- 1Department of Marine Zoology, Section Crustacea, Senckenberg Institute and Natural History Museum Frankfurt, Frankfurt am Main, Germany
- 2Institute for Ecology, Diversity and Evolution, Goethe University Frankfurt, Frankfurt am Main, Germany
Accurate taxonomic classification of deep-sea taxa is often impeded by the presence of highly morphologically similar but genetically distinct species. This issue is particularly pronounced in the isopods of the deep-sea family Macrostylidae, which exhibit remarkably low morphological variation despite significant genetic diversity. In this study, we present the first application of geometric morphometric techniques to 41 specimens across five species of deep-sea macrostylid isopods collected from Icelandic waters. Our results suggest that geometric morphometric techniques can effectively discriminate between macrostylid species. These techniques, hence, promise to be an important addition to the toolset of macrostylid taxonomists.
1 Introduction
Geometric morphometrics has emerged as an important addition to the taxonomic toolset (Mutanen and Pretorius, 2007; Ludoški et al., 2008; Francuski et al., 2009; Roggero et al., 2013; Mitrovski-Bogdanović et al., 2014; Siriwut et al., 2015; Karanovic et al., 2016; Li et al., 2016; Grinang et al., 2019). This approach combines multivariate statistics with Cartesian coordinates to quantify shape variation, making it effective for identifying the subtle morphological differences that traditional taxonomic approaches may overlook (see e.g., Fukami et al., 2004; Bridge et al., 2023). Numerous studies have successfully applied geometric morphometric techniques to investigate cryptic species (usually defined as co-occurring species indistinguishable by the human eye despite high genetic distinctness), discovering new taxa, and identifying new taxonomically informative traits across a wide range of taxa (insects: Mutanen and Pretorius, 2007; Ludoški et al., 2008; Francuski et al., 2009; centipedes: Siriwut et al., 2015; copepods: Karanovic et al., 2016; decapods: Grinang et al., 2019; Lovrenčić et al., 2020; Casaubon et al., 2023; ostracods: Ligios and Gliozzi, 2012; tardigrades: Fontoura and Morais, 2011). However, the application of geometric morphometrics is completely lacking for some taxonomic groups, including the deep-sea isopod family Macrostylidae Hansen, 1916.
The family Macrostylidae, comprising a single genus Macrostylis Sars, 1864, includes isopods with a global distribution spanning sublittoral (M. spinifera Sars, 1864, found at approximately 4 m) to hadal zones (M. mariana Mezhov, 1993, found at approximately 11,000 m) (Mezhov, 1992; Menzies, 1962; Brandt, 2002; 2004; Riehl et al., 2012). Despite an extensive distribution and significant molecular divergence, macrostylid isopods exhibit remarkably low morphologic variation (Riehl, 2014). Additionally, these isopods display varying degrees of sexual dimorphism, with copulatory (terminal) males having pronounced morphological differences from both subadult and females (Riehl et al., 2012), further complicating species diagnoses and allocation of conspecifics based solely on morphology.
Historically, macrostylid taxonomy has relied heavily on the comparative analysis of morphological characters and their linear measurements and ratios (Mezhov, 2003; Brandt, 2004; Vey and Brix, 2009; Riehl et al., 2012; Riehl and Brandt, 2013). Recent research on macrostylid isopods has increasingly employed integrative taxonomic techniques that combine molecular genetics and traditional morphometrics (Riehl et al., 2012; Riehl and Brandt, 2013; Bober et al., 2018). However, there are no known studies employing geometric morphometric techniques on Macrostylidae or other deep-sea isopods. This study represents the first application of geometric morphometric techniques in macrostylid taxonomy, aiming to: 1) evaluate the efficacy of these methods, and 2) to investigate various species of macrostylid isopods.
2 Materials and methods
Five species of macrostylid isopods (Figure 1) were used in this study: M. spinifera Sars, 1864, M. sp. aff. spinifera, M. subinermis Hansen, 1916, M. longiremis Hansen, 1916, and M. magnifica Wolff, 1962. The specimens used here (Table 1) were collected during research campaigns for several projects spanning from 1992 to 2014, including the BIOICE project (Benthic Invertebrates of Icelandic Waters; Brix and Svavarrson, 2010; Brattegard et al., 2019; Steingrímsson et al., 2020), the IceAGE project (Icelandic Marine Animals: Genetics and Ecology, 2008), and the PolySkag project (Polychaetes in coastal areas of the Skaggerak, 2014; Oug et al., 2015). All specimens analyzed here are deposited in the collections at the Senckenberg Natural History Museum in Frankfurt, Germany.
Initial species identifications were based on established taxonomic descriptions of macrostylid isopods (Sars, 1864, 1899; Meinert, 1890; Hansen 1916; Wolff, 1962). While there is some genetic data (16S and 18S, unpublished data) for macrostylids from the IceAGE and PolySkag projects, specimens collected during the BIOICE expedition lack genetic data due to the use of formaldehyde as a preservative.
A total of 41 subadult (preparatory) and adult (copulatory) specimens were used in geometric morphometric analyses. Only female isopods were used as they are more abundant in collections (Riehl et al., 2012) and are difficult to distinguish using morphology alone (Riehl et al., 2012), making them ideal candidates for use in geometric morphometric analyses.
The pleotelson was chosen as it is an important diagnostic character when used in conjunction with other morphological characters. It is also easier to position and photograph in a standard view compared to the third pereopod ischium or the operculum. As such, each pleotelson was photographed in dorsal view using a Leica M165C stereomicroscope equipped with a Leica DMC5400 20 Megapixel color CMOS camera. Images were saved in TIFF format using the Leica Application Suite (LAS X). The tpsUtil (Rohlf, 2015) program was used to prepare the images for landmarking and the tpsDig (Rohlf, 2015) program was used to digitize landmarks and semilandmarks. MorphoJ 1.07a (Klingenberg, 2011) was used for all subsequent analyses.
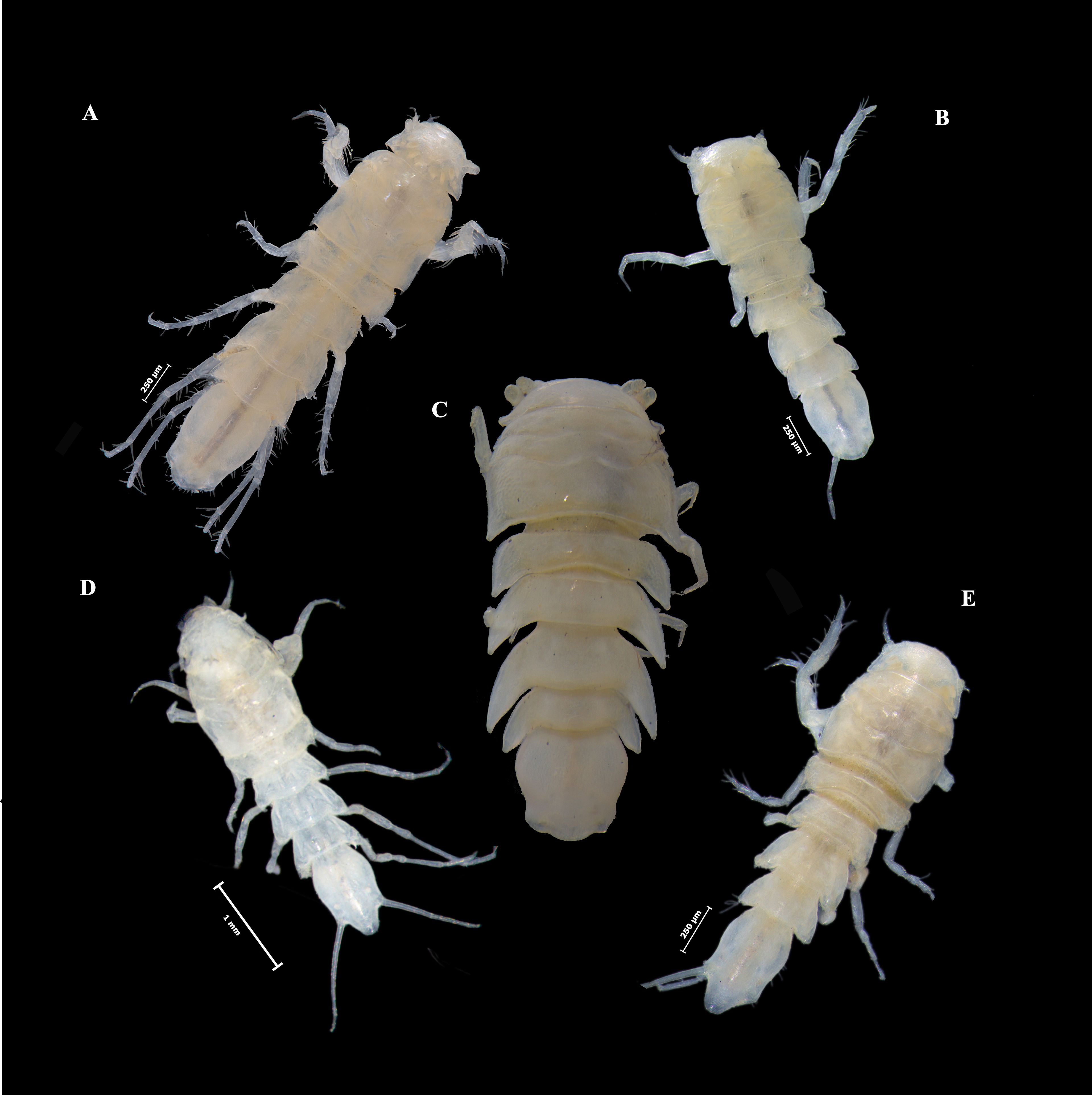
Figure 1. Female macrostylid isopods used in the present study. (A) Macrostylis longiremis; (B) M. subinermis; (C) M. magnifica; (D) M. sp. aff. spinifera; (E) M. spinifera.
Though there are a few studies that have applied geometric morphometric techniques to isopod taxa (Santamaria et al., 2013; Ismail 2021; Kamilari and Sfenthourakis, 2009; Kim et al., 2021), to our knowledge none have focused on macrostylid isopods. Previous studies on isopod taxa have focused primarily on body shape, on pleopodal appendages (Kamilari and Sfenthourakis, 2009), or have employed destructive techniques (Bertin et al., 2002). Here, we selected homologous landmarks across macrostylid species that captured the most amount of shape variation using non-destructive techniques. Additionally, given the pleotelson is a symmetrical character (Schultz, 1969) and an excessive number of landmarks can reduce statistical power (Rufino et al., 2006; Mitteroecker et al., 2013), we selected three landmarks and 66 semi-landmarks comprising half of the pleotelson (Figure 2). Landmark 1 (lmk1) represents the point where the lateral pleotelson outline meets the 7th pereonite. Landmark 2 (lmk2) represents the midpoint of the posterior apex of the pleotelson, the position and shape of which appears to vary widely between macrostylids (e.g., M. spinifera vs. M. subinermis). Landmark 3 (lmk3) represents the maximum curvature of the point where the uropod inserts into the pleotelson (see arrow in Figure 2). The semi-landmarks are anchored between lmk 1 and lmk 2 and capture the lateral and posterior margins of the pleotelson.
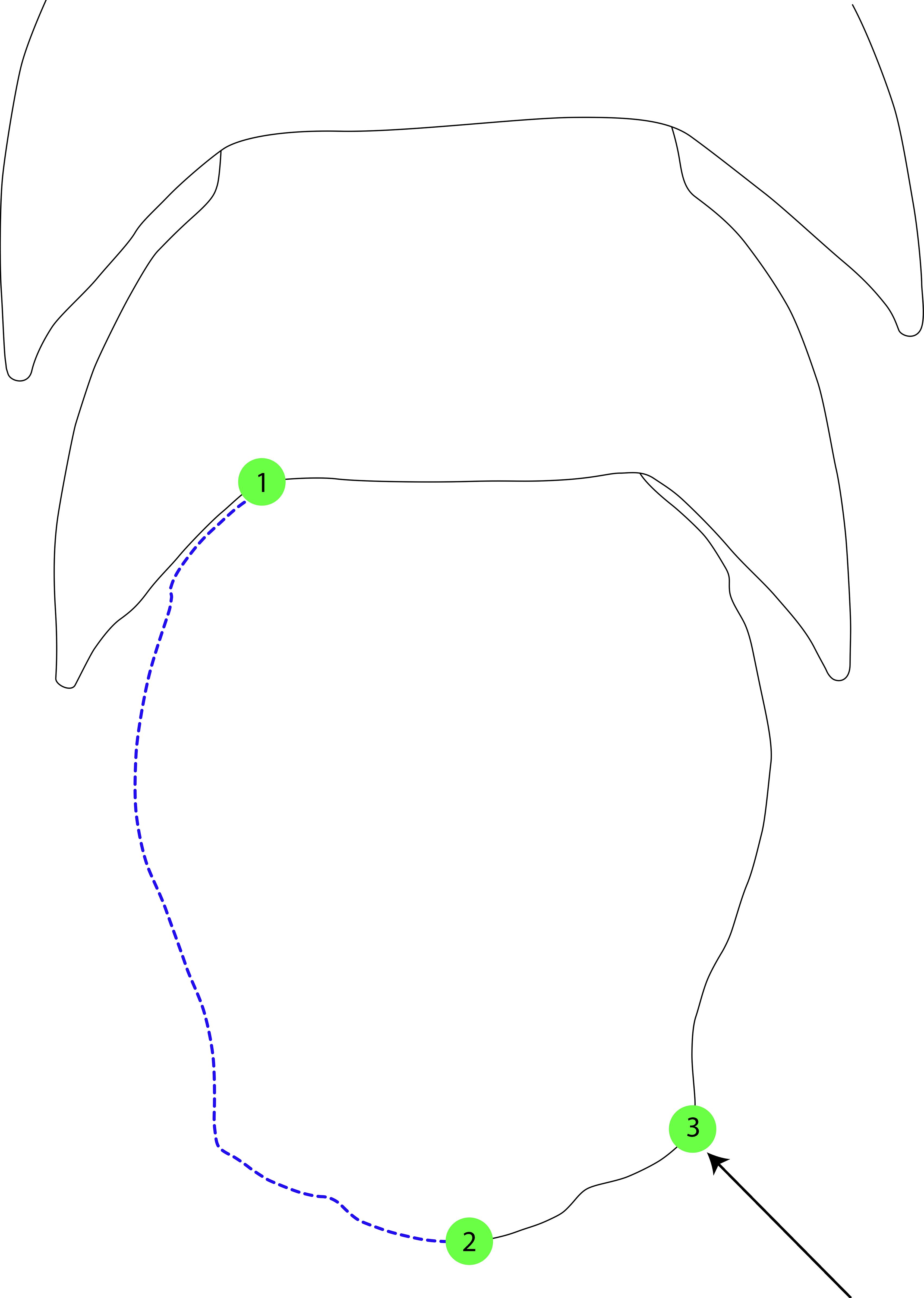
Figure 2. Landmarks and semi-landmarks on the pleotelson of Macrostylis spp. identified for use in geometric morphometric analyses. Closed red dots denote landmarks (n = 2), dashed blue lines denote the curve along which the semi-landmarks were placed.
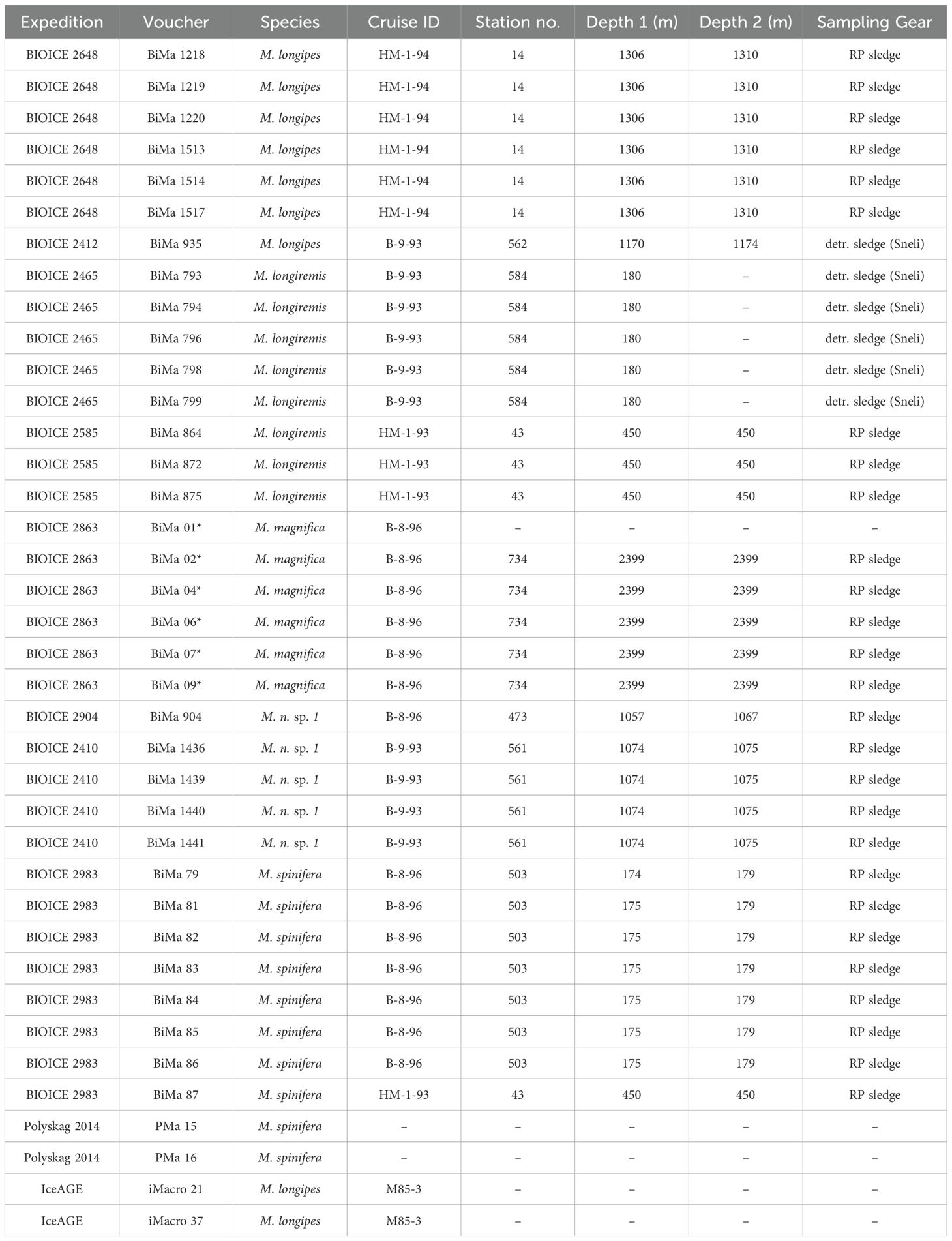
Table 1. Macrostylid specimens examined for the application of geometric morphometric techniques in this study.
A Procrustes superimposition method was used to standardize landmark data and generate Procrustes shape coordinates by translating, scaling, and rotating the raw coordinate data (Adams and Otárola-Castillo, 2013). The Procrustes shape coordinates generated were then used for principal component analyses (PCA) and canonical variate analyses (CVA). A PCA was performed to visualize and quantify pleotelson shape variation. The PCA created a morphospace (Figure 3) for visualizing shape variation, with each point representing the pleotelson shape of a macrostylid isopod. Points closer together on the PCA morphospace indicate more similar shapes, while points farther away indicate more dissimilar shapes. A CVA with a permutation test for pairwise distances (10,000 iterations) was performed to analyze interspecific shape variation in the pleotelson. The CVA (Figure 4) maximized the distance between individuals of different groups (i.e., species) while minimizing the distance between individuals of the same groups.
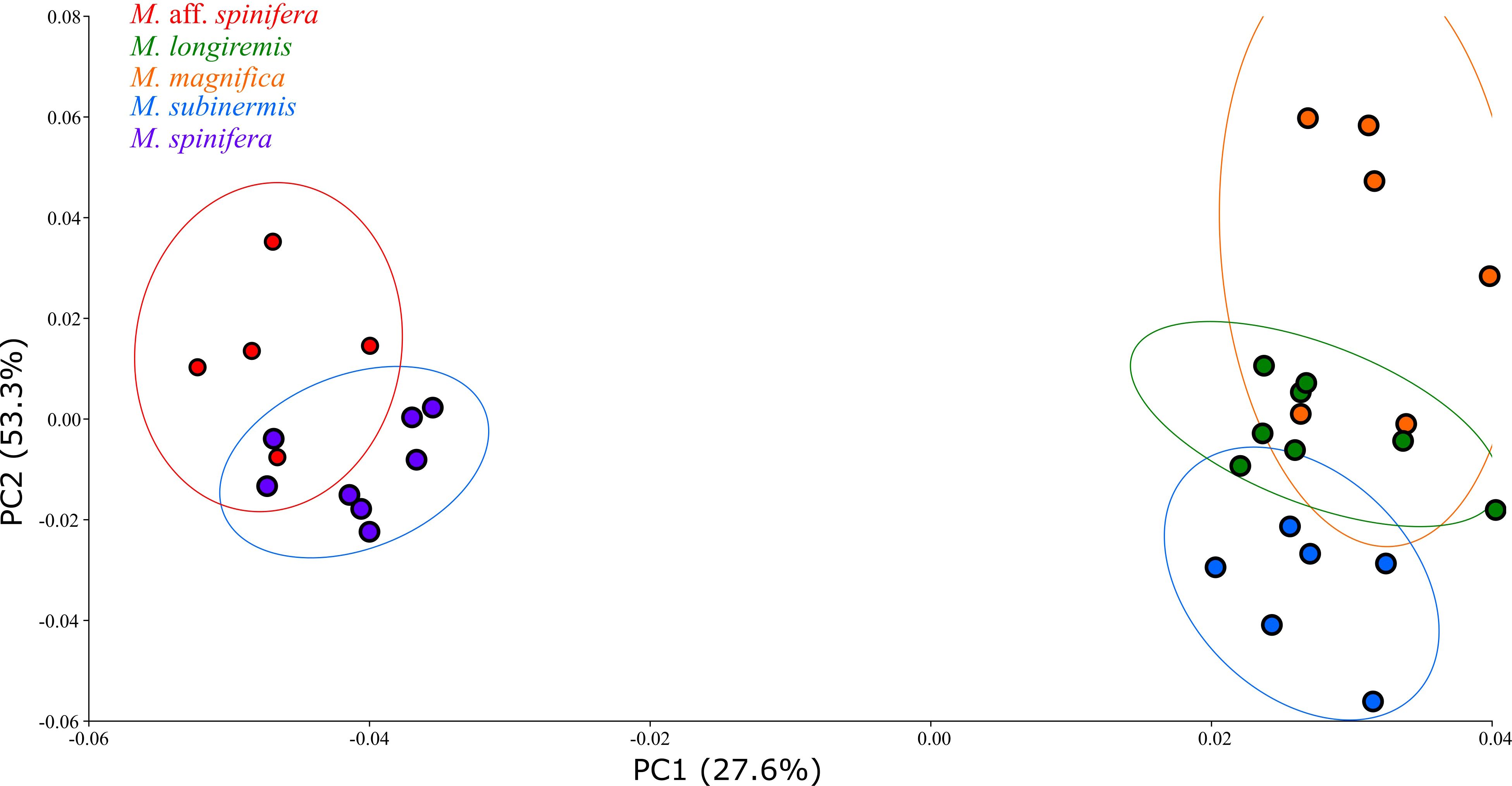
Figure 3. Scatter plot of the principal component analysis (PCA) performed on macrostylid isopods along the first two principal axes. Isopod specimens (dots) are colored by species.
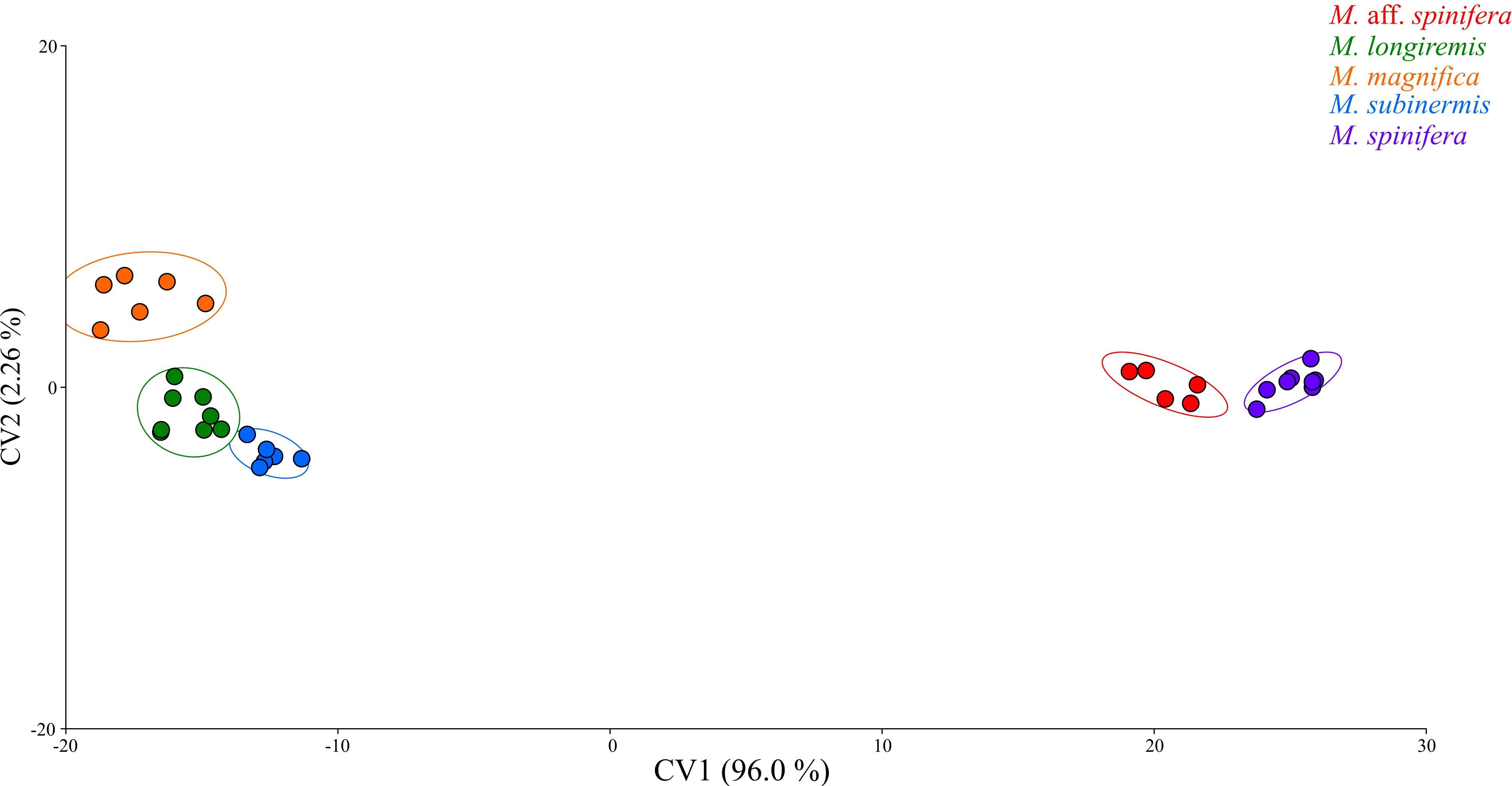
Figure 4. Canonical variate analysis (CVA) plot of pleotelson shape data of the macrostylid species used in the present study.
The Procrustes and Mahalanobis distances (Table 2) generated from the CVA were used to determine the statistical significance of the permutation tests. The Procrustes distance measured the absolute magnitude of shape deviation (Klingenberg and Monteiro, 2005), while the Mahalanobis distance measured how different an individual species was from others (Klingenberg and Monteiro, 2005).
Finally, a Procrustes ANOVA (analysis of variance; pANOVA) was conducted to assess the significance of pleotelson shape variation between the macrostylids studied here. The significance level (p-value) for all analyses was set to 0.05, with p-values less than 0.05 considered statistically significant.
3 Results
The results of the pANOVA revealed significant differences in pleotelson shape between macrostylid species (p < 0.0001; Table 2). The first two principal components accounted for 80.9% (PC1 53.3%, PC2 27.6%) of total variance. A scatter plot of the first two principal components (Figure 3) showed M. spinifera and M. sp. aff. spinifera clustering together but not overlapping in their distribution in the negative PC axis, M. subinermis forming its own distinct cluster in the positive PC axis, and M. magnifica slightly overlapping with M. longiremis in the positive PC axis. The first principal component accounted for most of the morphological variation and primarily showed changes in the posterolateral margins and the positioning of the pleotelson posterior apex. The pleotelson shape of species on the negative PC1, i.e., M. spinifera and M. sp. aff. spinifera, was more hourglass shaped with a waist. The posterolateral margins and the posterior apex of these two species are very different from the remaining species clustered on the positive PC2 axis. The second principal component primarily showed changes in the lateral margins and overall pleotelson shape.
The first two canonical variates accounted for 98.3% (CV1 96.0%, CV2 2.26%) of total variance. A scatter plot of the first two canonical variates (Figure 4) showed all five species completely separated. Macrostylis spinifera and M. sp. aff. spinifera clustered closely together but did not overlap in the positive CV1 axis, M. subinermis and M. longiremis overlapped in their distribution in the negative CV1 axis while M. magnifica clustered by itself in the extreme negative CV1 axis. On average, the species on the positive extremes, i.e., M. spinifera and M. sp. aff. spinifera, were characterized by an hourglass shaped pleotelson with a waist while the species on the negative extremes, i.e., M. magnifica, M. subinermis, and M. longiremis, were characterized by a pleotelson with more parallel lateral margins. Similarly, the second canonical variate (CV2) axis also demonstrated shape changes in the posterior end of the pleotelson, with the species on the positive extremes having a less pronounced waist, compared to the isopods on the negative extremes having a more pronounced waist.
4 Discussion
Our application of geometric morphometric techniques to the pleotelson of macrostylid isopods successfully differentiated between the five species studied here, revealing subtle morphological differences even between highly morphologically similar taxa. Despite their high levels of genetic divergence, macrostylid isopods exhibit remarkable morphological homogeneity (Riehl and Brandt, 2010; Riehl and Brandt, 2013) significantly impeding accurate species diagnoses and complicating efforts at taxonomic reorganization. Our results demonstrate that geometric morphometric techniques are excellent at detecting the subtle morphological differences that separate species which are highly morphologically similar.
As a prime example, the lack of overlap between M. spinifera and M. sp. aff. spinifera was unexpected given the high morphological similarity their females exhibit. Instead, geometric morphometric techniques clearly discriminated the two species. The distinction between M. spinifera and M. sp. aff. spinifera is ostensible in their males. The males of M. sp. aff. spinifera have strikingly elongated sixth and seventh pereopods that closely resemble those of M. longipes. However, this rather strong expression of sexual dimorphism impedes allocation of conspecific male and female specimens (see also, e.g., Riehl et al., 2012); without the application of geometric morphometrics or genetics, the females may easily be taken for M. spinifera by an unexperienced identifier while the males may be identified either as M. longipes, or as a separate species without conspecific females in the samples. Interestingly, Hansen (1916) was the first one to report variation within M. spinifera. He remarked on an “atypical form” of a female macrostylid collected from the Davis Strait, which differed from the “typical form” of M. spinifera in its pleotelson shape along with differences in other morphological characters. More recently, a Bayesian phylogenetic reconstruction using 16S mitochondrial DNA from macrostylids recovered M. spinifera in two clades (Riehl, 2014). Hansen’s (1916) remarks on a different form of M. spinifera, coupled with phylogenetic results (Riehl 2014, unpublished chapter1) suggest that there may be more than one species hidden under M. spinifera. As shown here, an approach integrating geometric morphometrics is useful for further investigating the morphological differences between females of these two species.
While our results demonstrated a successful application of geometric morphometric techniques, our study was limited in several respects. First, we were restricted by a small sample size (N = 5–10) for all species excluding M. spinifera. A small sample size is correlated with an increased risk of type II error and reduces the power of the statistical analyses being undertaken (Columb and Atkinson, 2016). Second, because of a limited number of specimens available to us and the enormous and cost-prohibitive sampling efforts required to collect deep-sea specimens, we avoided dissections or any techniques that would physically damage specimens. As such, this study was limited to only the pleotelson as it was the easiest to standardize without dissection. Still our results demonstrate the potential of geometric morphometrics as a powerful tool in macrostylid taxonomy, highlighting efficiency and applicability without the necessity of time-consuming and specimen-harming preparation.
Our findings introduce a promising new direction for research in macrostylid taxonomy and suggest that geometric morphometric techniques are a useful addition to the existing set of tools used in this field. As shown here, geometric morphometric techniques are especially useful for elucidating shape differences between female macrostylid isopods, which can be difficult to differentiate using traditional morphology. Within the scope of macrostylid taxonomy, we expect that future studies will integrate these techniques in their approach and expand the use of geometric morphometrics to not only assess other diagnostically informative body parts, such as the fossosome and the operculum but also assess the efficacy of new taxonomic characters in macrostylid taxonomy. Outside the scope of macrostylid taxonomy, geometric morphometric techniques may be applicable to other deep-sea isopod families to support taxonomic efforts. Geometric morphometric techniques may also be useful in other deep-sea asellotes that face similar difficulties in efforts at taxonomic reorganization (Raupach et al., 2009; Brix et al., 2011).
Data availability statement
The original contributions presented in the study are included in the article/supplementary material. Further inquiries can be directed to the corresponding author.
Ethics statement
The manuscript presents research on animals that do not require ethical approval for their study.
Author contributions
AC: Data curation, Formal analysis, Investigation, Methodology, Validation, Visualization, Writing – original draft, Writing – review & editing. TR: Data curation, Funding acquisition, Writing – review & editing.
Funding
The author(s) declare financial support was received for the research, authorship, and/or publication of this article. The present study is part of the Ph.D. project of the first author conducted in the framework of the Senckenberg Ocean Species Alliance (SOSA), which is a research and conservation program funded through a philanthropic donation. This is SOSA contribution #24.
Acknowledgments
We are grateful to Jörundur Svavarsson for his hospitality and support during a stay of TR at the University of Iceland and for providing the isopod material from the BIOICE expedition. We are thankful to Andreas H.J. Kelch for his assistance with the pleotelson illustration used in the present paper. We thank the two reviewers for their comments and suggestions. We are thankful to Nicolas Casaubon for his insightful comments and invaluable support. Lastly, we are thankful to Dr. Angelika Brandt and the teams of the Senckenberg Crustacea section and the Senckenberg Ocean Species Alliance (SOSA) for their invaluable support.
Conflict of interest
The authors declare that the research was conducted in the absence of any commercial or financial relationships that could be construed as a potential conflict of interest.
Publisher’s note
All claims expressed in this article are solely those of the authors and do not necessarily represent those of their affiliated organizations, or those of the publisher, the editors and the reviewers. Any product that may be evaluated in this article, or claim that may be made by its manufacturer, is not guaranteed or endorsed by the publisher.
References
Adams D. C., Otárola-Castillo E. (2013). geomorph: an r package for the collection and analysis of geometric morphometric shape data. Methods Ecol. Evol. 4, 393–399. doi: 10.1111/2041-210X.12035
Bertin A., et al. (2002). Quantification of sexual dimorphism in Asellus aquaticus (Crustacea: Isopoda) using outline approaches. Biol. J. Linn. Soc. 77, 523–533. doi: 10.1046/j.1095-8312.2002.00125.x
Bober S., et al. (2018). New Macrostylidae (Isopoda) from the Northwest Pacific Basin described by means of integrative taxonomy with reference to geographical barriers in the abyss. Zoological J. Linn. Soc. 182, 549–603. doi: 10.1093/zoolinnean/zlx042
Brandt A. (2002). Desmostylis gerdesi, a new species (Isopoda: Malacostraca) from kapp norvegia, weddell sea, antarctica. Proc. Biol. Soc. Washington 115 (3), 616–627.
Brandt A. (2004). New deep-sea species of Macrostylidae (Asellota: Isopoda: Malacostraca) from the Angola Basin off Namibia, South West Africa. Zootaxa 448, 1. doi: 10.11646/zootaxa.448.1.1
Brattegard T., Steingrímsson S.A., Svavarsson J., Helgason G.V., Gudmundsson G., Sneli J.A., et al. (2019). BIOICE: The Benthic Invertebrates of Icelandic waters stationlist (Marine Data Archive).
Bridge T. C. L., Cowman P. F., Quattrini A. M., Bonito V. E., Sinniger F., Harii S., et al. (2023). A tenuis relationship: traditional taxonomy obscures systematics and biogeography of the “Acropora tenuis” (Scleractinia: Acroporidae) species complex. Zoological J. Linn. Soc. 202, 1–24. doi: 10.1093/zoolinnean/zlad062
Brix S., Riehl T., Leese F. (2011). First genetic data for species of the genus haploniscus richardso(Isopoda: Asellota: Haploniscidae) from neighbouring deep-sea basins in the south atlantic. Zootaxa 2838 (1), 79–84.
Brix S., Svavarsson J. (2010). Distribution and diversity of desmosomatid and nannoniscid isopods (Crustacea) on the greenland–Iceland–Faeroe ridge. Polar Biol. 33, 515–530.
Casaubon A., Hultgren K. M., Murray C., Hanscom R. J., Hurt C. (2023). Application of integrative taxonomy combining phylogenetic and geometric morphometric techniques in a snapping shrimp (Alpheus) species complex (Decapoda: Caridea: Alpheidae). J. Crustacean Biol. 43. doi: 10.1093/jcbiol/ruad078
Columb M., Atkinson M. (2016). Statistical analysis: sample size and power estimations. BJA Educ. 16, 159–161. doi: 10.1093/bjaed/mkv034
Fontoura P., Morais P. (2011). Assessment of traditional and geometric morphometrics for discriminating cryptic species of the Pseudechiniscus suillus complex (Tardigrada, Echiniscidae). J. Zoological Systematics Evolutionary Res. 49, 26–33. doi: 10.1111/j.1439-0469.2010.00594.x
Francuski L., Vujić A., Kovačević A., Ludoški J., Milankov V. (2009). Identification of the species of the Cheilosia variabilis group (Diptera, Syrphidae) from the Balkan Peninsula using wing geometric morphometrics, with the revision of status of C. melanopa redi Vujic 1996. Contributions To Zool. - CONTRIB ZOOL 78, 129–140. doi: 10.1163/18759866-07803004
Fukami H., Budd A. F., Paulay G., Solé-Cava A., Allen Chen C., Iwao K., et al. (2004). Conventional taxonomy obscures deep divergence between Pacific and Atlantic corals. Nature 427, 832–835. doi: 10.1038/nature02339
Grinang J., Das I., Ng P. K. L. (2019). Geometric morphometric analysis in female freshwater crabs of Sarawak (Borneo) permits addressing taxonomy-related problems. PeerJ 7, e6205. doi: 10.7717/peerj.6205
Ismail T. G. (2021). Seasonal shape variations, ontogenetic shape changes, and sexual dimorphism in a population of land isopod Porcellionides pruinosus: a geometric morphometric study. J. Basic Appl. Zoology 82, 1–15. doi: 10.1186/s41936-021-00209-y
Kamilari M., Sfenthourakis S. (2009). A morphometric approach to the geographic variation of the terrestrial isopod species Armadillo tuberculatus (Isopoda: Oniscidea). J. Zoological Systematics Evolutionary Res. 47 (3), 219–226. doi: 10.1111/j.1439-0469.2008.00510.x
Karanovic T., Djurakic M., Eberhard S. M. (2016). Cryptic species or inadequate taxonomy? Implementation of 2D geometric morphometrics based on integumental organs as landmarks for delimitation and description of copepod taxa. Systematic Biol. 65, 304–327. doi: 10.1093/sysbio/syv088
Kim J., Kim J., Lee W., Karanovic I. (2021). The first insight into the patterns of size and shape variation of a microcerberid isopod. Water 13, 515. doi: 10.3390/w13040515
Klingenberg C. P., Monteiro L. R. (2005). Distances and directions in multidimensional shape spaces: implications for morphometric applications. Systematic Biol. 54 (4), 678–688. doi: 10.1080/10635150590947258
Klingenberg C. P. (2011). MorphoJ: an integrated software package for geometric morphometrics. Mol. Ecol. Resour. 11, 353–357. doi: 10.1111/j.1755-0998.2010.02924.x
Li L., Qi Y., Yang Y., Bai M. (2016). A new species of Falsopodabrus Pic characterized with geometric morphometrics (Coleoptera, Cantharidae). ZooKeys 614), 97–112. doi: 10.3897/zookeys.614.6156
Ligios S., Gliozzi E. (2012). The genus cyprideis jones 1857 (Crustacea, Ostracoda) in the Neogene of Italy: A geometric morphometric approach. Rev. Micropaléontologie 55, 171–207. doi: 10.1016/j.revmic.2012.09.002
Lovrenčić L., Pavić V., Majnarić S., Abramović L., Jelić M., Maguire I. (2020). Morphological diversity of the stone crayfish – traditional and geometric morphometric approach. Knowledge Manage. Aquat. Ecosyst. 421), 1. doi: 10.1051/kmae/2019042
Ludoški J., Francuski L., Vujić A., Milankov V. (2008). The Cheilosia canicularis group (Diptera: Syrphidae): Species delimitation and evolutionary relationships based on wing geometric morphometrics. Zootaxa 1825, 40–50. doi: 10.11646/zootaxa.1825.1.4
Meinert F. V. A. (1890). Crustacea Malacostraca. Videnskabelige udbytte af kanonbaden “Hauchs Dogter”, 147–230 (In Danish).
Menzies R. J. (1962). The isopods of abyssal depths in the atlantic ocean. Columbia Univ. Press Vema Res. Ser. 1, 79–206.
Mezhov B. V. (1992). Two new species of the genus macrostylis GO sar(Crustacea isopoda asellota macrostylidae) from the antarctic. Arthropoda Selecta 1 (2), 83–87.
Mezhov B. V. (1993). Three new species of macrostylis GO sar(Crustacea isopoda asellota macrostylidae) from the pacific ocean. Arthropoda Selecta 2 (3), 3–9.
Mezhov B. V. (2003). New abyssal species of the genus Macrostylis G.O. Sars 1864 (Crustacea: Isopoda: Macrostylidae) from the northwestern part of the Indian Ocean. 12 (1), 1–8.
Mitrovski-Bogdanović A., Tomanović Ž., Mitrović M., Petrović A., Ivanović A., Žikić V., et al. (2014). The Praon dorsale–yomenae s.str. complex (Hymenoptera, Braconidae, Aphidiinae): Species discrimination using geometric morphometrics and molecular markers with description of a new species. Zoologischer Anzeiger - A J. Comp. Zool. 253, 270–282. doi: 10.1016/j.jcz.2014.02.001
Mitteroecker P., Gunz P., Windhager S., Schaefer K. (2013). A brief review of shape, form, and allometry in geometric morphometrics, with applications to human facial morphology. Hystrix 24, 59. doi: 10.4404/hystrix-24.1-6369
Mutanen M., Pretorius E. (2007). Subjective visual evaluation vs. traditional and geometric morphometrics in species delimitation: A comparison of moth genitalia. Systematic Entomol. 32, 371–386. doi: 10.1111/j.1365-3113.2006.00372.x
Oug E., Christiansen M. E., Dobbe K., Rønning A. H., Bakken T., Kongsrud J. A. (2015). Mapping of marine benthic invertebrates in the oslofjord and the skagerrak: sampling data of museum collections from 1950–1955 and from recent investigations. doi: 10.5324/fn.v35i0.1944
Raupach M. J., Mayer C., Malyutina M., Wägele J. W. (2009). Multiple origins of deep-sea asellota (Crustacea: Isopoda) from shallow waters revealed by molecular data. Proc. R. Soc. B: Biol. Sci. 276 (1658), 799–808.
Riehl T. (2014). A phylogenetic approach to the classification of macrostylid isopods and faunal linkages between the deep sea and shallow-water environments (Doctoral dissertation, Hamburg, Germany, Universität Hamburg). doi: 10.13140/RG.2.1.3989.6084
Riehl T., Brandt A. (2010). Descriptions of two new species in the genus Macrostylis Sars 1864 (Isopoda, Asellota, Macrostylidae) from the Weddell Sea (Southern Ocean), with a synonymisation of the genus Desmostylis Brandt 1992 with Macrostylis. ZooKeys 57), 9–49. doi: 10.3897/zookeys.57.310
Riehl T., Brandt A. (2013). Southern ocean macrostylidae reviewed with a key to the species and new descriptions from maud rise. Zootaxa 3692 (1), 160–203.
Riehl T., Wilson G., Hessler R. (2012). New Macrostylidae Hansen 1916 (Crustacea: Isopoda) from the Gay Head-Bermuda transect with special consideration of sexual dimorphism. Zootaxa 3277, 1–26. doi: 10.11646/zootaxa.3277.1.1
Roggero A., Giachino P. M., Palestrini C. (2013). A new cryptic ground beetle species from the Alps characterised via geometric morphometrics. Contributions to Zool. 82, 171–183. doi: 10.1163/18759866-08204002
Rufino M. M., Abelló P., Yule A. B. (2006). Geographic and gender shape differences in the carapace of liocarcinus depurator (Brachyura: Portunidae) using geometric morphometrics and the influence of a digitizing method. J. Zoology 269 (4), 458–465.
Santamaria C. A., Mateos M., Taiti S., DeWitt T. J., Hurtado L. A. (2013). A complex evolutionary history in a remote archipelago: phylogeography and morphometrics of the Hawaiian endemic ligia isopods. PloS One 8, e85199. doi: 10.1371/journal.pone.0085199
Sars G. O. (1864). Om en anomal Gruppe af Isopoder. Forhandlinger i Videnskaps-selskabet i Christiania 1863, 205–221.
Sars G. O. (1899). An account of the crustacea of norway: with short descriptions and figures of all the species: Isopoda. Ed. Cammermeyer A. (Bergen: Bergen Museum).
Siriwut W., Edgecombe G.D., Sutcharit C., Panha S. (2015). The centipede genus scolopendra in Mainland Southeast Asia: molecular phylogenetics, geometric morphometrics and external morphology as tools for species delimitation. PloS One 10, e0135355. doi: 10.1371/journal.pone.0135355
Steingrímsson S. A., Gudmundsson G., Helgason G. V. (2020). The BIOICE station and sample list: revised compilation, March 2020. Zenodo. doi: 10.5281/zenodo.3728257
Vey A., Brix S. (2009). Macrostylis cerritus sp. nov., a new species of Macrostylidae (Isopoda: Asellota) from the Weddell Sea, Southern Ocean. Zootaxa 2096. doi: 10.11646/zootaxa.2096.1.21
Keywords: BIOICE, geometric morphometrics, integrative taxonomy, macrostylid, Iceland, IceAGE
Citation: Casaubon A and Riehl T (2024) Shape matters: investigating the utility of geometric morphometric techniques in the deep-sea isopod family Macrostylidae (Isopoda: Asellota). Front. Mar. Sci. 11:1380594. doi: 10.3389/fmars.2024.1380594
Received: 01 February 2024; Accepted: 19 September 2024;
Published: 30 October 2024.
Edited by:
Rachael Peart, National Institute of Water and Atmospheric Research (NIWA), New ZealandReviewed by:
Jörundur Svavarsson, University of Iceland, IcelandAmy Driskell, Smithsonian National Museum of Natural History (SI), United States
Copyright © 2024 Casaubon and Riehl. This is an open-access article distributed under the terms of the Creative Commons Attribution License (CC BY). The use, distribution or reproduction in other forums is permitted, provided the original author(s) and the copyright owner(s) are credited and that the original publication in this journal is cited, in accordance with accepted academic practice. No use, distribution or reproduction is permitted which does not comply with these terms.
*Correspondence: Anchita Casaubon, YW5jaGl0YS5jYXNhdWJvbkBzZW5ja2VuYmVyZy5kZQ==