. JH: Resources, Software, Supervision, Writing – review & editing. GZ: Supervision, Writing – review & editing, Conceptualization, Funding acquisition, Project administration, Writing – original draft. ZY: Conceptualization, Funding acquisition, Project administration, Supervision, Writing – original draft, Writing – review & editing.
Funding
The author(s) declare that financial support was received for the research, authorship, and/or publication of this article. This work was supported by the National Key Research and Development Program, China (2022YFD2401800 to GZ and 2022YFF1000300 to ZY), the National Natural Science Foundation, China (32230108 to ZY and 31972779 to GZ), the Foundation of Hubei Hongshan Laboratory (2021hszd021 to ZY and 2021hskf013 to GZ), the Major Science and Technology Program of Wuhan City (2022021302024854 to ZY), the Youth Innovation Promotion Association of CAS (2020336 to GZ), and the State Key Laboratory of Freshwater Ecology and Biotechnology (2016FBZ05 to ZY).
Acknowledgments
We are grateful to Professor Xiaochun Liu of Sun Yat-Sen University for the generous gift of the pgr-deficient zebrafish line. We thank Mr. Wenyou Chen of the Institute of Hydrobiology, Chinese Academy of Sciences, for handling the zebrafish stocks. We would also like to thank Ms. Guangxin Wang from the Analysis and Testing Center of Institute of Hydrobiology, Chinese Academy of Sciences for assistance with confocal microscopy.
Conflict of interest
The authors declare that the research was conducted in the absence of any commercial or financial relationships that could be construed as a potential conflict of interest.
The author(s) declared that they were an editorial board member of Frontiers, at the time of submission. This had no impact on the peer review process and the final decision.
Publisher’s note
All claims expressed in this article are solely those of the authors and do not necessarily represent those of their affiliated organizations, or those of the publisher, the editors and the reviewers. Any product that may be evaluated in this article, or claim that may be made by its manufacturer, is not guaranteed or endorsed by the publisher.
Supplementary material
The Supplementary Material for this article can be found online at: https://www.frontiersin.org/articles/10.3389/fmars.2024.1381305/full#supplementary-material
Supplementary Figure 1 | The gross appearance of offspring at diverse stages from control females, DHP-administrated lhβ- or star-deficient females naturally mated with WT males. (A–C) Representative image of the gross appearance of embryos at 12 hpf. (D–F) Representative image of the gross appearance of larvae at 72 hpf. (G–I) Representative image of the gross appearance of larvae at 5 dpf. (J–L) Representative image of the gross appearance of fish at 21 dpf. (A, D, G, J) Offspring from control females naturally mated with WT males. (B, E, H, K) offspring from lhβ-deficient females exposed to 100 μg/L DHP for 6 h and naturally mated with WT males. (C, F, I, L) Offspring from star-deficient females exposed to 300 μg/L DHP for 6 h and naturally mated with WT males. (M) The bar chart represents body weight of the fish at 1 mpf. (N) The bar chart represents body length of the fish at 1 mpf. (O) The bar chart represents full-length of fish at 1 mpf. The letter a in the bar charts indicates no significant difference.
References
Ascoli M., Fanelli F., Segaloff D. L. (2002). The lutropin/choriogonadotropin receptor, a 2002 perspective. Endocr. Rev. 23, 141–174. doi: 10.1210/edrv.23.2.0462
Chen S. X., Bogerd J., Schoonen N. E., Martijn J., de Waal P. P., Schulz R. W. (2013). A progestin (17alpha,20beta-dihydroxy-4-pregnen-3-one) stimulates early stages of spermatogenesis in zebrafish. Gen. Comp. Endocrinol. 185, 1–9. doi: 10.1016/j.ygcen.2013.01.005
Chu L. H., Li J. Z., Liu Y., Hu W., Cheng C. H. K. (2014). Targeted gene disruption in zebrafish reveals noncanonical functions of lh signaling in reproduction. Mol. Endocrinol. 28, 1785–1795. doi: 10.1210/me.2014-1061
Clelland E., Peng C. (2009). Endocrine/paracrine control of zebrafish ovarian development. Mol. Cell Endocrinol. 312, 42–52. doi: 10.1016/j.mce.2009.04.009
Crowder C. M., Lassiter C. S., Gorelick D. A. (2018). Nuclear androgen receptor regulates testes organization and oocyte maturation in zebrafish. Endocrinology 159, 980–993. doi: 10.1210/en.2017-00617
El Mohajer L., Bulteau R., Fontaine P., Milla S. (2022). Maturation inducing hormones in teleosts: Are progestogens always the first to be nominated? Aquaculture 546, 737315. doi: 10.1016/j.aquaculture.2021.737315
Gharib S. D., Wierman M. E., Shupnik M. A., Chin W. W. (1990). Molecular-biology of the pituitary gonadotropins. Endocr. Rev. 11, 177–199. doi: 10.1210/edrv-11-1-177
Gratacap R. L., Wargelius A., Edvardsen R. B., Houston R. D. (2019). Potential of genome editing to improve aquaculture breeding and production. Trends Genet. 35, 672–684. doi: 10.1016/j.tig.2019.06.006
Huang J. F., Shi C., Gao Y. P., Su J. Z., Shu Y. Q., Zeng N. M., et al. (2021). Heterozygous depletion of improves growth and feed conversion efficiency in gibel carp. Aquaculture 545, 737207. doi: 10.1016/j.aquaculture.2021.737207
Huhtaniemi I. (2006). Mutations along the pituitary-gonadal axis affecting sexual maturation: Novel information from transgenic and knockout mice. Mol. Cell Endocrinol. 254-255, 84–90. doi: 10.1016/j.mce.2006.04.015
Huhtaniemi I. T., Themmen A. P. (2005). Mutations in human gonadotropin and gonadotropin-receptor genes. Endocrine 26, 207–217. doi: 10.1385/ENDO:26:3:207
Kinkel M. D., Eames S. C., Philipson L. H., Prince V. E. (2010). Intraperitoneal injection into adult zebrafish. J. Vis. Exp. 42). doi: 10.3791/2126
Lau E. S., Zhang Z. W., Qin M. M., Ge W. (2016). Knockout of zebrafish ovarian aromatase gene (cyp19a1a) by talen and crispr/cas9 leads to all-male offspring due to failed ovarian differentiation. Sci. Rep. 6, 37357. doi: 10.1038/srep37357
Lei Z. M., Mishra S., Zou W., Xu B., Foltz M., Li X., et al. (2001). Targeted disruption of luteinizing hormone/human chorionic gonadotropin receptor gene. Mol. Endocrinol. 15, 184–200. doi: 10.1210/mend.15.1.0586
Levavi-Sivan B., Bogerd J., Mananos E. L., Gomez A., Lareyre J. J. (2010). Perspectives on fish gonadotropins and their receptors. Gen. Comp. Endocrinol. 165, 412–437. doi: 10.1016/j.ygcen.2009.07.019
Li J. Z., Ge W. (2020). Zebrafish as a model for studying ovarian development: Recent advances from targeted gene knockout studies. Mol. Cell Endocrinol. 507, 110778. doi: 10.1016/j.mce.2020.110778
Li J. Z., Niu C. Y., Cheng C. H. K. (2018). Igf3 serves as a mediator of luteinizing hormone in zebrafish ovulation. Biol. Reprod. 99, 1235–1243. doi: 10.1093/biolre/ioy143
Li N., Oakes J. A., Storbeck K. H., Cunliffe V. T., Krone N. P. (2020). The p450 side-chain cleavage enzyme cyp11a2 facilitates steroidogenesis in zebrafish. J. Endocrinol. 244, 309–321. doi: 10.1530/JOE-19-0384
Liu D. T., Carter N. J., Wu X. J., Hong W. S., Chen S. X., Zhu Y. (2018). Progestin and nuclear progestin receptor are essential for upregulation of metalloproteinase in zebrafish preovulatory follicles. Front. Endocrinol. (Lausanne). 9. doi: 10.3389/fendo.2018.00517
Liu D. T., Hong W. S., Chen S. X., Zhu Y. (2020). Upregulation of adamts9 by gonadotropin in preovulatory follicles of zebrafish. Mol. Cell Endocrinol. 499, 110608. doi: 10.1016/j.mce.2019.110608
Lu H. J., Cui Y., Jiang L. W., Ge W. (2017). Functional analysis of nuclear estrogen receptors in zebrafish reproduction by genome editing approach. Endocrinology 158, 2292–2308. doi: 10.1210/en.2017-00215
Ma X. P., Dong Y. L., Matzuk M. M., Kumar T. R. (2004). Targeted disruption of luteinizing hormone beta-subunit leads to hypogonadism, defects in gonadal steroidogenesis, and infertility. Proc. Natl. Acad. Sci. U S A. 101, 17294–17299. doi: 10.1073/pnas.0404743101
McGee E. A., Hsueh A. J. (2000). Initial and cyclic recruitment of ovarian follicles. Endocr. Rev. 21, 200–214. doi: 10.1210/edrv.21.2.0394
Nagahama Y., Yamashita M. (2008). Regulation of oocyte maturation in fish. Dev. Growth Differ. 50 Suppl 1, S195–S219. doi: 10.1111/j.1440-169X.2008.01019.x
Nagahama Y., Yoshikuni M., Yamashita M., Tokumoto T., Katsu Y. (1995). Regulation of oocyte growth and maturation in fish. Curr. topics Dev. Biol. 30, 103–145. doi: 10.1016/S0070-2153(08)60565-7
Okoli A. S., Blix T., Myhr A. I., Xu W. T., Xu X. D. (2022). Sustainable use of crispr/cas in fish aquaculture: The biosafety perspective. Transgenic Res. 31, 1–21. doi: 10.1007/s11248-021-00274-7
Qin Z. K., Li Y., Su B. F., Cheng Q., Ye Z., Perera D. A., et al. (2016). Editing of the luteinizing hormone gene to sterilize channel catfish, ictalurus punctatus, using a modified zinc finger nuclease technology with electroporation. Mar. Biotechnol. (NY). 18, 255–263. doi: 10.1007/s10126-016-9687-7
Shang G. H., Peng X. Y., Ji C., Zhai G., Ruan Y. L., Lou Q. Y., et al. (2019). Steroidogenic acute regulatory protein and luteinizing hormone are required for normal ovarian steroidogenesis and oocyte maturation in zebrafishdagger. Biol. Reprod. 101, 760–770. doi: 10.1093/biolre/ioz132
Shi S. C., Shu T. T., Li X., Lou Q. Y., Jin X., He J. Y., et al. (2022). Characterization of the interrenal gland and sexual traits development in cyp17a2-deficient zebrafish. Front. Endocrinol. (Lausanne). 13. doi: 10.3389/fendo.2022.910639
Shu T. T., Zhai G., Pradhan A., Olsson P. E., Yin Z. (2020). Zebrafish cyp17a1 knockout reveals that androgen-mediated signaling is important for male brain sex differentiation. Gen. Comp. Endocrinol. 295, 113490. doi: 10.1016/j.ygcen.2020.113490
Tang H. P., Chen Y., Wang L., Yin Y. K., Li G. F., Guo Y., et al. (2018). Fertility impairment with defective spermatogenesis and steroidogenesis in male zebrafish lacking androgen receptor. Biol. Reprod. 98, 227–238. doi: 10.1093/biolre/iox165
Tang H. P., Liu Y., Li J. Z., Yin Y. K., Li G. F., Chen Y., et al. (2016). Gene knockout of nuclear progesterone receptor provides insights into the regulation of ovulation by lh signaling in zebrafish. Sci. Rep. 6, 28545. doi: 10.1038/srep28545
Tokumoto T., Yamaguchi T., Ii S., Tokumoto M. (2011). In vivo induction of oocyte maturation and ovulation in zebrafish. PloS One 6, e25206. doi: 10.1371/journal.pone.0025206
Westerfield M. (2000). The zebrafish book, a guide for the laboratory use of zebrafish (danio rerio). 4th ed (Eugene, OR: University of Oregon Press).
Wu X. J., Liu D. T., Chen S. X., Hong W. S., Zhu Y. (2020). Impaired oocyte maturation and ovulation in membrane progestin receptor (mpr) knockouts in zebrafish. Mol. Cell Endocrinol. 511, 110856. doi: 10.1016/j.mce.2020.110856
Yin Y. K., Tang H. P., Liu Y., Chen Y., Li G. F., Liu X. C., et al. (2017). Targeted disruption of aromatase reveals dual functions of cyp19a1a during sex differentiation in zebrafish. Endocrinology 158, 3030–3041. doi: 10.1210/en.2016-1865
Yu G. Q., Zhang D. W., Liu W., Wang J., Liu X., Zhou C., et al. (2018). Zebrafish androgen receptor is required for spermatogenesis and maintenance of ovarian function. Oncotarget 9, 24320–24334. doi: 10.18632/oncotarget.v9i36
Zhai G., Shu T. T., Chen K. X., Lou Q. Y., Jia J. Y., Huang J. F., et al. (2022a). Successful production of an all-female common carp (cyprinus carpio l.) population using cyp17a1-deficient neomale carp. Engineering-Prc 8, 181–189. doi: 10.1016/j.eng.2021.03.026
Zhai G., Shu T. T., Xia Y. G., Lu Y., Shang G. H., Jin X., et al. (2018). Characterization of sexual trait development in cyp17a1-deficient zebrafish. Endocrinology 159, 3549–3562. doi: 10.1210/en.2018-00551
Zhai G., Shu T., Yu G., Tang H., Shi C., Jia J., et al. (2022b). Augmentation of progestin signaling rescues testis organization and spermatogenesis in zebrafish with the depletion of androgen signaling. Elife 11, 66118. doi: 10.7554/eLife.66118
Zhang F. P., Poutanen M., Wilbertz J., Huhtaniemi I. (2001). Normal prenatal but arrested postnatal sexual development of luteinizing hormone receptor knockout (lurko) mice. Mol. Endocrinol. 15, 172–183. doi: 10.1210/mend.15.1.0582
Zhang Z. W., Zhu B., Ge W. (2015). Genetic analysis of zebrafish gonadotropin (fsh and lh) functions by talen-mediated gene disruption. Mol. Endocrinol. 29, 76–98. doi: 10.1210/me.2014-1256
Zhu Y., Liu D. T., Shaner Z. C., Chen S. X., Hong W. S., Stellwag E. J. (2015). Nuclear progestin receptor (pgr) knockouts in zebrafish demonstrate role for pgr in ovulation but not in rapid non-genomic steroid mediated meiosis resumption. Front. Endocrinol. (Lausanne). 6. doi: 10.3389/fendo.2015.00037
Keywords: gonadotropin, luteinizing hormone, DHP, controllable sterility, eco-friendly manner
Citation: Shi S, Zhang Y, Huang J, Lou Q, Jin X, He J, Zhai G and Yin Z (2024) Effective “off-on” switch for fertility control in female zebrafish. Front. Mar. Sci. 11:1381305. doi: 10.3389/fmars.2024.1381305
Received: 03 February 2024; Accepted: 26 February 2024;
Published: 11 March 2024.
Edited by:
Jingzhen Wang, Beibu Gulf University, ChinaReviewed by:
Yong Zhu, East Carolina University, United StatesYao Zheng, Chinese Academy of Fishery Sciences (CAFS), China
Wensheng Li, Sun Yat-sen University, China
Copyright © 2024 Shi, Zhang, Huang, Lou, Jin, He, Zhai and Yin. This is an open-access article distributed under the terms of the Creative Commons Attribution License (CC BY). The use, distribution or reproduction in other forums is permitted, provided the original author(s) and the copyright owner(s) are credited and that the original publication in this journal is cited, in accordance with accepted academic practice. No use, distribution or reproduction is permitted which does not comply with these terms.
*Correspondence: Gang Zhai, zhaigang@ihb.ac.cn; Zhan Yin, zyin@ihb.ac.cn
†These authors have contributed equally to this work