- 1Department of Marine Zoology, Senckenberg Research Institute and Natural History Museum, Frankfurt, Germany
- 2Departamento de Estratigrafía y Paleontología, Universidad de Granada, Granada, Spain
- 3Department of Primary Industry, New South Wales Department of Primary Industries, Huskisson, NSW, Australia
- 4Faculty of Geography and Geology, Institute of Geological Sciences, Jagiellonian University, Kraków, Poland
- 5Department of Ocean BioGeosciences, National Oceanography Centre, Southampton, United Kingdom
- 6Department of Biological Sciences, Institute of Ecology, Evolution and Diversity, Johann Wolfgang Goethe University Frankfurt, Frankfurt am Main, Germany
Taxonomy is a dynamic and evolving scientific field in biological and paleontological research, which describes and systematises species and other taxa and thus lays the foundation to solve and structure biological questions. The study of past (fossil) and modern (present) seafloor traces holds huge potential for understanding the composition, behavioural ecology, and ecosystem impact of benthic communities, e.g., in terms of bioturbation and carbon cycling. In trace fossil research (paleoichnology), ichnotaxonomical analysis is based on the application of ichnotaxobases, morphology being the most important and useful criterion. However, marine neoichnological analyses (modern traces; lebensspuren) have no standardised ichnotaxobases. In the literature, classification based on morphological descriptions or behavioural assignations can be found, but without consensus. This lack of standardised terminology hinders comparisons among studies and regions. Through this manuscript, we discuss the limitations of marine lebensspuren characterisation through underwater observations and how further classification could be done (i.e., possible ichnotaxobases). Considering that most of the classifications will be based on marine still images, we propose a classification for marine lebensspuren based on a combination of three labels: 1) morphology (e.g., M-ridged trail), 2) behaviour determination (e.g., locomotion and feeding), and 3) tracemaker taxonomy (e.g., Echinocrepis rostrata). Accordingly, the classification will be 1) M-ridged trail 2) Locomotion and feeding 3) Echinocrepsis rostrata. A set of recommendations is given to address all three labels. Also, we describe some of the contrasting positions of paleo- and neoichnology, particularly where a solution presently seems difficult to find, and argue that open nomenclature could be useful as still images contain large amounts of information. Finally, we provide examples of the usage of this classification for input to a marine lebensspuren online repository and how this nomenclature can help to compare worldwide lebensspuren in a consistent way.
Introduction
Biologists and paleontologists seeking to understand the taxonomy, evolution and ecology of organisms have benefited from naming conventions (Patterson et al., 2010). By providing science-based names and classifications of organisms, researchers deliver globally consistent baseline information on the biosphere, crucially important to understand and interpret life and nature as a whole (Vences, 2020). Winston (2018) described the role of naming conventions in biology: “Nomenclature and taxonomy are complementary and distinct aspects of the study of biodiversity …. Taxonomy is the part of the science of systematics that deals with identifying, describing, and categorising organisms from species to higher taxa. Nomenclature is a system of giving names to organisms based on rules established for the process” [p.1122] (Winston, 2018). Codified rules for the names of plants and animals have been included and recently summarise in the International Code of Botanical Nomenclature and in the International Code of Zoological Nomenclature (ICZN), respectively (Ride, 1999). Moreover, the set of rules and recommendations that govern the ICZN is applicable to both living and extinct organism remains (e.g., fossils).
Principles governing the ICZN have been also applied to classifying trace fossils, the record of activities of past organisms imprinted in, on, or within the sediment/substrate, forming the basis of ichnotaxonomy (see Bertling et al., 2006, Bertling et al., 2022). Biological taxonomy employs meaningful morphological and molecular characteristics in the identification and description of organisms, while paleoichnology (i.e., the study of trace fossils) similarly uses specific morphological criteria for identifying, describing, and categorising trace fossils (Bromley, 2012). Which of these morphological criteria are selected as meaningful ichnotaxobases has been controversial among ichnologists (Bertling, 2007; Rindsberg, 2012; Bertling et al., 2022). During the beginning of ichnotaxonomy, the tracemaker taxonomy was the accepted standard of the field (Rindsberg, 2018). Trace fossil-associated features such as stratigraphic age, geographical location, and facies have been disregarded as suitable options (Bertling et al., 2006). A currently-favoured and accepted approach allows only the most important and useful morphological criteria, resulting from the behaviour of tracemakers, to be considered as an ichnotaxobase and the main types of substrate for the case of bioerosion traces (see Table 2 in Bertling et al., 2022). Hence, a classification scheme based on significant morphological features was proposed for trace fossils (Bertling et al., 2006; Knaust, 2012; Bertling et al., 2022). In parallel, particular ichnotaxobases were presented in order to assist in naming bioerosion trace fossils in bones (Pirrone et al., 2014). Despite these considerable advances towards a unified approach, not all discussion points have been settled in ichnotaxonomy of trace fossils (Rindsberg, 2012). A conscious effort to focus only on trace fossil morphological details is needed. However, a purely morphological classification is precluded by morphology being commonly permeated by our interpretation of organism behaviour in trace creation (Buatois and Mángano, 2011), suggesting that additional factors should be included. It is precisely this debate that has brought substantial progress to ichnotaxonomy over the past years (Bertling et al., 2006; Knaust, 2012; Bertling et al., 2022).
The approaches to taxonomy and nomenclature are quite different for the modern counterpart to trace fossils, neoichnology, which deals with lebensspuren (German origin, meaning ‘traces of life’). The classification and naming of marine lebensspuren (i.e., traces on and within the seafloor) is in relative infancy when compared with trace fossils or biological species, lacking objective criteria that could be applied in a universal lebensspuren classification and nomenclature. However, some classification bases have been developed. The initial classification of marine faunal lebensspuren takes into account five inferred behavioural categories: resting, crawling, feeding, grazing, and dwelling (Figure 1; Seilacher, 1954). Later approaches also used general morphological features to classify lebensspuren captured in marine photographic studies (Figure 1) (Ewing and Davis, 1967; Heezen and Hollister, 1971; Hollister et al., 1975; Kitchell et al., 1978; Young et al., 1985; Bett et al., 1995). Both the interpreted organism behaviour and morphology have continued to be used, sometimes in combination (Dundas and Przeslawski, 2009; Przeslawski et al., 2012; Bell et al., 2013) (Figure 1), and numerous additions and modifications have been introduced to defined classifications as more observations have been made (e.g., the introduction of ‘waste cast’ as a behavioural category by Dundas and Przeslawski, 2009). Only Althaus et al. (2015), based on the descriptions of Przeslawski et al. (2012) descriptions, codified marine lebensspuren in a more coherent way. Although they sometimes referred to the trace fossil literature, these classification systems were largely based on morphology and behaviour, ultimately resulting in nomenclature inconsistent with that used in paleoichnology.
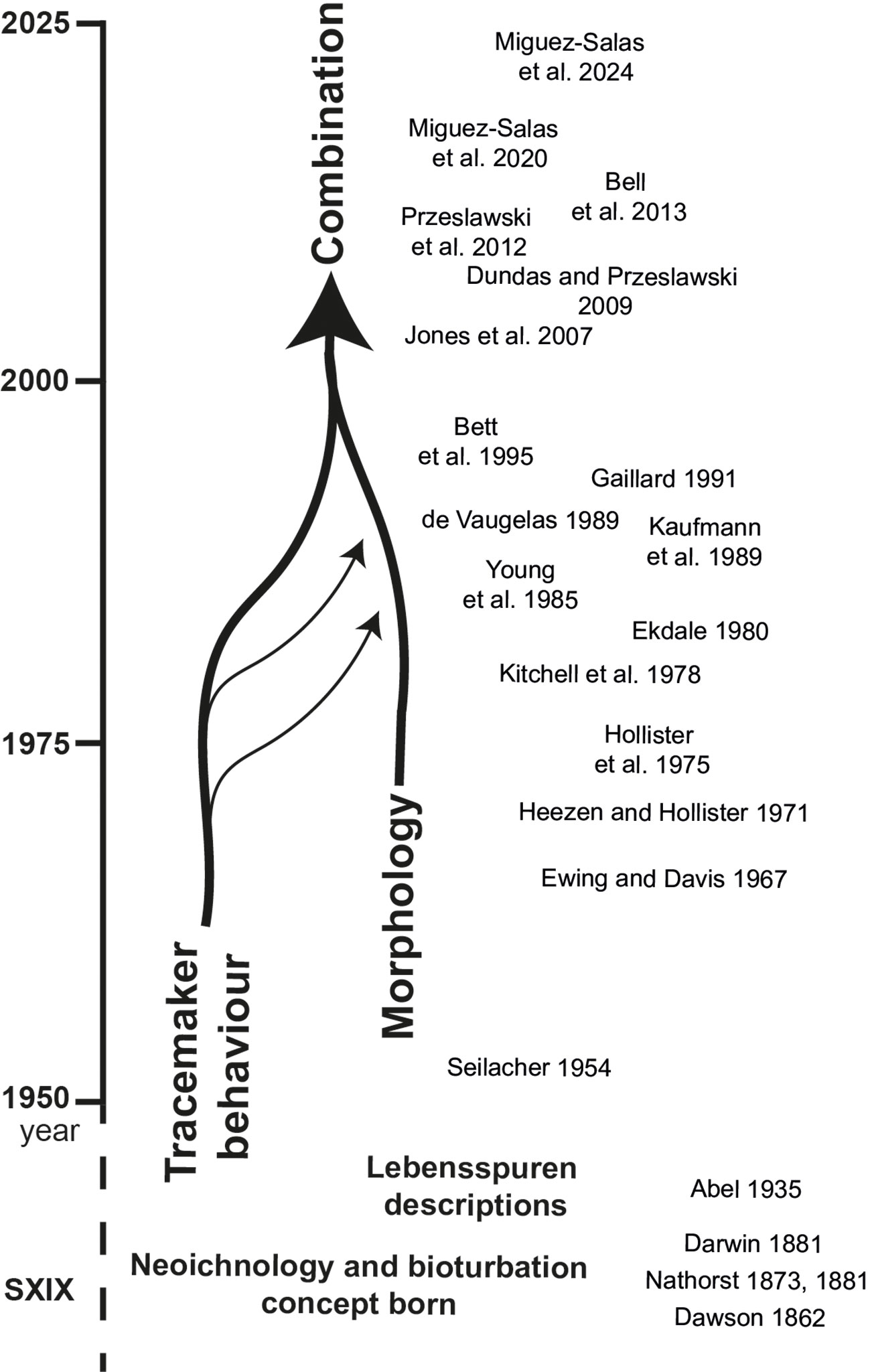
Figure 1 Time evolution of common lebensspuren classifications in the literature. References: (Dawson, 1862, Nathorst, 1873, Darwin, 1881, Nathorst, 1881; Abel, 1935; Seilacher, 1954; Ewing and Davis, 1967; Heezen and Hollister, 1971; Hollister et al., 1975; Kitchell et al., 1978; Ekdale, 1980; Young et al., 1985; De Vaugelas, 1989; Kaufmann et al., 1989; Gaillard, 1991; Bett et al., 1995; Jones et al., 2007; Dundas and Przeslawski, 2009; Przeslawski et al., 2012; Bell et al., 2013; Miguez-Salas et al., 2020).
This perspective paper evaluates the possibilities of establishing a systematic naming convention for marine lebensspuren based on the identification of traces in underwater images. We highlight the obstacles to a unified classification and review the important elements to nomenclature that have emerged as neoichnology and seafloor imaging have developed. Moreover, as recommended in previous studies (e.g., Przeslawski, 2022), we propose a standard framework for marine lebensspuren classification that combines the most important classification elements and will allow reliable worldwide lebensspuren comparisons. Finally, as we approach a consensus between ichnological and biological interpretation of modern seafloor traces, we describe some of the contrasting positions of the two disciplines, particularly where a solution currently seems out of reach. These unsolved issues and contrasting perspectives are reviewed here to provide a basis for future work to approach a consensus with yet additional insights available.
Marine lebensspuren identification: on the genesis of the problem
The origin of the major inconsistencies in marine lebensspuren classification lies mostly in the inherent difficulties of working in the marine environment. Inside the marine realm, most of the seafloor is out of reach for optical remote sensing. Thus, the exploration of these marine environments and the in-situ observation of tracemakers requires the expensive and time-consuming deployment of short-range observation gear, in many cases with inherent limitations, such as restricted fields of view in both spatial and temporal scales (Durden et al., 2016). Thus, many marine lebensspuren descriptions have been conducted in shallow environments (e.g., intertidal, supra tidal) where observations can be done outside the water, describing endobenthic lebensspuren (i.e., traces within the seafloor) (Gingras et al., 2008; Dashtgard and Gingras, 2012; La Croix et al., 2022).
Marine epibenthic lebensspuren (i.e., traces on the seafloor) are often observed in videos or still images captured by cameras on autonomous and remotely operated underwater vehicles transiting on or above the seafloor, or directly by divers on snorkel, SCUBA, or submersibles. However, these methods of observation, while popular and efficient, are two-dimensional and may result in some limitations to trace identification and classification (Durden et al., 2016; Purser et al., 2019). Many marine lebensspuren are endobenthic, extending inside the seafloor sediment, and can be mainly studied through resin casts (Seike et al., 2012; Seike, 2023) or sediment cores (Wetzel, 2008). These methods impose spatial limitations linked to the size of the samples (e.g., frame dimensions, resin cast size, core diameter/length, core volume), and temporal constraints associated with the time of observation. These two limiting factors are key when proceeding to an adequate marine lebensspuren classification, as in many cases we will not observe the full 3-dimensional extent and morphology of the traces, instead collecting only a snapshot observation without context for their state of degradation/formation. These limitations may be exacerbated by two additional factors: similar or morphologically indistinguishable lebensspuren can be produced by different organisms/species, and a single biological species can be responsible for a variety of different lebensspuren corresponding to different behavioural patterns or substrate properties.
A major drawback in lebensspuren classification is the taphonomic bias by the degradation of the traces, particularly epibenthic traces (Wheatcroft et al., 1989; Smith et al., 2005). Biotic and abiotic factors control lebensspuren degradation, being both complex dynamic and simultaneous processes that distort original morphology, leading to confusion and mischaracterisation of morphological parameters (Figure 2A). The relationship between biotic factors and lebensspuren degradation is not always obvious. For example, the diversity and composition of the benthic community, is spatially and temporally variable, impacting both trace creation and erasure (Wheatcroft et al., 1989). Among the abiotic degradation/preservation factors, sedimentation rate and seafloor hydrodynamic conditions (e.g., bottom currents) are most important. For example, high sedimentation rates or rapid burial by slight or non-destructive distal turbidites favour the preservation of specific lebensspuren (Seilacher, 1977; Gaillard, 1991), while high-energy bottom-current conditions induce the erosion of the unconsolidated surfaces, deleting most of the lebensspuren (Kaufmann et al., 1989; Miguez-Salas et al., 2020). However, even in low-disturbance environments, abiotic factors eventually ensure that lebensspuren do not persist over time, especially in the case of faecal casts (i.e., degradation occurs in as little as 48h; Smith et al. (2005); Figures 2B, C). Hence, lebensspuren classification based on morphology must consider the potential bias from degradation, particularly in underwater studies.
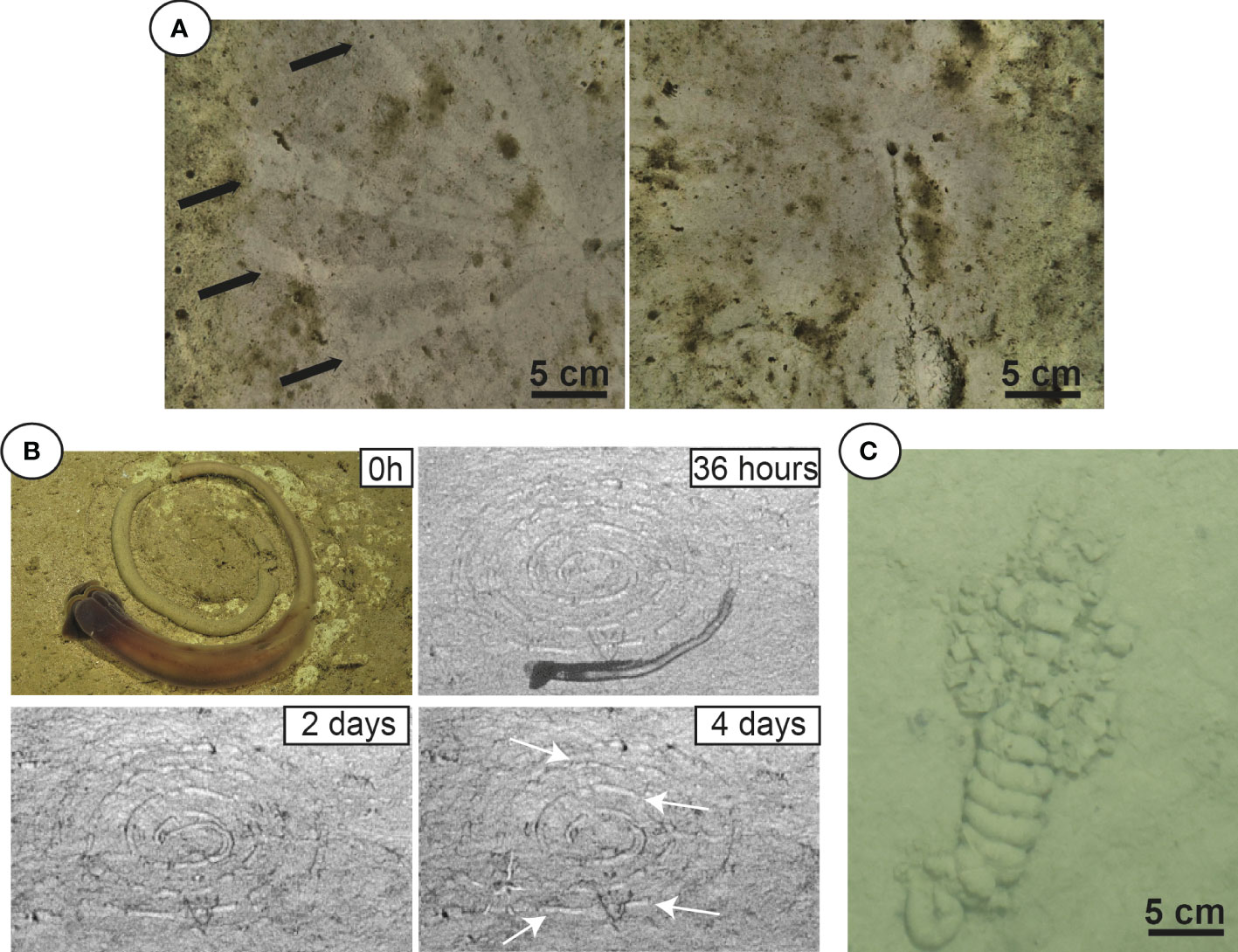
Figure 2 Examples of how degradation processes affect lebensspuren morphology. (A) Rosette lebensspuren with low degradation where the spokes can be clearly identified (black arrows; left) and rosette-shape lebensspuren with high degradation where spoke shape is nearly missed [Porcupine Abyssal Plain; (Miguez-Salas et al., 2023a)]. (B) Top left the enteropneust tracemaker; then, sequential example of photographs on the seafloor at Station M (4100 m depth) with the evolution of a spiral faecal cast and the elapsed time until the spiral is nearly gone [courtesy of (K) Smith (MBARI) (Smith et al., 2005)]. Note that at the end (4 days) the lebensspuren can be easily misclassified as smooth straight faecal cast (white arrows). (C) Coil and tailed faecal cast produced by the sea cucumber Psychropotes indet. with different stages of degradation. Note the difference in degradation even within the same lebensspuren.
A morphoethological approach
In an evaluation of ichnotaxobases, Bertling et al. (2006) concluded that “the taxonomic treatment of trace fossils needs a uniform approach, independent of the ethologic groups concerned…., with morphology resulting as the most important criterion” [p.265]. But is it appropriate for morphology to be the main lebensspuren ichnotaxobase?
Marine lebensspuren differ from trace fossils in that it is sometimes possible to observe tracemaking, and thus identify the tracemakers and their behaviour (Heezen and Hollister, 1971; Miguez-Salas et al., 2022; Miguez-Salas et al., 2023a, Miguez-Salas et al., 2023b). Hence, tracemaker taxonomy and the type of behaviour responsible for the traces left behind by the producer should not be disregarded as an important criterion for lebensspuren classification. This information is not just useful from a classification perspective, but also an ecological one. For example, behavioural categories of lebensspuren could be used as a functional group in various ecological studies (Baucon et al., 2021). This valuable opportunity to gain taxonomic and ethological information should not be neglected, as different traces can be produced by the same organisms and vice versa. The integration of all available information and, the flexible application of all three ichnotaxobases may be the most powerful approach to classifying marine lebensspuren in imagery.
We propose that lebensspuren classification be conducted based on the integration of three features (Figure 3): 1) morphological characterisation, 2) behavioural information and 3) tracemaker taxonomy. The structure of the suggested classification is based on the observed availability of the three data types from seafloor still images. Full two-dimensional morphology is the most commonly available type of information. Behavioural classification is possible in a sub-set of observations in which the behaviour is observed or inferred, while tracemaker identification is only possible in a small fraction of imagery, in which the act of tracemaking can be directly observed and classified to the lowest taxonomic rank possible. Our approach allows for a trace characterisation even without direct information about the tracemaker. The integration of the complete set of characteristics (where available) provides the most comprehensive trace classification, and can also aid in the interpretation and classification of other, incompletely characterised, traces.
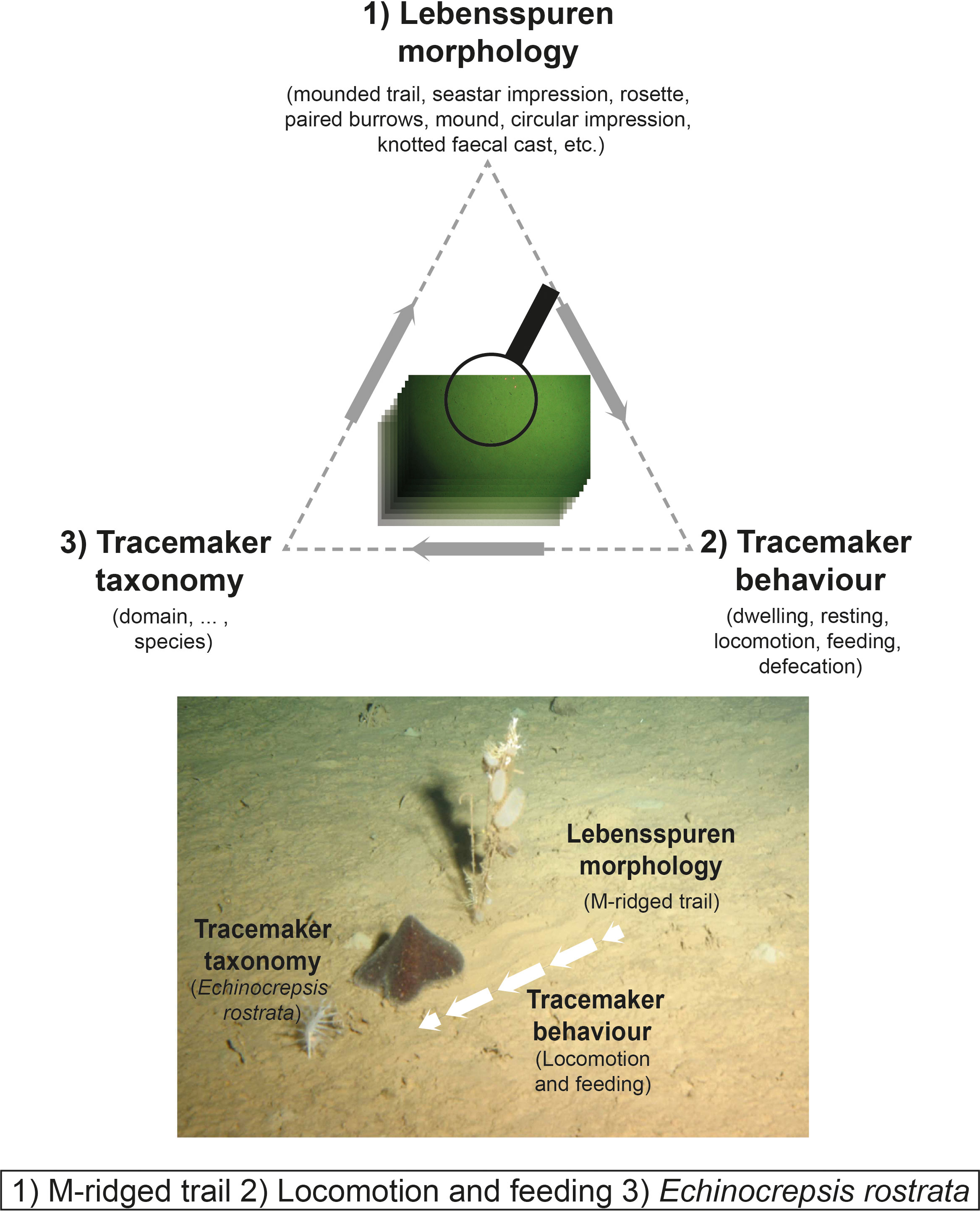
Figure 3 An example of the proposed morphoethological classification for marine lebensspuren based on three labels: 1) morphological characterisation, 2) behaviour determination, and 3) tracemaker taxonomy (image courtesy of Michael Vardaro).
We propose a morphoethological naming structure with three labels in the following sequence (Figures 4, 5):
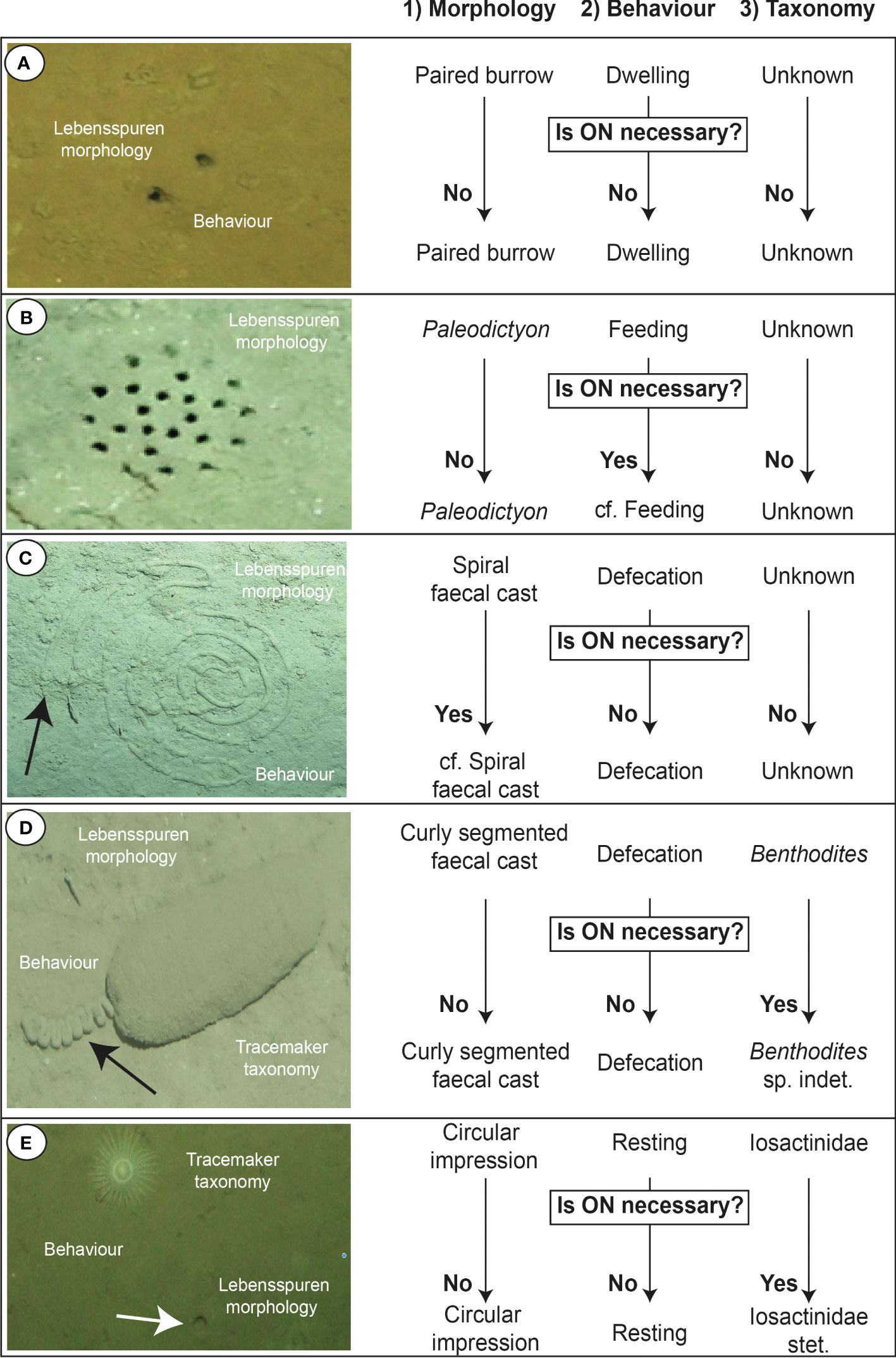
Figure 4 Examples of how to characterise marine lebensspuren based on the proposed morphoethological classification and how to apply open nomenclature (ON) if necessary (images from KuramBio 2012 and AleutBio 2022 expeditions). (A) 1) Paired burrow 2) Dwelling 3) Unknown; (B) 1) Paleodictyon 2) cf. feeding 3) Unknown (ON is necessary because this lebensspuren has been previously associated, but not conclusively, with a microbial farming feeding behaviour); (C) 1) cf. Spiral faecal cast 2) Defecation 3) Unknown (ON is necessary because the edge of the faecal cast seems to develop a switchback pattern instead of a spiral one); (D) 1) Curly segmented faecal cast 2) Defecation 3) Benthodytes sp. indet. (ON is necessary because it is not possible to identify the species of Benthodytes); (E) 1) Circular impression 2) Resting 3) Iosactinidae stet. (ON is necessary because it is not possible to continue with the taxonomic rank identification due to image resolution).
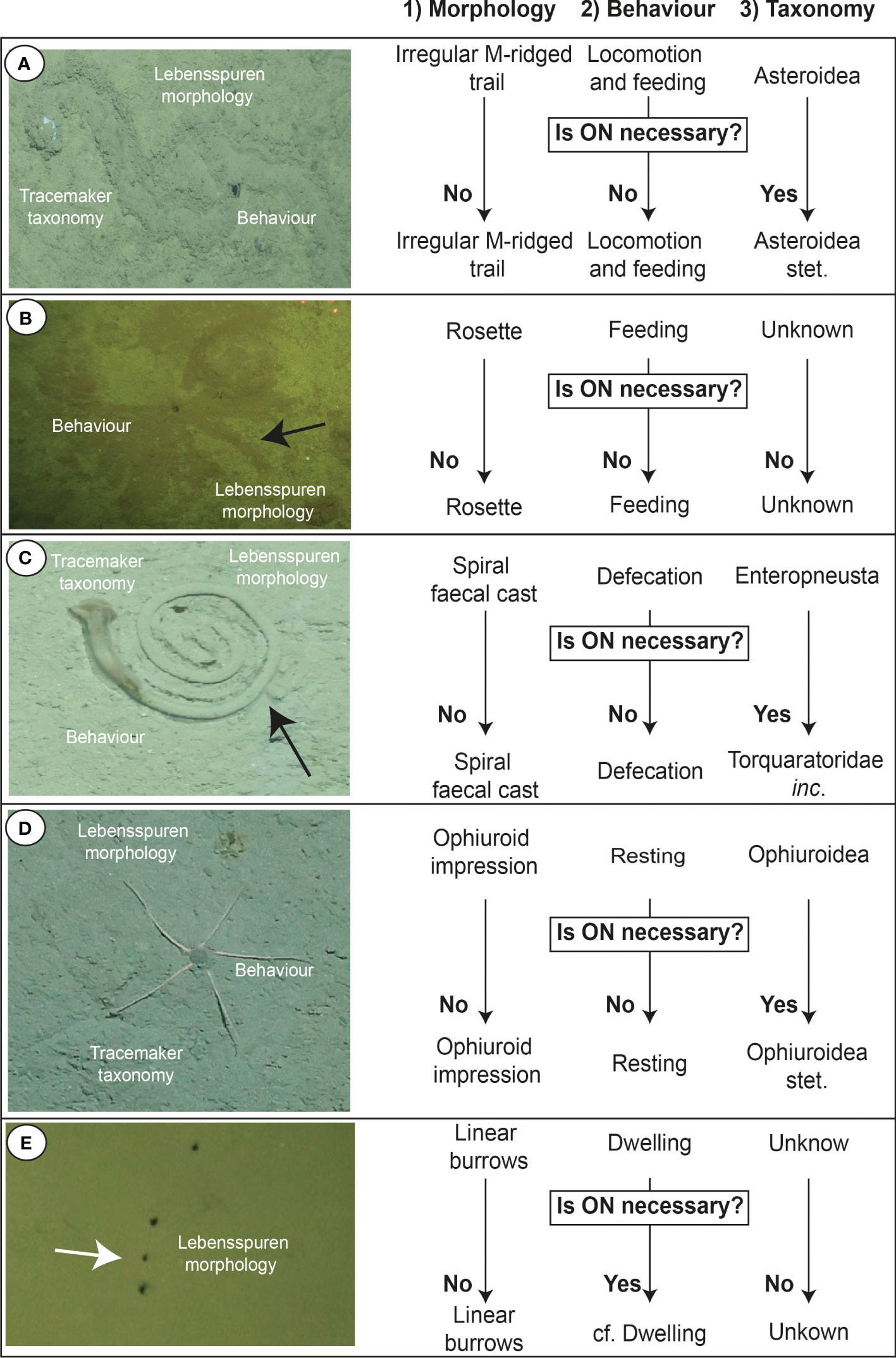
Figure 5 Examples of how to characterise marine lebensspuren based on the proposed morphoethological classification and how to apply open nomenclature (ON) if necessary (images from KuramBio 2012 and AleutBio 2022 expeditions). (A) 1) Irregular M-ridged trail 2) Locomotion and feeding 3) Asteroidea stet. (ON is necessary because it is not possible to continue with the taxonomic rank identification due to image resolution); (B) 1) Rosette 2) Feeding 3) Unknown; (C) 1) Spiral faecal cast 2) Defecation 3) Torquaratoridae inc. (ON is necessary because it is not possible to continue with the taxonomic rank identification due to image resolution); (D) 1) Ophiuroid impression 2) Resting 3) Ophiuroidea stet. (ON is necessary because it is not possible to continue with the taxonomic rank identification due to image resolution); (E) 1) Linear burrows 2) cf. dwelling 3) Unknown (ON is necessary because feeding behaviour cannot be discarded).
1) Morphological key information. This part provides the most important morphological features of the lebensspuren. It should be as concise as possible rather than a detailed description (e.g., without dimensional data), using previous morphological names where such exist (Ewing and Davis, 1967; Kitchell et al., 1978; Young et al., 1985; Dundas and Przeslawski, 2009; Przeslawski et al., 2012) (see the Supplementary Material: Lebensspuren Catalogue for a review of most common lebensspuren names). As general recommendations for new names: i) burrows should have some information about the overall distribution (e.g., single burrow; paired burrows; cluster burrows; circular burrow mesh); ii) impressions on the seafloor should have a word which characterises the shape (e.g., asteroid impression; ophiuroid impressions; rosette; circular crater); iii) faecal casts should have some information about the overall morphology (e.g., knotted faecal cast; rounded faecal cast; coiled faecal cast); and iv) trails should have some information about the epirelief morphology and ornamentation (e.g., mounded trail; W trail; punctuated trail; furrow trail). Also, since trace fossil ichnotaxonomy is based on morphology, in the rare case that their identification is unequivocal (e.g., Spiroraphe, Paleodictyon, Urohelminthoida (Ekdale, 1980; Gaillard, 1991), the trace fossil name should be used (Figure 4B). Thus here, we also underscore the statement of Bertling et al. (2006) (p. 269) that “the usage of existing and well-defined trace fossil names for modern structures is supported, whereas the establishment of ichnotaxa based on modern material is not”, with the ultimate aim of facilitating integration of ichnological knowledge gathered from the past and present.
The use of terms such as holes, perforated, striae among others should be avoided when it cannot be verified that the substrate is consolidated (Bromley, 1981). These terms should be only used for lebensspuren related stiff substrates and bioerosion (Bromley, 1981, Bromley, 2012; Pirrone et al., 2014). The use of size adjectives such as thick, thin, small or large may be useful with reservation. Ontogenetic changes of tracemaker size is naturally reflected in the scale of their traces (Mángano et al., 1997), but must not result in separate lebensspuren classification. Thus, the use of a single size parameter as a primary ichnotaxobase is not recommended; it should remain an auxiliary character (Bertling et al., 2022). However, morphometrics can be useful for further description but not constituents of the name. Only in those instances where statistical analysis shows a strong size difference (i.e., significantly different size classes within the same lebensspuren population) or where size variations are due to markedly different tracemakers, we recommend creating lebensspuren submorphotypes (see Figure 5 in Bertling et al., 2022). However, we suggest authors to diligently seek out additional criteria that may already exist.
2) Behavioural information. This part of the classification reflects the observed or inferred organism behaviour in producing the trace. After the initial five lebensspuren behavioural categories suggested by Seilacher (Seilacher, 1954), several more have been added to paleoichnological research, e.g., gardening, predation, equilibrium or escape among others (see Vallon et al., 2016 for an updated review). However, the recognition of some of them in still images (e.g., farming, equilibrium, predation, escaping) could be ambiguous and may lead to misclassifications. Thus, to make classification simpler and less susceptible to misinterpretation, in our view only the following behavioural categories should be considered (Table 1): i) resting lebensspuren (imprints of stationary animals); ii) locomotion lebensspuren (traces resulting from animals that move from one place to another); iii) feeding lebensspuren (feeding activities); iv) dwelling lebensspuren (permanent domiciles constructed by infaunal organisms); and v) defecation lebensspuren (faecal casts from the benthic fauna; usually classified as waste casts or wasting lebensspuren). Some marine lebensspuren could represent various behaviours and categories sometimes overlap (e.g., locomotion and feeding) (see Table 1), in which case both behaviours should be included in the ethological classification and dominant inferred behaviour should be indicated first (i.e., based on the inferred relative amount of time the organism spends on a certain behaviour for a given trace).
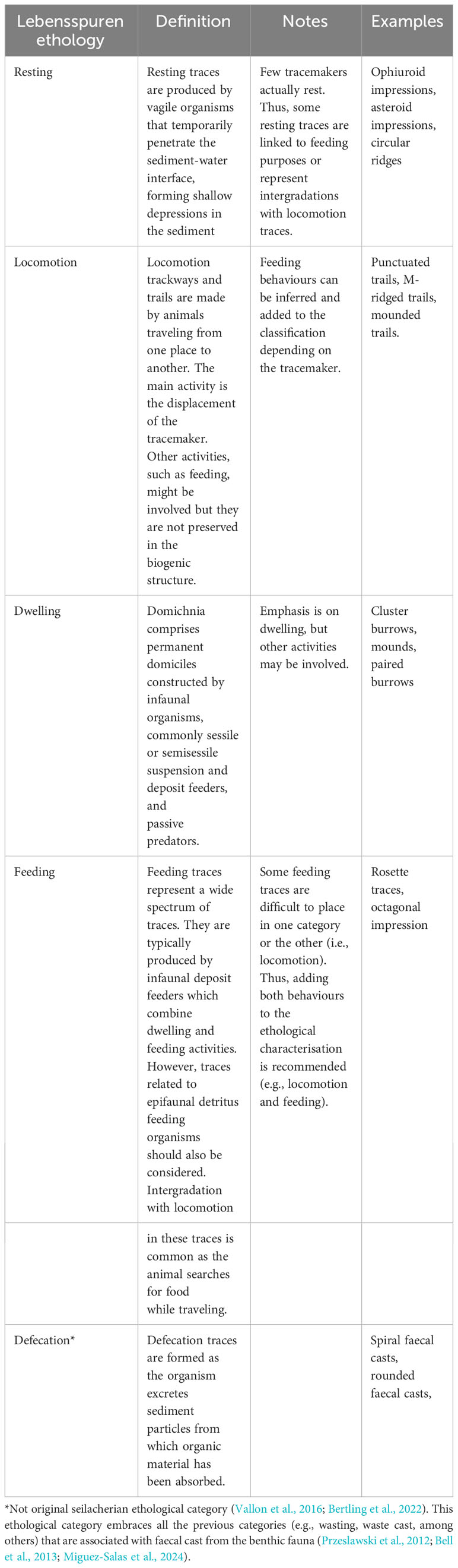
Table 1 Ethological classification of marine lebensspuren [based on (Seilacher, 1954; Frey, 1973; Buatois and Mángano, 2011)].
3) Tracemaker taxonomy follows biological classification (domain, kingdom, phylum, class, order, family, genus, species) (Figures 4, 5), to the lowest taxonomic rank possible. Since the same trace can be produced by multiple species and the same species can produce different traces, a specific three-labels combination can be added for each identified combination (e.g., 1) Flat trail 2) Locomotion and feeding 3) Molpadiodemas sp. and 1) Flat trail 2) Locomotion and feeding 3) Pseudostichopus cf.).
Open nomenclature
Uncertainties in classification may occur where any of the three labels may not be reliably determined (Figures 4, 5), so the use of open nomenclature (ON) may be useful to apply as it provides a means to explain this uncertainty during the identification (Bengtson, 1988; Sigovini et al., 2016; Horton et al., 2021). For the morphological and ethological labels, we suggest that the identifier uses the ON criteria proposed for paleontological research (Bengtson, 1988) which was later adapted for ichnotaxonomy (see Table 3 in Rindsberg, 2018 for a summary). For the tracemaker taxonomy, we suggest that the identifier uses the ON criteria proposed for image-based identification by Horton et al. (2021).
In terms of morphological classification, the added ON symbols would be: “cf.” (e.g., cf. Rosette; compare to Rosette, but incompletely known); and “aff.” (e.g., aff. Rosette; not Rosette, but an unnamed form with affinity to it) (see Figures 4, 5). In the case of the ethological label “cf.” will be used when the behaviour cannot be reliably inferred (cf. Dwelling; possibly dwelling but other behaviour can be possible as well) (Figures 4, 5). Additionally, since lebensspuren could represent various behaviours and categories could overlap, “cf.” should be added to each of the inferred categories that has not been clearly identify (e.g., Dwelling and cf. feeding, cf. locomotion and cf. feeding). The use of ON in the behaviour should be carefully considered by identifiers, reviewing inferred behaviours in the literature for similar marine lebensspuren. Finally, for the tracemaker taxonomy (Figures 4C–E, 5C, D), ON following the suggestions for image-based identifications by Horton et al. (2021) is highly recommended (see Horton et al., 2021 for a detailed explanation). When the tracemaker is absent in the still image, “Unknown” should be added (Figures 4A, B, 5B, E) unless the trace is known to be distinctive to a particular taxon (e.g., enteropneusts and spiral faecal casts).
Limitations
Two major challenges for the classification of lebensspuren in marine images are a lack of knowledge of the substrate consistency and lebensspuren state of degradation/formation. Image-based surveys are often used to study the deep sea where fine-scale knowledge of the seafloor’s physical (e.g., grain size, sediment consolidation) and faunal (e.g., microfauna) properties is often limited. It is known that substrate properties, such as grain size, shear strength or sediment consolidation, influence the life activity of benthic organisms and their traces. Usually, with increasing sediment consolidation, traces acquire additional morphological details (Uchman and Pervesler, 2006). Also, lebensspuren state of degradation/formation is strongly controlled by microbial degradation and microfauna which ultimately will affect the morphology (Figure 2). Thus, both factors will limit an appropriate lebensspuren description, inducing possible misclassifications. In these cases of uncertainty, observed traces may perhaps be addressed as different morphotypes within the same lebensspuren (Bett et al., 1995; Miguez-Salas et al., 2023c), but further considerations are needed before establishing morphotypes within the same lebensspuren category.
Size related lebensspuren categories (e.g., thin vs. thick trail or small mound vs. large mound), that have commonly been used in previous classifications and descriptions, should be applied cautiously (see above concerns). It is commonly observed that juvenile and adult tracemakers produce lebensspuren with different size, but their morphology usually remains the same. Thus, their classification should be based in these morphological features rather than size (i.e., overall shape, ornamentation, orientation). In most cases, observing the tracemaker is not possible, leading to numerous lebensspuren of varying sizes where it's uncertain if they belong to the same tracemaker in different ontogenetic phases. This means that the exclusion of size in the classification process poses a risk in itself. We strongly recommend using inferential statistics to test different size classes within the same lebensspuren and then creating submorphotypes if necessary. Nevertheless, as stated above, when possible, we suggest a morphological description in which size is not the anchor of that lebensspuren category.
Last but not least, in most of the lebensspuren that we observe, the tracemaker will be unknown (see Supplementary Material: Lebensspuren Catalogue for distinctive and common lebensspuren in which the tracemaker remains unknown). In future years, the number of core samples, high-quality marine images, and time-lapse data will increase, and consequently so will lebensspuren descriptions. Therefore, what today is a limitation when it comes to a full lebensspuren classification, in upcoming years these knowledge gaps will gradually decrease since there will be more chances to catch the tracemaker in the act (Miguez-Salas et al., 2022; Brandt et al., 2023; Miguez-Salas et al., 2023a). Classifications can then be retroactively expanded to include the third label (tracemaker taxonomy).
Further steps and considerations
The study of time-lapse data will be critical to clarify the uncertainties in lebensspuren marine classification. Time-lapse data will fill this knowledge gap as it provides the opportunity to observe the formation and degradation of lebensspuren as well as the specific associated behaviour. Only this way a deep understanding of the lebensspuren can be generated that is necessary to classify them in other scenarios where time-lapse data are not available. Nevertheless, the proposed morphoethological nomenclature offers the possibility to characterise marine lebensspuren from still images in a consistent manner. The absence of this consistency has hindered reliable diversity comparisons across surveys and among researchers. This underscores the significance of the proposed classification. Moreover, the three labels facilitate intuitive filtering and precise comparisons, proving invaluable when comparing lebensspuren assemblages globally.
The next logical step for the implementation of the proposed classification is the establishment of a curated open-access online repository which serves as a dynamic identification catalogue.. Unfortunately, there is currently no global repository for lebensspuren imagery, and images are instead scattered among existing institutional or regional repositories or inaccessably stored on researchers’ cloud storage or hard drives network or hard drives. In order to make imagery accessible and discoverable to all researchers, the community must decide on and actively use an appropriate platform to store, share, and annotate imagery using a standard classifications system such as that proposed here (Przeslawski et al., 2023). The ideal platform for this will have the following characteristics: 1) Allow any researcher to upload imagery and share it, 2) Require meta data for each image, including geographic coordinates and depth, 3) Provide an annotation tool for researchers to extract data from images, including classification of lebensspuren, 4) Allow experts to comment on and validate images of lebensspuren to identify new morphologies and match behaviours and tracemakers to a given trace, and 5) Contain a search function for the whole imagery collection so that annotated images of particular traces can be readily found. There are several online repositories and platforms that fulfil some of these characteristics (e.g. FathomNet, Ocean Biodiversity Information System, Squidle+, iNaturalist) but to our knowledge there are none that fulfil all of them. We plan to include the lebensspuren classification scheme described in this study into the next version of CATAMI, a broader marine imagery classification scheme (Althaus et al., 2015) which will in turn be integrated into the online image annotation platforms such as Squidle+ and Biigle. In parallel, following this new classification scheme, we will create a marine lebensspuren online repository in one of the aforementioned platforms (e.g., Fathomnet, iNaturalist) (Katija et al., 2022).
The creation of a three-label classification, incorporating behavior and morphology, serves the purpose of associating each trace fossil with distinct behaviors and morphologies. This framework facilitates comparisons between historical and contemporary benthic communities from a morphoethological perspective, offering a unique approach not found in other biological or paleontological disciplines. Nonetheless, future research should explore how this comparison can contribute to resolving broader environmental inquiries concerning marine benthic ecology.
Conclusions
We propose a three-labels classification for marine lebensspuren based on a combination of the most important classification elements: 1) morphology, 2) behaviour, and 3) tracemaker taxonomy. We suggest the use of open nomenclature to consistently document common classificatory uncertainties for each of these elements. The proposed integrative marine lebensspuren classification promises more systematic global comparisons and an integration of this information with other disciplines of marine research. Therefore, a blend of ethological and morphological characterization should serve as the basis for generating larger-scale diversity comparisons, as most observed lebensspuren will preserve this essential information.
Data availability statement
The original contributions presented in the study are included in the article/Supplementary Material. Further inquiries can be directed to the corresponding author.
Author contributions
OM-S: Conceptualization, Data curation, Formal analysis, Funding acquisition, Investigation, Methodology, Project administration, Resources, Software, Validation, Visualization, Writing – original draft, Writing – review & editing. RP: Conceptualization, Investigation, Methodology, Writing – review & editing. FR-T: Conceptualization, Investigation, Methodology, Writing – review & editing. AU: Conceptualization, Investigation, Methodology, Writing – review & editing. BB: Conceptualization, Investigation, Methodology, Writing – review & editing. JD: Conceptualization, Investigation, Methodology, Writing – review & editing. TR: Conceptualization, Funding acquisition, Investigation, Methodology, Supervision, Writing – original draft, Writing – review & editing.
Funding
The author(s) declare financial support was received for the research, authorship, and/or publication of this article. This contribution was funded by research project PID2019-104625RB-100 funded by MCIN/AEI/10.13039/501100011033. OM-S research was also funded through a Humboldt Postdoctoral Fellowship (Humboldt Foundation).
Acknowledgments
The authors want to dedicate this manuscript to Dr. Markus Bertling, who recently passed away, for the unquestionable value of his research on the ichnotaxonomical analysis. The research of A.U. was supported by the Strategic Programme Excellence Initiative from the Jagiellonian University. This is contribution #20 of the Senckenberg Ocean Species Alliance (SOSA). We are most grateful for the constructive comments of the referees who helped us to improve the clarity of the manuscript.
Conflict of interest
The authors declare that the research was conducted in the absence of any commercial or financial relationships that could be construed as a potential conflict of interest.
The author(s) declared that they were an editorial board member of Frontiers, at the time of submission. This had no impact on the peer review process and the final decision.
Publisher’s note
All claims expressed in this article are solely those of the authors and do not necessarily represent those of their affiliated organizations, or those of the publisher, the editors and the reviewers. Any product that may be evaluated in this article, or claim that may be made by its manufacturer, is not guaranteed or endorsed by the publisher.
Supplementary material
The Supplementary Material for this article can be found online at: https://www.frontiersin.org/articles/10.3389/fmars.2024.1371097/full#supplementary-material
References
Althaus F., Hill N., Ferrari R., Edwards L., Przeslawski R., Schönberg C. H. L., et al. (2015). A standardised vocabulary for identifying benthic biota and substrata from underwater imagery: The CATAMI Classification Scheme. PloS One 10, e0141039. doi: 10.1371/journal.pone.0141039
Baucon A., Carvalho C. N., Felletti F., Tosadori G., Antonelli A. (2021). Small-world dynamics drove Phanerozoic divergence of burrowing behaviors. Geology 49, 748–752. doi: 10.1130/G48523.1
Bell J. B., Jones D. O. B., Alt C. H. S. (2013). Lebensspuren of the bathyal mid-atlantic ridge. Deep Sea Res. Part II: Topical Stud. Oceanography 98, 341–351. doi: 10.1016/j.dsr2.2012.09.004
Bertling M. (2007). “CHAPTER 5 - what’s in a name? Nomenclature, systematics, ichnotaxonomy,” in Trace Fossils. Ed. Miller W. (Elsevier, Amsterdam), 81–91. doi: 10.1016/B978-044452949-7/50131-5
Bertling M., Braddy S. J., Bromley R. G., Demathieu G. R., Genise J., Mikuláš R., et al. (2006). Names for trace fossils: a uniform approach. Lethaia 39, 265–286. doi: 10.1080/00241160600787890
Bertling M., Buatois L. A., Knaust D., Laing B., Mángano M. G., Meyer N., et al. (2022). Names for trace fossils 2.0: theory and practice in ichnotaxonomy. Lethaia 55, 1–19. doi: 10.18261/let.55.3.3
Bett B. J., Rice A. L., Thurston M. H. (1995). A quantitative photographic survey of ‘Spoke-Burrow’ type lebensspuren on the Cape Verde Abyssal Plain. Internationale Rev. der gesamten Hydrobiologie und Hydrographie 80, 153–170. doi: 10.1002/iroh.19950800204
Brandt A., Chen C., Tandberg A. H. S., Miguez-Salas O., Sigwart J. D. (2023). Complex sublinear burrows in the deep sea may be constructed by amphipods. Ecol. Evol. 13, e9867. doi: 10.1002/ece3.9867
Bromley R. G. (1981). Concepts in ichnotaxonomy illustrated by small round holes in shells. Acta Geológica Hispánica 16, 56–64.
Bromley R. G. (2012). Trace Fossils: Biology, Taxonomy and Applications (London: Routledge), 380. doi: 10.4324/9780203059890
Buatois L., Mángano G. (2011). Ichnology: Organism-substrate interactions in space and time (Cambridge, UK: Cambridge University Press). doi: 10.1017/CBO9780511975622
Darwin C. (1881). The formation of vegetable mould through the action of worms with observation on their habits (London.: John Murray).
Dashtgard S. E., Gingras M. K. (2012). “Chapter 10 - Marine invertebrate neoichnology,” in Developments in Sedimentology, vol. 64 . Eds. Knaust D., Bromley R. G. (Amsterdam: Elsevier), 273–295. doi: 10.1016/B978-0-444-53813-0.00010-1
Dawson J. W. (1862). On the footprints of Limulus as compared with Protichnites of the Postdam sandstone. Can. Nat. Geologist 7, 271–277.
De Vaugelas J. (1989). Deep-sea lebensspuren: remarks on some echiuran traces in the Porcupine Seabight, northeast Atlantic, Deep Sea Research Part A. Oceanographic Res. Papers 36, 975–982. doi: 10.1016/0198-0149(89)90038-1
Dundas K., Przeslawski R. (2009). Deep sea lebensspuren: biological features on the seafloor on the Eastern and Western Australian margin (Camberra, Australia: Geoscience Australia).
Durden J. M., Schoening T., Althaus F., Friedman A., Garcia R., Glover A. G., et al. (2016). Perspectives in visual imaging for marine biology and ecology: from acquisition to understanding. Oceanogr. Mar. Biol. Ann. Rev. 54, 1–72. doi: 10.1201/9781315368597-2
Ekdale A. A. (1980). Graphoglyptid burrows in modern deep-sea sediment. Science 207, 304–306. doi: 10.1126/science.207.4428.304
Ewing M., Davis R. A. (1967). “Lebensspuren photographed on the ocean floor,” in Deep-Sea Photography (John Hopkins Press, Baltimore).
Frey R. W. (1973). Concepts in the study of biogenic sedimentary structures. SEPM JSR 43, 6-19. doi: 10.1306/74D726C1-2B21-11D7-8648000102C1865D
Gaillard C. (1991). Recent organism traces and ichnofacies on the deep-sea floor off New Caledonia, Southwestern Pacific. PALAIOS 6, 302. doi: 10.2307/3514910
Gingras M., Dashtgard S., MacEachern J., Pemberton S. (2008). Biology of shallow marine ichnology: a modern perspective. Aquat. Biol. 2, 255–268. doi: 10.3354/ab00055
Hollister C. D., Heezen B. C., Nafe K. E. (1975). “Animal traces on the deep-sea floor,” in The Study of Trace Fossils: A Synthesis of Principles, Problems, and Procedures in Ichnology. Ed. Frey R. W. (Springer, Berlin, Heidelberg), 493–510. doi: 10.1007/978-3-642-65923-2_21
Horton T., Marsh L., Bett B. J., Gates A. R., Jones D. O. B., Benoist N. M. A., et al. (2021). Recommendations for the standardisation of open taxonomic nomenclature for image-based identifications. Front. Mar. Sci. 8. doi: 10.3389/fmars.2021.620702
Jones D. O. B., Bett B. J., Tyler P. A. (2007). Megabenthic ecology of the deep Faroe–Shetland channel: A photographic study. Deep Sea Res. Part I: Oceanographic Res. Papers 54, 1111–1128. doi: 10.1016/j.dsr.2007.04.001
Katija K., Orenstein E., Schlining B., Lundsten L., Barnard K., Sainz G., et al. (2022). FathomNet: A global image database for enabling artificial intelligence in the ocean. Sci. Rep. 12, 15914. doi: 10.1038/s41598-022-19939-2
Kaufmann R. S., Wakefield W. W., Genin A. (1989). Distribution of epibenthic megafauna and lebensspuren on two central North Pacific seamounts. Deep Sea Res. Part A. Oceanographic Res. Papers 36, 1863–1896. doi: 10.1016/0198-0149(89)90116-7
Kitchell J. A., Kitchell J. F., Johnson G. L., Hunkins K. L. (1978). Abyssal traces and megafauna: comparison of productivity, diversity and density in the Arctic and Antarctic. Paleobiology 4, 171–180. doi: 10.1017/S0094837300005844
Knaust D. (2012). “Chapter 3 - trace-fossil systematics,” in Developments in Sedimentology, vol. 64 . Eds. Knaust D., Bromley R. G. (Amsterdam: Elsevier), 79–101. doi: 10.1016/B978-0-444-53813-0.00003-4
La Croix A. D., Ayranci K., Dashtgard S. E. (2022). Neoichnology of siliciclastic shallow-marine environments: Invertebrates, traces, and environmental conditions. Earth-Science Rev. 233, 104170. doi: 10.1016/j.earscirev.2022.104170
Mángano M. G., Buatois L. A., Maples C. G., Lanier W. P. (1997). Tonganoxichnus a new insect trace from the Upper Carboniferous of eastern Kansas. Lethaia 30, 113–125. doi: 10.1111/j.1502-3931.1997.tb00451.x
Miguez-Salas O., Bett B. J., Torres-Montilla S., Rodríguez-Tovar F. J., Durden J. M. (2023a). Comparison of rosette-shape traces in abyssal terrains: Environmental and faunal implications. Deep Sea Res. Part I: Oceanographic Res. Papers 197, 104051. doi: 10.1016/j.dsr.2023.104051
Miguez-Salas O., Brandt A., Knauber H., Riehl T. (2024). Diversity and density relationships between lebensspuren and tracemaking organisms: a study case from abyssal northwest Pacific. Biogeosciences 21, 641–655. doi: 10.5194/bg-21-641-2024
Miguez-Salas O., Brandt A., Moreau C. (2023b). Neoichnological analysis of sea stars in the deep sea near the Aleutian Trench: behavioral insights from in situ observations. Mar. Biodivers 54, 3. doi: 10.1007/s12526-023-01398-1
Miguez-Salas O., Huffard C. L., Smith K. L., McGill P. R., Rodríguez-Tovar F. J. (2020). Faunal assemblage changes, bioturbation and benthic storms at an abyssal station in the northeastern Pacific. Deep Sea Res. Part I: Oceanographic Res. Papers 160, 103277. doi: 10.1016/j.dsr.2020.103277
Miguez-Salas O., Rodríguez-Tovar F. J., Ekdale A. A., Kaiser S., Brandt A., Gooday A. J. (2023c). Northernmost (Subarctic) and deepest record of Paleodictyon: paleoecological and biological implications. Sci. Rep. 13, 7181. doi: 10.1038/s41598-023-34050-w
Miguez-Salas O., Vardaro M. F., Rodríguez-Tovar F. J., Pérez-Claros J. A., Huffard C. L. (2022). Deep-sea echinoid trails and seafloor nutrient distribution: present and past implications. Front. Mar. Sci. 9. doi: 10.3389/fmars.2022.903864
Nathorst A. G. (1873). Om nâgra förmodade växtfossilier, Öfversigt af Kgl. Vetensk. Akad. Förhandl. (Kongliga Svenska Vetenskaps-Akademiens Handlingar, Sweden) 9, 25–52.
Nathorst A. G. (1881). Om spâr af nagra evertebrerade djur M.M. och deras paleontologiska betydelse. 104 pp.
Patterson D. J., Cooper J., Kirk P. M., Pyle R. L., Remsen D. P. (2010). Names are key to the big new biology. Trends Ecol. Evol. 25, 686–691. doi: 10.1016/j.tree.2010.09.004
Pirrone C. A., Buatois L. A., Bromley R. G. (2014). Ichnotaxobases for bioerosion trace fossils in bones. J. Paleontology 88, 195–203. doi: 10.1666/11-058
Przeslawski R. (2022). Broad distribution of spider-shaped lebensspuren along the Australian continental margin. Front. Mar. Sci. 9. doi: 10.3389/fmars.2022.1086193
Przeslawski R., Barrett N., Carroll A., Foster S., Gibbons B., Jordan A., et al. (2023). Developing an ocean best practice: A case study of marine sampling practices from Australia. Front. Mar. Sci. 10. doi: 10.3389/fmars.2023.1173075
Przeslawski R., Dundas K., Radke L., Anderson T. J. (2012). Deep-sea Lebensspuren of the Australian continental margins. Deep Sea Res. Part I: Oceanographic Res. Papers 65, 26–35. doi: 10.1016/j.dsr.2012.03.006
Purser A., Marcon Y., Dreutter S., Hoge U., Sablotny B., Hehemann L., et al. (2019). Ocean floor observation and bathymetry system (OFOBS): A new towed camera/sonar system for deep-sea habitat surveys. IEEE J. Oceanic Eng. 44, 87–99. doi: 10.1109/JOE.2018.2794095
Ride W. D. J. L. (1999). International Code of Zoological Nomenclature (The Natural History Museum, London UK: International Trust for Zoological Nomenclature).
Rindsberg A. K. (2012). “Chapter 2 - ichnotaxonomy: finding patterns in a welter of information,” in Developments in Sedimentology, vol. 64 . Eds. Knaust D., Bromley R. G. (Amsterdam: Elsevier), 45–78. doi: 10.1016/B978-0-444-53813-0.00002-2
Rindsberg A. K. (2018). Ichnotaxonomy as a science. Annales Societatis Geologorum Poloniae 88, 91-110. doi: 10.14241/asgp.2018.012
Seike K. (2023). Diving neoichnology: underwater fieldwork focusing on organism and seafloor ecosystem interactions. Ichnos 30, 69–78. doi: 10.1080/10420940.2023.2270219
Seike K., Jenkins R. G., Watanabe H., Nomaki H., Sato K. (2012). Novel use of burrow casting as a research tool in deep-sea ecology. Biol. Lett. 8, 648–651. doi: 10.1098/rsbl.2011.1111
Seilacher A. (1954). Die geologische bedeutung fossiler lebensspuren. Z. der deutschen geologischen Gesellschaft 105, 214–227. doi: 10.1127/zdgg/105/1954/214
Seilacher A. (1977). “Chapter 11 evolution of trace fossil communities,” in Developments in Palaeontology and Stratigraphy, vol. 5 . Ed. Hallam A. (Amsterdam: Elsevier), 359–376. doi: 10.1016/S0920-5446(08)70331-5
Sigovini M., Keppel E., Tagliapietra D. (2016). Open Nomenclature in the biodiversity era. Methods Ecol. Evol. 7, 1217–1225. doi: 10.1111/2041-210X.12594
Smith K. L., Holland N. D., Ruhl H. A. (2005). Enteropneust production of spiral fecal trails on the deep-sea floor observed with time-lapse photography. Deep Sea Res. Part I: Oceanographic Res. Papers 52, 1228–1240. doi: 10.1016/j.dsr.2005.02.004
Uchman A., Pervesler P. (2006). Surface lebensspuren produced by amphipods and isopods (crustaceans) from the isonzo delta tidal flat, Italy. PALAIOS 21, 384–390. doi: 10.2110/palo.2005.P05-63e
Vallon L. H., Rindsberg A. K., Bromley R. G. (2016). An updated classification of animal behaviour preserved in substrates. Geodinamica Acta 28, 5–20. doi: 10.1080/09853111.2015.1065306
Vences M. (2020). The promise of next-generation taxonomy. Megataxa 1, 35–38. doi: 10.11646/megataxa.1.1.6
Wetzel A. (2008). Recent bioturbation in the deep south China Sea: A uniformitarian ichnologic approach. PALAIOS 23, 601–615. doi: 10.2110/palo.2007.p07-096r
Wheatcroft R. A., Smith C. R., Jumars P. A. (1989). Dynamics of surficial trace assemblages in the deep sea. Deep Sea Res. Part A. Oceanographic Res. Papers 36, 71–91. doi: 10.1016/0198-0149(89)90019-8
Winston J. E. (2018). Twenty-first century biological nomenclature—The enduring power of names. Integr. Comp. Biol. 58, 1122–1131. doi: 10.1093/icb/icy060
Keywords: lebensspuren, classification, underwater image, seafloor, benthic fauna
Citation: Miguez-Salas O, Przeslawski R, Rodríguez-Tovar FJ, Uchman A, Bett BJ, Durden JM and Riehl T (2024) Marine lebensspuren: improving the classification of seafloor traces from underwater imagery and observations. Front. Mar. Sci. 11:1371097. doi: 10.3389/fmars.2024.1371097
Received: 15 January 2024; Accepted: 12 February 2024;
Published: 21 March 2024.
Edited by:
Elva G. Escobar-Briones, National Autonomous University of Mexico, MexicoReviewed by:
Krzysztof Pabis, University of Łódź, PolandJan Marcin Weslawski, Polish Academy of Sciences, Poland
Copyright © 2024 Miguez-Salas, Przeslawski, Rodríguez-Tovar, Uchman, Bett, Durden and Riehl. This is an open-access article distributed under the terms of the Creative Commons Attribution License (CC BY). The use, distribution or reproduction in other forums is permitted, provided the original author(s) and the copyright owner(s) are credited and that the original publication in this journal is cited, in accordance with accepted academic practice. No use, distribution or reproduction is permitted which does not comply with these terms.
*Correspondence: Olmo Miguez-Salas, b2xtby5taWd1ZXotc2FsYXNAc2VuY2tlbmJlcmcuZGU=