- 1Key Laboratory for Coastal and Wetland Ecosystems, Ministry of Education, College of the Environment and Ecology, Xiamen University, Xiamen, China
- 2National Observation and Research Station for the Taiwan Strait Marine Ecosystem, Xiamen University, Xiamen, China
- 3Guangxi Key Lab of Mangrove Conservation and Utilization, Guangxi Academy of Marine Sciences, Guangxi Mangrove Research Center, Guangxi Academy of Sciences, Beihai, China
There is much controversy surrounding factors that affect the distribution of mangrove plants across the intertidal gradient. It was previously hypothesized that mangrove zonation was attributed to tidal sorting (TSH) of its propagules according to size (weight) or differential ability of propagules to establish in deep water. However, observational and experimental evidence have provided little support for the actual mechanism(s) of mangrove zonation. In general, species distribution pattern is the consequence of propagule dispersal. The specific gravity of water-borne mangrove propagules may affect their buoyancy, with inherent links to dispersal, thereby potentially influencing tree zonation. Propagule specific gravity can influence the distribution of mangroves in the context of global change, particularly in response to changes in seawater salinity. In this study, we measured the specific gravity and weight of 35 mangrove species propagules. There was no correlation between the weight of the propagule and its specific gravity. The specific gravity of propagules of true mangrove species was significantly greater than that of semi-mangrove. The results of the correlation between propagule specific gravity and the relative surface elevation of field distribution support the hypothesis that propagules are subject to tidal sorting and are not related to weight but to specific gravity. This newfound understanding of mangrove dispersal and distribution is critical in the context of mangrove protection and restoration, especially in projecting the effects of anthropogenic activities and global change on mangrove communities.
1 Introduction
On an intertidal scale, mangroves are commonly distributed in bands parallel to the tidal gradient, known as zonation (Duke et al., 1998; Wang et al., 2019). Zonation patterns of mangrove species have been a main research focus for several decades and have led to numerous hypotheses that attempt to explain mangrove zonation. There are four major explanations for mangrove zonation, including (1) propagule dispersal (Watson, 1928; Rabinowitz, 1978a, b; Bunt et al., 1985; McGuinness, 1997; Sousa et al., 2007; De Ryck et al., 2012; Crase et al., 2013; Van der Stocken et al., 2019a), (2) physiological adaptation (Ball, 1998, 2002), (3) animal predation (Smith, 1987a, b; Farnsworth, 1997) and (4) interspecific competition (McKee, 1995a). Mangrove zonation is the outcome of a number of processes driven by surface elevation and variables such as hydroperiod, soil salinity and soil physical-chemical characteristics (Crase et al., 2013; Tomlinson, 2016) The process of mangrove zonation is complex due to interaction of biotic and abiotic factors on the distribution and survival of mangrove seedlings. Therefore, no conclusion has yet satisfactorily explained the mechanism(s) that govern intertidal distribution of mangroves.
Propagule dispersal has been recognized as a main factor affecting population dynamics and community structure (Sousa et al., 2007; Van der Stocken et al., 2019a). Propagule dispersal is foundational to forest structure and dynamics, genetic diversity, and differentiation (Tonné et al., 2017). Additionally, understanding mangrove propagule dispersal is important for determining the potential for natural recovery of mangrove forest (e.g., natural regeneration of mangroves in abandoned ponds) and is critical to support management decisions for reforestation (Di Nitto et al., 2013; Van der Stocken et al., 2022).
Although the propagules of some mangrove species can be dispersed by wind or birds, most mangrove propagules are water-borne (Tomlinson, 2016). Interspecific differences in propagule dispersal patterns may contribute to mangrove zonation, a phenomenon commonly observed in mature mangrove communities (Rabinowitz, 1978b; McKee, 1995b; McGuinness, 1997). The Tidal Sorting Hypothesis (TSH), introduced by Rabinowitz (1978b), has been widely accepted as an explanation for this zonation. TSH hypothesizes that species with heavier propagules are more likely to be found in seaward areas, while species with lighter propagules are more common in landward areas. However, there are notable exceptions where TSH does not hold. For example, Avicennia marina and Sonneratia alba, both species with smaller propagules, are distributed in the lower intertidal zone, whereas species with larger propagules, such as Xylocarpus granatum, dominate the middle to upper intertidal zones (Smith, 1987a; Delgado et al., 2001). De Ryck et al. (2012) suggest that the number of propagules released, and their buoyancy can influence a species’ dispersal capacity. Despite these variations, Tomlinson (2016) maintains that propagule weight is a significant factor in determining zonation.
Propagule dispersal has been shown to be a main factor characterizing species distributions. While the reasons why TSH has not been supported in mangrove environments are unclear, there are marked species differences in dispersal and establishment strategies among mangrove plants (Robert et al., 2015). Ceriops tagal and Rhizophora mucronata (Robert et al., 2015); Bruguiera gymnorhiza and Rhizophora stylosa, which are in the same family (Kadoya and Inoue, 2015), have different propagule dispersal and establishment strategies. Previous studies investigated fewer species and did not consider propagules of different structure. Propagule structure is used to indicate the type of propagule and whether it is viviparous or not. Moreover, semi-mangrove, which commonly have higher tidal distribution and propagules of some species are also diffused by water (Tomlinson, 2016), were also omitted.
Few researchers have noted the influence of water salinity on dispersal ability of mangrove propagules (Alleman and Hester, 2011; Robert et al., 2015; Van der Stocken et al., 2015; Tonné et al., 2017; Van der Stocken et al., 2022). Water with greater salinity is denser therefore, for mangrove propagules whose densities are close to the sea water, small changes in salinity may determine whether propagules continue to float or settle. For example, a study found that the propagules of Aegiceras corniculatum could remain buoyant in full seawater for up to three months, but in brackish water, they sank within one week (Clarke, 1995). B. gymnorhiza and A. marina propagules also floated in saltwater and sank in freshwater (Clarke et al., 2001). Interspecific differences result in different floating states of propagules of different species, which is expressed in terms of species buoyancy (specific gravity: specific gravity refers to the ratio of the density of a substance to the density of a reference substance, typically water, at a specific temperature and pressure). However, data on the specific gravity of propagules of various mangrove plant species are incomplete.
In this study, the specific gravity and weight of 35 species of mangrove plants were measured; these included 25 true mangroves, 10 semi-mangroves, and mangrove species with different propagule structure. We posed two main research questions: (1) Does tidal sorting determine mangrove species zonation? (2) Which is the primary factor driving tidal sorting: propagule weight or propagule specific gravity? We hypothesized that mangrove zonation is determined by the tidal sorting of propagules. Furthermore, we hypothesized that tidal sorting is not driven by propagule weight but by their specific gravity. Propagules distributed along the intertidal zone were also collected and surface elevations were recorded. Additions to the tidal sorting hypothesis and the mangrove plant specific gravity database are critical for understanding mangrove distribution patterns, which are of great importance for mangrove restoration and predicting the distribution of mangrove plants in response to global changes.
2 Materials and methods
2.1 Study site
This study was conducted in Bamen Bay (19° 22′ - 19° 35′ N, 110° 40′ - 110°48′ E), Hainan Island, China (Figure 1), a small bay situated in the southeastern part of Hainan Island. The bay has a tropical monsoon climate, with an average annual temperature of 24°C and an average annual precipitation of 1974 mm (Wang et al., 2019). The tidal pattern in the bay is irregular all-day tide, with an average tidal range of 0.75 m and a maximum tidal range of 2.06 m. The specific gravity of seawater in the study area ranges from 1.00-1.02 g/cm3. Bamen Bay contains the highest biodiversity of mangrove species in China (Wang and Wang, 2007). Of the 25 true mangrove species found in China, 23 species are present in the bay. Bamen Bay is also home to 11 semi-mangroves (Wang and Wang, 2007). It is thought that these species include all propagule structure of mangrove propagules (Table 1). Therefore, all types of propagules naturally occurring at the same bay provide a unique opportunity to study the effects of propagule characteristics (i.e., propagule weight and specific gravity) on the intertidal distribution of mangrove species.
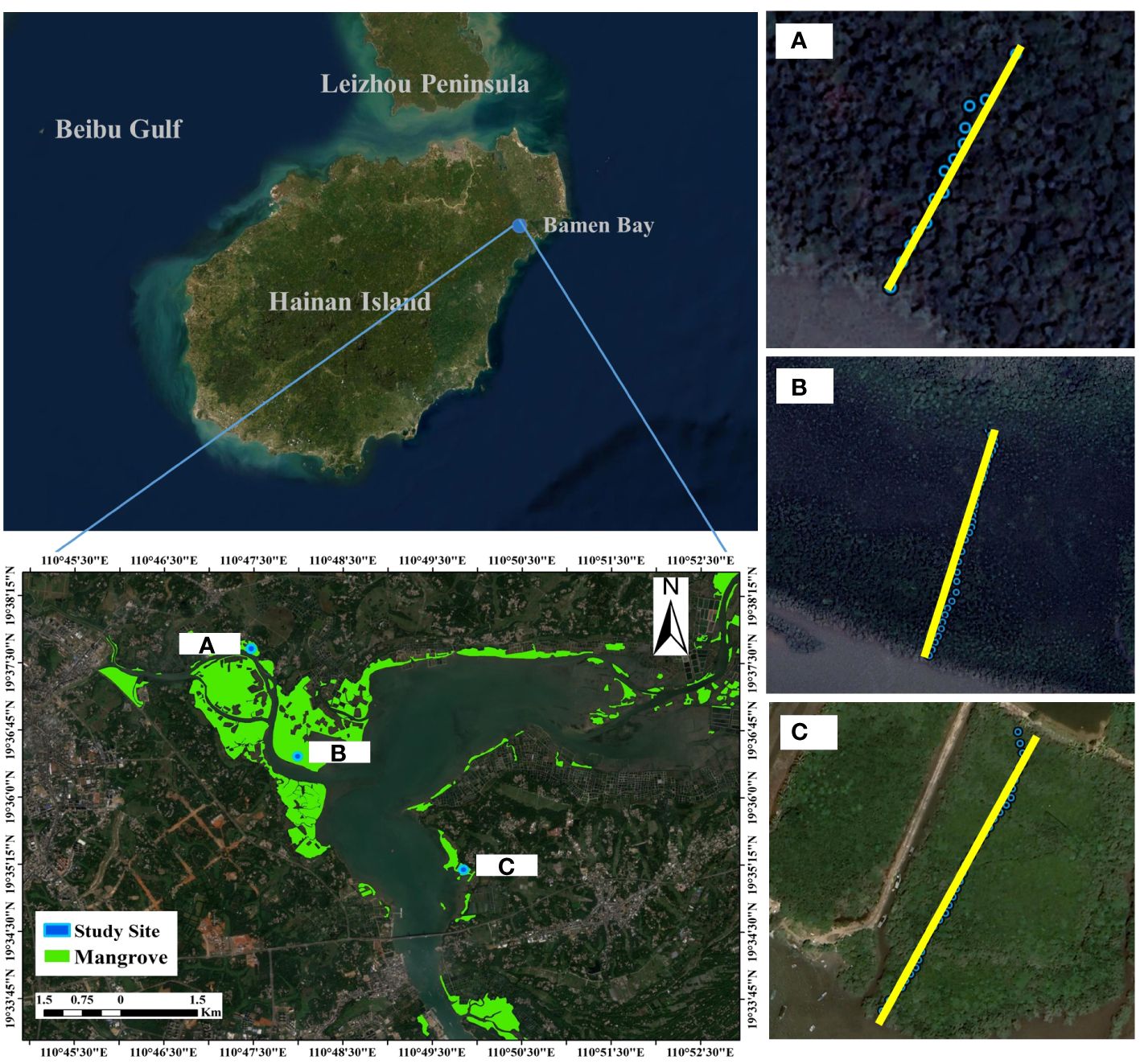
Figure 1 Study sites and mangrove vegetation attributes in Bamen bay, Hainan Island, China. The study site includes 3 transects (A-C). Each transect is vertical from the seaward forest edge to the landward forest edge.
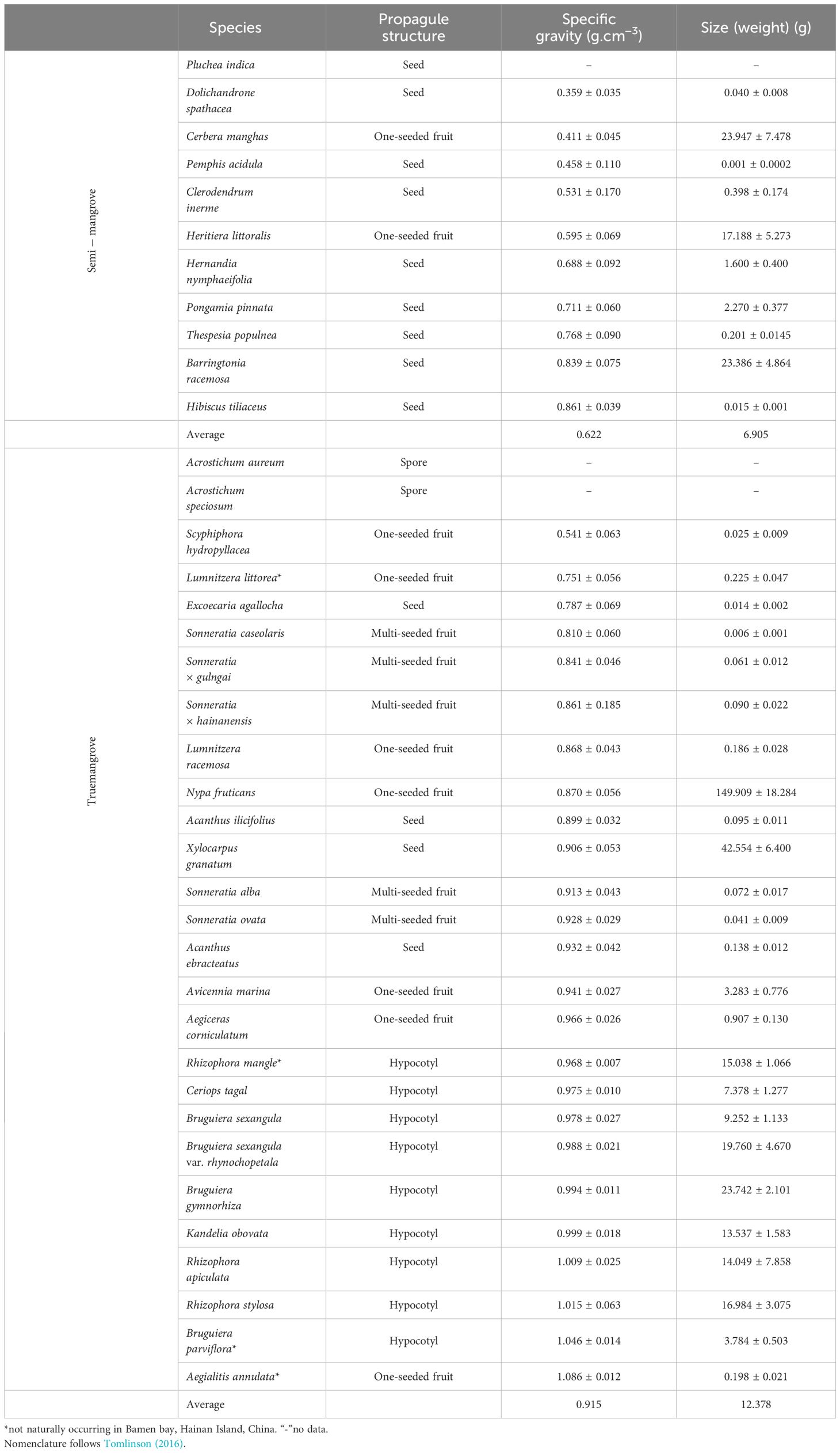
Table 1 Propagule structure, specific gravity and size (weight) ± standard deviation (SD) of the propagules of mangroves (n=20) in Bamen bay, Hainan Island, China.
2.2 Sample collection and analysis
Mature propagules of 35 mangroves, including 25 true mangrove species and 10 semi-mangroves, were collected when all propagules were available in order to compare their weight and specific gravity. Twenty mature propagules of each species were collected and measured during each species’ propagule maturation season from April to November in 2021. Five mature individuals of each mangrove species were randomly selected, and four healthy and undamaged mature propagules were collected from each plant. Additionally, the mature propagules of Lumnitzera littorea from Sanya of Hainan Island, and Aegialitis annulata and Rhizophora mangle from an introduction plant garden in Haikou of Hainan Island were also collected. A. annulata and R. mangle were introduced from Australia and Mexico from 1998 to 1999 (Liao et al., 2006).
In this study, propagule size was expressed as the fresh weight which is same as Tomlinson (2016) and Rabinowitz (1978b). Propagule volume was measured using a water-displacement method according to a revised Archimedes’ principle (Hughes, 2005). Fresh weight was measured with an analytical balance (1 mg resolution). For species with smaller propagules, an electronic balance with higher resolution (0.1 mg) and smaller beaker (10 ml) were used. The weight and volume were then used to calculate the specific gravity of each propagule.
According to the Archimedes’ theorem:
m: propagule weight
m0: weight of propagules submerged in water
ρ0: water specific gravity
ρ: propagule specific gravity
To explore the dispersal of propagules, three sample transects were set up along the river, from the seaward forest edge to the terrestrial edge of the land. The three transects were named A, B, and C in an upstream to downstream direction, with transect lengths of 70 m, 210 m and 185 m, respectively. Along each transect, 5 m × 5 m plots were set (Figure 1) to survey the mangrove propagule distribution. A total of 89 plots (transect A: 10 plots; transect B:42 plots; transect C:37 plots) were set up along the three transects, with mangrove propagules and relative surface elevation documented within each plot. The species and count of mature individuals of mangrove plants in each plot were also recorded. Trees over 1.8 m in height and shrubs over 0.6 m in height were recorded. Propagules were collected once a month after the spring tide, during July, August, and September (i.e., the main propagule dispersal period). The surface elevation of the study plots on the seaward side of transect A was assumed to be 0 cm, which allowed for comparisons between transects. The relative surface elevation of each plot was determined using a Global Navigation Satellite System-Real Time Kinematic GPS unit (iRTK10, Hi-Target Inc., Guangzhou, China). This unit has a vertical precision of 25 mm. The measurement of relative surface elevation was conducted using a five-point sampling method. Within each plot, five sampling points were randomly selected and measured, with the average serving as the relative surface elevation of the plot.
Mean and standard deviation (SD) values of propagule specific gravity and fresh weight of each species were calculated. A t-test was used to test whether differences are significant in specific gravity and weight between true mangroves and semi-mangrove. S. hydropyllacea propagules were most abortive and our study did not test for maturity on a case-by-case basis; thus, the correlation between their propagule specific gravity/weight and the relative surface elevation of their distribution was not explored. Then we determined correlations between specific gravity/weight and the relative surface elevation of propagule dispersal. The tests were conducted and figures created using RStudio (4.0.4).
3 Results
3.1 Weight and specific gravity of true mangroves and semi-mangrove
Propagule structure, weight and static specific gravity of the propagules of 35 mangrove species (25 true mangroves and 10 semi-mangrove) were measured (Table 1). The propagule specific gravity of 35 mangrove species ranged between 0.359 g/cm3 and 1.086 g/cm3. Among them, the propagule of Dolichandrone spathacea had the lowest specific gravity while propagules of A. annulata had the highest specific gravity. The true mangroves had significantly higher propagule specific gravity than the semi-mangrove (p<0.0001). The specific gravity of the propagules of 10 semi-mangrove ranged from 0.359 g/cm3 to 0.861 g/cm3 with a mean value of 0.622 g/cm3. The densities of the propagules of 25 true mangroves ranged from 0.541 g/cm3 to 1.086 g/cm3 with a mean value of 0.915 g/cm3. Except for Scyphiphora hydropyllacea and L. littorea, the densities of the propagules for 25 true mangroves were all higher than 0.800 g/cm3. However, for semi-mangrove, only the densities of the propagules of Hibiscus tiliaceus and Barringtonia racemosa were higher than 0.800 g/cm3.
The average fresh weight of propagules of true mangroves (12.378 g) appears to be heavier than that of semi-mangroves (6.905 g), while the Wilcoxon Rank-Sum Test results reported that there was no significant difference between these two groups (p=0.490, F=0.027). Of the true mangroves, Nypa fruticans had the largest propagule weight (149.909 g) and Sonneratia caseolaris had the smallest propagule weight (0.006 g). For the mangrove associates, the fresh weights of propagules were between 0.001 g and 23.947 g. The correlation between fresh weight and static specific gravity of 33 mangrove propagules was not significant (p=0.189) (Figure 2). N. fruticans had the heaviest propagule among the 33 mangrove species, but its specific gravity was lower than (p=0.04) propagules of Acanthus ilicifolis with a fresh weight of 0.122 g. Cerbera manghas and Clerodendrum inerme propagules had similar specific gravity, but fresh weights varied significantly, where C. manghas propagule was 300 times heavier than the propagules of C. inerme.
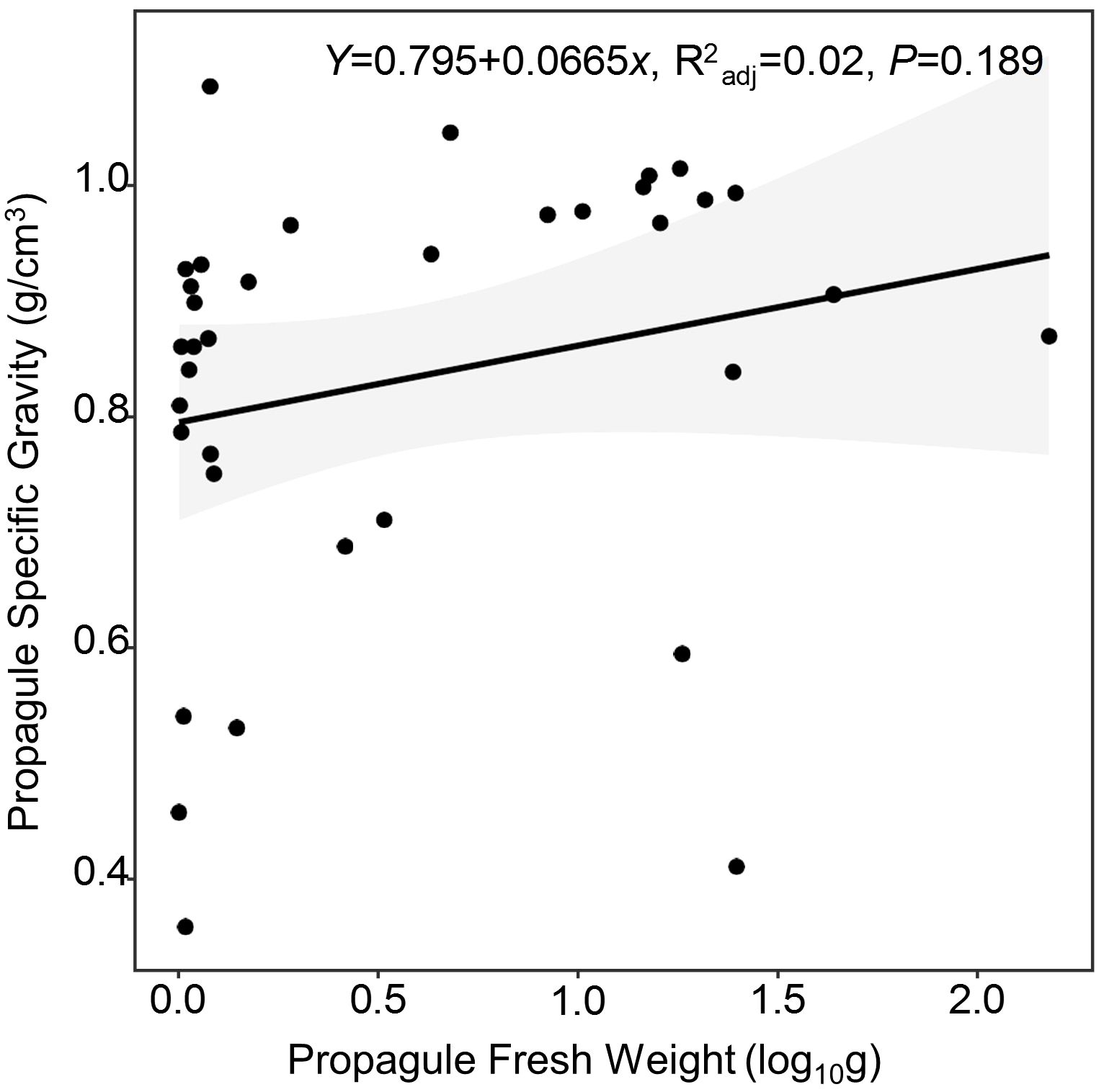
Figure 2 Relationship between fresh weight and static specific gravity of the propagules of 35 mangrove species (25 true mangrove species and 10 mangrove associates, p=0.189).
3.2 Distribution of propagules and relationship to specific gravity/weight
A total of ten mangrove species were recorded, including both propagules and mature individuals. Among these, three species were observed at transect A, five species at transect B, and eight species at transect C (Figure 3). All transects have distinct mangrove propagule zonation patterns (Figure 3A). The propagules at transect A included Sonneratia caseolaris, Rhizophora apiculata and B. sexangula. At transect B, propagules of A. marina, R. apiculate, B. sexangula, C. tagal and L. racemosa were ordered from the seaward edge to landward edge. At transect C, S. alba, R. apiculate, X. granatum, S. hydrophyllacea, C. tagal, L. racemosa, A. corniculatum and A. marina were the dominant species from the seaward to landward edge. Mature individuals of different mangrove plants (Figure 3B) show similar distribution characteristics to propagules.
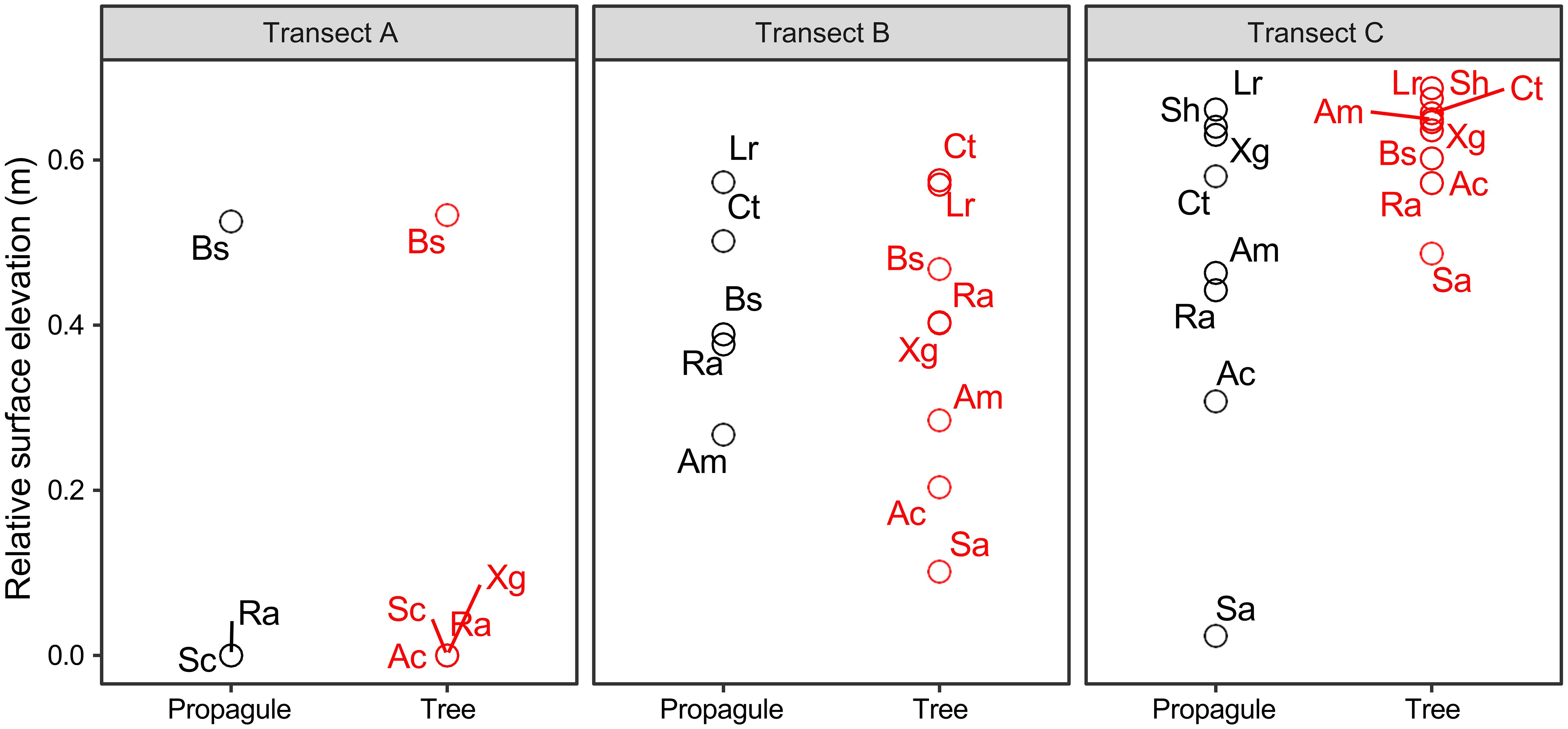
Figure 3 Relative surface elevations of mangrove propagules and mature individuals of mangrove plants (tree) in transect A-C along Bamen bay, Hainan Island, China. (Sa, Sonneratia alba; Sc, Sonneratia caseolaris; Ra, Rhizophora apiculata; Ct, Ceriops tagal; Ac, Aegiceras corniculatum; Bs, Bruguiera sexangula; Am, Avicennia marina; Lr, Lumntizera racemosa; Xg, Xylocarpus granatum; Sh, Scyphiphora hydropyllacea).
Of all the mangrove species recorded in Bamen bay, 10 mangrove species from total 3 transects were found. There was a significant negative linear relationship (p=0.017, Figure 4A) between the propagule specific gravity and relative surface elevations of mangroves. However, there is no significant correlation between weight and relative surface elevation (p=0.972, Figure 4B).
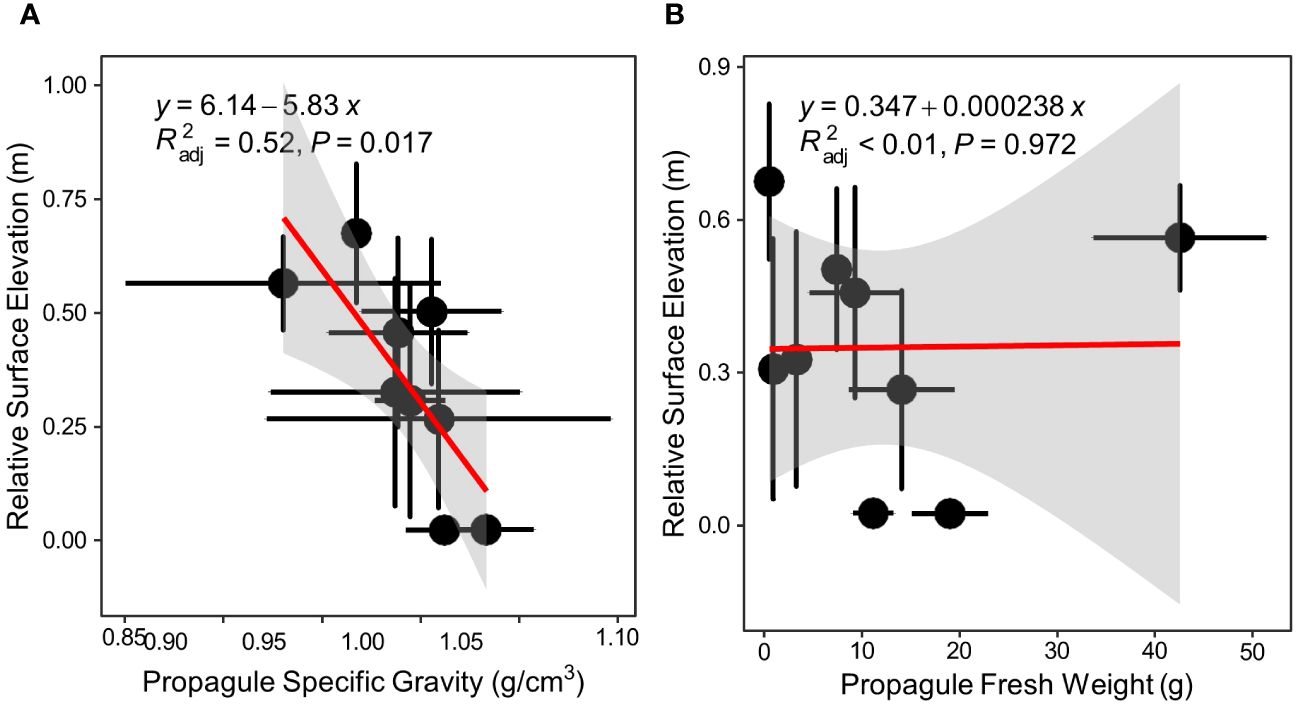
Figure 4 Correlation between propagule specific gravity/weight and relative surface elevation of plant distribution in Bamen bay, Hainan Island, China. (A) correlation between propagule specific gravity and relative surface elevation; (B) correlation between propagule weight and relative surface elevation (Sa, Sonneratia alba; Sc, Sonneratia caseolaris; Ra, Rhizophora apiculata; Ct, Ceriops tagal; Ac, Aegiceras corniculatum; Bs, Bruguiera sexangula; Am, Avicennia marina; Lr, Lumntizera racemosa; Xg, Xylocarpus granatum).
4 Discussion
In our study, we highlight the importance of specific gravity for small scale zonation and offer precise measurements for propagules across 35 mangrove species. The results of this study show that the mangrove propagules are all slightly less dense than seawater (1.00-1.02 g/cm3), which facilitates propagule dispersal and ensures sinking in suitable areas. Mangrove propagules can remain buoyant and viable for long periods to expand their effective range of dispersal (Steinke, 1975, 1986; Rabinowitz, 1978b; Duke et al., 1998). Propagule specific gravity determines their buoyancy, and propagule buoyancy is an important factor that influences dispersal in frequently flooded environments (Johansson et al., 1996). The propagules that had lower specific gravity than seawater could keep floating during dispersal and may move away from mature trees. In contrast, the propagules which have higher specific gravity than seawater would sink. This means that there are species-specific differences to water salinity during the propagule dispersal phase. We found that there was no relationship between propagule specific gravity and weight. Additionally, the dispersal of mangrove propagules was not explained by propagule weight but rather by propagule specific gravity (Figure 4). While many studies have used models to predict the future distributions or restoration outcomes of mangroves (Hamilton et al., 2017; Van der Stocken and Menemenlis, 2017; Van der Stocken et al., 2019b), our study through comparisons of more species, shows that mangrove propagules have significant interspecific differences in specific gravity and difference in settlement position, which further supports the conclusion of Tom Van der Stocken et al. (2019a). Almost all of the models ignore interspecific differences in propagules and assume that they can disperse anywhere with ocean currents. Therefore, the inclusion of different species propagule characteristics in the model is necessary in future predictions (Di Nitto et al., 2013; Wang et al., 2019), which is what our results want to emphasize.
In general, the seaward distance was positively correlated with surface elevation in the intertidal zone (Fu et al., 2019; Ma et al., 2020). The results indicate that the distribution pattern of mangrove propagules is perpendicular to the coastline of Bamen Bay, exhibiting a consistent zonation pattern with mangrove plants (Wang et al., 2019; Ma et al., 2020). It was once widely thought that mangrove propagules dispersed over the entire intertidal zone (Smith, 1992). The previous conclusion overlooked the influence of dispersal on species distribution and magnified the role of environmental factors. Meanwhile, our results revealed that both mangrove trees and propagules exhibit zonation patterns at the intertidal scale. The zonation of propagule was similarly obtained with the field mark-recapture experiment (Sousa et al., 2007), as well as inferences gathered from measured propagule specific gravity (Wang et al., 2019).
The closer to the downstream of the estuary, the higher the salinity of the sea water. Previous studies have shown that S. caseolaris seeds have a limited range of adaptation to salinity, with conditions below 10‰ being the most suitable for growth (Liao et al., 1997). The propagules and plants of S. caseolaris only occurred in transect A and were not found in the downstream transects B and C. This suggested that while the propagules of S. caseolaris cannot be carried to downstream of estuary, the seedlings are protected from high salinity. In contrast to transect A, the propagules and S. alba, C. tagal and L. racemosa were found in the downstream transects. The differences between propagule specific gravity and sea water specific gravity (Wang et al., 2019) prevented propagules from reaching the upper reaches of the river. Our results suggest that mangrove propagules can be dispersed to suitable habitats that influenced the distribution of mangroves. The similarity in the distribution of propagules and plants suggests propagule dispersal as a key driver of mangrove distribution patterns, alongside other biotic and abiotic factors (Sousa et al., 2007; Kadoya and Inoue, 2015; Wang et al., 2019; Van der Stocken et al., 2019a). Settlement of propagules in the intertidal is set to limit animal predation and is influenced by environmental factors such as tidal scour, water salinity, and inundation, which all contribute to the early establishment of mangrove plants (Krauss et al., 2008). The differences in settling positions of propagules, adaptability to water salinity, tolerance to inundation, and efficiency in nutrient utilization further contribute to the zonal distribution of mangrove plants (Tomlinson, 2016).
Both C. tagal and R. mucronata, belong to the same family and reproduce viviparously, however, R. mucronata was found to strand and establish in the lower intertidal zone (Robert et al., 2015). In the present study, although S. caseolaris and S. alba both belong to Sonneratiaceae, their propagules and plants were distributed in different locations along the river. R. apiculata, B. sexangular, and C. tagal belong to the family Rhizophoraceae, however, B. sexangula, R. apiculate, and C. tagal were distributed in order from sea edge to landedge in the intertidal zone. Differences in the intertidal positioning of propagules resulted from varying retention periods for species in the intertidal zone. The retention duration of mangrove propagules is in fact linked to the duration of the window for establishment (Balke et al., 2013; Van der Stocken et al., 2019a; van Hespen et al., 2022). This window denotes the timeframe during the retention period, when mangrove propagules have the opportunity to encounter favorable growth conditions within the intertidal zone, facilitating their establishment into plants (Balke et al., 2011; 2013).
The appearance of zonation with propagule dispersal and plant establishment also supported the tidal zonation hypothesis (Rabinowitz, 1978b), which results from tide sorting of propagules based on their traits or differences in propagule colonization ability. However, unlike Rabinowitz (1978b), we found that it was not weight but specific gravity that affects the distribution in the intertidal zone. Van der Stocken et al. (2022) proposed that the interaction between specific gravity and seawater can influence the distribution of mangrove plants, particularly in the context of human activities and climate change induced changes in sea water salinity (Cinco-Castro and Herrera-Silverira, 2020; Chen et al., 2021), which lead to changes in hydrological characteristics.
The influence of the propagule dispersal stage must not be overlooked in future discussions of the cause(s) or predictions of mangrove distribution patterns (Di Nitto et al., 2013; Van der Stocken et al., 2019a; Chen et al., 2020). Our study measured the specific gravity and weight of 35 species of mangrove plants, including 25 true mangroves, 10 semi-mangroves, and mangrove species with different propagule structures. This comprehensive data collection and analysis contribute to a deeper understanding of the ecological characteristics of different propagule structures of mangrove plants. We conducted a reassessment of the tidal sorting hypothesis, which has led to new insights into the distribution mechanisms of mangrove plants and proposed alternative perspectives diverging from traditional views. Our results indicate that dispersal has an impact on mangrove zonation, and environmental adaptation and interspecific competition may further intensify mangrove zonation. If interspecific differences are not properly accounted for, the effects of environmental filtering or interspecific competition on patterns may be magnified. In the case of mangrove conservation and restoration, it is desirable to obtain a community structure similar to that of natural mangrove forests. The complexity of elevation in the restored area should be set artificially in restoration to cope with species differences in propagule retention. Meanwhile, the setting of critical elevations is essential to ensure that propagules can sink and seedlings survive in all future mangrove restoration projects. We suggest that more consideration should be given to interactions of hydrodynamics with propagule dispersal processes in different species in future studies/investigations of mangrove plants. Additionally, the relationship between the survival and dispersal abilities of propagules should also be taken into account for more accurate estimations of mangrove distribution.
Data availability statement
Publicly available datasets were analyzed in this study. This data can be found here: https://zenodo.org/records/10476887.
Author contributions
CL: Writing – original draft, Visualization, Software, Project administration, Methodology, Formal analysis, Data curation, Conceptualization. LZ: Writing – review & editing, Software, Methodology, Conceptualization. XS: Writing – review & editing, Methodology, Investigation, Conceptualization. XL: Writing – review & editing, Methodology, Investigation, Conceptualization. YD: Writing – review & editing, Methodology, Investigation, Conceptualization. MW: Writing – review & editing, Validation, Supervision, Funding acquisition, Conceptualization. WW: Writing – review & editing, Validation, Supervision, Resources, Project administration, Methodology, Funding acquisition, Conceptualization.
Funding
The author(s) declare financial support was received for the research, authorship, and/or publication of this article. This study was supported by the National Natural Science Foundation of China (30200031, 40776046, 42176169).
Conflict of interest
The authors declare that the research was conducted in the absence of any commercial or financial relationships that could be construed as a potential conflict of interest.
Publisher’s note
All claims expressed in this article are solely those of the authors and do not necessarily represent those of their affiliated organizations, or those of the publisher, the editors and the reviewers. Any product that may be evaluated in this article, or claim that may be made by its manufacturer, is not guaranteed or endorsed by the publisher.
References
Alleman L. K., Hester M. W. (2011). Reproductive ecology of black mangrove (Avicennia germinans) along the louisiana coast: Propagule production cycles, dispersal limitations, and establishment elevations. Estuaries Coasts. 34, 1068–1077. doi: 10.1007/s12237-011-9404-8
Balke T., Bouma T., Horstman E., Webb E., Erftemeijer P., Herman P. (2011). Windows of opportunity: thresholds to mangrove seedling establishment on tidal flats. Mar. Ecol.-Prog. Ser. 440, 1–9. doi: 10.3354/meps09364
Balke T., Webb E. L., van den Elzen E., Galli D., Herman P. M. J., Bouma T. J. (2013). Seedling establishment in a dynamic sedimentary environment: a conceptual framework using mangroves. J. Appl. Ecol. 50, 740–747. doi: 10.1111/1365-2664.12067
Ball M. C. (1998). Mangrove species richness in relation to salinity and waterlogging: a case study along the Adelaide River floodplain, northern Australia. Glob. Ecol. Biogeogr. Lett. 7, 73–82. doi: 10.2307/2997699
Ball M. C. (2002). Interactive effects of salinity and irradiance on growth: implications for mangrove forest structure along salinity gradients. Trees-Struct. Funct. 16, 126–139. doi: 10.1007/s00468-002-0169-3
Bunt J. S., Wllliams T., Clay H. J. (1985). Detection of species sequences across environmental gradients. Mar. Ecol.-Prog. Ser. 24, 197–199. doi: 10.3354/meps024197
Chen G., Gu X., Li S., Wang Y., Zhang Y., Wang W., et al. (2021). Human-mediated dispersal redefines mangrove biogeography in the anthropocene. Ecography 44, 1845–1855. doi: 10.1111/ecog.05925
Chen L., Feng H., Gu X., Dong Y., Cheng P., Guo X. D., et al. (2020). Linkages of flow regime and micro-topography: prediction for non-native mangrove invasion under sea-level rise. Ecosyst. Health Sust. 6, 1780159. doi: 10.1080/20964129.2020.1780159
Cinco-Castro S., Herrera-Silverira J. (2020). Vulnerability of mangrove ecosystems to climate change effects: The case of the Yucatan Peninsula. Ocean Coast. Manage. 192, 105196. doi: 10.1016/j.ocecoaman.2020.105196
Clarke P. J. (1995). The population dynamics of the mangrove shrub Aegiceras corniculatum (Myrsinaceae): fecundity, dispersal, establishment and population structure. Proc. Linn. Soc N. S. W. 115, 35–44.
Clarke P. J., Kerrigan R. A., Westphal C. J. (2001). Dispersal potential and early growth in 14 tropical mangroves: do early life history traits correlate with patterns of adult distribution? J. Ecol. 89, 648–659. doi: 10.1046/j.0022-0477.2001.00584.x
Crase B., Liedloff A., Vesk P. A., Burgman M. A., Wintle B. A. (2013). Hydroperiod is the main driver of the spatial pattern of dominance in mangrove communities. Glob. Ecol. Biogeogr. 22, 806–817. doi: 10.1111/geb.12063
Delgado P., Hensel P., Jiménez J., Day J. (2001). The importance of propagule establishment and physical factors in mangrove distributional patterns in a Costa Rican estuary. Aquat. Bot. 71, 157–178. doi: 10.1016/S0304-3770(01)00188-7
De Ryck D. J. R., Robert E. M. R., Schmitz N., van der Stocken T., Di Nitto D., Dahdouh-Guebas F., et al. (2012). Size does matter, but not only size: Two alternative dispersal strategies for viviparous mangrove propagules. Aquat. Bot. 103, 66–73. doi: 10.1016/j.aquabot.2012.06.005
Di Nitto D., Erftemeijer P. L. A., van Beek J. K. L., Dahdouh-Guebas F., Higazi L., Quisthoudt K., et al. (2013). Modelling drivers of mangrove propagule dispersal and restoration of abandoned shrimp farms. Biogeosciences 10, 5095–5113. doi: 10.5194/bg-10-5095-2013
Duke N. C., BALL M. C., Ellison J. C. (1998). Factors influencing biodiversity and distributional gradients in mangroves. Glob. Ecol. Biogeogr. Lett. 7, 27–47. doi: 10.2307/2997695
Farnsworth E. J. (1997). Global patterns of pre-dispersal propagule predation in mangrove forests. Biotropica 29, 318–330. doi: 10.1111/j.1744-7429.1997.tb00433.x
Fu H., Zhang Y., Ao X., Wang W., Wang M. (2019). High surface elevation gains and prediction of mangrove responses to sea-level rise based on dynamic surface elevation changes at Dongzhaigang Bay, China. Geomorphology 334, 194–202. doi: 10.1016/j.geomorph.2019.03.012
Hamilton J. F., Osman R. W., Feller I. C. (2017). Modeling local effects on propagule movement and the potential expansion of mangroves and associated fauna: testing in a sub-tropical lagoon. Hydrobiologia 803, 173–187. doi: 10.1007/s10750-017-3231-2
Hughes S. W. (2005). Archimedes revisited: A faster, better, cheaper method of accurately measuring the volume of small objects. Phys. Education. 40, 468–474. doi: 10.1088/0031-9120/40/5/008
Johansson M. E., Nilsson C., Nilsson E. (1996). Do rivers function as corridors for plant dispersal? J. Veg. Sci. 7, 593–598. doi: 10.2307/3236309
Kadoya T., Inoue T. (2015). Spatio-temporal pattern of specific gravity of mangrove diaspore: Implications for upstream dispersal. Ecography 38, 472–479. doi: 10.1111/ecog.01074
Krauss K. W., Lovelock C. E., McKee K. L., López-Hoffman L., Ewe S. M. L., Sousa W. P. (2008). Environmental drivers in mangrove establishment and early development: A review. Aquat. Bot. 89, 105–127. doi: 10.1016/j.aquabot.2007.12.014
Liao B., Zheng S., Chen Y., Li M., Zeng W., Zheng D. (2006). Preliminary report on introduction of several alien mangrove plants in dongzhai harbour of hainan province. J. Cent. South forestry university. 26, 63–67. doi: 10.14067/j.cnki.1673-923x.2006.03.015
Liao B., Zheng D., Zheng S., Li Y. (1997). Seed germination conditions of Sonneratia caseolaris of mangrove. J. Cent. South Forestry University. 17, 25–31. doi: 10.14067/j.cnki.1673-923x.1997.01.005
Ma W., Wang W., Tang C., Chen G., Wang M. (2020). Zonation of mangrove flora and fauna in a subtropical estuarine wetland based on surface elevation. Ecol. Evol. 10, 7404–7418. doi: 10.1002/ece3.6467
McGuinness K. A. (1997). Dispersal, establishment and survival of Ceriops tagal propagules in a north Australian mangrove forest. Oecologia 109, 80–87. doi: 10.1007/s004420050061
McKee K. L. (1995a). Interspecific variation in growth, biomass partitioning, and defensive characteristics of neotropical mangrove seedlings: response to light and nutrient availability. Am. J. Bot. 82, 299–307. doi: 10.1002/j.1537-2197.1995.tb12634.x
McKee K. L. (1995b). Seedling recruitment patterns in a Belizean mangrove forest: effects of establishment ability and physico-chemical factors. Oecologia 101, 448–460. doi: 10.1007/BF00329423
Rabinowitz D. (1978a). Early growth of mangrove seedlings in Panama, and a hypothesis concerning the relationship of dispersal and zonation. J. Biogeogr. 5, 113–133. doi: 10.2307/3038167
Rabinowitz D. (1978b). Dispersal properties of mangrove propagules. Biotropica 10, 47–57. doi: 10.2307/2388105
Robert E. M. R., Oste J., van der Stocken T., De Ryck D. J. R., Quisthoudt K., Kairo J. G., et al. (2015). Viviparous mangrove propagules of Ceriops tagal and Rhizophora mucronata, where both Rhizophoraceae show different dispersal and establishment strategies. J. Exp. Mar. Biol. Ecology. 468, 45–54. doi: 10.1016/j.jembe.2015.03.014
Smith T. J. III. (1987a). Effects of light and intertidal position on seedling survival and growth in tropical tidal forests. J. Exp. Mar. Biol. Ecology. 110, 133–146. doi: 10.1016/0022-0981(87)90024-4
Smith T. J. III (1987b). Seed predation in relation to tree dominance and distribution in mangrove forests. Ecology 68, 266–273. doi: 10.2307/1939257
Smith T. J. III. (1992). “Forest structure,” in Tropical Mangrove. Eds. Robertson A. I., Alongi D. M. (American Geo-physical Union, Washington), 101–136.
Sousa W. P., Kennedy P. G., Mitchell B. J., Ordóñez L. M. O. (2007). Supply-side ecology in mangroves: Do propagule dispersal and seedling establishment explain forest structure? Ecol. Monogr. 77, 53–76. doi: 10.1890/05-1935
Steinke T. D. (1975). “Some factors affecting dispersal and establishment of propagules of Avicennia marina (Forsk.) Vierh,” in Proceedings of the International Symposium on Biology and Management of Mangroves. Eds. Walsh G. E., Snedaker S. C., Teas H. J. (Gainesville, Florida: Institute of Food and Agriculture Sciences, University of Florida, Gainesville), 402–414.
Steinke T. D. (1986). A preliminary study of buoyancy behaviour in Avicennia marina propagules. S. Afr. J. Bot. 52, 559–565. doi: 10.1016/S0254-6299(16)31492-2
Tonné N., Beeckman H., Robert E. M. R., Koedam N. (2017). Towards an unknown fate: The floating behaviour of recently abscised propagules from wide ranging Rhizophoraceae mangrove species. Aquat. Bot. 140, 23–33. doi: 10.1016/j.aquabot.2017.01.008
Van der Stocken T., Carroll D., Menemenlis D., Simard M., Koedam N. (2019b). Global-scale dispersal and connectivity in mangroves. Proc. Natl. Acad. Sci. U. S. A. 116, 915–922. doi: 10.1073/pnas.1812470116
Van der Stocken T., Menemenlis D. (2017). Modelling mangrove propagule dispersal trajectories using high-resolution estimates of ocean surface winds and currents. Biotropica 49, 472–481. doi: 10.1111/btp.12440
Van der Stocken T., Vanschoenwinkel B., Carroll D., Cavanaugh K. C., Koedam N. (2022). Mangrove dispersal disrupted by projected changes in global seawater density. Nat. Clim. Change 12, 685–691. doi: 10.1038/s41558-022-01391-9
Van der Stocken T., Vanschoenwinkel B., De Ryck D. J. R., Bouma T. J., Dahdouh-Guebas F., Koedam N. (2015). Interaction between water and wind as a driver of passive dispersal in mangroves. PloS One 10, e0121593. doi: 10.1371/journal.pone.0121593
Van der Stocken T., Wee A. K. S., De Ryck D. J. R., Vanschoenwinkel B., Friess D. A., Dahdouh-Guebas F., et al. (2019a). A general framework for propagule dispersal in mangroves. Biol. Rev. 94, 1547–1575. doi: 10.1111/brv.12514
van Hespen R., Hu Z., Peng Y., Zhu Z., Ysebaert T., Bouma T. J. (2022). Identifying trait-based tolerance to sediment dynamics during seedling establishment across eight mangrove species. Limnol. Oceanogr. 67, 2281–2295. doi: 10.1002/lno.12202
Wang W., Li X., Wang M. (2019). Propagule dispersal determines mangrove zonation at intertidal and estuarine scales. Forests 10, 245. doi: 10.3390/f10030245
Keywords: mangrove zonation, propagule, dispersal, weight, specific gravity, intertidal
Citation: Liu C, Zhang L, Shi X, Li X, Deng Y, Wang M and Wang W (2024) A re-evaluation of the tidal sorting hypothesis of mangrove zonation: propagule specific gravity matters. Front. Mar. Sci. 11:1368156. doi: 10.3389/fmars.2024.1368156
Received: 10 January 2024; Accepted: 29 April 2024;
Published: 17 May 2024.
Edited by:
Francesca Porri, South African Institute for Aquatic Biodiversity, South AfricaReviewed by:
Marco Fusi, Newcastle University, United KingdomSuhua Shi, Sun Yat-sen University, China
Copyright © 2024 Liu, Zhang, Shi, Li, Deng, Wang and Wang. This is an open-access article distributed under the terms of the Creative Commons Attribution License (CC BY). The use, distribution or reproduction in other forums is permitted, provided the original author(s) and the copyright owner(s) are credited and that the original publication in this journal is cited, in accordance with accepted academic practice. No use, distribution or reproduction is permitted which does not comply with these terms.
*Correspondence: Wenqing Wang, mangroves@xmu.edu.cn