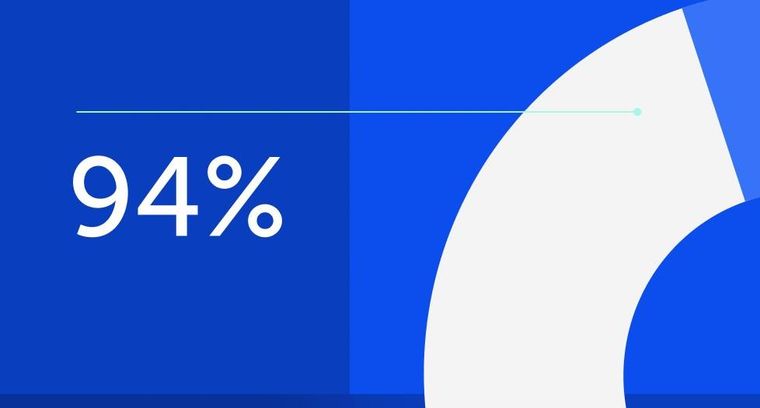
94% of researchers rate our articles as excellent or good
Learn more about the work of our research integrity team to safeguard the quality of each article we publish.
Find out more
ORIGINAL RESEARCH article
Front. Mar. Sci., 26 July 2024
Sec. Marine Fisheries, Aquaculture and Living Resources
Volume 11 - 2024 | https://doi.org/10.3389/fmars.2024.1379571
This article is part of the Research TopicBlue Foods Security and SustainabilityView all 15 articles
Introduction: Calcein was used to develop a shell marking method for Potamocorbula laevis.
Methods: The suitable conditions for marking were investigated, including marking concentration, immersion time, and water temperature. The impacts and feasibility of the marking method were assessed based on the survival rate of P. laevis, the success rate of fluorescence marking, marking quality, and alterations in activities of antioxidant enzymes in the digestive gland of the experimental bivalves. Two concentrations of calcein (20 and 50 mg/L) were used and the immersion time included 1 and 2 h, respectively. The experiment was performed in two rounds, with water temperatures of 12.84 ± 0.09 and 24.18 ± 0.04 °C, respectively.
Results and discussion: The results indicated that calcein did not significantly impact the survival of P. laevis after 7 d of recovery. The catalase activity and malondialdehyde content in low temperature-marked P. laevis showed significant decreases, and the relative abundances of certain fatty acids also exhibited significant changes within 2 h post exposure to 20 mg/L of calcein. However, these indicators returned to normal levels within 7 d. The marking impact of calcein was proportional to the calcein concentration and immersion time. Higher temperature generated a negative impact on the marking effect of 20 mg/L of calcein, while no obvious impacts were observed for 50 mg/L of calcein. The marking success rates and the recapture rates of P. laevis for in situ tests in the two experimental groups were both 100% and 4.44 ± 1.29% after one month. Also, the recapture marking rates and the marking good rates of the recaptured individuals were both 100%. There were no significant differences between these parameters for 50 and 75 mg/L of calcein. Given the cost and safety of labeling, a strategy in terms of an immersion in 50 mg/L of calcein for 2 h could be considered as an effective in situ labeling scheme for P. laevis. In conclusion, calcein can be employed as a marking method for P. laevis. These findings could be potentially beneficial for development of in situ labeling technology, proliferation as well as release of shellfish in tidal flats and resource conservation.
P. laevis belongs to the Bivalvia class, Myida order, and Corbulidae family. It is a eurythermal benthic bivalve commonly inhabiting the intertidal zone or shallow waters of the subtidal zone. It is a primary benthic species throughout many estuarine areas in East Asia (Sun et al., 2013; Chen et al., 2017). It is small and has an elongated oval or nearly triangular shape. The shell is hard, with the left valve being smaller than the enlarged right valve. The shell surface is smooth and lacks ribs, and the adult shell length is approximately 1.5 ~ 2.5 cm. P. laevis is rich in proteins, low in fat, and abundant in minerals. The meat of this bivalve is consumed by humans and can also serve as high-quality bait for shrimp, crabs, or fish (Liu and She, 2003; Liu, 2022). Recently, the natural resources of P. laevis have significantly declined due to overharvesting and environmental pollution. The ecological value (Zhou et al., 2017), nutritional value (Liu, 2022), reproductive habits (Liu and She, 2003), and responses to environmental factors (Li et al., 2015; Ning et al., 2016; Teng et al., 2018) of this bivalve have garnered significant attention. However, there is currently limited research regarding the population dynamics and dispersal patterns of this bivalve in its natural habitat.
Marking technology is a widespread method in shellfish proliferation and release. It can reflect the growth and population variability of shellfish based on changes in external traits, recapture position, quantity, and time before and after biomarker observation. Among these markers, chemical fluorescence labeling is predominantly employed for shellfish owing to its high labeling efficiency, high success rate, and high retention rate (Sugiura and Kikuya, 2017; Gancel et al., 2019; Mahé et al., 2021). The principle of chemical labeling relies on the complexation of fluorescent dyes with biological tissues to generate fluorescent markers visible under a specific wavelength light source.
Calcein is a calcium ion fluorescent indicator with an excitation wavelength of 495 nm and an emission wavelength of 515 nm (Xia and Li, 2015). Although direct contact with calcein may cause skin, respiratory, and eye irritation to humans, calcein typically binds to cells or biomolecules through internal or surface staining methods when labeling biological samples, and it has been shown to generate no significant toxicity to humans. Currently, there have not been detailed reports on the bioaccumulation and biodegradation of calcein in natural environments. The half-life of calcein in aquatic environments has been found to vary depending on environmental conditions, ranging from hours to days (Keller et al., 2004). The U.S. Federal Drug Administration approved a new veterinary drug research application (INADA) for calcein in 2011, allowing calcein to be used in edible animal feeding activities. The hypotoxic calcein has been used as a release marker for Crassostrea virginica (Spires et al., 2022), Perna Canacillus (Fitzpatrick et al., 2013), Argopecten irradians (Moran and Marko, 2005) and other aquatic organisms, such as fish, echinoderms (Zhao et al., 2011), etc (Supplementary Table S1). The available concentration range of calcein in bivalve mollusks generally varies between 25 - 600 mg/L, e.g., 25 - 250 mg/L for Ostreidae, 40 - 200 mg/L for Pteriidae, 50 - 600 mg/L for Pectinidae, and 200 - 300 mg/L for Arcidae, etc (Supplementary Table S2). However, it is worth noting that, the safety of calcein remains to be relative, and if the labeling concentration or exposure time of calcein is much higher or longer, certain adverse impacts appear to occur on clams or other labeling organisms. The growth of shellfish seems to be limited and the resulting death might even occur. Hence, the application of appropriate calcein labeling on P. laevis or other clams is potentially prospecting in large-scale stocking, and the food safety risks are likely within deemed safety range, to certain extent.
Organisms are able to clear large amounts of reactive oxygen species produced by exogenous factors through their antioxidant system, reducing oxidative damage. Antioxidant enzymes are an important component of this system, and their activity can reflect the extent of damage to the organism (Duan et al., 2016). Fatty acids are able to participate in various biochemical pathways such as energy supply, biological membrane formation, and signaling molecules, and are pivotal for maintaining normal body function (Lee et al., 2018). In addition, the fatty acids are very sensitive to pollutants and environmental changes, and often used as a bioindicator in response to external stressors in marine bivalves (Gonçalves et al., 2016). Thus, the fatty acids deserved to be focused on to evaluate the labeling effects of calcein on the clams in this work.
Geligang is a sandbank between the Liaohe River and the Shuangtaizi River in the northern part of the Liaodong Bay, China. The area is about 10,000 hm2, with an average altitude of –2 m. Its water quality is characterized by high turbidity, low transparency, and serious eutrophication. Approximately 80% of the beach surface is dry for about 3 h per day on average. The beach surface is flat, the surface water flow is turbulent, and there is a long freezing period in winter. It is rich in Mactra veneriformis, P. laevis, Solen grandis and other economic shellfish. In this study, the clam P. laevis from Geligang was focused on to investigate the appropriate marking conditions of the fluorescent dye calcein for both laboratory and in situ labeling. The antioxidant capacities as well as the fatty acid profiles served as biochemical indicators to explore the feasibility of chemical labeling and the optimal conditions of calcein on P. laevis. These results could be potentially beneficial for recovery and utilization of the clam P. laevis.
P. laevis was collected in April and July 2023, respectively, at Geligang, Liaodong Bay, China. The collected samples were transported back to the laboratory by express cold chain and temporarily maintained in a polyvinyl chloride tank with fine sand until the survival rate remained stable, and half of seawater was exchanged every day. Three commercial microalgal powders for two green algae, i.e., Dunaliella salina and Chlorella vulgaris, and one blue-green alga, i.e., Spirulina sp., were mixed in a mass ratio of 2:3:5, and the feeding proportion of algae was equivalent to 1% of the soft tissue mass of the bivalves.
The stock solution of calcein was prepared using distilled water, and stored in a light-protected container. To avoid discrepancies between experimental and theoretical concentrations, the calcein stock solutions used in the experiments were prepared within 24 h before use to prevent precipitation due to prolonged storage. During preparation, a small amount of NaHCO3 was added to adjust the pH, and stirring continued until calcein completely dissolved. Therefore, the actual concentrations used in the experiments remained relatively consistent with the theoretical concentrations. The stock concentration was set at 1 g/L. The stock solution was diluted into 20 and 50 mg/L using seawater, respectively.
The ambient seawater temperature was maintained at 12.84 ± 0.09°C. The experimental tests (Figure 1) included Group A (control, 0 mg/L), Group B (low concentration, 20 mg/L, 1 h), Group C (low concentration, 20 mg/L, 2 h), and Group D (high concentration, 50 mg/L, 2 h). Each group had 3 replicates with 30 P. laevis per replicate. The shell length, shell width, and wet weight of the tested clams were 22.45 ± 1.04 mm, 14.76 ± 0.75 mm, and 1.87 ± 0.27 g, respectively. The labeling process is carried out in an opaque foam box. The individuals were immersed into 10 L of seawater with 7.06 ± 0.08 mg/L of dissolved oxygen and 33.14 ± 0.06 of salinity during the marking process. The stocking density was 9 ind./L, and the clams were fasting throughout the marking process. Following the immersion, the residual calcein on the surface of the bivalve’s shell was gently rinsed using fresh seawater. Then the clams were transferred to a 0.16 m3 transparent glass tank covered with approximately 5 cm thick of sea sand that was filtered through 30~50 mesh for 7 d.
Nearly 70% of the cultured seawater was exchanged at regular intervals every day during acclimation. The clams were fed with algal powder in a mixed mass ratio of D. salina: C. vulgaris = 1:1, and the feeding proportion was 1% of the soft tissue mass of the clams. The seawater was aerated for 24 h prior to labeling. The temperature, dissolved oxygen, salinity and pH value of seawater were 12.80 ± 0.03°C, 7.54 ± 0.07 mg/L, 32.95 ± 0.03, and 7.96 ± 0.10 during the recovery culture, respectively.
The ambient seawater temperature during labeling was 24.18 ± 0.04°C. The experimental tests concluded one more Group E (high concentration, 50 mg/L, 1 h) in relative to Experiment 1. The shell length, shell width, and wet weight of P. laevis were 21.16 ± 1.55 mm, 13.83 ± 1.08 mm, and 1.45 ± 0.39 g, respectively. The dissolved oxygen and salinity were 6.26 ± 0.16 mg/L and 30.87 ± 0.55, and the stocking density was also 9 ind./L during labeling. The temperature, dissolved oxygen, salinity and pH value of seawater were 24.32 ± 0.24°C, 6.14 ± 0.16 mg/L, 31.40 ± 0.41, and 8.10 ± 0.05 during the recovery culture, respectively.
The in situ labeling experiment took place at Geligang in the Liaodong Bay, China. The calcein concentrations included 0, 50 and 75 mg/L and the immersion time was 2 h. Three parallel sampling sites were set for each calcein concentration. There were thirty individuals of P. laevis at each sampling site, and the native specimens were collected from Geligang, with a shell length of 24.42 ± 1.09 mm. Water temperature was 25.76 ± 0.08°C. The labeling process was performed within sealed light-free containers to avoid quenching of calcerin and the seawater volume was 20 L.
Seven sites with a distance of about 50 m were selected in Geligang tidal flat (40°N, 121°E). During the ebb tide, the in situ device was installed (Figures 2A, B), and the labeled P. laevis were transferred to the in situ device. After a month, the labeled individuals at each site were recaptured, and the recapture rate, the labeling rate of recaptured individuals, and the good labeling rate were then evaluated.
Figure 2 In situ labeled experimental model diagram and field device. (A) In situ device design diagram; (B) In situ device image.
After 7 d of marking, all the remaining smooth-shell clams were dissected, and the soft segments were removed. The shells were cleaned and dried at 50°C. Fluorescent markings on the shells were observed using a fluorescence flashlight (Luyor-3280LB) at 480 ~ 490 nm. The quality of fluorescent marking was assessed using Grade 0 ~ 5 (Table 1). Grade 0, 1, 2 and 3 indicated that the marking was not visible, blurry, clearly marking, and brightly marking under the fluorescence flashlight, respectively. Grade 4 and 5 indicated that the marking was visible, and clearly marking under the transmitted light, respectively (Lü et al., 2014). Markings with a quality of no less than Grade 2 were considered acceptable. The success rate of marking and the rate of good markings (Grade 2 or higher) were separately calculated for each experimental group.
Samples were obtained after 2 h following labeling and 7 d following temporary culture. Five individuals of P. laevis were randomly selected from each replicate. The digestive glands and soft tissues were dissected on ice and stored at –80°C. The digestive glands were used to determine the antioxidant enzymes activities and MDA content. The soft tissues were lyophilized and grounded into powder filtered through an 80-mesh sieve. The fine powder was then used to perform fatty acid analysis.
The antioxidant indexes in labeled P. laevis were determined by superoxide dismutase (SOD) assay kit, CAT assay kit, and glutathione peroxidase (GPX) assay kit from Nanjing Jiancheng Biological Co., Ltd. (Nanjing, China), and the MDA and total protein amounts were assessed using assay kits from Servicebio (Nanjing, China), respectively.
The fatty acids in labeled P. laevis was analyzed using the modified one-step acid-catalyzed esterification method (Liu et al., 2015). The accurately weighed powder (50 ~ 100 mg) were added to a 10-mL round-bottom flask followed by addition of 5 mL 2% H2SO4 in methanol. After complete ultra-sonication, the mixture was heated at 70°C for 1 h. The hexane-extracted fatty acid methyl esters (FAMEs) were quantified using a Bruker GC 456 equipped with FID and a DB-23 column (30 m length × 320 µm inner diameter × 0.25 µm thickness, Agilent Technologies, USA). C19:0-ME were used as internal standards for quantification of FAMEs. The injection volume was 1 µL and the carrier gas was nitrogen with purity of 99%. The split ratio was 30:1. Column temperature was programmed from 130 to 170°C increasing at 10°C/min, then to 210°C at 2.5°C/min and held at 210°C for 3 min. The running time was 23 min. The temperatures of the front inlet and front detector were both 270°C. The formula for calculating the relative (Pi) and absolute (Mi) content of each fatty acid were as follows.
Where Ai and ∑Ai in Equations 1, 2 indicated the peak area of the individual fatty acid and that of the total fatty acids, respectively. As, Ms and Ma in Equation 2 indicated the peak area of the internal standard, the addition mass of internal standard, and the amount of the added sample.
The data were statistically analyzed by SPSS 26.0 and denoted as average ± standard deviation (SD, n = 3). Statistical significance of the difference was determined by one-way analysis of variance Tukey’s honestly significant difference (HSD) test with the significance level of 0.05.
There were no significant differences in the survival rates of calcein-labeled P. laevis for both Experiment 1 and 2 after 7 d of recovery. The survival rates of individuals in Experiment 1 were 86 ~ 90%, and that in Experiment 2 were 83 ~ 86% (Figures 3A, B). The labeling scheme used in this study had no significantly negative impact on the short-term survival of P. laevis.
Figure 3 Survival rates of P. laevis during the marking period. (A) Experiment 1. (B) Experiment 2. Values are means of three biological replicates ± SD (n = 3).
The activities of SOD and GPX presented no notable differences between distinct groups in low (Figures 4A, C) and high (Figures 5A, C) temperatures labeling, after 2 h or 7 d of labeling. In addition, a majority of CAT activities and MDA contents in distinct groups showed no significant differences among different treatments (Figures 4B, D, 5B, D). In particular, the CAT activities in Groups B and C (20 mg/L, low-concentration of calcein) of Experiment 1 (low temperature labeling) were found to prominently decline by 95% and 71% relative to the control Group A following 2 h of labeling, respectively. The MDA content in Group B following 2 h of labeling was notably lower by 28% than that in Group A of Experiment 1 (low temperature labeling, Figure 4).
Figure 4 The antioxidant capacity of P. laevis in Experiment 1. (A) Experiment 1, 2 h post exposure (2 hpe). (B) Experiment 1, 7 d post exposure (7 hpe). (C) Experiment 2, 2 h post exposure. (D) Experiment 2, 7 d post exposure. Values are means of three biological replicates ± SD (n = 3). The a and b indicated significant difference between the treatment groups (*P< 0.05). SOD, CAT, GPX, and MDA indicated superoxide dismutase, catalase, glutathione peroxidase, and malondialdehyde, respectively.
Figure 5 The antioxidant capacity of P. laevis in Experiment 2. (A) Experiment 1, 2 h post exposure (2 hpe). (B) Experiment 1, 7 d post exposure (7 hpe). (C) Experiment 2, 2 h post exposure. (D) Experiment 2, 7 d post exposure. Values are means of three biological replicates ± SD (n = 3). SOD, CAT, GPX, and MDA indicated superoxide dismutase, catalase, glutathione peroxidase, and malondialdehyde, respectively.
In P. laevis, 28 species of fatty acids were detected, including 6 saturated fatty acids (SFA), 7 monounsaturated fatty acids (MUFA), and 15 polyunsaturated fatty acids (PUFA, Supplementary Tables S3, S4). The main fatty acids in P. laevis included C16:0, C20:5n3, and C22:6n3, while the minor fatty acids included C16:1n7 and C16:4n3 (Figures 6A–D). The relative abundances of the fatty acids with distinct saturation degrees were ranked as SFA< MUFA< PUFA, and the proportion of ω3 fatty acids was found to be much higher than that of ω6 fatty acids (Figures 7A–D). In both Experiment 1 and 2, there were almost no significant changes in the relative levels of the main fatty acids in calcein-labled P. laevis after 2 h and 7 d of labeling (Figures 6, 7). Additionally, all groups maintained relatively stable contents in the total fatty acid (Figures 8A, B). In conclusion, the labeling scheme used in this study does not have a significantly negative impact on the fatty acids of P. laevis.
Figure 6 The relative abundances of the major fatty acids in P. laevis. (A) Experiment 1, 2 h post exposure (2 hpe). (B) Experiment 1, 7 d post exposure (7 hpe). (C) Experiment 2, 2 h post exposure. (D) Experiment 2, 7 d post exposure. Values are means of three biological replicates ± SD (n = 3). The major fatty acids referred to C16:0, C20:5n3 and C22:6n3. The relative abundance of the individual fatty acid was more than 10% and the total relative abundances of the three fatty acids in P. laevis were more than 50%. The a and b indicated significant difference between the treatment groups (*P< 0.05).
Figure 7 The relative abundances of fatty acids with distinct saturation degrees in P. laevis. (A) Experiment 1, 2 h post exposure (2 hpe). (B) Experiment 1, 7 d post exposure (7 hpe). (C) Experiment 2, 2 h post exposure. (D) Experiment 2, 7 d post exposure. Values are means of three biological replicates ± SD (n = 3). The a and b indicated significant difference between the treatment groups (*P< 0.05). SFA, MUFA, PUFA indicated saturated, monounsaturated, and polyunsaturated fatty acids, respectively. ω3 referred to C16:4n3, C18:3n3, C18:4n3, C20:3n3, C20:4n3, C20:5n3, C22:5n3, and C22:6n3. ω6 referred to C16:2n6, C18:2n6, C20:2n6, C20:3n6, C20:4n6, and C22:4n6.
Figure 8 The total fatty acid contents of P. laevis under distinct labeling regimes. (A) Experiment 1. (B) Experiment 2. Values are means of three biological replicates ± SD (n = 3).
All the P. laevis individuals in Experiment 1 exhibited 100% marking success rates, except for Group B with a success rate of 93% (Table 2). Moreover, there were significant differences in the marking rate with an intensity level of 2 or higher among the groups. Group D had a significantly higher marking rate than Group C (*P< 0.05), while Group C had a significantly higher marking rate than Group B (*P< 0.05). In Experiment 2, the success rates of marking in all other groups exceeded 95% except for that in Group B. There were no significant differences in the marking rates between the low-concentration groups, but they were all significantly lower than the high-concentration groups. Among the high-concentration groups, Group C had a significantly higher labeling rate with a soaking time of 2 h, compared to Group E with a soaking time of 1 h. Under the same soaking scheme, the marking quality in Experiment 2 experienced varying degrees of decrease.
The marking success rates and the recapture rates of P. laevis for in situ tests in the two experimental groups were both 100% and 4.44 ± 1.29% after one month (Table 3; Supplementary Table S5). Also, the recapture marking rates and the marking good rates of the recaptured individuals were both 100%. There were no significant differences between these parameters for 50 and 75 mg/L of calcein (Table 3). Given the cost and safety of labeling, a strategy in terms of an immersion in 50 mg/L of calcein for 2 h could be considered as an effective in situ labeling scheme for P. laevis.
The mortality rate directly reflects the impact of fluorescent labeling on the organisms, indicating the feasibility of the proposed labeling scheme. When the dye concentration or labeling time is too high, it can result in death or growth inhibition on the labeled organisms. In Experiment 1, 4 h post exposure of labeling, acute deaths occurred in all experimental groups except for the control, but there were no significant differences between groups (Supplementary Table S6). In both rounds of experiments, there were no significant differences in the survival rates between the experimental and control groups following 7 d of labeling. This indicates that the calcein concentrations and immersion time employed in the experiment did not have a significantly negative impact on the short-term survival of P. laevis. In fact, several studies have demonstrated that calcein does not have a significant negative effect on the growth and survival of bivalves under suitable conditions (Zhou et al., 2017; Mahé et al., 2021; Spires and North, 2022), and it may even enhance the growth and survival of certain shellfish (Moran and Marko, 2005; Spires and North, 2022; Spires et al., 2022).
When exposed to stress, organisms generate a large amount of reactive oxygen species (ROS) in the body. Once produced in excess, ROS can result in lipid peroxidation in tissues and be harmful to the organism. The antioxidant system is important for defense against the detrimental effects of ROS, including SOD, CAT, and GPX. These antioxidants can effectively respond to exogenous substances impacting the body (Cao et al., 2012; Duan et al., 2016). ROS primarily include superoxide anion (O2-), hydroxyl radical (OH•), and non-radical hydrogen peroxide (H2O2) (Schieber and Chandel, 2014). SOD can convert superoxide anions (O2-) into H2O2 and O2 through dismutation, and the generated H2O2 is then converted into non-toxic H2O and O2 by CAT (Arockiaraj et al., 2012). GPX has a similar function to CAT, as it is able to eliminate H2O2 and lipid hydroperoxides in the body, inhibit lipid peroxidation, and prevent oxidative damage to cell membranes and tissues (Hiramatsu et al., 1992). MDA, in contrast, is a product generated from lipid peroxidation caused by free radicals induced by oxidative stress in the body. It can react with cellular proteins, DNA, and other components, resulting in cell damage. The MDA content in tissues can indirectly reflect the extent of cellular damage (Zhang et al., 2016).
In Experiment 1, the CAT activity across all experimental groups decreased within 2 h of labeling. As the concentration of calcein or immersion time decreased, CAT activity gradually decreased (Figure 4B). The reduction in CAT activity may be attributed to two factors. Firstly, the organism’s stress response could lead to the generation of a large amount of H2O2, consuming CAT. Secondly, immersion in calcein may reduce or inhibit the defense capacity of the organism’s antioxidant system, preventing the timely replenishment of CAT following consumption. Moreover, CAT activity in the digestive gland of smooth river blue mussels decreased when immersed in 20 mg/L of calcein. However, as the concentration of calcein increased, its activity also increased. This may be explained by the “toxicant hormesis effect”, wherein CAT activity is inhibited at low concentrations and stimulated at high concentrations. This observation is consistent with the observation in Meretrix meretrix under Cu2+ stress (Tian et al., 2022). Similar phenomena have also been observed in Chlamys farreri (Wand et al., 2008) and Mytilus edulis (Zhang et al., 2004). In this study, SOD and GPX activities in the visceral mass of P. laevis were not significantly impacted by the concentration of calcein or the immersion time (Figures 4A, C). This suggested that the labeling conditions employed in the experiment resulted in limited stress on P. laevis and did not perturb the organism’s antioxidant balance, resulting in minimal impact that can be overcome within a short period of time. Similar findings have been reported in low-concentration tetracycline hydrochloride labeled Danio rerio and Oreochromis niloticus (Oruc and Uner, 2001). Following 2 h of labeling, the MDA content in Group A significantly decreased compared to the control group (Figure 4D). This may be due to the production of ROS as a stress response, leading to the consumption of a large amount of CAT to eliminate ROS and limit the peroxidation level in the organism, causing a decrease in MDA content.
In contrast to Experiment 1, there were no significant changes in the activities of SOD, CAT, GPX, and MDA levels in all experimental groups in Experiment 2 after marking. The reason for this phenomenon may be related to the water temperature. P. laevis belongs to eurythermal and euryhaline bivalves, capable of surviving at temperatures as low as -4°C, but growth is nearly halted below 10°C and accelerated above 20°C (Sun, 1988). The water temperature in Experiment 2 was around 24°C, within the optimal range for P. laevis. The appropriate water temperature may have enhanced the ability of P. laevis to resist external stress, while the water temperature in Experiment 1 was around 13°C, which could have compromised the stress resistance of P. laevis due to lower temperatures.
The fatty acids in P. laevis were also influenced by the experimental water temperature, showing a similar pattern of changes to antioxidant capacity. In Experiment 1, significant differences in some fatty acids between Groups A and B were observed initially after marking, but they returned to normal levels after 7 d. Moreover, the magnitude of change in Experiment 1 was much greater than that in Experiment 2. However, it is worth noting that both Experiment 1 and 2 showed cases where there were no significant differences in fatty acids among the experimental groups initially after marking, but significant changes occurred after 7 days. This suggested that the fatty acids of P. laevis could respond to the stress caused by calcein, but this response exhibited delayed manifestation and required some time to become evident. Overall, the composition and content of fatty acids in P. laevis were indeed unaffected by fluorescent labeling. It was implicated that the labeling scheme used in this study appeared to cause no prominent stress on P. laevis, hinting on the safety of this labeling scheme, to certain extent. As the fatty acids in shellfish can change in response to environmental stress, the potential fatty acids biomarkers might exist in clams in adaption to fluorescent labeling. If the concentration or immersion time of calcein is further increased, it is expected to disclose the related fatty acids biomarkers responsive to fluorescent labeling in P. laevis, which deserves further exploration in our future work.
The concentration of dye and the labeling time significantly impacted the fluorescence labeling effect. The success rate and quality of labeling were augmented with increased concentration and incubation time. It was found that the concentration of calcein was the main factor affecting the labeling effect on P. laevis, and the soaking time took second place based on the batch labeling tests. When the calcein concentration reached ≥ 50 mg/L, the clams could be largely labeled with high quality in a short time. Moreover, if the soaking time of calcein extended, the labeling quality of P. laevis could also be further improved. When the labeling concentration was 50 mg/L or higher, the success rate of labeling reliably reached 100%. Under identical conditions, the high-concentration group consistently exhibited a significant advantage in labeling success rate compared to the low-concentration group. The experimental group immersed for 2 h had a higher labeling success rate than those immersed for 1 h (Table 2). The previous studies revealed that the labeling quality of calcein on Haliotis rubra (Chick, 2010), Perna canaliculus (Fitzpatrick et al., 2013) and Anadara broughtonii (Zhou et al., 2017) got enhanced with the increase of concentration and immersion time (Supplementary Table S7). The case for P. laevis in this work was also in line with these previous studies. In addition, a supplementary experiment at an ambient water temperature of 13.40°C (Experiment 1: 12.84 ± 0.09°C) was also conducted (Supplementary Methods). The results showed that the labeling success rates for 50 mg/L of calcein for 1 h and 2 h were both 100%, and the labeling good rate of 50 mg/L + 1 h was 43.33 ± 3.34%, which was significantly lower than that of 50 mg/L + 2 h (67.78 ± 5.09%) (Supplementary Table S8). As we speculated, the low temperature did not improve the labeling quality of 50 mg/L + 1 h. Following successful labeling, calcein will generate a blue-green fluorescent mark visible under blue light (480 ~ 490 nm) on the surface of the shell as well as the growth edge of the shell (Figures 9A–D). Differently from calcein-labeled Mactra veneriformis, the fluorescent markers on M. veneriformis were mainly concentrated on the outer surface of the shell (unpublished data), and the markers at the growth edge were more discrete, while in P. laevis, the clear fluorescent markers were more at the growth edge (Figures 10A, B). This phenomenon may be related to the differences in shellfish body size, growth rate and shell microstructure.
Figure 9 Fluorescent labeling of calcein on P. laevis. (A) Shell profiles of P. laevis under natural light. (B) Inner shell view of P. laevis under natural light. (C) Shell view of P. laevis irradiated using a Luyor–3280LB fluorescent flashlight. The white frame highlighted the presence of calcein. (D) Inner shell view of P. laevis irradiated using a Luyor–3280LB fluorescent flashlight. The white frame highlighted the presence of calcein.
Figure 10 Labeling effect of calcein on P. laevis and M. veneriformis. (A) Shell view of P. laevis and M. veneriformis irradiated using a Luyor–3280LB fluorescent flashlight. (B) Inner shell view of P. laevis and M. veneriformis irradiated using a Luyor–3280LB fluorescent flashlight.
Temperature can impact calcein labeling, and higher temperatures have a negative impact on low-concentration calcein labeling. In Experiment 1, the labeling water temperature was 12.84 ± 0.09°C, while in Experiment 2, the labeling water temperature was 24.18 ± 0.04°C. When the labeling concentration was 20 mg/L, the success rate of labeling in Experiment 2 was reduced, and the high-quality labeling rate was significantly lower than in Experiment 1 (*P< 0.05) over the same period. However, the effect of temperature increase on the high-concentration group was not significant (Table 2).
To our knowledge, the labeling method, especially chemical labeling, is characteristic in terms of species specificity, developmental stages and living habits of the labeled organism. The form, concentration, labeling time and labeling species of chemical dyes greatly affect the effect of chemical labeling. P. laevis is not only one of the main shellfish in the Geligang of Liaodong Bay, but also one of the main benthic species in many estuarine waters of East Asia. The edible clam can also be used as a high-quality feed for shrimp, crab or fish. In recent years, its natural resources have greatly declined due to large-scale harvesting and environmental pollution. However, the chemical labeling approach for the clam P. laevis has not been elucidated. In addition, fatty acids serve as the crucial components of lipids, and are vital for formation and permeability of cell membranes, resulting in their great sensitivity to multiple stressors and environmental alterations (Gonçalves et al., 2016). This trait has made fatty acids become the protruding biomarkers to indicate altered environmental changes. It is appropriate and innovative to take fatty acids as the evaluation factor in the labeling method of P. laevis. On the other hand, the in situ labeling tests combined with the laboratory experiments are applicable for resource stocking, conservation and assessment of the economic clams, to certain extent. In this study, the main complexation site of calcein was the calcified shell structure of P. laevis. This part is generally not ingested by predators, and it can further reduce the degree of calcein enrichment in organisms. Moreover, calcein is easily quenched under light. These factors suggested that calcein might be a relatively safe chemical dye with insignificant environmental impacts. Overall, the innovation of the methodology in our work lies in the establishment of chemical labeling method for the unreported clams P. laevis at distinct temperatures, the innovative evaluation of the sensitive biomarker fatty acids of clams, as well as the retests of in situ labeling experiments for P. laevis.
The calcein used in this study had a significant marking effect on P. laevis, and it did not cause any noticeably negative impacts. The most stable and bright marking effect was achieved by soaking the clams in 50 mg/L of calcein for 2 h. Although high temperature (24.18 ± 0.04°C) resulted in reduced marking effectiveness at 20 mg/L of calcein, the significantly marking effects were still observed when the soaking time was 2 h under low-temperature conditions (12.84 ± 0.09°C). Although this may induce certain oxidative stress on the clams, the stress extent was returned to normal levels within 7 d. Considering the marking cost, both options have practical value: (1) Water temperature at 13°C, soaking in 20 ~ 50 mg/L of calcein for 2 h; (2) Water temperature at 24°C, soaking in 50 mg/L of calcein for 2 h.
The data that support the findings of this study are available from the corresponding author upon reasonable request. Requests to access these datasets should be directed to Q-ZW, d3F6bG1AMTI2LmNvbQ==.
The manuscript presents research on animals that do not require ethical approval for their study.
JG: Writing – review & editing, Writing – original draft, Investigation, Data curation. XX: Writing – review & editing, Methodology, Data curation, Conceptualization. X-FL: Writing – original draft, Investigation. Y-AB: Writing – original draft, Resources. MY: Writing – review & editing, Data curation. W-MT: Writing – original draft, Investigation. H-JL: Writing – original draft, Investigation. Q-ZW: Writing – review & editing, Supervision, Resources, Funding acquisition, Conceptualization.
The author(s) declare financial support was received for the research, authorship, and/or publication of this article. This work was supported by Ocean Economic Development Grants of Liaoning Province (202222), National Key Research and Development Program of China (2022YFD2400305), China Scholarship Council, Science and Technology Foundation of Dalian (2021YF16SN015, 2021JB11SN035), a grant from Dalian Key Laboratory of Genetic Resources for Marine Shellfish, Foundation of Liaoning Academy of Agricultural Sciences (2022DD268339), Ocean and Fisheries Research Grants of Liaoning Province (201826, 201818) and Modern Agro-industry Technology Research System of China (CARS-49).
Author Y-AB was employed by the company Panjin Guanghe Crab Industry Co., LTD. Author H-JL was employed by the company Dalian Changhai-Yide Aquatic Products Co., LTD.
The remaining authors declare that the research was conducted in the absence of any commercial or financial relationships that could be construed as a potential conflict of interest.
All claims expressed in this article are solely those of the authors and do not necessarily represent those of their affiliated organizations, or those of the publisher, the editors and the reviewers. Any product that may be evaluated in this article, or claim that may be made by its manufacturer, is not guaranteed or endorsed by the publisher.
The Supplementary Material for this article can be found online at: https://www.frontiersin.org/articles/10.3389/fmars.2024.1379571/full#supplementary-material
Arockiaraj J., Easwvaran S., Vanaraja P., Singh A., Othman R. Y., Bhassu S. (2012). Molecular cloning, characterization and gene expression of an antioxidant enzyme catalase (MrCat) from Macrobrachium rosenbergii. Fish Shellf. Immunol. 32, 670–682. doi: 10.1016/j.fsi.2015.12.018
Cao L., Huang W., Shan X., Ye Z., Dou S. (2012). Tissue-specific accumulation of cadmium and its effects on antioxidative responses in Japanese flounder juveniles. Environ. Toxicol. Pharmacol. 33, 16–25. doi: 10.1016/j.fsi.2015.12.018
Chen W. Q., Wang W. X., Tan Q. G. (2017). Revealing the complex effects of salinity on copper toxicity in an estuarine clam Potamocorbula laevis with a toxicokinetic-toxicodynamic model. Environ. pollut. 222, 323–330. doi: 10.1016/j.envpol.2016.12.033
Chick R. C. (2010). Batch-tagging blacklip abalone (Haliotis rubra) for identification of hatchery-reared individuals on natural coastal reefs in New South Wales, Australia. J. Shellf. Res. 29, 209–215. doi: 10.2983/035.029.0117
Duan Y., Zhang J., Dong H., Wang Y., Liu Q., Li H. (2016). Effect of desiccation and resubmersion on the oxidative stress response of the kuruma shrimp Marsupenaeus japonicus. Fish Shellf. Immunol. 49, 91–99. doi: 10.1016/j.fsi.2015.12.018
Fitzpatrick M. P., Jeffs A. G., Dunphy B. J. (2013). Efficacy of calcein as a chemical marker of green-lipped mussel (Perna canaliculus) larvae and its potential use for tracking larval dispersal. Aquacult. Res. 44, 345–353. doi: 10.1111/are.2013.44.issue-3
Gancel H. N., Carmichael R. H., Park K., Krause J. W., Rikard S. (2019). Field mark-recapture of calcein-stained larval oysters (Crassostrea virginica) in a freshwater-dominated estuary. Estuaries Coasts. 42, 1558–1569. doi: 10.1007/s12237-019-00582-6
Gonçalves A. M. M., Mesquita A. F., Verdelhos T., Coutinho J. A. P., Marques J. C., Gonçalves F. (2016). Fatty acids’ profiles as indicators of stress induced by of a common herbicide on two marine bivalves species: Cerastoderma edule (Linnaeus 1758) and Scrobicularia plana (da Costa 1778). Ecol. Indic. 63, 209–218. doi: 10.1016/j.ecolind.2016.03.044
Hiramatsu M., Edamatsu R., Mori A. (1992). Free radicals, lipid peroxidation, SOD activity, neurotransmitters and choline acetyltransferase activity in the aged rat brain. EXS 62, 213–218. doi: 10.1007/978-3-0348-7460-1_21
Keller A., Mohamed A., Dröse S., Brandt U., Fleming I., Brandes R. (2004). Analysis of dichlorodihydrofluorescein and dihydrocalcein as probes for the detection of intracellular reactive oxygen species. Free Radical Res. 38, 1257–1267. doi: 10.1080/10715760400022145
Lee M. C., Park J. C., Lee J. S. (2018). Effects of environmental stressors on lipid metabolism in aquatic invertebrates. Aquat. Toxicol. 200, 83–92. doi: 10.1016/j.aquatox.2018.04.016
Li R., Ding J., Shen Y., Chang Y., Li Z. (2015). Analysis and fitted of the experimental data about the enzyme activity response of the clam Potamocorbula laevis to water temperature change above freezing. Mar. Environ. Sci. 34, 706–712. doi: 10.13634/j.cnki.mes.2015.05.011
Liu J. (2022). Analysis and evaluation of nutrient composition of Potamocorbula laevis North. Chin. Fish. 41, 6–11. doi: 10.3969/j.issn.1674-2419.2022.01.002
Liu J., Liu Y., Wang H., Xue S. (2015). Direct transesterification of fresh microalgal cells. Bioresource Technology. Bioresour. Technol. 176, 284–287. doi: 10.1016/j.biortech.2014.10.094
Liu J., She J. (2003). Reprodutctive behaviour of potamocorbula laevis (Hinds). Fish. Sci. 22, 12–13. doi: 10.16378/j.cnki.1003-1111.2003.05.003
Lü H., Zhang X., Xi D., Gao T. (2014). Use of calcein and alizarin red S for immersion marking of black rockfish Sebastes schlegelii juveniles. Chin. J. Oceanol. Limnol. 32, 88–98. doi: 10.1007/s00343-014-3022-9
Mahé K., Bellamy E., D’Amico F., Caill-Milly N. (2021). In situ fast marking study of manila clams (Ruditapes philippinarum). Int. J. Fish. Aquat. Stud. 9, 47–51. doi: 10.22271/fish.2021.v9.i1a.2387
Moran A. L., Marko P. B. (2005). A simple technique for physical marking of larvae of marine bivalves. J. Shellf. Res. 24, 567–571. doi: 10.2983/0730-8000(2005)24[567:ASTFPM]2.0.CO;2
Ning J., Chang Y., Liu W., Song J., Shi M. (2016). Stress responses of clam Potamocorbula laevis to sharp decrease in temperature. Fish. Sci. 35, 117–122. doi: 10.16378/j.cnki.1003-1111.2016.02.004
Oruc E. O., Uner N. (2001). Combined effects of 2, 4-D and azinphosmethyl on antioxidant enzymes and lipid peroxidation in liver of Oreochromis niloticus. Comp. Biochem. Phys. C. 127, 291–296. doi: 10.1016/S0742-8413(00)00159-6
Schieber M., Chandel N. S. (2014). ROS function in redox signaling and oxidative stress. Curr. Biol. 24, R453–R462. doi: 10.1016/j.cub.2014.03.034
Spires J. E., Dungan C. F., North E. W. (2022). Marking the shells of pediveliger eastern oysters Crassostrea virginica, with a calcein fluorochrome dye. J. Shellf. Res. 40, 479–487. doi: 10.2983/035.040.0304
Spires J. E., North E. W. (2022). Marking the shells of juvenile and adult eastern oysters, Crassostrea virginica, with the fluorochrome dye calcein and measuring growth and mortality after marking. J. Molluscan Stud. 88, eyac00. doi: 10.1093/mollus/eyac004
Sugiura D., Kikuya N. (2017). Validation of the age estimation method using the shell section of the manila clam Ruditapes philippinarum in Mutsu Bay, northern Japan. Aquacult. Sci. 65, 193–202. doi: 10.11233/aquaculturesci.65.193
Sun J. T. (1988). Preliminary study on the population dynamics of the clam Potamocorbula laevis (Hinds). Trans. Oceanol. Limnol. 10, 62–68. doi: 10.13984/j.cnki.cn37-1141.1988.04010
Sun C., Liu Z. H., Yang A. G., Zhou L. Q., Wu B., Yang J. K., et al. (2013). Genetic diversity of mitochondrial COI gene in three wild populations of Potamocorbula laevis. Hunan Agric. Sci. 07, 4–7. doi: 10.16498/j.cnki.hnnykx.2013.07.037
Teng W. M., Gao S. L., Liu X., Yan H. W., Xie X., Li X. D., et al. (2018). Effects of salinity on filtration and ingestion rates of surf clam Mactra veneriformis and clam Potamocorbula laevis in Liaodong Bay. Fish. Sci. 37, 622–627. doi: 10.16378/j.cnki.1003-1111.2018.05.007
Tian Z., Zhang Z. D., Chen A. H., Wu Y. P., Chen S. H., Zhang Y., et al. (2022). Effect of Cu2+on antioxidant capacity of red shell color breeding line and natural population of hard clam Meretrix meretrix. Fish. Sci. 41, 62–68. doi: 10.16378/j.cnki.1003-1111.20145
Wand F., Zhao Y., Lu J., Liu C. (2008). Effect of Cu2+ on the antioxidant enzyme of scallop Chlamys farreri’s viscera. Fish. Sci. 27, 622–624. doi: 10.16378/j.cnki.1003-1111.2008.12.004
Xia Y. Q., Li W. B. (2015). Study of calcein fluorescence properties. Guangdong Chem. Industry 42, 29–30. doi: 10.3969/j.issn.1007-1865.2015.07.014
Zhang S., Sun Y., Song Y., Yu Z. (2004). Kinrtic features of four heavy metals bioaccumulation of mussel Mytilus edulis. Oceanol. Limnol. Sin. (in Chinese). 05, 438–445. doi: 10.3321/j.issn:0029-814X.2004.05.007
Zhang Q., Wu X., Zheng J., Sun M. (2016). Progress of researches on methods for determination of malondialdehyde in biological samples. Phys. Test. Chem. Anal. 52, 979–985. doi: 10.11973/lhjy-hx201608026
Zhao P., Yang H. S., Wang X. Y., Lin C. G., Li X. P. (2011). Marking sea cucumber Apostichopus japonicus with calcein and its effects on antioxidant system. J. Fish. China 35, 372–378. doi: 10.3724/SP.J.1231
Keywords: Potamocorbula laevis, calcein, marking conditions, antioxidant capacity, fatty acid
Citation: Gao J, Xie X, Liu X-F, Bai Y-A, Yang M, Teng W-M, Liu H-J and Wang Q-Z (2024) Efficacy of calcein as a chemical marker of Potamocorbula laevis. Front. Mar. Sci. 11:1379571. doi: 10.3389/fmars.2024.1379571
Received: 31 January 2024; Accepted: 12 July 2024;
Published: 26 July 2024.
Edited by:
Tangtian He, Hong Kong Polytechnic University, Hong Kong SAR, ChinaReviewed by:
Alfonso Olaya Abril, University of Cordoba, SpainCopyright © 2024 Gao, Xie, Liu, Bai, Yang, Teng, Liu and Wang. This is an open-access article distributed under the terms of the Creative Commons Attribution License (CC BY). The use, distribution or reproduction in other forums is permitted, provided the original author(s) and the copyright owner(s) are credited and that the original publication in this journal is cited, in accordance with accepted academic practice. No use, distribution or reproduction is permitted which does not comply with these terms.
*Correspondence: Qing-Zhi Wang, d3F6bG1AMTI2LmNvbQ==
†These authors have contributed equally to this work
Disclaimer: All claims expressed in this article are solely those of the authors and do not necessarily represent those of their affiliated organizations, or those of the publisher, the editors and the reviewers. Any product that may be evaluated in this article or claim that may be made by its manufacturer is not guaranteed or endorsed by the publisher.
Research integrity at Frontiers
Learn more about the work of our research integrity team to safeguard the quality of each article we publish.