- Laboratory of Marine Ecotoxicology, V.I. Il’ichev Pacific Oceanological Institute, Russian Academy of Sciences, Vladivostok, Russia
The problem of environmental pollution by plastics is global in nature and needs to be addressed as soon as possible. Realization of the importance of this problem contributed to the study of degradation and biodegradation of synthetic polymers. It turned out that the driving force of plastic fragmentation along with abiotic factors can be biotic. Based on the above, we investigated the in vitro biodegradation of polypropylene (PP) fragments in digestive gland homogenates and crystalline styles of the bivalve mollusk Mizuhopecten yessoensis. Fourier transform infrared spectroscopy showed changes in the chemical composition of functional groups on the plastic surface. Enzyme complexes of crystalline styles enhanced the biodegradation of PP fragments to a larger extent than did digestive glands. The results obtained using M. yessoensis as an example suggest that marine phytophagous filter-feeding invertebrates may accelerate the biodegradation of synthetic polymers. The study provides a basis for rethinking the nature of relationships between marine invertebrates and microplastic polluting the marine environment.
1 Introduction
Extensive studies in recent years convincingly demonstrated that synthetic polymers spread widely in the environment and invaded all aquatic, terrestrial, and air ecosystems, thereby endangering their stability (Koelmans et al., 2014; Avio et al., 2015; Li et al., 2016; Ahmed et al., 2022; Xia et al., 2023). Microplastic particles have been recorded in representatives of various taxa (more than a thousand species) of different trophic levels (Bom and Sá, 2021; Dellisanti et al., 2023). There is a lot of evidence that microplastic has a harmful effect on biota (Jeong et al., 2016; Détrée and Gallardo-Escárate, 2018; Revel et al., 2019; Trifuoggi et al., 2019; Thomas et al., 2020; Chelomin et al., 2022). This danger sharply stimulated interest in studying the degradation and biodegradation processes of these man-made materials. Despite their strength and chemical inertness, synthetic polymers can not only break down because of physicochemical processes (photo, thermal, mechanical and chemical degradation) (Lambert and Wagner, 2016; Ward et al., 2019; Chamas et al., 2020; Liu et al., 2021; Mattsson et al., 2021; Born and Brüll, 2022; Duan et al., 2022; Xi et al., 2022) but also can degrade due to biota activity. In the latter case, this generally optimistic view is based on numerous studies on microorganisms, including mainly various bacterial species (Tokiwa et al., 2009; Wu et al., 2016; Matjašič et al., 2020; Jadaun et al., 2022; Miri et al., 2022; Idris et al., 2023), cyanobacteria, microalgae (Sarmah and Rout, 2018; Sarmah and Rout, 2020), and fungi (Liu et al., 2021). In addition, biodegradation of different types of polymers was observed with the participation of various insect larvae (Brandon et al., 2018; Kundungal et al., 2019; Ren et al., 2019; Peng et al., 2020; Yang et al., 2021).
Unlike abiotic degradation, biodegradation is carried out with the direct participation of biocatalysts - enzymes produced by living organisms. A large variety of these specific enzymes are known to be involved in various stages of polymer chain degradation (Lucas et al., 2008). In general terms, the activity of these enzymes ultimately leads to the oxidation and hydrolysis of polymers, followed by the fragmentation and release of low molecular-weight compounds (monomers and oligomers) that can be involved in metabolic processes. It should be emphasized that enzymes participate to varying degrees in the biodegradation processes of artificial polymers. They are primarily catalysts focused on the digestion of natural polymers (cellulose, lignin, mucopolysaccharides) to extract energy for essential processes. On the basis of these assumptions, it is logical to assume that digestive enzymes of herbivorous marine invertebrates involved in the digestion of cell walls of phytoplankton and algae, which include polymeric carbohydrates, are also capable to oxidative hydrolysis of plastic.
Since understanding the biodegradation and biological depolymerization of plastic is important, this study proposes a novel methodological approach to investigate the role of marine invertebrate digestive enzyme complexes in plastic biodegradation. The aim of this study is to evaluate the feasibility of enzymatic biodegradation of polypropylene (PP) pieces in Mizuhopecten yessoensis digestive organ homogenate.
2 Methods
2.1 Site of bivalves collection and material
Mollusks were collected in July 2023 in the waters of the Alekseev Bay in the Sea of Japan (42°59’ N; 131°43’ Е). Adult mollusks were selected by size so that they were in a similar physiological state. (in total, fifteen representatives). Mollusks were immediately dissected, and the digestive glands and crystalline styles were extracted. Tissue homogenate was prepared in 0.2 M of phosphate buffer, pH 7.5 (2:1, w/v).
The plastic polymers used in the experiment came from commercially available polypropylene rope, which chemical composition was determined by FTIR spectroscopy.
2.2 Experiment in vitro
The plastic fragments (2.5 cm long and 1 mm wide) used in the experiment were obtained by cutting unused polypropylene rope manufactured by TRUSCO NAKAYAMA Corporation (Cat No. PP-4300). The production technology and exact chemical composition of the rope is not specified by the manufacturer.
The plastic fragments were incubated in 1.5 ml tissue homogenate for 3 days (three times repeated for each animal tissue). The daily replacement of the homogenate was performed. When the homogenate was changed, the plastic pieces were washed in distilled water. Incubation was carried out with constant stirring (BIOSAN MultiBio RS-24) at room temperature. After 3 days of incubation, the plastic pieces were washed in distilled water to remove the homogenate residues and then in 70% ethanol to remove the sorbed organic substances (proteins and fats) for 24 h under constant agitation. After washing, the plastic pieces were dried at room temperature for one day before analysis. Control plastic samples were incubated in the homogenization buffer and were subjected to the same washing and processing steps. Biodegradation of the plastic surface were assessed using FTIR spectroscopy.
2.3 Fourier-transform infrared spectroscopy
FTIR spectra were acquired using an IRAffinity-1S (Shimadzu, Japan) equipped with attachment frustrated total internal reflection (wavenumber range of 4000–400 cm−1, 32 scans per spectrum, spectral resolution of 4 cm−1). The background was measured with the same settings against air. The obtained spectra were processed using LabSolutions IR software (Shimadzu, Japan).
The degree of biodegradation of plastic fragments was evaluated using three different indices: carbonyl index (CI), hydroxyl index (HI) and carboxyl index (COI). CI is the most commonly used index to measure the chemical oxidation of polyolefins such as polyethylene and polypropylene and reflects the deterioration of the mechanical properties of these polymers (Rouillon et al., 2016). CI was determined using the SAUB (specified area under band) technique described in Almond et al., 2020. CI was calculated from the ratio between the integrated band absorbance of the carbonyl (C=O) peak from 1850 to 1650 cm−1 and that of the methylene (CH2) scissoring peak from 1500 to 1420 cm−1 as expressed in the following equation: CI = (Area under band 1850–1650 cm−1)/(Area under band 1500–1420 cm−1).
The measurement of peak area rather than intensity at a particular wavelength is based on the fact that the cleavage of polyolefins produces not only ketones at 1714 cm-1, but also dozens of potentially different carbonyl products such as γ-lactones (1780 cm-1), esters and/or aldehydes (1733 cm-1), and carboxylic acids (1700 cm-1). The area between 1500 and 1420 cm-1 was chosen as the reference range and remains distinct throughout the degradation process during photo- and thermo-oxidation (Almond et al., 2020).
Similarly HI was calculated as the absorbance ratio of hydroxyl groups at 3300–3400 cm-1 and 986–952 cm-1 for reference peak (Campanale et al., 2023). COI was calculated as the ratio of the absorbance of carbon-oxygen groups at 1000–1200 cm-1 and the value of the reference peaks at 2885–2940cm-1 (Campanale et al., 2023).
The modification of the crystal structure of polypropylene was estimated according to Rouillon, Bussiere, 2016, where crystallinity = 998 cm-1/973 cm-1.
2.4 Statistics
Statistical processing of the results was performed using Statistica 7. The Mann-Whitney’s U test for non-parametric variables was used to assess reliability of parameter changes. Significance was established at p < 0.001 and p < 0.01.
2.5 Ethical aspects
All experimental procedures were performed in accordance with the ethical standards for animal experimentation, and meticulous efforts were made to ensure that the animals suffered as little as possible and to reduce external sources of stress, pain, and discomfort. The current study has not exceeded the number of animals needed to produce reliable scientific data. This article does not refer to any study with human participants performed by any authors.
3 Results
Using infrared spectroscopy method, we recorded several major changes in the IR spectra of PP after treatment with homogenates from the digestive gland and crystalline styles of the marine bivalve M. yessoensis (Figure 1). By its chemical structure, PP is a hydrocarbon, that lacks oxygen-containing functional groups (oxygen bonds), which can be clearly seen in the IR spectrum of the control sample of PP (Figure 1A). The pristine reference PP had characteristic absorption bands (Figure 1A), which were displayed at 2953 cm-1(–CH3 asymmetric stretching vibrations), 2837 cm-1and 1456 cm-1(-CH2 stretching and symmetric bending), 1375 cm-1 (–CH3 symmetric bending vibrations), 1166, 997 and 972 cm-1(–CH3 oscillating vibrations, respectively) (Gopanna et al., 2019; Campanale et al., 2023). These characteristic absorption peaks in the IR spectrum remained unchanged in the buffer solution. After 3-day treatment of the PP fragments with digestive gland and crystalline styles homogenates, new absorption peaks in the IR spectra of these samples were recorded in the areas of 3284 cm-1, 1734 cm-1 and 1653 cm-1 (Figures 1B, C), which are characteristic of hydroxyl (C–OH), carbonyl (C=O), and double (C=C) bonds, respectively (dePaoli, 2008; De Bomfim et al., 2019; Wu et al., 2021). In this context, the appearance of a new absorption band in the area of 3284 cm-1 is caused by the addition of a hydroxyl group to the polymer chain, indicating the initiation of oxidative degradation processes (Auta et al., 2018). The spectra in the area of 1900–1500 cm-1 reveal a known carbonyl component with a broad absorption band reflecting the formation of several products, among which the main absorption band (1734 cm-1), corresponding to the carbonyl group of esters, dominates (Rouillon et al., 2016). In addition to the appearance of oxygen-containing functional groups, a new absorption peak (1653 cm-1) was recorded in the structure of the polymer chain of the PP of the experimental samples, corresponding to the vinyl group (De Paoli, 2008; De Bomfim et al., 2019). The C=C bond is also not characteristic of an intact PP chain. It is assumed to arise due to degradation processes with C–C bond breaking of the main carbon chain initiated by substitution (–C–R) and C–H bond breaking (De Paoli, 2008; De Bomfim et al., 2019). Furthermore, from the comparative analysis of the IR spectra (Figures 1A–C), it is evident that exposure of digestive gland and crystalline styles homogenates to PP fragments leads not only to the appearance of new absorption bands but also to the enhancement of individual small bands. In both experimental samples, a significant increase in the intensity of the peak in the range of 1200–1100 cm-1 (centered around 1167 cm-1), corresponding to the spectral band of the carboxyl bond (C–O), is observed, which also indicates the accumulation of various products of oxidative degradation of the polymer (Campanale et al., 2023).
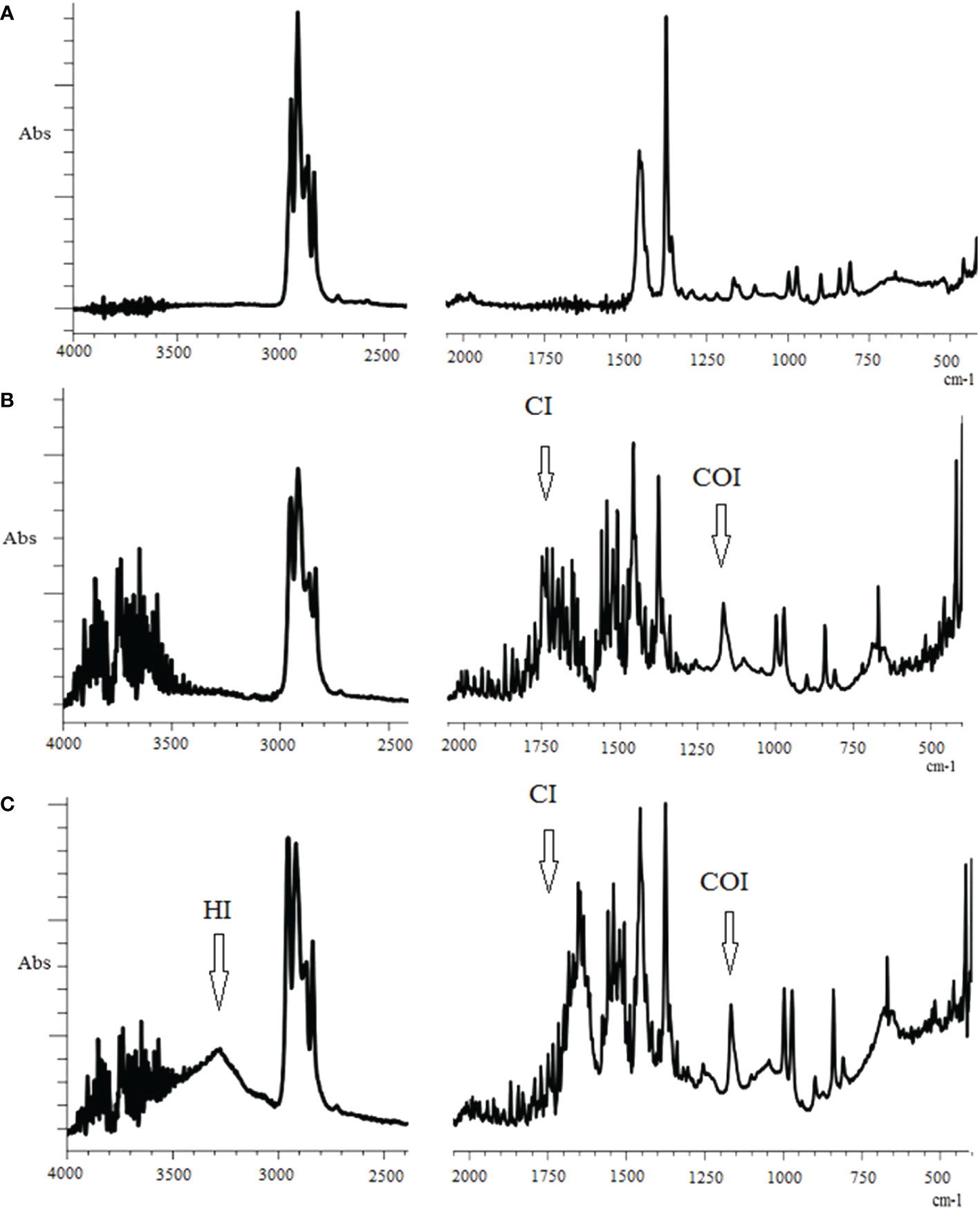
Figure 1 FTIR spectra of polypropylene after 3 days of incubation: (A) in the buffer (control), (B) in the digestive gland homogenate, (C) in the crystalline style homogenate. CI, сarbonyl index; HI, hydroxyl index; COI, сarbon-oxygen index.
To assess the extent of oxidative degradation of the PP polymer chain observed under our experimental conditions, we quantitatively estimated the functional group content and presented them as the relevant indices: carbonyl (CI), hydroxyl (HI), and carboxyl (COI) (Table 1).
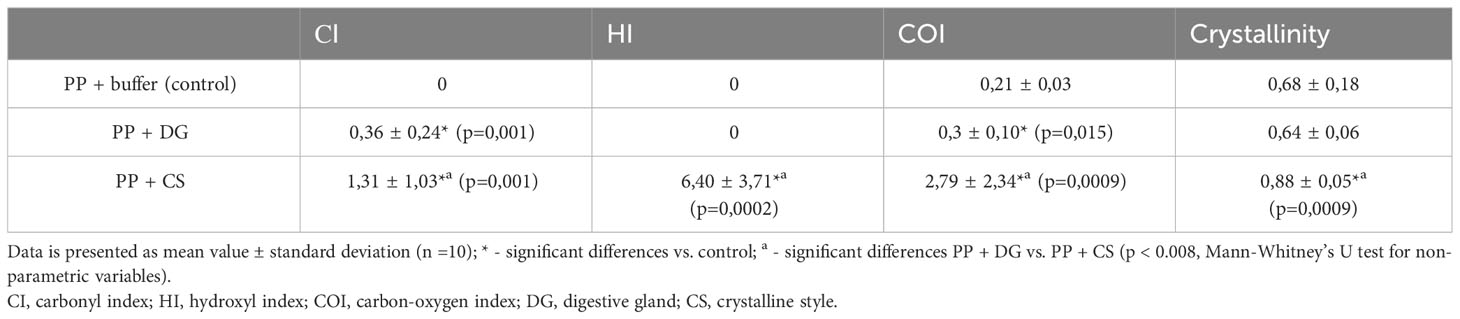
Table 1 Comparison of the polypropylene (PP) degradation indices and crystallinity after incubation in tissues homogenates.
Based on degradation indices, crystalline styles homogenate causes significant changes in the chemical composition of PP surface chains. In another case, treatment of PP fragments with digestive gland homogenate apparently affected these processes to a less extent, as it is manifested by a relatively small increase in CI and COI.
The oxidative degradation of PP, which results from the introduction of oxygen-containing functional groups, can affect the physical characteristics of the polymer. One of them is crystallinity, which characterizes the degree of ordering of the polymer chains (Rouillon et al., 2016; Wu et al., 2021). In our experiments, this index calculated from the IR spectra, increased significantly only in PP samples treated with crystalline styles homogenate (Table 1).
4 Discussion
Among various methods for studying the environmental behavior of synthetic polymers, one of the leading methods is infrared spectroscopy (FTIR). This approach is a highly selective and non-destructive method of analyzing functional groups in the structure of the polymer chain, which allows not only to identify the type of polymer, but also to detect changes in its structure. This method was particularly applied to characterize structural changes in plastics initiated by abiotic (temperature, oxygen concentration and UV) and biotic (microorganisms) influences (Skariyachan et al., 2018; Almond et al., 2020; Villalobos et al., 2022; Campanale et al., 2023).
In general, the whole complex of changes detected in experimental samples of PP indicates oxidative degradation of polymer chains, which can be considered as the first stage of the biodegradation process (Lucas et al., 2008). On the other hand, the addition of oxygen-containing functional groups to the polymer chains of PP increases the hydrophilicity of the polymer, making it more accessible for further biodegradation steps (Miri et al., 2022).
Indirect confirmation of our results can be found in single studies of microplastics in the digestive system or in the fecal remains of organisms. For example, in polyethylene (PE) and PP microplastics ingested by representatives of the mussels, changes in IR and Raman spectra were reported (Kumar et al., 2020). The IR spectra of polyethylene terephthalate (PET) in the feces of sea urchin Paracentrotus lividus differed from those of control samples (Parolini et al., 2020). A prime example is the study by Hamzah and colleagues (Hamzah et al., 2021), who used FTIR to reveal major changes in the composition of functional groups in PP and polyamide (PA) micro fragments extracted from the digestive system of the estuarine deposit-feeding polychaete Nomalycastis sp. Analysis of fragments of expanded polystyrene (EPS) isolated from the fecal remains of the terrestrial gastropod Achatinafulica showed that this polymer underwent severe chemical modification in the digestive tract (Song et al., 2020). It was also found that after staying in the digestive tract of periwinkles Littorina brevicula micro fragments of polymethylmethacrylate (PMMA), the physicochemical characteristics significantly changed (Odintsov et al., 2022).
Concerning the type of our experimental approach, it can be assumed that enzymes capable of degrading synthetic polymers, particular PP, are present in the digestive system of the mollusk, particular in the digestive gland and crystalline styles, which play a key role in digestion and assimilation of food. This assumption is not surprising if we take into account the digestive characteristics of marine bivalves, including the scallop M. yessoensis. Bivalves are planktonic filter feeders whose food composition is dominated by microalgae, bacteria, and macroalgae. Accordingly, the digestive gland and crystalline styles synthesize and actively secrete various digestive enzymes involved to varying degrees in the breakdown and degradation of natural polymers, particular complex polysaccharides that form the basis of the cell membrane structure of phytoplankton, marine bacteria, and algae. Hydrolases, esterase, and carbohydrase with different substrate specificities (amilase, cellulase, laminarinase, alginatelyase, etc.) were found as part of these digestive enzymes (Fernández-Reiriz et al., 2001; Milke et al., 2012; Lyu et al., 2016; Trestrail et al., 2021; González et al., 2022). It should be noted, however, that crystalline styles are characterized by a higher activity of carbohydrases (cellulase, laminarinase and amylase) compared with the digestive gland (Wojtowicz, 1972; Fernández-Reiriz et al., 2001). Based on the results obtained, an assumption can be made these features of the crystalline styles result in the stronger degradation of PP fragments compared with the digestive gland (Table 1).
Within the framework of this problem, it should be emphasized that, on the basis of the in vitro approach, we are among the first to demonstrate the potential ability of a marine invertebrate to accelerate PP biodegradation. Until now, the ability to degrade different types of polymers was attributed mainly to enzymes produced by diverse groups of microorganisms (Lucas et al., 2008; Skariyachan et al., 2018; Othman et al., 2021; Miri et al., 2022; Villalobos et al., 2022). In addition, there is strong evidence in scientific paper for the biodegradation of different types of polymers with the participation of insect larvae such as the common mealworm Tenebrio molitor (Brandon et al., 2018; Przemieniecki et al., 2019; Peng et al., 2020; Tsochatzis et al., 2021; Yang et al., 2021; Peng et al., 2023), waxworms Galleria mellanella (Ren et al., 2019; Billen et al., 2020; Lou et al., 2020) and Achroia grisella (Kundungal et al., 2019), and waxworms Zophabas atratus (Yang et al., 2020). However, in the case of examples with insect larvae, the possibility of the participation of enzymes of symbiotic microorganisms in the biodegradation of polymers cannot be excluded. Concerning that the digestive tract of marine bivalves, including the scallop M. yessoensis, also contains communities of specific symbiotic microorganisms (Lu et al., 2017; Ma et al., 2019), this possibility remains when explaining the authors’ results. Although specific investigations of this issue are beyond the scope of this study, it should be recognized that symbiotic microorganisms are an integral part of a single digestive process in which it is difficult to separate the digestive enzymes produced by microorganisms and host cells.
On the basis of the above reasoning, it is logical to assume that the appearance of defects in the polymer chain because of the introduction of functional groups (C=O, C–O–R, C–OH, and C=C) indicates the initiation and acceleration of the processes of oxidative degradation of PP after treatment of these fragments with a complex of digestive enzymes of the digestive gland and crystalline styles of the scallop. It should be emphasized that the authors detected accelerated chemical modification of PP during 3-day experiments, whereas under natural conditions, microplastics can be retained and subjected to enzymatic attack for longer periods of time. Furthermore, in marine ecosystems, this process may be repeated many times with other individuals of the species or by other organisms with different feeding behavior. In essence, from an ecological point of view, marine phytophagous filter-feeding invertebrates can accelerate the biotransformation of artificial polymers and ultimately participate in the removal of these anthropogenic compounds from marine ecosystems. Thus, the lifetime of plastic should be significantly less than it is currently assumed. Although this hypothesis requires further research, it is logical to assume that the results of these studies provide a basis for reconsidering the nature of the relationship between marine invertebrates and marine polluting microplastics.
Data availability statement
The raw data supporting the conclusions of this article will be made available by the authors, without undue reservation.
Ethics statement
The manuscript presents research on animals that do not require ethical approval for their study.
Author contributions
VC: Writing – original draft, Writing – review & editing. AI: Writing – original draft, Writing – review & editing. AM: Writing – original draft, Writing – review & editing. AZ: Writing – original draft, Writing – review & editing.
Funding
The author(s) declare financial support was received for the research, authorship, and/or publication of this article. The research was supported by the State assignment for research work of V.I. Il’ichev Pacific Oceanological Institute, FEB RAS (no. 121021500052-9) at the marine experimental station “Popov Island” Il’ichev Pacific Oceanological Institute. This research did not receive any specific grant from funding agencies in the public, commercial, or not-for-profit sectors.
Conflict of interest
The authors declare that the research was conducted in the absence of any commercial or financial relationships that could be construed as a potential conflict of interest.
Publisher’s note
All claims expressed in this article are solely those of the authors and do not necessarily represent those of their affiliated organizations, or those of the publisher, the editors and the reviewers. Any product that may be evaluated in this article, or claim that may be made by its manufacturer, is not guaranteed or endorsed by the publisher.
References
Ahmed R., Hamid A. K., Krebsbach S. A., He J., Wang D. (2022). Critical review of microplastics removal from the environment. Chemosphere. 293, 133557. doi: 10.1016/j.chemosphere.2022.133557
Almond J., Sugumaar P., Wenzel M. N., Hill G., Wallis C. (2020). Determination of the carbonyl index of polyethylene and polypropylene using specified area under band methodology with ATR-FTIR spectroscopy. e-Polymers. 20, 369–381. doi: 10.1515/epoly-2020-0041
Auta H. S., Emenike C. U., Jayanthi B., Fauziah S. H. (2018). Growth kinetics and biodeterioration of polypropylene microplastics by Bacillus sp. and Rhodococcus sp. isolated from mangrove sediment. Mar. pollut. Bull. 127, 15–21. doi: 10.1016/j.marpolbul.2017.11.036
Avio C. G., Gorbi S., Milan M., Benedetti M., Fattorini D., d.Errico G., et al. (2015). Pollutants bioavailability and toxicological risk from microplastics to marine mussels. Environ. pollut. 198, 211–222. doi: 10.1016/j.envpol.2014.12.021
Billen P., Khalifa L., Van Gerven F., Tavernier S., Spatari S. (2020). Technological application potential of polyethylene and polystyrene biodegradation by macro-organisms such as mealworms and wax moth larvae. Sci. Total Environ. 735, 139521. doi: 10.1016/j.scitotenv.2020.139521
Bom F. C., Sá F. (2021). Concentration of microplastics in bivalves of the environment: A systematic review. Environ. Monit. Assess. 193, 846. doi: 10.1007/s10661-021-09639-1
Born M. P., Brüll C. (2022). From model to nature — A review on the transferability of marine (micro-) plastic fragmentation studies. Sci. Total Environ. 811, 151389. doi: 10.1016/j.scitotenv.2021.151389
Brandon A. M., Gao S.-H., Tian R., Ning D., Yang S.-S., Zhou J., et al. (2018). Biodegradation of polyethylene and plastic mixtures in mealworms (larvae of Tenebrio molitor) and effects on the gut microbiome. Environ. Sci. Technol. 52, 6526–6533. doi: 10.1021/acs.est.8b02301
Campanale C., Savino I., Massarelli C., Uricchio V. F. (2023). Fourier transform infrared spectroscopy to assess the degree of alteration of artificially aged and environmentally weathered microplastics. Polymers. 15, 911. doi: 10.3390/polym15040911
Chamas A., Moon H., Zheng J., Qiu Y., Tabassum T., Jang J. H., et al. (2020). Degradation rates of plastics in the environment. ACS Sustain. Chem. Eng. 8, 3494–3511. doi: 10.1021/acssuschemeng.9b06635
Chelomin V. P., Mazur A. A., Slobodskova V. V., Kukla S. P., Dovzhenko N. V. (2022). Genotoxic properties of polystyrene (PS) microspheres in the filter-feeder mollusk mytilus trossulus (Gould 1850). J. Mar. Sci. Eng. 10 (2), 273. doi: 10.3390/jmse10020273
De Bomfim A. S. C., Maciel M.M.Á.D., Voorwald H. J. C., Benini K. C. C., de C., de Oliveira D. M., et al. (2019). Effect of different degradation types on properties of plastic waste obtained from espresso coffee capsules. Waste Management. 83, 123–130. doi: 10.1016/j.wasman.2018.11.006
De Paoli M. A. (2008). Degradação e Estabilização de Polímeros. Chemkeys 2, 221. Available at: https://docplayer.com.br/3259991-Degradacao-e-estabilizacao-de-polimeros.html.
Dellisanti W., Leung M. M. L., Lam K. W. K., Wan Y., Hu M., Lo H. S., et al. (2023). A short review on the recent method development for extraction and identification of microplastics in mussels and fish, two major groups of seafood. Mar. pollut. Bull. 186, 114221. doi: 10.1016/j.marpolbul.2022.114221
Détrée C., Gallardo-Escárate C. (2018). Single and repetitive microplastics exposures induce immune system modulation and homeostasis alteration in the edible mussel Mytilus galloprovincialis. Fish Shellfish Immunol. 83, 52–60. doi: 10.1016/j.fsi.2018.09.018
Duan J., Li Y., Gao J., Cao R., Shang E., Zhang W. (2022). ROS-mediated photoaging pathways of nano- and micro-plastic particles under UV irradiation. Water Res. 216, 118320. doi: 10.1016/j.watres.2022.118320
Fernández-Reiriz M. J., Labarta U., Navarro J. M., Velasco A. (2001). Enzymatic digestive activity in Mytilus Chilensis (Hupe 1854) in response to food regimes and past feeding history. J. Comp. Physiol. B. 171, 449–456. doi: 10.1007/s003600100194
González R., González D., Stambuk F., Ramírez F., Guzmán F., Mercado L., et al. (2022). A g-type lysozyme from the scallop Argopecten purpuratus participates in the immune response and in the stability of the hemolymph microbiota. Fish Shellfish Immunol. 123, 324–334. doi: 10.1016/j.fsi.2022.03.015
Gopanna A., Mandapati R. N., Thomas S. P., Rajan K., Chavali M. (2019). Fourier transform infrared spectroscopy (FTIR), Raman spectroscopy and wide-angle X-ray scattering (WAXS) of polypropylene (PP)/cyclic olefin copolymer (COC) blends for qualitative and quantitative analysis. Polymer Bull. 76, 4259–4274. doi: 10.1007/s00289-018-2599-0
Hamzah S. R., Altrawneh R. S., Anuar S. T., Khalik W.M.A.W.M., Kolandhasamy P., Ibrahim Y. S. (2021). Ingestion of microplastics by the estuarine polychaete, Namalycastis sp. in the Setiu Wetlands, Malaysia. Mar. pollut. Bull. 170, 112617. doi: 10.1016/j.marpolbul.2021.112617
Idris S. N., Amelia T. S. M., Bhubalan K., Lazim A. M. M., Zakwan N. A. M. A., Jamaluddin M. I., et al. (2023). The degradation of single-use plastics and commercially viable bioplastics in the environment: A review. Environ. Res. 231, 115988. doi: 10.1016/j.envres.2023.115988
Jadaun J. S., Bansal S., Sonthalia A., Rai A. K., Singh S. P. (2022). Biodegradation of plastics for sustainable environment. Bioresour. Technol. 347, 126697. doi: 10.1016/j.biortech.2022.126697
Jeong C. B., Won E. J., Kang H. M., Lee M. C., Hwang D. S., Hwang U., et al. (2016). Microplastic size-dependent toxicity, oxidative stress induction, and p JNK and p-p38 activation in the monogonont rotifer (Brachionus koreanus). Environ. Sci. Technol. 50 (16), 8849–8857. doi: 10.1021/acs.est.6b01441
Koelmans A. A., Gouin T., Thompson R., Wallace N., Arthur C. (2014). Plastics in the marine environment. Environ. Toxicol. Chem. 33, 5–10. doi: 10.1002/etc.2426
Kumar B. N. V., Löschel L. A., Imhof H. K., Löder M. G. J., Laforsch C. (2020). Analysis of microplastics of a broad size range in commercially important mussels by combining FTIR and Raman spectroscopy approaches. Environ. Pollut. 269, 116147. doi: 10.1016/j.envpol.2020.116147
Kundungal H., Gangarapu M., Sarangapani S., Patchaiyappan A., Devipriya S. P. (2019). Efficient biodegradation of polyethylene (HDPE) waste by the plastic-eating lesser waxworm (Achroia grisella). Environ. Sci. pollut. Res. 26, 18509–18519. doi: 10.1007/s11356-019-05038-9
Lambert S., Wagner M. (2016). Formation of microscopic particles during the degradation of different polymers. Chemosphere. 161, 510–517. doi: 10.1016/j.chemosphere.2016.07.042
Li W. C., Tse H. F., Fok L. (2016). Plastic waste in the marine environment: A review of sources, occurrence and effects. Sci. Total Environ. 566-567, 333–349. doi: 10.1016/j.scitotenv.2016.05.084
Liu L., Xu M., Ye Y., Zhang B. (2021). On the degradation of (micro)plastics: Degradation methods, influencing factors, environmental impacts. Sci. Total Environ. 806 Part 3, 151312. doi: 10.1016/j.scitotenv.2021.151312
Lou Y., Ekaterina P., Yang S., Lu B., Liu B.-F., Ren N., et al. (2020). Bio-degradation of polyethylene and polystyrene by greater wax moth larvae (Galleria mellonella L.) and the effect of co-diet supplementation on the core gut microbiome. Environ. Sci. Technol. 54, 2821–2831. doi: 10.1021/acs.est.9b07044
Lu G., Wang F., Yu Z., Lu M., Wang Y., Liu C., et al. (2017). Bacterial communities in gills and intestines of Yesso scallop (Patinopecten yessoensis) and its habitat waters in Changhai (Dalian, China). ISJ. 14, 340–351. doi: 10.25431/1824-307X/isj.v14i1.340-351
Lucas N., Bienaime C., Belloy C., Queneudec M., Silvestre F., Nava-Saucedo J.-E. (2008). Polymer biodegradation: Mechanisms and estimation techniques – A review. Chemosphere 73, 429–442. doi: 10.1016/j.chemosphere.2008.06.064
Lyu Q., Jiao W., Zhang K., Bao Z., Wang S., Liu W. (2016). Proteomic analysis of scallop hepatopancreatic extract provides insights into marine polysaccharide digestion. Sci. Rep. 6, 34866. doi: 10.1038/srep34866
Ma Y., Li M., Sun J., Hao Z., Liang J., Zhao X. (2019). Characterization of bacterial community associated with four organs of the yesso scallop (Patinopecten yessoensis) by high-throughput sequencing. J. Ocean Univ. China. 18, 493–500. doi: 10.1007/s11802-019-3791-z
Matjašič T., Simčič T., Medvešček N., Bajt O., Dreo T., Mori N. (2020). Critical evaluation of biodegradation studies on synthetic plastics through a systematic literature review. Sci. Total Environ. 752, 141959. doi: 10.1016/j.scitotenv.2020.141959
Mattsson K., Björkroth F., Karlsson T., Hassellöv M. (2021). Nano fragmentation of expanded polystyrene under simulated environmental weathering (Thermooxidative degradation and hydrodynamic turbulence). Front. Mar. Sci. 7. doi: 10.3389/fmars.2020.578178
Milke L. M., Bricelj V. M., Ross N. W. (2012). Changes in enzymatic activity during early development of bay scallops Argopecten irradians and sea scallops Placopecten magellanicus. Aquat. Biol. 14, 207–216. doi: 10.3354/ab00398
Miri S., Saini R., Davoodi S. M., Pulicharla R., Brar S. K., Magdouli S. (2022). Biodegradation of microplastics: Better late than never. Chemosphere 286, 131670. doi: 10.1016/j.chemosphere.2021.131670
Odintsov V. S., Karpenko A. A., Karpenko M. A. (2022). Degradation of micro−nano−sized polytetrafluoroethylene and acrylic fluorinated copolymer particles in the periwinkle digestive tract. Environ. Sci. pollut. Res. 30, 1–9. doi: 10.1007/s11356-022-23996-5
Othman A. R., Hasan H. A., Muhamad M. H., Ismail N.’I., Abdullah S. R. S. (2021). Microbial degradation of microplastics by enzymatic processes: a review. Environ. Chem. Lett. 19, 3057–3073. doi: 10.1007/s10311-021-01197-9
Parolini M., Ferrario C., De Felice B., Gazzotti S., Bonasoro F., Candia Carnevali M. D., et al. (2020). Interactive effects between sinking polyethylene terephthalate (PET) microplastics deriving from water bottles and a benthic grazer. J. Hazard. Mater. 398, 122848. doi: 10.1016/j.jhazmat.2020.122848
Peng B.-Y., Li Y., Fan R., Chen Z., Chen J., Brandon A. M., et al. (2020). Biodegradation of low-density polyethylene and polystyrene in superworms, larvae of Zophobas atratus (Coleoptera: Tenebrionidae): Broad and limited extent depolymerization. Environ. Pollut. 266 (Pt1), 115206. doi: 10.1016/j.envpol.2020.115206
Peng B.-Y., Sun Y., Zhang X., Sun J., Xu Y., Xiao S., et al. (2023). Unveiling the residual plastics and produced toxicity during biodegradation of polyethylene (PE), polystyrene (PS), and polyvinyl chloride (PVC) microplastics by mealworms (larvae of Tenebrio molitor). J. Hazard. Mater. 452, 131326. doi: 10.1016/j.jhazmat.2023.131326
Przemieniecki S. W., Kosewska A., Ciesielski S., Kosewska O. (2019). Changes in the gut microbiome and enzymatic profile of Tenebrio molitor larvae biodegrading cellulose, polyethylene and polystyrene waste. Environ. Pollut. 256, 113265. doi: 10.1016/j.envpol.2019.113265
Ren L., Men L., Zhang Z., Guan F., Tian J., Wang B., et al. (2019). Biodegradation of polyethylene by Enterobacter sp. D1 from the guts of wax moth Galleria mellonella. Int. J. Environ. Res. Public Health 16, 1941. doi: 10.3390/ijerph16111941
Revel M., Lagarde F., Perrein-Ettajani H., Bruneau M., Akcha F., Sussarellu R., et al. (2019). Tissue-specific biomarker responses in the blue mussel Mytilus spp. exposed to a mixture of microplastics at environmentally relevant concentrations. Front. Environ. Sci. 7. doi: 10.3389/fenvs.2019.00033
Rouillon C., Bussiere P.-O., Desnoux E., Collin S., Vial C., Therias S., et al. (2016). Is carbonyl index a quantitative probe to monitor polypropylene photodegradation? Polym. Degrad. Stab. 128, 200–208. doi: 10.1016/j.polymdegradstab.2015.12.011
Sarmah P., Rout J. (2018). Efficient biodegradation of low-density polyethylene by cyanobacteria isolated from submerged polyethylene surface in domestic sewage water. Environ. Sci. pollut. Res. 25, 33508–33520. doi: 10.1007/s11356-018-3079-7
Sarmah P., Rout J. (2020). “Role of algae and cyanobacteria in bioremediation: prospects in polyethylene biodegradation,” Advances Cyanobacterial Biology, ed. Singh P. K., Kumar A., Singh K., Shrivastava A. K. (Academic Press), 333–349. doi: 10.1016/b978-0-12-819311-2.00022-x
Skariyachan S., Patil A. A., Shankar A., Manjunath M., Bachappanavar N., Kiran S. (2018). Enhanced polymer degradation of polyethylene and polypropylene by novel thermophilic consortia of Brevibacillus sps. and Aneurinibacillus sp. screened from waste management landfills and sewage treatment plants. Polym. Degrad. Stab. 149, 52–68. doi: 10.1016/j.polymdegradstab.2018.01.018
Song Y., Qiu R., Hu J., Li X., Zhang X., Chen Y., et al. (2020). Biodegradation and disintegration of expanded polystyrene by land snails Achatina fulica. Sci. Total Environ. 746, 141289. doi: 10.1016/j.scitotenv.2020.141289
Thomas P. J., Oral R., Pagano G., Tez S., Toscanesi M., Ranieri P., et al. (2020). Mild toxicity of polystyrene and polymethylmethacrylate microplastics in Paracentrotus lividus early life stages. Mar. Environ. Res. 161, 105132. doi: 10.1016/j.marenvres.2020.105132
Tokiwa Y., Calabia B., Ugwu C., Aiba S. (2009). Biodegradability of plastics. Int. J. Mol. Sci. 10, 3722–3742. doi: 10.3390/ijms10093722
Trestrail C., Walpitagama M., Miranda A., Nugegoda D., Shimeta J. (2021). Microplastics alter digestive enzyme activities in the marine bivalve, Mytilus galloprovincialis. Sci. Total Environ. 779, 146418. doi: 10.1016/j.scitotenv.2021.146418
Trifuoggi M., Pagano G., Oral R., Pavičić-Hamer D., Burić P., Kovačcić I., et al. (2019). Microplastic-induced damage in early embryonal development of sea urchin Sphaerechinus granularis. Environ. Res. 179, 108815. doi: 10.1016/j.envres.2019.108815
Tsochatzis E. D., Berggreen I. E., Nørgaard J. V., Theodoridis G., Dalsgaard T. K. (2021). Biodegradation of expanded polystyrene by mealworm larvae under different feeding strategies evaluated by metabolic profiling using GC-TOF-MS. Chemosphere 281, 130840. doi: 10.1016/j.chemosphere.2021.130840
Villalobos N. F., Costa M. C., Marín-Beltrán I. (2022). A community of marine bacteria with potential to biodegrade petroleum-based and biobased microplastics. Mar. pollut. Bull. 185, 114251. doi: 10.1016/j.marpolbul.2022.114251
Ward C. P., Armstrong C. J., Walsh A. N., Jackson J. H., Reddy C. M. (2019). Sunlight converts polystyrene to carbon dioxide and dissolved organic carbon. Environ. Sci. Technol. Lett. 6 (11), 669–674. doi: 10.1021/acs.estlett.9b00532
Wojtowicz M. B. (1972). Carbohydrases of the digestive gland and the crystalline style of the atlantic deep-sea scallop (Placopecten magellanicus, gmelin). Comp. Biochem. Physiol. A: Physiol. 43, 131–141. doi: 10.1016/0300-9629(72)90475-6
Wu X., Liu P., Wang H., Huang H., Shi Y., Yang C., et al. (2021). Photo aging of polypropylene microplastics in estuary water and coastal seawater: Important role of chlorine ion. Water Res. 202, 117396. doi: 10.1016/j.watres.2021.117396
Wu W.-M., Yang J., Criddle C. S. (2016). Microplastics pollution and reduction strategies. Front. Environ. Sci. Eng. 11, 6. doi: 10.1007/s11783-017-0897-7
Xi B., Wang B., Chen M., Lee X., Zhang X., Wang S., et al. (2022). Environmental behaviors and degradation methods of microplastics in different environmental media. Chemosphere. 299, 134354. doi: 10.1016/j.chemosphere.2022.134354
Xia Y., Niu S., Yu J. (2023). Microplastics as vectors of organic pollutants in aquatic environment: A review on mechanisms, numerical models, and influencing factors. Sci. Total Environ. 887, 164008. doi: 10.1016/j.scitotenv.2023.164008
Yang S.-S., Ding M.-Q., Zhang Z.-R., Ding J., Bai S.-W., Cao G.-L., et al. (2021). Confirmation of biodegradation of low-density polyethylene in dark- versus yellow- mealworms (larvae of Tenebrio obscurus versus Tenebrio molitor) via. gut microbe-independent depolymerization. Sci. Total Environ. 789, 147915. doi: 10.1016/j.scitotenv.2021.147915
Keywords: biotransformation of plastic, Fourier transform infrared spectroscopy, carbonyl index, hydroxyl index, carboxyl index
Citation: Chelomin VP, Istomina AA, Mazur AA and Zhukovskaya AF (2024) Biodegradation of polypropylene by filter-feeding marine scallop Mizuhopecten yessoensis: infrared spectroscopy evidence. Front. Mar. Sci. 11:1362231. doi: 10.3389/fmars.2024.1362231
Received: 27 December 2023; Accepted: 09 February 2024;
Published: 21 February 2024.
Edited by:
Jeff Shimeta, RMIT University, AustraliaReviewed by:
Tan Suet May Amelia, University of Malaysia Terengganu, MalaysiaNur Adeela Yasid, Universiti Putra Malaysia, Malaysia
Copyright © 2024 Chelomin, Istomina, Mazur and Zhukovskaya. This is an open-access article distributed under the terms of the Creative Commons Attribution License (CC BY). The use, distribution or reproduction in other forums is permitted, provided the original author(s) and the copyright owner(s) are credited and that the original publication in this journal is cited, in accordance with accepted academic practice. No use, distribution or reproduction is permitted which does not comply with these terms.
*Correspondence: Aleksandra Anatolyevna Istomina, s-istomina1@mail.ru