- 1Department of Coastal systems, NIOZ Royal Netherlands Institute for Sea Research, Den Burg, Texel, Netherlands
- 2Department of Ecology, Radboud Inst. for Biological and Environmental Sciences, Radboud Univ. Nijmegen, Faculty of Science, Nijmegen, Netherlands
- 3South Florida Water Management District, Everglades Systems Assessment, West Palm Beach, FL, United States
- 4Florida Fish Wildlife Conservation Commission, Fish and Wildlife Research Institute, St. Petersburg, FL, United States
- 5B-WARE Research Centre, Radboud University, Nijmegen, Netherlands
- 6Biological Sciences, Florida International University, Miami, FL, United States
- 7Groningen Institute for Evolutionary Life Sciences (GELIFES), University of Groningen, Groningen, Netherlands
Coastal systems often depend on foundation species such as seagrasses that are supported by self-facilitation. Seagrass meadows, however, are threatened worldwide due to climate change and local human impact, disrupting self-facilitation leading to system instability. Florida Bay is a large seagrass dominated coastal ecosystem that suffered from multiple seagrass mortality events over the last half century driven by hypoxia, high water temperatures, hypersalinity, and high biological oxygen demand. These conditions reduce the amount of photosynthetically-derived oxygen in the plant causing sulphide intrusion into meristematic tissues. Using a bay-wide sampling design and long-term monitoring trends of seagrass condition, we investigated the current state of the meadows, sediment characteristics (e.g., organic matter, sulphide, nutrients) and discuss how climate stressors interact with plant and sediment oxygen dynamics. Our survey revealed that at sites where seagrass had been previously denuded by die-off, the dominant seagrass Thalassia testudinum had not recovered, while the pioneering seagrass Halodule wrightii recolonized the impacted areas. Organic matter and sulphide levels were higher at the impacted sites, apparently a persistent characteristic of the formerly dense T. testudinum meadows in central and western Florida Bay. These sediment conditions promote sulphide intrusion of T. testudinum belowground tissue under anoxic conditions. Plant oxidation initially buffers sulphide intrusion, but disruption of this oxidation mechanism due to changing environmental conditions results in widespread mortality and seagrass community collapse. Climate change cannot be fully mitigated by local management, however, attempts can be made to control critical salinity and oxygen levels by increasing freshwater input, reducing hypersalinity and aiming to keep the internal seagrass oxidation feedback intact. Our study shows that the Florida Bay seagrass ecosystem is still recovering four years post die-off and continues to be susceptible to future climate change and system degradation.
Introduction
Seagrass meadows are a key ecological component of many coastal ecosystems. They are essential foundation species due to their habitat-formation, the high biodiversity they support and their socio-economic importance; they serve as carbon and nutrient sinks, control coastal erosion and contribute to secondary production (Heck et al., 2003; Orth et al., 2006; Waycott et al., 2009). However, seagrass meadows are rapidly declining due to human impacts such as eutrophication, coastal development, and climate change, resulting in the loss of valuable ecosystem services (Costanza et al., 1997, 2008; Waycott et al., 2009) Increased severity and frequency of drought and heat waves are expected to drive further decline through hypersalinity, desiccation, hypoxia, and heat stress, all of which can disrupt internal processes important for seagrass ecosystem resilience. For example, drought was recently shown to cause desiccation stress in a large intertidal seagrass-dominated system in Africa, disrupting system stabilizing mutualism, leading to a systemwide die-off event (de Fouw et al., 2016, 2018). In 2010, Shark Bay in Australia was exposed to a severe marine heat wave that led to a widespread loss of seagrass (Fraser et al., 2014; Strydom et al., 2020). Increasing fluctuations in coastal salinity levels due to climate change are expected to affect seagrass survival and distribution (Durako and Howarth, 2017; Sandoval-Gil et al., 2023). This illustrates how extreme meteorological and climatic events can disrupt otherwise stable, long-lived foundation species adapted to dynamic environmental conditions.
Florida Bay is a large seagrass-dominated coastal ecosystem that has suffered from multiple seagrass mortality events over the last half century (Zieman et al., 1989; Robblee et al., 1991; Schmidt, 2002; Hall et al., 2016). Between 1974-1975, 1987-1991 and again in 2015, high water temperatures, hypersalinity, and/or high biological oxygen demand all contributed to the periods of hypoxia and sulphide toxicity that primarily affected the climax seagrass species, Thalassia testudinum (Zieman, 1997; Zieman et al., 1999; Koch et al., 2007d; Hall et al., 2016). After the 1987 event, exposed sediments, loss of T. testudinum as a stable nutrient sink and liberated nutrients from sediments and seagrass detritus led to cyanobacterial blooms and sediment resuspension events that persisted for nearly a decade. Hence, this contributed to widespread light limitation and secondary losses to a wider range of seagrass species (e.g., Halodule wrightii and Syringodium filiforme) (Robblee et al., 1991; Fourqurean and Robblee, 1999; Rodemann et al., 2021). System recovery from die-off events lasted 17-23 years (Hall et al., 2021).
In addition to largescale die-off events, sulphide-driven T. testudinum mortality occurs annually in Florida Bay, typically at the end of summer and in early autumn when plant biomass, salinity and water temperatures are highest and daylength begins to shorten, reducing the amount of photosynthetically-derived oxygen in the plant and the meadow canopy (Hall et al., 1999; Koch et al., 2007d). Hypersaline conditions in Florida Bay are most frequent and severe in the west-central bay (Fourqurean et al., 1992; Hall et al., 2016) where 40-50 PSU often occurs at the end of the dry season (April-May) and at times 70 PSU has been recorded (Fourqurean and Robblee, 1999; Nuttle et al., 2000; Hall et al., 2016). Combined with high temperature (>33°C) leading to reduced photosynthetic efficiency in seagrasses (>60 PSU) (Koch et al., 2007a, c; Johnson et al., 2020) and widespread bottom-water anoxia, all capable of increasing oxygen imbalance in the plant, particularly in the densest stands of T. testudinum (Hall et al., 2021). Simultaneously, high water temperatures increase anaerobic decomposition by sulphate-reducing bacteria, producing sulphide as waste product (Jørgensen, 1982; Lamers et al., 2013). Finally, these external stressors lead to sulphide intrusion in the plant and is thought to be the proximate cause of mortality (Borum et al., 2005; Koch et al., 2007c; Johnson et al., 2018).
Under more benign environmental conditions, seagrasses typically facilitate their own growing conditions through porewater oxygenation and by increasing water clarity, by suspended particle trapping and sediment stabilization (van der Heide et al., 2007; Fourqurean et al., 2012; Hansen and Reidenbach, 2012). A consequence of this self-facilitation process is enhanced organic matter accumulation, however, stimulating anaerobic microbial activity resulting in increased sulphide concentrations (Jørgensen, 1982; Lamers et al., 2013). Organic matter sufficient to initiate sulphide-driven seagrass mortality events may routinely occur in Florida Bay sediments, as evidenced by patchy, small-scale mortality observed in west-central Florida Bay. This area of the bay is where die-off is most pronounced and has been the locus for all major events documented thus far. Due to extensive water management modifications in the Everglades and infrastructure development of the Florida Keys over the last century, it is currently the least-well flushed region of the bay, receiving relatively low influx of freshwater from the Everglades and has limited hydrologic connectivity to either the Gulf of Mexico or the Atlantic Ocean (Lee et al., 2016).
During the day, seagrasses photosynthetically produce oxygen which is released into the water within the phyllosphere and through their roots into the surrounding rhizosphere (radial oxygen loss). At night, oxygen gradients across the sediment water interface help to drive oxygen from the water column to sediment porewater through roots and rhizomes (Figure 1) (Koch et al., 2022). Oxygen can detoxify the sediment by converting free sulphides to sulphate (Pedersen et al., 2004; Borum et al., 2005). However, this plant detoxification mechanism can be disrupted by high water temperatures and hypersalinity that can compromise the photosynthetic capacity of T. testudinum, reducing oxygen concentrations in the bottom water and limiting downward diffusive flux of oxygen into the sediment at night (Johnson et al., 2020). In such cases, sulphide can outpace oxygen, particularly under warmer temperature conditions when sulphide production and metabolic demand increases. This situation may cause higher sulphide intrusion in the plant meristem increasing plant mortality (Koch et al., 2022).
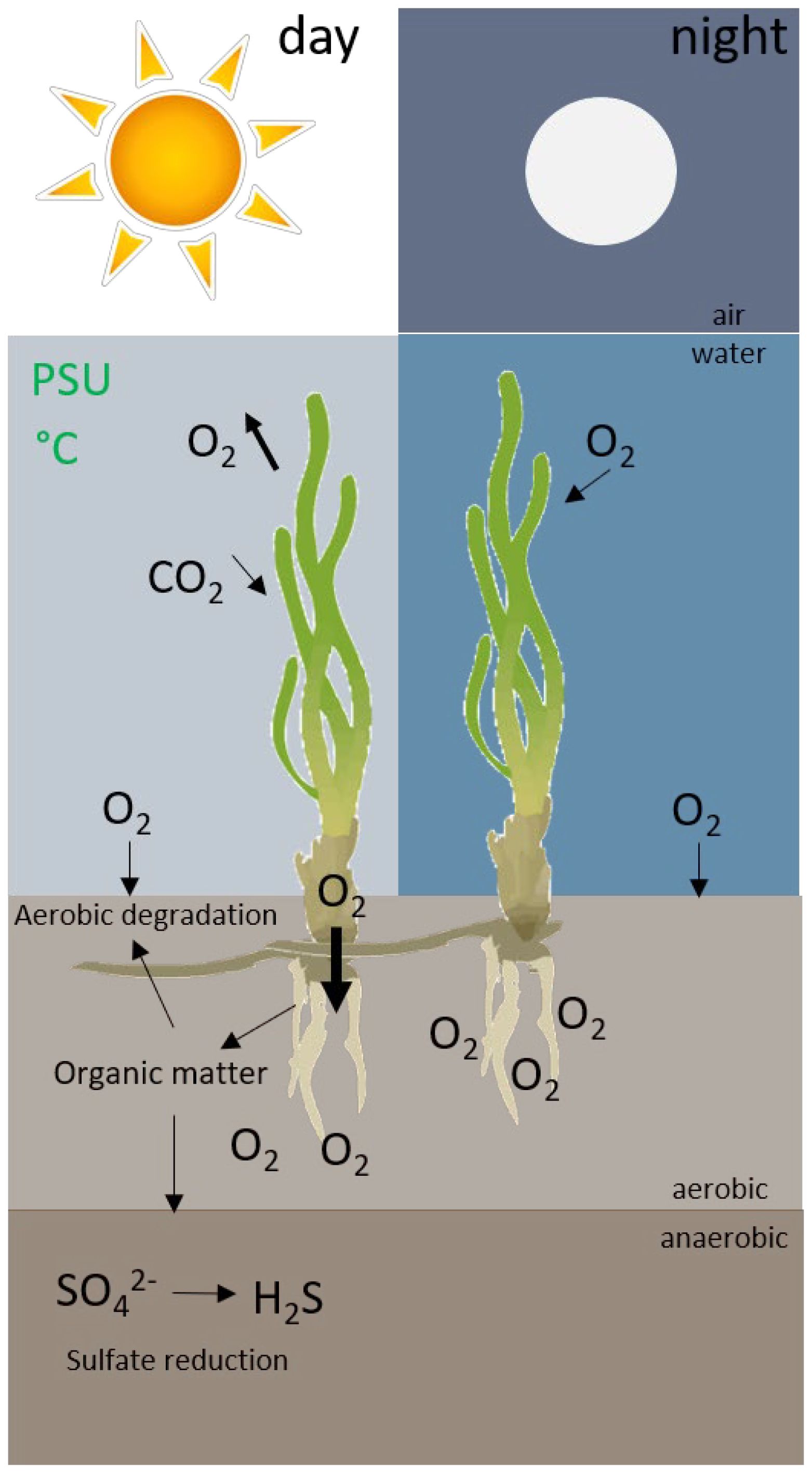
Figure 1 Conceptual model of the photosynthetically-derived oxygen sulphide detoxification mechanism in Thalassia testudinum. During the day, seagrasses photosynthetically produce oxygen which is released into the water within the canopy layer and through their roots into the surrounding rhizosphere in a process called radial oxygen loss (ROL). At night, oxygen gradients across the sediment water interface help to drive oxygen from the water column to sediment porewater through roots and rhizomes. Oxygen can detoxify the sediment by converting free sulphides to sulphate.
To get insight in the current state of the seagrass meadows and understand how external stressors interact with plant dynamics and sediment conditions, we conducted a landscape-level examination of edaphic condition (e.g., organic matter, sulphide, nutrients), sediment morphology and seagrass condition in Florida Bay. Four years after the most recent die-off event in 2015, we conducted a bay-wide study using a gridded sampling design comprising 104 stations. At each station we investigated sediment variables, porewater sulphide concentrations and seagrass status. For long term trends of seagrass condition 15 permanent transects across the bay were used. We hypothesized that 1) based on recovery trajectories of past die-off events, sites impacted would not have fully recovered to pre-die-off conditions; 2) impacted sites would differ in seagrass species and biomass from unimpacted sites and 3) impacted sites would exhibit higher sediment organic matter and sulphide levels than unimpacted sites a legacy from the die-off event. We discuss what these findings mean for the plant oxidation feedback and how the system may be more vulnerable to external environmental stressors due to the greater potential of sulphide build-up.
Methods
Study area
Florida Bay is located at the southern end of the Florida peninsula, bordering the Everglades in the north, the Florida Keys in the east and south, and the Gulf of Mexico in the west (Supplementary Figure S1). Historically the bay was covered with a heterogenous macrophyte community of seagrass species like Halodule wrightii, Ruppia martima and T. testudinum (Fourqurean and Robblee, 1999). For more than a century, development and water management have reduced freshwater flows to the bay from the Everglades. Freshwater flows into Florida Bay were 2-3 times greater and salinities averaged 3-9 PSU lower prior to intense drainage and water diversion in the upstream Everglades in the 20th century (Marshall et al., 2014, 2020), with a lower and more variable salinity regime supporting the mixed macrophyte assemblages (Fourqurean et al., 2003). The reduction of freshwater input changed a formerly heterogenous macrophyte community to a T. testudinum dominated system (Zieman et al., 1989), with high organic biomass that can precede mortality events (Hall et al., 2021). This is one of the largest populations of T. testudinum in the world covering about 2,000 km2 (Fourqurean and Robblee, 1999). The construction of roads and railways between the Florida Keys islands also changed the input of saltwater from the Atlantic Ocean. The combined fresh- and saltwater input changes from the north and the east increased the residence time of the water in the bay considerably (Madden, 2013). In addition, Florida Bay consists of more than 50 shallow basins (0.5 to 3.0 m depth) separated by a network of shallow mudbanks (Lee et al., 2016). This complex geomorphology results in limited water exchange between basins and large differences in water residence times within basins, ranging from a month to a year (Boyer et al., 1999; Lee et al., 2006, 2008, 2016).
Spatio-temporal transect sampling
Temporal trends of aboveground seagrass biomass were collected yearly by the Florida Fish Habitat Assessment Program (FHAP) in 50-meter transects in 15 basins since 2006. Five basins were impacted by the die-off event in 2015 and others were unimpacted (see: Fredley et al., 2019; Peñalver et al., 2020; Hall et al., 2021). In 2016, visual surveys were conducted by FHAP to determine impacted sites and converted to polygon maps (Supplementary Figure S1) (Hall et al., 2016). Biomass was sampled with sediment cores (ø: 15 cm) from three haphazardly selected locations adjacent of each transect line, and dry mass was determined accordingly (see below). We used mean aboveground dry mass per basin per year (2006 to 2021) in autumn for historical trends.
Gridded spatial sampling
Seagrass biomass and sediment characteristics were collected in a large monitoring campaign between 2 October and 20 October in 2019 covering the entirety of Florida Bay. The gridded sampling design consisted of sites taken at 5 km intervals and several additional sites targeting the die-off areas (Supplementary Figure S1). In total 16 stations at impacted sites and 86 stations at unimpacted areas were visited. At each sample location sediment depth was measured with a calibrated pole (220 cm) inserted into the sediment until contact with the solid carbonate platform below. Water depth was similarly measured from the surface to the sediment-water interface. Seagrass was collected with sediment cores (ø: 15 cm) to a depth of about 20 cm and then sieved in 1-mm mesh bags. Live seagrass was separated from shell debris and detritus and sorted by species in the laboratory. Aboveground and belowground biomass was separated, and dry weight was determined after drying for 48 hours at 60°C.
To measure porewater sulphide concentrations and sediment parameters, a separate small sediment core (ø 10 cm) was taken to a depth of 15 cm. The intact sediment core was immediately transferred to small buckets (ø 10 cm) and covered with a lid to prevent oxygen contamination. In the laboratory the sulphide was measured by anaerobically collecting sediment pore water samples in 60-ml vacuumed syringes connected to 10-cm rhizon samplers (Eijkelkamp Agrisearch Equipment, Giesbeek, the Netherlands). Total dissolved sulphide concentration in the pore water was measured immediately after sampling, in a mixture of 50% sample and 50% Sulphide Anti-Oxidation Buffer (SAOB) using an ion-specific silver-sulphide electrode (van der Heide et al., 2012; de Fouw et al., 2016). Sediment samples were taken with a small core (ø 1.5 cm) to a depth of 5 cm from the sediment in the buckets. Sediment samples were freeze-dried in the laboratory and grainsize by using a particle size analyzer with auto sampler (Coulter LS 13 320), and organic matter as loss on ignition (LOI; 5 hrs. at 550°C) were determined. Total phosphorus of the sediment was determined after digestion of dried sediment with nitric acid and analyzed using an inductively coupled plasma emission spectrophotometer (ICP-OES iCAP 6000; Thermo Fisher Scientific, Waltham, MA,USA). Sediment total nitrogen was determined of dried sediment on an elemental analyzer (Thermo Scientific).
Statistics
Differences in organic matter content, porewater sulphide, sediment depth, water depth, sediment phosphorus and -nitrogen between sites impacted and unimpacted by the die-off were tested within one-way analyses of variance. Difference of mean seagrass biomass of the transects before 2015 and after the die-off impact were also tested in a two-way analyses of variance, and Tukey HSD for post-hoc comparisons. We tested whether the independent variables had a significant effect at a significance level of P=0.05. Values are reported as means ± standard deviation. We investigated the occurrence of impacted or unimpacted in relation to current environmental variables (organic matter content, porewater sulphide, sediment depth, water depth, sediment phosphorus and -nitrogen) with logistic regression models (i.e., binary linear mixed models). By using a logistic regression model with a stepwise backward elimination of variables procedure we predicted the occurrence of the die-off. To detect multicollinearity between explanatory variables, variance inflation factors (VIF) were computed with the car R package (Fox and Weisberg, 2019) in combination with Pearson correlation coefficient. Sediment nitrogen content was highly correlated with organic matter content (Pearson rho=0.93) and sediment depth and water depth were also correlated (Pearson rho=0.72), therefore nitrogen content and water depth were dropped before starting the stepwise procedure. This resulted in a VIF of 1.2, indicating that we successfully reduced collinearity (Zuur et al., 2009). All statistical analysis were performed with R statistical and programming environment (R Core Team version 4.1.1, 2021).
Results
At 73% of the gridded stations T. testudinum was observed with an average aboveground biomass of 41.10 ± 58.32 g m-2. Aboveground biomass between die-off impacted and unimpacted sites did not differ (Figure 2, F1,100 = 0.07, P=0.79). However, highest aboveground biomass of H. wrightii was found at the impacted area in the north-west part of the bay (Figure 2, F1,100 = 10.97, P<0.01). Die-off impacted sites had 1.6 times significantly higher organic matter (Figure 3, F1,100 = 27.5, P<0.001) and porewater sulphide concentrations where 2.6 times higher compared to unimpacted sites (Figure 3, F1,100 = 17.89, P<0.001). Impacted sites were also characterized by shallower water depths (1.25 m vs 1.85 m; Supplementary Figure S2, F1,100 = 10.85, P<0.01) and sediment depths were twice greater (1.45 m vs 0.75 m; Supplementary Figure S2, F1,100 = 6.75, P<0.05). Sediment nutrient concentrations of phosphorus (F1,100 = 11.59, P<0.001) and nitrogen (F1,100 = 23.50, P<0.001) were both higher at impacted sites than unimpacted sites (Supplementary Figure S3).
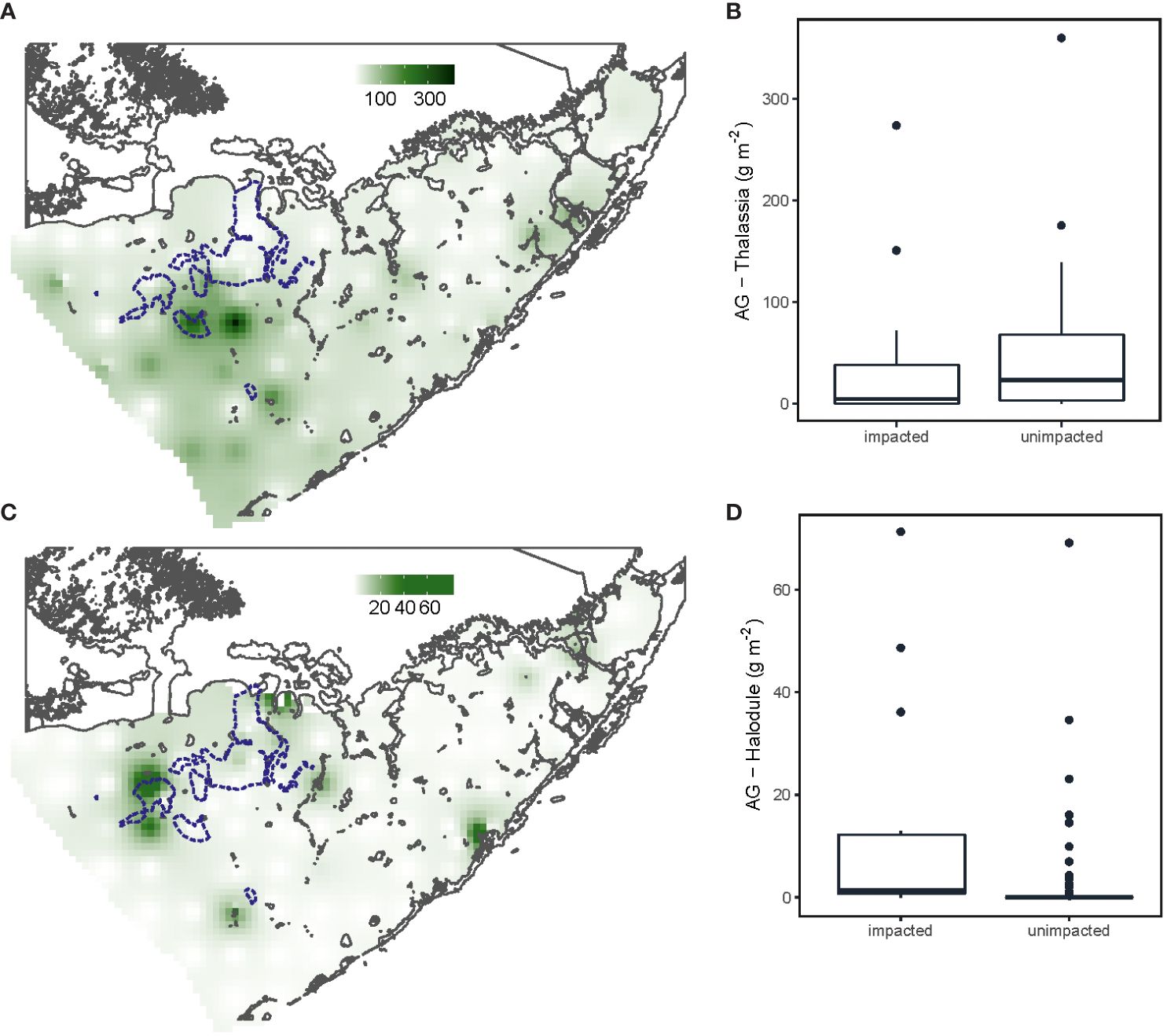
Figure 2 Thalassia testudinum aboveground biomass across the Florida Bay in sampling grid (A) and in impacted and unimpacted die-off areas (B). Halodule wrightii aboveground biomass across the Florida Bay (C) and in impacted and unimpacted die-off areas (D). Die-off area from visual surveys conducted in 2016 by FHAP to determine impacted sites (blue lines).
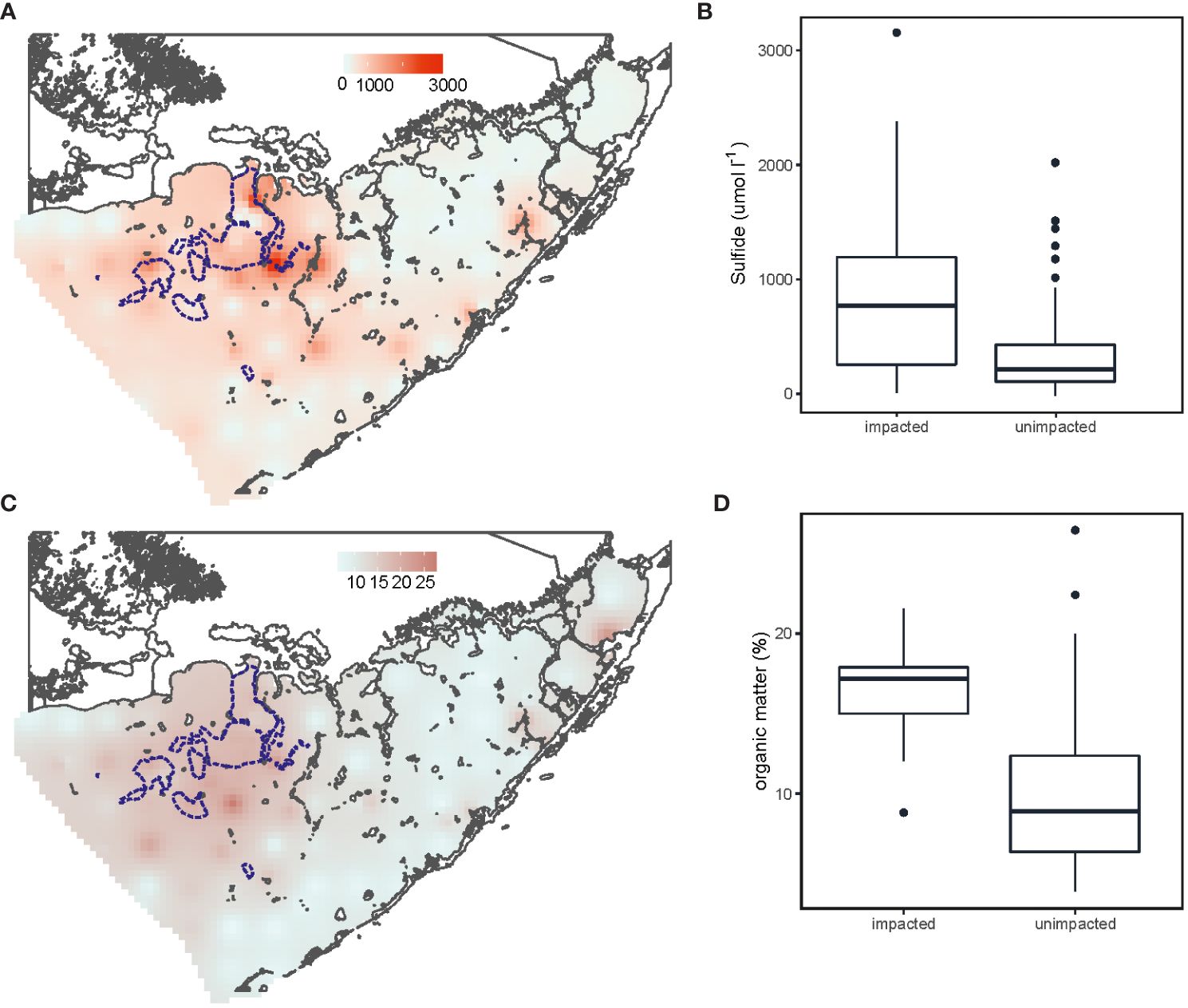
Figure 3 Sediment organic matter (A, B) and sulphide concentrations (C, D) across the Florida Bay and in impacted and unimpacted die-off areas. Die-off area from visual surveys conducted in 2016 by FHAP to determine impacted sites (blue lines).
When explanatory variables were included in the stepwise backward logistic regression procedure, the occurrence of the die-off was best explained by sediment organic matter (χ2 = 21.55, P< 0.001; Figure 4A). From our temporal analysis, there was a significant decrease in mean aboveground T. testudinum biomass before and after the die-off at basins that were impacted (134.2 versus 47.7 g m-2), but no difference in basins that were not impacted (Figure 4B). Consequently, we detected a significant two-way interaction between whether a site was impacted/unimpacted and moment before/after the die-off event (F1,234 = 54.64, P<0.001).
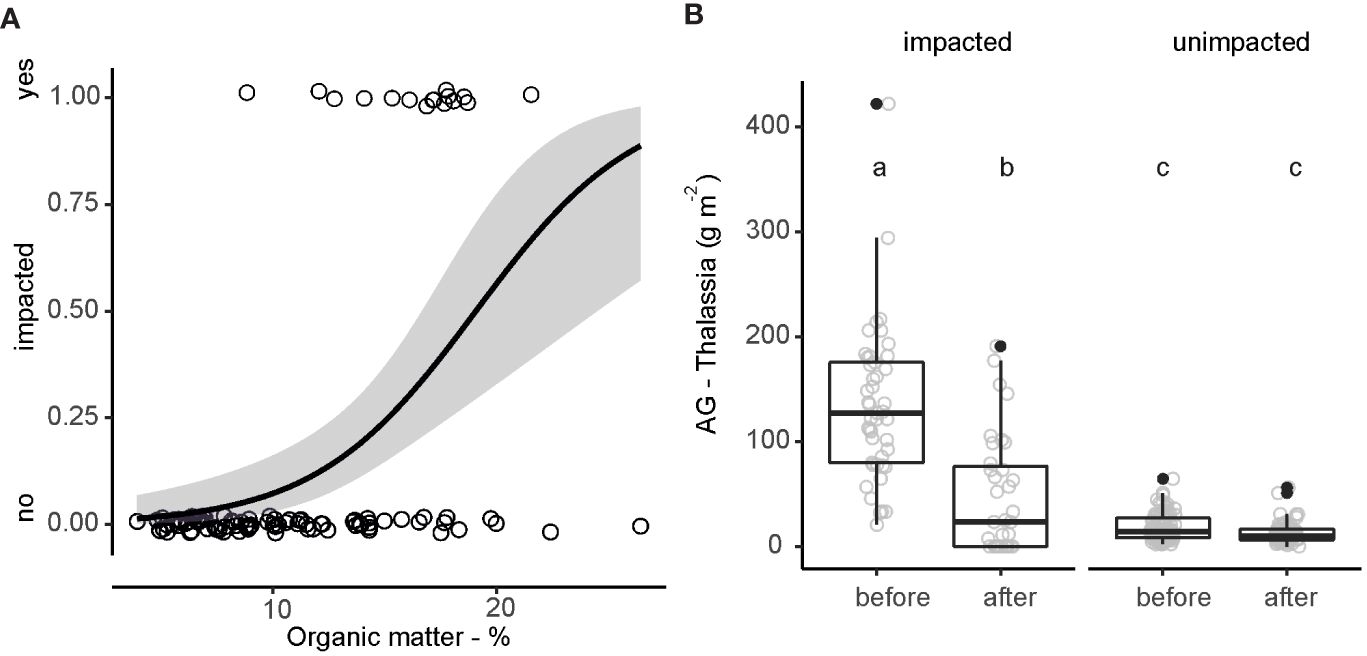
Figure 4 The occurrence of impacted (1) and unimpacted sites (0) plotted against organic matter with prediction of logistic regression with 95% confidence interval (A) and aboveground T. testudinum biomass at impacted and unimpacted sites before (2006-2014) and after the die-off (2015-2021). Letters above the plots depict Tukey post hoc outcome (B).
Discussion
Our field study provides recent insights into local conditions – sediment characteristics in particular – in relation to seagrass standing stock following a large die-off event in Florida Bay. Sites impacted by the die-off in 2015 have higher organic matter content and sulphide concentrations than unimpacted areas. In areas were T. testudinum biomass was reduced by die-off, the pioneering seagrass H. wrightii had recolonized the impacted areas and had higher biomass at those sites than elsewhere in the bay. T. testudinum standing stock in the gridded sampling data did not differ between impacted and unimpacted sites. However, our temporal transect analysis showed that T. testudinum biomass was higher pre die-off at the impacted sites, indicating that high biomass was a precursor of die-off, and that impacted sites had not yet fully recovered four years after the event. The higher organic matter and sulphide levels at the impacted sites suggests that these sites are vulnerable to unfavorable environmental perturbations (e.g. high temperatures and hypersalinity) leading to hypoxia, resulting in seagrass degradation.
Organic matter was the most important predictor explaining the occurrence of the die-off in our analyses. The origin of the higher sediment organic matter content at the impacted sites is a combination of processes. Water quality measurements show that the die-off areas in the central bay have the highest concentration of total organic carbon, -nitrogen and -phosphorus in the water column of any region of the bay and high import of organic matter from the upstream marsh and mangrove zone (Childers et al., 2006). As such in the central bay there is a strong self-facilitating internal feedback, leading to two effects: a more nutrient-rich substrate in which seagrass can grow to potentially high densities (Fourqurean and Robblee, 1999); and inputs of labile organic material from both internal seagrass production and external import that increases respiratory demand and encourages anaerobic processes leading to sulphide production (Koch et al., 2007d, 2022). Both of these processes are self-reinforcing, making the community more vulnerable to system degradation via unfavorable environmental conditions. When system degradation occurs, seagrass die-off causes a spike in organic material and nutrients in the sediment matrix. Thus, the build-up of sediment organic matter due to the combination of internal dynamics and die-off event, creates ideal circumstances for sulphide concentrations to increase. After the die-off, seagrass biomass is low or absent, causing organic matter and sulphide to decrease again, allowing seagrass to recover over time. Overall, this combination of processes may result in cyclic collapse-and-recovery dynamics.
Porewater sulphide concentrations were considerably higher in impacted sites four year post die-off, similar as what was found at post-die-off sites in Florida Bay in the past (Carlson et al., 1994; Kavanagh, 2016). Under favorable environmental seagrass conditions, concentrations found in our study (max 3.2 mM) are not directly toxic for T. testudinum (Koch and Erskine, 2001). Under stress conditions, increased temperature (35°C) and salinity (55 PSU), sulphide concentrations of 6 mM increased T. testudinum mortality (Koch and Erskine, 2001). The dense T. testudinum canopies at the west central site allow for oxygen saturated water retained in the phyllo-sphere and radial oxygen loss through roots to keep a positive oxygen balance preventing sulphide intrusion. When environmental conditions deteriorates, increase of water temperature (>33°C) and salinity (>55 PSU), the risk of disruption of internal plant oxidation increases, and compromise oxygen balance and contributed to anoxia and sulphide intrusion and plant mortality (Figure 5B) (Johnson et al., 2020; Koch et al., 2022; MacLeod et al., 2023).
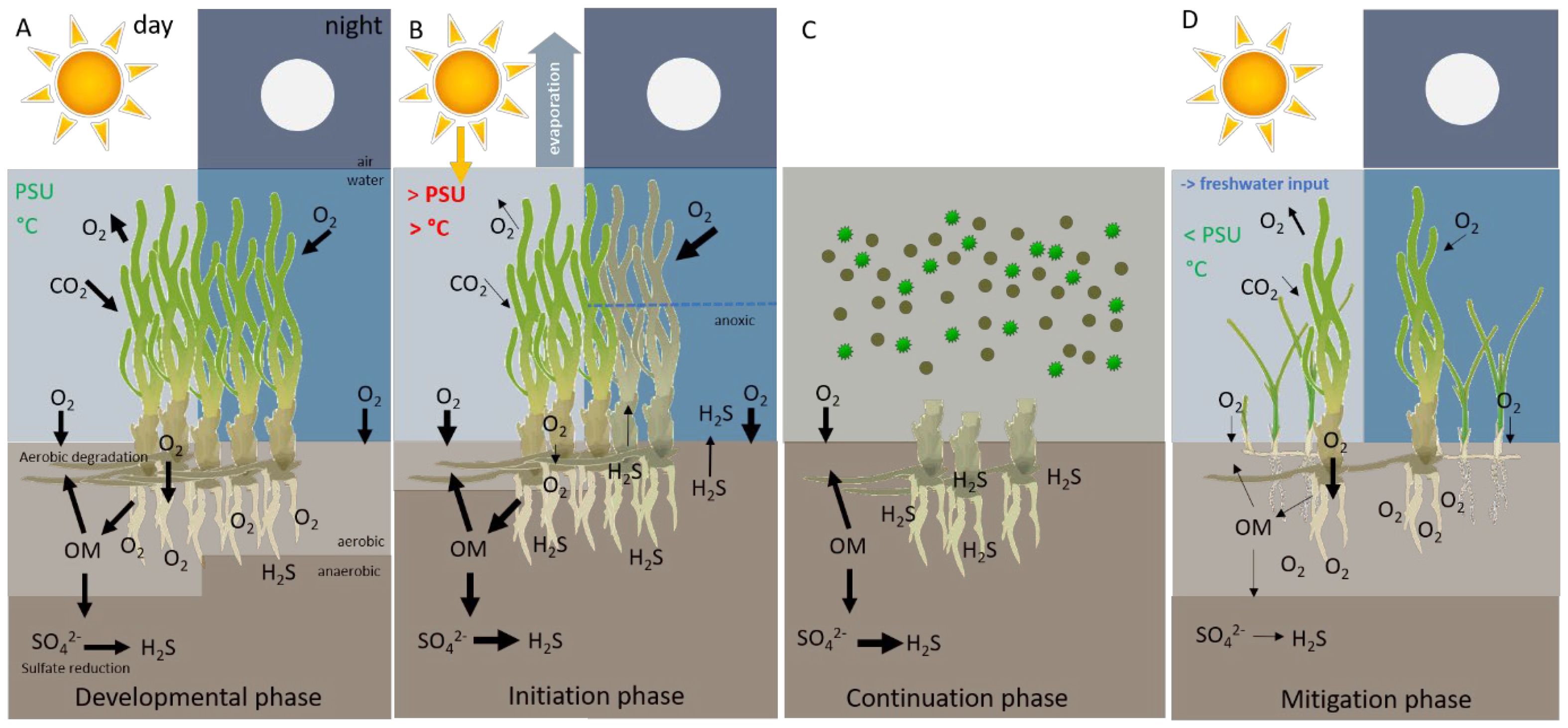
Figure 5 High light and high sediment nutrient concentrations create good growth conditions and dense meadows develop, leading to increased photosynthetically-derived oxygen that pressurizes aerenchymal tissues and supersaturates bottom waters, which drives ROL during the day and night. Dense meadows also increase labile organic material from both internal seagrass production and external import that encourages sulphide production and increases respiratory demand (A). The plant detoxification mechanism can be disrupted by high water temperatures, hypersalinity and or stratification that can compromise photosynthetic capacity, reduce oxygen concentrations in the bottom water and limit downward diffusive flux of oxygen into the sediment at night. In such cases, sulphide can outpace oxygen and causes higher sulphide intrusion in the plant meristem increasing plant mortality, leading to large die-off events (B). After the initial die-off, seagrass mortality increases turbidity and decreases water clarity through destabilization and re-suspension of sediments in the water column, as well as release of nutrients that can support algal blooms and further reduce irradiance (C). Restoration of historic increasing seasonal freshwater flow and flushing rates, enhance toxic conditions, limit hypersalinity and provide a more natural hydrologic and estuarine gradient into north-central Florida Bay. Potentially leading to less stress for macrophyte community, increase oxygen in the water column, leading to a lower risk of sulphide intrusion and promote a more heterogenous macrophyte community with more H wrightii and S. filiforme are believed to be less susceptible to environmental perturbations than communities dominated by monotopic of T. testudinum (D) (Based on Zieman, 1997, Koch et al., 2007d).
In central and western Florida Bay, cycles of periods of increasing T. testudinum density were punctuated by large die-offs and followed by seagrass succession and recovery of healthy T. testudinum meadows. High organic matter and sulphide is a characteristic of the dense T. testudinum meadows in central and western Florida Bay and may create a ‘time bomb effect’ ready to flood the T. testudinum meristems with toxic sulphide when conditions permit. This is similar to cyclic shifts in shallow lakes in Europe where accumulation of phosphorus creates an unsustainable condition (time bomb) due to slow internal eutrophication (van Nes et al., 2007). A recent study in a tropical intertidal seagrass system showed similar dynamics. Here a facultative lucinid-seagrass mutualism buffers sulphide toxicity by excessive accumulation of organic matter leading to slow-fast cycles, characterized by a long-term degraded- and seagrass dominated state, with fast transitions in between (de Fouw et al., 2018). In the Florida Bay system, the internal plant oxidation initially buffers sulphide intrusion (Figure 5A), but disruption of the internal oxidation feedback due to environmental perturbation eventually leads to bottom anoxia during the night and increased sulphide production by high organic matter, ending in sulphide intrusion and plant mortality (Figure 5B). Furthermore, seagrass mortality increases turbidity and decreases water clarity through destabilization and re-suspension of sediments in the water column, as well as release of nutrients that can support algal blooms and further reduce irradiance (Figure 5C). Which explains the slow recovery trajectory at impacted sites.
A specific goal of the Comprehensive Everglades Restoration Plan (CERP) by the South Florida Water Management District (SFWMD) and the U.S. Army Corps of Engineers is supplying additional water from the Everglades to Florida Bay via management of Everglades hydrology toward the regime historically present prior to Everglades drainage (see Madden et al. 2009). This is intended to increase seasonal freshwater flow and flushing rates, enhance oxic conditions, limit hypersalinity and provide a more natural hydrologic and estuarine gradient in north-central Florida Bay. This may lead to less stress for the benthic macrophyte community, increase oxygen in the water column, lower the risk of sulphide intrusion (Koch et al., 2007b; Fredley et al., 2019). For the upstream mangrove community, reduced saltwater intrusion should help maintain intact substrate (peat) and prevent nutrient and organic matter export to the downstream seagrass meadows. For the downstream seagrass community, restoration will promote a more heterogeneous macrophyte community (Fourqurean et al., 2003; Madden et al., 2009). Such mixed-species communities with more H. wrightii and S. filiforme are believed to be less susceptible to environmental perturbations than communities dominated by monotopic stands of T. testudinum (Figure 5D). In addition to altered hydrology, historic loss of megafaunal grazers such as green turtles and manatees (Chelonia mydas and Trichechus manatus) has been suggested to allow development of monospecific T. testudinum meadows with canopy high biomass in Florida Bay and the Caribbean (Jackson, 1997; Jackson et al., 2001). Very dense T. testudinum meadows with high ratio of below- to above-ground biomass have a very high oxygen demand and are less resilient to hypoxia then a heterogenous macrophyte community (Fourqurean et al., 2003; Fredley et al., 2019).
Apart from the human-altered hydrology, sea level rise has also increased saltwater intrusion into the Everglades freshwater ecosystems, altering the availability and distribution of nutrients and carbon (Charles et al., 2019; Servais et al., 2019). Historically, freshwater from the Everglades is not likely to increase nutrients in Florida Bay significancy, as N and P input from the Gulf of Mexico far exceeds Everglades input (Rudnick et al., 1999). However, as sea level rise, more organic material is exported from upstream freshwater marshes as saltwater intrusion cause substrate breakdown and peat collapse (Wilson et al., 2018). Further, as hurricane intensity and frequency has increased, peat collapse in coastal mangroves has resulted in turbidity plumes and additional nutrient mobility in the lower Everglades (Rodemann et al., 2021). This can affect the composition and concentration of nutrients reaching Florida Bay, potentially increasing algal blooms (Shangguan et al., 2017), reducing subsurface irradiance impairing the internal dynamics in T. testudinum meadows. Overall, sea level rise and hurricanes may thus play a significant role in shaping nutrient dynamics and ecosystem health in the transition from the Everglades to Florida Bay.
Increasing temperature due to climate change is another important challenge facing Florida Bay. In the summer of 2023 water temperatures in Florida Bay exceeded 38°C for an extended period (unpub. data C. Madden), well above the 33°C threshold for thermal breakdown of T. testudinum and H. wrightti physiological processes (Koch et al., 2007a). Temperature projections for the next 50 years for South Florida region are in the range of zero to +2.5°C, however precipitations projections show no clear pattern in the sign of change and range from -20 to +30% (Obeysekera et al., 2015). Climate change will probably increase water temperature and subsequently salinity levels due to increased evaporation, which will put more pressure on the internal dynamics of dense T. testudinum meadows. The potentially cumulative increased organic matter load in the western central part of the bay due to die-off events makes the meadows less resistant to climate change. Thus, restoration of the historic greater freshwater input from the Everglades may be imperative to maintain healthy seagrass meadows in the bay and help to reduce the risk of sudden die-offs.
Our study shows that the Florida Bay seagrass ecosystem continues to be susceptible to die-off. Local anthropogenic impact of the last decades has significantly changed the bay and in the combination with climate change, recurrent and severe die-offs events have been a direct result of this (Hall et al., 2016, 2021; Rodemann et al., 2021). There is now increasing evidence that coastal macrophyte communities rely on positive interactions (e.g. facilitation and mutualism), to reduce environmental stress (Bruno et al., 2003; Maxwell et al., 2017; Valdez et al., 2020; van der Heide et al., 2021). Recent studies have shown the importance of a facultative mutualistic interactions between bivalves and macrophytes in seagrass meadows and salt marshes during drought and increasing temperatures (Angelini et al., 2016; de Fouw et al., 2016, 2018). At the same time, however, disruption of positive interactions can exacerbate the coastal ecosystem degradation by compromising its ability to reduce stress (de Fouw et al., 2016; Strydom et al., 2020). Due to global change, disruption of plant oxidation mechanism may therefore become a more common phenomenon for the Florida Bay seagrass communities. Freshwater input, which favors a heterogenous macrophyte community, may reduce that risk. To better track progress toward this goal and to constrain estimates of critical thresholds, we suggest that monitoring programs consider additional in situ measurements such as primary production rates, porewater sulphide concentrations, bottom water temperatures, bottom- and pore-water salinities and diurnally resolved dissolved oxygen estimates to better understand, predict and prevent future community or even ecosystem collapse.
Data availability statement
The raw data supporting the conclusions of this article will be made available by the authors, without undue reservation.
Author contributions
JD: Conceptualization, Data curation, Formal analysis, Funding acquisition, Investigation, Methodology, Project administration, Resources, Software, Supervision, Validation, Visualization, Writing – original draft, Writing – review & editing. CM: Conceptualization, Data curation, Investigation, Methodology, Project administration, Resources, Supervision, Validation, Writing – review & editing. BF: Conceptualization, Data curation, Investigation, Methodology, Project administration, Resources, Validation, Writing – review & editing. MH: Data curation, Investigation, Resources, Validation, Writing – review & editing. YV: Investigation, Methodology, Resources, Writing – review & editing. SH: Investigation, Methodology, Resources, Writing – review & editing. TF: Conceptualization, Investigation, Methodology, Resources, Writing – review & editing. TS: Investigation, Resources, Writing – review & editing. MB: Investigation, Resources, Writing – review & editing. TV: Conceptualization, Funding acquisition, Investigation, Methodology, Resources, Supervision, Validation, Writing – review & editing.
Funding
The author(s) declare that financial support was received for the research, authorship, and/or publication of this article. The research was supported by NWO Open Competition #ALWOP.203 and NWO-Vidi Career no. 16588. NWO - The Dutch Research Council is the most important science funding bodies in the Netherlands and realizes quality and innovation in science.
Acknowledgments
We are grateful for technical assistance provided by Roy Peters and Sebastian Krosse for nutrient and elemental analysis. National Park (ENP) provided logistical support and permits supporting the field research.
Conflict of interest
The authors declare that the research was conducted in the absence of any commercial or financial relationships that could be construed as a potential conflict of interest.
Publisher’s note
All claims expressed in this article are solely those of the authors and do not necessarily represent those of their affiliated organizations, or those of the publisher, the editors and the reviewers. Any product that may be evaluated in this article, or claim that may be made by its manufacturer, is not guaranteed or endorsed by the publisher.
Supplementary material
The Supplementary Material for this article can be found online at: https://www.frontiersin.org/articles/10.3389/fmars.2024.1366939/full#supplementary-material
References
Angelini C., Griffin J. N., van de Koppel J., Lamers L. P. M., Smolders A. J. P., Derksen-Hooijberg M., et al. (2016). A keystone mutualism underpins resilience of a coastal ecosystem to drought. Nat. Commun. 7, 12473. doi: 10.1038/ncomms12473
Borum J., Pedersen O., Greve T. M., Frankovich T. A., Zieman J. C., Fourqurean J. W., et al. (2005). The potential role of plant oxygen and sulphide dynamics in die-off events of the tropical seagrass, Thalassia testudinum. J. Ecol. 93, 148–158. doi: 10.1111/j.1365-2745.2004.00943.x
Boyer J. N., Fourqurean J. W., Jones R. D. (1999). Seasonal and long-term trends in the water quality of Florida Bay, (1989–1997). Estuaries 22, 417–430. doi: 10.2307/1353208
Bruno J. F., Stachowicz J. J., Bertness M. D. (2003). Inclusion of facilitation into ecological theory. Trends Ecol. Evol. 18, 119–125. doi: 10.1016/S0169-5347(02)00045-9
Carlson P., Yarbro L., Barber T. (1994). Relationship of sediment sulfide to mortality of thalassia testudinum in Florida bay. Bull. Mar. Sci. 54, 733–746.
Charles S. P., Kominoski J. S., Troxler T. G., Gaiser E. E., Servais S., Wilson B. J., et al. (2019). Experimental saltwater intrusion drives rapid soil elevation and carbon loss in freshwater and brackish everglades marshes. Estuaries Coasts 42, 1868–1881. doi: 10.1007/s12237-019-00620-3
Childers D. L., Boyer J. N., Davis S. E., Madden C. J., Rudnick D. T., Sklar F. H. (2006). Relating precipitation and water management to nutrient concentrations in the oligotrophic “upside-down” estuaries of the Florida Everglades. Limnol. Oceanogr. 51, 602–616. doi: 10.4319/lo.2006.51.1_part_2.0602
Costanza R., dArge R., deGroot R., Farber S., Grasso M., Hannon B., et al. (1997). The value of the world’s ecosystem services and natural capital. Nature 387, 253–260. doi: 10.1038/387253a0
Costanza R., Pérez-Maqueo O., Martinez M. L., Sutton P., Anderson S. J., Mulder K. (2008). The value of coastal wetlands for hurricane protection. Ambio 37, 241–248. doi: 10.1579/0044-7447(2008)37[241:tvocwf]2.0.co;2
de Fouw J., Govers L. L., Van Belzen J., van de Koppel J., Dorigo W., Christianen M. J. A., et al. (2016). Drought, mutualism breakdown and landscape-scale degradation of seagrass beds. Curr. Biol. 26, 1051–1056. doi: 10.1016/j.cub.2016.02.023
de Fouw J., van der Heide T., van Belzen J., Govers L. L., Cheikh M. A. S., Olff H., et al. (2018). A facultative mutualistic feedback enhances the stability of tropical intertidal seagrass beds. Sci. Rep. 8, 12988. doi: 10.1038/s41598-018-31060-x
Durako M. J., Howarth J. F. (2017). Leaf Spectral Reflectance Shows Thalassia testudinum Seedlings More Sensitive to Hypersalinity than Hyposalinity. Front. Plant Sci. 8. doi: 10.3389/fpls.2017.01127
Fourqurean J. W., Boyer J. N., Durako M. J., Hefty L. N., Peterson B. J. (2003). Forecasting responses of seagrass distributions to changing water quality using monitoring data. Ecol. Appl. 13, 474–489. doi: 10.1890/1051-0761(2003)013[0474:FROSDT]2.0.CO;2
Fourqurean J. W., Duarte C. M., Kennedy H., Marba N., Holmer M., Mateo M. A., et al. (2012). Seagrass ecosystems as a globally significant carbon stock. Nat. Geosci. 5, 505–509. doi: 10.1038/ngeo1477
Fourqurean J. W., Robblee M. B. (1999). Florida Bay: A history of recent ecological changes. Estuaries 22, 345–357. doi: 10.2307/1353203
Fourqurean J. W., Zieman J. C., Powell G. V. N. (1992). Phosphorus limitation of primary production in Florida Bay: Evidence from C:N:P ratios of the dominant seagrass Thalassia testudinum. Limnol. Oceanogr. 37, 162–171. doi: 10.4319/lo.1992.37.1.0162
Fraser M. W., Kendrick G. A., Statton J., Hovey R. K., Zavala-Perez A., Walker D. I. (2014). Extreme climate events lower resilience of foundation seagrass at edge of biogeographical range. J. Ecol. 102, 1528–1536. doi: 10.1111/1365-2745.12300
Fredley J., Durako M. J., Hall M. O. (2019). Multivariate analyses link macrophyte and water quality indicators to seagrass die-off in Florida Bay. Ecol. Indic. 101, 692–701. doi: 10.1016/j.ecolind.2019.01.074
Hall M., Bell S., Furman B., Durako M. (2021). Natural recovery of a marine foundation species emerges decades after landscape-scale mortality. Sci. Rep. 11, 6973. doi: 10.1038/s41598-021-86160-y
Hall M. O., Durako M. J., Fourqurean J. W., Zieman J. C. (1999). Decadal changes in seagrass distribution and abundance in Florida Bay. Estuaries 22, 445–459. doi: 10.2307/1353210
Hall M. O., Furman B. T., Merello M., Durako M. J. (2016). Recurrence of Thalassia testudinum seagrass die-off in Florida Bay: initial observations. Mar. Ecol. Prog. Ser. 560, 243–249. doi: 10.3354/meps11923
Hansen J. C. R., Reidenbach M. A. (2012). Wave and tidally driven flows in eelgrass beds and their effect on sediment suspension. Mar. Ecol. Prog. Ser. 448, 271–287. doi: 10.3354/meps09225
Heck K. L., Hays G., Orth R. J. (2003). Critical evaluation of the nursery role hypothesis for seagrass meadows. Mar. Ecol. Prog. Ser. 253, 123–136. doi: 10.3354/meps253123
Jackson J. B. C., Kirby M. X., Berger W. H., Bjorndal K. A., Botsford L. W., Bourque B. J., et al. (2001). Historical overfishing and the recent collapse of coastal ecosystems. Science 293, 629–638. doi: 10.1126/science.1059199
Johnson C. R., Koch M. S., Pedersen O., Madden C. J. (2018). Hypersalinity as a trigger of seagrass (Thalassia testudinum) die-off events in Florida Bay: Evidence based on shoot meristem O2 and H2S dynamics. J. Exp. Mar. Biol. Ecol. 504, 47–52. doi: 10.1016/j.jembe.2018.03.007
Johnson C. R., Koch M. S., Pedersen O., Madden C. J. (2020). Hypersalinity affects leaf and meristem O2 dynamics exposing meristems to H2S in the dominant tropical seagrass Thalassia testudinum. J. Exp. Mar. Biol. Ecol. 533, 151458. doi: 10.1016/j.jembe.2020.151458
Jørgensen B. B. (1982). Mineralization of organic matter in the sea bed—the role of sulphate reduction. Nature 296, 643–645. doi: 10.1038/296643a0
Kavanagh C. (2016). 2015 Florida Bay seagrass die-off. South Florida Natural Resour. Center. Available at: https://www.nps.gov/ever/learn/nature/sfnrc-publications.htm.
Koch M. S., Erskine J. M. (2001). Sulfide as a phytotoxin to the tropical seagrass Thalassia testudinum: interactions with light, salinity and temperature. J. Exp. Mar. Biol. Ecol. 266, 81–95. doi: 10.1016/S0022-0981(01)00339-2
Koch M. S., Johnson C. R., Madden C. J., Pedersen O. (2022). Low irradiance disrupts the internal O2 dynamics of seagrass (Thalassia testudinum) leading to shoot meristem H2S intrusion. Aquat. Bot. 181, 103532. doi: 10.1016/j.aquabot.2022.103532
Koch M. S., Schopmeyer S. A., Holmer M., Madden C. J., Kyhn-Hansen C. (2007b). Thalassia testudinum response to the interactive stressors hypersalinity, sulfide and hypoxia. Aquat. Bot. 87, 104–110. doi: 10.1016/j.aquabot.2007.03.004
Koch M. S., Schopmeyer S., Kyhn-Hansen C., Madden C. J. (2007a). Synergistic effects of high temperature and sulfide on tropical seagrass. J. Exp. Mar. Biol. Ecol. 341, 91–101. doi: 10.1016/j.jembe.2006.10.004
Koch M. S., Schopmeyer S. A., Kyhn-Hansen C., Madden C. J., Peters J. S. (2007c). Tropical seagrass species tolerance to hypersalinity stress. Aquat. Bot. 86, 14–24. doi: 10.1016/j.aquabot.2006.08.003
Koch M. S., Schopmeyer S. A., Nielsen O. I., Kyhn-Hansen C., Madden C. J. (2007d). Conceptual model of seagrass die-off in Florida Bay: Links to biogeochemical processes. J. Exp. Mar. Biol. Ecol. 350, 73–88. doi: 10.1016/j.jembe.2007.05.031
Lamers L. P. M., Govers L. L., Janssen I. C. J. M., Geurts J. J. M., van der Welle M. E. W., Van Katwijk M. M., et al. (2013). Sulfide as a soil phytotoxin - A review. Front. Plant Sci. 4. doi: 10.3389/fpls.2013.00268
Lee T., Johns E., Melo N., Smith R., Ortner P., Smith D. (2006). On Florida Bay hypersalinity and water exchange. Bull. Mar. Sci. 79, 301–327.
Lee T., Melo N., Johns E., Kelble C., Smith R., Ortner P. (2008). On water renewal and salinity variability in the northeast subregion of Florida Bay. Bull. Mar. Sci. 82, 83–105.
Lee T., Melo N., Smith N., Johns E., Kelble C., Smith R., et al. (2016). Circulation and water renewal of Florida Bay, USA. Bull. Mar. Sci. -Miami- 92, 153–180. doi: 10.5343/bms.2015.1019
MacLeod K., Koch M. S., Johnson C. R., Madden C. J. (2023). Resilience of recruiting seagrass (Thalassia testudinum) to porewater H2S in Florida Bay. Aquat. Bot. 187, 103650. doi: 10.1016/j.aquabot.2023.103650
Madden C. J. (2013). Use of models in ecosystem-based management of the southern Everglades and Florida Bay, Florida (College Station, TX: Texas A&M University Press).
Madden C. J., Rudnick D. T., McDonald A. A., Cunniff K. M., Fourqurean J. W. (2009). Ecological indicators for assessing and communicating seagrass status and trends in Florida Bay. Ecol. Indic. 9, S68–S82. doi: 10.1016/j.ecolind.2009.02.004
Marshall F. E., Bernhardt C. E., Wingard G. L. (2020). Estimating late 19th century hydrology in the greater everglades ecosystem: an integration of paleoecologic data and models. Front. Environ. Sci. 8, 1449–1466. doi: 10.3389/fenvs.2020.00003
Marshall F., Wingard G., Pitts P. (2014). Estimates of natural salinity and hydrology in a subtropical estuarine ecosystem: implications for greater everglades restoration. Estuaries Coasts 37. doi: 10.1007/s12237-014-9783-8
Maxwell P. S., Eklöf J. S., Katwijk M. M., O’Brien K., de la Torre-Castro M., Boström C., et al. (2017). The fundamental role of ecological feedback mechanisms for the adaptive management of seagrass ecosystems - a review. Biol. Rev. 92, 1521–1538. doi: 10.1111/brv.12294
Nuttle W. K., Fourqurean J. W., Cosby B. J., Zieman J. C., Robblee M. B. (2000). Influence of net freshwater supply on salinity in Florida Bay. Water Resour. Res. 36, 1805–1822. doi: 10.1029/1999WR900352
Obeysekera J., Barnes J., Nungesser M. (2015). Climate sensitivity runs and regional hydrologic modeling for predicting the response of the greater Florida Everglades ecosystem to climate change. Environ. Manage 55, 749–762. doi: 10.1007/s00267-014-0315-x
Orth R. J., Carruthers T. J. B., Dennison W. C., Duarte C. M., Fourqurean J. W., Heck K. L., et al. (2006). A global crisis for seagrass ecosystems. Bioscience 56, 987–996. doi: 10.1641/0006-3568(2006)56[987:agcfse]2.0.co;2
Pedersen O., Binzer T., Borum J. (2004). Sulphide intrusion in eelgrass (Zostera marina L.). Plant Cell Environ. 27, 595–602. doi: 10.1111/j.1365-3040.2004.01173.x
Peñalver M. M., Durako M., Furman B. T., Hall M. (2020). Multiple stressors result in reduced reproductive effort by Thalassia testudinum in Florida Bay, USA. Mar. Ecol. Prog. Ser. 647, 65–78. doi: 10.3354/meps13395
R Core Team version 4.1.1 (2021). R: A language and environment for statistical computing (Vienna, Austria: R Foundation for Statistical Computing).
Robblee M. B., Barber T., Carlson P. Jr., Durako M., Fourqurean J. W., Muehlstein L., et al. (1991). Mass mortality of the tropical seagrass Thalassia testudinum in Florida Bay (USA). Mar. Ecol. Prog. Ser. 71, 297–299. doi: 10.3354/meps071297
Rodemann J., James W. R., Rolando O., Furman B., Fratto Z., Bautista V., et al. (2021). Impact of extreme disturbances on suspended sediment in western Florida Bay: implications for seagrass resilience. Front. Mar. Sci. 8. doi: 10.3389/fmars.2021.633240
Rudnick D., Chen Z., Childers D., Fontaine T. (1999). Phosphorus and nitrogen inputs to Florida Bay: the importance of the everglades watershed. Estuaries 22, 398–416. doi: 10.2307/1353207
Sandoval-Gil J. M., Ruiz J. M., Marín-Guirao L. (2023). Advances in understanding multilevel responses of seagrasses to hypersalinity. Mar. Environ. Res. 183, 105809. doi: 10.1016/j.marenvres.2022.105809
Schmidt T. W. (2002). 1979 ecological study of fishes and the water quality characteristics of Florida Bay, Everglades National Park, Florida.
Servais S., Kominoski J. S., Charles S. P., Gaiser E. E., Mazzei V., Troxler T. G., et al. (2019). Saltwater intrusion and soil carbon loss: Testing effects of salinity and phosphorus loading on microbial functions in experimental freshwater wetlands. Geoderma 337, 1291–1300. doi: 10.1016/j.geoderma.2018.11.013
Shangguan Y., Glibert P. M., Alexander J., Madden C. J., Murasko S. (2017). Phytoplankton assemblage response to changing nutrients in Florida Bay: Results of mesocosm studies. J. Exp. Mar. Biol. Ecol. 494, 38–53. doi: 10.1016/j.jembe.2017.05.006
Strydom S., Murray K., Wilson S., Huntley B., Rule M., Heithaus M., et al. (2020). Too hot to handle: Unprecedented seagrass death driven by marine heatwave in a World Heritage Area. Global Change Biol. 26, 3525–3538. doi: 10.1111/gcb.15065
Valdez S. R., Zhang Y. S., van der Heide T., Vanderklift M. A., Tarquinio F., Orth R. J., et al. (2020). Positive ecological interactions and the success of seagrass restoration. Front. Mar. Sci. 7. doi: 10.3389/fmars.2020.00091
van der Heide T., Angelini C., de Fouw J., Eklöf J. S. (2021). Facultative mutualisms: A double-edged sword for foundation species in the face of anthropogenic global change. Ecol. Evol. 11, 29–44. doi: 10.1002/ece3.7044
van der Heide T., Govers L. L., de Fouw J., Olff H., van der Geest M., van Katwijk M. M., et al. (2012). A three-stage symbiosis forms the foundation of seagrass ecosystems. Science 336, 1432–1434. doi: 10.1126/science.1219973
van der Heide T., van Nes E. H., Geerling G. W., Smolders A. J. P., Bouma T. J., van Katwijk M. (2007). Positive feedbacks in seagrass ecosystems: Implications for success in conservation and restoration. Ecosystems 10, 1311–1322. doi: 10.1007/s10021-007-9099-7
van Nes E. H., Rip W. J., Scheffer M. (2007). A theory for cyclic shifts between alternative states in shallow lakes. Ecosystems 10, 17–27. doi: 10.1007/s10021-006-0176-0
Waycott M., Duarte C. M., Carruthers T. J. B., Orth R. J., Dennison W. C., Olyarnik S., et al. (2009). Accelerating loss of seagrasses across the globe threatens coastal ecosystems. Proc. Natl. Acad. Sci. 106, 12377–12381. doi: 10.1073/pnas.0905620106
Wilson B. J., Servais S., Mazzei V., Kominoski J. S., Hu M., Davis S. E., et al. (2018). Salinity pulses interact with seasonal dry-down to increase ecosystem carbon loss in marshes of the Florida Everglades. Ecol. Appl. 28, 2092–2108. doi: 10.1002/eap.1798
Zieman J. C. (1997). Florida Bay seagrass die-off. The Florida Keys Environmental Story, A Panorama of the Environment, Culture and History of Monroe County, Florida. Seacamp Association.
Zieman J. C., Fourqurean J. W., Frankovich T. A. (1999). Seagrass die-off in Florida Bay: Long-term trends in abundance and growth of turtle grass, Thalassia testudinum. Estuaries 22, 460–470. doi: 10.2307/1353211
Zieman J., Fourqurean J. W., Iverson R. L. (1989). Distribution, abundance and productivity of seagrasses and macroalgae in Florida Bay. Bull. Mar. Sci. 44, 292–311.
Keywords: Florida bay, climate change, hypoxia, sulphide toxicity, seagrass die-off, turtlegrass Thalassia testudinum
Citation: de Fouw J, Madden CJ, Furman BT, Hall MO, Verstijnen Y, Holthuijsen S, Frankovich TA, Strazisar T, Blaha M and Van Der Heide T (2024) Reduced seagrass resilience due to environmental and anthropogenic effects may lead to future die-off events in Florida Bay. Front. Mar. Sci. 11:1366939. doi: 10.3389/fmars.2024.1366939
Received: 07 January 2024; Accepted: 10 June 2024;
Published: 15 July 2024.
Edited by:
Stelios Katsanevakis, University of the Aegean, GreeceReviewed by:
Gidon Winters, Dead Sea and Arava Science Center, IsraelJohn Lehrter, University of South Alabama, United States
Copyright © 2024 de Fouw, Madden, Furman, Hall, Verstijnen, Holthuijsen, Frankovich, Strazisar, Blaha and Van Der Heide. This is an open-access article distributed under the terms of the Creative Commons Attribution License (CC BY). The use, distribution or reproduction in other forums is permitted, provided the original author(s) and the copyright owner(s) are credited and that the original publication in this journal is cited, in accordance with accepted academic practice. No use, distribution or reproduction is permitted which does not comply with these terms.
*Correspondence: Jimmy de Fouw, amltLmRlZm91d0BydS5ubA==
†Present address: Sander Holthuijsen, Rijkswaterstaat, Ministry of Infrastructure and Water Management, The Hague, The Netherlands