- 1Instituto de Oceanografía y Cambio Global, IOCAG, Universidad de Las Palmas de Gran Canaria, Las Palmas de Gran Canaria, Spain
- 2Department of Ecology and Coastal Management, Instituto de Ciencias Marinas de Andalucía (ICMAN-CSIC), Cádiz, Spain
- 3Institute of Zoology, University of Regensburg, Regensburg, Germany
- 4Biodiversidad, Ecología marina y Conservación Research Group (BIOECOMAC) Research Group, Universidad de La Laguna, San Cristobal de La Laguna, Spain
The early life of most decapod crustaceans takes place in the water column as larvae before they settle in benthic habitats. The spatial and temporal variability in the settling of larval stages offers valuable insights into the potential recruitment of natural populations. To explore this, we studied megalopa assemblages at various segments of coastline on El Hierro and Lanzarote islands, both within and outside the Marine Protected Areas (MPAs) of each island. The study spanned four consecutive oceanographic periods in the Canary Islands (NW Africa), employing light traps for sample collection. The low number of recorded species suggested that light traps exhibit selectivity, particularly for megalopae belonging to Portunidae and Grapsidae. El Hierro, which experienced warmer sea surface temperatures, displayed higher megalopa abundance values than Lanzarote and distinct larval assemblages was observed between these two islands. Similarly, we identified significant variations in abundance and species composition between stratified and mixing seasons. These seasonal differences were influenced by the dominance of Achelous hastatus, Percnon gibessi, and Cronius ruber during the stratified season. In Lanzarote, C. ruber was not recorded during the mixing season, suggesting that the colder conditions there may constrain its reproduction. Interestingly, we observed that species traditionally harvested from the intertidal zone for human consumption (Plagusia depressa) or used as bait for recreational fishing (Pachygrapsus spp. and P. gibessi) exhibited higher megalopa abundances within the MPAs. In contrast, we noted lower megalopa densities of the non-indigenous species C. ruber within the protected areas. These results indicate a positive effect of MPAs controlling indigenous and non-indigenous crab populations. Moreover, the study provides novel data, showing that light traps are suitable for monitoring the temporal occurrence, abundance, and spatial distribution of non-indigenous and commercially exploited species. This is key for adopting an ecosystem-based approach to manage marine resources.
1 Introduction
Marine brachyuran crabs have a complex life-cycle consisting of two main phases: one that occurs in the benthic environment during the juvenile-adult period and another in the pelagic realm as larvae (Anger, 2006). Both phases are crucial and interdependent as the larval success depends on the maternal investment, and the maintenance of adult populations requires a sufficient level of larval recruitment (Oliphant & Thatje, 2021). The larval phase consists of a series of zoea stages, which are specialized for the planktonic life and finish with a megalopa stage. This last stage emerges after a metamorphosis to prepare the larvae in the transition plankton-benthos. Although these larval stages have limited size and swimming capabilities, they have developed different morphologies and behaviors to ensure an optimum foraging, growth, and predator avoidance to survive (Bashevkin et al., 2020).
The interdependence of pelagic and benthic phases makes the study of planktonic larval stages of paramount importance to understand changes at population-level (Hjort, 1914). Consequently, many efforts have been made to increase our knowledge of early life stages of decapod crustaceans. These include the analyses of the biodiversity in sensitive ecosystems (Brandão et al., 2016), the investigation of reproductive phenology (González-Gordillo & Rodríguez, 2003; Landeira & Lozano-Soldevilla, 2018), the identification of transport pathways and population connectivity (Pires et al., 2020; Clavel-Henry et al., 2021), the early detection of non-indigenous species (Torres et al., 2012; Marco-Herrero et al., 2018), the assessment of the effectiveness of Marine Protected Areas (MPAs) (Whomersley et al., 2018), and the examination of the impact of climate change (Lindley et al., 2010). Considering their significance, a comprehensive understanding of the early life stages of decapods is a prerequisite for adopting an ecosystem-based approach to management. This is particularly crucial under the EU Marine Strategy Framework Directive (MSFD - Directive 2008/56/EC), where understanding of the early life stages serves as a descriptor for determining good environmental status and plays a role in establishing MPAs. Additionally, it is essential for monitoring the temporal occurrence, abundance, and spatial distribution of non-indigenous and commercially exploited species.
The Canary Islands archipelago is an interesting region to investigate larval dynamics of marine invertebrates. The archipelago is located in a subtropical transition area in the NE Atlantic and shows clear signs of tropicalization due to ongoing warming of the waters (González et al., 2017; González-Delgado et al., 2018). In terms of faunistic composition, it presents high diversity of marine species, and the decapod crustaceans are well studied comprising more than 374 species reported (González, 2018). To preserve and restore the biodiversity, as well as to enhance the total biomass of commercial species and therefore, support the local artisanal fisheries, the Canary Island government has established MPAs in the islands of Lanzarote, La Palma and El Hierro (Sanabria-Fernandez et al., 2019). The Canary Islands extends westward from near the NW African coast to the open ocean, acting as a barrier to the flow of both the Canary Current and the Trade winds inducing an intense mesoscale activity southward the islands (Barton et al., 1998). This mesoscale activity is a major driver in the distribution of decapod larvae in the open waters. Eddies and the lee zone formed downstream of the archipelago due to the island-mass effect (Sangrà et al., 2007) serve as retention zones for larvae of neritic species (Landeira et al., 2009, Landeira et al., 2010, Landeira et al., 2013). Moreover, it has been observed that upwelling filaments from the continental shelf transport larvae from African populations towards the island coasts, serving as potential pathways of connectivity (Landeira et al., 2012; Landeira et al., 2017). The year-round upwelling system off NW Africa generates high variability in the physical, chemical, and biological conditions (Arístegui et al., 2009). Thus, islands closer to Africa are more influenced by the upwelling system, showing colder and nutrient-richer waters than the western side of the archipelago. Moreover, the temporal variability of the oceanographic conditions characterizes two distinct seasons in the region. The productive season (Late Winter Bloom) takes place from January to May due to surface waters cooling and vertical mixing of nutrients (Arístegui et al., 2001). The rest of the year (from late spring to autumn) the region is characterized by a strong stratification of the water column giving rise to surface warming and oligotrophy (Couret et al., 2023a). This seasonality determines temporal changes in plankton biomass and composition (Schmoker et al., 2012; Couret et al., 2023b), and reproductive strategies of decapod crustaceans (Landeira & Lozano-Soldevilla, 2018).
Despite the advances in understanding the planktonic dynamics of decapod crustacean larvae over the past decade, spatial and temporal patterns of settlement larval stages near the coast remain poorly known in the Canary Island region. Only observations on colonizing artificial collectors in different habitats have provided insights on the settlement preferences of post-larval stages of decapod crustaceans through an annual cycle (García-Sanz et al., 2014; Herrera et al., 2014). Filling the gap of knowledge is fundamental for understanding recruitment processes and population functioning. Ultimately, it can serve as a valuable tool for policymakers to improve the capacities for the management of marine resources and ecosystem services. This study aimed to provide information on spatial and temporal variability of the abundance, composition, and assemblages of settlement stage of brachyuran crabs in the Canary Islands by sampling with light traps during four consecutive environmental seasons. Moreover, we investigated the possible effect of MPAs controlling larval assemblages in relation to non-indigenous species and indigenous species with commercial interest.
2 Material and methods
2.1 Decapod larvae collection
Settlement stages of decapod crustaceans (referred to megalopa) were collected with the Ecocean CARE® light trap. The CARE light trap consisted of a float with a watertight block containing a rechargeable light, and a 2 m conical net (2 mm mesh size) hanging 1.5 m below the sea surface (Lecaillon, 2004). The sampling was designed to ensure segments of coastline with contrasting environmental conditions in the Canary Islands (Central Atlantic, NW Africa, Figure 1A). Considering the longitudinal gradient of temperature and productivity generated by the NW African upwelling system, we targeted the islands of Lanzarote (eastern side of the archipelago, with high upwelling influence) and El Hierro (westernmost island, non-upwelling influence) (Figure 2; Table 1). Thus, samples were collected during the stratified-warm season (years 2019 and 2020) and mixing-cold season (years 2020 and 2021) at 4 segments of coastline in Lanzarote and 4 in El Hierro (Figures 1B, C; Table 1). Two of those segments were placed inside each MPA of “Isla de La Graciosa e Islotes del Norte’’ in Lanzarote, and of “La Restinga-Mar de las Calmas” in El Hierro (Figures 1B, C; Table 1). Samples were not obtained at Lanzarote’s MPA stations (traps A1-3, F1-3) because of adverse weather conditions during the 2019 campaign. Furthermore, one sample was lost during the 2021 campaign due to a mesh breakage in the trap Q2. At each segment of coastline, three light traps were moored nearshore, at 10-25 m depth and at 20-300 m from the coastline. The traps were set maintaining a minimum distance of 300 m apart from each other to prevent light overlapping (Félix-Hackradt et al., 2013; Moreno-Borges et al., in press). Traps were left overnight; usually deployed and retrieved 1-2 hours before the sunset and after sunrise, respectively. Thus, for each campaign (seasonal sampling on an island), the segments of coastlines were sampled on consecutive days. For example, during the stratified sampling season of 2019 on El Hierro island, the Orchilla coastline segment was sampled on October 20th, Bonanza on the 21st, Tacorón on the 22nd, and Bahía de Naos on the 23rd. On board, the samples were recovered, labelled, and preserved in 95% ethanol. In the laboratory, decapod larvae from each sample were sorted and transferred to new preservation liquid (ethanol 95%) for posterior taxonomic identifications.
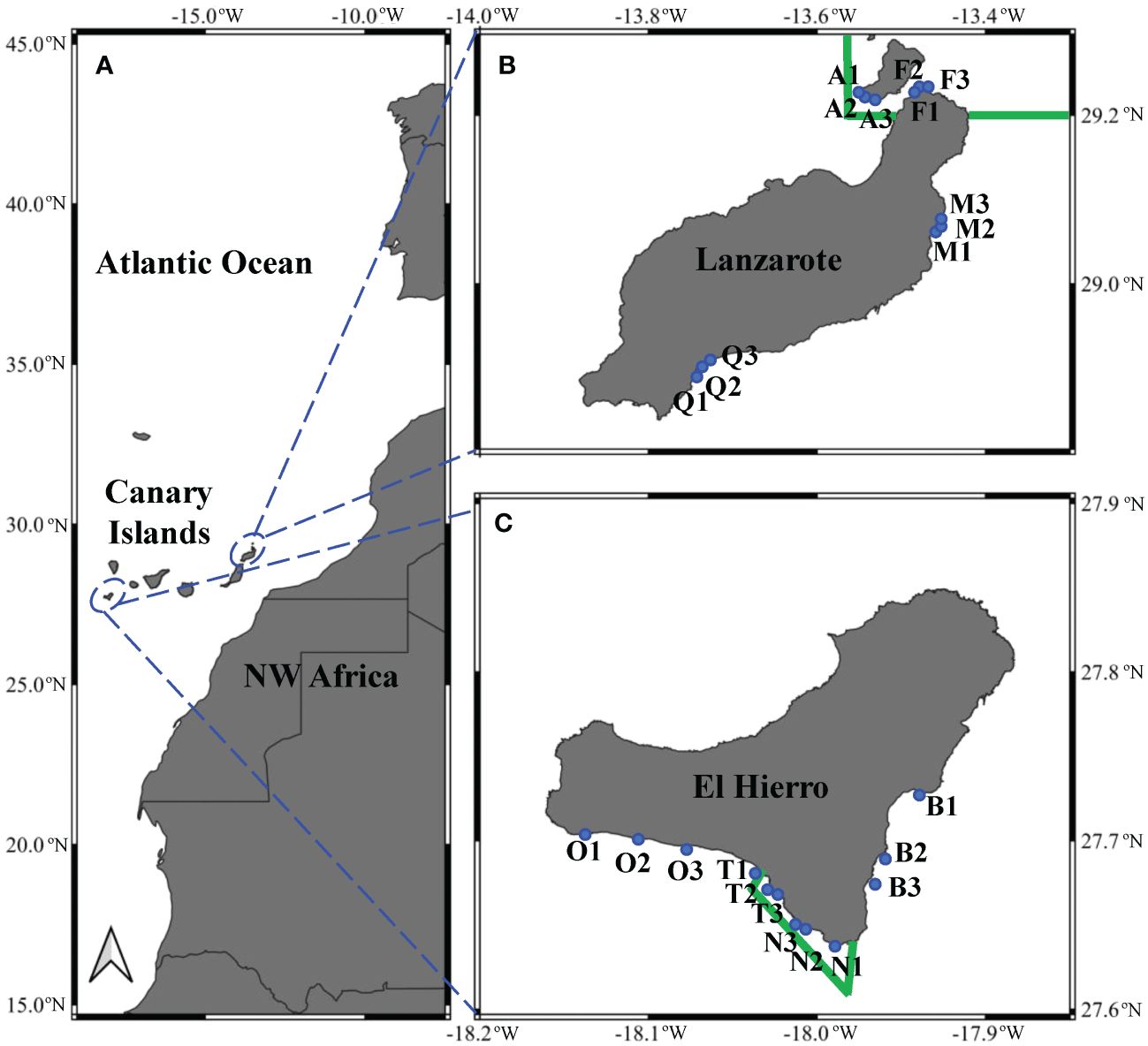
Figure 1 Location of the sampling stations in the Canary Islands, NW Africa (A). Green lines delimit the boundaries of the Marine Protected Areas in Lanzarote (B) and El Hierro (C) islands. Blue dots stand for section of coastline: A = Montaña Amarilla, F = Punta Fariones, M = Mala, P = Playa Quemada, B = Bonanza, N = Bahía de Naos, T = Tacorón, O = Orchilla.
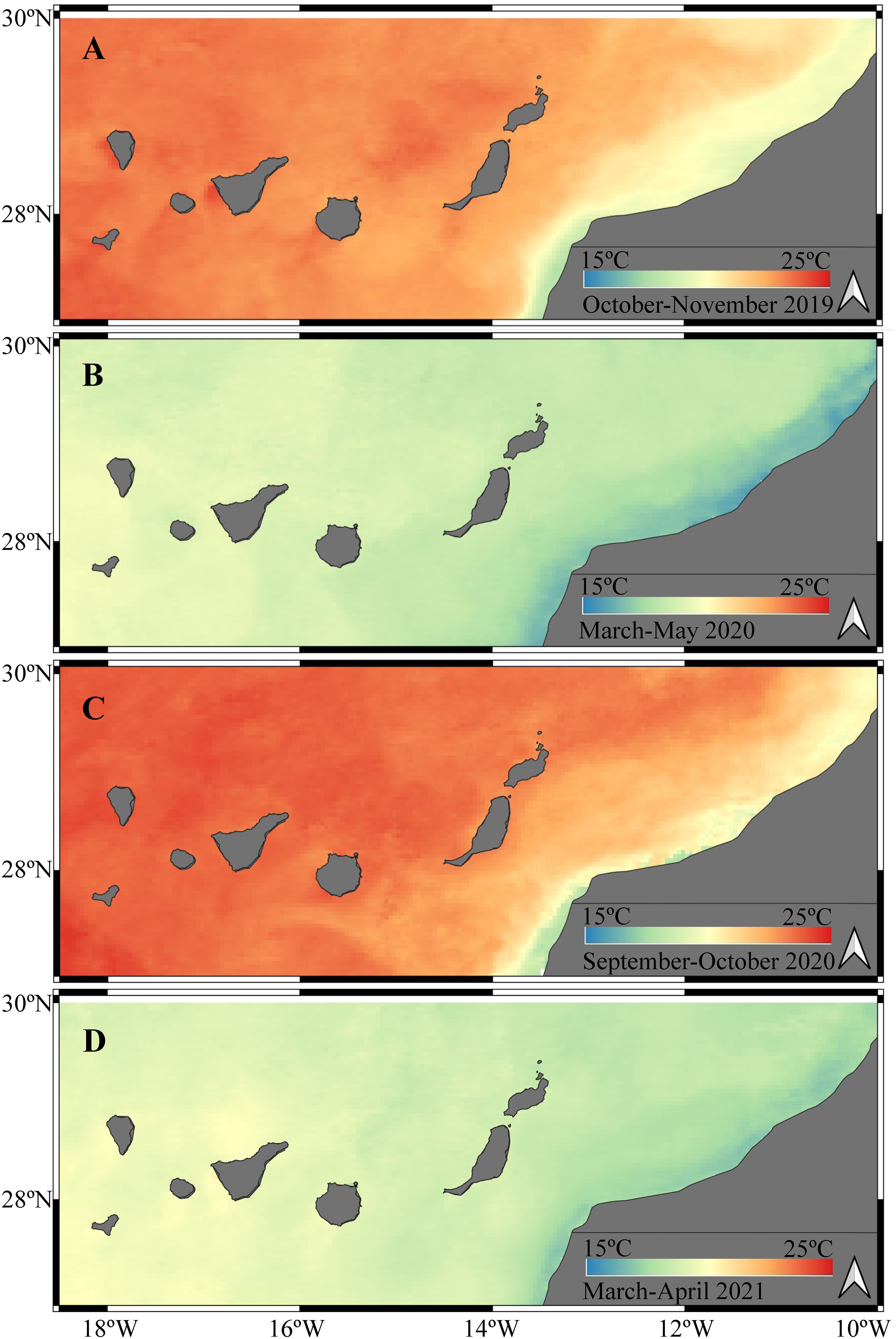
Figure 2 Sea surface temperature derived from satellite data. Maps correspond to averaged values of sea surface temperature during each sampling period: October-November 2019 (A), March-May 2020 (B), September-October 2020 (C), March-April 2021 (D).
2.2 Taxonomic identifications
Most of the larvae collected were in megalopa stage; however, occasionally few zoea stage larvae occurred in the catches. As this study is focused on settlement stages, only the megalopa was analyzed. In the laboratory, each megalopa was identified using a stereomicroscope and following the taxonomic key of Ingle (1992) and Marco-Herrero (2015), as well as specific larval morphology descriptions of decapod crustaceans recommended by González-Gordillo et al. (2001). As the knowledge on the morphology of megalopae is limited in subtropical areas such as the Canary Islands, we removed the fifth pereiopod of those unidentified megalopae with distinct phenotype for molecular identification.
2.3 Molecular identification
The identification of the megalopae, and one adult specimen of Thalamita poissonii, was based on partial sequences of the 16S rRNA or cytochrome oxidase unit I (COI) mitochondrial genes. Total genomic DNA of the megalopa specimen, and the adult, was extracted from a pereiopod following a modified Chelex 10% protocol by Estoup et al. (1996). The 16S rRNA and COI genes were amplified with polymerase chain reaction (PCR) using the following cycling conditions: 2 min at 95°C, 35 cycles of 20s at 95°C, 20s at 45-48°C, 45s (16S) or 47s (COI) at 72°C, and 5 min at 72°C. The primers 1472 (5’-AGA TAG AAA CCA ACC TGG-3’) (Crandall & Fitzpatrick, 1996) and 16L2 (5’-TGC CTG TTT ATC AAA AAC AT-3’) (Schubart et al., 2002) were used to amplify a maximum of 545 bp of 16S rRNA, and for the COI gene the primers COH6 (5´-TAD ACT TCD GGR TGD CCA AAR AAY CA-3´) and COL6b (5’-ACA AAT CAT AAA GAT ATY GG-3’) (Schubart & Huber, 2006) were used to amplify a maximum of 670 bp.
PCR products were sent to Stab Vida company to be purified and then bidirectionally sequenced. Sequences were edited using the software Chromas version 2.0. With the obtained final DNA sequences were performed a BLAST (Basic Local Alignment Search Tool) on NCBI (National Center for Biotechnology Information) web facility on GenBank sequences database (http://www.ncbi.nlm.nih.gov/genbank/) to get the best matches for identification. The COI sequences were also searched in the official Barcode of Life database (BOLD) (http://v3.boldsystems.org/index.php/IDS_OpenIdEngine). Identifications were considered as positive when retrieved sequences showed similarity values greater than 99%, only differed in 1–3 or 1–7 mutations in 16S or COI, respectively. This is a more conservative limit than other previous works identifying decapod larvae considering > 98% (Brandão et al., 2016). Larval sequences for both genes are deposited in Genbank, and the megalopa and adult specimens DNA vouchers were deposited in “Museos de Tenerife - Naturaleza y Arqueología” (MUNA).
2.4 Data analysis and statistics
Sea surface temperature derived from long-wave (11-12 µm) thermal radiation satellite data were obtained from the Ocean Color web site (https://oceancolor.gsfc.nasa.gov/) using long-wave infrared algorithm and Aqua MODIS information (Kilpatrick et al., 2015). Averaged data were computed for the study period of each sampling season and plotted using the geographic information system QGIS 3.18 (QGIS Development Team, 2022).
Abundance of megalopa were standardized into catch per unit of effort (CPUE), with ‘effort’ being both number of traps and time fished (megalopae per trap x night) (Félix-Hackradt et al., 2013). This standardization was important in those samplings with trap failures. The Shannon–Wiener diversity index (H′), (where S is the number of taxa and pi is the proportion of individuals in taxa i), was based on all identified taxa.
Regarding statistical analysis, 3-way PERMANOVA (Anderson et al., 2008), based on Bray-Curtis distances matrix calculated from log (x+1) transformed CPUE data of each taxon. PERMANOVA tested differences in the CPUE of megalopa assemblages between islands (El Hierro vs Lanzarote), level of protection (inside vs outside the MPA) and sampling season (mixing vs stratified). In this model design, “island”, “season”, and “protection” were fixed factors, being “protection” nested in “island”. P-values were calculated from 999 unrestricted permutations of the data. Multivariate analysis of assemblage structure using multidimensional scaling (MDS) was applied to visualize differences in the structure of the entire community, and the individual contribution of each species to the dissimilarity for each factor (island, season, and protection) was calculated by the SIMPER routine (Clarke et al., 2014). All statistical analyses were carried out using the software PRIMER 7.0.23 + PERMANOVA routine.
3 Results
3.1 Environmental conditions
Satellite imagery pictured the spatio-temporal variability of sea surface temperature (SST) in the Canary Islands region (Figure 2). Upwelling waters were present in the African shelf showing cold SST (16.68-18.03 °C). The limited longitudinal extension of the upwelled waters generated a westward increase of SST in the study region up to 4-5 °C during the stratified season and 3-3.5 °C during the mixing season. Thus, El Hierro exhibited warmer SST values than Lanzarote in both seasons. For example, during the stratified season Lanzarote showed a mean SST of 22.48 ± 0.36 °C, whereas in El Hierro the mean SST was 23.67 ± 0.41 °C (Figures 2A, C). During the mixing season, typical lower SST values were observed in Lanzarote (18.96 ± 0.42 °C) and El Hierro (19.72 ± 0.30 °C) (Figures 2B, D).
3.2 Molecular identification
We identified a subset of megalopae as A. hastatus by matching their sequences with those deposited by Mantelatto et al. (2009); Schubart & Reuschel (2009), and Marco-Herrero et al. (2021). Additionally, using sequences published by González et al. (2017), we identified other portunid megalopae as C. ruber. Sequences obtained from two adult specimens we deposited in the MUNA allowed us to identify further megalopae as T. poissonii. Big-sized grapsid megalopae aligned with sequences of Grapsus adscensionis from Schubart (2011). Molecular analysis revealed significant similarity between a group of megalopae and sequences of Cryptosoma spp. from GenBank, as well as with sequences of Dromia erythropus submitted by Mantelatto et al. (2018). Considering the known distribution of these species and the species documented in the Canary Islands (González, 2016), we assigned our sequences to C. cristatum and D. marmorea. For comprehensive details on the molecular identifications, please refer to Supplementary Material 1.
3.3 Spatio-temporal patterns of megalopa larvae
In the framework of this study, light traps collected a total of 65,639 crab megalopae belonging to 12 different taxa (Table 2). The maximum abundance value of 8,832 megalopae per trap x night occurred in El Hierro Island within the Marine Protected Area at Tacorón (T3 trap) during the stratified season of 2019. In contrast, significantly lower megalopa catches were observed in Lanzarote, averaging 78.75 megalopae per trap x night, compared to El Hierro, where the average was 1,300 megalopae per trap x night. In terms of seasonality, megalopae were more abundant during the stratified period (averaging 1,375.64 megalopae per trap x night) than during the mixing period (averaging 160.64 megalopae per trap x night). With respect to total abundance, more larvae were collected outside the MPA (averaging 868.81 megalopae per trap x night) than inside (averaging 590.59 megalopae per trap x night). Shannon diversity index was low (ranging from 0.43 to 1.99) due to the limited number of taxa found. Similar diversity values were observed between seasons (mixing: 1.19; stratified: 1.27) and islands (El Hierro: 1.22; Lanzarote: 1.17). However, there was a weak difference in level of protection where the average value of diversity was higher (1.35) inside the MPA than outside (1.13).
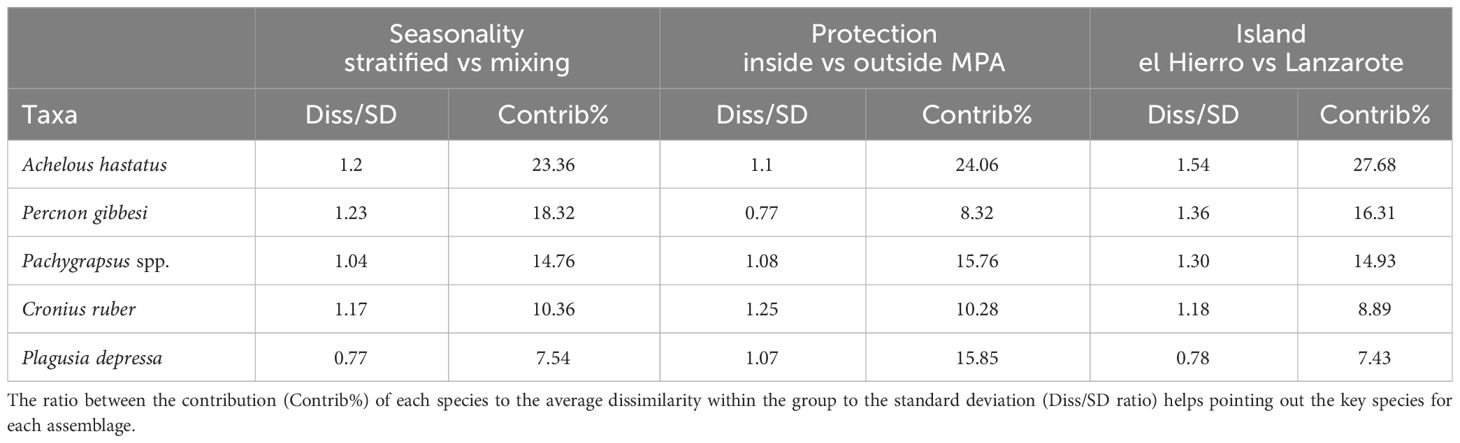
Table 2 Similarity percentage (SIMPER) results for seasonal, protection, and island assemblages, showing the most important species contributing to each group (cut-off for lower contributions 70%).
Permutational multivariate analysis of variance (PERMANOVA) revealed significant differences in megalopae abundance and species composition for factors “seasonality” (PERMANOVA pseudo-F=27.87; P=0.001), “islands” (PERMANOVA pseudo-F=51.00; P=0.001) and “protection” (PERMANOVA pseudo-F=5.69; P=0.001). The non-metric multidimensional scaling analysis (nMDS), based on species composition and abundance, separated the samples among both seasons and islands (Figures 3A, B). However, when considering the factor “protection”, the nMDS did not show a clear separation, despite the significant differences detected in the PERMANOVA analysis (Figure 3C). Moreover, overlaying the total megalopa abundance on the nMDS plots not only aided in visualizing the higher values both during the stratified season (Figure 3D) and in El Hierro island (Figure 3E), but also the weak separation of sample collected inside and outside the MPAs (Figure 3F). Similarly, overlaying the Shannon diversity indexes confirmed the similar values across factors (Figures 3G–I).
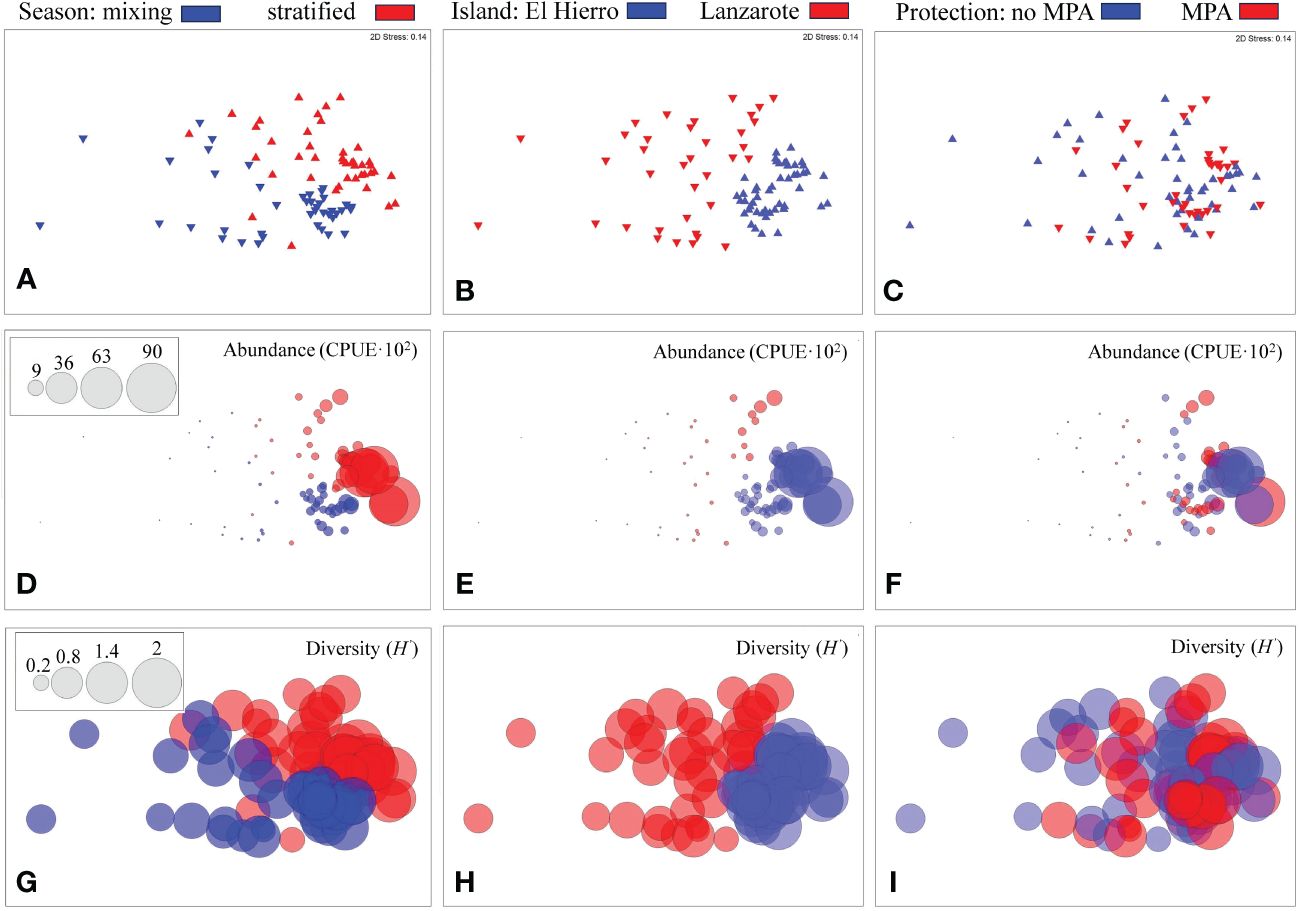
Figure 3 Non-metric Multidimensional Scaling (nMDS) plots based on megalopae abundance and composition for each factor: season (A, D, G), islands (B, E, H) and protection (C, F, I). Bubble size stand for total abundance, CPUE·10-2 (D, E, F) or Shannon diversity index, H’ (G, H, I).
Spatial and temporal distributions of five taxa accounted for 70% of the differences observed in both “seasonality” and “level of protection” (Table 2). The SIMPER analysis showed the high contribution of A. hastatus, P. gibessi, C. ruber, Pachygrapsus spp. and P. depressa to the average dissimilarity between seasons (57.93%) and islands (61.06%) (Table 2). Temporal distribution of A. hastatus, P. gibessi, C. ruber indicated a dominance during the stratified season (Figures 4, 5). Interestingly, megalopae of A. hastatus, the most abundant taxa, and P. gibessi occurred in both seasons, but exhibited a clear seasonality in El Hierro (Figure 4) that was not so evident in Lanzarote (Figure 5). In the case of C. ruber, the phenological seasonality was observed in both islands. However, its megalopae occurred year-round in El Hierro (Figure 4), whereas in Lanzarote they were present only during the stratified period (Figure 5). On the other hand, the higher abundance of Pachygrapsus spp., P. depressa, and P. gibessi, and the lower abundance of C. ruber inside the MPA (Figures 6, 7) contributed to differentiate the community assemblages based on the protection level, exhibiting an average dissimilarity of 56.56% (Table 2). Again, this pattern of protection was less consistent in Lanzarote (Figure 7).
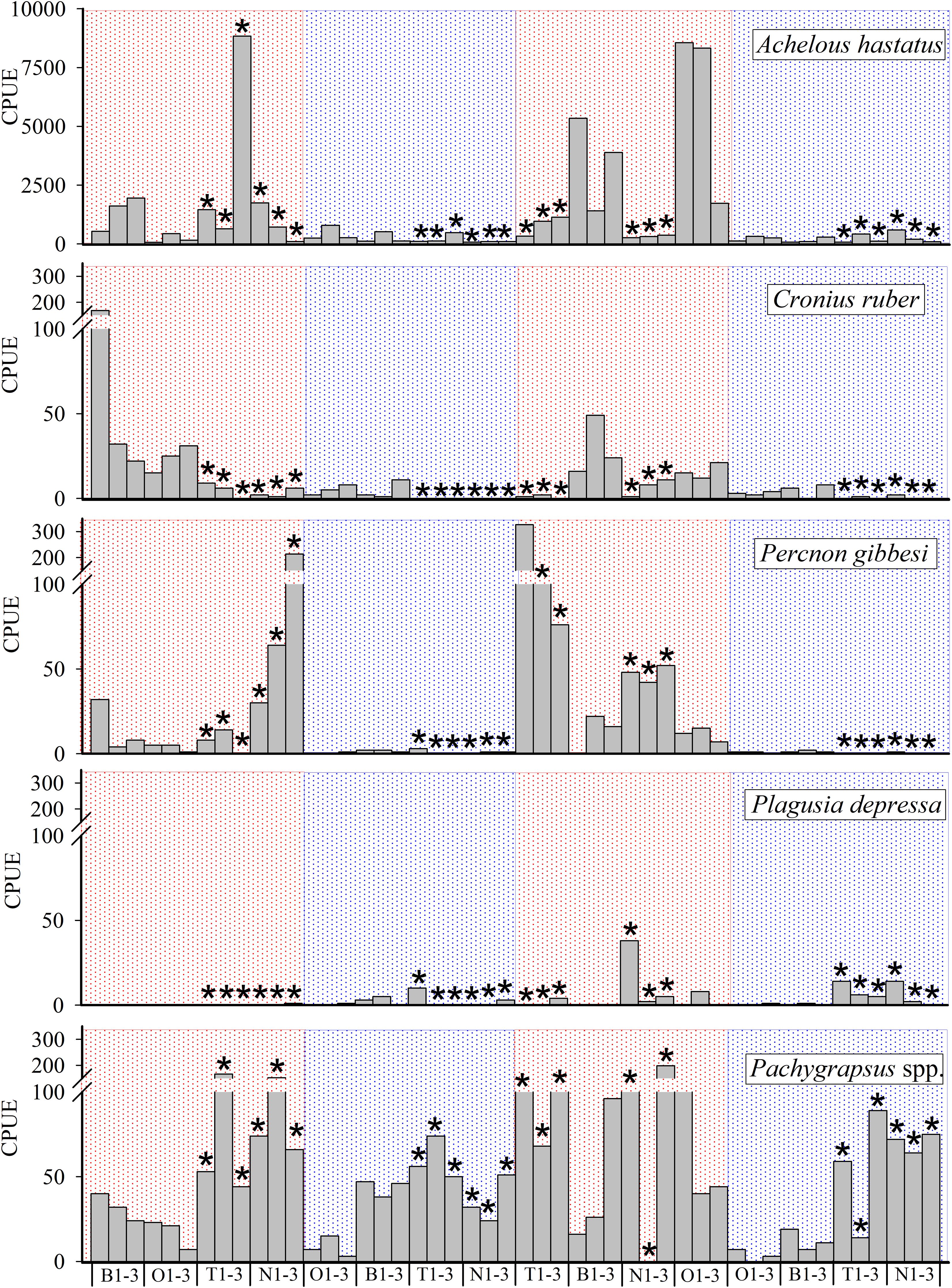
Figure 4 Temporal distribution of megalopae abundance (CPUE·10-2) for key species in El Hierro. Asterisks stand for samples collected inside the Marine Protected area (MPA). Red and blue areas stand for mixing and stratified periods, respectively.
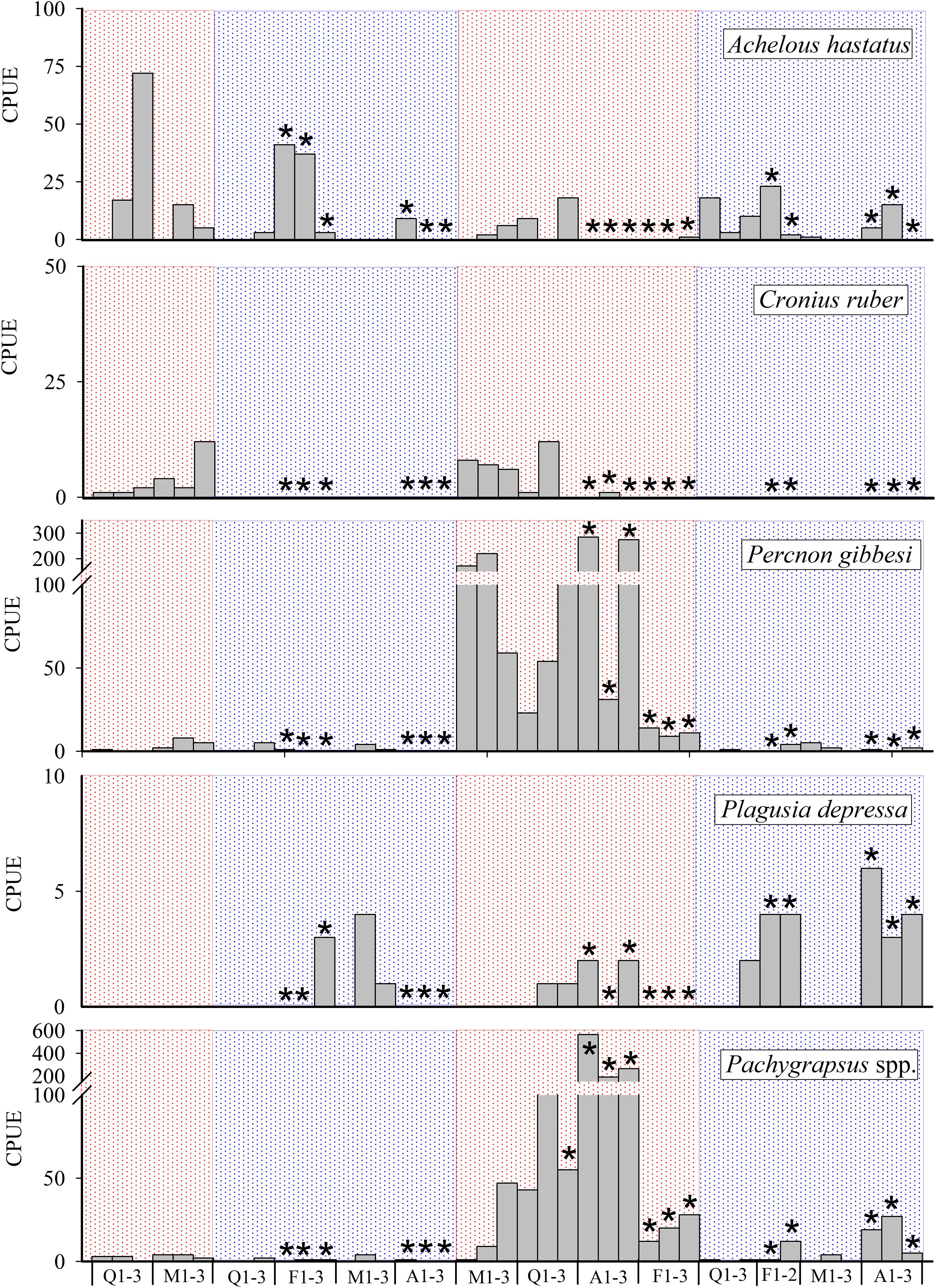
Figure 5 Temporal distribution of megalopa abundance (CPUE·10-2) for key species in Lanzarote. Asterisks stand for samples collected inside the Marine Protected area (MPA). Red and blue areas stand for mixing and stratified periods, respectively.
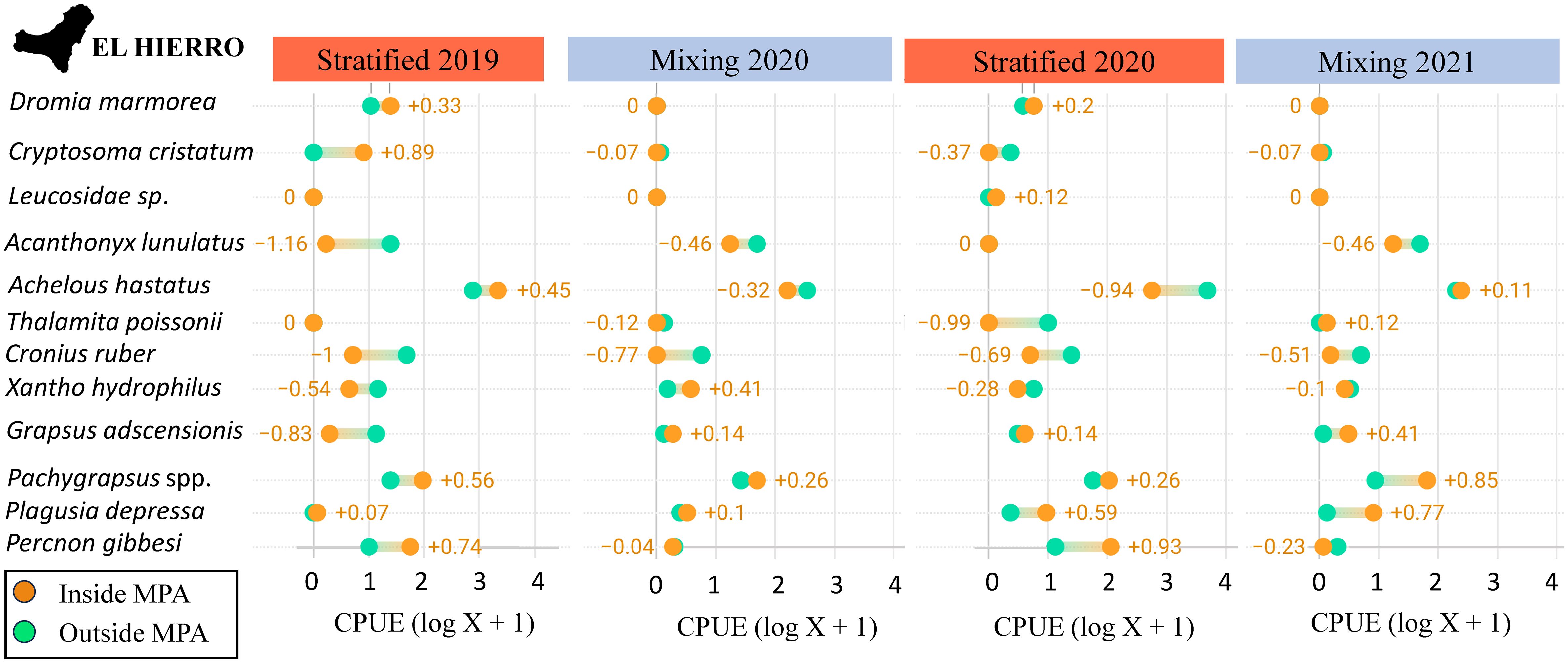
Figure 6 Mean abundance values, log (CPU +1), of each taxon within and outside the Marine Protected Area (MPA) for each sampling period in El Hierro. Orange values are obtained subtracting the abundance within the MPA from the abundance outside the MPA.
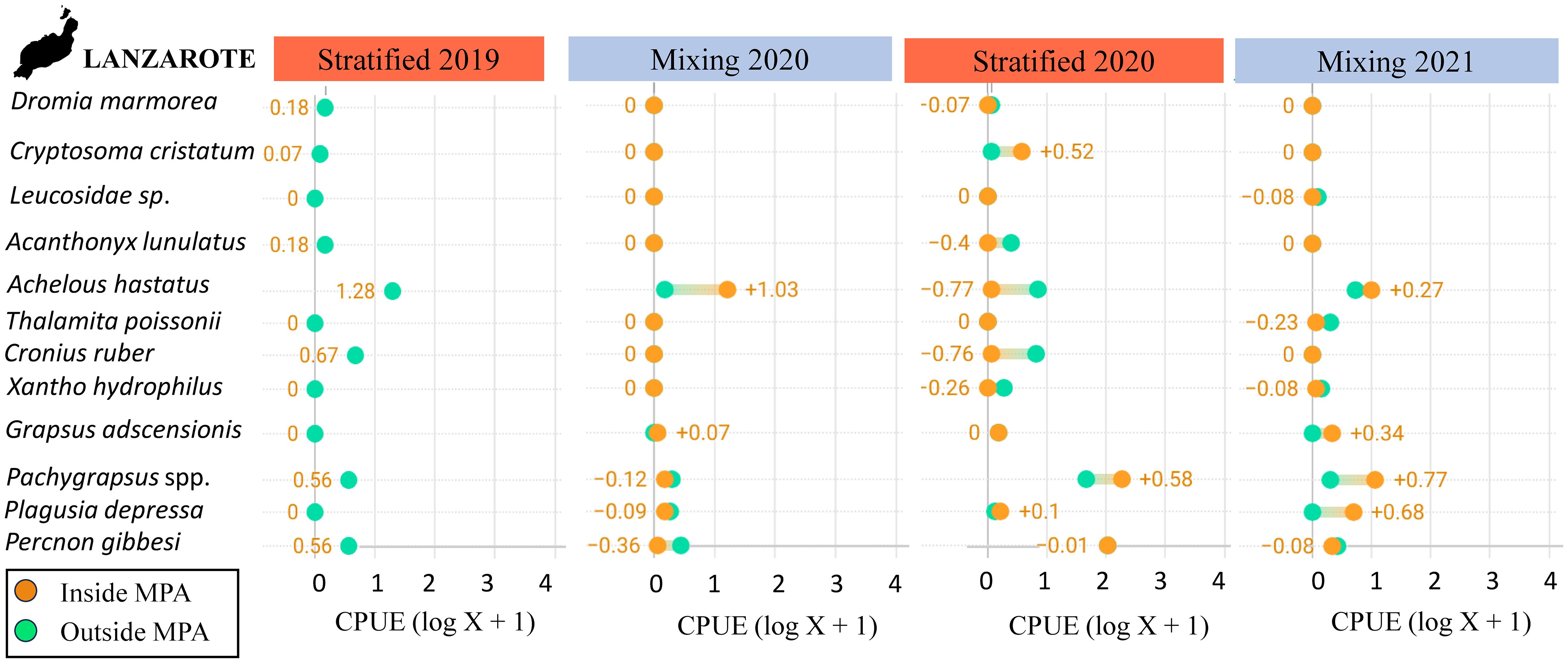
Figure 7 Mean abundance values, log (CPU +1), of each taxon within and outside the Marine Protected Area (MPA) for each sampling period in Lanzarote. Orange values are obtained subtracting the abundance within the MPA from the abundance outside the MPA.
4 Discussion
4.1 Methodological considerations
Light traps offer a passive sampling method suitable for shallow coasts and reefs where working with plankton nets can be challenging. Their ability to collect undamaged and even live specimens at a low cost has led to their widespread utilization in the collection of larval stages of marine organisms (McLeod & Costello, 2017). While light traps have predominantly been employed for the collection of fish larvae (e.g. Félix-Hackradt et al., 2013), their effectiveness in capturing invertebrate larvae, including decapod crustaceans, has also been demonstrated (Reyns & Sponaugle, 1999; Jeffs et al., 2003; Miller and Shanks, 2004; Herter & Eckert, 2008; Sigurdsson et al., 2014; Moreno-Borges et al, in press). Previous studies on decapod larvae have involved adaptations of the common box traps, cylindrical traps, quatrefoil traps, and tube traps. However, only the sampling framework analyzed in the present study utilized a net trap like the CARE light trap (see Moreno-Borges et al., in press) for additional methodological information). Interestingly, despite the utilization of different collecting methods, it appears that all light traps exhibited high selectivity for individuals in settlement stage. In terms of selectivity, the light traps also captured various organisms, including fish larvae, hyperbenthic taxa (mysids, amphipods, and isopods), as well as certain planktonic copepods and ostracods. Nevertheless, it is noteworthy that the light traps showed remarkable selectivity towards the megalopa stage of crabs, comprising a dominant proportion exceeding 95% of the total yield of decapod larvae. Indeed, when Porter et al. (2008) conducted a comparative analysis of the effectiveness of light traps and plankton tows for sampling brachyuran crab larvae, they observed that traps were more efficient at capturing fast-swimming megalopae, which possessed the ability to evade the nets. This observation is substantiated by the limited presence of megalopae in plankton samples previously examined in the Canary Islands region (Landeira et al., 2013, Landeira et al., 2017; Landeira & Lozano-Soldevilla, 2018). Hence, the concurrent utilization of both plankton nets and light traps appears to be a suitable approach for investigating dispersive and settling processes of zoea and megalopa larval stages. However, the ability of the CARE light trap to capture a broad spectrum of species is limited. Given the presence of 132 crab species within the marine fauna of the Canary Islands (González, 2016) and the identification of approximately 40 species from plankton samples (Landeira et al., 2013, Landeira et al., 2017; Landeira & Lozano-Soldevilla, 2018), the identification of only 12 taxa from light traps appear to be a relatively limited number.
On the contrary, the low number of species may also indicate that light traps are very selective for certain species. More specifically, we collected high numbers of portunid megalopae, with A. hastatus as the most abundant species, as well as grapsoid megalopae particularly represented by Pachygrapsus spp. Similarly, using quatrefoil traps in Caribbean coral reefs, Reyns and Sponaugle (1999) found in the catches a significant contribution of the families Portunidae and Grapsidae along with Majidae. As light traps are designed to attract (McLeod & Costello, 2017), it is reasonable to infer that the megalopa stage of these two families may exhibit a phototaxis behavior that attracts them towards the trap amidst the darkness. Portunidae is one of the crab families that encompasses a higher number of non-indigenous species worldwide. Their large body size, swimming ability and aggressive behavior, as well as its high fecundity and broad environmental tolerance have facilitated the expansion of several species far from their distribution ranges such as Callinectes sapidus in the Mediterranean Sea and Atlantic Ocean (González-Ortegón et al., 2022), Charybdis japonica in New Zealand (Fowler and McLay, 2013) or C. ruber in the Canary Islands (González et al., 2017). Hence, we suggest employing light traps for the monitoring or early detection of non- indigenous portunid species, that can enable a prompt response for the management and mitigation of the potential invasion. Our study demonstrates that for non- indigenous species lacking taxonomic descriptions of larval morphology, such as C. ruber, the combination of light trap sampling and molecular identification can indeed serve as a potent tool.
4.2 Spatial and seasonal patterns
The study was designed to test differences in species composition between distant islands with contrasting oceanographic conditions. The oceanographic gradient from east to west across the archipelago plays a significant role in shaping the distribution of biota (Brito et al., 2001). In the western islands, characterized by warmer waters, there is a greater prevalence of tropical species. Conversely, in the eastern islands with temperate waters, species exhibiting temperate affinities are more commonly found (Tuya et al., 2004; Sangil et al., 2012a). As expected, we observed warmer temperatures in El Hierro than in Lanzarote which was clearly influenced by colder waters from the NW African upwelling (Barton et al., 1998). The relatively low number of species observed in the samples, resulted from the high light trap’s selectivity for capturing megalopae of certain species, hindered the ability to characterize the expected east-west diversity gradient. However, differences in megalopa abundance A. hastatus, C. ruber, Pachygrapsus spp., P. gibbesi, and P. depressa allowed the separation of two distinct assemblages for Lanzarote and El Hierro.
The seasonality of decapod larvae in the plankton is primarily governed by the reproductive phenology of adult populations. In the present study, we found significant differences in megalopae abundance and species composition between stratified and mixing seasons. Data derived from plankton nets in the Canary Islands has indicated that most decapod species exhibit continuous reproduction throughout the year, with peaks in abundance coinciding with periods of water stratification and mixing (Landeira & Lozano-Soldevilla, 2018). Consistent with our findings, colonization experiments conducted in various habitats including, seagrass meadows, sandy patches, and macroalgal-dominated beds on Gran Canaria Island (located centrally in the archipelago) have likewise noted a seasonality in the settlement of postlarval stages of decapods linked to warm and cold periods of the year (García-Sanz et al., 2014; Herrera et al., 2014). In the Atlantic Ocean, this seasonality becomes progressively more pronounced as one moves northward (González-Gordillo & Rodríguez, 2003; Pan et al., 2011; Stübner et al., 2016). In future investigations, it would be interesting to conduct weekly light trap samplings throughout an entire year to better understand the annual cycle of settlement larval stages.
In the present study, seasonal differences were driven by the dominance of A. hastatus, P. gibessi, and C. ruber during the stratified season. Interestingly, the tropical-subtropical species like P. gibessi and C. ruber were particularly abundant in El Hierro, where higher temperature occurs. Monthly samplings were conducted to assess the status and population structure of these species on Gran Canaria Island. Regarding P. gibessi, Guerra-Marrero et al. (2023) identified two primary reproductive seasons (March-April and August-September) based on the presence of ovigerous females. Similarly, for C. ruber, females with mature ovaries were observed consistently throughout the year, with a higher abundance noted from July to November, coinciding with the peak of ovigerous females (Triay-Portella et al.)1. These reproduction strategies are consistent with our observations of megalopa dynamics. Nevertheless, it is important to delve into the differing temporal distribution of C. ruber megalopae for each island. In El Hierro, we observed megalopae in both seasons, with a higher abundance during the stratified season, aligning with the reproduction pattern detailed by Triay-Portella et al1. However, in Lanzarote, no megalopae were encountered during the samplings conducted in the mixing season. The differences observed between the islands may suggest that during the colder season (mixing) when reproductive activity is reduced, the colder conditions of Lanzarote (more than 1°C colder than El Hierro) may not facilitate a proper reproduction for this subtropical species. Given that C. ruber is considered a non- indigenous species in the study region, its limited reproductive performance during certain times of the year could potentially reduce its expansion in the eastern islands, such as Lanzarote, which are more influenced by the cold waters originating from the NW Africa upwelling. It is well-established that temperature plays a pivotal role in driving spatial and temporal variations in the reproductive biology of crab species, as demonstrated in the case of Portunus armatus on the West coast of Australia (Johnston & Yeoh, 2021). However, as there are no documented instances of marine invertebrates displaying spatial phenological variations within the Canary Islands, it is important to approach this pattern with caution until further data become available.
4.3 Effect of marine protected area
The present study was also designed to test differences in megalopa abundance inside and outside the MPAs of “La Restinga-Mar de las Calmas” in El Hierro, and of “Isla de La Graciosa e Islotes del Norte’’ in Lanzarote. Interestingly, we found that species traditionally harvested from the intertidal zone for human consumption (P. depressa) or used as bait for recreational fishing (Pachygrapsus spp. and P. gibessi) (González et al., 2016) presented higher megalopa abundances inside the MPAs. Artisanal fisheries in the Canary Islands have benefited from more stable yields since the MPAs were established, compared to the overexploitation of most of demersal target fish species (Sanabria-Fernandez et al., 2019). Protection areas from fishing pressure not only has increased the fish biomass within the MPAs but also the densities of harvested intertidal invertebrates, such as limpets (López et al., 2012). Unfortunately, there are no data available to understand the protection effect on crustaceans in the Canary Islands, but we expect similar positive influence since it has been proved to be effective to increase the catches of portunid crabs inside MPAs of Thailand (Jones et al., 2017).
On the other hand, we found lower megalopa densities of the non-indigenous species C. ruber in the protected areas. Inside the MPAs various mechanisms can interplay to control the population densities of non-indigenous species within their boundaries. One such mechanism is the potential barrier created by the high indigenous species richness found within MPAs, which may deter the introduction and establishment of alien species (Levine & D’Antonio, 1999). Faunistic and botanic studies have described how the seascape recovers inside the MPAs of the Canary Islands, not only increasing the number of species but also producing a more balanced and better-structured ecosystem (Sangil et al., 2012b; Sanabria-Fernandez et al., 2019). A better-structured ecosystem, which allows more competitive native species, could be preventing the development of large populations of C. ruber within the MPA. Additionally, the restoration of top predator populations within MPAs can play a pivotal role in regulating the populations of certain non-indigenous species within these protected areas (Mumby et al., 2011). In this sense, there is already evidence of the top-down role of fish predators controlling the populations of a key herbivore, the sea urchin Diadema africanum (Clemente et al., 2009) in the Canary Islands. These authors observed that the presence of higher fish predator densities within MPAs led to an increase in post-settlement and post-recruitment mortality events among D. africanum. Therefore, it is plausible that top-down ecological processes, involving fish predation on megalopae, juveniles, and even adult crabs, are influencing the populations of the non-indigenous crab C. ruber. It is known that lower megalopa densities may indicate both small adult populations and lower larval survival rate (McLeod & Costello, 2017). Thus, the increased density of fish predators within the MPAs may explain the observed lower megalopa densities there. Consequently, our results might indicate that the invasiveness capability C. ruber, is reduced within the MPA. This finding has global relevance due to the scarcity of quantitative data to estimate the effect of protection on the densities of non-indigenous species (Giakoumi & Pey, 2017). In the future, it would be interesting to concurrently assess the size of adult populations alongside larval densities using light traps within and outside the MPAs to empirically test this hypothesis.
Data availability statement
The datasets presented in this study can be found in online repositories. The names of the repository/repositories and accession number(s) can be found in the article/Supplementary Material.
Ethics statement
The manuscript presents research on animals that do not require ethical approval for their study.
Author contributions
JL: Formal analysis, Visualization, Writing – original draft. EF: Data curation, Formal analysis, Investigation, Writing – review & editing. JC: Formal analysis, Investigation, Writing – original draft. CS: Investigation, Writing – review & editing. SM: Formal analysis, Investigation, Writing – review & editing. AR: Funding acquisition, Investigation, Project administration, Supervision, Writing – review & editing.
Funding
The author(s) declare financial support was received for the research, authorship, and/or publication of this article. This work was funded by the project “Seguimiento de especies y hábitats indicadores de cambio climático a largo plazo en el archipiélago canario”, cofunded by Operational Program FEDER Canarias (2014-2010) and Gobierno de Canarias. Effrosyni Fatira was funded from the European Union’s Horizon Europe research and innovation programme under the Marie Skłodowska-Curie grant agreement No 101090322 PLEASE. JL was supported by the “Beatriz Galindo” individual grant (BEAGAL 18/00172) from the Spanish Ministry of Science and Innovation. SM-B was funded by the Canarian Agency for Research, Innovation and Information Society of the Ministry of Economy, Industry and Competitiveness and by the European Social Fund (ESF) integrated operational program of the Canary Islands 2014-2020. Adriana Rodríguez was funded by the Viera and Clavijo Senior Excellence Programme of the University of La Laguna, financed by the Canary Islands Agency for Research, Innovation, and the Information Society.
Acknowledgments
We would like to thank the colleagues who took part in the sampling expeditions and/or with the sample processing at the lab: José Carlos Mendoza, David Martorell, Sandra Montañes and Daniel Álvarez Canali. We thank the Spanish Ministries of “Agriculture, Fisheries and Food” and “Ecological Transition and Demographic Challenge”, and “Reservas Marinas de España” for processing and granting the necessary permissions for the field work. As well as, the staff directly involved in the management and vigilance of Marine Protected Areas (Zona Especial Canaria – ZEC, and Zonas de Especial Protección para las Aves – ZEPA). During the development of this study our dear friend and colleague Christoph D. Schubart tragically passed away, for this reason we would like to dedicate this work in fond memory of him.
Conflict of interest
The authors declare that the research was conducted in the absence of any commercial or financial relationships that could be construed as a potential conflict of interest.
Publisher’s note
All claims expressed in this article are solely those of the authors and do not necessarily represent those of their affiliated organizations, or those of the publisher, the editors and the reviewers. Any product that may be evaluated in this article, or claim that may be made by its manufacturer, is not guaranteed or endorsed by the publisher.
Supplementary material
The Supplementary Material for this article can be found online at: https://www.frontiersin.org/articles/10.3389/fmars.2024.1371782/full#supplementary-material
Footnotes
- ^ Triay-Portella, R., Martı́n, J. A., and Pajuelo, J. G. Reproductive features of the invasive crab Cronius ruber (Brachyura, Portunidae) on the Canary Islands (central eastern Atlantic). Spain. Reg. Stud. Mar. Sci. (Submitted to).
References
Anderson M. J., Gorley R. N., Clarke K. R. (2008). PERMANOVA+ for PRIMER: Guide to Software and Statistical Methods (Plymouth, UK: PRIMER-E).
Anger K. (2006). Contributions of larval biology to crustacean research: a review. Invertebr. Reprod. Dev. 49, 175–205. doi: 10.1080/07924259.2006.9652207
Arístegui J., Barton E. D., Álvarez-Salgado X. A., Santos A. M. P., Figueiras F. G., Kifani S., et al. (2009). Sub-regional ecosystem variability in the Canary Current upwelling. Prog. Oceanogr. 83, 33–48. doi: 10.1016/j.pocean.2009.07.031
Arístegui J., Hernández-León S., Montero M. F., Gómez M. (2001). The seasonal planktonic cycle in coastal waters of the Canary Islands. Sci. Mar. 65, 51–58. doi: 10.3989/scimar.2001.65s151
Barton E. D., Arístegui J., Tett P., Canton M., García-Braun J., Hernández-León S., et al. (1998). The transition zone of the Canary Current upwelling region. Prog. Oceanogr. 41, 455–504. doi: 10.1016/S0079-6611(98)00023-8
Bashevkin S. M., Christy J. H., Morgan S. G. (2020). Adaptive specialization and constraint in morphological defenses of planktonic larvae. Funct. Ecol. 34, 217–228. doi: 10.1111/1365-2435.13464
Brandão M. C., Freire A. S., Burton R. S. (2016). Estimating diversity of crabs (Decapoda: Brachyura) in a no-take marine protected area of the SW Atlantic coast through DNA barcoding of larvae. Syst. Biodivers. 14, 288–302. doi: 10.1080/14772000.2016.1140245
Brito A., Falcón J. M., Aguilar N., Pascual P. (2001). “Fauna vertebrada marina,” in Naturaleza de las Islas Canarias: Ecología y, Conservación. Eds. Fernández-Palacios J. M., Martín-Esquivel J. L. (Turquesa, Santa Cruz de Tenerife), pp 219–pp 229.
Clarke K. R., Gorley R. N., Somerfield P. J., Warwick R. M. (2014). Change in marine communities: an approach to statistical analysis and interpretation. 3rd edition (Plymouth: PRIMER-E).
Clavel-Henry M., Solé J., Bahamon N., Carretón M., Company J. B. (2021). Larval transport of Aristeus antennatus shrimp (Crustacea: Decapoda: Dendrobranchiata: Aristeidae) near the Palamós submarine canyon (NW Mediterranean Sea) linked to the North Balearic Front. Prog. Oceanogr. 192, 102515. doi: 10.1016/j.pocean.2021.102515
Clemente S., Hernández J. C., Brito A. (2009). Evidence of the top–down role of predators in structuring sublittoral rocky-reef communities in a Marine Protected Area and nearby areas of the Canary Islands. ICES J. Mar. Sci. 66, 64–71. doi: 10.1093/icesjms/fsn176
Couret M., Landeira J. M., Santana del Pino Á., Hernández-León S. (2023a). A 50-year, (1971–2021) mesozooplankton biomass data collection in the Canary Current System: Base line, gaps, trends, and future prospect. Prog. Oceanogr. 216, 103073. doi: 10.1016/j.pocean.2023.103073
Couret M., Landeira J. M., Tuset V. M., Sarmiento-Lezcano A. N., Vélez-Belchí P., Hernández-León S. (2023b). Mesozooplankton size structure in the Canary Current System. Mar. Environ. Res. 188, 105976. doi: 10.1016/j.marenvres.2023.105976
Crandall K. A., Fitzpatrick J. F. J. (1996). Crayfish molecular systematics: using a combination of procedures to estimate phylogeny. Syst. Biol. 45, 1–26. doi: 10.1093/sysbio/45.1.1
Estoup A. C. R. L., Largiader C. R., Perrot E., Chourrout D. (1996). Rapid one-tube DNA extraction for reliable PCR detection of fish polymorphic markers and transgenes. Mol. Mar. Biol. Biotech. 5, 295–298.
Félix-Hackradt F. C., Hackradt C. W., Treviño-Otón J., Pérez-Ruzafa A., García-Charton J. A. (2013). Temporal patterns of settlement, recruitment and post-settlement losses in a rocky reef fish assemblage in the South-Western Mediterranean Sea. Mar. Biol. 160, 2337–2352. doi: 10.1007/s00227-013-2228-2
Fowler A. E., McLay C. L. (2013). Early stages of a new zealand invasion by charybdis japonica () (Brachyura: Portunidae) from asia: Population demography. J. Crust. Biol. 33, 224–234. doi: 10.1163/1937240X-00002127
García-Sanz S., Navarro P. G., Landeira J. M., Tuya F. (2014). Colonization patterns of decapods into artificial collectors: seasonality between habitat patches. J. Crustac. Biol. 34, 431–441. doi: 10.1163/1937240X-00002242
Giakoumi S., Pey A. (2017). Assessing the effects of marine protected areas on biological invasions: A global review. Front. Mar. Sci. 4. doi: 10.3389/fmars.2017.00049
González J. A. (2016). Brachyuran crabs (Crustacea: Decapoda) from the Canary Islands (eastern Atlantic): checklist, zoogeographic considerations and conservation. Sci. Mar. 80, 89–102. doi: 10.3989/scimar.04350.10A
González J. A. (2018). Checklists of Crustacea Decapoda from the Canary and Cape Verde Islands, with an assessment of Macaronesian and Cape Verde biogeographic marine ecoregions. Zootaxa 4413, 401–448. doi: 10.11646/zootaxa.4413.3.1
González J. A., Triay-Portella R., Escribano A., Cuesta J. A. (2017). Northernmost record of the pantropical portunid crab Cronius ruber in the eastern Atlantic (Canary Islands): natural range extension or human-mediated introduction? Sci. Mar. 81, 81–89. doi: 10.3989/scimar.04551.17B
González-Delgado S., López C., Brito A., Clemente S. (2018). Marine community effects of two colonial zoanthids in intertidal habitats of the Canary Islands. Reg. Stud. Mar. Sci. 23, 23–31. doi: 10.1016/j.rsma.2018.03.006
González-Gordillo J., Dos Santos A., Rodríguez A. (2001). Checklist and annotated bibliography of decapod crustacean larvae from the Southwestern European coast (Gibraltar Strait area). Sci. Mar. 65, 275–305. doi: 10.3989/scimar.2001.65n4
González-Gordillo J. I., Rodríguez A. (2003). Comparative seasonal and spatial distribution of decapod larvae assemblages in three coastal zones off the south-western Iberian Peninsula. Acta Oecol. 24, S219–S233. doi: 10.1016/S1146-609X(03)00032-8
González-Ortegón E., Berger S., Encarnação J., Chairi H., Morais P., Teodósio M. A., et al. (2022). Free pass through the Pillars of Hercules? Genetic and historical insights into the recent expansion of the Atlantic blue crab Callinectes sapidus to the West and the East of the Strait of Gibraltar. Front. Mar. Sci. 9. doi: 10.3389/fmars.2022.918026
Guerra-Marrero A., Bonino-Pérez A., Espino-Ruano A., Couce-Montero L., Jiménez-Alvarado D., Castro J. J. (2023). Life history parameters and fishing aspects of the alien nimble spray crab percnon gibbesi in a native area of the central-east atlantic. Animals 13, 1427. doi: 10.3390/ani13081427
Herrera A., Landeira J. M., Tuya F., Packard T., Espino F., Gómez M. (2014). Seasonal variability of suprabenthic crustaceans associated with Cymodocea nodosa seagrass meadows off Gran Canaria (eastern Atlantic). Cont. Shelf Res. 88, 1–10. doi: 10.1016/j.csr.2014.06.014
Herter H., Eckert G. L. (2008). Transport of Dungeness crab Cancer magister megalopae into Glacier Bay, Alaska. Mar. Ecol. Prog. Ser. 372, 181–194. doi: 10.3354/meps07667
Hjort J. (1914). Fluctuations in the great fisheries of northern Europe viewed in light of biological research. Rapp. P-V Réun. Cons. Int. Expl. Mer. 20, 1–288.
Ingle R. W. (1992). Larval Stages of Northeastern Atlantic Crabs (London: An Illustrated Key. Chapman & Hall). 363 pp.
Jeffs A., Tolimieri N., Montgomery J. C. (2003). Crabs on cue for the coast: The use of underwater sound for orientation by pelagic crab stages. Mar. Freshw. Res. 54, 841–845. doi: 10.1071/MF03007
Johnston D. J., Yeoh D. E. (2021). Temperature drives spatial and temporal variation in the reproductive biology of the blue swimmer crab Portunus armatus A. Milne-Edwards 1861 (Decapoda: Brachyura: Portunidae). J. Crustac. Biol. 41, ruab032. doi: 10.1093/jcbiol/ruab032
Jones E. V., Macintosh D., Stead S., Gray T. (2017). How effective are MPAs in conserving crab stocks? A comparison of fisheries and conservation objectives in three coastal MPAs in Thailand. Ocean Coast. Manage. 149, 186–197. doi: 10.1016/j.ocecoaman.2017.09.012
Kilpatrick K. A., Podestá G., Walsh S., Williams E., Halliwell V., Szczodrak M., et al. (2015). A decade of sea surface temperature from MODIS. Remote Sens. Environ. 165, 27–41. doi: 10.1016/j.rse.2015.04.023
Landeira J. M., Brochier T., Mason E., Lozano-Soldevilla F., Hernández-León S., Barton E. D. (2017). Transport pathways of decapod larvae under intense mesoscale activity in the Canary-African coastal transition zone: implications for population connectivity. Sci. Mar. 81, 299–315. doi: 10.3989/scimar.2017.81n3
Landeira J. M., Lozano-Soldevilla F. (2018). Seasonality of planktonic crustacean decapod larvae in the subtropical waters of Gran Canaria Island, NE Atlantic. Sci. Mar. 82, 119–134. doi: 10.3989/scimar.04683.08A
Landeira J. M., Lozano-Soldevilla F., Hernández-León S. (2013). Temporal and alongshore distribution of decapod larvae in the oceanic island of Gran Canaria (NW Africa). J. Plankton Res. 35, 309–322. doi: 10.1093/plankt/fbs089
Landeira J. M., Lozano-soldevilla F., Hernández-León S., Barton E. D. (2009). Horizontal distribution of invertebrate larvae around the oceanic island of Gran Canaria: the effect of mesoscale variability. Sci. Mar. 73, 761–771. doi: 10.3989/scimar.2009.73n4
Landeira J. M., Lozano-Soldevilla F., Hernández-León S., Barton E. D. (2010). Spatial variability of planktonic invertebrate larvae in the Canary Islands area. J. Mar. Biol. Assoc. UK 90, 1217–1225. doi: 10.1017/S0025315409990750
Landeira J. M., Lozano-Soldevilla F., Barton E. D. (2012). Mesoscale advection of upogebia pusilla larvae through an upwelling filament in the canaries coastal transition zone (CTZ). Helgol. Mar. Res. 66, 537–544. doi: 10.1007/s10152-011-0289-5
Lecaillon G. (2004). The “C.A.R.E.” (collect by artificial reef eco-friendly) system as a method of producing farmed marine animals for the aquarium market: An alternative solution to collection in the wild. SPC Live Reef Fish Inf. Bull. 12, 17–20.
Levine J. M., D’Antonio C. M. (1999). Elton revisited: a review of evidence linking diversity and invasibility. Oikos 87, 15–26. doi: 10.2307/3546992
Lindley J. A., Beaugrand G., Luczak C., Dewarumez J.-M., Kirby R. R. (2010). Warm-water decapods and the trophic amplification of climate in the North Sea. Biol. Lett. 6, 773–776. doi: 10.1098/rsbl.2010.0394
López C., Poladura A., Hernández J. C., Martín L., Concepción L., Sangil C., et al. (2012). Contrasting effects of protection from harvesting in populations of two limpet species in a recently established marine protected area. Sci. Mar. 76, 799–807. doi: 10.3989/scimar.0.0n0
López C., Poladura A., Hernández J. C., Martín L., Concepción L., Sangil C., et al. (2012). Contrasting effects of protection from harvesting in populations of two limpet species in a recently established marine protected area. Sci. Mar. 74, 799–807. doi: 10.3989/scimar.03601.15
Mantelatto F. L., Robles R., Schubart C. D., Felder D. L. (2009). “Molecular phylogeny of the genus Cronius Stimpson 1860, with reassignment of C. tumidulus and several American species of Portunus to the genus Achelous de Haan 1833(Brachyura: portunidae),” in Decapod Crustacean Phylogenetics. Crustac, vol. 18 . Eds. Martin J. W., Crandall K. A., Felder D. L., 567–579.
Mantelatto F. L., Terossi M., Negri M., Buranelli R. C., Robles R., Magalhães T., et al. (2018). DNA sequence database as a tool to identify decapod crustaceans on the São Paulo coastline. Mitochondrial DNA A DNA Mapp. Seq. Anal. 29, 805–815. doi: 10.1080/24701394.2017.1365848
Marco-Herrero E. (2015). Aplicación de técnicas morfológicas y moleculares en la identificación de la megalopa de decápodos braquiuros de la Península Ibérica (Universidad de Valencia). 143 pp.
Marco-Herrero E., Cuesta J. A., González-Gordillo J. I. (2021). DNA barcoding allows identifcation of undescribed crab megalopas from the open sea. Sci. Rep. 11, 1–19. doi: 10.1038/s41598-021-99486-4
Marco-Herrero E., Drake P., Cuesta J. A. (2018). Larval morphology and DNA barcodes as valuable tools in early detection of marine invaders: a new pea crab found in European waters. J. Mar. Biol. Assoc. 98, 1675–1683. doi: 10.1017/S0025315417000996
McLeod L. E., Costello M. J. (2017). Light traps for sampling marine biodiversity. Helgol. Mar. Res. 71, 1–8. doi: 10.1186/s10152-017-0483-1
Miller J. A., Shanks A. L. (2004). Ocean-estuary coupling in the Oregon upwelling region: Abundance and transport of juvenile fish and of crab megalopae. Mar. Ecol. Prog. Ser. 271, 267–279. doi: 10.3354/meps271267
Moreno-Borges S., Mendoza J. C., Martorell D., Montañes S., Rodríguez A. (2024). Spatio-temporal patterns in the inshore plankton community of the Canary Islands. In: Herrera R., González J., Ayza O., Moro L. (eds). Global Change in the Macaronesian Region. Gobierno de Canarias and GESPLAN S.A.U.
Mumby P. J., Harborne A. R., Brumbaugh D. R. (2011). Grouper as a natural biocontrol of invasive lionfish. PLoS One 6, e21510. doi: 10.1371/journal.pone.0021510
Oliphant A., Thatje S. (2021). Variable shrimp in variable environments: reproductive investment within Palaemon varians. Hydrobiologia 848, 469–484. doi: 10.1007/s10750-020-04455-z
Pan M., Pierce G. J., Cunningham C. O., Hay S. J. (2011). Seasonal and interannual variation of decapod larval abundance from two coastal locations in Scotland, UK. J. Mar. Biol. Assoc. UK 91, 1443–1451. doi: 10.1017/S0025315411000191
Pires R. F. T., Peliz Á., Pan M. (2020). “There and back again” – How decapod megalopae find the way home: A modelling exercise for Pachygrapsus marmoratus. Prog. Oceanogr. 184, 102331. doi: 10.1016/j.pocean.2020.102331
Porter S. S., Eckert G. L., Byron C. J., Fisher J. L. (2008). Comparison of light traps and plankton tows for sampling brachyuran crab larvae in an Alaskan fjord. J. Crust. Biol. 28, 175–179. doi: 10.1651/06-2818R.1
QGIS Development Team (2022). QGIS Geographic Information System (Open Source Geospatial Foundation). Available at: https://www.qgis.org/es/site/.
Reyns N., Sponaugle S. (1999). Patterns and processes of brachyuran crab settlement to Caribbean coral reefs. Mar. Ecol. Prog. Ser. 185, 155–170. doi: 10.3354/meps185155
Sanabria-Fernandez J. A., Alday J. G., Lazzari N., Riera R., Becerro M. A. (2019). Marine protected areas are more effective but less reliable in protecting fish biomass than fish diversity. Mar. pollut. Bull. 143, 24–32. doi: 10.1016/j.marpolbul.2019.04.015
Sangil C., Clemente S., Martín-García L., Hernández J. C. (2012b). No-take areas as an effective tool to restore urchin barrens on subtropical rocky reefs. Estuar. Coast. Shelf Sci. 112, 207–215. doi: 10.1016/j.ecss.2012.07.025
Sangil C., Sansón M., Afonso-Carrillo J., Herrera R., Rodríguez A., Martín-García L., et al. (2012a). Changes in subtidal assemblages in a scenario of warming: Proliferations of ephemeral benthic algae in the Canary Islands (eastern Atlantic Ocean). Mar. Environ. Res. 77, 120–128. doi: 10.1016/j.marenvres.2012.03.004
Sangrà P., Auladell M., Marrero-Díaz A., Pelegrí J. L., Fraile-Nuez E., Rodríguez-Santana A., et al. (2007). On the nature of oceanic eddies shed by the Island of Gran Canaria. Deep-Sea Res. I 54, 687–709. doi: 10.1016/j.dsr.2007.02.004
Schmoker C., Arístegui J., Hernández-León S. (2012). Planktonic biomass variability during a late winter bloom in the subtropical waters off the Canary Islands. J. Mar. Syst. 95, 24–31. doi: 10.1016/j.jmarsys.2012.01.008
Schubart C. D. (2011). Reconstruction of phylogenetic relationship within Grapsidae (Crustacea: Brachyura) and comparison of trans-isthmian versus amphi-atlantic gene flow based on mtDNA. Zool. Anz. 250, 472–478. doi: 10.1016/j.jcz.2011.06.003
Schubart C. D., Cuesta J. A., Felder D. L. (2002). Glyptograpsidae, a new brachyuran family from Central America: larval and adult morphology, and a molecular phylogeny of the Grapsoidea. J. Crust. Biol. 22, 28–44. doi: 10.1163/20021975-99990206
Schubart C. D., Huber M. G. J. (2006). Genetic comparisons of German populations of the stone crayfish, Austropotamobius torrentium (Crustacea: Astacidae). Bull. Fr. pêche piscic. 380–381, 1019–1028. doi: 10.1051/kmae:2006008
Schubart C. D., Reuschel S. (2009). Proposal for a new classification of Portunoidea and Cancroidea (Brachyura: Heterotremata) based on two independent molecular phylogenies. Crustacean issues: decapod crustacean Phylogenet. 18, 533–549.
Sigurdsson G. M., Morse B., Rochette R. (2014). Light traps as a tool to sample pelagic larvae of American lobster (Homarus americanus). J. Crustac. Biol. 34, 182–188. doi: 10.1163/1937240X-00002219
Stübner E. I., Søreide J. E., Reigstad M., Marquardt M., Blachowiak-Samolyk K. (2016). Year-round meroplankton dynamics in high-Arctic Svalbard. J. Plankton Res. 38, 522–536. doi: 10.1093/plankt/fbv124
Torres A. P., Dos Santos A., Cuesta J. A., Carbonell A., Massutí E., Alemany F., et al. (2012). First record of Palaemon macrodactylus Rathbun 1902 (Decapoda, Palaemonidae) in the Western Mediterranean. Mediterr. Mar. Sci. 13, 278–282. doi: 10.1163/1568540041181466
Tuya F., Boyra A., Sánchez-Jerez P., Barbera C., Haroun R. (2004). Relationships between rocky-reef fish assemblages, the sea urchin Diadema antillarum and macroalgae throughout the Canarian Archipelago. Mar. Ecol. Progr. Ser. 278, 157–169. doi: 10.3354/meps278157
Keywords: megalopa, light trap, phenology, recruitment, protection, Canary Islands
Citation: Landeira JM, Fatira E, Cuesta JA, Schubart CD, Moreno-Borges S and Rodríguez A (2024) Larval dynamics suggest phenological strategies and positive effect of marine protected areas controlling indigenous and non-indigenous crab populations. Front. Mar. Sci. 11:1371782. doi: 10.3389/fmars.2024.1371782
Received: 17 January 2024; Accepted: 10 April 2024;
Published: 25 April 2024.
Edited by:
Rachael Peart, National Institute of Water and Atmospheric Research (NIWA), New ZealandReviewed by:
Ajit Kumar Mohanty, Indira Gandhi Centre for Atomic Research (IGCAR), IndiaHenrique Queiroga, University of Aveiro, Portugal
Copyright © 2024 Landeira, Fatira, Cuesta, Schubart, Moreno-Borges and Rodríguez. This is an open-access article distributed under the terms of the Creative Commons Attribution License (CC BY). The use, distribution or reproduction in other forums is permitted, provided the original author(s) and the copyright owner(s) are credited and that the original publication in this journal is cited, in accordance with accepted academic practice. No use, distribution or reproduction is permitted which does not comply with these terms.
*Correspondence: José M. Landeira, am9zZS5sYW5kZWlyYUB1bHBnYy5lcw==
†Deceased