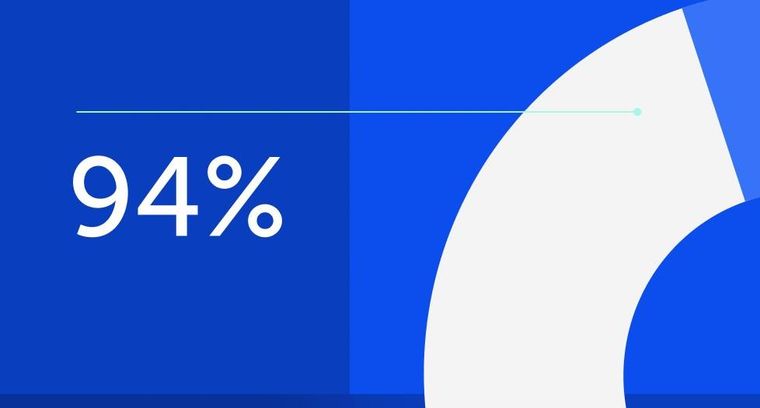
94% of researchers rate our articles as excellent or good
Learn more about the work of our research integrity team to safeguard the quality of each article we publish.
Find out more
BRIEF RESEARCH REPORT article
Front. Mar. Sci., 25 March 2024
Sec. Marine Fisheries, Aquaculture and Living Resources
Volume 11 - 2024 | https://doi.org/10.3389/fmars.2024.1360596
The razor clam Sinonovacula constricta, is one of the most commercially important cultured bivalves in China and Southeast Asia, while S. rivularis is its closer relatives discovered more than a decade ago. In order to obtain offspring with faster growth rate and stronger salt tolerance of S. constricta and S. rivularis, interspecific hybrids were produced, and the hybridity of the interspecific hybrids was confirmed by microsatellite markers. Microsatellite markers exhibit a high potential for transfer through cross-amplification in related species, and the transferability of 48 pairs of microsatellite marker primers from S. constricta were assessed in S. rivularis. Here, 24 universal microsatellite markers were successfully amplified in S. rivularis, of which 18 were polymorphic with the allele number from 2 to 5. The genetic diversity of two razor clams evaluated by 18 polymorphic microsatellite markers indicated that two species were both above the middle level, with a relatively higher genetic diversity, while S. constricta showed higher genetic diversity than S. rivularis according to the genetic parameters of Na, Ho, He and PIC. Furthermore, a total of two species-specific microsatellite markers were screened, which could be used for quick genetic identification of S. constricta, S. rivularis and their hybrids. The results suggest the induced interspecific hybrids are true hybrids between S. constricta and S. rivularis, which provide a basis for breeding, subsequent protection, and germplasm resources utilization of the razor clams.
The razor clam Sinonovacula constricta is one of the most commercially and ecologically important bivalves in China and Southeast Asia, while S. rivularis is the closer relative of S. constricta, belongs to genus Sinonocavula, and is first found in Minjiang estuary waters of Fujian province (China) in 2007 (Huang and Zhang, 2007). It has the characteristics of fast growth, high economic value and environmental friendliness, and has been promoted for aquaculture in coastal areas of Fujian province of China. For a long time, S. rivularis was considered as S. constricta because of its overlapping ecological habits and similar morphology. However, S. rivularis was identified as a new species by shell length/shell height ratio, sperm morphology and so on (Huang and Zhang, 2007). As for S. constricta, it has high tolerance to broad range of salinities and can adapt to salinity around 6.5-26.2 ppt (Ran et al., 2017). Unlike S. constricta, S. rivularis possesses strong low salt tolerance (salinity 5.2-7.8 ppt), which is suitable for living in low salt water areas, and can even survive for more than 4 d in freshwater (Huang and Zhang, 2007; Huang et al., 2011; Weng et al., 2013).
Hybridization has been considered as a possibly efficient method to increase growth rate and adaptability to environmental conditions, which has been widely used in classic breeding in plant and livestock production. Crossbreeding has been widely used for genetic improvement in commercial shellfish species, such as oysters, scallops and abalones (Guo, 2009; Luo et al., 2010; You et al., 2015). Some interspecies hybrids of shellfish have been commercially used to improve growth rate, survival rate, food conversion and stress resistance (Lafarga de la Cruz and Gallardo-Escárate, 2011). For example, Pacific abalone Haliotis discus hannai and red abalone H. rufescens were successfully hybridized to obtain hybrids showing positive heterosis in growth and survival rates compared to parental species (Lafarga de la Cruz et al., 2010), and H. discus hannai were successfully hybridized with green abalone H. fulgens, with hybrids exhibited hybrid vigor and adaptation to the higher temperature tolerance (You et al., 2015). S. constricta and S. rivularis are close relatives, and the hybrids with faster growth rate and stronger salt tolerance (strong lower and higher salt tolerance) have been successfully produced. However, identification of hybridized individuals is a primary importance for breeding and the germplasm conservation of S. constricta and S. rivularis.
To date, previous studies have reported several methods to distinguish S. constricta and S. rivularis. Huang et al. (2011) compared the ultrastructure of mature sperms of S. rivularis and S. constricta by scanning electron microscope and transmission electron microscopy for differentiating them. Subsequently, molecular identification of S. constricta and S. rivularis were conducted by mitochondrial COІ and 16S rRNA analysis (Weng et al., 2013). However, there has been no method to effectively identify their hybrids, which is critical for breeding projects and conservation of two species.
Microsatellites, also known as simple sequence repeats (SSRs), are molecular markers developed in the late 1980s. At present, they have been widely used in genetic diversity analysis, genetic relationship analysis, pedigree identification, genetic map construction and many other fields due to their wide distribution, rich polymorphism, co-dominant inheritance and convenient detection in aquatic animals (Li, 2006). In mollusks, microsatellites have been developed in a wide number of species, such as Zhikong scallop Chlamys farreri (Zhan et al., 2008), Pacific oyster Crassostrea gigas (Li et al., 2011), blood clam Tegillarca granosa (Dong et al., 2012; Liu et al., 2012a), hard clam Meretrix meretrix (Dong et al., 2018) and so on. Since the flanking sequences of microsatellite markers are highly conserved between species, so microsatellite markers have been shown to possess a high potential for inter-specific transferability, which is of great significance to develop microsatellites for species lacking of genome and transcriptome information (Zhang et al., 2018). Cross-species amplification of microsatellites has been successfully performed in mollusks. For example, 21 microsatellite markers from scallop Patinopecten yessoensis were amplified in C. farreri (Zhang et al., 2018), 30 microsatellite markers of M. meretrix were tested for versatility in M. lamarckii and M. lyrata (Qi et al., 2013), and 34 polymorphic microsatellite markers of T. granosa were amplified in Anadara craticulata (Dong et al., 2013a), etc. Moreover, microsatellites are somehow conserved in related species, and have also been used as species-specific markers to distinguish related species and their hybrids if exclusive alleles are present for each species (Luo et al., 2010). It has been reported that 20 polymorphic microsatellite markers developed from abalone H. discus hannai were used in cross-amplification in H. gigantean, and eight were polymorphic, two of which could be used as species-specific markers to distinguish the hybrids and their parental species (Luo et al., 2010). Abalone H. fulgens, H. rufescens and their hybrids could also be distinguished by species-specific microsatellites (Cruz et al., 2005).
Until now, some microsatellites of S. constricta have been developed (Jiang et al., 2010; Liu et al., 2012b; Ma et al., 2015; Dong et al., 2016; Wang et al., 2016), and polymorphic loci were amplified in Solen linearis by cross-species amplification (Dong et al., 2016). However, the microsatellite markers of S. rivularis have not been developed yet due to lacking of genomic and transcriptomic information. Moreover, other methods for the development of microsatellite markers are costly and inefficient. In the present study, we have performed a quantitative assessment of microsatellites from S. constricta to be transferred to closely related species S. rivularis, and these microsatellites were then used to identify S. constricta, S. rivularis and their hybrids, which lay the foundation for breeding, subsequent protection, and germplasm resources utilization of the razor clams.
In October 2021, each 1000 clams from S. constricta (average shell length 52.45 ± 3.70 mm and total body weight 11.98 ± 2.02g, one year old) and S. rivularis (average shell length 52.19 ± 3.20 mm and total body weight 9.96 ± 2.62 g, one year old) were sampled from Ninghai, Zhejiang province and Changle, Fujian province, China, respectively. Before crossing of S. constricta and S. rivularis, the parental samples have been pre-assessed to confirm pure individuals of each species by the morphology and genetic methods followed by Weng et al. (2013). When the brood stocks of two species were sexually mature, the interspecific hybridization trials were conducted. Mature clams were chosen and firstly kept dry in the shade, and then stimulated by flowing seawater to induce spawning. Sperms and eggs from different individuals of the same species were collected separately. The eggs from S. constricta were fertilized by sperms from S. rivularis to produce CR group (S. constricta♀×S. rivularis♂), while the eggs of S. rivularis were fertilized by sperms of S. constricta to generate RC group (S. rivularis♀×S. constricta♂). So two hybrids were produced: CR (S. constricta♀×S. rivularis♂) and RC (S. rivularis♀×S. constricta♂). After artificial insemination, the foot tissues of parental clams (n=30) were sampled and stored in 95% ethanol for DNA extraction.
The reciprocal hybrids were reared at tanks separately to prevent intermix, and foot tissues of live juvenile clams (n=30) of CR (shell length 12.45 ± 1.10 mm) and RC (shell length 11.47 ± 1.20 mm) were randomly sampled at 6 months of age and used for DNA extraction.
Total genomic DNA was extracted using a marine animal DNA extraction kit (Tiangen, China), and the quality and concentration of DNA were assessed by 1.2% agarose gel electrophoresis and Nanodrop ND-1000 spectrophotometer (Thermo Scientific, USA). All DNA samples were diluted to 100 ng/µL with Trise-EDTA buffer (pH 8.0) and stored at -20°C.
48 microsatellite markers from S. constricta (Liu et al., 2012b; Dong et al., 2016; Wang et al., 2016, Table 1) were selected and their primers were synthesized (Sangon Bioengineering, Shanghai). Cross-species amplification was carried out in S. rivularis and the polymorphic loci were identified. PCR amplifications were performed with Mastercycler Pro S thermal cycler in a 25 µL reaction system (12.5 µL 2×Taq Master Mix (Takara), 9.5 µL ddH2O, 1.0 µL DNA and 1 µL positive and negative primers). The reaction program was as follows: first denaturation at 94°C for 5 min, followed by 35 cycles of denaturation at 94°C for 45 s, the primer-specific annealing temperature (Table 1) for 45 s, and extension at 72°C for 45 s with a final extension at 72°C for 10 min. The PCR products were separated by electrophoresis on 8% nondenaturing polyacrylamide gel and visualized by ethidium bromide staining under UV light. Allele size was determined by a 500 bp ladder marker. Then, the expected PCR products were sequenced in Biotechnology Co., LTD, and sequence alignments were conducted by ClustalX 2.1 software.
Table 1 Characteristics of 24 universal microsatellites and their primer information used in the experiment.
The developed polymorphic microsatellite markers that stably amplified in S. rivularis were firstly used to analyze the genetic diversity of parental clams (S. constricta and S. rivularis), and species-specific microsatellite markers were then screened to identify two species and their hybrids (CR and RC) using 30 individuals, respectively.
The number of alleles (Na), direct count heterozygotes and homozygotes, and polymorphism information content (PIC) were calculated by CERVUS 3.0 genotype frequencies software (Kalinowski et al., 2007). The observed heterozygosity (Ho), expected heterozygosity (He) and effective number of alleles (Ne) were estimated by Popgen 32 software. The GENEPOP online software (http://genepop.curtin.edu.au) was used to calculate the Hardy-Weinberg Equilibrium (HWE), and all significant levels were adjusted by the Bonferroni correction.
48 pairs of microsatellite primers from S. constricta were assessed in S. rivularis, and the results revealed that 24 pairs of universal primers with clear bands were stably amplified in S. rivularis, with the transferability rate of 50.0% (Table 1). Furthermore, 18 pairs of primers were polymorphic, accounting for 37.5% of the total primers (Table 2).
Table 2 Cross-species amplification of 18 polymorphic microsatellite markers in S. constricta and S. rivularis.
The 18 polymorphic SSR markers were then used to evaluate the genetic diversity of S. constricta and S. rivularis, which displayed polymorphic differences between two species (Table 2). The number of alleles in S. constricta and S. rivularis populations ranged from 2.00~6.00 (an average of 3.1667 alleles) and 2.00~5.00 (an average of 2.7222 alleles), respectively. The Ho and He changed from 0.1333 to 1.0000 (0.6958 on average) and 0.1266 to 0.7339 (0.5386 on average) in S. constricta, respectively, while Ho and He of S. rivularis varied from 0.0333 to 1.0000 (0.5574 on average) and 0.0333 to 0.7006 (0.4376 on average), respectively. The polymorphic information content (PIC) ranged from 0.117 to 0.677 (0.4556 on average) in S. constricta, while it changed from 0.141 to 0.677 (0.4101 on average) in S. rivularis. After Bonferroni correction, 8 and 5 loci deviated from Hardy-Weinberg equilibrium in S. constricta and S. rivularis, respectively. In general, S. constricta showed higher genetic diversity than S. rivularis.
Of all 24 universal microsatellites, a total of two species-specific markers (comp147145_c0 and comp149438_c0) were screened between two species S. constricta and S. rivularis (Table 3; Supplementary Figure S1). Furthermore, the PCR products of these species-specific markers were sequenced, and the results showed that the repeat numbers of core repeating units were different between two species, which were consistent with above genotyping results (Table 3; Supplementary Figure S1). Overall, there is a significant difference in the number and length of fragments amplified and no allele overlap between two species by two species-specific microsatellites, which is useful for distinguishing S. constricta and S. rivularis.
Table 3 Sequence characteristics and parameters of two species-specific microsatellite markers in S. constricta and S. rivularis.
According to the principle that hybrid bands are parent-based complementary bands, the purity of hybrid can be identified quickly, accurately and efficiently. These two species-specific microsatellites were then used to verify the hybrids nature of the reciprocal hybrids (Figure 1). For microsatellite marker comp147145_c0, two bands (about 290 and 310bp) were amplified in S. constricta, only one band about 250bp was observed in S. rivularis, while the hybrids CR and RC crosses possessed two bands (about 290bp and 250bp) that inherited one band from each parent, respectively (Figure 1A). For microsatellite marker comp149438_c0, only one band about 165bp was amplified in S. constricta, only one band about 195bp appeared in S. rivularis, while these two bands (about 165bp and 195bp) were both appeared in the hybrids CR and RC that come from their parents (Figure 1B). Consequently, the hybrids CR and RC possessed bands derived from both parents amplified by microsatellites comp147145_c0 and comp149438_c0, which could be used for quick genetic identification of S. constricta, S. rivularis and their reciprocal hybrids.
Figure 1 The species-specific microsatellite markers in S. constricta (Sc), S. rivularis (Sr), CR (S. constricta♀×S. rivularis♂) and RC (S. rivularis♀×S. constricta♂). (A) comp147145_c0; (B) comp149438_c0.
Owing to relatively conservatism, microsatellite markers show a considerable degree of transferability to related species, and can be cross-species amplified in closely related species, which is especially important for some species lacking of research of interspecific evolutionary relationships, interspecific comparison mapping, molecular marker assisted breeding and so on (Li, 2006). Especially for some species lacking of microsatellite markers, the transferability is of great significance for the evaluation of genetic diversity and germplasm identification.
Previous studies have reported the cross-species amplification of microsatellite markers in mollusks. In two scallops, 21 microsatellite markers of P. yessensis could amplify specific bands in C. farreri, of which 17 markers were polymorphic, with a polymorphism proportion of 28.33% (Zhan et al., 2008). In another example, 15 microsatellite markers from Argopecten irradians irradians were used to cross-species amplified in C. farreri, P. yesoensis and Amusium pleuronectes, and the transferability rates were 60%, 60% and 20%, respectively (Li et al., 2008). In hard clams, the interspecific transferability of 53 polymorphic microsatellite markers from M. meretrix revealed that 19 polymorphic markers in M. lamarckii and 10 in M. lyrata were developed, resulting in the transferability rates of 35.8% and 18.9%, respectively (Dong et al., 2013b). In this study, 48 microsatellite markers of S. constricta were tested in S. rivularis, of which 24 markers were successfully amplified and 18 were polymorphic, with the transferability rate of 50.0%. Meanwhile, the polymorphic microsatellite markers of S. constricta were also cross-species amplified in S. linearis, and 20 markers could be amplified and displayed polymorphisms, resulting in a transferability rate of 33.3% (Dong et al., 2016), which was lower than that of S. rivularis in the present study. Previous studies have reported that the cross-species transferability rate of microsatellites depends upon the evolutionary distance between the source and target species, and the higher the genomic homology, the greater conservation of flanking regions, the higher transferability rate of microsatellite markers (FitzSimmons et al., 1995). One possible explanation was that S. constricta was more closely related to S. rivularis than S. linearis, and S. constricta and S. rivularis had a relatively closer affinity. Therefore, the microsatellite markers developed by cross-species amplification could enrich some species with fewer markers available, and especially could be utilized for genetic diversity, comparative mapping and evolutionary biology among different species.
Microsatellite markers are highly polymorphic and widely distributed in eukaryotic genomes (Schlötterer, 2000), which has been widely used in genetic diversity analysis, construction of genetic linkage maps, variety identification and so on (Li, 2006). In mollusks, microsatellite markers have also been widely used in genetic diversity analysis in Japanese scallop P. yesoensis (Li et al., 2014), Manila clam Ruditapes philippinarum (Tan et al., 2020), Eastern oyster Crassostrea virginica (Wang and Guo, 2007), etc. In the present study, 18 polymorphic microsatellite markers were developed by cross-species amplification, and were used to evaluate the genetic diversity of S. constricta and S. rivularis. From the genetic parameters of Na, Ho, He and PIC, S. constricta showed higher genetic diversity than S. rivularis. Genetic diversity of a population or species could be evaluated by PIC value, and PIC < 0.25 represents low polymorphism, 0.25 ≤ PIC ≤ 0.5 represents moderate polymorphism, and PIC ≥ 0.5 represents high polymorphism (Botstein et al., 1980). The average PIC of S. constricta and S. rivularis were 0.4556 and 0.4101, respectively, suggesting that the genetic diversity of two species both above the middle level, with a higher genetic diversity.
S. rivularis is similar in shape to S. constricta, including similar size, elongate quadrate, shell surface covered with a yellow-green periostracum, and an oblique furrow running from the umbo to ventral margin on the center of the shell, which is the unique character of S. constricta. Therefore, S. rivularis has always been considered to be S. constricta until it was recently identified as a new species in 2007. To identify two razor clams, shell phenotype (shell length/shell height ratio) and sperm morphology were used to distinguish them by Huang and Zhang (2007). Afterwards, the ultrastructure of mature sperms by electron microscope and transmission electron microscopy was applied to differentiate two species (Huang et al., 2011). With the advance of molecular genetics in recent decades, mitochondrial COІ and 16S rRNA fragments were also used to identify S. rivularis and S. constricta (Weng et al., 2013). Generally, the external morphological measurement method may have great instability and imprecision, because their shell morphology may change under the habitat condition influence. Moreover, this method is difficult to identify juvenile clams and their hybrids. The observation of mature sperm ultrastructure has to rely on expensive instruments (electron microscope and transmission electron microscopy), and can only be used in breeding season due to collecting mature sperms, while female clams and hybrids cannot be distinguished. The COІ and 16S rRNA, belonging to mitochondrial DNA, are maternally inherited, so that they cannot be used for hybrid identification. Therefore, it is of great significance to explore a simple, accurate and reliable method for identification of S. constricta, S. rivularis and their hybrids.
Hybridization has been used for the genetic improvement and played an important role in the development shellfish aquaculture, and the Pacific oyster is a good example (Guo, 2009). Meanwhile, hybridization identification is essential for breeding, conservation, and understanding of their biological and genetic differences. Hybrids of closely related species often possess intermediated morphological traits, including body shape and other meristic traits (Konopiński and Amirowicz, 2018; Elliott et al., 2020). In many cases, minor deviations away from one or the other parental species can lead to difficulty in correctly diagnosing pure from F1 individuals, particularly when relying on morphology alone (Lessios, 2007). So the identification of pure and hybrid lineages in fundamental in developing policies for the conservation and management of native species (Allendorf et al., 2001), and DNA molecular marker techniques have been widely applied for the identification of aquaculture species and their hybrids in mollusks. For example, nuclear and mitochondrial primers were used to identify hybrids of Mytilus coruscus × Mytilus galloprovincialis in mussel hatcheries of China (Zhang et al., 2020), and two-step PCR method using three primers was conducted to identify abalones H. discus hannai, H. fulgens and their hybrids (You et al., 2015).
In comparison with other molecular markers, microsatellite markers are highly abundant in various eukaryotic genomes, and provide more polymorphism information for distinguishing different species or identify hybridization in aquatic animals (Li, 2006; Luo et al., 2010). For example, abalones H. fulgens, H. rufescens and their hybrids could be distinguished by species-specific microsatellite markers (Luo et al., 2010). Cruz et al. (2005) also developed a quick diagnosis for genetic identification of hybrids between red and Pacific abalone using microsatellites, and two species-specific microsatellite markers of H. discus hannai and H. fulgens could be used to distinguish the hybrids and their parents. In the present study, two species-specific microsatellite markers (comp147145_c0 and comp149438_c0) were both amplified in two closely related species, and there is a significant difference in the number and length of fragments amplified without allele overlap in two razor clams, which could be used as species-specific markers to clearly distinguish two razor clams. Furthermore, these two microsatellite loci confirmed that the hybrids CR and RC consisted of two haploid sets, one originating from each parental species, which could be used for quick genetic identification of S. constricta, S. rivularis and their reciprocal hybrids.
Consequently, these results provide a valuable and convenient method for distinguishing among two species and crossbred offspring.
In the present study, interspecific hybrids of S. constricta and S. rivularis were produced, and the hybridity of the interspecific hybrids was confirmed by microsatellite markers. 24 microsatellite markers of S. constricta were successfully amplified in S. rivularis by cross-species amplification, of which 18 were polymorphic, resulting in the transferability rates of 50.0%. The genetic diversity of the two razor clams evaluated by 18 polymorphic markers indicated that S. constricta showed higher genetic diversity than S. rivularis. Furthermore, two species-specific microsatellite markers were screened, which could be used for quick genetic identification of S. constricta, S. rivularis and their hybrids. The results strongly suggest the induced interspecific hybrids are true hybrids, which is critical for breeding projects and the conservation of two razor clams.
The original contributions presented in the study are included in the article/Supplementary Material. Further inquiries can be directed to the corresponding authors.
The animal study was approved by Institutional Review Board of Institutional Animal Care and Use Committee (IACUC) of Zhejiang Wanli University, China. The study was conducted in accordance with the local legislation and institutional requirements.
SW: Data curation, Formal analysis, Visualization, Writing – original draft. YS: Formal analysis, Investigation, Visualization, Writing – review & editing. YD: Conceptualization, Funding acquisition, Writing – review & editing. YM: Data curation, Methodology, Writing – review & editing. HY: Funding acquisition, Investigation, Project administration, Resources, Writing – review & editing. LH: Conceptualization, Methodology, Visualization, Writing – review & editing.
The author(s) declare financial support was received for the research, authorship, and/or publication of this article. This research was funded by Ningbo Major Project of Science and Technology (2021Z114), Zhejiang Major Program of Science and Technology (2021C02069-7) and National Marine Genetic Resource Center Program.
We would like to thank Ningbo Ocean and Fishery Science and Technology Innovation Base for the provision of experimental sample.
The authors declare that the research was conducted in the absence of any commercial or financial relationships that could be construed as a potential conflict of interest.
All claims expressed in this article are solely those of the authors and do not necessarily represent those of their affiliated organizations, or those of the publisher, the editors and the reviewers. Any product that may be evaluated in this article, or claim that may be made by its manufacturer, is not guaranteed or endorsed by the publisher.
The Supplementary Material for this article can be found online at: https://www.frontiersin.org/articles/10.3389/fmars.2024.1360596/full#supplementary-material
Allendorf F. W., Leary R. F., Spruell P., Wenburg J. K. (2001). The problems with hybrids: setting conservation guidelines. Trends Ecol. Evol. 16, 613–622. doi: 10.1016/S0169-5347(01)02290-X
Botstein D., White R. L., Skolnick M. H., Davis R. W. (1980). Construction of a genetic linkage map in man using restriction fragment length polymorphisms. Am. J. Hum. Genet. 32, 314–331.
Dong Y. H., Jing Y., Ruan W. B., Yao H. H., He L., Lin Z. H. (2018). Development and evaluation of a set of 135 EST-SNP markers in the transcriptome dataset of hard clam, Meretrix meretrix. Conserv. Genet. Resour. 10, 651–658. doi: 10.1007/s12686-017-0894-y
Cruz P., Ibarra A. M., Fiore-Amaral G., Galindo C., Mendoza-Carrion G. (2005). Isolation of microsatellite loci in green abalone (Haliotis fulgens) and cross-species amplification in two other North American abalones, red (H. rufescens) and pink (H. corrugata). Mol. Ecol. Notes 05, 857–859. doi: 10.1111/j.1471-8286.2005.01088.x
Dong Y. H., Wu G. X., Yao H. H., Bian P. J., Zhao D. M., Lin Z. H. (2013a). Characterization of 34 polymorphic EST-SSR markers in Tegillarca granosa and their transferability in Anadara craticulata. J. Fish. China 37, 70–77. doi: 10.3724/SP.J.1231.2013.38307
Dong Y. H., Yao H. H., Lin Z. H., Sun C. S., You Z. J. (2013b). Development of 53 novel polymorphic EST-SSR markers for the hard clam Meretrix meretrix and cross-species amplification. Conserv. Genet. Resour. 05, 811–816. doi: 10.1007/s12686-013-9914-8
Dong Y. H., Yao H. H., Lin Z. H., Zhang L. L., Bao Z. M. (2012). Characterization of 62 polymorphic EST-SSR markers in the blood clam (Tegillarca granosa) and their cross-amplication in Scapharca subcrenata. Conserv. Genet. Resour. 04, 991–997. doi: 10.1007/s12686-012-9691-9
Dong Y. H., Yao H. H., Sun C. S., Lv D. M., Li M. Q., Lin Z. H. (2016). Development of polymorphic SSR markers in the razor clam (Sinonovacula constricta) and cross-species amplification. Genet. Mol. Res. 15, gmr7285. doi: 10.4238/gmr.15017285
Elliott L. D., Ward H. G. M., Russello M. A. (2020). Kokanee-sockeye salmon hybridization leads to intermediate morphology and resident life history: Implications for fisheries management. Can. J. Fish. Aquat. Sci. 77, 355–364. doi: 10.1139/cjfas-2019-0034
FitzSimmons N. N., Moritz C., Moore S. (1995). Conservation and dynamics of microsatellite loci over 300 million years of marine turtle evolution. Mol. Biol. Evol. 12, 432–440. doi: 10.1093/oxfordjournals.molbev.a040218
Guo X. (2009). Use and exchange of genetic resources in molluscan aquaculture. Rev. Aquac. 1, 251–259. doi: 10.1111/j.1753-5131.2009.01014.x
Huang R., Huang B. W., Li L. C., Zhang Y. F. (2011). Comparative ultrastructure of spermatozoa of Sinonovacula rivularis sp. nov. and Sinonovacula constricta. J. Fish. China 35, 58–65. doi: 10.3724/SP.J.1231.2011.17128
Huang R., Zhang Y. F. (2007). A new species of the genus Sinonovacula. J. Oceanogr. Taiwan Strait 01, 115–120. doi: 10.3969/j.issn.1000-8160.2007.01.015
Jiang Q., Yuan Y., Kong L. F. (2010). Development and characterization of 14 polymorphic microsatellite loci in the razor clam (Sinonovacula constricta). Conserv. Genet. Resour. 02, 81–83. doi: 10.1007/s12686-010-9185-6
Kalinowski S. T., Taper M. L., Marshall T. C. (2007). Revising how the computer program CERVUS accommodates genotyping error increases success in paternity assignment. Mol. Ecol. 16, 1006–1099. doi: 10.1111/j.1365-294X.2007.03089.x
Konopiński M. K., Amirowicz A. (2018). Genetic composition of a population of natural common bream Abramis brama × roach Rutilus rutilus hybrids and their morphological characteristics in comparison with parent species. J. Fish Biol. 92, 365–385. doi: 10.1111/jfb.13506
Lafarga de la Cruz F., Amar-Basulto G., Río-Portilla M. Á. D., Gallardo-Escárate C. (2010). Genetic analysis of an artificially produced hybrid abalone (Haliotis rufescens × Haliotis discus hannai) in Chile. J. Shellfish Res. 29, 717–724. doi: 10.2983/035.029.0324
Lafarga de la Cruz F., Gallardo-Escárate C. (2011). Intraspecies and interspecies hybrids in Haliotis: natural and experimental evidence and its impact on abalone aquaculture. Rev. Aquac. 3, 74–99. doi: 10.1111/j.1753-5131.2011.01045.x
Lessios H. A. (2007). Reproductive isolation between species of sea urchins. B. Mar. Sci. 81, 191–208.
Li Q. (2006). Development of microsatellite DNA markers and their applications in genetic studies in marine mollusks. J. Fish. Sci. China 13, 502–509. doi: 10.3321/j.issn:1005-8737.2006.03.027
Li Y. F., Gao X. G., Liu W. D., Tian Y., Gao L., Li S. L., et al. (2014). Microsatellite markers derived from Japanese scallop (Mizuhopecten yessoensis) expressed sequence tags. Genet. Mol. Res. 13, 1989–1992. doi: 10.4238/2014.March.24.3
Li R. H., Li Q., Yu R. H. (2008). A set of polymorphic expressed sequence tag-derived microsatellites from the bay scallop, Argopecten irradians irradians, and their transportability in three other scallop species. J. World. Aquacult. Soc 39, 138–141. doi: 10.1111/j.1749-7345.2007.00142.x
Li Q., Wang Q. Z., Qi M. J., Ge J. L., Ge J. L., Cong R. H. (2011). Development, characterization, and inheritance of 113 novel EST-SSR markers in the Pacific oyster (Crassostrea gigas). Genes Genom. 33, 313–316. doi: 10.1007/s13258-011-0021-5
Liu B., Shao Y. Q., Teng S. S., Chai X. L., Xiao G. Q. (2012b). Characterization, development and utilization of EST-derived microsatellites in Sinonovacula constricta. Oceanol. Limnol. Sin. 43, 132–137. doi: 10.11693/hyhz201201022022
Liu B., Teng S. S., Shao Y. Q., Chai X. L., Xiao G. Q. (2012a). Isolation and characterization of 39 novel polymorphic EST-SSR loci for the blood clam, Tegillarca granosa. Conserv. Genet. Resour. 04, 375–378. doi: 10.1007/s12686-011-9552-y
Luo X., Ke C. H., You W. W., Wang D. X., Chen F. (2010). Molecular identification of interspecific hybrids between Haliotis discus hannai Ino and Haliotis gigantea Gmelin using amplified fragment-length polymorphism and microsatellite markers. Aquac. Res. 41, 1827–1834. doi: 10.1111/j.1365-2109.2010.02568.x
Ma H. T., Jiang H. B., Liu X. Q., Wu X. P., Wei X. M. (2015). Polymorphic microsatellite loci for the razor clam, Sinonovacula constricta. Genet. Mol. Res. 14, 145–148. doi: 10.4238/2015.January.15.17
Qi X. Y., Dong Y. H., Yao H. H., Zhou X. L., Lin Z. H. (2013). Identification of 30 microsatellite markers in Meretrix meretrix and their transferability in Meretrix lamarckii and Meretrix lyrata. J. Fish. Sci. China 37, 1147–1154. doi: 10.3724/SP.J.1231.2013.38484
Ran Z., Li S., Zhang R., Xu J., Liao K., Yu X., et al. (2017). Proximate, amino acid and lipid compositions in Sinonovacula constricta (Lamarck) reared at different salinities. J. J. Sci. Food Agr. 97, 4476–4483. doi: 10.1002/jsfa.8311
Schlötterer C. (2000). Evolutionary dynamics of microsatellite DNA. Chromosoma 109, 365–371. doi: 10.1007/s004120000089
Tan Y., Fang L., Qiu M., Huo Z. M., Yan X. W. (2020). Population genetics of the Manila clam (Ruditapes philippinarum) in East Asia. Sci. Rep. 10, 21890. doi: 10.1038/s41598-020-78923-w
Wang Z., Du W. J., Chen S., Dong H. G., Niu D. H., Shen H. D., et al. (2016). Analysis of microsatellite polymorphism and its application in parentage determination of Sinonovacula constricta. J. Shanghai Ocean Univ. 25, 807–813. doi: 10.12024/jsou.20160301694
Wang Y. P., Guo X. M. (2007). Development and characterization of EST-SSR markers in the Eastern oyster Crassostrea virginica. Mar. Biotechnol. 9, 500–511. doi: 10.1007/s10126-007-9011-7
Weng Z. H., Xie Y. J., Xiao Z. Q., Ren P., Wang Z. Y., Gui J. F. (2013). Molecular identification of the taxonomic status of Sinonnvacula rivularis and genus Sinonovacula using mitochondrial COI and 16S rRNA fragments. Acta Hydrobiol. Sin. 37, 684–690. doi: 10.7541/2013.80
You W. W., Guo Q., Fan F. L., Ren P., Luo X., Ke C. H. (2015). Experimental hybridization and genetic identification of Pacific abalone Haliotis discus hannai and green abalone H. fulgen. Aquaculture 448, 243–249. doi: 10.1016/j.aquaculture.2015.05.043
Zhan A. B., Bao Z. M., Hu X. L., Wang S., Peng W., Wang M. L., et al. (2008). Characterization of 95 novel microsatellite markers for Zhikong scallop Chlamys farreri using FIASCO-colony hybridization and EST database mining. Fishries Sci. 74, 516–526. doi: 10.1111/j.1444-2906.2008.01554.x
Zhang W. J., Li R. H., Chen X. Q., Wang C. L., Gu Z. Q., Mu C. K., et al. (2020). Molecular identification reveals hybrids of Mytilus coruscus × Mytilus galloprovincialis in mussel hatcheries of China. Aquacult. Int. 28, 85–93. doi: 10.1007/s10499-019-00445-8
Keywords: Sinonovacula constricta, Sinonovacula rivularis, microsatellite marker, cross-species amplification, hybrids, genetic identification
Citation: Wang S, Shi Y, Dong Y, Meng Y, Yao H and He L (2024) Molecular identification of Sinonovacula constricta, Sinonovacula rivularis and their interspecific hybrids using microsatellite markers. Front. Mar. Sci. 11:1360596. doi: 10.3389/fmars.2024.1360596
Received: 23 December 2023; Accepted: 12 March 2024;
Published: 25 March 2024.
Edited by:
Kwang-Sik Albert Choi, Jeju National University, Republic of KoreaReviewed by:
Youji Wang, Shanghai Ocean University, ChinaCopyright © 2024 Wang, Shi, Dong, Meng, Yao and He. This is an open-access article distributed under the terms of the Creative Commons Attribution License (CC BY). The use, distribution or reproduction in other forums is permitted, provided the original author(s) and the copyright owner(s) are credited and that the original publication in this journal is cited, in accordance with accepted academic practice. No use, distribution or reproduction is permitted which does not comply with these terms.
*Correspondence: Hanhan Yao, eWFvaGFuaGFuMTAyMEAxMjYuY29t; Lin He, aGVsaW5Aend1LmVkdS5jbg==
†These authors have contributed equally to this work
Disclaimer: All claims expressed in this article are solely those of the authors and do not necessarily represent those of their affiliated organizations, or those of the publisher, the editors and the reviewers. Any product that may be evaluated in this article or claim that may be made by its manufacturer is not guaranteed or endorsed by the publisher.
Research integrity at Frontiers
Learn more about the work of our research integrity team to safeguard the quality of each article we publish.