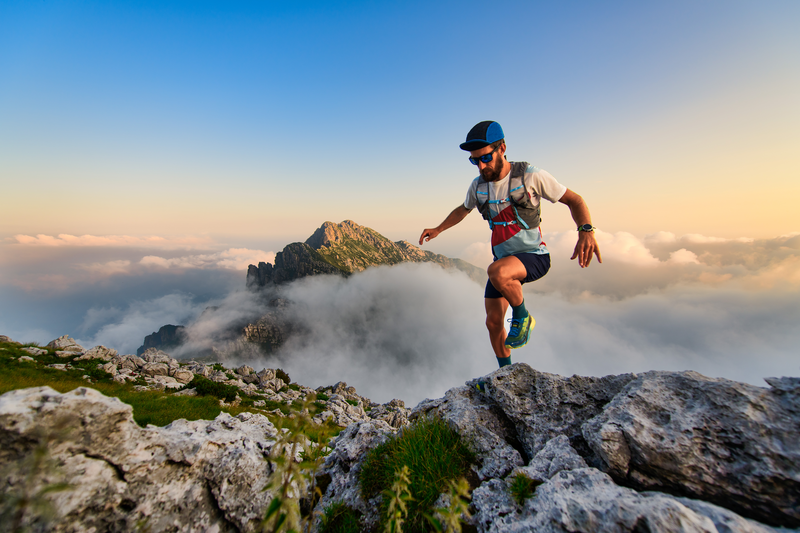
94% of researchers rate our articles as excellent or good
Learn more about the work of our research integrity team to safeguard the quality of each article we publish.
Find out more
REVIEW article
Front. Mar. Sci. , 23 January 2025
Sec. Global Change and the Future Ocean
Volume 11 - 2024 | https://doi.org/10.3389/fmars.2024.1363863
This article is part of the Research Topic ICYMARE - Early Career Researchers in Marine Science View all 32 articles
Increasing global temperatures, biodiversity loss, and pollution are among the major transformative changes affecting terrestrial and marine ecosystems. The marine biome can be observed and monitored using ocean observations with satellite-based platforms, tagging of animals, autonomous platforms and vehicles, and ship-based measurements. Sustaining ocean observations at a given location over time are known as ocean time series. For example, stationary platforms like moorings record data autonomously at one location over time, while ship-based time series stations are sampled using discrete measurements at varying sampling frequencies throughout a given year. Ocean observations feed into networks that either focus on obtaining data, e.g., similar instruments, or a social focus that aims to connect participants, e.g., early career or science-policy interface. These platforms and associated networks feed into the Framework for Ocean Observing (FOO) with a set of Essential Ocean Variables (EOVs) and the Ocean Best Practices System (OBPS) developed by the global ocean observing community. The range of ocean observing activities around the world creates a complex landscape, which can be particularly difficult to navigate for early career ocean professionals (ECOPs). ECOPs face higher barriers to entering the United Nations Decade of Ocean Science for Sustainable Development (“UN Ocean Decade”) than others despite actively contributing to ocean observations and playing the most significant role in the success of sustainability transformations. The review aims to provide an overview of the organizations and networks associated with ocean observations, motivate ECOPs to actively get involved in the ongoing UN Ocean Decade (2021-2030), and join or create new initiatives within the existing landscape. Ocean observations harbor an extraordinary benefit for a large diversity of end-users far beyond the scientific community, and there is a need to engage the next generation of ocean leaders as we transition to live and manage this blue planet sustainably.
The oceans cover 70% of the Earth’s surface and extend an average of 4000 meters below sea level. The fascination with the ocean has persisted to the test of time, from the history of navigation to the Challenger expedition that marked the start of modern oceanography in the late 19th Century. Since then, modern research efforts have advanced considerably and made attempts at estimating oceanic element budgets and diversity, such as the Tara Oceans expeditions (Sunagawa et al., 2015) or Ocean Sampling Day (Kopf et al., 2015). Regardless, the oceans remain largely undersampled. The increasing efforts of large-scale and sustained ocean observations are revealing the complexity of small-scale oceanographic dynamics and variability in marine ecosystems.
Oceanic processes and marine life naturally vary across spatial and temporal scales, which are best studied using ocean observations (Figure 1). Ocean observations are made with a diverse range of platforms that can be classified into four categories: autonomous remote sensing, autonomous drifting devices, autonomous fixed or stationary devices, and discrete sampling. Remote sensing satellites provide a high spatial and temporal resolution, but only at the ocean surface. Within the ocean, autonomous drifting or tagged devices can resolve the spatial variability at the ocean surface to 2000 meters depth (e.g., Argo floats or Autonomous Underwater Vehicles) and track marine animals (Visser et al., 2021), while autonomous stationary platforms can resolve the temporal variability (i.e., moorings or anchored buoys). Autonomous observations are typically operated using standardized protocols for a range of sensors to monitor physical and some biological and chemical variables, such as temperature, salinity, oxygen, and fluorescence for phytoplankton biomass (Boss et al., 2022). In addition, stationary platforms can also be coupled with autonomous sampling devices to sample for a wider range of variables, such as sediment traps or remote access samplers (RAS). However, many variables, particularly biological and ecological variables, require visual monitoring and discrete hand-sampling of the water column or sediment to survey the spatiotemporal variability. Both, autonomous and discrete ocean observations, rely on trained personnel and major scientific infrastructures. Research vessels provide an essential observation infrastructure across scientific disciplines to deploy a variety of oceanographic instruments (Nieuwejaar and Mazauric, 2019). Modern research vessels host a range of permanently installed sensors and handling capabilities of mobile instruments that include the conductivity-temperature-depth profiler (CTD) coupled to a rosette that provides discrete water samples, towed seismic equipment, box- and multicorer (MUC) systems that provide discrete sediment samples, and nets that passively collect megafauna in the water column or the seafloor (see Paulus, 2021). In 2019, European countries operated 99 research vessels with a greater number and easier access to coastal/local classes (approx. five scientific births) compared to ocean and global classes (>20 scientific births) (Nieuwejaar and Mazauric, 2019).
Figure 1. Temporal and spatial scales for a variety of ocean processes and oceanographic platforms. Adapted from O'Brien et al. (2017), copyright UNESCO, with permission. AUVs, Autonomous Underwater Vehicles.
Repeating observations at a given location over time are known as time series (Box 1). Ocean time series are temporally resolved datasets that are valuable in characterizing ocean climate, biogeochemistry, and ecosystem change (International Group for Marine Ecological Time Series, 2017). The ocean climate and biogeochemistry can be monitored using autonomous platforms, like moorings, while ecosystem changes still crucially rely on monitoring at ship-based time series that, in the case of open ocean time series, can be registered with the OceanSITES global network. Examples of these long-term open-ocean time series sites that combine autonomous and ship-based time series include: Bermuda Atlantic Time-series Study (BATS), DYFAMED Observatory, Hawaii Ocean Time Series (HOT), HAUSGARTEN Observatory, Porcupine Abyssal Plain (PAP), Santander Atlantic Time-Series (SATS), and the Southern Ocean Time-Series (SOTS). These combined sites emphasize the value of cross-validation and allow for the highest degree of observation, while typically also reducing the observation bias of meso- and bathypelagic zones that are also affected by anthropogenic climate change (González-Dávila and Santana-Casiano, 2023).
Box 1. The definition of a time series
Time series are temporally resolved datasets that provide one of the most valuable tools scientists have to characterize ocean climate, biogeochemistry, and ecosystem change. A single time series is only relevant at a local scale, with a limited potential for extrapolation to a larger region. However, if multiple time series are combined, regional and global variability can be examined using large spatial-scale analyses to improve our understanding of marine ecosystem change. Time series harbor an extraordinary and currently unexploited strength in numbers.
International Group for Marine Ecological Time Series (IGMETS)
Open ocean fixed time series are located in all ocean basins (Figure 2A), but most ship-based ecological time series are more predominant in shallow coastal areas (Figure 2B). However, not all ship-based ecological time series are currently not actively curated in a global database and have to be independently retrieved to perform specific metanalyses (Raes et al., 2024). Previously, efforts of the International Group for Marine Ecological Time Series (IGMETS) demonstrated the collective value of integrating and assessing ship-based time series (O’Brien et al., 2017). Merging the catalogs from IGMETS marine ecological time series metadata (METabase), EuroSea Project (Ratnarajah, 2021), the International Ocean Carbon Coordination Project network (IOCCP, April 2016), and Buttigieg et al. (2018) showed that 54% of time series stations are located in the Atlantic Ocean, which includes the English Channel, Irish Sea, and North Sea (Supplementary Table 1). Next, time series stations exist in the Arctic Ocean ~14%, the Baltic Sea ~12%, the Mediterranean Sea (incl. the Black Sea) ~9%, the Pacific Ocean ~8%, the Indian Ocean, and the Southern Ocean <2% (Figure 3; Supplementary Table 1). The accessibility of the station often pre-determines the sampling frequency, which ranges from daily sampling events ~2% to annual sampling campaigns ~24%, although ~5% were listed as variable and unknown (Figure 3). Monitoring Essential Ocean Variables (EOVs) and standardizing sampling protocols would raise the comparability of global datasets since ~82% of ship-based time series observe temporal variability of the pelagic realm with a strong focus on phytoplankton, zooplankton, and microbes (here, bacteria and archaea; Figure 3).
Figure 2. Map of (A) fixed and (B) ship-based time series stations around the world. The fixed ocean stations were based on the OceanSITES network from January 2024. Ship-based time series are based on the marine ecological time series metadata (METabase) from the International Group for Marine Ecological Time Series (IGMETS), EuroSea project database (Ratnarajah, 2021), and the International Ocean Carbon Coordination Project (IOCCP, April 2016), see Supplementary Table 1.
Figure 3. Sampling strategies of ship-based time series. The (A) location by ocean basin, (B) sampling frequency, (C) focus on the pelagic or benthic realm, and (D) ecological focus (pelagic only). The data is based on the marine ecological time series metadata (METabase) from the International Group for Marine Ecological Time Series (IGMETS), EuroSea project database (Ratnarajah, 2021), International Ocean Carbon Coordination Project (IOCCP, April 2016), and Buttigieg et al. (2018).
Monitoring the natural variability and health of the ocean is becoming more pressing than ever with increasing warming, acidification, pollution, eutrophication, and hypoxic events (Gruber, 2011). Ocean time series are central in quantifying the temporal variability with an additional number of benefits from testing new technologies, conducting in situ experiments within a context of known environmental variability, validating autonomous and remote platforms used in forecasting systems, to informing policy decisions (Karl, 2010; Neuer et al., 2017; O’Brien et al., 2017). Currently, data discoverability and synthesis products limit the full scientific potential of ship-based time series, but efforts are being made to close this gap (Lange et al., 2023, Lange et al., 2024). The scientific needs and future policies for a sustainable ocean are currently being addressed as part of the United Nations Decade of Ocean Science for Sustainable Development (henceforth UN Ocean Decade) between 2021-2030.
Ocean observations typically begin with producing data that feeds into databases, which can then be used for communication and eventually result in end-user interoperability as science-based policy, education, and technology development. User benefits vary based on the key sector, such as safety through monitoring and managing the ocean, food safety, or tourism.
Ocean observations are financially supported through individual projects, technical infrastructure services, or a combination of both. Individual projects are usually hypothesis-driven research questions for a limited time of two to five years. Monitoring ocean time series with project-based funding could cover additional personnel costs, while a drawback would be the focus on short time scales due to the project’s timeline. Sustaining ocean time series with multiple projects can be highly subject to personnel turnover and increased likelihood of sampling gaps that can compromise the utility of datasets and assessments of ecosystem health (Benway et al., 2019). Instead, long-term funding might cover analytical services and maintaining technical infrastructure through observation programs and research infrastructures (RI). An observation program or RI tends to financially support measurements of a defined set of EOVs at national or international partner research institutions. The major advantage of an infrastructure-based funding strategy is long-term monitoring efforts of ecosystem health and benefits to the community at large. A drawback of an infrastructure-based national funding strategy could restrict funding for out-of-the-box studies. In both cases, ocean time series contribute to observation networks and provide evidence-based datasets that can enable policymakers to make well-informed decisions about marine management (Giering et al., 2022).
Networks bundle and connect parts according to similarity. In the ocean, networks connect ocean observations on a national, regional, and global level. Ocean observing networks typically align around three dimensions: platform-based observing networks, thematic networks, and scale-based networks (Tanhua et al., 2019). Additionally, programs and RIs create internal network structures that can be part of an observation network and network-of-networks. The intrinsic complexity of the ocean observations was visualized by the European Observatory of Earth Observation Networks (ENEON) in-situ network graph (Masó et al., 2020; ENEON, 2020), which has been simplified and focused on ocean time series (Figure 4). The landscape of ocean observations and observation networks is complex because contributions to many of the existing networks rely on voluntary submission and are coordinated by scientists in a bottom-up manner (Tanhua et al., 2019).
Figure 4. The complex landscape of ocean observations infrastructures and networks. The networks are arranged on local, national, regional, and global levels, aiming to inform end-user community actions and raise ocean health and sustained observations. Please note that the figure does not aim to show a complete representation but works exemplarily based on the networks elaborated under the ConnectinGEO H2020 (ENEON, 2020). Abbreviations include (from top-to-bottom): National Institute for Space Research (portuguese INPE) in Brazil, National Centre for Scientific Research (french CNRS) in France, Federal Ministry of Education and Research (ger. BMBF) in Germany, National Oceanic and Atmospheric Administration (NOAA) in the USA, Mercator Ocean International (MOi), North Pacific Marine Science Organisation (PICES), Group on Earth Observations (GEO), International Council for the Exploration of the Sea (ICES), Scientific Committee on Oceanic Research (SCOR), Integrated Marine Observing System (IMOS) in Australia, US Integrated Ocean Observing System (IOOS) in the USA, Joint Programming Initiative (JPI), Surface Ocean - Lower Atmosphere Study (SOLAS), Coastal Monitoring Network (CoastNET) in Portugal, Mediterranean Ocean Observing System for the Environment (MOOSE) in France, Ocean Observatories Initiative (OOI) in the USA, European Multidisciplinary Seafloor and water column (EMSO) and European Marine Biological Resource Centre (EMBRC) in Europe, Oceanic Research Institute (ORI) in Australia, German Alliance for Marine Research (ger. DAM) in Germany, Ocean Carbon and Biogeochemistry Program (OCB) in the USA, Irish Ocean Literacy Network (IOLN) in Ireland, GEO European Marine Omics Biodiversity Observation Network (GEO EMO BON), Deep-Ocean Stewardship Initiative (DOSI) in the UK, Marine Biodiversity Observation Network (MBON) in the USA, Group on Earth Observations Biodiversity Observations Network (GEO BON), European Long-Term Ecosystem and socio-ecological Research Infrastructure (eLTER), All-Atlantic Ocean Observing System (AtlantOS), European Global Ocean Observing System (EuroGOOS), Southern Ocean Observing System (SOOS), Indian Ocean GOOS (IOGOOS), German Marine Research Consortium (ger. KDM) in Germany, Helsinki Commission (HELCOM), European Marine Board (EMB), European Ocean Observing System (EOOS), Partnership for Observation of the Global Ocean (POGO), Global Earth Observation System of Systems (GEOSS).
The first dimension is that of platform-based observing networks, which act on a global scale (Figure 4). Observing networks contribute to the Ocean Coordination Group (OCG), which monitors and reports on the progress of global ocean observations to the Global Ocean Observing System (GOOS, Table 1). GOOS is organized into a steering committee, expert panels developing EOVs, regional alliances to integrate national needs into regional systems, and the OCG; for details, please see GOOS (2024) and Moltmann et al. (2019). GOOS endorses the Framework for Ocean Observing (FOO), which provides guidelines for the processes, requirements settings, and best practices in ocean observation (Tanhua et al., 2019; McCurdy, 2023). Hence, the OCG endorsed observing networks all operate under well-defined criteria and best practices to promote open data sharing. The 13 platform-based observing networks that contribute to GOOS OCG include:
● Argo
● Data Buoy Cooperation Panel (DBCP)
● Ship Observations Team (SOT)
● Ship-of-Opportunity Program (SOOP)
● Automated Shipboard Aerological Program (ASAP)
● Voluntary Observing Ships (VOS)
● Global Sea Level Observing System (GLOSS)
● Animal-Borne Ocean Sensors (AniBOS)
● Global Ocean Ship-Based Hydrographic Investigations Program (GO-SHIP)
● OceanSITES
● The Global High Frequency Radar Network (HFRNet)
● Ocean Gliders
● Tsunami Buoys
Table 1. Common abbreviations of terms of best practices, organizations, and networks related to ocean observations.
The second dimension is that of thematic observation networks, which center around a broader and multidisciplinary audience across geographic areas. For example, the Group on Earth Observations Biodiversity Observation Networks (GEO BON) is a large international network of networks of experts that monitor biodiversity to improve the acquisition, delivery, and application of information on biodiversity change for decision-making and allocation processes. Within GEO BON, the thematic global BONs include Marine Biodiversity Observation Network (MBON), Freshwater Observing System (FWBON), Soil Biodiversity Observation Network (SoilBON), and Omic Biodiversity Observation Network (OmicBON). The BON networks operate within a set of essential biodiversity variables (EBVs) that are used to identify indicators for biodiversity (Zilioli et al., 2021; GEOBON, 2023). Additionally, national BONs monitor biodiversity and plan for a global biodiversity observing system (GBiOS) that could interlink existing capacities and organizations in the national governments (Gonzalez et al., 2023). In general, thematic ocean observation networks serve to communicate a consensus about ocean health from the international scientific community within a specific field to inform policy and accelerate actions. Examples of thematic ocean observation networks include (excluding internal programs and RI networks):
● GEO BON Marine Biodiversity Observation Network (MBON) focuses on the effective management of marine biodiversity and ecosystem services
● Global Alliance of Continuous Plankton Recorder Surveys (GACS)/Continuous Plankton Recorder focuses on environmental changes in plankton biodiversity
● Ocean Tracking Network focuses on animal tracking
● Chlorophyll Globally Integrated Network (ChloroGIN) focuses on ocean color and related satellite observations
● Global Ocean Acidification Observing Network (GOA-ON) focuses on the drivers of ocean acidification
● Deep Ocean Observing Strategy (DOOS) is a network of networks that focuses on the research and modeling community as well as maturing EOVs at the deep ocean
The third dimension is that of scale-based observation networks, which operate on the national, basin, and regional levels (Figure 4). This third dimension is among the most diverse as networks can indirectly stem from currently or formerly funded projects, programs, or RIs that may still hold a residual active community funding and international non-profit associations. Therefore, funding can play a critical role in observing networks that also impact the network’s governance. Network governance can range from relatively strong governance at a national level to little or no governance across nations. Although regional observation networks might share many interests, e.g., local oceanography, fisheries biodiversity, and pollution, transboundary governance is often bound to a limiting factor (Tanhua et al., 2019). Instead, much greater success has been seen with observation networks that focus on specific ocean basins due to the wider resources provided by several nations to achieve observing strategies. Examples of scale-based ocean observation and coordination networks include (active and discontinued):
● National scale
○ Ocean Carbon and Biogeochemistry (OCB) program is a US-based network where the staff coordinate and facilitate activities relevant to carbon cycle science, climate, and global change issues (Benway and Doney, 2014). OCB is a valuable resource and organizes webinars, workshops, and a monthly newsletter.
○ National nodes of the International Long Term Ecological Research Network (ILTER) that focuses on long-term, site-based research, and monitoring of marine biome but also alpine, forests, grasslands, lakes, and rivers across one country.
● Regional scale
○ ANTARES project focused on coastal ecosystems in sites around Latin America (2012-2017).
○ European Marine Omics Biodiversity Observation Network (EMO BON), launched by the European Marine Biological Resource Centre (EMBRC), focuses on the European contribution to global genomic observation efforts (Santi et al., 2023).
○ A regional network of the International Long Term Ecological Research Network (ILTER), e.g., Long-Term Ecosystem Research in Europe (LTER-Europe), identifies drivers of ecosystem change across European environmental and economic gradients (Mollenhauer et al., 2018).
● Basin-scale
○ GOOS Regional Alliances (GRA) integrate national needs into regional systems, e.g., the Southern Ocean Observing System (SOOS) (Newman et al., 2019).
○ All-Atlantic Ocean Observing System (AtlantOS) was a former EU Horizon 2020 project that focused on multidisciplinary observations in the North and South Atlantic.
○ Tropical Pacific Observing System (TPOS) focuses on USVs, moored arrays, and BGC-Argo observations in the tropical band of the Pacific Ocean (Smith et al., 2019).
○ Mediterranean Ocean Observing System for the Environment (MOOSE) is part of the French National Observation Services (fr. Services Nationaux d’Observation), which focuses on monitoring the temporal and spatial processes between the atmosphere and coastal-open ocean components in the North-Western Mediterranean basin.
To support long-term ocean observations, nations can tailor their funding strategy towards observing programs. Programs are large inter- and multidisciplinary projects across national research institutions that can have multiple funding phases. The first round of funding supports the “build phase” with continued support for the “maintenance phase” and “growth phase”. However, there are no set commitments to indefinitely support programs. A valuable funding strategy is to support several ocean observing programs that differ in foci but complement each other to increase multi-scale ocean observations. For example, the Integrated Ocean Observing System (IOOS), Ocean Observatories Initiative (OOI), and the Northeast United States Shelf (NES) observation program in the USA. The focus of IOOS is to provide information for the safety, economic, and stewardship of the nation, while a science-driven focus is on moorings and cabled arrays within OOI and discrete samples within Northeast United States Shelf (NES) Long-Term Ecological Research (LTER) (Trowbridge et al., 2019; IOOS, 2024; OOI, 2024). Examples of established ocean observing programs include:
● Integrated Ocean Observing System (IOOS) in the USA
● Ocean Observatories Initiative (OOI, formerly ORION) in the USA
● Northeast United States Shelf (NES) Long-Term Ecological Research (LTER) in the USA
● Integrated Marine Observing System (IMOS) in Australia
● Ocean Networks Canada (ONC) in Canada
● East China Sea Seafloor Observation System (ECSSOS) in China (Yu et al., 2012)
● Dense Ocean Floor Network System for Earthquakes and Tsunamis (DONET) in Japan
Research infrastructures (RIs) are centralized facilities that provide resources and services to foster innovation (European Commission, 2016). RIs offer access to cutting-edge instruments or scientific equipment that promote knowledge, process management, and development of products within teams and institutions from academia, industry, and public sectors (European Commission, 2016). The services of RIs extend past offering access to instruments or measurement services, given the strong focus on inter-calibration efforts that improve the measurement validation and data output across institutes. Data output can vary based on the extraction method, instrument settings, the assumed homogeneity of duplicate samples, and the absence of certified standards analyzed at regular time intervals (Canuti, 2023) but can be controlled using inter-laboratory comparisons (ILCs). Annual ILC exercises implemented within the coastal monitoring sites of SOMLIT, funded through the French National Observation Services, have increased the data quality over the last 21 years (Breton et al., 2023).
Coordinating ocean observations can be a challenge at national levels but becomes increasingly difficult at supra-national levels (e.g., European Union). To address this, the European Union established the European Strategy Forum on Research Infrastructures (ESFRI) in 2002. The ESFRI brings together a senior science-policy official from the European Commission and delegates from research ministers of the member and associate countries to develop a coherent strategy for European investments in RIs with the aim of realizing a well-functioning market for research innovation and technology as part of the European Research Area (ERA), launched in 2000 (Table 1). ESFRI awards the “Project” status to RIs in the preparation phase and may be upgraded to the “Landmark” status to implemented RIs with the option of receiving the specific legal form of a European Research Infrastructure Consortium (ERIC, Table 1). As of 2021, the EU landscape had 22 ESFRI Projects and 41 ESFRI Landmarks, of which 20 were ERIC ESFRI Landmarks. The ERIC allows the establishment and operation of new or existing RIs on a non-economic basis (Dañobeitia et al., 2023). Marine European ERICs bring structure to research communities, implement guidelines, and best practices laid out in international frameworks of the Global Ocean Observing System (GOOS), Genomics Standards Consortium (GSC), and the European Ocean Biodiversity Information System (EurOBIS) (Dañobeitia et al., 2023). Established European ERICs and RIs for the ocean include:
● European Multidisciplinary Seafloor and water-column Observatory (EMSO ERIC) focuses on climate change, marine ecosystems, and marine hazards at 14 sites with glider transects, buoys, moorings, and seafloor nodes.
● European contribution to the International Argo program (EURO ARGO ERIC) focuses on maintaining approximately one-fourth of the global Argo-profiling floats.
● E-Science Technology European Infrastructure for Biodiversity and Ecosystem Research (LifeWatch ERIC) focuses on mobilizing and integrating data and algorithms for biodiversity and ecosystem research.
● European Marine Biological Resource Centre (EMBRC ERIC) focuses on promoting the development of blue biotechnologies by providing access to resources, services, facilities, and technology platforms across nine member countries for more than 70 sites.
● Integrated Carbon Observation System (ICOS ERIC) Ocean Thematic Centre (OTC) focuses on the carbon cycle in the ocean at 29 sites.
● International Centre of Advanced Studies on River-Sea Systems (DANUBIUS-RI) focuses on facilitating excellent science at river-sea systems.
European RIs are specific to a scientific field, but ocean observations naturally overlap across domains (Dañobeitia et al., 2023). For example, sensor-based measurements of water temperature occur on moorings within EMSO ERIC and Argo floats within EURO ARGO ERIC. The continued efforts to promote new and consolidate existing marine RIs and ERICs (e.g., InSEA Project; see De Santis et al., 2022) fosters inter- and multidisciplinary research to innovation across several nations (Lantéri et al., 2022) that encourages a collective and deeper understanding of the range of geographical scales in the global oceans.
Ocean observations generate a tremendous volume of data and metadata. Many platform-based networks and ship-based time series have or are in the process of implementing the standards and best practices guided by FAIR principles to make data more findable, accessible, interoperable, and reusable (Wilkinson et al., 2016). Additionally, platform-based networks have standards for best practices, such as GO-SHIP (Talley et al., 2016; Sloyan et al., 2019), Argo (Roemmich et al., 2009), and OceanSITES (Send et al., 2010). A useful foundation is provided by the Ocean Best Practices System (OBPS), which provides technological solutions and community approaches to best practices that are centered around adopting methods for every activity in ocean observation to provide coordinated and sustained global access to methods across ocean sciences and foster collaboration and innovation (Pearlman et al., 2019). Clearly outlining the lifecycle of data and metadata to ensure compliance with FAIR data principles can be described as part of a data management plan to outline a data submission to public databases. In general, applying a data management plan compliant with FAIR principles to time series generates additional value and a sustainable life cycle of ocean datasets to ensure availability to a broader set of end-users (Benway et al., 2019).
Data synthesis products to enhance efficient and interoperable data usage vary based on discipline and sampled variables. In particular, discrete ship-based data might be either uploaded as supplementary files to a scientific publication, PANGAEA is a data repository for earth system and environmental science (Diepenbroek et al., 2002), or displayed in near real-time on the designated website of an ocean time series. Data interoperability to synthesis products make data mining increasingly difficult and time-consuming but community-driven efforts have produced data products around specific EOVs: MarinE MethanE and NiTrous Oxide (MEMENTO) (Bange et al., 2009), Surface Ocean CO2 Atlas (SOCAT) (Pfeil et al., 2013; Sabine et al., 2013), Global Ocean Data Analysis Project (GLODAP, version 2) (Olsen et al., 2016), and the Global Ocean Oxygen Database and Atlas (GO2DAT) (Grégoire et al., 2021). Unfortunately, the data products have provided varying degrees of FOO readiness levels (Lange et al., 2023). Additionally, ocean profile data is provided by World Ocean Database (Boyer et al., 2018), the European Marine Observation and Data Network (EMODnet) integrates data across themes (Martín Míguez et al., 2019), and the Ocean Biodiversity Information System (OBIS) maintains biodiversity and biogeographic data on marine life. Therefore, striving towards a full integration of the OBPS with fit-for-purpose data synthesis products is essential to make the data more available to a broader set of end-users (Lange et al., 2023, Lange et al., 2024).
Data synthesis is still predominantly community-driven and easier said than done. The most important step towards broader global datasets is effective communication within scientific networks, which can set standards to define protocols for uniform data processing of specific parameters, resembling recommendations for plankton measurements in the OceanSITES network (Boss et al., 2022). Likewise, optimization of observatory instruments (Guo et al., 2023; Zhang et al., 2023), refurbishment of existing and expansion of observatory infrastructures (Ford et al., 2022; Zielinski et al., 2022), and data mining with methods of transfer functions, for example, artificial neural networks or deep learning can also work towards processing global datasets (Sauzède et al., 2017; Fourrier et al., 2020; Contractor and Roughan, 2021; Lee et al., 2022; Wang et al., 2023).
Ocean observations serve as the baseline for scientific evidence-based recommendations and inform a broad range of end users, including decision- or policymakers and actors. Actors, occasionally referred to as stakeholders, are non-academic individuals and organizations from many sectors are interested in ocean observations. For example, offshore energy and shipping industry, to industries such as aviation, (re-)insurance, and retail/logistics, to indigenous communities and non-governmental organizations that ultimately generate societal value (Mackenzie et al., 2019). Elevating the science-policy and public-private interfaces improves the blue economy in ocean-based industries (e.g., Palmer et al., 2020; Yoon et al., 2023) and develops effective management actions (Gallo et al., 2022). Moreover, businesses that commit to becoming sustainable can simultaneously promote ocean literacy among employees and elevate the economic, societal, and environmental benefits of ocean observations (Mackenzie et al., 2019).
Ocean time series can play a vital role in building ocean literacy and help transform habits within a community. Ocean time series can support educators from schools, higher institutes, and the ocean literacy of the public (Benway et al., 2019). In the last decade, the public’s perception of the ocean has changed with increasing awareness through (social) media outreach and ocean literacy initiatives, such as the Irish Ocean Literacy Network (2023). Yet, in cases where funding is awarded to ocean time series, the deliverables are typically restricted to scientific output and rarely dedicated to outreach and the translation of scientific evidence into action (Neuer et al., 2017; Benway et al., 2019; Rölfer et al., 2021). Incorporating time series into educational modules could teach children the tremendous value of long-term marine ecosystem monitoring, while equating ocean observations to ocean weather reports could expand and instill the value of marine ecosystem monitoring among adults.
Knowledge and action gaps related to the ocean are currently addressed through social networks. Social networks perform a variety of functions from knowledge sharing, advisory actions, building management capacities, or all three as hybrid networks (Dalton et al., 2020). Knowledge-sharing networks intend to connect individuals with access to information relevant to their research, their jobs, or other aspects of their livelihoods of a specific focus group. Advisory-focused networks bring together scientists and actors for the co-design of ocean science and intend to inform ocean governance and marine policy at multiple levels, such as the Ocean Knowledge Action Network and the Deep Ocean Stewardship Initiative (DOSI; Figure 4). Additionally, networks of networks can bridge the gap between science and policy, such as the GEOBluePlanet or the European Marine Board (EMB). Finally, engaging in programs, projects, and actions endorsed by the UN Ocean Decade, like the OceanPractices program, will support all ocean actors in sustainably managing our oceans.
An Early Career Ocean Professional (ECOP) is an individual with ten years or less of professional experience in any field related to the ocean (Box 2). (ECOP Program, 2024). By definition, ECOPs include early career researchers (ECR) or scientists (ECS) as part of the academic community, which is perhaps the most obvious ocean career. ECRs considerably contribute to ocean observations through their thesis and communicate these results through scientific publications and at scientific conferences. There are conferences dedicated specifically to ECOPs, which allow them to present network and gain confidence in communicating current research and work, e.g., at sessions focused on ocean time series (von Jackowski et al., 2020, von Jackowski et al., 2022; Beltran-Perez and Waniek, 2022; Beltran-Perez et al., 2023; Schnier et al., 2024; Hepach et al., 2024). Unfortunately, scientific publications are still the only measure of scientific value, but the success and impact metrics in science are shifting (Davies et al., 2021). The transition toward inclusive metrics, capacity sharing, and co-design processes with non-academic actors in research projects will benefit the scientific system and global ocean sustainability initiatives (Davies et al., 2021; Rölfer et al., 2021; Satterthwaite et al., 2022). Furthermore, incorporating multi-sectoral collaborations in research projects will also sustainably foster career paths of ECOPs since the majority of ECRs transition from the high number of unpaid work and extremely competitive positions in natural sciences to social sciences (Osiecka et al., 2021; Rölfer et al., 2022; Shellock et al., 2023). ECOPs need to position themselves across sectors (i.e., science, policy, and society), build support networks (see section 3.2), and influence conversations to undoubtedly play the most significant role in bridging generational gaps and ensuring the success of the UN Ocean Decade in the upcoming years (Giron-Nava and Harden-Davies, 2021).
Box 2. Definition of an ECOP
An Early Career Ocean Professional (ECOP) is an individual with then years or less of professional experience in any field related to the ocean. The universal definition of “early career” varies across sectors and is often tied to criteria such as years since terminal degree. Here, the definition aims to be an inclusive term for all professionals near the beginning of their career trajectory. ECOPs are individuals in academia, industry, consultancy, foundations, non-profit organizations, and government positions. For example, doctoral researchers, divers, environmental lawyers, ship mechanics, marine engineers, politicians, and many more.
Based on the definition from the UN Ocean Decade ECOP Program
Networking is the process of communicating and making connections that can build your own network. Over time, continued networking will build your personal network. Establishing your personal network is not always easy, but there are numerous social networks and opportunities specifically designed for ECOPs to enable networking and early career engagement; examples include:
● UN Ocean Decade ECOP Program with regional and national nodes
● Sustainable Ocean Alliance (SOA) Ocean Leadership Program with regional and national “Ocean Hub” nodes
● Association of Polar Early Career Scientists (APECS) with national committee nodes
● Youth4Ocean
● European Marine Board (EMB) ECOP Network
● Global Youth Biodiversity Network
● All-Atlantic Ocean Research and Innovation Alliance (AAORIA) initiatives
● Membership-based IEEE Society Young Professionals
● Membership-based Black In Marine Science (BIMS)
● Membership-based Institute of Marine Engineering, Science & Technology (IMarEST)
● Sign up for newsletters and hackathons
● Attend webinars, see Ocean Carbon and Biogeochemistry program and Iliad DTO series
● Attend workshops and training sessions; see Iliad Academy and OceanPractices program with their annual Ocean Best Practices System Workshop
● Attend conferences, including UN Ocean Decade conferences
Once you have identified one or multiple network(s) you would like to join, get in contact! Although it can seem overwhelming at first, the individuals already within the network are interested in communication and will welcome interested ECOPs. Joining a network will give you the opportunity to connect with like-minded individuals from different walks of life, backgrounds, perspectives, and expertise to build an engaged community with the cause. Here are some recommendations and pointers to get started:
● There is no knowledge threshold that you need to get started - just go for it!
● There are many more networks than those mentioned above. Just find the community that you would like to get involved in, whether on a thematic or geographical scale. You can e-mail them or attend a conference to connect with a member of the network that inspires you.
● ENGAGE! Active engagement in a social network is frequently on a voluntary basis, but interacting with ECOPs or cross-disciplinary fields will positively impact your career.
● Learn from the community by attending meetings and webinars and perhaps taking on a leadership role in one network you have joined.
● Maintain the community over time and collaborate with other networks to reach out of your comfort zone and bridge the gaps between networks.
Climate change is increasing the frequency of natural disasters and the risk of crossing several marine-related tipping points (IPCC, 2023). Although the oceans considerably regulate the Earth’s climate, the Sustainable Development Goal (SDG) 14 “Life Below Water”, within the 2030 Agenda for Sustainable Development, receives the least amount of long-term funding of any of the SDGs (United Nations, 2022). Therefore, ocean observations are critical to understanding the changing role of oceans in buffering and moderating the ongoing anthropogenic climate change (e.g., Moran et al., 2022; Przeslawski et al., 2023). Ocean observations are gaining more and more attention with an increasing number of designated journal sections and conferences, such as OceanObs’ conference series (Speich et al., 2019) and UN Ocean Decade conferences as part of the UN Ocean Decade, to inform the relevant industry sectors and end-users. The UN Ocean Decade aims to connect people and transform ocean science solutions for sustainable development using ten challenges between 2021-2030. Specifically, two of the ten challenges focus on ocean observations (Challenge 7) and a digital representation of the ocean (Challenge 8), described thoroughly in a White Papers that outlines the user needs, gaps in science, and strategic measures required to fulfill the objectives by the end of the decade (UNESCO, 2024).
Ocean observations are becoming increasingly important for society, but ocean time series are not sustainably supported despite their far-reaching benefits. There is a large possibility that ocean time series stations are, to a degree or fully, dependent on voluntary contributions from institutional budgets or short-term project-based funding. Only a few ocean observations are supported through program-based funding and RIs for multiple years to decades. In the near future, national governments should recognize that adequate and reliable funding infrastructure of ocean observations is fundamental to meeting user needs and important to build innovation and capacity. Ocean time series elevate the societal and economic benefits of ocean data. In an ideal scenario, national strategic investments combine short-term project funding with long-term funding to maximize the scientific knowledge output of curiosity-driven research and monitoring efforts that feed into the digital twins of the oceans (DITTO).
Ocean observations and ECOPs are the main pillars of sustainable ocean solutions in the future. It is undeniable that human activities are responsible for climate change, which is a complex problem that requires transformative and transdisciplinary approaches. Such transformations require co-design approaches that share knowledge for inclusive engagement and facilitate multi-sectoral collaborations to develop a shared commitment among end-users. Ocean time series are a great tool to observe the ocean, building ocean literacy, and engage a range of end-users. End-users range across sectors, disciplines, regions, generations, and career stages. For example, ECOPs are present in science, policy, and society, where they might ask questions and start new conversations with out-of-the-box ideas to catalyze culture change for inclusivity. In conclusion, promoting inclusion and transparency of the current structures will strengthen the engagement in networks and actions across end-users, in particular among ECOPs that can bridge generational gaps, develop innovative solutions, and play a significant role in ensuring the success of the UN Ocean Decade to shape the ocean we want and need in the future.
AvJ: Conceptualization, Formal analysis, Investigation, Visualization, Writing – original draft, Writing – review & editing.
The author(s) declare financial support was received for the research, authorship, and/or publication of this article. AvJ was funded through the French National Centre for Scientific Research (CNRS) MicroPump project (ANR-20-CE01-0007). Open-access publication fees were covered by the state of Bremen. ICYMARE provides the editorial service.
I would like to thank all the ECOPs that attended the International Conference for YOUNG Marine Researchers (ICYMARE), especially those interested in a session on ocean observations, in Bremerhaven (Germany) between 13-16th September 2022. My recognition to the ICYMARE organization team for putting together such a successful conference and space for ECOPs as an action to the United Nations Decade of Ocean Science for Sustainable Development 2021-2030. Special thanks to Todd O’Brien (NOAA), Laura Lorenzoni (NASA), and the many contributors who curated METabase and Alexandra Kraberg (AWI) who added information from the AWI-LTO webgis. Thank you for the time and effort provided by the reviewers.
The author declares that the research was conducted in the absence of any commercial or financial relationships that could be construed as a potential conflict of interest.
The authors declare that no Gen AI was used in the creation of this manuscript.
All claims expressed in this article are solely those of the authors and do not necessarily represent those of their affiliated organizations, or those of the publisher, the editors and the reviewers. Any product that may be evaluated in this article, or claim that may be made by its manufacturer, is not guaranteed or endorsed by the publisher.
The Supplementary Material for this article can be found online at: https://www.frontiersin.org/articles/10.3389/fmars.2024.1363863/full#supplementary-material
Bange H. W., Bell T. G., Cornejo M., Freing A., Uher G., Upstill-Goddard R. C., et al. (2009). MEMENTO: a proposal to develop a database of marine nitrous oxide and methane measurements. Environ. Chem. 6, 195. doi: 10.1071/EN09033
Beltran-Perez O. D., Voss M., Pollehne F., Liskow I., Waniek J. J. (2023). Temporal variability of particle flux and its components in the Gotland Basin, eastern Baltic Sea. Front. Earth Sci. 11. doi: 10.3389/feart.2023.1171917
Beltran-Perez O. D., Waniek J. J. (2022). Inter-annual variability of spring and summer blooms in the eastern baltic sea. Front. Mar. Sci. 9. doi: 10.3389/fmars.2022.928633
Benway H., Doney S. (2014). Scientific outcomes and future challenges of the ocean carbon and biogeochemistry program. oceanog 27, 106–107. doi: 10.5670/oceanog.2014.13
Benway H. M., Lorenzoni L., White A. E., Fiedler B., Levine N. M., Nicholson D. P., et al. (2019). Ocean time series observations of changing marine ecosystems: an era of integration, synthesis, and societal applications. Front. Mar. Sci. 6. doi: 10.3389/fmars.2019.00393
Boss E., Waite A. M., Karstensen J., Trull T., Muller-Karger F., Sosik H. M., et al. (2022). Recommendations for plankton measurements on oceanSITES moorings with relevance to other observing sites. Front. Mar. Sci. 9. doi: 10.3389/fmars.2022.929436
Boyer T. P., Baranova O. K., Coleman C., Garcia H. E., Grodsky A., Locarnini R. A., et al. (2018). World ocean database WOD18. Available online at: https://ncei.noaa.gov/products/world-ocean-database. (Accessed December 21, 2023)
Breton E., Savoye N., Rimmelin-Maury P., Sautour B., Goberville E., Lheureux A., et al. (2023). Data quality control considerations in multivariate environmental monitoring: experience of the French coastal network SOMLIT. Front. Mar. Sci. 10. doi: 10.3389/fmars.2023.1135446
Buttigieg P. L., Fadeev E., Bienhold C., Hehemann L., Offre P., Boetius A. (2018). Marine microbes in 4D — using time series observation to assess the dynamics of the ocean microbiome and its links to ocean health. Curr. Opin. Microbiol. 43, 169–185. doi: 10.1016/j.mib.2018.01.015
Canuti E. (2023). Phytoplankton pigment in situ measurements uncertainty evaluation: an HPLC interlaboratory comparison with a European-scale dataset. Front. Mar. Sci. 10. doi: 10.3389/fmars.2023.1197311
Contractor S., Roughan M. (2021). Efficacy of feedforward and LSTM neural networks at predicting and gap filling coastal ocean timeseries: oxygen, nutrients, and temperature. Front. Mar. Sci. 8. doi: 10.3389/fmars.2021.637759
Dalton K., Skrobe M., Bell H., Kantner B., Berndtson D., Gerhardinger L. C., et al. (2020). Marine-related learning networks: shifting the paradigm toward collaborative ocean governance. Front. Mar. Sci. 7. doi: 10.3389/fmars.2020.595054
Dañobeitia J. J., Pouliquen S., Pade N., Arvanitidis C., Sanders R., Stanica A., et al. (2023). The role of the marine research infrastructures in the European marine observation landscape: present and future perspectives. Front. Mar. Sci. 10. doi: 10.3389/fmars.2023.1047251
Davies S. W., Putnam H. M., Ainsworth T., Baum J. K., Bove C. B., Crosby S. C., et al. (2021). Promoting inclusive metrics of success and impact to dismantle a discriminatory reward system in science. PloS Biol. 19, e3001282. doi: 10.1371/journal.pbio.3001282
De Santis A., Chiappini M., Marinaro G., Guardato S., Conversano F., D’Anna G., et al. (2022). InSEA project: initiatives in supporting the consolidation and enhancement of the EMSO infrastructure and related activities. Front. Mar. Sci. 9. doi: 10.3389/fmars.2022.846701
Diepenbroek M., Grobe H., Reinke M., Schindler U., Schlitzer R., Sieger R., et al. (2002). PANGAEA—an information system for environmental sciences. Comput. Geosciences 28, 1201–1210. doi: 10.1016/S0098-3004(02)00039-0
ECOP Program (2024). Available online at: https://ecopdecade.org/. (Accessed April 22, 2024)
ENEON (2020). European observatory of earth observation networks. Available online at: https://eneon.net/ENEON_insitu.htm. (Accessed September 20, 2023)
European Commission (2016). European Charter for access to research infrastructures - Principles and Guidelines for Access and Related Services Brussels, Belgium. Available online at: https://op.europa.eu/en/publication-detail/-/publication/78e87306-48bc-11e6-9c64-01aa75ed71a1/. (Accessed September 20, 2023)
Ford D. A., Grossberg S., Rinaldi G., Menon P. P., Palmer M. R., Skákala J., et al. (2022). A solution for autonomous, adaptive monitoring of coastal ocean ecosystems: Integrating ocean robots and operational forecasts. Front. Mar. Sci. 9. doi: 10.3389/fmars.2022.1067174
Fourrier M., Coppola L., Claustre H., D’Ortenzio F., Sauzède R., Gattuso J.-P. (2020). A regional neural network approach to estimate water-column nutrient concentrations and carbonate system variables in the mediterranean sea: CANYON-MED. Front. Mar. Sci. 7. doi: 10.3389/fmars.2020.00620
Gallo N. D., Bowlin N. M., Thompson A. R., Satterthwaite E. V., Brady B., Semmens B. X. (2022). Fisheries surveys are essential ocean observing programs in a time of global change: A synthesis of oceanographic and ecological data from U.S. West coast fisheries surveys. Front. Mar. Sci. 9. doi: 10.3389/fmars.2022.757124
GEOBON (2023). GEOBON what are EBVs. Available online at: https://geobon.org/ebvs/what-are-ebvs/. (Accessed November 02, 2023)
Giering S. L. C., Culverhouse P. F., Johns D. G., McQuatters-Gollop A., Pitois S. G. (2022). Are plankton nets a thing of the past? An assessment of in situ imaging of zooplankton for large-scale ecosystem assessment and policy decision-making. Front. Mar. Sci. 9. doi: 10.3389/fmars.2022.986206
Giron-Nava A., Harden-Davies H. (2021). A generational shift in ocean stewardship. Oceanog 34, 1-5. doi: 10.5670/oceanog.2021.402
Gonzalez A., Vihervaara P., Balvanera P., Bates A. E., Bayraktarov E., Bellingham P. J., et al. (2023). A global biodiversity observing system to unite monitoring and guide action. Nat. Ecol. Evol. 7, 1947-1952. doi: 10.1038/s41559-023-02171-0
González-Dávila M., Santana-Casiano J. M. (2023). Long-term trends of pH and inorganic carbon in the Eastern North Atlantic: the ESTOC site. Front. Mar. Sci. 10. doi: 10.3389/fmars.2023.1236214
GOOS (2024). Available online at: https://goosocean.org/who-we-are/. (Accessed October 15, 2023)
Grégoire M., Garçon V., Garcia H., Breitburg D., Isensee K., Oschlies A., et al. (2021). A global ocean oxygen database and atlas for assessing and predicting deoxygenation and ocean health in the open and coastal ocean. Front. Mar. Sci. 8. doi: 10.3389/fmars.2021.724913
Gruber N. (2011). Warming up, turning sour, losing breath: ocean biogeochemistry under global change. Phil. Trans. R. Soc A. 369, 1980–1996. doi: 10.1098/rsta.2011.0003
Guo L., Yang X., Xue G., Wang C., Fei Z., Zhang S., et al. (2023). Development and application of a 6000-meter double decelerating lander. Front. Mar. Sci. 10. doi: 10.3389/fmars.2023.1090940
Hepach H, Piontek J, Bange HW, Barthelmeß T, von Jackowski A, Engel A.. (2024). Enhanced warming and bacterial biomass production as key factors for coastal hypoxia in the southwestern Baltic. Sci. Rep. 4 (1), 29442. doi: 10.1038/s41598-024-80451-w
IGMETS. (2017). International group for marine ecological time series. Available online at: https://igmets.net/. (Accessed September 10, 2023)
IOOS (2024). Integrated ocean observing system. Available online at: https://ioos.noaa.gov/about/about-us/. (Accessed October 15, 2023)
IPCC (2023). Sixth assessment report. Available online at: https://ipcc.ch/assessment-report/ar6/. (Accessed April 17, 2024)
Irish Ocean Literacy Network (2023). Irish ocean literacy network. Available online at: https://irishoceanliteracy.ie/. (Accessed December 22, 2023)
Karl D. (2010). Oceanic ecosystem time-series programs: ten lessons learned. Oceanog. 23, 104–125. doi: 10.5670/oceanog.2010.27
Kopf A., Bicak M., Kottmann R., Schnetzer J., Kostadinov I., Lehmann K., et al. (2015). The ocean sampling day consortium. GigaSci 4, 27. doi: 10.1186/s13742-015-0066-5
Lange N., Fiedler B., Álvarez M., Benoit-Cattin A., Benway H., Buttigieg P. L., et al. (2024). Synthesis Product for Ocean Time Series (SPOTS) – a ship-based biogeochemical pilot. Earth Syst. Sci. Data 16, 1901–1931. doi: 10.5194/essd-16-1901-2024
Lange N., Tanhua T., Pfeil B., Bange H. W., Lauvset S. K., Grégoire M., et al. (2023). A status assessment of selected data synthesis products for ocean biogeochemistry. Front. Mar. Sci. 10. doi: 10.3389/fmars.2023.1078908
Lantéri N., Ruhl H. A., Gates A., Martínez E., Del Rio Fernandez J., Aguzzi J., et al. (2022). The EMSO generic instrument module (EGIM): standardized and interoperable instrumentation for ocean observation. Front. Mar. Sci. 9. doi: 10.3389/fmars.2022.801033
Lee E.-J., Kim K., Park J.-H. (2022). Reconstruction of long-term sea-level data gaps of tide gauge records using a neural network operator. Front. Mar. Sci. 9. doi: 10.3389/fmars.2022.1037697
Mackenzie B., Celliers L., Assad L. P. D. F., Heymans J. J., Rome N., Thomas J., et al. (2019). The role of stakeholders in creating societal value from coastal and ocean observations. Front. Mar. Sci. 6. doi: 10.3389/fmars.2019.00137
Martín Míguez B., Novellino A., Vinci M., Claus S., Calewaert J.-B., Vallius H., et al. (2019). The european marine observation and data network (EMODnet): visions and roles of the gateway to marine data in europe. Front. Mar. Sci. 6. doi: 10.3389/fmars.2019.00313
Masó J., Serral I., Domingo-Marimon C., Zabala A. (2020). Earth observations for sustainable development goals monitoring based on essential variables and driver-pressure-state-impact-response indicators. Int. J. Digital Earth 13, 217–235. doi: 10.1080/17538947.2019.1576787
McCurdy A. (2023). FOO 2.0 system report. Available online at: https://www.goosocean.org/index.php?option=com_oe&task=viewDocumentRecord&docID=32427. (Accessed October 15, 2023)
Mollenhauer H., Kasner M., Haase P., Peterseil J., Wohner C., Frenzel M., et al. (2018). Long-term environmental monitoring infrastructures in Europe: observations, measurements, scales, and socio-ecological representativeness. Sci. Total Environ. 624, 968–978. doi: 10.1016/j.scitotenv.2017.12.095
Moltmann T., Turton J., Zhang H.-M., Nolan G., Gouldman C., Griesbauer L., et al. (2019). A global ocean observing system (GOOS), delivered through enhanced collaboration across regions, communities, and new technologies. Front. Mar. Sci. 6. doi: 10.3389/fmars.2019.00291
Moran K., Juniper S. K., Bligh S., Loock D., Kulin I., Paulson M., et al. (2022). Canada’s internet-connected ocean. Front. Mar. Sci. 8. doi: 10.3389/fmars.2021.805134
Neuer S., Benway H., Bates N., Carlson C., Church M., DeGrandpre M., et al. (2017). Monitoring ocean change in the 21st century. Eos. 98. doi: 10.1029/2017EO080045
Newman L., Heil P., Trebilco R., Katsumata K., Constable A., Van Wijk E., et al. (2019). Delivering sustained, coordinated, and integrated observations of the southern ocean for global impact. Front. Mar. Sci. 6. doi: 10.3389/fmars.2019.00433
Nieuwejaar P., Mazauric V. (2019). Next generation european research vessels. Available online at: https://epic.awi.de/id/eprint/50523/1/EMB_PP25_Research_Vessels_Web_v2.pdf. (Accessed September 25, 2023)
O’Brien T., Lorenzoni L., Isensee K., Valdés L. (2017). What are Marine Ecological Time Series telling us about the ocean? A status report (IOC-UNESCO). Available at: https://www.uncclearn.org/wp-content/uploads/library/ioc_unesco.pdf.
Olsen A., Key R. M., Van Heuven S., Lauvset S. K., Velo A., Lin X., et al. (2016). The Global Ocean Data Analysis Project version 2 (GLODAPv2) – an internallyconsistent data product for the world ocean. Earth Syst. Sci. Data 8, 297–323. doi: 10.5194/essd-8-297-2016
OOI (2024). Ocean observatories initiative. Available online at: https://oceanobservatories.org/. (Accessed October 15, 2023)
Osiecka A. N., Quer S., Wróbel A., Osiecka-Brzeska K. (2021). Unpaid work in marine science: A snapshot of the early-career job market. Front. Mar. Sci. 8. doi: 10.3389/fmars.2021.690163
Palmer S. C. J., Gernez P. M., Thomas Y., Simis S., Miller P. I., Glize P., et al. (2020). Remote sensing-driven pacific oyster (Crassostrea gigas) growth modeling to inform offshore aquaculture site selection. Front. Mar. Sci. 6. doi: 10.3389/fmars.2019.00802
Paulus E. (2021). Shedding light on deep-sea biodiversity—A highly vulnerable habitat in the face of anthropogenic change. Front. Mar. Sci. 8. doi: 10.3389/fmars.2021.667048
Pearlman J., Bushnell M., Coppola L., Karstensen J., Buttigieg P. L., Pearlman F., et al. (2019). Evolving and sustaining ocean best practices and standards for the next decade. Front. Mar. Sci. 6. doi: 10.3389/fmars.2019.00277
Pfeil B., Olsen A., Bakker D. C. E., Hankin S., Koyuk H., Kozyr A., et al. (2013). A uniform, quality controlled Surface Ocean CO<sub<2</sub< Atlas (SOCAT). Earth Syst. Sci. Data 5, 125–143. doi: 10.5194/essd-5-125-2013
Przeslawski R., Barrett N., Carroll A., Foster S., Gibbons B., Jordan A., et al. (2023). Developing an ocean best practice: A case study of marine sampling practices from Australia. Front. Mar. Sci. 10. doi: 10.3389/fmars.2023.1173075
Raes E. J., Myles S., MacNeil L., Wietz M., Bienhold C., Tait K., et al. (2024). Seasonal patterns of microbial diversity across the world oceans. Limnol Oceanogr Lett. 9 (5), lol2.10422. doi: 10.1002/lol2.10422
Ratnarajah L. (2021). Map of bioEco observing networks/capability D1.2. Eurosea. (Available at). doi: 10.3289/eurosea_d1.2
Roemmich D., Johnson G., Riser S., Davis R., Gilson J., Owens W. B., et al. (2009). The argo program: observing the global oceans with profiling floats. Oceanog. 22, 34–43. doi: 10.5670/oceanog.2009.36
Rölfer L., Elias Ilosvay X. E., Ferse S. C. A., Jung J., Karcher D. B., Kriegl M., et al. (2022). Disentangling obstacles to knowledge co-production for early-career researchers in the marine sciences. Front. Mar. Sci. 9. doi: 10.3389/fmars.2022.893489
Rölfer L., Liconti A., Prinz N., Klöcker C. A. (2021). Integrated research for integrated ocean management. Front. Mar. Sci. 8. doi: 10.3389/fmars.2021.693373
Sabine C. L., Hankin S., Koyuk H., Bakker D. C. E., Pfeil B., Olsen A., et al. (2013). Surface Ocean CO2 Atlas (SOCAT) gridded data products. Earth Syst. Sci. Data 5, 145–153. doi: 10.5194/essd-5-145-2013
Santi I., Beluche O., Beraud M., Buttigieg P. L., Casotti R., Cox C. J., et al. (2023). European marine omics biodiversity observation network: a strategic outline for the implementation of omics approaches in ocean observation. Front. Mar. Sci. 10. doi: 10.3389/fmars.2023.1118120
Satterthwaite E. V., Komyakova V., Erazo N. G., Gammage L., Juma G. A., Kelly R., et al. (2022). Five actionable pillars to engage the next generation of leaders in the co-design of transformative ocean solutions. PloS Biol. 20, e3001832. doi: 10.1371/journal.pbio.3001832
Sauzède R., Bittig H. C., Claustre H., Pasqueron De Fommervault O., Gattuso J.-P., Legendre L., et al. (2017). Estimates of water-column nutrient concentrations and carbonate system parameters in the global ocean: A novel approach based on neural networks. Front. Mar. Sci. 4. doi: 10.3389/fmars.2017.00128
Schnier J., Hasemann C., Mokievsky V., Martínez Arbizu P., Soltwedel T. (2023). Nematode communities along a bathymetric transect in the deep eastern Fram Strait (Arctic Ocean): Interrelations between diversity, function and environment. Front. Mar. Sci. 10, 1271447. doi: 10.3389/fmars.2023.1271447
Send U., Send U., Send U., Send U., Send U., Send U., et al. (2010). Proceedings of oceanObs’09: sustained ocean observations and information for society (European Space Agency), 854–863. doi: 10.5270/OceanObs09.cwp.79
Shellock R. J., Cvitanovic C., Badullovich N., Catto D., DelBene J. A., Duggan J., et al. (2023). Crossing disciplinary boundaries: motivations, challenges, and enablers for early career marine researchers moving from natural to social sciences. ICES J. Mar. Sci. 80, 40–55. doi: 10.1093/icesjms/fsac218
Sloyan B. M., Wanninkhof R., Kramp M., Johnson G. C., Talley L. D., Tanhua T., et al. (2019). The global ocean ship-based hydrographic investigations program (GO-SHIP): A platform for integrated multidisciplinary ocean science. Front. Mar. Sci. 6. doi: 10.3389/fmars.2019.00445
Smith N., Kessler W. S., Cravatte S., Sprintall J., Wijffels S., Cronin M. F., et al. (2019). Tropical pacific observing system. Front. Mar. Sci. 6. doi: 10.3389/fmars.2019.00031
Speich S., Lee T., Muller-Karger F., Lorenzoni L., Pascual A., Jin D., et al. (2019). Oceanobs’19: an ocean of opportunity. Front. Mar. Sci. 6. doi: 10.3389/fmars.2019.00570
Sunagawa S., Coelho L. P., Chaffron S., Kultima J. R., Labadie K., Salazar G., et al. (2015). Structure and function of the global ocean microbiome. Science 348, 1261359. doi: 10.1126/science.1261359
Talley L. D., Feely R. A., Sloyan B. M., Wanninkhof R., Baringer M. O., Bullister J. L., et al. (2016). Changes in ocean heat, carbon content, and ventilation: A review of the first decade of GO-SHIP global repeat hydrography. Annu. Rev. Mar. Sci. 8, 185–215. doi: 10.1146/annurev-marine-052915-100829
Tanhua T., McCurdy A., Fischer A., Appeltans W., Bax N., Currie K., et al. (2019). What we have learned from the framework for ocean observing: evolution of the global ocean observing system. Front. Mar. Sci. 6. doi: 10.3389/fmars.2019.00471
Trowbridge J., Weller R., Kelley D., Dever E., Plueddemann A., Barth J. A., et al. (2019). The ocean observatories initiative. Front. Mar. Sci. 6. doi: 10.3389/fmars.2019.00074
UNESCO (2024). Ocean decade vision 2030 white papers. Available online at: https://oceandecade.org/publications/ocean-decade-vision-2030-white-papers/. (Accessed June 21, 2024)
United Nations (2022). Accelerating investments in sdg 14 and the sustainable blue economy. Available online at: https://sdgs.un.org/events/accelerating-investments-sdg-14-and-sustainable-blue-economy-48934. (Accessed April 21, 2024)
Visser F., Keller O. A., Oudejans M. G., Nowacek D. P., Kok A. C. M., Huisman J., et al. (2021). Risso’s dolphins perform spin dives to target deep-dwelling prey. R. Soc Open Sci. 8, 202320. doi: 10.1098/rsos.202320
von Jackowski A., Becker K. W., Wietz M., Bienhold C., Zäncker B., Nöthig E., et al. (2022). Variations of microbial communities and substrate regimes in the eastern Fram Strait between summer and fall. Environ. Microbiol. 24, 4124–4136. doi: 10.1111/1462-2920.16036
von Jackowski A., Grosse J., Nöthig E.-M., Engel A. (2020). Dynamics of organic matter and bacterial activity in the Fram Strait during summer and autumn. Phil. Trans. R. Soc A. 378, 20190366. doi: 10.1098/rsta.2019.0366
Wang M., Wang D., Xiang Y., Liang Y., Xia R., Yang J., et al. (2023). Fusion of ocean data from multiple sources using deep learning: Utilizing sea temperature as an example. Front. Mar. Sci. 10. doi: 10.3389/fmars.2023.1112065
Wilkinson M. D., Dumontier M., Aalbersberg I. J., Appleton G., Axton M., Baak A., et al. (2016). The FAIR Guiding Principles for scientific data management and stewardship. Sci. Data 3, 160018. doi: 10.1038/sdata.2016.18
Yoon Y. G., Han D.-G., Choi J. W. (2023). Measurements of underwater operational noise caused by offshore wind turbine off the southwest coast of Korea. Front. Mar. Sci. 10. doi: 10.3389/fmars.2023.1153843
Yu Y., Xu H., Xu C., Qin R. (2012). A study of the remote control for the east China sea seafloor observation system. J. Atmospheric Oceanic Technol. 29, 1149–1158. doi: 10.1175/JTECH-D-11-00115.1
Zhang S., Tian C., Zhou F. (2023). Design and optimization of buoy mooring with single-point cable for seafloor observatories. Front. Mar. Sci. 9. doi: 10.3389/fmars.2022.1048048
Zielinski O., Pieck D., Schulz J., Thölen C., Wollschläger J., Albinus M., et al. (2022). The spiekeroog coastal observatory: A scientific infrastructure at the land-sea transition zone (Southern north sea). Front. Mar. Sci. 8. doi: 10.3389/fmars.2021.754905
Keywords: climate change, ocean observations, time series, human well-being, policy-making, early-career
Citation: von Jackowski A (2025) Navigating the current landscape of ocean observations: an overview from platform infrastructures to networks related to ocean time series. Front. Mar. Sci. 11:1363863. doi: 10.3389/fmars.2024.1363863
Received: 31 December 2023; Accepted: 22 November 2024;
Published: 23 January 2025.
Edited by:
Yvonne Schadewell, University of Duisburg-Essen, GermanyReviewed by:
Jinlin Liu, Tongji University, ChinaCopyright © 2025 von Jackowski. This is an open-access article distributed under the terms of the Creative Commons Attribution License (CC BY). The use, distribution or reproduction in other forums is permitted, provided the original author(s) and the copyright owner(s) are credited and that the original publication in this journal is cited, in accordance with accepted academic practice. No use, distribution or reproduction is permitted which does not comply with these terms.
*Correspondence: Anabel von Jackowski, YW5hYmVsLnZvbmphY2tvd3NraUBvYnMtYmFueXVscy5mcg==
Disclaimer: All claims expressed in this article are solely those of the authors and do not necessarily represent those of their affiliated organizations, or those of the publisher, the editors and the reviewers. Any product that may be evaluated in this article or claim that may be made by its manufacturer is not guaranteed or endorsed by the publisher.
Research integrity at Frontiers
Learn more about the work of our research integrity team to safeguard the quality of each article we publish.