- 1Delft University of Technology, Civil Engineering and Geosciences, Delft, Netherlands
- 2Van Oord Dredging and Marine Contractors, Engineering & Estimating, Rotterdam, Netherlands
The marine environment faces continuous anthropogenic pressures, including infrastructural developments at a global scale. Integration of nature-inclusive measures in the design of infrastructural development is increasingly encouraged, but a lack of coordination results in fragmentation of project-based measures, failing to meet the desired overall effects. To realize impact at system-scale, i.e. the seascape dimension required to achieve the set objective for a selected ecosystem component, overarching policies with shared targets towards effective nature-inclusive marine infrastructure are needed. We present a stepwise approach to work towards operational objectives for promoting selected ecosystem components that can be species, habitats or ecosystem processes, in which ruling policies, environmental conditions and the use of infrastructural development are aligned, and agreement on achievable ambitions is reached. Having clear targets will provide guidance to project developers in designing the infrastructure nature-inclusive, and in setting up relevant monitoring programs to evaluate the measures taken. We demonstrate how this stepwise approach could be applied to derive operational objectives for the design of nature-inclusive marine infrastructure in the context of offshore windfarm development in the North Sea, currently one of the most prominent infrastructure developments that changes the marine environment drastically. The European flat oyster Ostrea edulis has been selected as target species in the case study, as its once abundant population is now nearly extinct from the North Sea due to human disturbances, and there’s growing interest to restore its reefs. The application of the stepwise approach indicates the potential for oyster reef restoration in the area, based upon a clear match between ruling policy, environmental conditions, and habitat suitability within offshore wind farms. An agreement between the main stakeholders on achievable ambitions can likely be established and would translate into the operational objective to actively introduce oysters to reach an initial critical mass and optimize settlement habitat in all future offshore wind farms in an area with suitable habitat characteristics. Such an agreement on overarching objectives is crucial to align separate initiatives to promote targeted ecosystem components and to jointly become most effective, which is ultimately in the best interest of the larger community using the system.
1 Introduction
Rapid changes in the marine environment are taking place, driven by human usages and climate change (Halpern et al., 2019; Smale et al., 2019). One of the most extreme human modifications to global seascapes is the extent of marine construction (Bugnot et al., 2020). Marine infrastructure comes in many forms and covers functionalities for multiple usages, including recreation, residency, fisheries, coastal defense, and offshore energy installations (Dafforn et al., 2015b). It is primarily designed to meet engineering and financial criteria, without considering its value as habitat (Browne and Chapman, 2011; Laboyrie et al., 2018). Marine infrastructure modifies seascapes by replacing natural habitats and changing environmental conditions critical to habitat persistence (Bishop et al., 2017; Bugnot et al., 2020). While these effects are primarily viewed as negative, marine infrastructure can also be designed to incorporate ecological principles that benefit marine life (Dafforn et al., 2015b; Laboyrie et al., 2018). This so-called ‘nature enhancement’ and derivatives thereof are prone to broad interpretation. To avoid ambiguity, we refer primarily to ‘nature-inclusive marine infrastructure’, which we define as marine infrastructure designed to improve the condition of targeted components of the ecosystem during its operational lifetime. These components would be selected species, habitats or ecosystem processes, and improvement refers to comparison with their condition prior to the infrastructural development. In recent years, a wide variety of nature-inclusive designs were applied to real projects (e.g. O’Shaughnessy et al., 2020 for review). However, the fragmented character of individual measures has so far not led to significant impact at system-scale (Abelson et al., 2020; Duarte et al., 2020), by which we refer to a seascape of the dimension required to achieve a set objective for the targeted ecosystem component. The variety in measures applied in individual projects could partly be due to underlying competitive differences between the developers. Without shared objectives, parallel efforts to include nature-inclusive elements in the design of marine infrastructure might not lead to a desired overall effect, and could even interfere with each other. To achieve a significant system-scale effect, individual initiatives to promote selected ecosystem components should be defragmented into a coordinated system-wide approach, following shared objectives. The process of setting those objectives for different systems involving different usages, would benefit from a generic stepwise approach to do so.
When defining objectives for ecological values as part of infrastructural development in the marine environment, one should aim to limit the negative environmental impact, and try to stimulate positive impact with the usage function. Such potential impact on the environment of marine infrastructural development is generally evaluated through an Environmental Impact Assessment (EIA) (e.g. Carroll et al., 2020). In practice, as most infrastructural designs are optimized for their economic and technical objectives, the EIA process subsequently applies mitigation measures to reduce any significant negative effects identified. But a recent development is that an EIA also addresses the potential of a project to have beneficial effects to the environment, both natural and socio-economic (Laboyrie et al., 2018). Furthermore, priority should be given to the implementation of monitoring programs, in order to be able to assess the long-term effects of newly build infrastructure (Dafforn et al., 2015b). In the end, it is up to authorities in close cooperation with the scientific community and other stakeholders, to determine an approach for implementing the environmental goals and policy objectives for the infrastructural development within the system.
A well-established tool to structurally align policy objectives with technical solutions to meet these objectives is the ‘Frame of Reference’ approach (Van Koningsveld, 2003). It cyclically defines both a strategic and an operational objective and operationalizes these objectives in a 4-step decision recipe determining (i) a quantitative state concept, (ii) a bench marking procedure, (iii) an intervention procedure and (iv) an evaluation procedure (see Figure 1). Originally derived to evaluate and re-define a sustainable coastal policy for the Netherlands (van Koningsveld and Mulder, 2004), the ‘Frame of Reference’ approach has since then been applied successfully for a range of civil engineering disciplines. For example, it was used to define coastal management policies for beach areas (Jiménez et al., 2007; Gault et al., 2011; Sutherland and Thomas, 2011), to develop environmental monitoring schemes for offshore renewable energy projects (Garel et al., 2014), and proposed as a tool to assess the sustainability of for example dredging (Laboyrie et al., 2018) and port and waterway projects (Van Koningsveld et al., 2023).
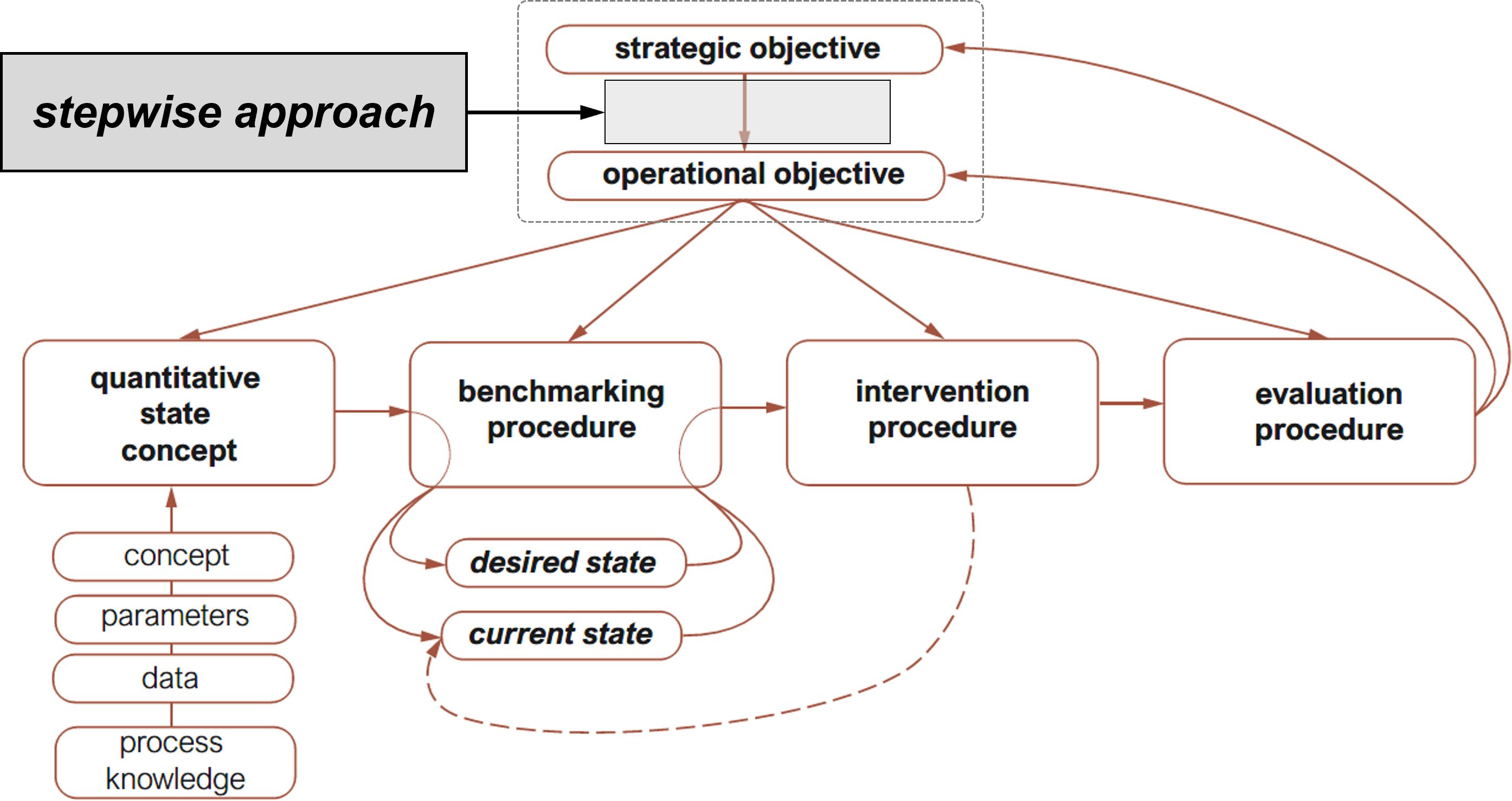
Figure 1 The basic Frame of Reference for policy development by Van Koningsveld (2003). The grey rectangle indicates the fit of the stepwise approach for alignment towards operational objectives. (Figure after Van Koningsveld et al., 2023).
A key element of establishing a coordinated, system-wide approach for implementing nature-inclusive elements within infrastructural development, is to define the strategic and operational objectives and to break these down into a number of logical elements (De Vries et al., 2020b). Strategic objectives provide the long-term context for a policy, express the vision for a system and its usage, and tend to change slowly (Van Koningsveld et al., 2005). Operational objectives are the concrete implementation of strategic objectives, by expressing how to handle the system and its usage, and include and explicit indication of the spatial and temporal scales involved (Van Koningsveld et al., 2005). Turning strategic objectives into operational ones, as also shown in the ‘Frame of Reference’ methodology, is a crucial though complicated process. It would benefit from a generic approach that is applicable for different systems involving different usages. Such an approach should include standards for defining the objectives as well as for implementing targets to achieve them, both temporarily and spatially. Although the need to specify clear operational objectives in coastal and marine management is generally recognized (e.g. Van Koningsveld, 2003; Cormier et al., 2017; De Vries et al., 2020a), a methodology for facilitating the process of turning strategic objectives into operational ones has not yet been described. This paper is the first in its kind to address an approach to set effective operational objectives for promoting targeted components of the subtidal ecosystem, i.e. the environment below the surface of the sea, in areas designated for infrastructural development. It entails a structured methodology, that aligns the ruling socio-economical and environmental conditions of the system with the potential offered by nature-inclusive marine infrastructure to achieve long-term benefits for selected ecosystem components. This stepwise approach is demonstrated by setting operational objectives for the nature-inclusive design of offshore windfarms the Dutch part of the North Sea. We selected offshore wind farms as it is currently one of the most prominent infrastructure developments that severely changes the marine environment (Ter Hofstede et al., 2023a). This rapid development in renewable energy production has been attributed to the goals set in the Paris Agreement (UNFCCC, 2018), aiming at the reduction of CO2 emissions, and by several of the Sustainable Development Goals of the “2030 UN Agenda for Sustainable Development” (UN, 2015) (Danovaro et al., 2024). We selected the Dutch part of the North Sea as incorporation of nature-inclusive measures in offshore wind farms is highly encouraged by the Dutch government (Ministry of Economic Affairs, 2018). A key driver behind this encouragement is commitment to European policies such as the Green Deal, stating that the development of economic activities should “Do No Significant Harm” to the EU environmental objectives (European Commission, 2019), and the Marine Strategy Framework Directive (MSFD) targeting Good Environmental Status (GES) of the EU marine ecosystems (European Commission, 2008). However, current initiatives for implementation of nature-inclusive design measures in offshore wind farms in the Dutch part of the North Sea are yet uncoordinated and likely not meeting their full potential. Therefore, clear objectives are needed to ensure that the condition of targeted ecosystem components is at least maintained to meet the existing policies, or can even be improved effectively through interventions taken along with the development of offshore wind farms.
2 Approach for alignment towards operational objectives
When considering the design of nature-inclusive marine infrastructure, one should first identify the strategic objectives for the ecosystem in which the development is planned, and then define operational objectives to achieve desired environmental targets. Although the inherent dynamic variability of ecosystems makes it difficult to design marine infrastructure such that it contributes to an improved condition of certain targeted components, setting operational objectives is fundamental to enable the implementation of nature-inclusive design measures (De Vries et al., 2020a). This applies for individual infrastructure projects, but even more so for the combined effect of multiple interventions on a system-scale. Addressing large-scale issues will reveal the true impact of measures and support their well-considered selection and implementation to enable their full potential (De Vries et al., 2020b). However, current practices focus too little on their collective impact to reach system-scale effects. On the contrary, current designs of nature-inclusive marine infrastructure still result in an uncoordinated sprawl of individual measures that each may be effective to achieve their individual project objectives, but collectively don’t contribute to the system-scale objective to achieve the desired impact for the targeted ecosystem component (De Vries et al., 2020b). To mitigate this shortfall, we present a stepwise approach for alignment of the nature-inclusive designs of marine infrastructure that is to be developed in a system, to support setting operational objectives for making an impact at system-scale (see Figure 2). The approach starts with assessing three fundamental elements of the system that are to be aligned: Policy assessment (I), identifying and prioritizing the objectives of existing and future policies and legislation towards nature; Environmental assessment (II), identifying and prioritizing the potential of the environmental conditions for improving ecosystem components; and Infrastructural assessment (III), identifying and prioritizing the potential of nature-inclusive marine infrastructure, including defining design modifications. Next, matchmaking (IV) has to be done between ruling policy, environmental conditions, and infrastructural potential, to determine whether the identified measures don’t conflict with each other, preferably even have mutual positive effects, and to achieve a set objective for the targeted ecosystem component, which we defined as system-scale impact. Finally, an achievable agreed ambition (V) between the relevant stakeholders for implementation of potential measures is needed, for which operational objectives can be defined.
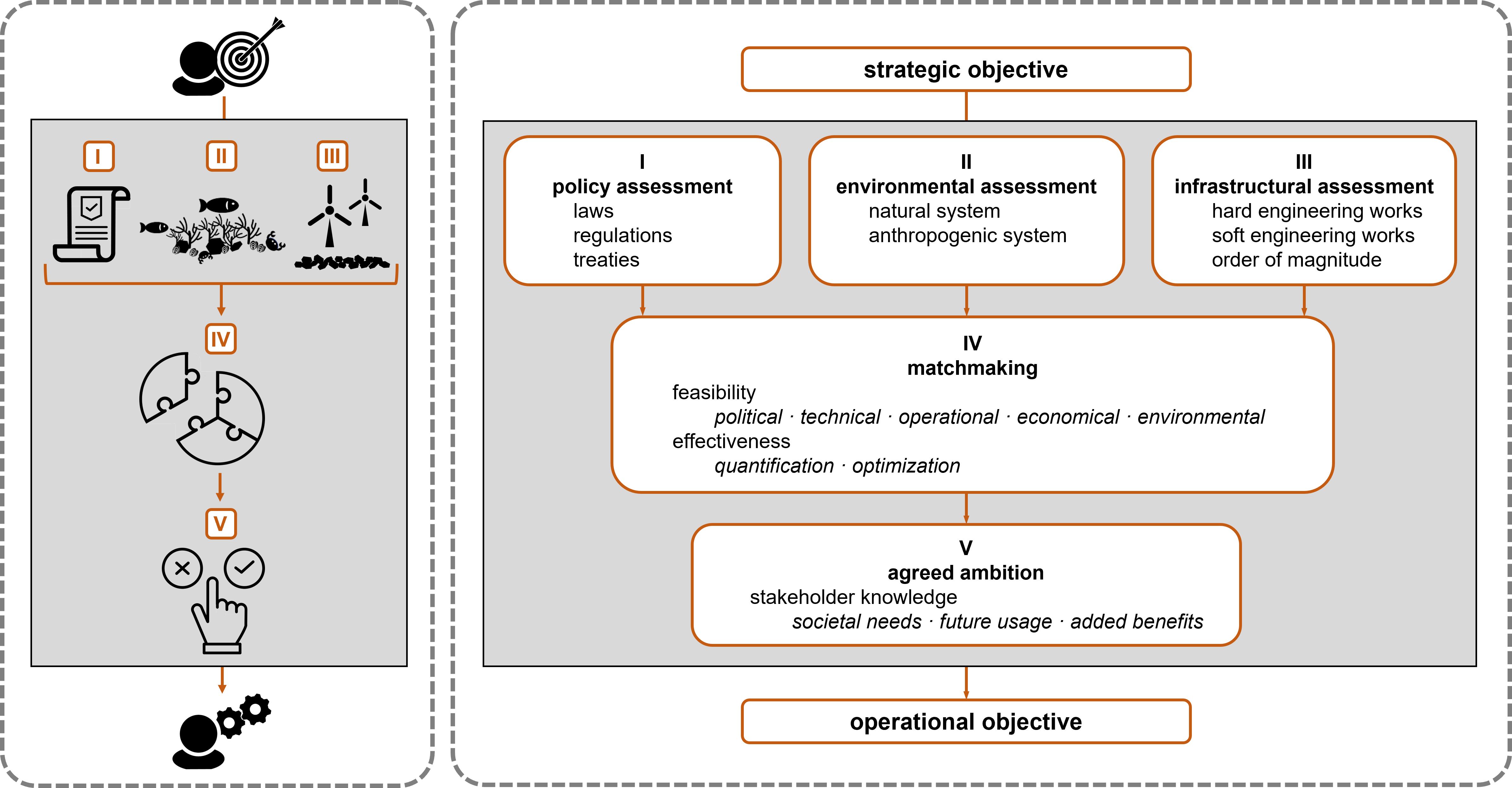
Figure 2 Stepwise approach for alignment towards operational objectives for designing nature-inclusive marine infrastructure.
2.1 Step I - policy assessment
Policies adhere to (inter)national laws, regulations and treaties, and implementing nature-inclusive elements in infrastructural designs is subject to legislative frameworks and associated permitting processes. Sometimes these processes are complex and uncoordinated, thereby impeding the implementation of nature-inclusive design measures in infrastructural development projects (Shumway et al., 2021). For instance, legislation may require specific requirements of constructions being built using certain materials, inhibiting the use of nature-inclusive elements (Dhakal and Chevalier, 2017). Another example is the observation that local legislation in the Netherlands does not allow sand borrow pits to exceed 2 m in depth, though it has been observed that creating a seabed with deep pits of 20 m during sand extraction would increase benthic biodiversity (De Jong et al., 2015). On the contrary, policy and legislation can also enable incentives for the implementation of nature-inclusive infrastructural development, when policy makers adhere to these, such as the United Nations Decade of Ocean Science for Sustainable Development (2021–2030), the United Nations Decade of Ecosystem Restoration (2021–2030), and the European Green Deal (Abelson et al., 2020). The EU Floods Directive, for example, has inspired at least 26 EU member states to include nature-based solutions in their water retention plans (Gerritsen et al., 2021). Another example, but at a local level, exists in Maryland (USA) where living shorelines are promoted by the Living Shoreline Protection act from 2008, stating that by default natural and nature-based infrastructure should be used for shoreline protection, unless a property owner can demonstrate the need to put in a built feature (Sutton-Grier et al., 2018). Depending on the ruling authority, policies vary in extent from local to international seascapes, which should be recognized when defining feasible objectives for nature-inclusive marine infrastructure.
2.2 Step II - environmental assessment
For nature-inclusive marine infrastructure to achieve its full potential at the system-scale, a thorough understanding of the functioning of that system is required. It is important to consider both the historic and present situation (Ter Hofstede et al., 2023a), but also future site conditions given current projections of global climate change and changes in ecosystem services (Suding, 2011; Howie and Bishop, 2021). The local environment contains both the natural system, which includes abiotic as well as biotic components, and the anthropogenic system. For the description of the conditions of a system is it advisable to adhere to those provided in a standard procedure as commonly used for an Environmental and Social Impact Assessment (Laboyrie et al., 2018), such as to the one mandatory in the European Union, the EU’s Environmental Impact Assessment Directive (European Commission, 2014), or to principles practiced more globally and provided by the International Association for Impact Assessment (www.iaia.org/best-practice.php). An impact assessment generally covers basic variables of the physical environment (e.g. geology, meteorology, hydrology, water and air quality, etc.), biological environment (e.g. fish and benthic communities, marine megafauna, birds, etc.), the anthropogenic environment (e.g. fisheries and aquaculture, socio-economic profile, traffic and navigation, cultural and archeological heritage, etc.) (Laboyrie et al., 2018).
Essential for nature-inclusive design of marine infrastructure is to clearly define which components of the ecosystem are to be targeted. One could for example strive for establishing more biodiversity, or for promoting threatened species or habitats. Consensus on the nature-inclusivity target allows for the selection of measures to be incorporated in the design of the foreseen marine infrastructure, and the determination of the system-scale required to achieve the target. Sometimes a specific species is considered to represent a range of co-occurring species, which are assumed to co-develop similarly as that species (Fleishman et al., 2000; Lengkeek et al., 2017). Selecting such a so-called umbrella species as target for nature-inclusive design can be favored, as focusing on one species eases the design process of measures, while the effect of the measures is assumed to benefit a range of species. Also, when monitoring the effect of the measures, it is often more cost-effective to only sample one species than an entire assembly (Fleishman et al., 2000). However, it is always preferred to monitor the impact of a rigorous intervention such as the construction of marine infrastructure on all abiotic and biotic components of the system, in order to determine whether the desired effect has been achieved and side-effects have occurred.
2.3 Step III - infrastructural assessment
Man-made marine infrastructure such as dredged channels, breakwaters, sea-walls and scour protection can provide important habitat for marine organisms to spawn, to nurse, to forage, or to find shelter (Dafforn et al., 2015b; Ter Hofstede et al, 2023b). There is a vast potential to include elements that can benefit selected ecosystem components in the design of the infrastructure. The generally long-term lifetime of marine infrastructure allows the associated marine life at and around it to develop, and designs can be optimized to target desired species, habitats or processes (Ter Hofstede et al., 2023b). Marine infrastructure can be categorized under hard engineering works and soft engineering works. Hard engineering comprises marine infrastructural development using hard structures, including rubble mound structures (e.g. breakwaters), gravity-based structures (e.g. seawalls), pile foundations (e.g. offshore platforms) and floating structures (e.g. offshore wind turbines). Soft engineering involves human control on natural processes primarily through dredging works, including for example beach nourishment, salt marsh creation and capital dredging of channels. Within both categories, conventional engineering solutions can already benefit marine ecosystem components, and optimizations in the design can further increase these benefits.
In order to the determine the potential of using infrastructure development for promoting ecosystem components, one should first identify which design options are available and could function in the system. Second, one should consider design optimizations (Ter Hofstede et al., 2023b). It is recognized that marine construction works first serve human needs, not nature goals, but optimizing the infrastructure does provide an opportunity to benefit ecological values at system-scale. This should never be used as excuse to ignore or down-play the negative impact that infrastructural developments may have on a marine system (Firth et al., 2020). However, marine construction works can be synergized with the functioning of the ecosystem in which they are build much better than is currently practiced, and one should always strive for nature-inclusive features in their designs (Pioch et al., 2018; Ter Hofstede et al., 2023b).
2.3.1 Hard engineering works
The hard substrate used in marine infrastructure is known to act as artificial reef substrate (e.g. Bishop et al., 2017; Coolen et al., 2020; Degraer et al., 2020), though the associated communities are often observed to be less diverse and abundant than natural assemblages and nonindigenous species due to their low surface complexity and non-natural materials (Glasby et al., 2007; Gittman et al., 2016). Hard engineering works can be adjusted to increase the habitat complexity by bringing in more variety in use of materials and their texture, shape and dimensions, which is expected to result in a higher biodiversity (e.g. Dafforn et al., 2015a; Pioch et al., 2018; Strain et al., 2018). For example, the use of calcareous rock such as limestone or marble will trigger increased settlement by shellfish (Hidu et al., 1975; Soniat et al., 1991). If concrete is used as a construction material, it can be enriched with calcium carbonate, making it potentially a more preferable settlement substrate for shellfish larvae (Cuadrado-Rica et al., 2016; Potet et al.). The texture of concrete can also be roughened to mimic natural rock to promote the colonization by pioneering species (Moschella et al., 2005; Potet et al., 2021). The downside of using concrete is its toxicity as the cement mortars often leach trace metals over time (Hillier et al., 1999; Wilding and Sayer, 2002), which can be reduced by using nature-friendly adhesives in the mortar (Perkol-Finkel and Sella, 2014). Irregular extensions of infrastructure in both vertically and horizontally directions will increase surface area and provide leesides for marine organisms to shelter (Firth et al., 2014; Consoli et al., 2018; Ter Hofstede et al., 2023a). Narrowing down the rock grading in rubble mound structures will results in more crevices, and variation in rock size at different locations will increase habitat diversity, serving a wide range of rock-dwelling species (Ter Hofstede et al., 2022). All such measures can be incorporated into the design of nature-inclusive marine infrastructure.
2.3.2 Soft engineering works
Soft engineering works may also positively affect marine species (e.g. Todd et al., 2014). For instance, dredged channels were observed to be favored over other habitat types by dolphins as the structural features aid to trap prey (Allen et al., 2001), and beach nourishments can be used to restore or create nesting habitats for shorebirds and turtles (Jones & Mangun, 2001). The potential optimization of soft engineering works to achieve benefits for the ecosystem lies particularly in the contours created in the seabed. Leaving borrow areas with steep sand ridges and deep pits after sand extraction, leads to a decrease in bed shear stress and settlement of fine sediment and organic matter. This diversity in bedform can result in a 10- to 20-fold higher biomass of benthic and demersal organisms than would be the case with a plane seabed (De Jong et al., 2014; 2015). Applying a mega-nourishment for coastal protection instead of regular nourishment strategies would increase beach volume and the opportunity to vary habitat relief, leading to distinct communities and higher species richness of coastal fauna (Van Egmond et al., 2018).
2.3.3 Order of magnitude
Actions to promote targeted components of an ecosystem should be executed at a scale large enough to be functionally successful and cost effective (Abelson et al., 2020). Making use of infrastructural development can support this by offering technological advances to reach both efficiency of scale (Abelson et al., 2020) and economy of scale (Price and Toonen, 2017). To estimate the potential effect on ecosystem components, and to determine the required scale of interventions to be taken to become significantly effective, one should quantify the potential effects of the measures prior to their implementation (Ter Hofstede et al, 2023a). The outcomes may support decision-making when designing nature-inclusive marine infrastructure to contribute to the desired system-scale impact. Predicting the effects of such measures will provide insight into the magnitude of effort required to reach the desired impact (Ter Hofstede et al., 2023a).
2.4 Step IV - matchmaking
Matchmaking is the process of evaluating the system against its ruling policy (I), its environmental conditions (II), and its foreseen infrastructural development (III), to determine its potential for benefitting selected ecosystem components. These three elements should match in a manner that their combination reveals opportunities for nature-inclusive design at the required system-scale, promoting effectively a targeted component of the ecosystem within the area. An example of a good match, though not at system-scale, is the development of the Sand Motor in front of the Dutch coastline, a large foreshore nourishment of 128 hectares to contribute to long-term coastal protection. The design of the Sand Motor included a lagoon area with the target to become an appealing feeding and resting place for birds, and indeed was observed to have a positive effect on some species of waders, seagulls and cormorants (Huisman et al., 2021). In the case of the Sand Motor, local policy (I) states to protect all wild bird species and to protect and restore their habitats (e.g. European Commission, 2009). Furthermore, the area lies within the distribution range of many of these birds and offering a suitable environment (II) to host them, and the infrastructural design (III) was optimized with the lagoon area to provide optimal feeding and resting grounds. A similar match would not apply in the area if the infrastructural element would be for example a wind farm, which is recognized for causing negative impact on bird populations (e.g. Furness et al., 2013; Garthe et al., 2023). Although this nature-based foreshore nourishment solution is likely able to create an impact at system-scale if also implemented at other locations along the Dutch coastline, the potential effect of multiple applications has yet not been assessed (De Vries et al., 2020b).
Part of matchmaking is checking the feasibility and effectiveness of potential nature-inclusive measures to meet the prospective. A feasibility check comprises for example assessing the political, technical, operational, economical, and environmental elements. The feasibility assessment includes political, technical, operational, economical and environmental aspects. Political feasibility relates to the societal readiness for the implementation of measures, whether these are possible within the local regulations and socially acceptable. Technical feasibility concerns assessing whether the implementation of a foreseen measure is technically possible, for which the Technology Readiness Level (TRL) is a good parameter. The more mature a technology is, the more likely it can be implemented. The operational feasibility includes organizational issues, operability, accessibility of a location, required effort and limitations due to legal aspects. Economical feasibility relates to the costs of a measure to be successful, strongly determined by being active or passive, the latter generally being less expensive, as this requires less labor, technologies and personnel, e.g. the limitation of fishing activities (Fox et al., 2019). Environmental feasibility concerns whether any proposed intervention with the intention to promote selected ecosystem components would fit within the ecological boundaries of the system.
Assessing the effectiveness of measures involves quantifying their potential effect, determining the required scale for implementation, and optimizing designs to achieve the highest results. A quantitative assessment of each intervention would be based upon existing knowledge and should take into account the prevailing conditions in the designated area (Ter Hofstede et al., 2023a). Knowing the potential effect will allow to make informed decisions on the selection of measures for implementation.
2.5 Step V - agreed ambition
Once the potential has been identified for designing nature-inclusive marine infrastructure to benefit targeted ecosystem components, it is required to reach agreement on achievable ambitions for the system in which actions are foreseen. For this step, knowledge of the system is key, as weighing and ranking ambitions depends on multiple aspects such as, societal demands for the system, whether the identified required scale of a measure fits the system, knowing the future usage of the area, and any additional side-benefits from a measure. During this process of marine spatial planning, the spatial and temporal distribution of human activities in marine areas are analyzed and allocated, with the aim to achieve ecological, economic, and social objectives (Ehler and Douvere, 2009). It has been accepted as a practical tool to sustainably manage the marine environment through a participatory approach around the globe, though it is recognized that the process of stakeholder engagement still faces some challenges (e.g. Ehler, 2021; Santos et al., 2021). Profound stakeholder engagement will ensure that all knowledge from different user groups is incorporated when defining interventions that can promote selected ecosystem components. The combination of engineering, ecological and governance perspectives can yield new opportunities to improve the feasibility of nature-inclusive infrastructural development projects in sensitive environments while meeting societal demands and legislative constraints (Laboyrie et al., 2018). To achieve success after implementation, all relevant users of the system should commit to jointly set objectives, long-term as well as financially (Saunders et al., 2020). Tools for stakeholder involvement are available to ensure that their ideas, interests, and concerns are consistently addressed, and include for example open collaboration in policy modelling through building ICT-based scenario’s (Wimmer et al., 2012), engaging panels of experts through surveys such as the Delphi method (Linstone and Turoff, 1975), or group model building that includes simulating policy choices through role playing (Vennix et al., 1996).
During the process of agreeing upon an achievable ambition, the potential impact of measures on the original environment should carefully considered. For example the addition of hard structures in a sandy environment will change the available habitats, affecting the diversity and function of the system (Davis et al., 1982; Martin et al., 2005; Bulleri and Chapman, 2010). The losses of the original habitat need be assessed and in general be minimized if possible (Dafforn et al., 2015b). In situations where hard structures cannot be avoided, or are even desired, there is the potential for eco-engineering to mitigate the impacts of these structures and to maximize potential ecological outcomes (e.g. Chapman and Blockley, 2009).
Once an agreed ambition has been reached, the operational objectives can be defined to achieve the desired ecological impact. These objectives are fundamental for making the design of a nature-inclusive infrastructural development. The ‘Frame of Reference’ approach can be used to transfer the operational objectives into functional engineering designs, and to assess their performance once applied in practice (Van Koningsveld, 2003; De Vries et al., 2020a; Figure 1).
3 Application of the approach
To be able to exploit the full potential of infrastructural development to strengthen the ecological values of a system, it is key to set clear operational objectives. Our stepwise approach to determine such operational objectives for a system involves the assessment of the ruling policies in an area, the local natural and anthropogenic system, and the benefits of potential infrastructural developments. Next, an inventory of potential design measures for nature-inclusive marine infrastructure is made and assessed for their feasibility and effectiveness. Finally, an agreed ambition on measures to be taken should be reached between relevant stakeholders, for which then operational objectives can be defined. The functionality of this stepwise approach will be demonstrated in a fictive case for setting operational objectives to promote components of the subtidal ecosystem in the Dutch North Sea, aligned with offshore windfarm development.
3.1 Case description
3.1.1 Offshore windfarm development in the Dutch North Sea
The offshore wind energy industry is rapidly growing in the North Sea. In the southern North Sea alone, 62 windfarms with total surface of 3,388 km2 and capacity of 20,6 GW have been installed during the first two decades of this millennium, and the tenfold in surface area is designated to develop offshore wind energy production (Ter Hofstede et al., 2023a). These areas are generally closed for bottom-disturbing activities such as bottom-dwelling fisheries or sand extraction during the lifetime of the wind farms. They also offer hard substrate by means of the wind turbine foundations and rock material placed at the base of the turbine foundations and on top of cable crossings to prevent scouring of the seabed (Ter Hofstede et al., 2023a). For these reasons, offshore wind farms provide an environment ideal for the development of the epibenthic ecosystem, offering opportunities for biogenic reefs to develop and other organisms to forage and find shelter from human disturbances (Petersen and Malm, 2006; Coolen et al., 2020; Degraer et al., 2020).
The Dutch government has the strategic objective to rehabilitate the North Sea ecosystem, and to make offshore wind farms (see Figure 2) contribute to it through implementing nature-inclusive design measures (e.g. Ministry of Economic Affairs, 2018). However, in order to reach strategic objectives, one requires a coherent overarching realization scheme for such measures (De Vries et al., 2020b). Enabling large scale ecosystem restoration and/or ecological development in offshore wind farms requires setting up an overarching coordinated framework that sets clear targets. Otherwise, well-meant initiatives are bound to range widely in technical solutions per wind farm, being suboptimal or even ineffective at the larger scale (De Vries et al., 2020b). The systematic approach that we present supports in this process to identify and align applicable policies, the ruling environmental conditions, and the potential of the infrastructure, and determine measures that could promote targeted components of the subtidal ecosystem at offshore wind farms in the Dutch part of the North Sea (see Figure 3).
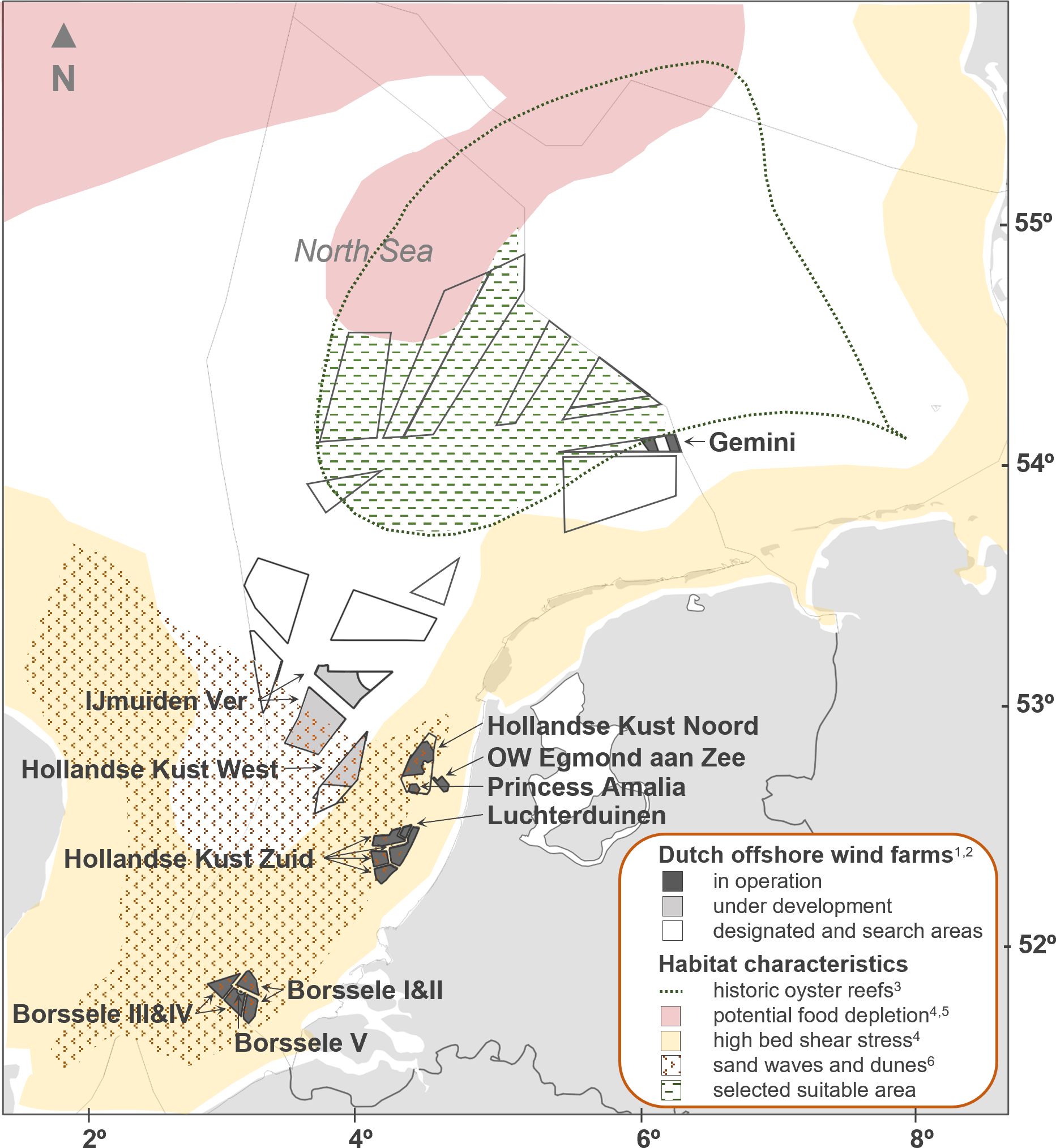
Figure 3 Offshore wind farms in the Dutch part of the North Sea. Compiled with QGIS 3.22; data sourced from 1emodnet.ec.europa.eu; 2Ministry of Infrastructure and Water Management, 2022, 3Bennema et al., 2020; 4Kamermans et al., 2018a; 5Van Leeuwen et al., 2015; 6Van der Veen et al., 2006.
3.1.2 Evolving ecological requirements for offshore wind farms
Efforts to protect and improve ecosystem components in the Dutch part of the North Sea using offshore wind farms are evolving. The first Offshore Wind farm Egmond aan Zee is in use since 2007 and its development did not include any nature-inclusive measures, though its impact on the environment was concisely monitored (Lindeboom et al., 2011). The installation of following wind farms Princess Amalia (2008), Luchterduinen (2015) and Gemini (2017) focused mainly on the protection of marine organisms such as mammals and fish from impulsive underwater sound caused by piling of the turbine foundations. A decade after the construction of the first offshore wind farm, their development required an ‘obligation to undertake demonstrable efforts’ to contribute to the strengthening of a healthy sea and to the preservation and sustainable use of endemic species and habitats in the Netherlands. This commitment was firstly formally included in the site decision of Borssele OWF lots I in 2016 (Staatscourant, 2016), and is considered an effort to utilize the momentum of the large-scale development of offshore wind farms for ecological benefits. Also the extension of the Borssele windfarms (2020) and Hollandse Kust Zuid (2021) and -Noord (2022) required to adhere to this commitment. Currently, preserving and improving the ecology of the North Sea has even become a strong requisite in the design, construction and operation of offshore wind farms, being a determinative component in the most economically advantageous tender (MEAT) criteria for offshore wind farms Hollandse Kust West Lot IV (Staatscourant, 2022) and IJmuiden Ver Lot Alpha (Staatscourant, 2023). Processes like these lead to the commercial incentivization of ecosystem restoration and creation in offshore wind farms (Stechele et al., 2023a).
A way of integrating nature-inclusive designs in offshore wind farms is to promote target species, e.g. being either umbrella species covering ‘overall native biodiversity’ or policy relevant species (Lengkeek et al., 2017). The umbrella species Atlantic cod (Gadus morhua) and policy relevant species European flat oyster (Ostrea edulis) were explicitly addressed in the Site Decisions for the recent Dutch wind farms Hollandse Kust Noord (Staatscourant, 2019) and Hollandse Kust West (Staatscourant, 2022). In the Site Decision for the latest offshore wind farm development project IJmuiden Ver, a new policy-relevant species was introduced, namely the biogenic reef building species Ross worm (Sabellaria spinulosa) (Staatscourant, 2023). To illustrate the process of the stepwise approach for setting operational objectives for nature-inclusive design of offshore wind farms in the Dutch part of the North Sea, we’ve selected the European flat oyster as target species (see Table 1).
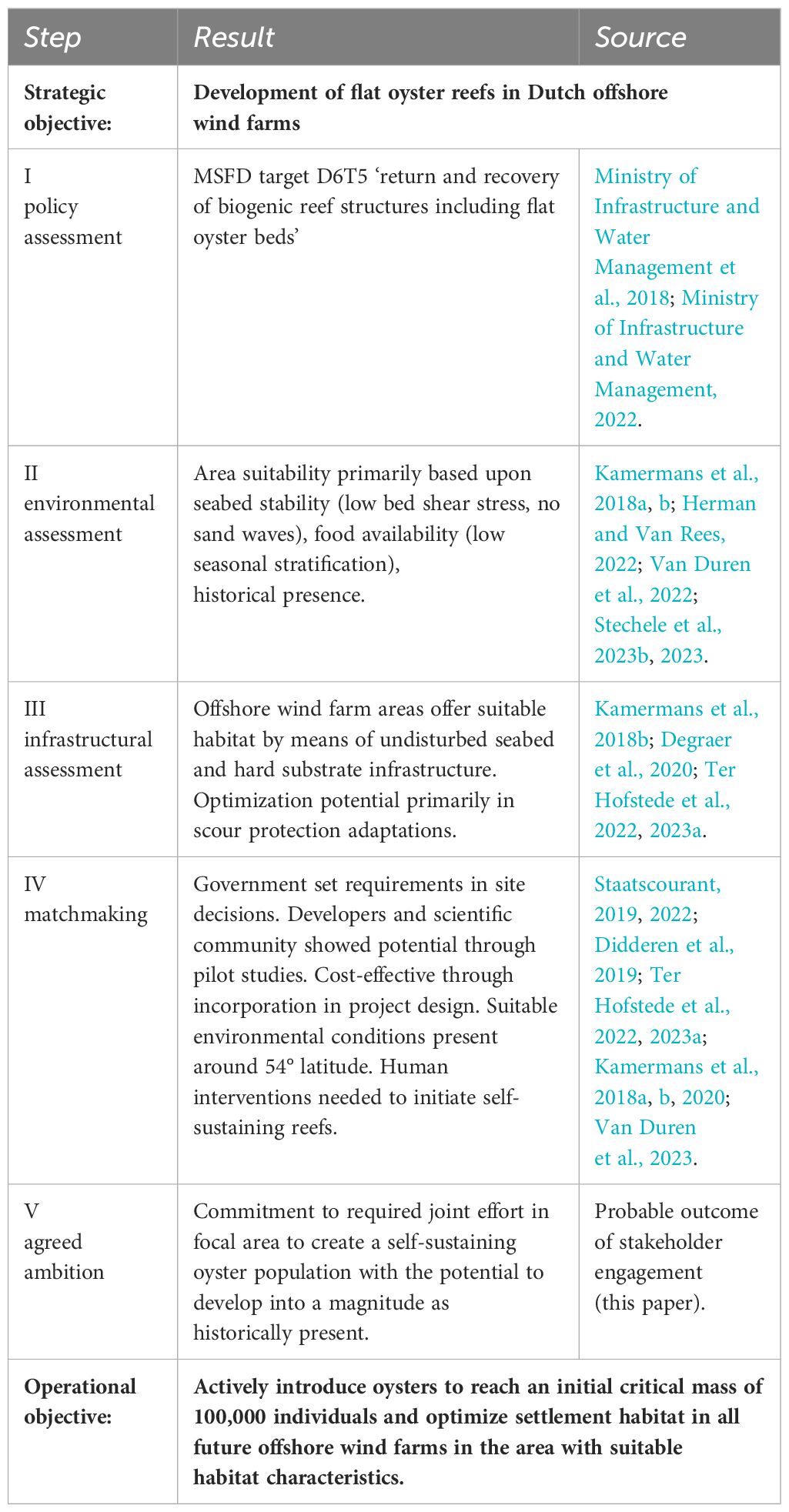
Table 1 Overview of the main outcomes when applying the stepwise approach on the case study to derive operational objectives for oyster reef development in offshore wind farms in the Dutch part of the North Sea.
3.2 Step I - policy assessment
The nature policy for the Dutch part of the North Sea is driven by the strategic objective “to restore and conserve the integrity of the ecosystem and sustainably use the ecosystem services and products” (Ministry of Infrastructure and Water Management, 2022). The objective is practiced through commitment to European treaties, primarily by the Marine Strategy Framework Directive providing 11 Descriptors for Good Environmental Status (2008/56/EC; European Commission, 2008), which have the characteristics of operational objectives, and complemented by the Birds- and Habitat Directives (2009/147/EC; European Commission, 2009) (Mulder, 2022). With respect to the development of offshore wind energy, the Dutch nature policy focuses on the nature-inclusive design, installation and operation new wind farms. This approach offers opportunities for strengthening species populations and habitats that occur naturally in the North Sea and for carrying out nature restoration projects within wind farms (Mulder, 2022).
A Dutch policy relevant for the development of the European flat oysters comes from commitment to the European Marine Strategy Framework Directive. Dutch government has set the environmental target (D6T5) for the ‘return and recovery of biogenic reef structures including flat oyster beds’ in part 1 of the Dutch Strategy for the period 2018–2024 (Ministry of Infrastructure and Water Management et al., 2018), and incorporated it as well in the Dutch North Sea Program 2022–2027 (Ministry of Infrastructure and Water Management, 2022).
3.3 Step II - environmental assessment
Wind farm locations relatively suitable for oyster reef development have been appointed based upon habitat suitability, larval retention, food availability, and historical presence (Smaal et al., 2017; Kamermans et al., 2018a, b; Herman and Van Rees, 2022; Van Duren et al., 2022; Stechele et al., 2023b, 2023). The most important characteristics of habitat suitability relate to the presence of a stable seabed, meaning little seabed mobility (sand waves), low bed shear stress, and a composition that provides a consolidated foundation, such as stable sands, stiff muds, shells or rock (Héral and Deslous-Paoli, 1991; Houziaux et al., 2008; Smaal et al., 2017; Hughes et al., 2023). Larval retention is assumed to be highest in areas at or near their production, and therefore its assessment accounted for the source locations and dispersal rates (Herman and Van Rees, 2022). Food availability is related to the stratification of the North Sea during the summer season, reducing the transport of the main food source phytoplankton to the seabed. Areas with high seasonal stratification are therefore assumed less suitable for oyster reef development, opposed to areas that are nearly fully mixed (Van Leeuwen et al., 2015; Kamermans et al., 2018a; Stechele et al., 2023b). For estimating the historical presence of oyster reefs, data was used from Olsen (1883), Houziaux et al. (2008), and Bennema et al. (2020). Note that the potential for oyster reef development offered by the infrastructure in offshore windfarms (Ter Hofstede et al., 2023a), in particular in terms of hard substrate offered by the rock material used as scour protection, was not taken into account in these studies. Other main factors of importance for the survival of flat oysters are oxygen content in the water and food availability by means of phytoplankton, but these are not limiting in the Dutch part of the North Sea (Van Leeuwen et al., 2015; Van Duren et al., 2022). The potential suitable area for oyster reef development in the Dutch part of the North Sea is visualized in Figure 3, drafted from their historic distribution (Bennema et al., 2020) and the prime environmental factors that hamper their development, i.e. bed shear stress (Kamermans et al., 2018a), sand waves (Van der Veen et al., 2006), and potential food depletion based upon stratification (Van Leeuwen et al., 2015).
3.4 Step III - infrastructural assessment
Once in operation, offshore wind farms provide an undisturbed seabed and hard substrate infrastructure which both make them suitable for oyster reef development (Kamermans et al, 2018b; Degraer et al., 2020; Ter Hofstede et al., 2022, 2023a). For safety reasons, the wind farm developers have successfully excluded bottom disturbing activities such as bottom-trawl fisheries from the concession zones around their underwater infrastructure such as turbine foundations and cable routes. The exclusion of disturbing activities from these concession zones has resulted in quasi-marine protected areas providing refuge for benthic habitats and species such as oysters (Hammar et al., 2016). The infrastructure of offshore wind farms inherently provides artificial habitat, allowing long-term development of targeted ecosystem components, and its design can even be optimized to target desired species (Ter Hofstede et al., 2023b). The type of infrastructure used for offshore wind farms is dependent upon the system in which it is build. In particular the support structures for the wind turbines will vary, either fixed-support or floating. The fixed-support types are gravity-based and the turbines are placed on for example monopile foundations or jackets. Their primary constraint is the limited depth in which they can be build, as in waters of over 60 m depth, they become commercially inviable due to a considerable increase in costs (The Carbon Trust, 2015). Floating wind turbines are held in place through mooring cables connected to the seabed. Their costs are generally higher than gravity-based turbines, and also significantly increase with water depth (Kausche et al., 2018). Gravity-based designs of wind turbines can likely include the highest benefits for increasing epibenthic biodiversity, in particularly when placed on sandy substrate. Here they require a scour protection, generally by means of rocky substrate, which forms a suitable habitat for the development of marine flora and fauna (Petersen & Malm, 2006; Degraer et al., 2020; Glarou et al., 2020). Such benefits for epibenthic biodiversity should be taken into account when selection the type of windfarm in the design process. To increase biodiversity or the presence of targeted rock-dwelling species using scour protection, small adaptations in material use, texture, and shape can improve the conditions for settlement, growth and use by a variety of marine organisms even more, while keeping the function of the scour protection intact (Ter Hofstede et al., 2023a). In case of oyster reef development, the main adaptations in the scour protection design for would include the use of calciferous rock material such as limestone or marble, containing a high amount of calcium which is beneficial to shellfish species (Hidu et al., 1975; Soniat et al., 1991). Furthermore, the scour protection should be designed with rock material of a grading size large enough to provide stable substrate (Van Velzen et al., 2014), as moving rocks cause physical damage or even mortality to the oysters. Also, extending the dimensions of the scour protection would increase the area of hard substrate for settlement by oyster larvae, and variation in shape could create areas with reduced flow velocity to improve the opportunities for settlement (Korringa, 1940; Smaal et al., 2017). Over time, the oyster reef development is even expected to contribute to the stabilization of the scour protection by effectively binding the rocks, in particular those of a smaller grading size (Domisse, 2020).
3.5 Step IV - matchmaking
The combination of the ruling policy, environmental conditions, and presence of offshore wind farms in the Dutch part of the North Sea offers great potential for oyster reef development. This match is based upon an assessment of both the feasibility and effectiveness of the potential measures that can be taken to establish oyster reefs using the infrastructure in offshore wind farms.
3.5.1 Feasibility
The feasibility assessment includes political, technical, operational, economical and environmental aspects.
From a political perspective, it is highly feasible to develop oyster reefs in Dutch offshore wind farms, as there’s general support from government, developers, scientists, and the public society. The government has been setting requirements for oyster reef development in the site decisions for new wind farms, e.g. in windfarms Hollandse Kust Noord and -West (Staatscourant, 2019; 2022). Wind farm operators have shown their willingness by taking measures to initiate reef development in wind farms Gemini, Borssele 3&4, Borssele V, and Luchterduinen (e.g. Didderen et al., 2019). The scientific community has stressed the potential to use wind farms for oyster reef restoration practices (e.g. Lengkeek et al., 2017; Kamermans et al., 2018b). From public society, no opposition has been reported, and oyster reef development is generally advocated by non-governmental organizations (e.g. Sas et al., 2019; Vrooman et al., 2019).
From a technical perspective, Dutch offshore wind farms offer great potential for oyster reef development. The seabed in the area refrains from disturbance as no bottom disturbing activities are allowed during the operational lifetime of the wind farms. The infrastructure of the windfarms by means of the scour protection at the base of the turbine foundations and at the cable crossings, offers excellent substrate for settlement of larvae. The infrastructure could even be further optimized by making small adaptations in material use, texture, and shape to further improve settlement conditions (Ter Hofstede et al., 2023a).
From an operational perspective, multiple pilot studies have shown that interventions can be taken in offshore wind farms to trigger oyster reef development. For example, adjustments to the scour protection were made to facilitate oyster larvae settlement in several Dutch offshore wind farms: In Borssele 3&4, 20 m3 of clean shell material was placed in the scour protection at the base of 8 wind turbines foundations; in Hollandse Kust Zuid, a sprinkler layer of marble rock was placed at 4 cable crossings; and in Hollandse Kust Noord, berms of marble rock were placed at the scour protection of 42 wind turbines. In addition, to initiate larvae production and kickstart reef development, oyster broodstock was installed in wind farms Borssele 3&4, Borssele V, Luchterduinen, and Gemini (Didderen et al., 2019), and at all locations the broodstock was shown to survive, grow and reproduce after installation.
From an economical perspective, feasibility relates to the costs of a measure to be successful. All activities in the offshore environment are generally considered to be costly due to the required vessel time. However, the fact that many activities have already taken place to develop oyster reefs in offshore wind farms, demonstrates their feasibility, independent of the costs. Cost reductions can be achieved by wisely selecting and implementing interventions. For example on a small scale, the deployment of oyster broodstock fixed on stable structures will provide a dense and lasting source of adult oysters to ensure local larvae production, while deployment via loose distribution would require a far greater amount of oysters to ensure the same sized broodstock over time, due the high risk of losses caused by severe hydrodynamic conditions. On a larger scale, costs of vessel time include the expensive mobilization and demobilization of a vessel for a specific purpose. The longer a vessel can be at sea, the daily costs of the activity becomes relatively lower. Therefore, it is recommended to combine as much as feasible the various activities that are aimed at promoting targeted ecosystem components, or even align them fully with the standard wind farm installation and operation activities. For example, the deployment of calciferous rock aimed at increasing oyster larvae settlement rates should be executed in line with the installation of the functional scour protection, and preferably even be fully integrated in its basic design; post-construction deployment as an add-on should be avoided at all times to save on costs.
From an environmental perspective, offshore wind farms in the Dutch part of the North Sea are generally considered suitable for oyster reef development. The environmental conditions however vary throughout the region, leading to different levels of suitability. Although all current and likely future Dutch offshore wind farms offer substrate for oyster reef development by means of their scour protection at the base of the turbine foundations, the vast seabed area in between the turbines does not. Oysters require a stable seabed with low hydrodynamic forces such as bed shear stress. These conditions are not present in the southern part of the Dutch EEZ (Kamermans et al., 2018b; Van Duren et al., 2023; see Figure 3). In order to utilize wind farm areas for oyster reef development to its full potential, meaning not only at the scour protections, but also at the seabed in between, it is therefore recommended to focus efforts to establish oyster reefs in the current and future wind farms in the northern part of the Dutch EEZ (Van Duren et al., 2023). However, the tip of the Dutch EEZ around 55° latitude is considered unsuitable due to poor food availability in the summer season as a consequence of stratification (Kamermans et al., 2018a; see Figure 3). These considerations would leave an area assumed most suitable for oyster reef development around the 54° latitude (see Figure 3).
3.5.2 Effectiveness
In order to select measures to establish oyster reefs in offshore wind farms, their potential effect needs to be quantified first. Knowing the impact of the presence of the wind farm itself, and of the additional interventions to increase it, allows to make informed decisions in setting the operational objectives for oyster reef development in an area. The quantification provides insight in the required order of magnitude of the potential effects, needed to determine the type and scale for selecting measures to achieve a desired result. A stepwise procedure designed in particular to guide the selection of appropriate interventions and their required scale for pro-actively facilitating flat oyster reef development in offshore wind farms, was presented by Ter Hofstede et al. (2023a). The procedure makes use of available knowledge, allowing inclusion of most recent insights. An assessment of the wider Southern North Sea bordered by England (UK) on the west, and Belgium, The Netherlands, Germany and Denmark on the east, learned that oyster reef development in offshore wind farms at least requires a human-induced accumulation of broodstock in the wind farms due to the lack of connectivity with the scarce natural reefs (Ter Hofstede et al., 2023a). Succeeding development of oyster reefs within a wind farm area is suggested to be facilitated by providing suitable substrate for larvae settlement. Provision of clean shell material would be the most beneficial, in potential offering oyster densities 150 times higher than on rock material and 8000 times higher than on a soft seabed. However, the supply of shell material is not unlimited, and also not without impact on the existing environment when being collected. Therefore, a focus on providing suitable settlement substrate using rock material, such as already applied in the scour protections in wind farms, would be a good alternative. Optimization of these scour protections for oyster reef development can be achieved by using most suitable rock material, and adjustment of the conventional shape. Calciferous rock material such as marble could in increase settlement rates by factor 1.33 (Tonk et al., 2020), and simply extending the scour protection horizontally would increase settlement opportunities linearly, particularly if done for the armour layer which won’t disappear on a layer of sand as is the case for the filter layer (Ter Hofstede et al., 2022).
3.6 Step V - agreed ambition
Now that the potential for oyster reef development in the Dutch part of the North Sea has been identified, it is needed to reach agreement on achievable ambitions to do so, for which operational objectives can be defined next. The ambition should be agreed upon by relevant stakeholders, of whom their knowledge of the political and environmental system and of the potential for implementation of interventions are key to ensure that the ambition will be achievable. The main four stakeholder groups for this case study would at least include i) relevant Dutch Ministries having legislative authority of the area, ii) research institutes with knowledge about the environmental conditions, iii) marine contractors providing engineering solutions, and iv) wind energy developers being the owners of the offshore wind farms.
The demonstration of the functionality of our stepwise approach is merely applied for a fictive case. Therefore an actual stakeholder involvement process was not performed, but we confined ourselves to assuming a probable perspective for each of the four stakeholders towards oyster reef development in Dutch offshore windfarm: Governmental authorities (i) would be supportive, following their incentive to “return and recover biogenic reef structures including flat oyster beds”, and have the powerful tool of setting requirements to enforce oyster reef development in site decisions for new offshore wind farms. The scientific community (ii) would stress the need for windfarms to have suitable environmental conditions, and a connected, preferably continuous, areal large enough to host a self-sustainable population. The wind farm developers (iii) would be willing to invest in taking nature-inclusive measures to meet contractual obligations and to strengthen their corporate image, though a predictable income from energy production should be guaranteed. Marine contractors (iv) would offer the capability to design and implement engineering solutions to support oyster reef development, but require guidance on the required type and extent of interventions.
Considering the stakeholder perspectives, it can be concluded that habitat suitability would be the main driver for agreeing upon development of oyster reefs in specific offshore wind farms, as it is the only aspect that varies across the Dutch part of the North Sea (see Figure 3). On the contrary, the Dutch policy (to recover flat oyster beds) and the type of existing and future wind farm infrastructure (a monopile foundation with rocky scour protection at its base), are generally more uniformly distributed.
An area suitable for oyster reef development that offers a stable seabed and year-round food availability, is present in the Dutch part of the North Sea broadly around the 54° latitude (Kamermans et al., 2018b; Van Duren et al., 2023; see Figure 3). The area is partly overlapping with the historic presence of oyster beds (Olsen, 1883; Houziaux et al., 2008; Bennema et al., 2020). The highest potential to establish oyster reefs successfully is generally thought to be in an area with both suitable environmental conditions and historic presence of oyster reefs (Kamermans et al., 2018a, b; Stechele et al., 2023b). Therefore, the area around 54° latitude and between 4° and 6° longitude could be appointed to initiate oyster reef development in the Dutch part of the North Sea (see Figure 3). This area also includes search areas for future offshore wind farms (see Figure 3), eventually offering the valuable infrastructure for hosting oyster reefs, which could be optimized even further to provide the most suitable conditions.
Currently, observations of flat oyster reefs presence have not been reported for the area, nor is connectivity with existing reefs to be expected (Ter Hofstede et al., 2023a). Therefore, active introduction of oysters for local larvae production to initiate reef development would be required. A preferred starting population would be one that can become self-sustaining over time. An amount of 20,000 oysters was suggested as the minimum starting population size to exceed a limited critical mass of 100,000 oysters (at high densities of 82 m-2) within 3 years (Smyth et al., 2016; Kamermans et al., 2020). For such a population to be able to develop into a size as used to be present in the late 19th century, a large area, exceeding individual wind farms, is needed (Ter Hofstede et al., 2023a). This means that all future offshore wind farms in the area should embrace the ambition to host oyster reefs. Further increase of the oyster population can be achieved by optimizing the wind farm areas to provide suitable hard substrate habitat. Optimizations could include increasing the habitat complexity of conventional scour protection by bringing in more variety in use of materials, shapes and dimensions (Ter Hofstede et al., 2023a), and the installation of longlines hosting vertical mussel reefs that provide a continuous supply of shell material and will thereby provide most suitable settlement substrate for oyster larvae (Ter Hofstede et al., 2023a, b).
The agreed ambition between the prime stakeholders to restore oyster reefs in the Dutch part of the North Sea could be threefold, i.e. 1) to concentrate efforts in the future offshore wind farms located in the area that is considered most suitable for oyster reefs, i.e. around 54° latitude and between 4° and 6° longitude (see Figure 3); 2) to initiate oyster reef development by deploying sufficient broodstock for the population to become self-sustainable; and 3) to provide settlement substrate as part of the wind farm infrastructure for the oyster reefs to thrive upon in higher densities than at the existing seabed. The operational objective would accordingly become: Actively introduce oysters to reach an initial critical mass of 100,000 individuals and optimize settlement habitat at all future offshore wind farms in the area with suitable habitat characteristics. This operational objective would provide the starting point for the next step, i.e. defining and actually implementing quantified technical solutions needed to reach the objective, using a tool like the ‘Frame of Reference’ approach (see Figure 1). The application of this approach was demonstrated to further stimulate the integration and cooperation of science, policy and management during the process of defining functional engineering designs and assessing their performance (Van Koningsveld, 2003).
4 Discussion
Marine infrastructure offers huge potential to improve the ecological functioning of the system in which it is build (Dafforn et al., 2015b; Laboyrie et al., 2018), and it is becoming more and more common to include nature-inclusive elements in marine construction projects (e.g. Borsje et al., 2011; Firth et al., 2014; Ter Hofstede et al., 2023a). In most cases, these nature-inclusive measures are designed to meet individual project requirements, fragmented, and insufficiently taking into account the opportunity to make a system-scale impact. To do so, a coherent overarching realization scheme of design measures for nature-inclusive marine infrastructure is required, with clear objectives to make the efforts effective at a larger scale beyond individual projects (De Vries et al., 2020b). Strategic objectives are required to address the desired future condition of a system, defining a long-term plan with clear priorities, along with a means to monitor and assess progress (Tunnicliffe et al., 2020). Operational objectives are needed to provide tangible direction to implement strategic objectives, with a clear indication of the spatial and temporal scales involved (Van Koningsveld et al., 2005).
Our paper presents a stepwise approach for defining clear operational objectives for nature-inclusive marine infrastructure to achieve impact at system-scale, in which ruling polices, environmental conditions and the potential use of marine infrastructure are aligned. It includes careful consideration of the full potential nature-inclusive infrastructure can offer, based upon an assessment of the feasibility of measures and their estimated effects. Our approach can support policy makers in achieving their environmental targets, while at the same time meeting societal demands for infrastructural development. For example member states of the European Union have set policy targets to achieve a Good Environmental Status of their marine ecosystems, being implemented by the Marine Strategy Framework Directive (European Commission, 2008), while at the same time their marine environment is increasingly used for wind energy production in order to meet renewable energy goals (European Commission, 2023). Using our approach could facilitate EU member states in the planning process of their marine waters, by identifying measures that can be implemented in the future marine infrastructure to optimize the potential benefits for ecosystem components targeted by the MSFD. Herewith policy makers might overcome the struggles they face during the marine spatial planning process, e.g. in establishing a shared transboundary vision and in aligning the different interests of stakeholders (e.g. Fraschetti et al., 2018; Santos et al., 2021). The final selection of operational objectives for a system is still to be made through strong involvement of these stakeholders. This is to ensure that all required knowledge and expertise from various disciplines are covered, and to achieve commitment to the jointly established objectives. For example, inclusion of the scientific community, allows for the assessment of the objectives to determine their ecological feasibility and their consequences, which is often too little understood by decision makers and developers only (Lackey, 2003). Scientists provide advice on the ecological, social and economic repercussions of the objectives, and can determine courses of actions to be taken (Cormier et al., 2017). However, scientists might also display implicit preferences and advocate a certain ecological state for a system, which should be avoided when providing advice for setting objectives (Lackey, 2003). This illustrates the importance of a balanced stakeholder engagement process, in which the various relevant disciplines are evenly represented.
Reaching agreement upon achievable ambitions between different stakeholder disciplines can be a difficult process. For example, going from overarching strategic objectives towards clear operational objectives was observed to lead to confusion about terminology, baseline and reference states, and defining them quantitatively (Leadley et al., 2022). The process of setting operational objectives for including nature-inclusive elements in the design of marine infrastructure requires strong leadership and political will. A lack of motivation to improve legislation, vested interest in conventional infrastructural development, and insufficient funds and resources will hamper the development and implementation of measures that could benefit marine life (Dhakal and Chevalier, 2017; Johns, 2019). Stakeholder engagement during the stepwise approach to reach operational objectives for nature-inclusive marine infrastructure allows all relevant users of the system to express their interests, essential to reach long-term commitment to the set objectives.
Our stepwise approach to define operational objectives to embed nature-inclusive measures into marine infrastructure has been demonstrated for use to promote oyster reef development in offshore wind farms in the Dutch part of the North Sea. To establish oyster reef restoration in the North Sea, it is recommended to follow a coordinated basin-wide approach to reach connectivity between natural oyster beds, restoration sites, offshore infrastructure, and aquaculture sites (Stechele et al., 2023a). Such can only be achieved if an overarching vision is developed for an area, including the setting of clear operational objectives for implementing measures wisely. Assessing the three major elements within the Dutch North Sea system, i.e. policy, environment and infrastructure, it is concluded that these elements match when striving for oyster reef development in offshore wind farms. The European flat oyster has been characterized as a ‘policy relevant species’ (Lengkeek et al., 2017) and is already explicitly addressed as a target species in Site Decisions for new offshore wind farms (Staatscourant, 2019; 2022). The environmental conditions are considered most suitable for oyster reefs around the 54° latitude and between 4° and 6° longitude (see Figure 3), which is primarily influenced by food availability, the presence of a stable seabed with low hydrodynamic forces, and their historic presence (Kamermans et al., 2018a; b; Bennema et al., 2020; Van Duren et al., 2023). The area is furthermore prone to offshore wind farm development in the near future (Ministry of Infrastructure and Water Management, 2022), providing infrastructure that offers suitable substrate for oyster reef development. Considering the interests of the main stakeholders, it is highly likely that an agreement on achievable ambitions can be established, which would result in the operational objective to actively introduce oysters to reach an initial critical mass and optimize settlement habitat in all future offshore wind farms in the area.
Offshore wind farms outside of the identified area with the most suitable habitat characteristics should not be completely discarded from consideration to support oyster reef restoration. In general, at the base of each wind turbine foundation and on top of cable crossings, rock material is placed to prevent scouring of the seabed, providing good conditions for oyster reef formation (Ter Hofstede et al., 2023a). Although the majority of the seabed within a wind farm in the southern part of the Dutch Nort Sea might not be stable enough for oyster reef development, their scour protections do provide suitable substrate, and could function as steppingstones for the spread of oyster larvae (Adams et al., 2014), thereby contributing to oyster reef restoration throughout the North Sea. The suitability of scour protections within these offshore wind farms can also be further optimized for hosting oyster reefs by the design of their shape and dimension and by the type of rock material used (Ter Hofstede et al., 2023a). Whichever design optimizations in a scour protection are feasible while still preserving its primary function to prevent seabed erosion, is location-specific and depends on the willingness of the developer to invest, if a cost-increase is applicable.
The final selection of which and where to implement design measures for nature-inclusive marine infrastructure is always to be made through careful consideration of the different interests of relevant stakeholders. If only individual interests are pursued, there’s a risk of an uncoordinated fragmentation of well-intended though ineffective measures to promote ecosystem components, which fail the need to strengthen one another, or even may be counteracting. In order for interventions to be truly benefitting marine life, it is required to implement measures at a predetermined scale, large enough to be create impact within the larger system (Abelson et al., 2020; Ter Hofstede et al., 2023b). When feasible, one should even consider targeting cross-habitat effects, by facilitating positive interactions that occur when processes generated in one habitat benefits other (Vozzo et al., 2023). For effective improvement of targeted components of the ecosystem, an interdisciplinary approach with the involvement of different stakeholders is needed, covering all required aspects with regards to knowledge, expertise, finance, and legislation (Gann et al., 2019; Saunders et al., 2020). Finding mutual ground and reaching agreement on achievable ambitions between all relevant parties is key for setting operational objectives to take measures for nature-inclusive design with and within marine infrastructure at a system-wide scale. This can be achieved through following our stepwise approach in which the potential for nature-inclusive marine infrastructure is determined by matching the ruling policy, its environmental conditions, and its foreseen infrastructural development, followed by jointly determining the most effective operational objectives.
Data availability statement
The original contributions presented in the study are included in the article/supplementary material. Further inquiries can be directed to the corresponding author/s.
Author contributions
RH: Conceptualization, Data curation, Investigation, Methodology, Project administration, Software, Visualization, Writing – original draft, Writing – review & editing. Mv: Conceptualization, Funding acquisition, Investigation, Methodology, Project administration, Supervision, Writing – original draft, Writing – review & editing.
Funding
The author(s) declare that financial support was received for the research, authorship, and/or publication of this article. This work was supported by the Dutch Research Council NWO [grant number 17671 (North Sea ReVIFES)].
Conflict of interest
The authors declare the research was conducted in the absence of any commercial or financial relationships that could be construed as a potential conflict of interest.
Publisher’s note
All claims expressed in this article are solely those of the authors and do not necessarily represent those of their affiliated organizations, or those of the publisher, the editors and the reviewers. Any product that may be evaluated in this article, or claim that may be made by its manufacturer, is not guaranteed or endorsed by the publisher.
References
Abelson A., Reed D. C., Edgar G. J., Smith C. S., Kendrick G. A., Orth R. J., et al. (2020). Challenges for restoration of coastal marine ecosystems in the anthropocene. Front. Mar. Sci. 7, 544105. doi: 10.3389/fmars.2020.544105
Adams T., Miller R. G., Aleynik D., Burrows M. T. (2014). Offshore marine renewable energy devices as stepping stones across biogeographical boundaries. J. Appl. Ecol. 51, 330–338. doi: 10.1111/1365-2664.12207
Allen M. C., Read A. J., Gaudet J., Sayigh L. S. (2001). Fine-scale habitat selection of foraging bottlenose dolphins Tursiops truncates near Clearwater, Florida. Mar. Ecol. Prog. Ser. 222, 253–264. doi: 10.3354/meps222253
Bennema F. P., Engelhard G. H., Lindeboom H. (2020). Ostrea edulis beds in the central North Sea: delineation, ecology, and restoration. ICES J. Mar. Sci. 77, 2694–2705. doi: 10.1093/icesjms/fsaa134
Bishop M. J., Mayer-Pinto M., Airoldi L., Firth L. B., Morris R. L., Loke L. H., et al. (2017). Effects of ocean sprawl on ecological connectivity: impacts and solutions. J. Exp. Mar. Biol. Ecol. 492, 7–30. doi: 10.1016/j.jembe.2017.01.021
Borsje B. W., van Wesenbeeck B. K., Dekker F., Paalvast P., Bouma T. J., Katwijk v., et al. (2011). How ecological engineering can serve in coastal protection. Ecol. Eng. 37, 113–122. doi: 10.1016/j.ecoleng.2010.11.027
Browne M. A., Chapman M. G. (2011). Ecological informed engineering reduces loss of intertidal biodiversity on artificial shorelines. Environ. Sci. Technol. 45, 8204–8207. doi: 10.1021/es201924b
Bugnot A., Mayer-Pinto M., Airoldi L., Heery E., Johnston E., Critchley L., et al. (2020). Current and projected global extent of marine built structures. Nat. Sustain. 4, 33–41. doi: 10.1038/s41893-020-00595-1
Bulleri F., Chapman M. G. (2010). The introduction of coastal infrastructure as a driver of change in marine environments. J. Appl. Ecol. 47, 26–35. doi: 10.1111/j.1365-2664.2009.01751.x
Carroll B., Fothergill J., Murphy J., Turpin T. (2020). Environmental impact assessment handbook: a practical guide for planners, developers and communities (London, UK: ICE Publishing), 144. doi: 10.1680/eiah3e.61415
Chapman M. G., Blockley D. J. (2009). Engineering novel habitats on urban infrastructure to increase intertidal biodiversity. Oecologia 161, 625–635. doi: 10.1007/s00442-009-1393-y
Consoli P., Mangano M. C., Sarà G., Romeo T., Andaloro F. (2018). The influence of habitat complexity on fish assemblages associated with extractive platforms in the central Mediterranean Sea. Adv. Oceanogr. Limnol. 9, 59–67. doi: 10.4081/aiol.2018.7918
Coolen J. W. P., van der Weide F. B. E., Cuperus J., Blomberg M., van Moorsel G. W. N. M., Faasse M. A., et al. (2020). Benthic biodiversity on old platforms, young wind farms and rocky reefs. ICES J. Mar. Sci. 77, 1250–1265. doi: 10.1093/icesjms/fsy092
Cormier R., Kelble C. R., Anderson M. R., Allen J. I., Grehan A., Gregersen Ó. (2017). Moving from ecosystem-based policy objectives to operational implementation of ecosystem-based management measures. ICES J. Mar. Sci. 74, 406–413. doi: 10.1093/icesjms/fsw181
Cuadrado-Rica H., Sebaibi N., Boutouil M., Boudart B. (2016). Properties of ordinary concretes incorporating crushed queen scallop shells. Mater. Struct. 49, 1805–1816. doi: 10.1617/s11527-015-0613-7
Dafforn K. A., Glasby T. M., Airoldi L., Rivero N. K., Mayer-Pinto M., Johnston E. L. (2015a). Marine urbanization: an ecological framework for designing multifunctional artificial structures. Front. Ecol. Environ. 13, 82–90. doi: 10.1890/140050
Dafforn K. A., Mayer-Pinto M., Morris R. L., Waltham N. J. (2015b). Application of management tools to integrate ecological principles with the design of marine infrastructure. J. Environ. Manage. 158, 61–73. doi: 10.1016/j.jenvman.2015.05.001
Danovaro R., Bianchelli S., Brambilla P., Brussa G., Corinaldesi C., Del Borghi A., et al. (2024). Making eco-sustainable floating offshore wind farms: Siting, mitigations, and compensations. Renew. Sustain. Energy Rev. 197, 114386. doi: 10.1016/j.rser.2024.114386
Davis N., Van Blaricom G. M., Dayton P. K. (1982). Man-made structures on marine sediments: effects on adjacent benthic communities. Mar. Biol. 70, 295–303. doi: 10.1007/BF00396848
Degraer S., Carey D. A., Coolen J. W. P., Hutchison Z. L., Kerckhof F., Rumes B., et al. (2020). Offshore wind farm artificial reefs affect ecosystem structure and functioning. Oceanogr 33, 48–57. doi: 10.5670/oceanog
De Jong M. F., Baptist M. J., Lindeboom H. J., Hoekstra P. (2015). Short-term impact of deep sand extraction and ecosystem-based landscaping on macrozoobenthos and sediment characteristics. Mar. pollut. Bull. 97, 294–308. doi: 10.1016/j.marpolbul.2015.06.002
De Jong M. F., Baptist M. J., van Hal R., De Boois I., Lindeboom H. J., Hoekstra P. (2014). Impact on demersal fish of a large-scale and deep sand extraction site with ecosystem-based landscaped sandbars. Estuar. Coast. Shelf Sci. 146, 83–94. doi: 10.1016/j.ecss.2014.05.029
De Vries M., van Koningsveld M., Aarninkhof S., de Vriend H. (2020a). A systematic design approach for objectifying Building with Nature solutions. Res. Urban Ser. 7, 29–50. doi: 10.47982/rius.7.124
De Vries M., van Koningsveld M., Aarninkhof S., de Vriend H. (2020b). Objectifying Building with Nature strategies: towards scale resolving policies. Res. Urban Ser. 7, 51–72. doi: 10.47982/rius.7.128
Dhakal K. P., Chevalier L. R. (2017). Managing urban stormwater for urban sustainability: barriers and policy solutions for green infrastructure application. J. Environ. Manage. 203, 171–181. doi: 10.1016/j.jenvman.2017.07.065
Didderen K., Bergsma J. H., Kamermans P. (2019). Offshore flat oyster pilot Luchterduinen wind farm: Results campaign 2 (July 2019) and lessons learned. Rep. Bureau Waardenburg (Culemborg, The Netherlands) 48, 19–184.
Domisse W. (2020). “Shellfish as a protection of revetments: A case study in the port of Rotterdam,” in Master thesis Delft University of Technology(Delft, The Netherlands), 107.
Duarte C. M., Agusti S., Barbier E., Britten G. L., Castilla J. C., Gattuso J. P., et al. (2020). Rebuilding marine life. Nature 580, 39–51. doi: 10.1038/s41586-020-2146-7
Ehler C. N. (2021). Two decades of progress in Marine Spatial Planning. Mar. Policy 132, 104134. doi: 10.1016/j.marpol.2020.104134
Ehler C. N., Douvere F. (2009). Marine Spatial Planning: A Step-by-Step Approach Toward Ecosystem-Based Management. Intergovernmental Oceanographic Commission and Man and the Biosphere Programme. IOC Manual and Guides No. 53, ICAM Dossier No. 6 (UNESCO, Paris), 99.
European Commission (2008). “Directive 2008/56/EC of the European Parliament and of the Council of 17 June 2008 establishing a framework for community action in the field of marine environmental policy (Marine Strategy Framework Directive),” in Official Journal of the European Union L164(Brussels, Belgium), 22.
European Commission (2009). “Council Directive 2009/147/EC of 30 November 2009 on the conservation of wild birds,” in Official Journal of the European Union L20(Brussels, Belgium), 7–25.
European Commission (2014). “Directive 2014/52/EU of the European Parliament and of the Council of 16 April 2014, amending Directive 2011/92/EU on the assessment of the effects of certain public and private projects on the environment,” in Official Journal of the European Union L124(Brussels, Belgium), 18.
European Commission (2019). “The european green deal,” in Communication from the Commission COM/2019/640 final(Brussels, Belgium), 24.
European Commission (2023). “Directive (EU) 2023/2413 of the European Parliament and of the Council of 18 October 2023 amending Directive (EU) 2018/2001, Regulation (EU) 2018/1999 and Directive 98/70/EC as regards the promotion of energy from renewable sources, and repealing Council Directive (EU) 2015/652,” in Official Journal of the European Union L series(Brussels, Belgium), 77.
Firth L. B., Airoldi L., Bulleri F., Challinor S., Chee S.-Y., Evans A. J., et al. (2020). Greening of grey infrastructure should not be used as a Trojan horse to facilitate coastal development. J. Appl. Ecol. 57, 1762–1768. doi: 10.1111/1365-2664.13683
Firth L. B., Thompson R. C., Bohna K., Abbiati M., Airoldi L., Bouma T. J., et al. (2014). Between a rock and a hard place: environmental and engineering considerations when designing coastal defence structures. Coast. Eng. 87, 122–135. doi: 10.1016/j.coastaleng.2013.10.015
Fleishman E., Murphy D. D., Brussard P. F. (2000). A new method for selection of umbrella species for conservation planning. Ecol. Appl. 10, 569–579. doi: 10.1890/1051-0761(2000)010[0569:ANMFSO]2.0.CO;2
Fox A. D., Corne D. W., Mayorga Adame C. G., Polton J. A., Henry L.-A., Roberts J. M. (2019). An efficient multi-objective optimization method for use in the design of marine protected area networks. Front. Mar. Sci. 6, 17. doi: 10.3389/fmars.2019.00017
Fraschetti S., Pipitone C., Mazaris A. D., Rilov G., Badalamenti F., Bevilacqua S., et al. (2018). Light and shade in marine conservation across european and contiguous seas. Front. Mar. Sci. 5, 420. doi: 10.3389/fmars.2018.00420
Furness R. W., Wade H., Masden E. A. (2013). Assessing vulnerability of seabird populations to offshore wind farms. J. Environ. Manage. 119, 56–66. doi: 10.1016/j.jenvman.2013.01.025
Gann G. D., McDonald T., Walder B., Aronson J., Nelson C. R., Johnson J., et al. (2019). International principles and standards for the practice of ecological restoration. Second edition. Restor. Ecol. 27, S1–S46. doi: 10.1111/rec.13035
Garel E., Camba C., Ferreira Ó., Van Koningsveld M. (2014). “Applicability of the “Frame of Reference” approach for environmental monitoring of offshore renewable energy projects”. J. Environ. Manage. 141, 16–28. doi: 10.1016/j.jenvman.2014.02.037
Garthe S., Schwemmer H., Peschko V., Markones L., Muller S., Schwemmer P. (2023). Large-scale effects of offshore wind farms on seabirds of high conservation concern. Sci. Rep. 13. doi: 10.1038/s41598-023-31601-z
Gault J., O’Hagan A. M., Cummins V., Murphy J., Vial T. (2011). Erosion management in inch beach, south west Ireland. Ocean Coast. Manage. 54, 930–942. doi: 10.1016/j.ocecoaman.2011.05.005
Gerritsen E., Kopsieker L., Naumann S., Röschel L., Davis M. (2021). “Using nature based solutions to foster synergies between biodiversity and climate: Missed chances and new opportunities for a sustainable future,” in Think2030 policy paper by the Institute for European Environmental Policy (IEEP) and the Ecologic Institute, 31.
Gittman R. K., Scyphers S. B., Smith C. S., Neylan I. P., Grabowski J. H. (2016). Ecological consequences of shoreline hardening: a meta-analysis. BioScience 66, 763–773. doi: 10.1093/biosci/biw091
Glarou M., Zrust M., Svendsen J. C. (2020). Using artificial-reef knowledge to enhance the ecological function of offshore wind turbine foundations: Implications for fish abundance and diversity. J. Mar. Sci. Eng. 8, 332. doi: 10.3390/jmse8050332
Glasby T. M., Connell S. D., Holloway M. G., Hewitt C. L. (2007). Nonindigenous biota on artificial structures: could habitat creation facilitate biological invasions? Mar. Biol. 151, 887–895. doi: 10.1007/s00227-006-0552-5
Halpern B. S., Frazier M., Afflerbach J., Lowndes J. S., Micheli F., O’Hara C., et al. (2019). Recent pace of change in human impact on the world’s ocean. Sci. Rep. 9, 1–8. doi: 10.1038/s41598-019-47201-9
Hammar L., Perry D., Gullström M. (2016). Offshore wind power for marine conservation. Open J. Mar. Sci. 6, 66–78. doi: 10.4236/ojms.2016.61007
Héral M., Deslous-Paoli J. M. (1991). “Oyster culture in European countries,” in Estuarine and marine bivalve mollusk culture. Ed. Menzel W. (Baton Rouge, USA: CRC Press), 154–190.
Herman P. M. J., Van Rees F. F. (2022). “Mapping reef forming north sea species,” in Report Deltares 11207716–000-ZKS-0001(Delft, the Netherlands), 40.
Hidu H., Chapman S., Soule P. W. (1975). Cultchless setting of European oysters, Ostrea edulis, using polished marble. Proc. Natl. Shellfish Ass 65, 13–14.
Hillier S. R., Sangha C. M., Plunkett B. A., Walden P. J. (1999). Long-term leaching of toxic trace metals from Portland cement concrete. Cem. Concr. Res. 29, 515–529. doi: 10.1016/S0008-8846(98)00200-2
Houziaux J. S., Kerckhof F., Degrendele K., Roche M., Norro A. (2008). “The Hinder banks: yet an important area for the Belgian marine biodiversity?,” in Belgian Science Policy, Scientific support plan for as sustainable development policy (SPSD II), Final Report, Research contract n° EV/36/45(Brussels, Belgium), 259.
Howie A. H., Bishop M. J. (2021). Contemporary oyster reef restoration: responding to a changing world. Front. Ecol. Evol. 9, 689915. doi: 10.3389/fevo.2021.689915
Hughes A., Bonacic K., Cameron T., Collins K., da Costa F., Debney A., et al. (2023). Site selection for European native oyster (Ostrea edulis) habitat restoration projects: An expert-derived consensus. Aquat. Conserv. 33, 721–736. doi: 10.1002/aqc.3917
Huisman B. J. A., Wijsman J. W. M., Arens S. M., Vertegaal C. T. M., van der Valk L., Van Donk S. C., et al. (2021). “10-years evaluation of the Sand Motor,” in Deltares report 11201431–003-ZKS-0014(Delft, The Netherlands), 84.
Jiménez J. A., Osorio A., Marino-Tapia I., Davidson M., Medina R., Kroon A., et al. (2007). Beach recreation planning using video-derived coastal state indicators. Coast. Eng. 54, 507–521. doi: 10.1016/j.coastaleng.2007.01.012
Johns C. M. (2019). Understanding barriers to green infrastructure policy and stormwater management in the City of Toronto: a shift from grey to green or policy layering and conversion? J. Environ. Plan Manage. 62, 1377–1401. doi: 10.1080/09640568.2018.1496072
Jones S. R., Mangun W. R. (2001). Beach nourishment and public policy after Hurricane Floyd: where do we go from here? Ocean Coast. Manage. 44, 207–220. doi: 10.1016/S0964-5691(01)00046-1
Kamermans P., Blanco A., Van Dalen P. (2020). “Sources of European flat oysters (Ostrea edulis L.) for restoration projects in the Dutch North Sea,” in Report Wageningen Marine Research C086/20 (Yerseke, The Netherlands), 56.
Kamermans P., Van Duren L., Kleissen F. (2018a). “European flat oysters on offshore wind farms: additional locations; Opportunities for the development of European flat oyster (Ostrea edulis) populations on planned wind farms and additional locations in the Dutch section of the North Sea,” in Wageningen Marine Research report C053/18(Yerseke, The Netherlands), 33. doi: 10.18174/456358
Kamermans P., Walles B., Kraan M., van Duren L., Kleissen F., van der Have T., et al. (2018b). Offshore wind farms as potential locations for flat oyster (Ostrea edulis) restoration in the Dutch North Sea. Sustainability 10, 3942. doi: 10.3390/su10113942
Kausche M., Adam F., Dahlhaus F., Grossmann J. (2018). Floating offshore wind - Economic and ecological challenges of a TLP solution. Renew. Energy 126, 270–280. doi: 10.1016/j.renene.2018.03.058
Korringa P. (1940). “Experiments and observations on swarming, pelagic life and setting in the European flat oyster, Ostrea edulis L,” in PhD thesis University of Amsterdam(Amsterdam, the Netherlands), 249.
Laboyrie P., van Koningsveld M., Aarninkhof S., Van Parys M., Lee M., Jensen A. A. C. (2018). “Dredging for sustainable infrastructure,” in CEDA / IADC CEDA / IADC(The Hague, The Netherlands), 336.
Lackey R. T. (2003). “Setting goals and objectives in managing for healthy ecosystems,” in Managing for healthy ecosystems. Eds. Rapport D. J., Lasley W. L., Rolston D. E., Nielsen N. O., Qualset C. O., Damania A. B. (Lewis Publishers, Florida, USA), 165–166.
Leadley P., Archer E., Bendandi B., Cavender-Bares J., Davalos L., DeClerck F., et al. (2022). Setting ambitious international restoration objectives for terrestrial ecosystems for 2030 and beyond. PloS Sustain. Transform 1, e0000039. doi: 10.1371/journal.pstr.0000039
Lengkeek W., Didderen K., Teunis M., Driessen F., Coolen J. W. P., Bos O. G., et al. (2017). “Eco-friendly design of scour protection: potential enhancement of ecological functioning in offshore wind farms,” in Report Bureau Waardenburg No 17–001(Culemborg, The Netherlands), 98.
Lindeboom H. J., Kouwenhoven H. J., Bergman M. J. N., Bouma S., Brasseur S., Daan R., et al. (2011). Short-term ecological effects of an offshore wind farm in the Dutch coastal zone; a compilation. Environ. Res. Lett. 6, 035101–035113. doi: 10.1088/1748-9326/6/3/035101
Linstone H., Turoff M. (1975). The Delphi method: techniques and applications (London, United Kingdom: Addison-Wesley), 616.
Martin D., Bertasi F., Colangelo M. A., de Vries M., Frost M., Hawkins S. J., et al. (2005). Ecological impact of coastal defence structures on sediments and mobile infauna: evaluating and forecasting consequences of unavoidable modifications of native habitats. Coast. Eng. 52, 1027–1051. doi: 10.1016/j.coastaleng.2005.09.006
Ministry of Economic Affairs (2018). Hollandse Kust (zuid) Wind Farm Zone - Wind Farm Sites III and IV - Project and Site Description (Utrecht, The Netherlands: Netherlands Enterprise Agency), 69.
Ministry of Infrastructure and Water Management (2022). Programma Noordzee 2022–2027 (The Netherlands: The Hague), 161.
Ministry of Infrastructure and Water Management, Ministry of Agriculture, Nature and Food Quality (2018). Mariene Strategie (deel 1) 2018–2024; Actualisatie van huidige milieutoestand, goede milieutoestand, milieudoelen en indicatoren (the Netherlands: The Hague), 268.
Moschella P. S., Abbiati M., Åberg P., Airoldi L., Anderson J. M., Bacchiocchi F., et al. (2005). Low-crested coastal defence structures as artificial habitats for marine life: using ecological criteria in design. Coast. Eng. 52, 1053–1071. doi: 10.1016/j.coastaleng.2005.09.014
Mulder J. P. M. (2022). Herstel onderwaternatuur en bevorderen passieve visserij/aquacultuur in windparken op zee. Een analyse van doelstellingen en mogelijkheden tot operationalisering. Rep. Mulder Coast. Consultancy (Oegstgeest, The Netherlands) 34.
Olsen O. T. (1883). The Piscatorial Atlas of the North Sea, English and St. George’s Channels, Illustrating the Fishing Ports, Boats, Gear, Species of Fish (How, Where, and When Caught), and Other Information Concerning Fish and Fisheries (London, United Kingdom: Taylor and Francis), 50.
O’Shaughnessy K. A., Hawkins S. J., Evans A. J., Hanley M. E., Lunt P., Thompson R. C., et al. (2020). Design catalogue for eco-engineering of coastal artificial structures: A multifunctional approach for stakeholders and end-users. Urban Ecosyst. 23, 431–443. doi: 10.1007/s11252-019-00924-z
Perkol-Finkel S., Sella I. (2014). “Ecologically active concrete for coastal and marine infrastructure: innovative matrices and designs,” in From Sea to shore – meeting the challenges of the Sea. Eds. Allops W., Burgess K. (ICE Publishing, London, United Kingdom), 1139–1149.
Petersen J. K., Malm T. (2006). Offshore windmill farms: Threats to or possibilities for the marine environment. Ambio 35, 75–80. doi: 10.1579/0044-7447(2006)35[75:OWFTTO]2.0.CO;2
Pioch S., Relini G., Souche J. C., Stive M. J. F., De Monbrison D., Nassif S., et al. (2018). Enhancing eco-engineering of coastal infrastructure with eco-design: moving from mitigation to integration. Ecol. Eng. 120, 574–584. doi: 10.1016/j.ecoleng.2018.05.034
Potet M., Fabien A., Chaudemanche S., Sebaibi N., Guillet T., Gachelin S., et al. (2021). Which concrete substrate suits you? ostrea edulis larval preferences and implications for shellfish restoration in Europe. Ecol. Eng. 162, 106159. doi: 10.1016/j.ecoleng.2021.106159
Price M. R., Toonen R. J. (2017). Scaling up restoration efforts in the pacific islands: a call for clear management objectives, targeted research to minimize uncertainty, and innovative solutions to a wicked problem. Pac Sci. 71, 391–399. doi: 10.2984/71.4.1
Santos C. F., Agardy T., Andrade F., Crowder L. B., Ehler C. N., Orbach M. K. (2021). Major challenges in developing marine spatial planning. Mar. Policy 132, 103248. doi: 10.1016/j.marpol.2018.08.032
Sas H., Didderen K., van der Have T., Kamermans P., van den Wijngaard K., Reuchlin E. (2019). “Recommendations for flat oyster restoration in the North Sea,” in Report ARK/WWF(The Netherlands), 34.
Saunders M. I., Doropoulos C., Bayraktarov E., Babcock R. C., Gorman D., Eger A. M., et al. (2020). Bright spots in coastal marine ecosystem restoration. Curr. Biol. 30, 1500–1510. doi: 10.1016/j.cub.2020.10.056
Shumway N., Bell-James J., Fitzsimons J. A., Foster R., Gillies C., Lovelock C. E. (2021). Policy solutions to facilitate restoration in coastal marine environments. Mar. Policy 134, 104789. doi: 10.1016/j.marpol.2021.104789
Smaal A., Kamermans P., Kleissen F., van Duren L. (2017). “Flat oysters on offshore wind farms,” in Report Wageningen Marine Research C052/17(Yerseke, The Netherlands), 54.
Smale D. A., Wernberg T., Oliver E. C. J., Thomsen M., Harvey B. P., Straub S. C., et al. (2019). Marine heatwaves threaten global biodiversity and the provision of ecosystem services. Nat. Clim. Change 9, 306–312. doi: 10.1038/s41558-019-0412-1
Smyth D., Kregting L., Elsäßer B., Kennedy R., Roberts D. (2016). Using particle dispersal models to assist in the conservation and recovery of the overexploited native oyster (Ostrea edulis) in an enclosed sea lough. J. Sea Res. 108, 50–59. doi: 10.1016/j.seares.2015.12.009
Soniat T. M., Broadhurst R. C., Haywood E. L. (1991). Alternatives to clamshell as cultch for oysters, and the use of gypsum for the production of cultchless oysters. J. Shellfish Res. 10, 405–410. doi: 10.2983/0730-8000(2005)24[483:ACOTEO]2.0.CO;2
Staatscourant (2016). Kavelbesluit I windenergiegebied Borssele. Nr.14428 (The Hague, The Netherlands: Koninkrijk der Nederlanden).
Staatscourant (2019). Kavelbesluit VI windenergiegebied Hollandse Kust (noord), Ministerie van Economische Zaken en Klimaat. Nr. 24545 (The Hague, The Netherlands: Koninkrijk der Nederlanden).
Staatscourant (2022). Kavelbesluit VI windenergiegebied Hollandse Kust (west), Ministerie van Economische Zaken en Klimaat. Nr. 4381 (The Hague, The Netherlands: Koninkrijk der Nederlanden).
Staatscourant (2023). Kennisgeving ontwerpkavelbesluiten kavels Alpha en Beta in het windenergiegebied IJmuiden Ver, Ministerie van Economische Zaken en Klimaat. Nr. 20377 (The Hague, The Netherlands: Koninkrijk der Nederlanden).
Stechele B., Barbut L., Lacroix G., van Duren L. A., Van Lancker V., Degraer S., et al. (2023a). Northern Europe’s suitability for offshore European flat oyster (Ostrea edulis) habitat restoration based on population dynamics. Front. Mar. Sci. 10, 1224346. doi: 10.3389/fmars.2023.1224346
Stechele B., Hughes A., Degraer S., Bossier P., Nevejan N. (2023b). Northern Europe’s suitability for flat oyster (Ostrea edulis) reef restoration: A mechanistic niche modelling approach. Aquat Conserv.: Mar. Freshw. Ecosyst. 33, 7. doi: 10.1002/aqc.3947
Strain E. M. A., Olabarria C., Mayer-Pinto M., Cumbo V., Morris R. L., Bugnot A. B., et al. (2018). Eco-engineering urban infrastructure for marine and coastal biodiversity: Which interventions have the greatest ecological benefit? J. Appl. Ecol. 55, 426–441. doi: 10.1111/1365-2664.12961
Suding K. N. (2011). Toward an era of restoration in ecology: successes, failures, and opportunities ahead. Annu. Rev. Ecol. Evol. Syst. 42, 465–487. doi: 10.1146/annurev-ecolsys-102710-145115
Sutherland J., Thomas I. (2011). The management of Pevensey shingle barrier. Ocean Coast. Manage. 54, 919–929. doi: 10.1016/j.ocecoaman.2011.07.004
Sutton-Grier A. E., Gittman R. K., Arkema K. K., Bennett R. O., Benoit J., Blitch S., et al. (2018). Investing in Natural and Nature-Based Infrastructure: Building better along our coasts. Sustainability 10, 523. doi: 10.3390/su10020523
Ter Hofstede R., Bouma T. J., Van Koningsveld M. (2023b). Five golden principles to advance marine reef restoration by linking science and industry. Front. Mar. Sci. 10, 1143242. doi: 10.3389/fmars.2023.1143242
Ter Hofstede R., Driessen F. M. F., Elzinga P. J., Van Koningsveld M., Schutter M. (2022). Offshore wind farms contribute to epibenthic biodiversity in the north Sea. J. Sea Res. 185, 102229. doi: 10.1016/j.seares.2022.102229
Ter Hofstede R., Williams G., Van Koningsveld M. (2023a). The potential impact of human interventions at different scales in offshore wind farms to promote flat oyster (Ostrea edulis) reef development in the southern north Sea. Aquat Living Resour. 36, 4. doi: 10.1051/alr/2023001
The Carbon Trust (2015). “Offshore wind industry review of GBs: identifying the key barriers to large scale commercialization of gravity-based structures in the offshore wind industry,” in Report The Carbon Trust(London, UK), 54.
Todd V. L. G., Todd I. B., Gardiner J. C., Morrin E. C. N., MacPherson N. A., DiMarzio N. A., et al. (2014). Review of impacts of marine dredging activities on marine mammals. ICES J. Mar. Sci. 72, 328–340. doi: 10.1093/icesjms/fsu187
Tonk L., Witte S., Kamermans P. (2020). Flat oyster (Ostrea edulis) settlement on scour protection material. Rep. Wageningen Mar. Res. C063/20 (Yerseke, The Netherlands), 56.
Tunnicliffe V., Metaxas A., Le J., Ramirez-Llodra E., Levin L. A. (2020). Strategic environmental goals and objectives: Setting the basis for environmental regulation of deep seabed mining. Mar. Policy 114, 103347. doi: 10.1016/j.marpol.2018.11.010
UN (2015). “Transforming our world: the 2030 agenda for sustainable development,” in Resolution A/RES/70/1 adopted by the General Assembly on 15 September 2015, (New York, USA: United Nations), 41.
UNFCCC (2018). “The paris agreement,” in Publication of the Paris Climate Change Conference – November 2015, COP21, (New York, USA: United Nations), 60.
Van der Veen H. H., Hulscher S. J. M. H., Knaapen M. A. F. (2006). Grain size dependency in the occurrence of sand waves. Ocean Dyn 56, 228–234. doi: 10.1007/s10236-005-0049-7
Van Duren L., Kamermans P., Kleissen F. (2022). Suitability for the development of flat oyster populations in new offshore wind farm zones and two search areas for restoration projects in the Dutch section of the North Sea. Deltares Rep. 11208312–002-ZKS-0001 (Delft, The Netherlands), 24.
Van Duren L., Kamermans P., Kleissen F. (2023). “Suitability for the development of flat oyster populations in future offshore wind farm zones in the Dutch section of the North Sea,” in Deltares report 11208312–003-ZKS-0001(Delft, The Netherlands), 39.
Van Egmond E. M., van Bodegom P. M., Berg M. P., Wijsman J. W. M., Leewis L., Janssen G. M., et al. (2018). A mega-nourishment creates novel habitat for intertidal macroinvertebrates by enhancing habitat relief of the sandy beach. Estuar. Coast. Shelf Sci. 207, 232–241. doi: 10.1016/j.ecss.2018.03.003
Van Koningsveld M. (2003). “Matching Specialist Knowledge with End User Needs. Bridging the gap between coastal science and coastal management,” in PhD Thesis, University of Twente(Enschede, The Netherlands), 173.
Van Koningsveld M., Davidson M. A., Huntley D. A. (2005). Matching science with coastal management needs: The search for appropriate coastal state indicators. J. Coast. Res. 21, 399–411. doi: 10.2112/03-0076.1
van Koningsveld M., Mulder J. P. M. (2004). Sustainable coastal policy developments in the Netherlands. A systematic approach revealed. J. Coast. Res. 202, 375–385. doi: 10.2112/1551-5036(2004)020[0375:SCPDIT]2.0.CO;2
Van Koningsveld M., Verheij H. J., Taneja P., De Vriend H. J. (Eds.) (2023). “Ports and Waterways - Navigating the changing world. Revision no 1349,” in Open textbook Delft University of Technology(Delft, The Netherlands), 517.
Van Leeuwen S., Tett P., Mills D., van der Molen J. (2015). Stratified and nonstratified areas in the North Sea: Long-term variability and biological and policy implications. J. Geophys. Res. 120, 4670–4686. doi: 10.1002/2014JC010485
Van Velzen B., Raaijmakers T., Mattieu J. (2014). “Scour protection GEMINI offshore wind farm,” in Deltares Technical Report unpublished(Delft, The Netherlands).
Vennix J. A. M., Akkermans H. A., Rouwette E. (1996). Group model-building to facilitate organizational change: an exploratory study. Syst. Dyn Rev. 12, 39–58. doi: 10.1002/(ISSN)1099-1727
Vozzo M. L., Doropoulos C., Silliman B. R., Steven A., Reeves S. E., Ter Hofstede R., et al. (2023). To restore coastal marine areas, we need to work across multiple habitats simultaneously. Proc. Natl. Acad. Sci. U.S.A. 120, e2300546120. doi: 10.1073/pnas.2300546120
Vrooman J., Schild G., Rodriguez A. G., Van Hest F. (2019). “North Sea wind farms: ecological risks and opportunities,” in Report North Sea Foundation(Utrecht, the Netherlands), 19.
Wilding T. A., Sayer M. D. J. (20002). The physical and chemical performance of artificial reef blocks made using quarry by-products. ICES J. Mar. Sci. 59, 250–257. doi: 10.1006/jmsc.2002.1267
Keywords: offshore wind, policy, restoration, nature-inclusive, infrastructure, oyster
Citation: ter Hofstede R and van Koningsveld M (2024) Defining operational objectives for nature-inclusive marine infrastructure to achieve system-scale impact. Front. Mar. Sci. 11:1358851. doi: 10.3389/fmars.2024.1358851
Received: 20 December 2023; Accepted: 20 May 2024;
Published: 06 June 2024.
Edited by:
Stelios Katsanevakis, University of the Aegean, GreeceReviewed by:
Sofia Spyridonidou, Aristotle University of Thessaloniki, GreeceSimonetta Fraschetti, University of Naples Federico II, Italy
Copyright © 2024 ter Hofstede and van Koningsveld. This is an open-access article distributed under the terms of the Creative Commons Attribution License (CC BY). The use, distribution or reproduction in other forums is permitted, provided the original author(s) and the copyright owner(s) are credited and that the original publication in this journal is cited, in accordance with accepted academic practice. No use, distribution or reproduction is permitted which does not comply with these terms.
*Correspondence: Remment ter Hofstede, ci50ZXJob2ZzdGVkZUB0dWRlbGZ0Lm5s