- 1School of Marine Science, Ningbo University, Ningbo, China
- 2Collaborative Innovation Center for Zhejiang Marine High-efficiency and Healthy Aquaculture, Ningbo University, Ningbo, China
The caudal neurosecretory system (CNSS) of teleost is intricately linked to reproduction. This study sought to explore the association between the CNSS and the reproductive cycle of Pampus argenteus. Utilizing microscopy and transmission electron microscopy, we observed the morphological alterations within the CNSS. Furthermore, we quantified the levels of urotensin-I (U-I), urotensin-II (U-II), cortisol, and corticotropin-releasing hormone (CRH) throughout the reproductive process. Findings revealed that Dahlgren cells, distributed along the spinal cord adjacent to the terminal 6 vertebrae of the silver pomfret’s tail, were primarily concentrated within the final 3 vertebrae. The neurohypophysis was characterized by an elongated oval form when viewed laterally and an almost spherical configuration in ventral perspective. Post-breeding season, the average diameters of Type I and Type II Dahlgren cells decreased by 21.8% and 15.44%, respectively (P < 0.05), accompanied by a reduction in neurosecretory granules to merely 60% of their initial pre-reproductive state (P < 0.05). Variations in the serum and gonadal concentrations of U-I, U-II, cortisol, and CRH indicated a significant correlation with gonadal maturation. In summary, the CNSS plays a crucial role in gonadal development, directly or indirectly influencing and modulating the reproductive activities of P. argenteus.
1 Introduction
The Caudal Neurosecretory System (CNSS) resides at the extremity of the spinal cord and bears resemblance to the hypothalamus-pituitary axis found in vertebrates (Winter et al., 2000). This distinctive neurosecretory system in fish comprises three primary components: the caudal neurosecretory cells (Dahlgren cells), axon bundles stemming from these cells, and the neurohypophysis. The CNSS is instrumental in various adaptive physiological processes, including osmoregulation, reproduction, nutrition, and stress responses (Lu et al., 2004, 2006). Previous study suggested that the CNSS could undergo functional reprogramming to accommodate physiological demands during seasonal fluctuations (Chen and Mu, 2008). Additionally, the CNSS secretes urotensin, a peptide crucial for regulating reproduction, osmoregulation, and cardiovascular functions via the circulatory system (Berlind, 1972; Audet and Chevalier, 1981; Owada et al., 1985; Cioni et al., 1998; McCrohan et al., 2007). Investigations have identified the co-expression of up to three peptides, corticotropin-releasing hormone (CRH), urotensin I and II (U-I, U-II), within a single Dahlgren cell, indicating their capability to synthesize and secrete these peptides (McCrohan et al., 2007). These secretory molecules are transported through axons to a distinct neurohemal organ for release into the bloodstream (Lu et al., 2012). The previous studies on teleost species have highlighted the negative impacts of stress, including cortisol-induced effects on the gonadotropic axis and reproductive efficiency. Stress is related to reduced levels of plasma vitellogenin (Vg) (Campbell et al., 1994) and luteinizing hormone (LH) (Van Der Kraak et al., 1992), alongside decreased mRNA expression of hypothalamic gonadotropin-releasing hormone 1 (GnRH1) (Imanaga et al., 2014), leading to delayed ovulation (Campbell et al., 1992), smaller egg size in females (Mileva et al., 2011), decreased sperm count in males (Campbell et al., 1994), and lowered fecundity (Contreras-Sanchez et al., 1998).
The silver pomfret (Pampus argenteus), a migratory pelagic species, is found across various regions including the Indian Ocean, North Sea, Persian Gulf, Oman Sea, and the coastal zones of the China Sea, from the Bohai Sea to the South China Sea (Zhao et al., 2011; Archangi et al., 2013). The demand for this fish, coupled with overfishing, has led to a decline in its natural populations. Despite the shift toward large-scale farming facilitated by technological advancements in P. argenteus culture, challenges such as high mortality rates and poor developmental outcomes persist. The CNSS is recognized for its pivotal role in the reproductive regulation of fish; however, studies detailing the morphology, tissue structure, and contribution of the CNSS to the reproductive processes of P. argenteus are lacking. Thus, deepening our understanding of the CNSS in P. argenteus will significantly enhance our comprehension of the neuroendocrine system in higher vertebrates.
2 Materials and methods
2.1 Animal and sample collection
The experiment utilized Pampus argenteus reared in Zhoushan, Zhejiang, China. These were 1-year-old adult fish, raised in controlled artificial breeding ponds, selected based on their health, activity, and regular feeding habits. Prior to the spawning season, a monthly sampling of 20 individuals was conducted. The body length of P. argenteus ranged from 12.6 to 15.0 cm, averaging of 13.83 ± 0.74 cm, and a body weight varied from 67.15 to 107.58 g, with an average weight of 84.50 ± 14.05 g. In total, 200 P. argenteus were collected. During the sampling period, the water temperature in the aquaculture ponds was maintained at 24.0 ± 1.0°C, pH at 8.0 ± 0.3, and salinity at 25.0 ± 1.0. For sampling the fish, MS-222 (100 mg/L, Tricaine methanesulfonate, Sigma-Aldrich Co. LLC.) was used to anesthetize the fish for sampling. Samples of both female and male fish, including gonads, blood, and CNSS, were collected at stages IV-VI. The gonads were used for histological examination to confirm the gonadal status according to Guo et al. (2024) and hormones test. Blood was collected from each specimen, then centrifuged at 4500 rpm for 10 minutes. The supernatant was stored at -20°C for future serum hormone analysis. Meanwhile, all the CNSS was collected and fixed in different fixation solutions. After histological analysis of the gonads, the fixed CNSS was grouped for different experiments.
2.2 Morphological observation of CNSS
When collected the CNSS, the fish was placed on an ice tray for dissection. Muscle tissue from the tail at the 10th to last vertebra, was carefully removed, and one side of the neural arch was sequentially retracted, fully revealing the spinal cord and neurohypophysis associated with the last 10 vertebrae. External morphology was documented and measured using a Nikon C-BD230 stereomicroscope.
2.3 The histology of CNSS
CNSS samples were fixed in Bouin’s solution at 4°C for 24 hours. Subsequently, the samples were dehydrated by replacing the fixative with 70% ethanol, followed by a series of alcohol gradients for further dehydration, then cleared in xylene, and finally embedded in paraffin. Cross and longitudinal continuous sections with a thickness of 5 μm were prepared, mounted, dried, deparaffinized, dehydrated, and stained with hematoxylin and eosin (H&E). Typical tissue structures were examined using a Nikon Ni-U research-grade microscope, with images captured using the Nikon DS-Ri2 imaging system (CCD camera).
2.4 The transmission electron microscopy of CNSS
For transmission electron microscopy (TEM), the tails of fish were dissected to obtain the spinal cord and attached neurohypophysis corresponding to the terminal vertebra. These samples were immediately fixed in pre-cooled 2.5% glutaraldehyde for 8-10 hours. Afterwards, the samples were trimmed to dimensions of 1mm×1mm×1mm and stored overnight at 4°C. The samples were then washed thrice for 15 minutes each in 0.1M phosphate buffer (PBS). This was followed by fixation in 1% osmium tetroxide for 1-2 hours at 4°C, and then rinsed twice in PBS for 15 minutes. Dehydration was achieved through a graded series of ethanol and propylene oxide treatments (30%, 50%, 70%, 90% ethanol, followed by 90% and 100% propylene oxide) for 15 minutes each, with four changes to 100% propylene oxide. The samples were embedded in Epon 812 epoxy resin and polymerized in an oven with temperature gradients of 37°C, 45°C, and 65°C, each for 24 hours. Ultra-thin sections (40-60 nm) were prepared using an Ultracut ultramicrotome and double-stained with uranyl acetate and lead citrate for observation under an H-7650 transmission electron microscope (Hitachi, Japan).
2.5 The detection of hormones
The fish ELISA Kit (Jiangsu Meimian Industrial Co., Ltd) was used for measuring U-I (26297), U-II (26300), Cortisol (25076), and CRH (26299) in serum, gonads, and CNSS of P. argenteus. For gonads and CNSS tissue analyses, samples were homogenized in ice-cold physiological saline solution at a specific volume ratio, then centrifuged at 2,000 g for 5 minutes at 4°C to separate the supernatant. Total protein was quantified using the supernatant and the Micro BCA Protein Assay Kit (CWBIO) following the manufacturer’s guidelines. Three independent biological replicates were used for each analysis. After that, the specific commercial kits was used to detect the levels of U-I, U-II, Cortisol, and CRH.
2.6 Data analysis
ImageJ software facilitated the random selection of cells and their internal organelles for measurement and statistical analysis. In each fish, 10 fields were randomly chosen from 10 slices around the central canal of the spinal cord. Within these fields, nuclear Dahlgren cells, their neurosecretory granules, and clear vacuoles were enumerated. Data were presented as the mean ± SD (standard deviation). All the data was statistically evaluated with SPSS 19.0 software. The Shapiro–Wilk method was used to analyze the normality of data distribution. Statistical analysis was conducted using one-way ANOVA, followed by Tukey’s test, with P < 0.05 considered statistically significant.
3 Results
3.1 The morphology and histological structure of CNSS in P. argenteus
The location of the CNSS in P. argenteus is illustrated in Figure 1, identified within an enlarged spherical structure connected to the ventral side of the spinal cord corresponding to the last 6 vertebrae. The CNSS mainly composed Dahlgren cells, axons, and the neurohypophysis. The spinal cord noticeably narrowed from the position corresponding to the third-to-last vertebra, with its diameter progressively reducing from approximately 150 μm to less than 70 μm. The spherical enlarged structure resided in the socket of the tail’s last vertebra, presenting two forms: elongated and spherical. The spinal cord narrowed towarded the tail, and at the socket of the last vertebra, an enlarged protruding structure on the ventral side, which identified as the neurohypophysis was observed (Figure 2A). The neurohypophysis, connected to the posterior spinal cord and enclosed by the outer spinal membrane, features a distinctive concave structure on the dorsal side of the corresponding vertebral body. Extending backward from the dorsal side of the neurohypophysis was the terminal filament of the spinal cord. The neurohypophysis in P. argenteus primarily exhibited an elongated (Figure 2B) and a spherical shape (Figure 2C), with dimensions of approximately 3870 μm × 990 μm × 820 μm for the elongated type and 2060 μm × 1070 μm× 900 μm.
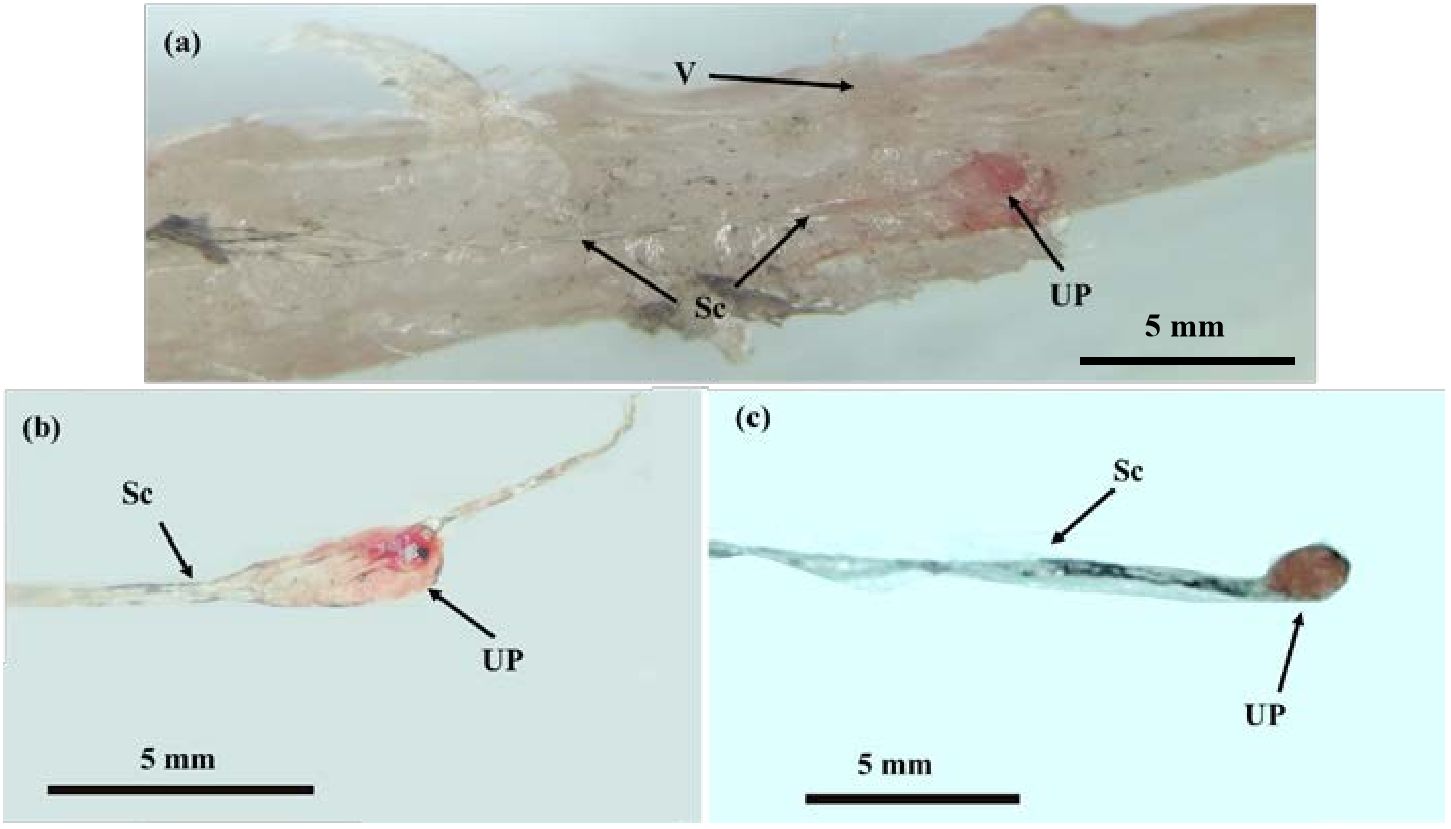
Figure 2 The Morphological structure of the CNSS in P. argenteus. (A) The microstructure of CNSS in P. argenteus; (B) The lateral view of single elongated neurohypophysis; (C) The ventral view of a single spherical neurohypophysis. V, vertebra; UP, neurohypophysis; Sc, spinal cord. Schematic diagram of CNSS of P. argenteus.
As showed in Figure 3, Dahlgren cells clustered and distributed at the spinal cord’s end, surrounding the central canal with diverse morphologies. Predominantly, they were located on both sides of the central canal. Dahlgren cells were differentiated into two main types: type I Dahlgren cells and type II. Type I Dahlgren cells, larger in volume with an irregular morphology, typically contain 2 nuclei, occasionally 3, and had a lighter cytoplasm, identified as large caudal neurosecretory cells. Conversely, Type II Dahlgren cells, smaller in cross-sectional area and generally possessing a single nucleus, exhibited either elongated or spherical shapes with a relatively darker cytoplasm compared to Type I cells. Dahlgren cells were concentrated in the spinal cord at the last 3 vertebrae. Their numbers diminish towarded the anterior part of the spinal cord, becoming sparser within the region corresponding to the last 4 to 6 vertebrae and absented in the spinal cord beyond the 7th vertebra from the tail’s end.
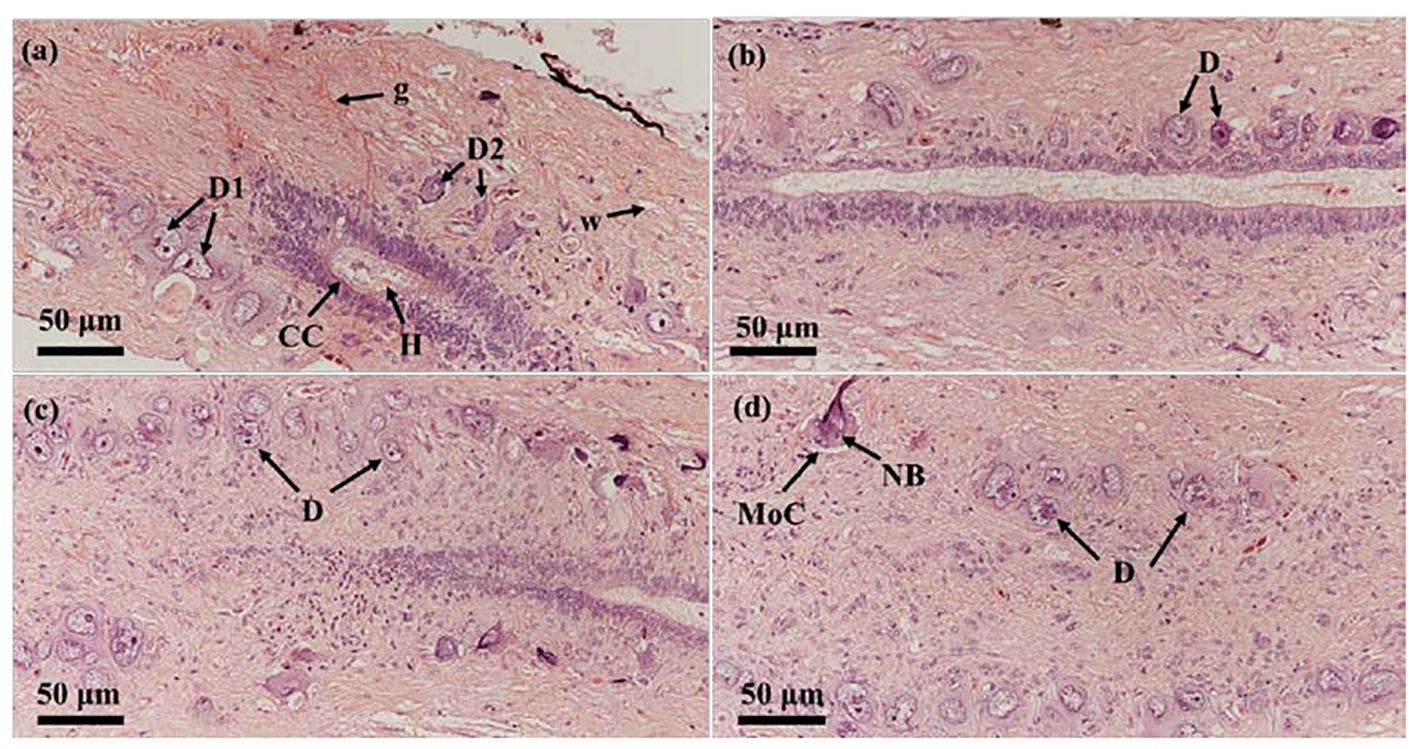
Figure 3 The two types of Dahlgren cells in P. argenteus and their distribution in the last three segments of the spinal cord. (A) Dahlgren I and II cell types; (B) Dahlgren cells in the third-to-last segment of the spinal cord; (C) Dahlgren cells in the second-to-last segment of the spinal cord; (D) Dahlgren cells in the last segment of the spinal cord. D, Dahlgren cells; D1, Dahlgren I cells; D2, Dahlgren II cells; g, gray matter; w, white matter; CC, central canal; MoC, motor cells; H, Herring bodies; NB, Nissl bodies.
3.2 Microstructural and numerical changes in the CNSS throughout the reproductive process
HE staining showed that Dahlgren cells, prior to breeding season, were characterized by large volumes, intense staining, abundant inclusions, and a dense arrangement, encircled by numerous other nerve cells (Figure 4A). Post-breeding season, these cells displayed reduced volumes, vacuolation, a sparse distribution, and fewer surrounding nerve cells. During the reproductive period, significant content discharged from the cell bodies led to diminished volume and vacuolation (Figure 4B). Prior to breeding season, within the spinal cord at the tail’s last vertebra and the neurohypophysis, Herring bodies were distinctly visible, deeply stained, indicating they were secretion-rich (Figure 4C). Post-breeding season, at the same sites, Herring bodies appeared noticeably reduced in size and number, with increased surrounding vacuoles (Figure 4D).
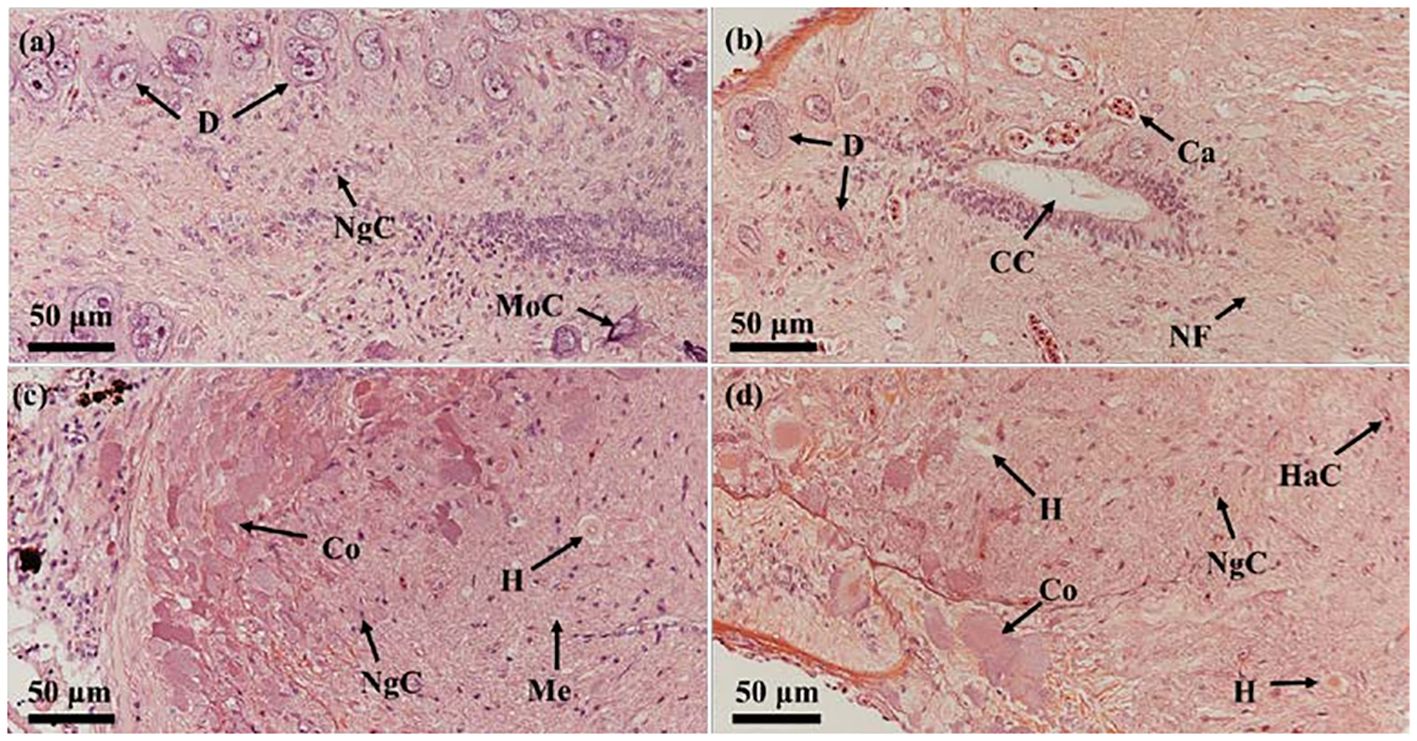
Figure 4 Comparison of the histological structure of Dahlgren cells and neurohypophysis throughout breeding season. (A) Morphology of Dahlgren cells before breeding season (gonadal stage IV); (B) Morphology of Dahlgren cells after breeding season (gonadal stage VI); (C) Morphology of Herring bodies before breeding season (gonadal stage V); (D) Morphology of Herring bodies after breeding season (gonadal Stage VI). D, Dahlgren cells; MoC, motor cells; Ca, capillary; CC, central canal; NsC, neurosecretory cells; NgC, neuroglial cells; NF, nerve fiber; H, Herring body; HaC, hematocyte; Co, cortex; Me, medulla.
During the breeding season process of P. argenteus, there were significant differences in the size of Dahlgren cells. As showed in Table 1, in the anterior and middle sections, the diameter of Dahlgren cells during the reproductive stage (gonadal stage V) was marginally larger than in stage IV, though not statistically (P > 0.05). Conversely, in the posterior section, a significant increase in diameter between stages IV and V before breeding season was noted, with a 24.75% increase in stage V (P < 0.05). The diameters in stage IV were slightly larger than in stage VI, but the difference was not significant (P > 0.05). In stage V, the diameters of Dahlgren cells in the anterior, middle, and posterior sections were 41.89 ± 2.08 μm, 26.35 ± 1.63 μm, and 22.95 ± 5.11 μm, respectively. After breeding season in stage VI, the diameters of Dahlgren cells decreased by an average of 26.12%, 12.64%, and 29.85%, respectively (P < 0.05). Table 2 showed that the diameters of Type I and Type II Dahlgren cells in stage V before breeding season were marginally larger than in stage IV, with no significant difference (P > 0.05). The diameters of these two cell types in stage V were 39.59 ± 4.04 μm and 21.24 ± 2.62 μm, respectively. After breeding season in stage VI, Type I and Type II Dahlgren cells decreased by an average of 21.8% and 15.44%, respectively (P < 0.05).
3.3 Ultrastructure in the CNSS throughout the reproductive process
As shown in Figure 5, the Dahlgren cells prior to breeding season were rich in organelles, including mitochondria, endoplasmic reticulum, Golgi apparatus, ribosomes, and lysosomes, with an abundance of secretory and glycogen granules encircling the cell. Post-breeding season, there was a notable decrease in the variety of organelles within the Dahlgren cell body, which then featured numerous vacuoles and sparsely distributed secretory granules throughout the cytoplasm. Initially, a significant presence of lysosomes with a lighter hue was observed in the Dahlgren cell body; however, post-breeding season, primary lysosomes were seldom seen, and there was a reduction in both their number and size, accompanied by a darker coloration (Figures 5A–D). The analysis of Dahlgren cell protrusions and terminal neurosecretory granules throughout the breeding season cycle was presented in Figure 5E. Before breeding season, at the gonadal stage IV, Dahlgren cell protrusions and both types of neurosecretory granules within the terminals were densely arranged, with a density of 17.3 per unit area. Following breeding season, at the gonadal stage VI, the quantity of neurosecretory granules significantly diminished (P < 0.05), constituting only 60% of the count observed at gonadal stage V.
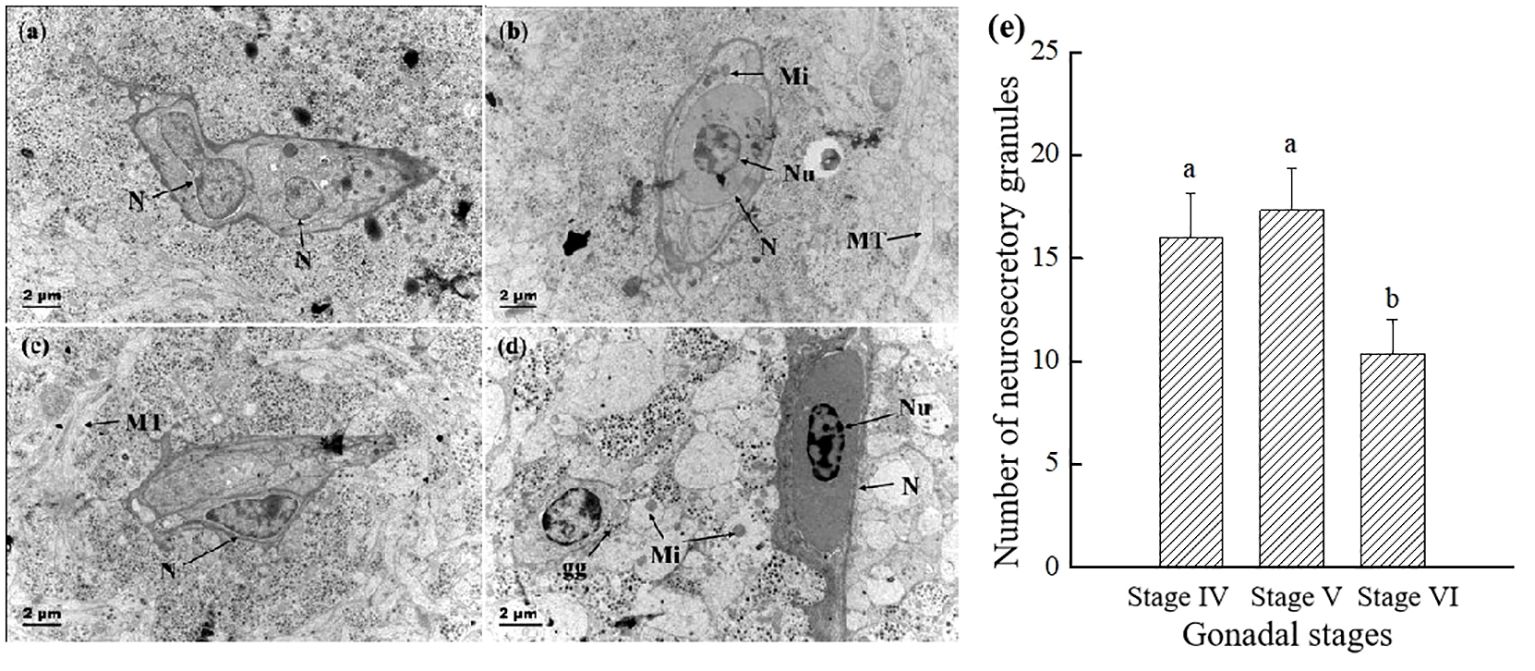
Figure 5 Comparison of the electron micrograph of two types of Dahlgren cells and the number of neurosecretory granules throughout breeding season. (A) Type I Dahlgren cells before breeding season (gonadal stage IV); (B) Type I Dahlgren cells after breeding season (gonadal stage VI); (C) Type II Dahlgren cells before breeding season (gonadal stage IV); (D) Type II Dahlgren cells after breeding season (gonadal stage VI); (E) Number of neurosecretory granules per unit area in the CNSS (n = 6 fish for each value). N, Nucleus; MT, microtubules; Nu, nucleolus; nl, lipid droplet; Mi, mitochondria; gg: glycogen granules. Superscript letters indicate one-way ANOVA, followed by Tukey’s test (P < 0.05).
The changes of the Herring’s body and mitochondria throughout reproductive process were showed in Figure 6. Before spawning, the bodies of herrings are filled with abundant secretory granules, large in volume, and contain a small number of electron-transparent vesicles (Figures 6A, B). Prior to breeding season, mitochondria were abundant and numerous, with clear and distinct cristae structures. After breeding season, the cristae within mitochondria decreased, appeared vacuolated (Figures 6C, D).
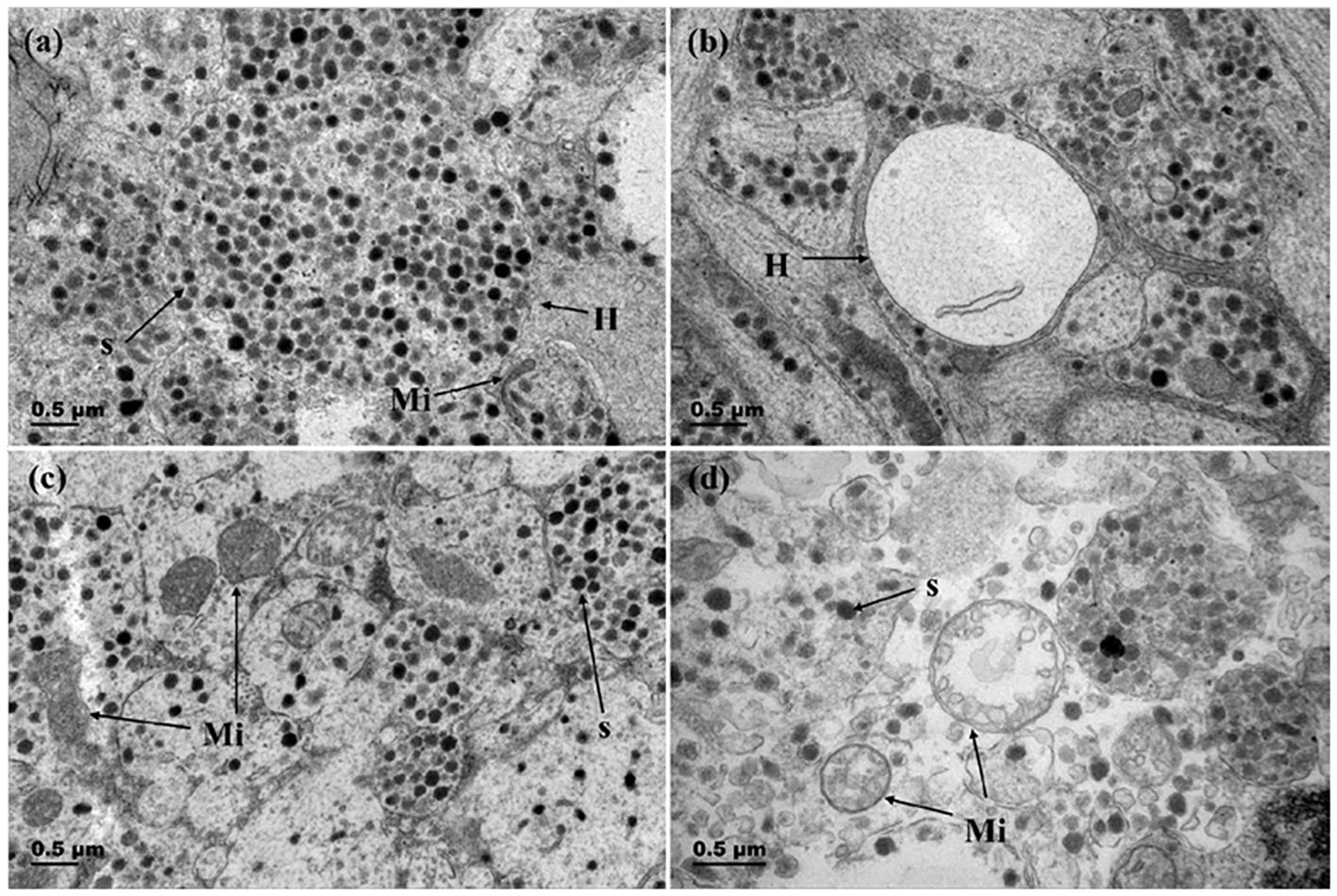
Figure 6 Changes in Herring bodies and mitochondria before and after breeding season. (A) Herring body before breeding season (gonadal stage IV); (B) Herring body after breeding season (gonadal stage VI); (C) Mitochondria in Dahlgren cells before breeding season (gonadal stage IV); (D) Mitochondria in Dahlgren cells after breeding season (gonadal stage VI). H, Herring body; S, neurosecretory granules; Mi, Mitochondria.
Figure 7 showed that before breeding season, the nuclei of Dahlgren cells were predominantly irregular in shape, featuring complex invaginations in the nuclear membrane, and the capillary lumen was large and smooth. The endothelial cell nuclei were either elliptical or irregular and prominently visible, with the luminal surface closely associated with cell protrusions or axon terminals filled with secretory granules. After breeding season, Dahlgren cell nuclei mostly appeared smooth, and the capillaries presented a constricted appearance with endothelial cells exhibiting increased folds protruding into the lumen. The number of secretory granules within axon terminals adjacent to the basement membrane decreased, occasionally showing vacuolation.
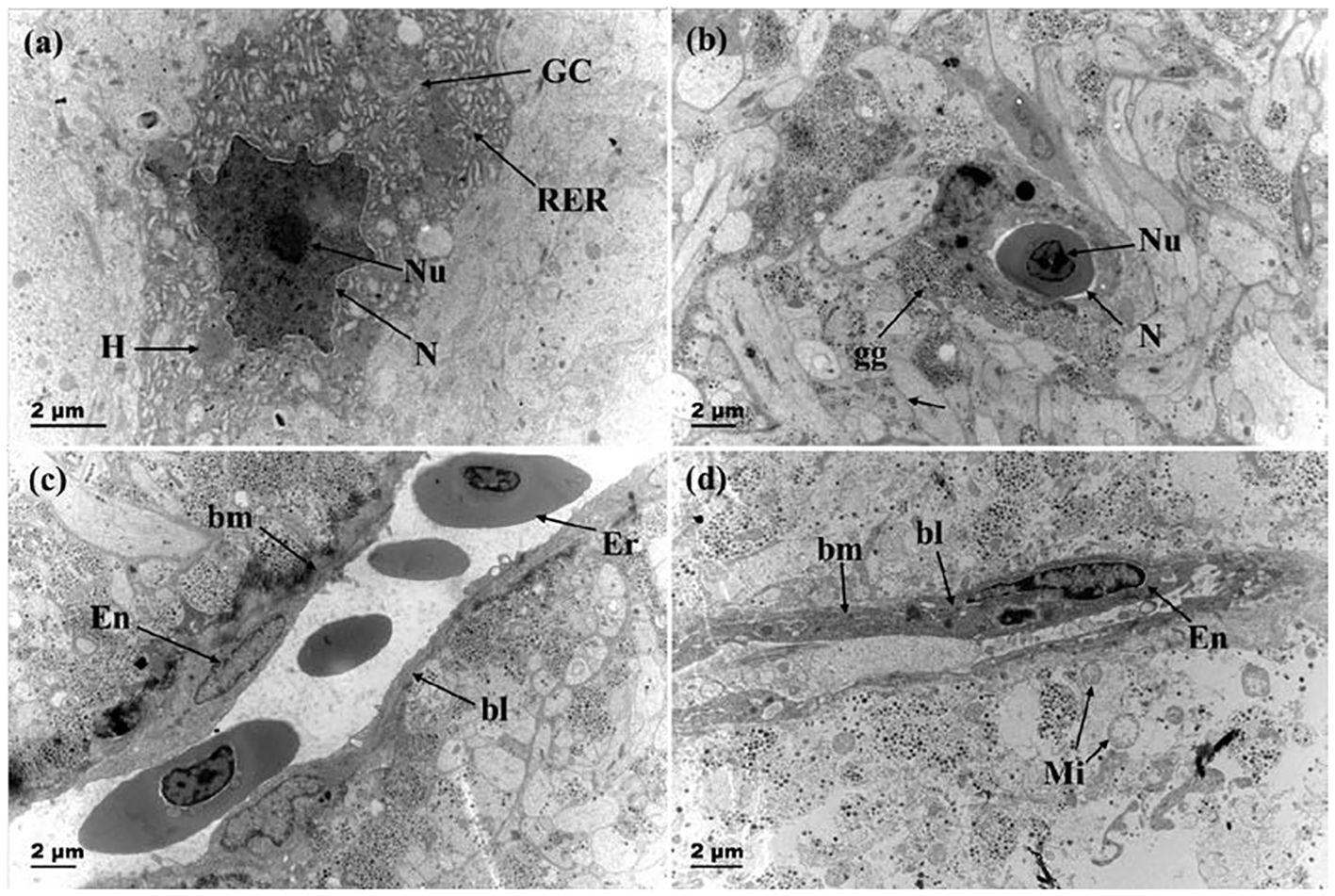
Figure 7 Changes in the nucleus of neurosecretory cells and capillaries before and after breeding season. (A) Dahlgren cells with invaginated nuclear membrane before breeding season (gonadal stage IV); (B) Smooth nuclear membrane of Dahlgren cell after breeding season (gonadal stage VI); (C) Capillary in adult fish before breeding season (gonadal stage IV); (D) Capillary after breeding season (gonadal stage VI). RER, rough endoplasmic reticulum; GC: Golgi apparatus; N, Nucleus; Nu, Nucleolus; gg, Glycogen granules; Mi, Mitochondria; Ca, Capillary; bm, basement membrane; bl, basal lamina; Er, red blood cell; En, Capillary endothelial cell.
3.4 Changes in hormone levels in gonads and plasma throughout the reproductive process
As shown in Figure 8, the levels of U-I, U-II, Cortisol, and CRH were measured in the gonads and plasma. The findings indicated that U-I levels remained consistent across stages IV to VI in both gonads and plasma (Figures 8A, B). U-II levels in gonads at stage VI were significantly lower than stage IV and V (Figure 8C). However, U-II levels in plasma showed no significant changes throughout the reproductive process (Figure 8D). Cortisol levels in gonads significantly decreased from stage IV to stage V, followed by a significant increase from stage V to VI (Figure 8E). In plasma, cortisol levels at gonadal stage VI were significantly elevated compared to stages IV and V (Figure 8F). CRH levels in both gonads and plasma exhibited no significant fluctuations throughout the reproductive process (Figures 8G, H).
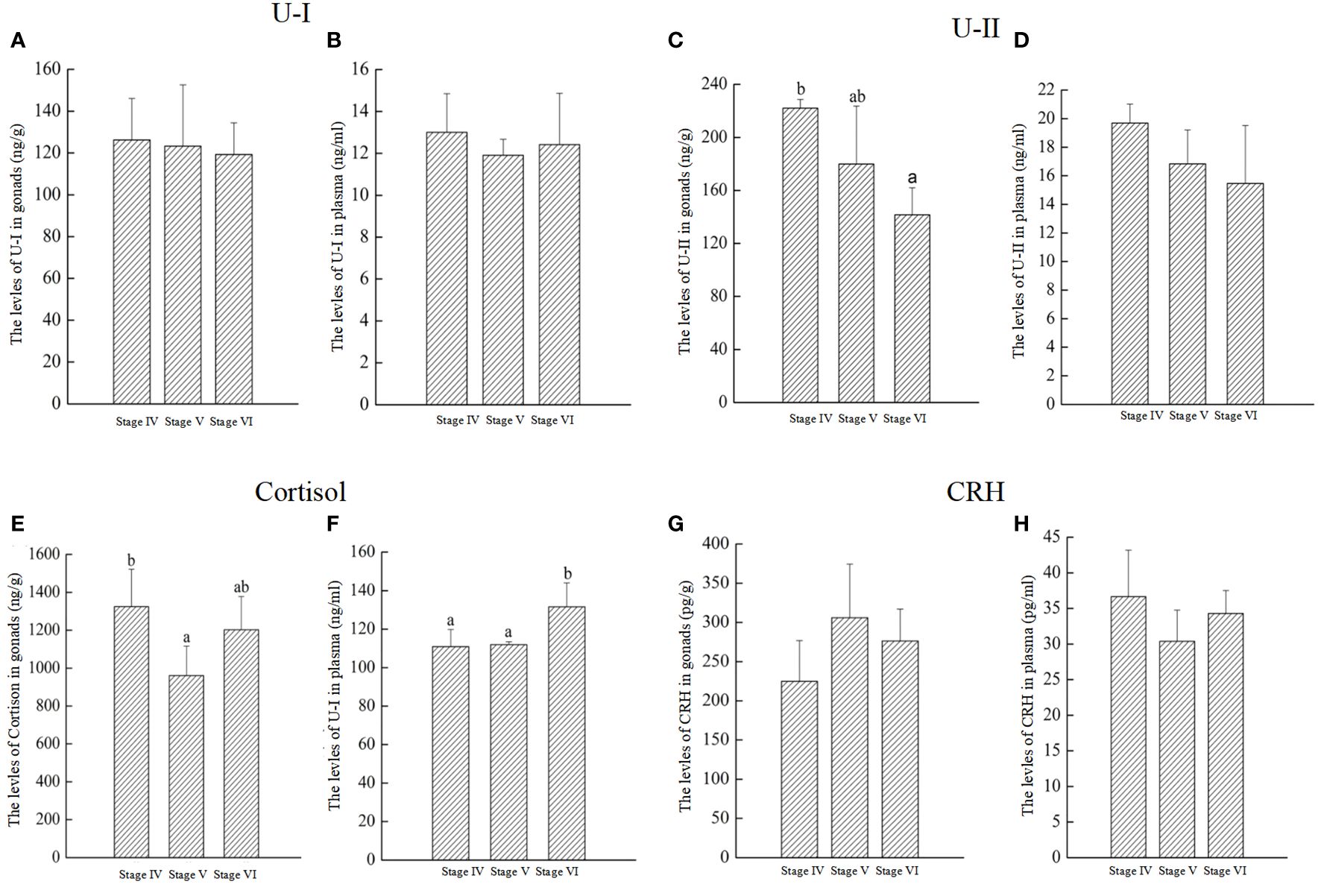
Figure 8 The levels of U-I, U-II, Cortisol, and CRH throughout reproductive process in P. argenteus. The U-I levelsin gonads (A) and plasma (B); The U-II levelsin gonads (C) and plasma (D), respectively; The cortisol levels in gonads (E) and plasma (F), respectively; The CRH levels in gonads (G) and plasma (H), respectively. n = 6 for each value. Superscript letters indicate one-way ANOVA, followed by Tukey’s test (P < 0.05).
4 Discussion
4.1 The two unique morphological forms of the neurohypophysis in P. argenteus
The neurohypophysis, a neuroendocrine gland exclusive to fish and integral to the Central Nervous System (CNS), plays a pivotal role in its functionality This gland, characterized as the ventral enlargement at the terminal end of the spinal cord, exhibits significant morphological diversity among fish species (Fridberg and Bern, 1968; Sun et al., 2013). Previous research has identified that in cartilaginous fish, the axonal nerve bundle-like terminals and capillaries of Dahlgren cells are closely associated yet do not coalesce into a distinct enlargement. Contrastingly, in certain teleost species, such as the Japanese eel (Anguilla japonica), northern pike (Esox lucius), and Pachycormus, the axonal terminals and capillaries within their tail spinal cord terminals aggregated, forming noticeable enlargements. Nonetheless, these structures maintain continuity with the spinal cord without significant morphological differentiation (Sano et al., 1962; Fridberg and Bern, 1968). Unique among these, Albula vulpes, demonstrated a distinct tail gland enlargement with a conspicuous narrowing at the transition from the tail gland to the spinal cord, coupled with a neural vascular aggregation zone, epitomizing a transitional state between aggregation and dispersion (Fridberg and Bern, 1968). In most teleost species, such as Neothynnus albacora (Sano et al., 1962), Platichthys flesus (Kriebel, 1980), and Rita rita (Sharma and Sharma, 1975), exhibited a markedly enlarged structure in the tail spinal cord, namely the tail gland (Imai et al., 1965). Our findings revealed a unique structural adaptation in P. argenteus, where a marked concavity in the last vertebra corresponds with a pronounced enlargement of the ventral side of the tail spinal cord, forming the neurohypophysis concealed within this concavity. Additionally, the size of the neurohypophysis varied significantly across species, with dimensions in the North American blueback herring averaging 1.0 mm × 1.3 mm × 0.5 mm (Richard et al., 1979), in the channel catfish approximately 1.5 mm in length and 1.0 mm in diameter (Kriebel, 1980), and in Nile tilapia (Oreochromis niloticus) around 4.0 mm in length and 0.3 mm in width (Cioni et al., 2000). In this study, the neurohypophysis in both morphologies of P. argenteus was found to be relatively smaller, a trait possibly attributed to the slender vertebral bones in their tail region. Taken together, the neurohypophysis of P. argenteus is notably smaller compared to that in most fish species, with the occurrence of fish exhibiting two distinct neurohypophysis morphologies being exceptionally rare.
4.2 Dahlgren cells were concentrated in the CNSS of P. argenteus
The neurosecretory systems of fish showcase a vast diversity, with the distribution of neurosecretory cells within the CNSS of the spinal cord varying markedly across species. In teleost, Dahlgren cells within the CNSS are notably concentrated within the spinal cord. Conversely, in cartilaginous fish, these cells are dispersed more broadly throughout the spinal cord. In A. vulpes, Dahlgren cells are primarily localized within the spinal cord adjacent to the last 6 vertebrae of the tail (Fridberg and Nishioka, 1966), whereas in Labeo rohita, they are spread throughout the spinal cord near the final 9 vertebrae (Fridberg and Bern, 1968). In Elasmobranchs, such as Raja batis, a wide distribution of Dahlgren cells were observed along the spinal cord corresponding to 55 vertebrae in the tail (Fridberg and Bern, 1968). In Poecilia sphenops, these cells were distributed in the spinal cord adjacent to the last 8 vertebrae (Kriebel, 1980), and in Pomolobus aestivalis, they were found near the last 6 or 8 vertebrae (Richard et al., 1979). The Japanese eel shows a distribution of Dahlgren cells in the spinal cord near the last 6 or 7 vertebrae. In Nile tilapia (Oreochromis niloticus), these cells were located in the spinal cord adjacent to the last 5 vertebrae of the tail (Fridberg and Nishioka, 1966). Typically, Dahlgren cells were absent at the terminal end of the spinal cord (cauda equina). However, in Ompak bimaculatus, Dahlgren cells were identified at the cauda equina (Sureshwar, 1973). Our findings indicated a more concentrated distribution of Dahlgren cells of P. argenteus, localized in the spinal cord near the last 6 vertebrae of the tail. Significantly, these cells were primarily concentrated around the final 3 vertebrae, with a less dense distribution from the 4th to the 6th last vertebrae.
4.3 Neuroendocrine changes in P. argenteus during breeding season
The neurosecretory system in fish, in conjunction with the hypothalamus-pituitary axis, plays a pivotal role in regulating various physiological functions, including reproduction. In Rita rita, it was observed that the contents of the Herring bodies in the CNNS were depleted throughout the reproductive process, leaving behind cavities. This depletion coincided with the dilation of blood vessel lumens, suggesting that the substances from the Herring bodies were released into the bloodstream and transported to target organs, where they executed their physiological roles (Sano and Knoop, 1959; Sharma and Sharma, 1975). In this study, prior to breeding season, Dahlgren cells in P. argenteus, were observed to be rich in organelles such as mitochondria, ribosomes, lysosomes, Golgi apparatus, and rough endoplasmic reticulum. Post-breeding season, there was a noticeable decrease in these organelles, accompanied by diminished mitochondrial cristae and the emergence of vacuolization, and a pattern also observed in Albula vulpes (Fridberg and Nishioka, 1966). Before breeding season, the lumens of blood vessels in P. argenteus were notably enlarged and smooth, enabling close contact with cell protrusions or axonal terminals filled with secretory granules. After breeding season, these lumens narrowed, endothelial cells exhibited increased wrinkling protruding into the lumen, and a decrease in the quantity of secretory granules in axonal terminals was observed, with occasional vacuolization. This phenomenon might be closely associated with the decrease in the diameter of Dahlgren cells following the reproductive season of P. argenteus. Additionally, the reproductive process in P. argenteus featured both neurosecretory granules and electron-transparent vesicles within the CNSS. Neurosecretory granules were dominant before breeding season, whereas electron-transparent vesicles became more prevalent afterward, likely serving as residues post-release of granule contents. These secretory granules were speculated to release their contents into the bloodstream (Sano and Knoop, 1959; Holmgren and Chapman, 1960). Previous studies suggested a close connection between the bone membrane and the tail gland, facilitating the release of neurosecretory granules into adjacent areas (Fridberg and Nishioka, 1966). Within the CNSS of P. argenteus, neurosecretory granules could reach axonal nerve terminals via axons. Following secretion release, residual electron-empty vesicles were formed. These secretions, upon interacting with endothelial cells of capillaries, were transported into the capillary lumen, exerting physiological effects pertinent to breeding season.
4.4 Changes in related hormones throughout breeding season
The CNSS is the major circulating source of U-I, U-II, and CRH in fish. U-I and CRH are known to regulate and integrate the neuroendocrine, autonomic, immune, and behavioral response of fish to stressors by affecting stress-related cortisol production (Yuan et al., 2020). U-II plays an important role in regulating the body-energy reserves and nutrients for reproduction and survival (Kiss et al., 2011; Chen et al., 2019). In this study, U-II levels were significantly decreased in gonads after breeding season. This may indicate that U-II is involved in reproduction. However, there were no significant difference in U-I and CRH levels during breeding season, which indicate that the U-I and CRH might not be related to the reproduction of P. argenteus. In the orange-spotted grouper (Epinephelus coioides), U-II mirrors the function of hCG, influencing the secretion of estradiol (E2) in ovarian tissue culture (Sun et al., 2013). Additionally, the expression of U-II in Paralichthys olivaceus is temperature-sensitive (Yuan et al., 2020, 2021; Wei et al., 2022). Although the primary function of cortisol during stress is to redistribute energy metabolically, enabling animals to cope with stressor challenges, its impact on reproduction is typically regarded as inhibitory (Faught and Vijayan, 2018). Stressor-mediated elevation of cortisol suppresses the functioning of the hypothalamus-pituitary-gonadal (HPG) axis, leading to reduced expression of gonadotropins in the pituitary and decreased gonad size (Lethimonier et al., 2002; Milla et al., 2009). In this study, the cortisol levels decreased from stage IV to stage V in gonad, and were significant increased from stage V to stage VI in gonad and plasma. This might indicate that the cortisol involved in the regulation of breeding of P. argenteus. In P. flesus, plasma cortisol levels peak during breeding season, reflecting a dynamic interplay with the gonadosomatic index alongside the mRNA expression levels of U-I, U-II, and CRH (Lu et al., 2007; Jiang et al., 2021). In summary, the concentrations of U-I, U-II, cortisol, and CRH in P. argenteus are intimately linked to gonadal development, highlighting the CNSS’s instrumental function in facilitating seasonal adaptation and the reproductive process.
Data availability statement
The raw data supporting the conclusions of this article will be made available by the authors, without undue reservation.
Ethics statement
All fish experiments were conducted in accordance with the recommendations of the National Institutes of Health Guide for the Care and Use of Laboratory Animals. The Animal Care and Use Committee of Ningbo University approved the protocols. The study was conducted in accordance with the local legislation and institutional requirements.
Author contributions
CG: Conceptualization, Data curation, Formal analysis, Funding acquisition, Investigation, Methodology, Project administration, Resources, Software, Supervision, Validation, Visualization, Writing – original draft, Writing – review & editing. XZ: Conceptualization, Data curation, Formal analysis, Funding acquisition, Investigation, Methodology, Project administration, Resources, Software, Supervision, Validation, Visualization, Writing – original draft. HS: Conceptualization, Data curation, Formal analysis, Funding acquisition, Investigation, Methodology, Project administration, Resources, Software, Supervision, Validation, Visualization, Writing – original draft. XW: Conceptualization, Data curation, Formal analysis, Funding acquisition, Investigation, Methodology, Project administration, Resources, Software, Supervision, Validation, Visualization, Writing – review & editing. SX: Conceptualization, Data curation, Formal analysis, Funding acquisition, Investigation, Methodology, Project administration, Resources, Software, Supervision, Validation, Visualization, Writing – review & editing. DW: Conceptualization, Data curation, Formal analysis, Funding acquisition, Investigation, Methodology, Project administration, Resources, Software, Supervision, Validation, Visualization, Writing – original draft, Writing – review & editing.
Funding
The author(s) declare financial support was received for the research, authorship, and/or publication of this article. This research was funded by grants from Ningbo University Talent Introduction Research Initiation Fund Project (Natural Science Category), the National Natural Science Foundation of China (42306114), and KC Wong Magna Fund in Ningbo University.
Conflict of interest
The authors declare that the research was conducted in the absence of any commercial or financial relationships that could be construed as a potential conflict of interest.
Publisher’s note
All claims expressed in this article are solely those of the authors and do not necessarily represent those of their affiliated organizations, or those of the publisher, the editors and the reviewers. Any product that may be evaluated in this article, or claim that may be made by its manufacturer, is not guaranteed or endorsed by the publisher.
References
Archangi B., Bazrafshan K. H., Ronagh M. T., Savari A., Abadi M. S. A. (2013). Population genetic structure of Silver pomfret (Pampus argenteus) in Persian Gulf and Oman Sea, inferred from 11 microsatellite loci. World J. Fish Mar. Sci. 5, 227–232. doi: 10.5829/idosi.wjfms.2013.05.02.7148
Audet C., Chevalier G. (1981). Monaminergic innervation of the caudal neurosecretory system of the brook trout Salvelinus fontinalis in relation to osmotic stimulation. Gen. Comp. Endocrinol. 45, 189–203. doi: 10.1016/0016-6480(81)90104-0
Berlind A. (1972). Teleost caudal neurosecretory system: sperm duct contraction induced by urophysial material. J. Endocrinol. 52, 567–574. doi: 10.1677/joe.0.0520567
Campbell P. M., Pottinger T. G., Sumpter J. P. (1992). Stress reduces the quality of gametes produced by rainbow trout. Biol. Reprod. 47, 1140–1150. doi: 10.1095/biolreprod47.6.1140
Campbell P. M., Pottinger T. G., Sumpter J. P. (1994). Preliminary evidence that chronic confinement stress reduces the quality of gametes produced by brown and rainbow trout. Aquaculture 120, 151–169. doi: 10.1016/0044-8486(94)90230-5
Chen H., Mu R. (2008). Seasonal morphological and biochemical changes of Dahlgren cells implies a potential role of the caudal neurosecretory system (CNSS) in the reproduction cycle of teleostean fish. Fish Physiol. Biochem. 34, 37–42. doi: 10.1007/s10695-007-9143-8
Chen X., Yin L., Jia W. H., Wang N. Q., Xu C. Y., Hou B. Y., et al. (2019). Chronic urotensin-II administration improves whole-body glucose tolerance in high-fat diet-fed mice. Front. Endocrinol. 10. doi: 10.3389/fendo.2019.00453
Cioni C., De Vito L., Greco A., Pepe A. (1998). The caudal neurosecretory system and its afferent synapses in the goldfish, Carassius auratus: morphology, immunohistochemistry, and fine structure. J. Morphol. 235, 59–76. doi: 10.1002/(SICI)1097-4687(199801)235:1<59::AID-JMOR5>3.0.CO;2-J
Cioni C., Francia N., Greco A., De Vito L., Bordieri L., Crosetti D. (2000). Development of the caudal neurosecretory system of the nile tilapia Oreochromis niloticus: an immunohistochemical and electron microscopic study. J. Morphol. 243, 209–218. doi: 10.1002/(SICI)1097-4687(200002)243:2<209::AID-JMOR9>3.0.CO;2-J
Contreras-Sanchez W., Schreck C., Fitzpatrick M., Pereira C. (1998). Effects of stress on the reproductive performance of rainbow trout (Oncorhynchus mykiss). Biol. Reprod. 58, 439–447. doi: 10.1095/biolreprod58.2.439
Faught E., Vijayan M. (2018). Maternal stress and fish reproduction: The role of cortisol revisited. Fish. Fish. 19, 1016–1030. doi: 10.1111/faf.12309
Fridberg G., Bern H. A. (1968). The urophysis and the caudal neurosecretory system of fishes. Biol. Rev. Camb. Philos. Soc 43, 175–199. doi: 10.1111/j.1469-185x.1968.tb00958.x
Fridberg G., Nishioka R. S. (1966). The caudal neurosecretory system of the isospondylous teleost, Albula vulpes, from different habitats. Gen. Comp. Endocrinol. 6, 195–212. doi: 10.1016/S0016-6480(66)80009-6
Guo C., Zhang K., Li C., Xing R., Xu S., Wang D., et al. (2024). Cyp19a1a promotes ovarian maturation through regulating E2 synthesis with estrogen receptor 2a in Pampus argenteus (Euphrasen 1788). Int. J. Mol. Sci. 25 (3), 1583. doi: 10.3390/ijms25031583
Holmgren U., Chapman G. B. (1960). The fine structure of the urophysis spinalis of the teleost fish, Fundulus heteroclitus L. J. Ultrastruct Res. 4, 15–25. doi: 10.1016/s0022-5320(60)80003-2
Imai K., Stanley J. G., Fleming W. R., Bern H. A. (1965). On the suggested ionoregulatory role of the teleost caudal neurosecretory system. Proc. Soc Exp. Biol. Med. 118, 1102–1106. doi: 10.3181/00379727-118-30055
Imanaga Y., Nyuji M., Amano M., Takahashi A., Kitano H., Yamaguchi A., et al. (2014). Characterization of gonadotropin-releasing hormone and gonadotropin in jack mackerel (Trachurus japonicus): Comparative gene expression analysis with respect to reproductive dysfunction in captive and wild fish. Aquaculture 428–429, 226–235. doi: 10.1016/j.aquaculture.2014.03.003
Jiang P., Pan X., Zhang W., Dai Z., Lu W. (2021). Neuromodulatory effects of GnRH on the caudal neurosecretory Dahlgren cells in female olive flounder. Gen. Comp. Endocrinol. 307, 113754. doi: 10.1016/j.ygcen.2021.113754
Kiss R. S., You Z., Genest J. J., Behm D. J., Giaid A. (2011). Urotensin II differentially regulates macrophage and hepatic cholesterol homeostasis. Peptides 32, 956–963. doi: 10.1016/j.peptides.2011.02.016
Kriebel R. M. (1980). The caudal neurosecretory system of Poecilia sphenops (Poeciliidae). J. Morphol. 165, 157–165. doi: 10.1002/jmor.1051650204
Lethimonier C., Tujague M., Kern L., Ducouret B. (2002). Peptide insertion in the DNA-binding domain of fish glucocorticoid receptor is encoded by an additional exon and confers particular functional properties. Mol. Cell. Endocrinol. 194, 107–116. doi: 10.1016/S0303-7207(02)00181-8
Lu W., Dow L., Gumusgoz S., Brierley M. J., Warne J. M., McCrohan C. R., et al. (2004). Coexpression of corticotropin-releasing hormone and urotensin I precursor genes in the caudal neurosecretory system of the euryhaline flounder (Platichthys flesus): a possible shared role in peripheral regulation. Endocrinology 145, 5786–5797. doi: 10.1210/en.2004-0144
Lu W., Greenwood M., Dow L., Yuill J., Worthington J., Brierley M. J., et al. (2006). Molecular characterization and expression of urotensin II and its receptor in the flounder (Platichthys flesus): a hormone system supporting body fluid homeostasis in euryhaline fish. Endocrinology 147, 3692–3708. doi: 10.1210/en.2005-1457
Lu W., Liu S., Zhong Y. (2012). Research progress on the caudal neurosecretory system of fish. J. Shanghai Ocean Univ. 21, 1743–1749. doi: 10.1007/s11783-011-0280-z
Lu W., Worthington J., Riccardi D., Balment R. J., McCrohan C. R. (2007). Seasonal changes in peptide, receptor and ion channel mRNA expression in the caudal neurosecretory system of the European flounder (Platichthys flesus). Gen. Comp. Endocrinol. 153, 262–272. doi: 10.1016/j.ygcen.2007.05.004
McCrohan C. R., Lu W., Brierley M. J., Dow L., Balment R. J. (2007). Fish caudal neurosecretory system: a model for the study of neuroendocrine secretion. Gen. Comp. Endocrinol. 153, 243–250. doi: 10.1016/j.ygcen.2006.12.027
Mileva V. R., Gilmour K. M., Balshine S. (2011). Effects of maternal stress on egg characteristics in a cooperatively breeding fish. Comp. Biochem. Physiol. A Mol. Integr. Physiol. 158, 22–29. doi: 10.1016/j.cbpa.2010.08.017
Milla S., Wang N., Mandiki S. N. M., Kestemont P. (2009). Corticosteroids: Friends or foes of teleost fish reproduction? Comp. Biochem. Phys. A. 153, 242–251. doi: 10.1016/j.cbpa.2009.02.027
Owada K., Kawata M., Akaji K., Takagi A., Moriga M., Kobayashi H. (1985). Urotensin II-immunoreactive neurons in the caudal neurosecretory system of freshwater and seawater fish. Cell Tissue Res. 239, 349–354. doi: 10.1007/BF00218014
Richard M. K., Burke J. D., Meetz G. D. (1979). Morphologic features of the caudal neurosecretory system in the blueback herring, Pomolobus aestivalis. Anat. Rec. 195, 553–572. doi: 10.1002/ar.1091950314
Sano Y., Hamana K., Kawamato M. (1962). On the segmental formation of the neurophysis spinalis caudalis in various fish species. Z. Zellforsch. Mikrosk. Anat. 57, 881–887. doi: 10.1007/BF00332468
Sano Y. A., Knoop (1959). Elektronenmikroskopische untersuchungen am Kaudalen neurosekretorischen system von Tinca vulgaris. Z. Zellforsch. 49, 464–492. doi: 10.1007/BF00320055
Sharma S., Sharma A. (1975). A note on the cavdal neurosecretory system and seasonal changes observed in the urophysis of Rita rita (Blecker). Can. J. Zool. 53, 357–360. doi: 10.1139/z75-045
Sun C., Duan D., Li B., Qin C., Jia J., Wang B., et al. (2013). UII and UT in grouper: cloning and effects on the transcription of hormones related to growth control. J. Endocrinol. 220, 35–48. doi: 10.1530/JOE-13-0282
Van Der Kraak G. J., Munkittrick K. R., McMaster M. E., Portt C. B., Chang J. P. (1992). Exposure to bleached kraft pulp mill effluent disrupts the pituitary-gonadal axis of white sucker at multiple sites. Toxicol. Appl. Pharmacol. 115, 224–233. doi: 10.1016/0041-008x(92)90327-o
Wei Y., Zhang X., Yuan H., Lu W. (2022). The individuality affects the hormone secretion of the caudal neurosecretory system (CNSS) and correlation with respiratory metabolism, spontaneous activity and appetite in olive flounder (Paralichthys olivaceus). Aquaculture 548, 737684. doi: 10.1016/j.aquaculture.2021.737684
Winter M. J., Ashworth A., Bond H., Brierley M. J., McCrohan C. R., Balment R. J. (2000). The caudal neurosecretory system: control and function of a novel neuroendocrine system in fish. Biochem. Cell Biol. 78, 193–203. doi: 10.1111/jne.12876
Yuan M. Z., Li X. X., Lu W. Q. (2020). The caudal neurosecretory system: A novel thermosensitive tissue and its signal pathway in olive flounder (Paralichthys olivaceus). J. Neuroendocrinol. 32, e12876. doi: 10.1111/jne.12876
Yuan M. Z., Zhang X., Louro B., Li X., Canário A. V. M., Lu W. (2021). Transcriptomics reveals that the caudal neurosecretory system in the olive flounder (Paralichthys olivaceus) is more responsive in bold individuals and to chronic temperature change. Aquaculture 544, 737032. doi: 10.1016/j.aquaculture.2021.737032
Keywords: CNSS, Dahlgren cell, neurohypophysis, urotensin, cortisol, breeding season, fish
Citation: Guo C, Zhang X, Song H, Wang X, Xu S and Wang D (2024) The distinctive morphology of caudal neurosecretory system and its impact on Pampus argenteus throughout the breeding season. Front. Mar. Sci. 11:1358901. doi: 10.3389/fmars.2024.1358901
Received: 20 December 2023; Accepted: 28 March 2024;
Published: 11 April 2024.
Edited by:
Ana M. Faria, MARE-ISPA, PortugalReviewed by:
Jinxiang Liu, Ocean University of China, ChinaShiming Peng, Chinese Academy of Fishery Sciences, China
Copyright © 2024 Guo, Zhang, Song, Wang, Xu and Wang. This is an open-access article distributed under the terms of the Creative Commons Attribution License (CC BY). The use, distribution or reproduction in other forums is permitted, provided the original author(s) and the copyright owner(s) are credited and that the original publication in this journal is cited, in accordance with accepted academic practice. No use, distribution or reproduction is permitted which does not comply with these terms.
*Correspondence: Danli Wang, d2FuZ2RhbmxpQG5idS5lZHUuY24=