- 1Research Unit Food and Lipids, KU Leuven Kulak, Kortrijk, Belgium
- 2Leuven Food Science and Nutrition Research Centre (LFoRCe), KU Leuven, Leuven, Belgium
- 3Analytical and Circular Chemistry (ACC), Institute for Materials Research (IMO), Hasselt University, Hasselt, Belgium
Microalgae have rigid, complex cell walls hindering direct lipid extraction. Cell disruption techniques are used to rupture these cellular structures to increase lipid extraction. Researchers investigating the downstream processing of microalgae do not always have access to microalgal cultivation systems to generate large amounts of fresh microalgal biomass. Using resuspended freeze-dried microalgal biomass as a model laboratory system for concentrated fresh biomass during cell disruption experiments offers greater flexibility in experimental planning and omits investment costs of microalgal cultivation equipment. So far, it however remains unclear whether freeze-dried resuspended biomass can be used as a model laboratory system to represent concentrated fresh biomass during cell disruption and lipid extraction experiments. This paper thus evaluated the suitability of resuspended freeze-dried Nannochloropsis as a model laboratory system for concentrated fresh Nannochloropsis during cell disruption. Ultrasound assisted cell disruption was used as example cell disruption technique and lipid extraction efficiency and free fatty acid content were investigated. Tap water and 3% sodium chloride are both suitable resuspension media for the resuspension of freeze-dried Nannochloropsis. Resuspension duration should be limited (< 120 min) to prevent the formation of free fatty acids. The condition of the biomass (concentrated fresh, or resuspended freeze-dried) prior to ultrasound assisted cell disruption did not influence the resulting lipid extraction efficiency. Resuspended freeze-dried Nannochloropsis biomass in tap water or 3% sodium chloride can thus be used as a model laboratory system for fresh microalgal biomass during research on ultrasound assisted lipid extraction. The generalization of the results to other cultivation conditions, cell disruption techniques, components of interest or microalgal species should be carefully assessed.
1 Introduction
Microalgae are unicellular organisms able to produce commercially interesting components such as proteins, lipids, carbohydrates, pigments, and vitamins. These microalgae can be used to produce feed, food, and pharmaceuticals either as whole cell biomass or as extracts of these major or minor constituents. One microalgae genus that is extensively studied regarding lipid production is the marine microalga Nannochloropsis (Barbosa et al., 2023). Nannochloropsis can accumulate lipids (up to 60%DM) with a high content of the valuable omega-3 long-chain polyunsaturated fatty acid (n-3 LC-PUFA) eicosapentaenoic acid (Chua and Schenk, 2017; Xu, 2022). This n-3 LC-PUFA exhibits health promoting effects as it plays a role in the prevention of cardiovascular diseases (Calder, 2021).
Microalgae, and especially Nannochloropsis, have rigid, complex cell walls hindering direct lipid extraction by organic solvents (Lee et al., 2012; Alhattab et al., 2019; de Carvalho et al., 2020). To increase lipid extraction efficiency, cell disruption techniques are used to change and/or rupture cellular structures (Halim et al., 2012). The lipids can then either be directly released from the interior of the cells into the bulk extraction solvent or the modified and ruptured cellular structures pose less of a barrier to organic solvent penetration into the cell and diffusion out of the cells (Halim et al., 2012). Altogether, these mechanisms assist in lipid extraction and can result in increased lipid extraction efficiencies. Throughout this paper, the term ‘cell disruption’ is used to describe all these disruption-related phenomena.
Microalgal cell disruption and lipid extraction is subject of extensive laboratory-scale research. Multiple cell disruption techniques such as microwave (Rezaei Motlagh et al., 2021), ultrasound (UAE) (Yao et al., 2018) and enzymatic (Maffei et al., 2018; Mienis et al., 2023) treatment and high pressure homogenization (Balduyck et al., 2018; Bernaerts et al., 2019) have been studied. These cell disruption techniques require the microalgae to be in suspension (Halim et al., 2012). Resuspended freeze-dried (also referred to as lyophilized) microalgal biomass is often utilized in these laboratory-scale set-ups as a model laboratory system to represent concentrated fresh microalgal biomass during cell disruption and lipid extraction experiments for several reasons. First of all, laboratory-scale cell disruption set-ups involve the optimization of multiple process parameters [e.g., time, temperature, solid to liquid ratio, power input, enzyme concentration (Zuorro et al., 2016; Yao et al., 2018; Zhao et al., 2022)], requiring the use of several to hundreds of grams of microalgae over multiple days. Concentrated fresh microalgal biomass (suspensions of 5-10%DM) however has limited stability and shelf life due to the formation of free fatty acids (FFA) (Balduyck et al., 2017) and fermentation products (Verspreet et al., 2020). Furthermore, the cultivation and harvesting of fresh microalgae requires specific facilities that not all research laboratories have access to, and if microalgae cultivation facilities are present, the total microalgal biomass yield [generally between 0.5 and 5 g/L (de Carvalho et al., 2020)] and total volume of the dilute culture is low. The demand for the required amount of concentrated fresh microalgae can therefore often not be met. Compared to concentrated fresh biomass, the use of resuspended freeze-dried microalgae thus requires less investment costs for specific equipment and it allows for flexible planning of large cell disruption optimization experiments executed at different moments in time while using the same batch of biomass. In the present study, the term ‘resuspension’ is used to describe the act of suspending (freeze-)dried microalgal biomass into an aqueous medium. Some authors refer to this as ‘suspension’ or ‘hydration’. As microalgae in their native state are already suspended in aqueous medium, using the term ‘resuspension’ is considered more specific.
Within cell disruption experiments aimed at extracting lipids, the intention is to study the effect of the applied disruption technique on the lipid extraction efficiency. Therefore, it is of importance that the increase in lipid extraction efficiency can be solely attributed to the applied cell disruption and is not caused by any preceding processing steps, sometimes also referred to as pretreatment, such as freeze-drying and subsequent resuspension of biomass. However, in papers using resuspended freeze-dried biomass as a model laboratory system, it is (inexplicitly) assumed that the condition of the biomass (resuspended freeze-dried or concentrated fresh) prior to cell disruption has no influence on the resulting lipid extraction efficiency. For further advancements in the field of microalgal cell disruption and lipid extraction it would be very useful to verify whether resuspended freeze-dried biomass can actually be used to represent concentrated fresh biomass during cell disruption and lipid extraction experiments. To the best of the authors’ knowledge, this assumption has however not been verified. Furthermore, different resuspension media such as demineralized water (Martínez-Sanz et al., 2020; Quesada-Salas et al., 2021), tap water, but also growth medium or saline media to mimic saline environment have been used. However, to the best of our knowledge, assessing the effect of resuspension medium and duration on lipid extraction efficiency has also not been studied.
In addition to lipid extraction efficiency, FFA content is another relevant lipid parameter to study during resuspension and cell disruption. FFA content of concentrated fresh Nannochloropsis increased during storage and during cell disruption by high pressure homogenization which was attributed to endogenous lipolytic enzyme activity (Balduyck et al., 2017, 2018). High FFA content is associated with increased rancidity (Mannion et al., 2016) and can thus be considered a lipid quality indicator. FFA content has however not been extensively studied in previous research regarding resuspension or cell disruption of microalgal biomass.
The overall objective of this study was to assess the suitability of resuspended freeze-dried Nannochloropsis biomass as a model laboratory system for concentrated fresh Nannochloropsis biomass in cell disruption and lipid extraction experiments. The study has been divided into two parts. The first assessed the effect of resuspension medium (tap water or 3% NaCl) and resuspension duration of freeze-dried Nannochloropsis on the lipid extraction efficiency. In the second experiment, UAE was chosen as example cell disruption technique to compare the effectiveness of cell disruption on concentrated fresh and resuspended freeze-dried biomass. In both experiments the FFA content was analyzed to assess the effect on lipid quality.
2 Materials and methods
2.1 Materials
Commercial freeze-dried Nannochloropsis sp. (COM-FD-Nsp) (NannoPrime) biomass was obtained from Proviron (Hemiksem, Belgium) and was stored at -80°C until further use. Sodium chloride (≥ 99.5%) and isopropanol (≥ 99.5%, GC/HPLC-grade) were obtained from Sigma-Aldrich (Buch, Switzerland), sodium sulphate (> 99%) and chloroform (≥ 99.9%, HPLC-grade) were obtained from Carl Roth (Karlsruhe, Germany). Ammonium acetate (≥ 98.0, HPLC-grade) was obtained from Merck (Darmstadt, Germany). Methanol (≥ 99.9%, HPLC-grade) and toluene (≥ 99.8%, HPLC-grade) were obtained from Chemlab (Zedelgem, Belgium) and hexane (95%, HPLC-grade) was obtained from Biosolve (Valkenswaard, The Netherlands), Tridecanoic acid (C13:0) was obtained from Sigma-Aldrich (Buch, Switzerland). Myristic acid (C14:0), palmitic acid (C16:0), palmitoleic acid (C16:1), stearic acid (C18:0), oleic acid (C18:1), linoleic acid (C18:2), linolenic acid (C18:3), arachidonic acid (C20:4) and eicosapentaenoic acid (C20:5) were obtained from Nu-Chek Prep (Elysian, USA). All FFA standards had a purity of > 99%.
2.2 Methods
This paper investigated the use of resuspended freeze-dried Nannochloropsis biomass as a model laboratory system to represent concentrated fresh biomass during cell disruption and lipid extraction experiments. Experiment 1 assessed the effect of resuspension medium (tap water or 3% NaCl) and resuspension duration of freeze-dried Nannochloropsis on the lipid extraction efficiency. During experiment 2, UAE was chosen as example cell disruption technique to compare the effectiveness of cell disruption on concentrated fresh and resuspended freeze-dried biomass. The FFA content was analyzed in both experiments to assess the effect of respectively resuspension or UAE on the lipid quality.
Both experimental set-ups (Figure 1) are first discussed separately while the details of the subsequent analyses of total and extractable lipids, lipid extraction efficiency, FFA, ash content, and the statistics are discussed thereafter. An overview of the types of biomasses used in the two experiments and corresponding abbreviations are provided in Table 1.
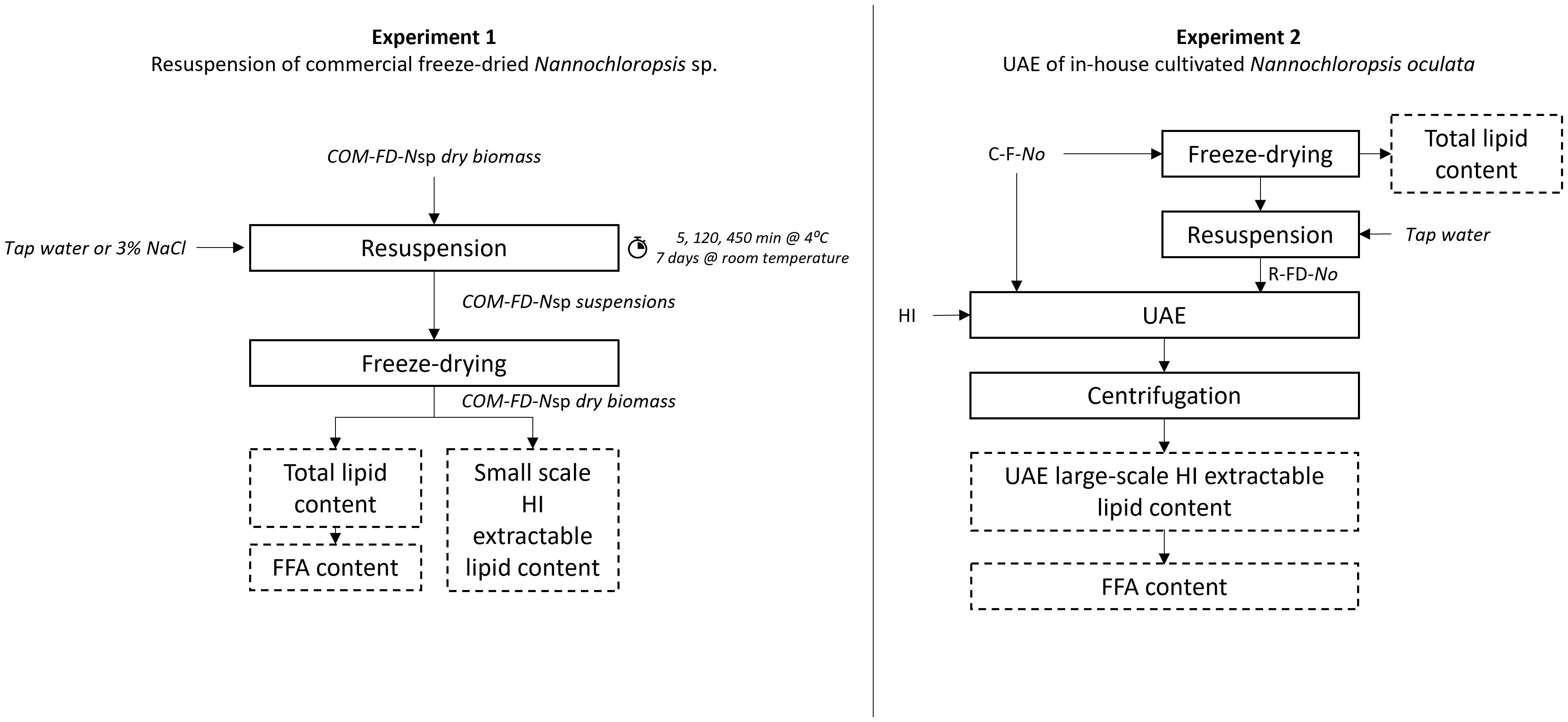
Figure 1 Schematic overview of the experimental set-up of experiment 1 and experiment 2 including the lipid analysis with COM-FD-Nsp, Commercial freeze-dried Nannochloropsis sp.; FFA, free fatty acids; HI, hexane/isopropanol; C-F-No, concentrated; fresh Nannochloropsis oculata; R-FD-No, resuspended freeze-dried Nannochloropsis oculata and UAE, ultrasound assisted lipid extraction.
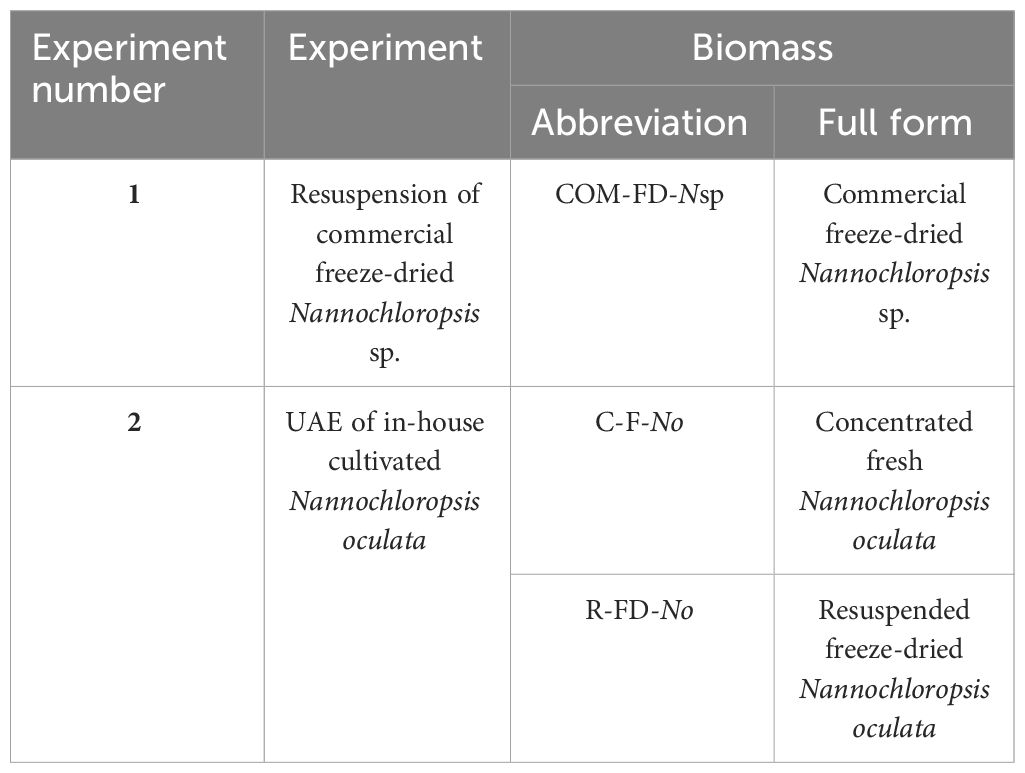
Table 1 Nannochloropsis biomasses and corresponding abbreviations used in the two experimental set-ups.
2.3 Resuspension of commercial freeze-dried Nannochloropsis sp.
In this first experimental set-up, COM-FD-Nsp was resuspended, in duplicate, in sterile tap water or sterile 3% w/v sodium chloride (NaCl) solution to a concentration of 11.1% w/v, to mimic a concentrated microalgal suspension, by stirring for 5 min. No demineralized water was used as Nannochloropsis is a marine microalga for which resuspension in demineralized water could cause hypotonic osmotic shock resulting in a small degree of cell disruption (Halim et al., 2021). Instead, 3% NaCl was used to mimic marine cultivation medium. The Nannochloropsis sp. suspensions were stored in closed Falcon tubes in the dark at 4°C for 5, 120 and 450 min. During storage, the suspensions were agitated using a rotary shaking plate at 100 rpm to prevent sedimentation of the microalgal cells. After 450 min, storage continued at room temperature for 7 days as a stress test to study the potential influence of endogenous lipases. After each time interval, the microalgal suspensions were frozen at -80°C, subsequently freeze-dried and stored at -80°C until further analyses.
2.4 UAE of in-house cultivated Nannochloropsis oculata
2.4.1 In-house cultivation and harvesting of Nannochloropsis oculata
Nannochloropsis oculata 38.85 (Sammlung von Algenkulturen, Göttingen, Germany) stock culture was kept in modified Wright’s Cryptophyte (WC) medium (Guillard & Lorenzen, 1972) with double the amount of NaNO3 (170 mg/L) and to which artificial sea salt (30 g/L) (Homarsel, Zoutman Industries, Roeselare, Belgium) was added. The stock culture was introduced into five 30 L bubble column photobioreactors (cylindrical, 20 cm diameter) containing WC medium with adjusted NaNO3 concentration of 110 mg/L and artificial sea salt (30 g/L). A pH-stat system set at pH 8.5 was used to control the pH by addition of filtered CO2 (0.2 μm) to acidify the medium. Each bioreactor was continuously aerated with filtered air (0.2 μm) for biomass mixing and irradiated by two daylight fluorescent tubes (Sylvania GRO-LUX T8 F36W/Gro) in a room with temperature set at 23.5°C. Microalgal growth in each bioreactor was monitored daily by measuring the optical density at 750 nm and showed slight variations indicating biological variation between the cultures within the different bioreactors. The microalgae were harvested and pooled after ten days of cultivation using a disc stack centrifuge (4000 g) with further concentration by centrifugation at 7500 g for 10 min (Sigma 6-16KS, Sigma Laborzentrifugen, Osterode am Harz, Germany).The resulting microalgal paste was diluted with supernatant to obtain a concentrated microalgal suspension (10.89 ± 0.01%DM). Half of the suspension volume was stored overnight at 4°C (for practical reasons) and then directly subjected to UAE (Section 2.4.2), and this is referred to as concentrated fresh N. oculata (C-F-No) biomass. The remaining biomass was frozen at -80°C, freeze-dried, grinded into a fine powder and stored at -80°C.
The in-house cultivated, freeze-dried N. oculata was resuspended in tap water by stirring for 10 min at 550 rpm to obtain a suspension with dry matter content of 11.05 ± 0.03%, similar to the dry matter content of C-F-No. Tap water was chosen as resuspension medium based on the outcome of the results of the resuspension set-up (Section 3.1) This microalgal suspension is referred to as resuspended freeze-dried N.oculata (R-FD-No) biomass.
2.4.2 Ultrasound disruption
C-F-No and R-FD-No biomass suspensions were subjected, in duplicate, to five min of UAE in the presence of hexane/isopropanol (3:2 v/v) (HI (3:2)) for simultaneous cell disruption and lipid extraction. A HI (3:2) solvent system was chosen as this solvent is accepted in the food supply chain (EU Directive 2009/32/EC) (EU, 2009), is less toxic compared to other organic solvents, and is able to extract non-polar as well as polar lipids (Ryckebosch et al., 2014). The dry biomass to organic solvent ratio was 1:13.7. A Branson 450 Sonifier with operating frequency of 20 kHz was used. The output control, which determines the amplitude, was set at 6, which corresponded to an initial power of 0.45 W/mL. 50% pulse mode, corresponding to an ultrasound duration of 0.5 second per second, was used. The sonicator tip (13 mm diameter tapped, stepped horn tip) was submerged in the center of the mixture with the probe tip at 2 cm below the liquid surface. After UAE, the biomass-solvent mixture was centrifuged at 3005 g for 10 min and the upper lipid-rich organic solvent layer was filtered (Whatmann n°1) through a sodium sulphate layer, collected in a round-bottom flask and evaporated by rotary evaporation. The lipid yield was gravimetrically determined and is referred to as UAE large-scale HI (3:2) extractable lipid content. Lipid extracts were redissolved in HI (3:2 v/v) and stored in amber vials at -80°C until further analyses.
2.5 Analyses
For the total lipid content, small-scale hexane/isopropanol (3:2) extractable lipid content and the ash content determination all samples are required to be dry. The suspensions obtained in the resuspension experiment (Section 2.3) were thus freeze-dried before all three analyses. Likewise, the suspensions of C-F-No and R-FD-No obtained in the UAE experiment (Section 2.4.1) were also freeze-dried prior to ash content determination and total lipid content determination (only for C-F-No).
2.5.1 Total lipid content of Nannochloropsis biomass
The total lipid content of all samples was determined using chloroform/methanol (1:1 v/v) according to EN 17908:2023 CEN (2023) and Ryckebosch et al. (2012). Briefly, 4 mL methanol, 2 mL chloroform and 0.4 mL demineralized water were added to 100 mg freeze-dried biomass. The sample was vortexed followed by addition of 2 mL of chloroform and 2 mL of demineralized water. The samples were then vortexed again and centrifuged at 750 g for 10 min. The upper layer was discarded, and the bottom organic solvent layer was collected. The pellet was re-extracted with 4 mL chloroform/methanol (1:1 v/v), vortexed and centrifuged. The organic solvent was pooled with the previously collected solvent. These steps were repeated on the remaining pellet and the organic solvent was pooled with the previously collected organic solvent. The organic solvent was filtered (Whatmann n°1) through a sodium sulfate layer, the filter was rinsed with chloroform/methanol (1:1 v/v), and the filtered solvent was removed by rotary evaporation. The mass of the extracted lipids after solvent evaporation was gravimetrically determined. The total lipid content of all samples was determined minimally in duplicate.
2.5.2 Small scale hexane/isopropanol (3:2) extractable lipid content
The small scale HI (3:2) extractable lipid content of samples obtained during the resuspension experiment was determined using the method described by Ryckebosch et al. (2013) with modifications. In brief, 6 mL of HI (3:2) was added to 100 mg of freeze-dried biomass and homogenized. Subsequently, the samples were centrifuged for 10 min at 750 g, and the organic solvent layer collected. The remaining pellet was re-extracted three more times using the same procedure. Solvent layers were pooled, filtered (Whatmann n°1) and evaporated to obtain the extracted lipids. The small-scale HI (3:2) extractable lipid content was determined minimally in duplicate.
2.5.3 Dry matter and ash content
The dry matter content of C-F-No and R-FD-No was determined at least in triplicate by comparing the weight pre- and post- freeze-drying. The ash content of samples was determined at least in duplicate according to the NREL protocol ‘Determination of Total Solids and Ash in Algal Biomass’ (Van Wychen & Laurens, 2015), with slight modifications. In brief, crucibles were pre-conditioned at 575 ± 25°C. 100 mg freeze-dried biomass was prepared in pre-weighted crucibles. Samples were dried in an oven at 60 ± 10°C for at least 18 hours until constant weight. The dried samples were ashed in a muffle furnace at 575 ± 25°C for 24 ± 6 hours. The weight of the ashed sample was recorded until constant weight. The ash content was used to determine the lipid extraction efficiency on ash-free dry weight in the resuspension experiment by expressing both the small-scale HI (3:2) extractable lipid content and total lipid content on ash-free dry weight (Section 2.5) to correct for the contribution of added NaCl.
2.5.4 Free fatty acid content
The FFA content of the total lipid extracts obtained in the resuspension experiment and of UAE large-scale HI (3:2) lipid extracts obtained during the UAE experiments were determined according to a method based on Van Meulebroek et al. (2017) with modifications and described previously by Van Wayenbergh et al. (2023) for one FFA (C13:0)). For each experiment, a separate calibration curve was constructed for C14:0, C16:0, C16:1, C18:0, C18:1, C18:2, C18:3, C20:4 and C20:5 in toluene/methanol (1:2) (Table 2). Each lipid sample was standardized to a final lipid concentration of 500 ng/µL in toluene/methanol (1:2). Tridecanoic acid was used as internal standard in the samples and in each level of the calibration curve.
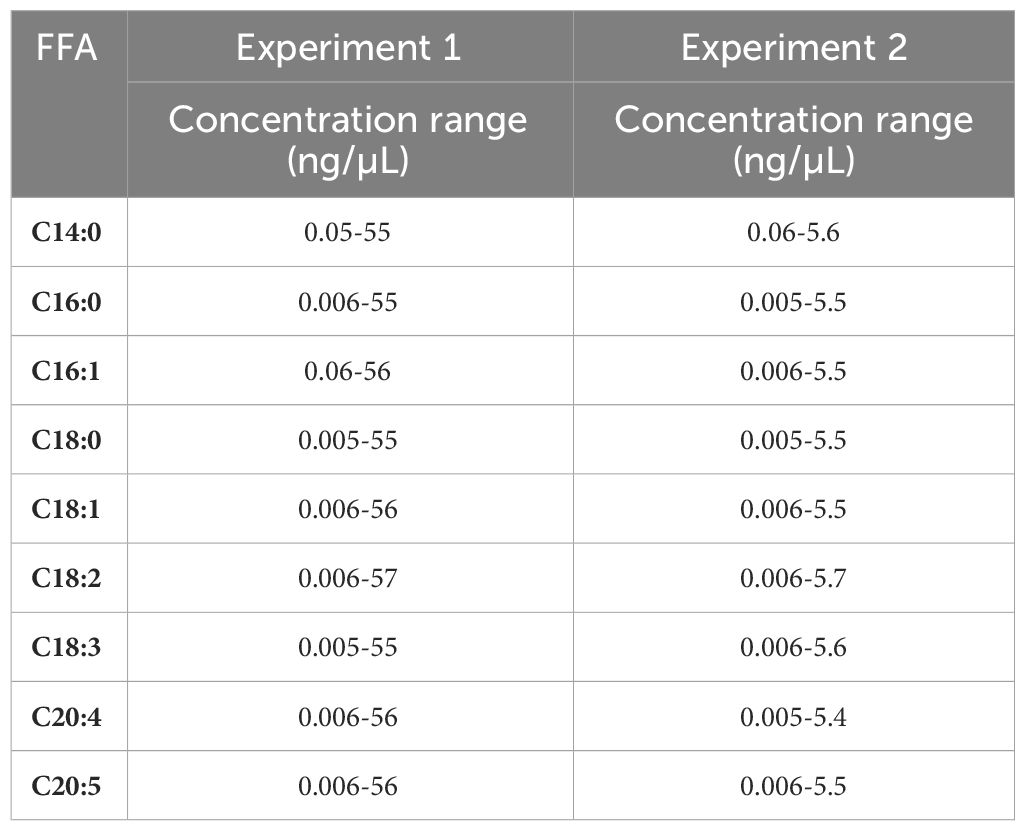
Table 2 FFA concentration range (ng/µL) of each FFA used for the construction of the calibration curves in experiment 1 and 2.
LC-MS was used for the quantitative analysis of the nine FFAs. FFA separation was achieved by UHPLC on an Vanquish Flex UHPLC system operated at 40°C with an Accucore 80A-C18 reverse phase column with a universal guard column (Uniguard, Thermo Fischer, holder for 2.1/3.0MM). A binary pump system was used for mixing two mobile phases: 20 mM ammonium acetate in MilliQ water (mobile phase A) and 20 mM ammonium acetate in methanol (mobile phase B). Gradient elution was applied (expressed in % of mobile phase B) for a total run time of 25 min with mobile phase flow of 0.3 mL/min:
- 0-1 min: isocratic 75%
- 1-2 min: linear 75-90%
- 2-6 min: linear 90-98%
- 6-15 min: linear 98-100%
- 15-22 min: isocratic 100%
- 22-25 min: linear 75%
5 µL of sample was injected for analysis. Q Exactive MS with electrospray ionization (ESI) and orbitrap detector was used to detect the FFA. The MS was run in negative ion mode for detection of FFA as ionization occurs due to proton loss. The scan range was 153.4 – 2300 m/z with sheath, auxiliary and sweep gas flow rate of 60, 20 and 2 arbitrary units, respectively. Capillary temperature was 285°C and the auxiliary gas heater temperature was 370°C. The mass resolution was 70 000 full-width half maximum (fwhm, 1 Hz). The ESI spray voltage was 3 kV, and the S-Lens radio frequency level was 70.
Data analysis was performed using TraceFinder 5.1 (Thermo Fisher Scientific). Identification of the FFAs of interest was based on the m/z value of the molecular ion with maximum m/z variation of 3 ppm. The peak areas of FFA in the calibration curves were integrated and corrected for IS. Linear calibration curves were created for each FFA based on the calibration levels. Peak areas of the FFA in the samples were corrected for IS and quantitative determination of the FFAs was achieved using the calibration curves. Total FFA content within the samples was determined by summation of the content of the individual detected FFA (C14:0, C16:0, C16:1, C18:1, C18:2, C20:4 and C20:5).
2.6 Lipid extraction efficiency
In the resuspension experiment (Section 2.3) the lipid extraction efficiency was determined according to Equation 1. The small-scale HI (3:2) extractable lipid content (Section 2.5.2) was divided by the total lipid content as determined according to Section 2.5.1. Both were expressed on ash free dry weight.
The lipid extraction efficiency in the UAE experiment was determined by dividing the UAE large-scale HI (3:2) extractable lipid content (Section 2.4.2) by the total lipid content as determined according to Section 2.5.1 (Equation 2). The lipid extraction efficiency of the initial, undisrupted in-house cultivated N. oculata prior to UAE was determined by Equation 1.
2.7 Statistics
Statistical analysis was performed using JMP Pro 16.2.0 (SAS Institute Inc, Cary, USA) with a significance level of 0.05. For the resuspension experiment, a two-way analysis of variance (ANOVA) was conducted to assess the impact of resuspension duration (5, 120 and 450 minutes) and resuspension medium (tap water or 3% NaCl) on the lipid extraction efficiency and the FFA content. The presence of interaction effects was tested by using a multiplicative model. When no significant interaction effects were present, an additive model was applied. In case of a significant effect, a post-hoc Tukey-HSD test was performed. A one-way ANOVA was conducted to study the effect of resuspension treatment (no resuspension, in tap water or 3% NaCl) on the lipid extraction efficiency and FFA content. A two-way ANOVA was performed to study the effect of resuspension treatment (450 minutes at 4°C or 7 days at room temperature) and resuspension medium (tap water or 3% NaCl) on the FFA content.
For UAE, t-tests were conducted to assess the effect of the condition of the biomass (concentrated fresh or resuspended freeze-dried) on the UAE large-scale HI (3:2) extractable lipid content and FFA content in the HI-extractable lipids.
3 Results
This paper investigated the use of resuspended freeze-dried Nannochloropsis biomass as a model laboratory system to represent concentrated fresh biomass during cell disruption and lipid extraction experiments. Experiment 1 assessed the effect of resuspension medium (tap water or 3% NaCl) and resuspension duration of freeze-dried Nannochloropsis on the lipid extraction efficiency. During experiment 2, UAE was chosen as example cell disruption technique to compare the effectiveness of cell disruption on concentrated fresh and resuspended freeze-dried biomass. The FFA content was analyzed in both experiments to assess the effect of respectively resuspension or UAE on the lipid quality.
3.1 Experiment 1: resuspension of commercial freeze-dried Nannochloropsis sp.
3.1.1 Lipid extraction efficiency
The lipid extraction efficiency (according to Equation 1) of initial, not-resuspended COM-FD-Nsp and COM-FD-Nsp after resuspension (5, 120 and 450 min) in tap water or 3% NaCl was determined (Figure 2). The total lipid content of the initial, not resuspended COM-FD-Nsp was 30.5 ± 0.2% on ash free dry weight. The total lipid content of the resuspended biomass was similar. The two-way ANOVA indicated that there was no significant impact of resuspension medium nor resuspension duration on the lipid extraction efficiency. The lipid extraction efficiency of initial not-resuspended COM-FD-Nsp was 32.4 ± 0.7% on ash free dry weight and was compared to five minutes of resuspension in tap water or 3% NaCl (respectively 38.5 ± 2.3 and 40.3 ± 1.4% on ash free dry weight) by a one-way ANOVA. No significant effect of the resuspension treatment (not resuspended, in tap water or 3% NaCl) was found on the lipid extraction efficiency. In summary, no effects for resuspension itself nor for resuspension medium (tap water or 3% NaCl) or resuspension duration on lipid extraction efficiency were observed.
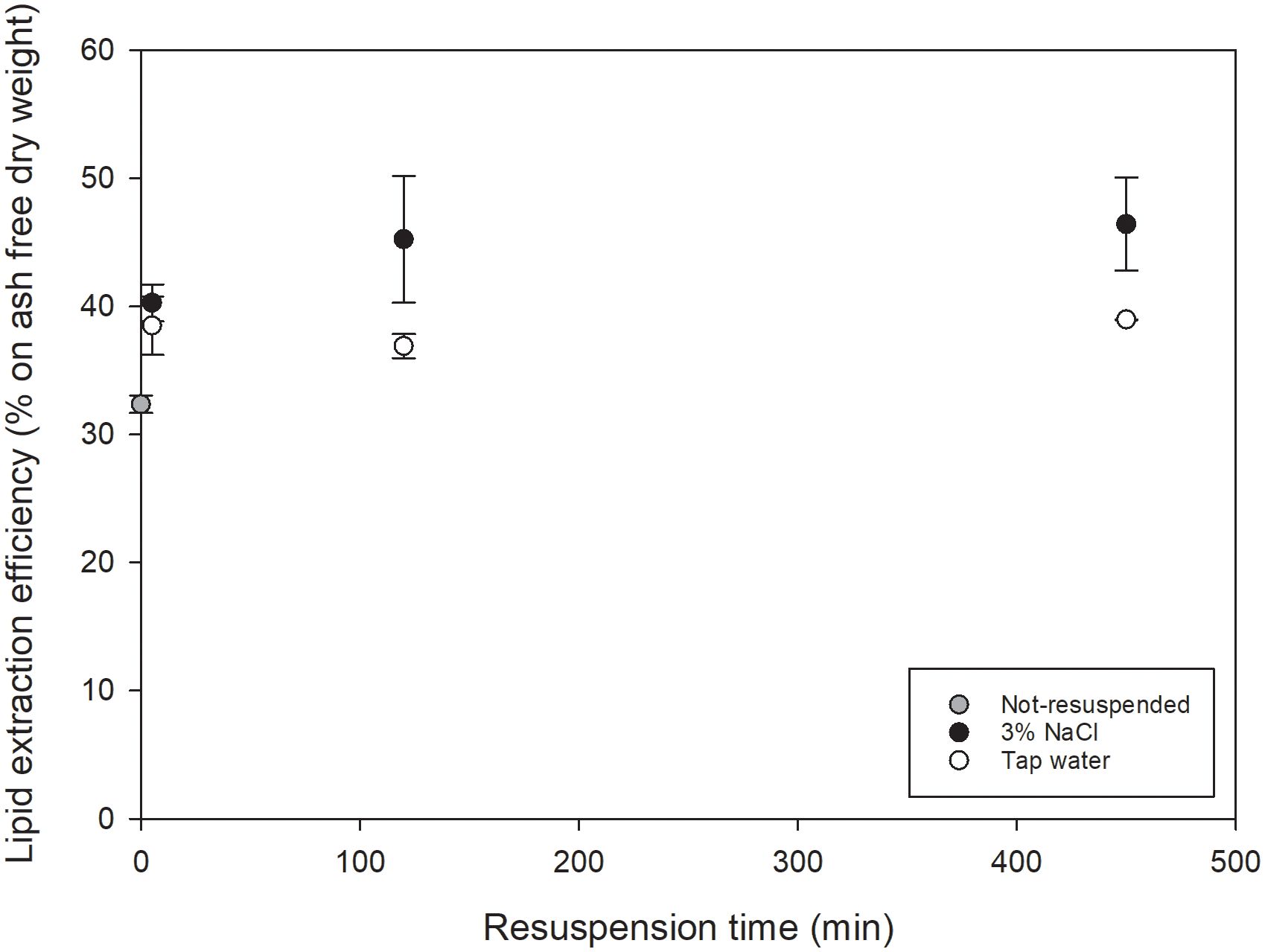
Figure 2 Lipid extraction efficiency (% on ash free dry weight) as function of resuspension time (min) for COM-FD-Nsp that was not resuspended (gray symbol), resuspended in 3% NaCl (black symbols) or resuspended in tap water (white symbols) reported as mean +/- st.dev with n=2*2 for resuspended biomass and n=4 for not-resuspended biomass. No significant differences (α=0.05) were observed for the effect of resuspension, nor of resuspension duration nor resuspension medium.
3.1.2 FFA content
The FFA content of initial, not-resuspended COM-FD-Nsp and COM-FD-Nsp after resuspension (5, 120, 450 min, and 7 days) in tap water or 3% NaCl was quantified by LC-MS (Figure 3). Based on the two-way ANOVA, no significant interaction effect between resuspension medium and resuspension duration was found. The resuspension duration had a significant effect on the FFA content (p<0.0001), where 120 and 450 minutes of resuspension each led to a significant increase in FFA content compared to the preceding time point. No significant influence of resuspension medium was found. The FFA content of initial not-resuspended COM-FD-Nsp was 7.3 ± 0.2% of total lipids. Compared to not-resuspended COM-FD-Nsp, resuspension for 5 minutes did not significantly increase the FFA content, independent of resuspension medium (Figure 3).
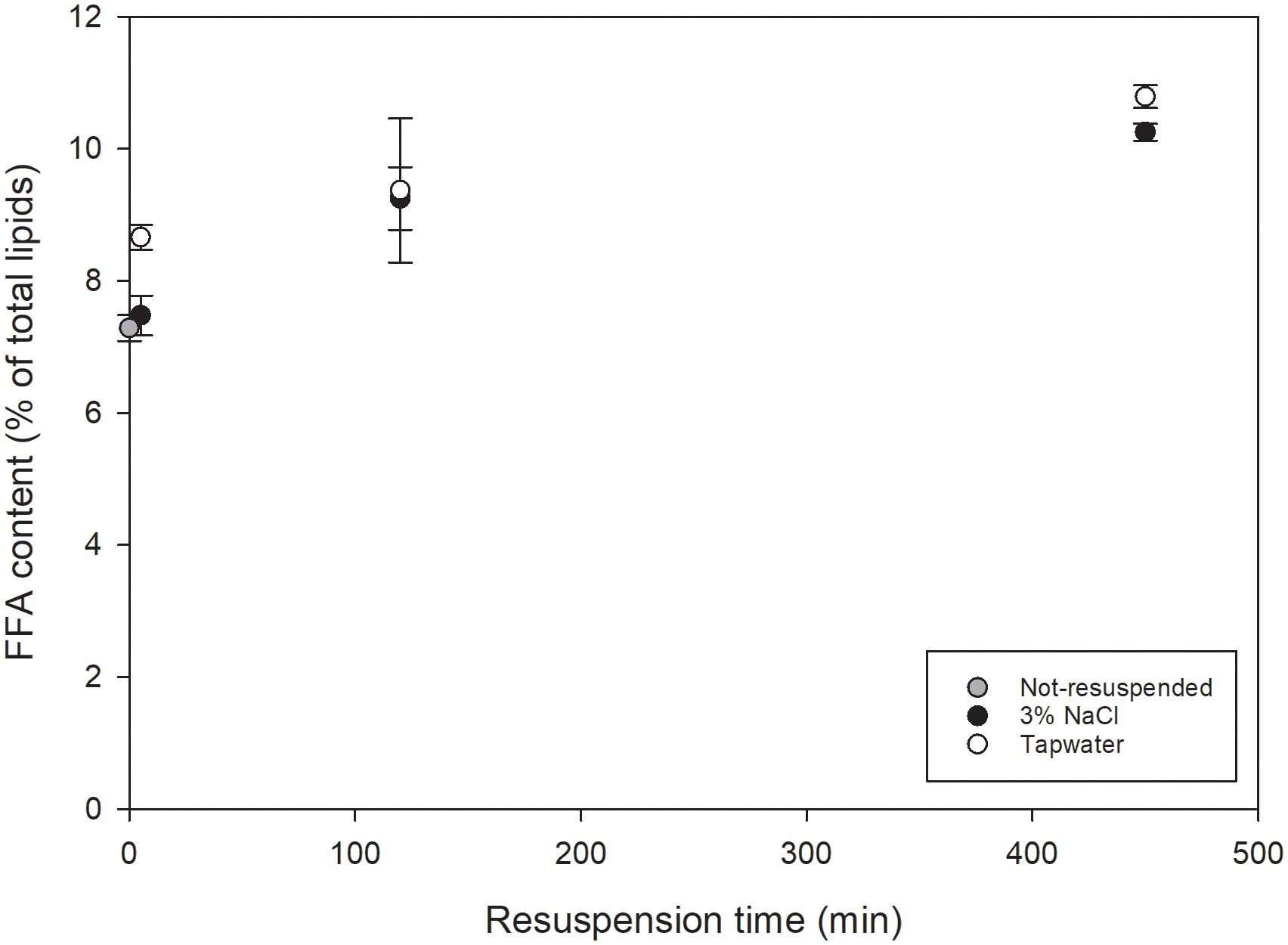
Figure 3 FFA content expressed as % of total lipids as function of resuspension time (min) for COM-FD-Nsp that was not resuspended (gray symbols), resuspended in 3% NaCl (black symbols) or resuspended in tap water (white symbols) reported as mean +/- st.dev with n=2*2 for resuspended biomass and n=4 for not-resuspended biomass. A significant effect of resuspension duration was observed. Neither resuspension medium nor the resuspension itself had a significant effect.
The activity of endogenous lipases in COM-FD-Nsp was assessed by a stress test. After 450 minutes of resuspension at 4°C, storage of the resuspended samples was continued at room temperature in the dark for 7 days. This change in storage temperature was deliberately chosen to create circumstances that have shown to extensively activate endogenous lipases in concentrated fresh N. oculata resulting in an increased FFA content (Balduyck et al., 2017). Unlike Balduyck et al. (2017), this paper utilized resuspended freeze-dried Nannochloropsis sp. to verify its suitability as a model laboratory system for concentrated fresh biomass, but it was uncertain whether the endogenous lipases in COM-FD-Nsp retained their lipolytic activity after undergoing freeze-drying. The FFA content of COM-FD-Nsp resuspended in tap water or 3% NaCl after seven days of storage at 20°C was 32.0 ± 0.3 and 28.9 ± 2.9% of total lipids, respectively. Based on a two-way ANOVA, the prolonged storage of 7 days at room temperature significantly increased (p = 0.001) the FFA content compared to resuspension for 450 min at 4°C confirming the presence of active endogenous lipases in COM-FD-Nsp. Exogenous lipase activity was excluded as autoclaved resuspension media were used. No effect of resuspension medium was found.
3.2 Experiment 2: UAE of in-house cultivated Nannochloropsis oculata
Based on the results in Section 3.1, freeze-dried in-house cultivated N. oculata was resuspended in tap water for 10 min. The total lipid content of this in-house cultivated batch of N. oculata was 25.6 ± 0.7%. The lipid extraction efficiency (Equation 2) of C-F-No and R-FD-No suspensions after UAE was not statistically different (Table 3). Unlike in Section 3.1.1, lipid extraction efficiency was not expressed on ash free dry weight, as the ash content of C-F-No and R-FD-No suspensions was similar (16.8 ± 0.1% and 16.6 ± 0.2%, respectively) and correction for different ash content of the biomass was thus not required. The FFA content in the HI (3:2) lipid extract obtained after UAE was (marginally) significantly higher (p=0.044) for R-FD-No compared to C-F-No (Table 3). These results indicated that the condition of the N. oculata biomass, either as concentrated fresh or resuspended freeze-dried biomass before UAE has no influence on the obtained lipid extraction efficiency. However, there was a (marginally) significant effect of the condition of the biomass on the FFA content in the obtained HI (3:2) lipid extract.
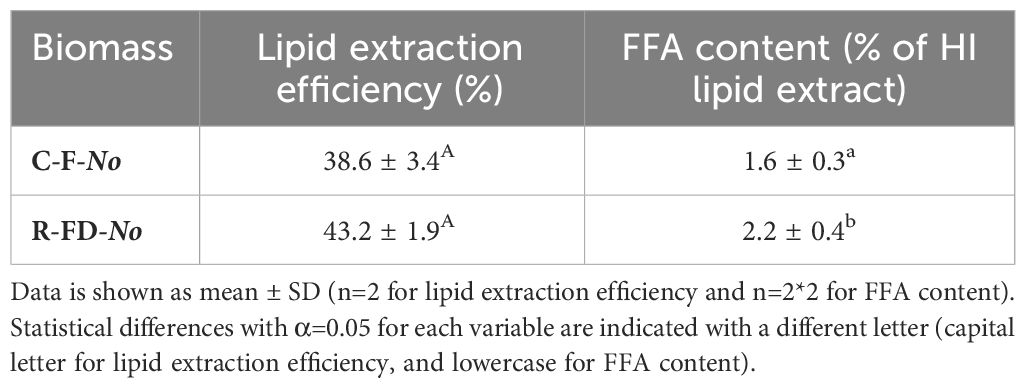
Table 3 Lipid extraction efficiency (%) and FFA content (% of HI lipid extract) of C-F-No and R-FD-No after UAE for 5 min (experiment 2).
4 Discussion
Resuspended freeze-dried microalgal biomass is often used as model laboratory system to represent concentrated fresh microalgal biomass during cell disruption and lipid extraction experiments. For correct interpretation of the effect of cell disruption on the increase in lipid extraction efficiency, it is important that the increase in lipid extraction efficiency is exclusively caused by the applied cell disruption technique, and not by any preceding processing steps. Such a preceding processing step, the resuspension of COM-FD-Nsp in tap water or 3% NaCl and subsequent freeze-drying was evaluated in this study. The resuspension and subsequent freeze-drying will be referred to as ‘resuspension’ to increase readability in the remainder of the text. Resuspension itself did not influence the lipid extraction efficiency nor FFA content independent of resuspension medium. In addition, resuspension medium (tap water or 3% NaCl) and resuspension duration (5, 120 or 450 min) did not significantly increase the lipid extraction efficiency. In contrast, the FFA content used as lipid quality indicator in this study, increased significantly for longer resuspension duration (120, 450 min and 7 days), confirming the presence of endogenous lipases. This implies that tap water or 3% NaCl can both be used to resuspend freeze-dried Nannochloropsis, but the resuspension duration should be short (< 120 min) to avoid lipid degradation.
The condition of N. oculata biomass (concentrated fresh or resuspended freeze-dried) prior to cell disruption by UAE did not influence the lipid extraction efficiency. However, the FFA content in the lipid extract of resuspended freeze-dried biomass was only slightly, yet statistically significant, higher compared to concentrated fresh biomass (2.2 ± 0.4 and 1.6 ± 0.3% of HI lipid extract, respectively). However, as the total amount of FFA detected is low, the increase in FFA with respect to the total amount of lipids is low, the sample size of each treatment is small, the p-value is only marginally significant, and the lipid extraction efficiency is unaffected, this statistical significance should not be used to disprove the suitability of resuspended freeze-dried biomass as a model laboratory system for concentrated fresh biomass.
The lipid extraction efficiency of initial, undisrupted in-house cultivated N. oculata used in the UAE experiment and calculated using Equation 1 was 11.0 ± 0.6%. A different extraction method with different contact time between organic solvent and biomass, presence or absence of cell disruption technique and different organic solvent to biomass ratio and water content, was used after the UAE experiment to calculate the lipid extraction efficiency, resulting in two different equations (Equations 1, 2). Due to these different methods and equations, a direct statistical comparison between the lipid extraction efficiency prior to and post UAE cannot be made. However, the results indicated that the UAE increased the lipid extraction efficiency (lipid extraction efficiency of 11% prior to and ~40% post UAE). These results are in correspondence with literature, where an increase in lipid extraction efficiency is observed after ultrasound treatment of Nannochloropsis (Bermúdez Menéndez et al., 2014; Yao et al., 2018; Figueiredo et al., 2019) The selection of UAE as example cell disruption technique was thus appropriate.
The lipid extraction efficiencies and FFA content of the two Nannochloropsis biomasses used in the two experimental set-ups discussed in this paper differ. Comparison of the absolute values regarding lipid extraction efficiency and FFA content obtained from different batches of microalgae, even on species level, should always be performed with caution as strain and cultivation, harvesting and storage conditions can have an influence on the properties (e.g. cell wall thickness, activation of lipases) of the microalgae (Beacham et al. (2014); Balduyck et al., 2017. In turn, this can influence the susceptibility to cell disruption and resulting lipid extraction efficiency and FFA content (Halim et al., 2019). Due to these variations, trends (e.g., increases or decreases) should be considered instead.
In a similar fashion, extrapolation of the results obtained in this paper (in tap water resuspended, freeze-dried Nannochloropsis can be used as a model laboratory system for concentrated fresh Nannochloropsis during cell disruption, more specifically UAE) to other microalgae, cultivation conditions, cell disruption techniques and release of other components of interest should be done with caution. Nannochloropsis is a marine microalgae with a complex cell wall consisting of algeanan and (hemi)cellulose (Scholz et al., 2014) and a small size (2-5 µm) which greatly differs from the cell wall composition and size of other (freshwater) microalgae (de Carvalho et al., 2020). The different size and composition of cell walls, or lack thereof, can influence the response of the microalgal cell to resuspension medium and duration, the interaction with lipid extraction solvent and the susceptibility to cell disruption (Natarajan et al. (2014). In addition, the proposed underlying mechanism of cell disruption techniques (e.g. weakening of the cell wall, increased temperature, large shear forces, or particle-size reduction) varies depending on disruption technique and microalgal species will likely respond differently to these techniques (Zhang et al., 2019). Further investigation is needed to verify if the results can be transferred to other cell disruption techniques, (lipid) extraction systems, and biorefinery products for Nannochloropsis and microalgae in general. Nevertheless, the results discussed in this paper are highly relevant for research involving the resuspension and ultrasound cell disruption of (freeze-dried) Nannochloropsis for the extraction of lipids.
5 Conclusion
The suitability of resuspended freeze-dried Nannochloropsis biomass as a model laboratory system for concentrated fresh biomass during ultrasound cell disruption and lipid extraction experiments was assessed. Tap water as well as 3% NaCl can be used as resuspension medium for a resuspension duration up to 450 min. If FFA content is a relevant quality indicator, the resuspension duration should be minimized (< 120 min) to avoid lipid degradation. The lipid extraction efficiency after UAE was not affected by the condition of the biomass (concentrated fresh or resuspended freeze-dried). Resuspended freeze-dried microalgal biomass is thus a versatile alternative for fresh biomass during ultrasound cell disruption. Generalization of the results to other cultivation conditions, cell disruption techniques, components of interest or microalgal species should be carefully assessed. Nevertheless, the findings presented in this paper hold significant relevance for researchers applying (ultrasound) cell disruption techniques to resuspended freeze-dried Nannochloropsis and other microalgae.
Data availability statement
The data presented in the study are deposited in the KU Leuven Research Data Repository repository, accession number doi:10.48804/C3YCPZ.
Author contributions
EM: Conceptualization, Formal Analysis, Investigation, Methodology, Project administration, Visualization, Writing – original draft, Writing – review & editing. DV: Conceptualization, Funding acquisition, Writing – review & editing. IF: Conceptualization, Funding acquisition, Methodology, Project administration, Resources, Writing – review & editing.
Funding
The author(s) declare that financial support was received for the research, authorship, and/or publication of this article. This work was supported by Flanders’ Food and funded by Flanders Innovation and Entrepreneurship (VLAIO) through the cSBR project EffSep (Grant number HBC.2019.0012).
Acknowledgments
The authors wish to thank Dr. Jonas Blockx for proofreading the paper.
Conflict of interest
The authors declare that the research was conducted in the absence of any commercial or financial relationships that could be construed as a potential conflict of interest.
Publisher’s note
All claims expressed in this article are solely those of the authors and do not necessarily represent those of their affiliated organizations, or those of the publisher, the editors and the reviewers. Any product that may be evaluated in this article, or claim that may be made by its manufacturer, is not guaranteed or endorsed by the publisher.
Abbreviations
n-3 LC-PUFA, Omega-3 long-chain polyunsaturated fatty acid; FFA, free fatty acids; HI, Hexane/isopropanol; COM-FD-Nsp, Commercial freeze-dried Nannochloropsis sp.; C-F-No, Concentrated fresh Nannochloropsis oculata; R-FD-No, Resuspended freeze-dried Nannochloropsis oculata.
References
Alhattab M., Kermanshahi-Pour A., Brooks M. S.-L. (2019). Microalgae disruption techniques for product recovery: influence of cell wall composition. J. Appl. Phycol. 31, 61–88. doi: 10.1007/s10811-018-1560-9
Balduyck L., Bruneel C., Goiris K., Dejonghe C., Foubert I. (2018). Influence of high pressure homogenization on free fatty acid formation in nannochloropsis sp. Eur. J. Lipid Sci. Technol. 120, 1700436. doi: 10.1002/ejlt.201700436
Balduyck L., Stock T., Bijttebier S., Bruneel C., Jacobs G., Voorspoels S., et al. (2017). Integrity of the microalgal cell plays a major role in the lipolytic stability during wet storage. Algal Res. 25, 516–524. doi: 10.1016/j.algal.2017.06.013
Barbosa M. J., Janssen M., Südfeld C., D’Adamo S., Wijffels R. H. (2023). Hypes, hopes, and the way forward for microalgal biotechnology. Trends Biotechnol. 41, 452–471. doi: 10.1016/j.tibtech.2022.12.017
Beacham T. A., Bradley C., White D. A., Bond P., Ali S. T. (2014). Lipid productivity and cell wall ultrastructure of six strains of Nannochloropsis: Implications for biofuel production and downstream processing. Algal Res. 6, 64–69. doi: 10.1016/j.algal.2014.09.003
Bermúdez Menéndez J. M., Arenillas A., Menéndez Díaz J.Á., Boffa L., Mantegna S., Binello A., et al. (2014). Optimization of microalgae oil extraction under ultrasound and microwave iradiation. J. Chem. Technol. Biotechnol. 89, 1779–1784. doi: 10.1002/jctb.4272
Bernaerts T. M. M., Gheysen L., Foubert I., Hendrickx M. E., Van Loey A. M. (2019). Evaluating microalgal cell disruption upon ultra high pressure homogenization. Algal Res. 42, 101616. doi: 10.1016/j.algal.2019.101616
Calder P. C. (2021). “Chapter 2 - Health benefits of omega-3 fatty acids,” in Omega-3 delivery systems. Eds. García-Moreno P. J., Jacobsen C., Sørensen A.-D. M., Yesiltas B. (London, United Kingdom: Academic Press), 25–53. doi: 10.1016/b978-0-12-821391-9.00006-5
Chua E. T., Schenk P. M. (2017). A biorefinery for Nannochloropsis: Induction, harvesting, and extraction of EPA-rich oil and high-value protein. Bioresour. Technol. 244, 1416–1424. doi: 10.1016/j.biortech.2017.05.124
de Carvalho J. C., Magalhães A. I., de Melo Pereira G. V., Medeiros A. B. P., Bittencourt Sydney E., Rodrigues C., et al. (2020). Microalgal biomass pretreatment for integrated processing into biofuels, food, and feed. Bioresour. Technol. 300, 122719. doi: 10.1016/j.biortech.2019.122719
EU (2009). Directive 2009/32/EC. Commision Directive 2009/32/EC of the European Parliament and of the Council of 23 April 2009 on the approximation of the laws of the Member States on extraction solvents used in the production of foodstuffs and food ingredients (Official Journal of the European Union). L 141/3 = L 141/11.
Figueiredo A. R. P., da Costa E., Silva J., Domingues M. R., Domingues P. (2019). The effects of different extraction methods of lipids from Nannochloropsis oceanica on the contents of omega-3 fatty acids. Algal Res. 41, 101556. doi: 10.1016/j.algal.2019.101556
Guillard R. R. L., Lorenzen C. J. (1972). Yellow-green algae with chlorophyllide. C. J.Phycol. 8, 10–14. doi: 10.1111/j.1529-8817.1972.tb03995.x
Halim R., Danquah M. K., Webley P. A. (2012). Extraction of oil from microalgae for biodiesel production: A review. Biotechnol. Adv. 30, 709–732. doi: 10.1016/j.biotechadv.2012.01.001
Halim R., Hill D. R. A., Hanssen E., Webley P. A., Blackburn S., Grossman A. R., et al. (2019). Towards sustainable microalgal biomass processing: Anaerobic induction of autolytic cell-wall self-ingestion in lipid-rich: Nannochloropsis slurries. Green Chem. 21, 2967–2982. doi: 10.1039/C8GC03186J
Halim R., Papachristou I., Kubisch C., Nazarova N., Wüstner R., Steinbach D., et al. (2021). Hypotonic osmotic shock treatment to enhance lipid and protein recoveries from concentrated saltwater Nannochloropsis slurries. Fuel 287, 119442. doi: 10.1016/j.fuel.2020.119442
Lee A. K., Lewis D. M., Ashman P. J. (2012). Disruption of microalgal cells for the extraction of lipids for biofuels: Processes and specific energy requirements. Biomass Bioenergy 46, 89–101. doi: 10.1016/j.biombioe.2012.06.034
Maffei G., Bracciale M. P., Broggi A., Zuorro A., Santarelli M. L., Lavecchia R. (2018). Effect of an enzymatic treatment with cellulase and mannanase on the structural properties of Nannochloropsis microalgae. Bioresour. Technol. 249, 592–598. doi: 10.1016/j.biortech.2017.10.062
Mannion D. T., Furey A., Kilcawley K. N. (2016). Free fatty acids quantification in dairy products. Int. J. Dairy Technol. 69, 1–12. doi: 10.1111/1471-0307.12301
Martínez-Sanz M., Garrido-Fernández A., Mijlkovic A., Krona A., Martínez-Abad A., Coll-Marqués J. M., et al. (2020). Composition and rheological properties of microalgae suspensions: Impact of ultrasound processing. Algal Res. 49, 101960. doi: 10.1016/j.algal.2020.101960
Mienis E., Vandamme D., Foubert I. (2023). Enzyme-assisted disruption of oleaginous microalgae to increase the extraction of lipids: Nannochloropsis as a case study. Curr. Opin. Food Sci. 51, 101034. doi: 10.1016/j.cofs.2023.101034
Natarajan R., Ang W. M. R., Chen X., Voigtmann M., Lau R. (2014). Lipid releasing characteristics of microalgae species through continuous ultrasonication. Bioresour. Technol. 158, 7–11. doi: 10.1016/j.biortech.2014.01.146
Quesada-Salas M. C., Delfau-Bonnet G., Willig G., Préat N., Allais F., Ioannou I. (2021). Optimization and comparison of three cell disruption processes on lipid extraction from microalgae. Processes 9, 1–20. doi: 10.3390/pr9020369
Rezaei Motlagh S., Harun R., Awang Biak D. R., Hussain S. A., Omar R., Khezri R., et al. (2021). Ionic liquid-based microwave-assisted extraction of lipid and eicosapentaenoic acid from Nannochloropsis oceanica biomass: experimental optimization approach. J. Appl. Phycol. 33, 2015–2029. doi: 10.1007/s10811-021-02437-9
Ryckebosch E., Bermúdez S. P. C., Termote-Verhalle R., Bruneel C., Muylaert K., Parra-Saldivar R., et al. (2014). Influence of extraction solvent system on the extractability of lipid components from the biomass of Nannochloropsis gaditana. J. Appl. Phycol. 26, 1501–1510. doi: 10.1007/s10811-013-0189-y
Ryckebosch E., Bruneel C., Termote-Verhalle R., Lemahieu C., Muylaert K., Van Durme J., et al. (2013). Stability of omega-3 LC-PUFA-rich photoautotrophic microalgal oils compared to commercially available omega-3 LC-PUFA oils. J. Agric. Food Chem. 61, 10145–10155. doi: 10.1021/jf402296s
Ryckebosch E., Muylaert K., Foubert I. (2012). Optimization of an analytical procedure for extraction of lipids from microalgae. J. Am. Oil Chem. Soc 89, 189–198. doi: 10.1007/s11746-011-1903-z
Scholz M. J., Weiss T. L., Jinkerson R. E., Jing J., Roth R., Goodenough U., et al. (2014). Ultrastructure and composition of the Nannochloropsis gaditana cell wall. Eukaryot. Cell 13, 1450–1464. doi: 10.1128/EC.00183-14
Van Meulebroek L., De Paepe E., Vercruysse V., Pomian B., Bos S., Lapauw B., et al. (2017). Holistic lipidomics of the human gut phenotype using validated ultra-high-performance liquid chromatography coupled to hybrid orbitrap mass spectrometry. Analytical Chem. 89, 12502–12510. doi: 10.1021/acs.analchem.7b03606
Van Wayenbergh E., Blockx J., Langenaeken N. A., Foubert I., Courtin C. M. (2023). Conversion of retinyl palmitate to retinol by wheat bran endogenous lipase reduces vitamin A stability. Foods 13, 80. doi: 10.3390/foods13010080
Van Wychen S., Laurens L. M. L. (2015) Determination of Total Solids and Ash in Algal Biomass. Tech. Rep. NREL/TP-5100-60956. Available online at: http://www.osti.gov/servlets/purl/1118077/.
Verspreet J., Kreps S., Bastiaens L. (2020). Evaluation of microbial load, formation of odorous metabolites and lipid stability during wet preservation of Nannochloropsis gaditana concentrates. Appl. Sci. 10, 3419. doi: 10.3390/app10103419
Xu Y. (2022). Biochemistry and biotechnology of lipid accumulation in the microalga nannochloropsis oceanica. J. Agric. Food Chem. 70, 11500–11509. doi: 10.1021/acs.jafc.2c05309
Yao S., Mettu S., Law S. Q. K., Ashokkumar M., Martin G. J. O. (2018). The effect of high-intensity ultrasound on cell disruption and lipid extraction from high-solids viscous slurries of Nannochloropsis sp. biomass. Algal Res. 35, 341–348. doi: 10.1016/j.algal.2018.09.004
Zhang R., Parniakov O., Grimi N., Lebovka N., Marchal L., Vorobiev E. (2019). Emerging techniques for cell disruption and extraction of valuable bio-molecules of microalgae Nannochloropsis sp. Bioprocess Biosyst. Eng. 42, 173–186. doi: 10.1007/s00449-018-2038-5
Zhao K., Zhang L., Zhang M., Tian H., He D., Zheng J. (2022). Response surface optimization of enzyme pretreatment improves yield of ethanol-extracted lipids from nannochloropsis oceanica. Eur. J. Lipid Sci. Technol. 124, 2100200. doi: 10.1002/ejlt.202100200
Keywords: microalgae, Nannochloropsis, disruption, resuspension, lipid extraction efficiency, free fatty acid, ultrasonication
Citation: Mienis E, Vandamme D and Foubert I (2024) Resuspended freeze-dried Nannochloropsis as a model laboratory system for concentrated fresh Nannochloropsis in ultrasound cell disruption experiments. Front. Mar. Sci. 11:1359090. doi: 10.3389/fmars.2024.1359090
Received: 20 December 2023; Accepted: 22 April 2024;
Published: 03 May 2024.
Edited by:
Jelena Vladic, NOVA University of Lisbon, PortugalReviewed by:
Tonmoy Ghosh, Durban University of Technology, South AfricaRonald Halim, University College Dublin, Ireland
Copyright © 2024 Mienis, Vandamme and Foubert. This is an open-access article distributed under the terms of the Creative Commons Attribution License (CC BY). The use, distribution or reproduction in other forums is permitted, provided the original author(s) and the copyright owner(s) are credited and that the original publication in this journal is cited, in accordance with accepted academic practice. No use, distribution or reproduction is permitted which does not comply with these terms.
*Correspondence: Imogen Foubert, aW1vZ2VuLmZvdWJlcnRAa3VsZXV2ZW4uYmU=