- Key Laboratory of the Coastal and Wetland Ecosystems (Xiamen University), Ministry of Education, College of the Environment and Ecology, Xiamen University, Xiamen, China
Background: In natural mangrove communities, mangrove species are often distributed zonally. Leaf-eating crabs are one of the most abundant and iconic arboreal brachyurans in mangrove forests, but variation in the composition of crab diets in different mangrove tidal zones is unknown.
Methods: To determine the contributions of mangrove leaves and other organic carbon (C) sources to leaf-eating crab diets, dual stable C and nitrogen (N) isotope signatures (δ13C and 1δ5N) were used in a Bayesian stable isotope mixing model. We conducted experiments at various tidal levels in the Dongzhaigang Bay National Natural Reserve in China. We analyzed δ13C and δ15N of leaf-eating crabs, mangrove leaves, sediment organic matter (SOM), and animal tissues (prey).
Results: The food composition of the dominant crab species, Parasesarma continentale, exhibited significant differences among the four tidal zones. From the margin to the high tide zone, the main food source shifted from predominantly mangrove leaves and SOM to primarily SOM and animal tissues. We observed a significant negative relationship between the C/N ratios of mangrove leaves and the proportion of leaves consumed by leaf-eating crabs. Additionally, as the tidal level increased, the C/N ratio of mangrove leaves also increased, whereas the proportion of leaves consumed by crabs decreased.
Conclusion: Leaf-eating crab diets vary significantly across tidal zones, highlighting the importance of considering tidal zone differentiation when studying consumer diets in mangrove ecosystems.
Introduction
Many benthic invertebrates rely, either partially or entirely, on mangrove habitats to complete their life cycles (Basyuni et al., 2022). They participate in the trophic dynamics of mangrove ecosystems through nutrient cycling, bioturbation, and as prey or predator (Bui and Lee, 2014; Medina-Contreras et al., 2022). Among benthic invertebrates in mangrove forests, leaf-eating crabs play a pivotal role as “ecosystem engineers” (Araújo et al., 2012; Gao and Lee, 2022; Medina-Contreras et al., 2022) because of their top-down role in food web energy flow (Swindells et al., 2017; Xie et al., 2022). Crab activities include feeding, burrowing, and crawling (Lee, 1997). Leaf-eating crabs have downstream effects on mangroves by consuming a substantial portion of annual mangrove primary production (Robertson and Daniel, 1989; Lee, 2008; Medina-Contreras et al., 2022). In the Indo-West Pacific region, species of the Sesarmidae dominate the intertidal zone and rely mainly on mangrove leaves as their food source (Kristensen et al., 1995; Thongtham and Kristensen, 2005; Bui and Lee, 2014; MacKenzie et al., 2020). High leaf consumption rates result in rapid utilization of nutrients in mangrove leaves before they are exported by the tide (Nagelkerken et al., 2008). For instance, leaf-eating crabs living in mangrove forests along the semi-arid coast of Brazil consume more sediment organic matter (SOM) and omnivorous invertebrates to replenish nitrogen, in addition to feeding on mangrove leaf litter because of its high C/N ratio (Pereira et al., 2019). Several studies have shown that leaf-eating crabs supplement their nitrogen-poor diet of leaves by consuming sediment-associated bacteria, microbenthos and fungi, and animal tissues (Kristensen et al., 2010; Oakes et al., 2010; Medina-Contreras et al., 2022). In most mangrove environments, leaf-eating crabs contribute substantially to the detritus-based food chain, resulting in important effects on nutrient cycling, energy flow, litter decomposition, and export of mangrove production (Robertson, 1986; Nordhaus et al., 2006; Chen et al., 2017; Medina-Contreras et al., 2022).
Previous experiments typically explored crab food sources at the estuarine level, primarily in areas dominated by a single mangrove species (Thongtham et al., 2008; Tue et al., 2012; Medina-Contreras et al., 2022). However, mangrove species in natural communities often exhibit zonal stratification (Duke et al., 1998). According to Feng et al. (2017), changes in the structure of mangrove vegetation communities can affect the availability and palatability of food sources for leaf-eating crabs. Van Hieu et al. (2020) discovered that crabs primarily feed on phytoplankton and benthic microalgae in mudflats, while in Sonneratia caseolaris and Kandelia candel forests, their diet consists mainly of benthic microalgae and sediment organic matter. Past studies have shown differences in the food sources of crabs in mangrove margin areas and forest interior areas (Kruitwagen et al., 2010). However, it remains unclear whether tidal zones composed of different plant communities affect the food composition of leaf-eating crabs.
Previously, it was possible to determine the food composition of leaf-eating crabs by analyzing gastrointestinal tract content (Thongtham and Kristensen, 2005; Kristensen, 2008). Analyzing gastrointestinal tract content has limitations, however, and offers insight into consumer diet composition only in the short term and does not reveal long-term dietary preferences (Gao et al., 2018). Furthermore, the gastrointestinal contents of crabs are influenced by factors such as food digestion rates, food size, and the content of nitrogen, vitamins, and fatty acids in the food (Wolcott and O’Connor, 1992). Stable isotope tracing methods can precisely measure feeding over extended periods and therefore provide a more realistic view of energy flow among organisms in a food web (Mieczan et al., 2015; Preciado et al., 2017; Donázar-Aramendía et al., 2019; Medina-Contreras et al., 2022). Bayesian stable isotope mixing models (SIMMR) based on the Bayesian statistical framework also have been developed to use statistical distributions to characterize the isotopic values of food sources and consumers based on mass-balance mixing models (Moore and Semmens, 2008; Parnell et al., 2010, 2013; Stock et al., 2018; Medina-Contreras et al., 2022). Furthermore, SIMMR can be used to investigate the effects of different food sources on numerous species or populations (Parnell et al., 2013; Stock et al., 2018). However, it is restricted to evaluating the contributions of various foods within the same ecosystem (Parnell et al., 2013).
In this study, we used stable isotopes and C/N ratios to determine variations in food sources among the dominant invertebrate taxon in Dongzhaigang Bay’s mangrove ecosystem: the leaf-feeding crab (P. continentale), across different tidal zones. Given the significant ecological role played by leaf-feeding crabs, there is a pressing need for a comprehensive investigation into the intricate interrelationships between leaf-feeding crabs and the tidal zones of mangrove ecosystems. Addressing this knowledge gap is crucial for advancing our comprehension of the dynamics in crab diet.
Materials and methods
Study area
The Dongzhaigang Bay National Natural Reserve, the first mangrove wetland nature reserve established in China, is situated in the northeast of Hainan Island, China (19°55′N, 110°36’E) (Figure 1). Importantly, the mangrove forest in Dongzhaigang Bay remains pristine and is devoid of human-induced influences, such as urban or agricultural runoff. No other systems, such as seagrasses or salt marshes, affect the mangrove forests. The bay covers approximately 3,337 hectares, and this reserve boasts one of the most extensive mangrove forests in China, accounting for 25 distinct mangrove species (Xiong et al., 2017). The mangrove forests within the reserve are predominantly composed of naturally occurring mangrove species. Avicennia marina is a pioneer species at forest margins; Rhizophora stylosa is in the low intertidal or fringe zone, with frequent flooding by tidal waters; Bruguiera sexangula inhabits the intermediate or middle intertidal area; and Ceriops tagal is in the high intertidal or landward zone, where tidal inundation rarely occurs. Thus, Dongzhaigang Bay presents a natural laboratory for investigating the food habits of leaf-eating crabs in mangrove forests across diverse intertidal locations. Notably, the mangrove forest zones lack an understory, and in each zone, continuous mangrove leaf litter is the dominant source of litter. Dongzhaigang Bay experiences mixed semidiurnal tides, featuring an average tidal range of 1.6 to 1.8 meters. The bay undergoes two high tides and two low tides of different sizes every lunar day (Zhang et al., 2020). The duration of inundation is short during dry season tides and long during wet season tides. The prevailing climate in the region is characterized as a tropical maritime monsoon, marked by distinct rainy and dry seasons. The rainy season spans from May to October, with an average monthly rainfall of 222 mm and an average monthly air temperature of 27.5°C. Conversely, the dry season persists from November to April, which is accompanied by an average monthly rainfall of 54 mm and an average monthly air temperature of 20.8°C. Salinity levels in the bay exhibit seasonal variations, ranging from 24.9 to 31.8 during the rainy season and from 29.8 to 32.35 during the dry season.
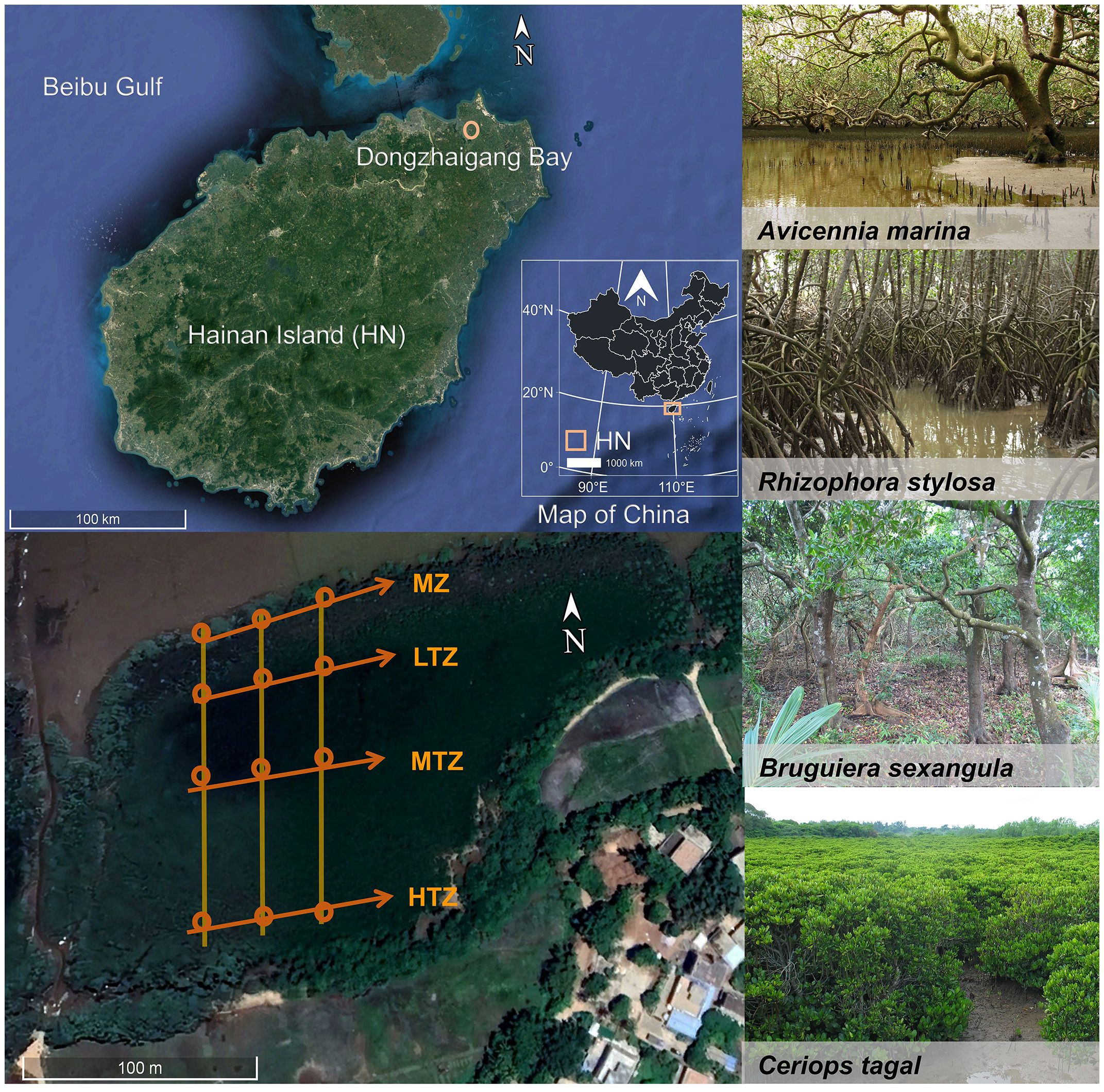
Figure 1 Sampling sites along transects in four intertidal zones of the mangrove ecosystem in Dongzhaigang Bay, Hainan Island, China. The locations of sampling sites are represented by circles in the figure. The dominant species in each zone are as follows: MZ, Margin zone (Avicennia marina); LTZ, low intertidal zone (Rhizophora stylosa); MTZ, middle intertidal zone (Bruguiera sexangula); HTZ, high intertidal zone (Ceriops tagal).
Sampling
In this study, samples were collected monthly during November 2007 and February 2008 (dry season) and May and August 2007 (rainy season). This approach yielded a comprehensive dataset enabling the capture of seasonal and tidal zone variations in the food sources of leaf-feeding crabs. Three repetitive north-south transects were established, each extending approximately 150 meters in length and spaced approximately 50 meters apart, from the mangrove margin to the highest tide mark. Along each transect, sampling sites were strategically chosen across four distinct tidal zones: (1) the margin of the mangrove zone (MZ), where A. marina is the pioneer species; (2) the low intertidal zone (LTZ), where R. stylosa dominates; (3) the middle intertidal zone (MTZ), where B. sexangula is the dominant species; and (4) the high intertidal zone (HTZ), where C. tagal prevails (Figure 1). One sampling plot was designated at intervals of 0 m (MZ), 50 m (LTZ), 100 m (MTZ), and 150 m (HTZ) within each tidal zone along each transect. We conducted visual counts of crab populations (1 m × 1 m) within each mangrove tidal zone. The crabs from each tidal zone in three transects were pooled for combined analysis. In our field observations, we’ve noted that leaf-eating crabs (Parasesarma continentale) consume not just mangrove leaves but also soil organic matter (SOM) and animal tissues, including those from Uca arcuata and U. borealis. During low tide, three individuals of the dominant crab species, Parasesarma continentale, were manually captured within each sampling plot. We subsequently cleaned, froze, and stored the leaf-eating crabs at -20°C before transporting them back to the laboratory. Additionally, we collected a total of three individuals of U. arcuata and U. borealis from each sampling plot. Similarly, the samples underwent cleaning, freezing, and storage at -20°C before being transported back to the laboratory. After thawing the crab samples in the laboratory, muscle tissue was extracted. For P. continentale, the chelae from three individuals at each sampling plot were utilized for composite sample analysis. As for U. arcuata and U. borealis, the gut system was initially removed, and muscle tissue from the body was used, total of three individuals from each sampling plot were pooled to form a single sample. In each sampling plot, we collected three brown mangrove leaves at the stage of decomposition preferred by leaf-eating crabs for composite sample analysis (Lee, 1997; Kristensen et al., 2017). Then, after removing branches, leaves, benthic organisms, animal carcasses, and small rocks, we repeatedly scraped three samples of sediment organic matter (SOM) containing bacteria, microalgae, and fungi at each sampling plot for composite sample analysis.
Sample preparation for stable isotope and nutrient content analyses
Before processing, both leaf and consumer samples underwent a thorough washing with deionized water. Subsequently, all samples were subjected to a drying process at 60°C for 48 h. The dried tissues were then meticulously ground to a fine powder using a mortar and pestle. SOM and plant leaves were analyzed in two subsamples. For δ13C analysis, subsamples were soaked in 10% HCl to eliminate carbonates. In contrast, subsamples designated for δ15N analysis did not undergo acid treatment to prevent any impact on δ15N (Ryba and Burgess, 2002). From each sample, 0.5–1.0 mg was carefully folded into a tin capsule for subsequent analysis. An isotope ratio mass spectrometer (DELTA plus XP, Thermo Finnigan, CA, USA) was used to determine sample δ13C and δ15N with a standard deviation of 0.3 per mil for both C and N. Total organic C (TOC) and total N (TN) contents were determined by an elemental analyzer (FLASH EA 1112, Thermo, CA, USA) and used to calculate the C/N ratio. Percentage values of C and N were measured using atropine (Carlo Erba, Milano) as the reference material. The isotopic values were determined against in-house standards, which were calibrated using internationally recognized reference materials: L-glutamic acid USGS 40 (IAEA, Vienna, Austria) and sugar (IAEA-CH-6) for δ13C, and L-glutamic acid USGS 40 and potassium nitrate IAEA-NO3 for δ15N. The uncertainty associated with stable isotopic determinations was 0.3‰ for both δ13C and δ15N. The detection limit of the method for C and N was 0.6 mmol and 0.9 mmol, respectively. The stable isotope contents were expressed as δ values, following the equation:
where X represents either isotope δ13C or δ15N and R is the 13C/12C ratio for C or 15N/14N for N, using Vienna Pee Dee Belemnite and N2 in air as the standards, respectively.
Bayesian stable isotope mixing models
The Bayesian SIMMR, which was developed by Parnell and Inger (2016), served as the analytical framework for estimating the contributions of potential food to the diets of leaf-eating crabs (Parnell et al., 2013). This model leverages the Markov chain Monte Carlo (MCMC) method, incorporating a posterior distribution based on 10,000 iterations in this study. The MCMC technique uses random sampling based on prior knowledge of the proportional contributions from different foods and the probability distribution of each input variable (Shang et al., 2020). To assess the model’s performance, we employed Gelman–Rubin convergence statistics, with a diagnostic value below 1.1 indicating a satisfactory run (Gelman et al., 2013).
In instances in which a plethora of food sources existed, the model’s ability to differentiate between similar sources may have diminished, subsequently amplifying the uncertainty in model results (Phillips et al., 2014). To address this issue, similar food sources can be combined based on a priori information such as the isotopic space (Isospace) location of the food source and the results of cluster analysis. Alternatively, for foods that are close ecologically, SIMMR provides a ‘combine_sources’ function that combines similar sources a posteriori based on the analysis results. Through field observations and literature searches, we identified and selected three primary sources for the model: mangrove leaves, SOM, and animal tissues (prey). This careful selection was based on their ecological relevance and their distinct isotopic signatures, ensuring a robust and accurate representation of the leaf-eating crab’s dietary contributions.
Bayesian mixing models assess the likelihood of the proportion of specific foods in a diet by assessing the mean and standard deviation of the stable isotope ratios of producers, as well as the trophic enrichment factor (TEF) for each isotope ratio (Moore and Semmens, 2008; Caut et al., 2009; Ward et al., 2010; Parnell et al., 2013). In our study, we adjusted the isotopic shifts from various food sources to consumers using the “correction_means” parameter in SIMMR, based on mean values of TEF. For leaf-eating crabs, TEF was established as 3.3‰ ± 0.26‰ for δ15N and 1.3‰ ± 0.3‰ for δ13C (McCutchan et al., 2003).
Statistical analyses
Before analysis, we checked the variance of the residuals for normality and homoscedasticity using Shapiro-Wilk’s (p > 0.05) and Levene’s tests (p > 0.05). Student’s t-tests were used to compare the differences in stable isotope signatures of leaf-eating crabs between rainy and dry seasons, as well as the differences in stable isotopes of their food sources. We used one-way ANOVA to compare differences in stable isotopes of leaf-feeding crabs across tidal zones in the rainy and dry seasons. To examine variations in the stable isotope signatures of diverse food sources among different mangrove zones, we combined rainy and dry season data in a one-way ANOVA and Tukey HSD as post hoc test when differences with ANOVA were detected. We used linear regression analysis to investigate the relationships between C/N ratio of mangrove leaves and their contribution to food, and 95% confidence intervals were determined for the regression. The explanatory power of simple linear regression models was assessed using the modified coefficient of determination R2. The significance level of linear regressions was set at 0.05 (p< 0.05). All reported values are expressed as the mean ± SD.
All statistical analyses and data visualization were carried out using R software v4.0.1 (R Core Team, 2017), with a focus on utilizing the ggplot2 and ggpubr packages for robust analysis and effective data representation (Wickham, 2016).
Results
Carbon and nitrogen isotopic compositions of leaf-eating crabs and food sources
The relative abundance of the leaf-eating crabs (P. continentale) exhibited variation among zones, with the highest densities observed in the MZ and LTZ, followed by the MTZ, and the lowest densities recorded in the HTZ (Figure 2). Similarly, we noted variability in prey relative abundance across different zones, with the highest densities found in the LTZ and MTZ, followed by the MZ, and the lowest densities in the HTZ (Figure 2). The stable isotope values for leaf-eating crabs in different mangrove zones are shown in Figure 3. In all four zones, the δ13C signatures of leaf-eating crabs were relatively higher compared to those of mangrove leaves, while the δ15N values of the mangrove leaves were comparatively lower than those of the leaf-eating crabs. Additionally, the δ13C and δ15N values of SOM were comparatively lower than those of the leaf-eating crabs. Conversely, the δ13C and δ15N values of prey were both relatively higher compared to those of the leaf-eating crabs.
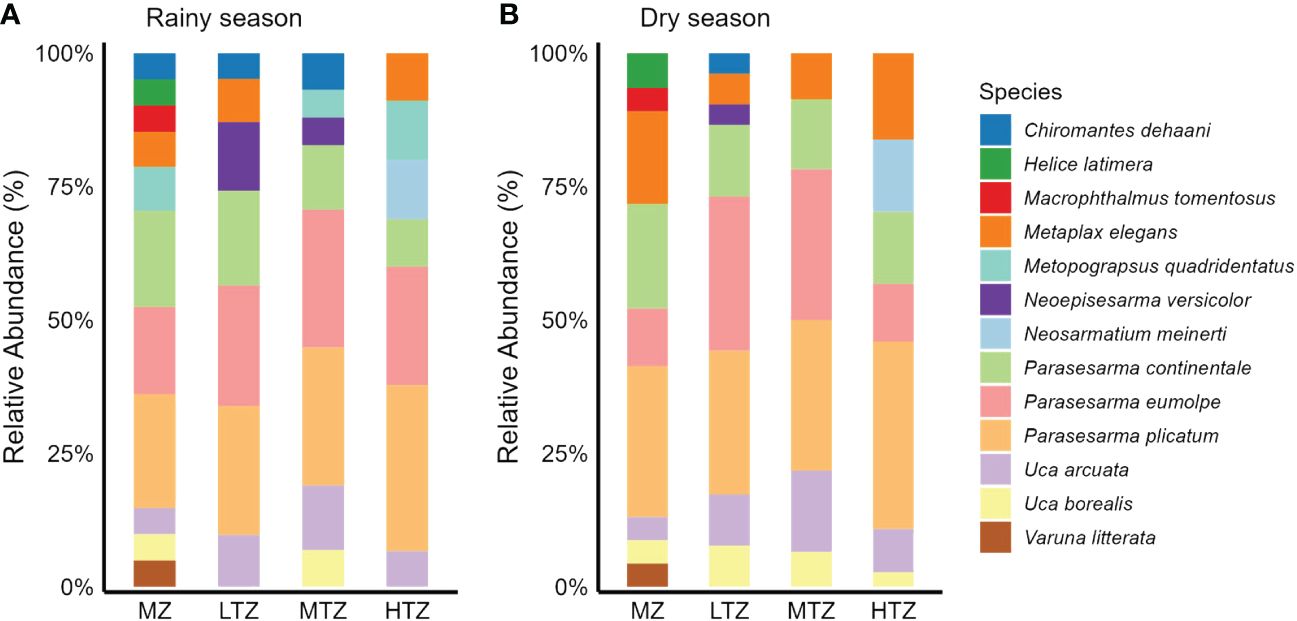
Figure 2 Relative abundance of crabs in rainy and dry seasons at different intertidal zones (MZ, margin zone; LTZ, low intertidal zone; MTZ, middle intertidal zone; HTZ, high intertidal zone) of a mangrove forest in Dongzhaigang Bay, Hainan Island, China. (A) Rainy season; (B) Dry season.
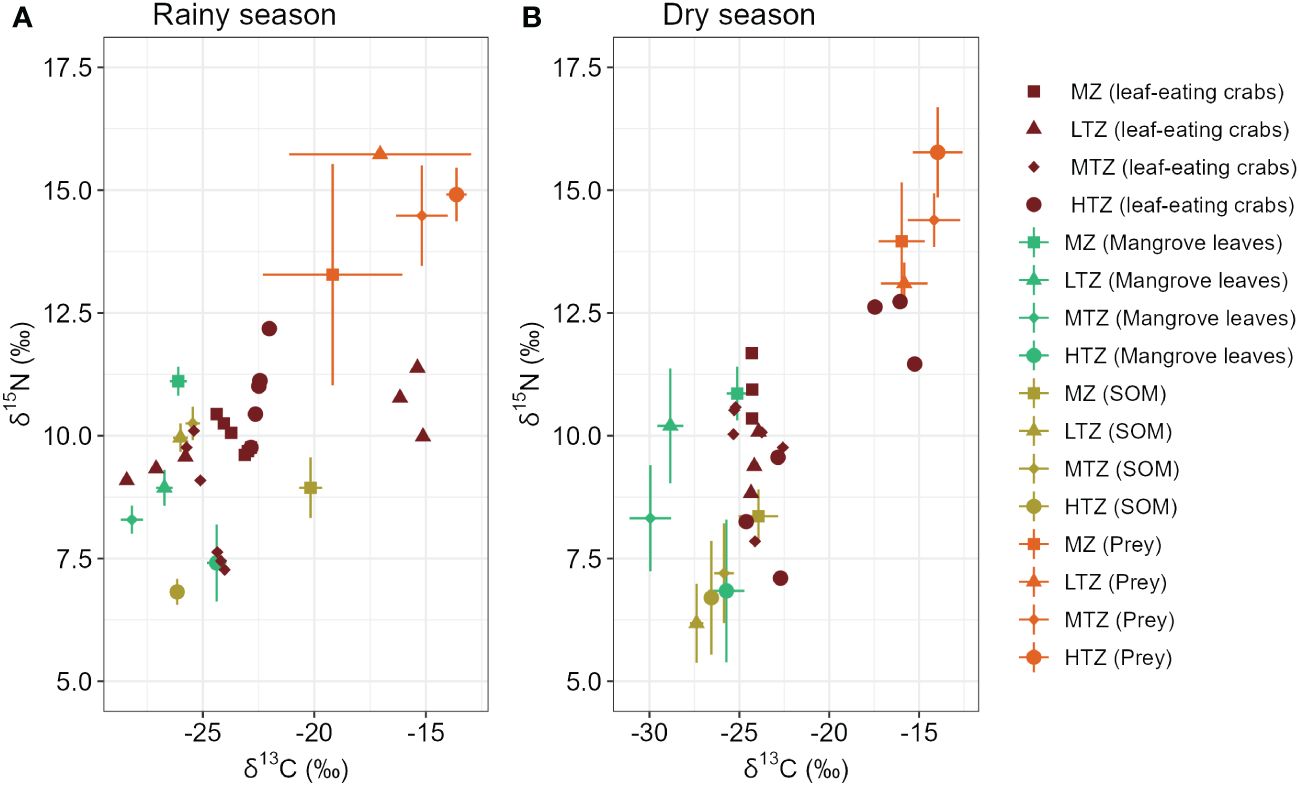
Figure 3 Mean ± SD δ13C and δ15N values of leaf-eating crabs, leaves of mangroves, sediment organic matter (SOM), and prey (animal tissues) in different intertidal zones (MZ, Margin zone; LTZ, low intertidal zone; MTZ, middle intertidal zone; HTZ, high intertidal zone) of a mangrove forest in Dongzhaigang Bay, Hainan Island, China (n = 6). (A) Rainy season; (B) Dry season. The δ13C and δ15N values were adjusted based on Trophic Enrichment Factor (TEF).
In the rainy season, there were no significant differences in the δ13C values of leaf-eating crabs across tidal intervals (p = 0.315, ANOVA) (Supplementary Table S1). However, the δ15N values showed significant differences (p = 0.002, ANOVA) (Supplementary Table S1). Post hoc analyses revealed that the MTZ differed significantly from both the HTZ (p< 0.001) and the LTZ (p = 0.042) (Supplementary Table S2). Nonetheless, no significant differences were observed among the other tidal zones. In the dry season, significant variations were observed in the δ13C values of leaf-eating crabs across tidal zones (p = 0.024, ANOVA) (Supplementary Table S1). Post hoc analyses indicated a significant difference between the MTZ and HTZ (p = 0.032). However, no significant differences were observed among the other tidal zones. Interestingly, no statistical difference was detected in the δ15N signature of leaf-eating crabs among the zones (p = 0.622, ANOVA) (Supplementary Table S1). The δ13C values of leaf-eating crabs exhibited significant seasonal variations (Supplementary Table S3), with significant differences observed in MZ (p = 0.029, t-test). However, the δ15N values of leaf-eating crabs showed no significant differences between seasons across the four intertidal zones.
Isotopic signatures of the available food sources were categorized into mangrove leaves, SOM, and prey tissues (Figure 3). The δ13C values of mangrove leaves exhibited significant seasonal variations (Supplementary Table S3), with notable differences observed in MZ (p = 0.003, t-test), LTZ (p< 0.001, t-test), MTZ (p = 0.010, t-test), and HTZ (p = 0.017, t-test). However, the δ15N values of mangrove leaves only showed significant differences in LTZ across different seasons (p = 0.041, t-test). Similarly, the δ13C values of SOM varied significantly among different seasons, with notable distinctions observed in MZ (p = 0.015, t-test), LTZ (p = 0.002, t-test), except for MTZ (p > 0.05, t-test), and HTZ (p = 0.042, t-test). However, the δ15N values of SOM only exhibited significant differences in LTZ and MTZ among different seasons (p = 0.011, p = 0.028, t-test). The δ13C and δ15N values of prey showed no significant differences between seasons across the four intertidal zones.
Despite variations in dry and rainy seasons, the trends of δ13C content and δ15N values across different intertidal zones remain consistent. Therefore, to assess differences in food sources across tidal zones, we pooled data from both seasons and conducted ANOVA analysis and post hoc tests (Figures 4A, B). The results showed significant differences in the δ13C values of mangrove leaves between the MZ and the LTZ and MTZ, as well as between the HTZ and the LTZ and MTZ. Due to differences in mangrove species, the δ15N values of mangrove leaves decrease with increasing tidal levels. The δ13C values of mangrove leaves and SOM were significantly different in this study (p< 0.001, ANOVA), with SOM enriched by 1.2‰ to 5.9‰ compared with mangrove leaves. The δ13C values of SOM in the MZ were significantly higher than those in the other zones. We did not detect any statistically significant difference in the δ15N values between different tidal zones. The δ13C values of prey in the MZ differed significantly from those in the MTZ and HTZ. Additionally, the δ15N values of prey increased with increasing tide level, but we did not find any statistically significant difference in the δ15N signatures among different tidal zones.
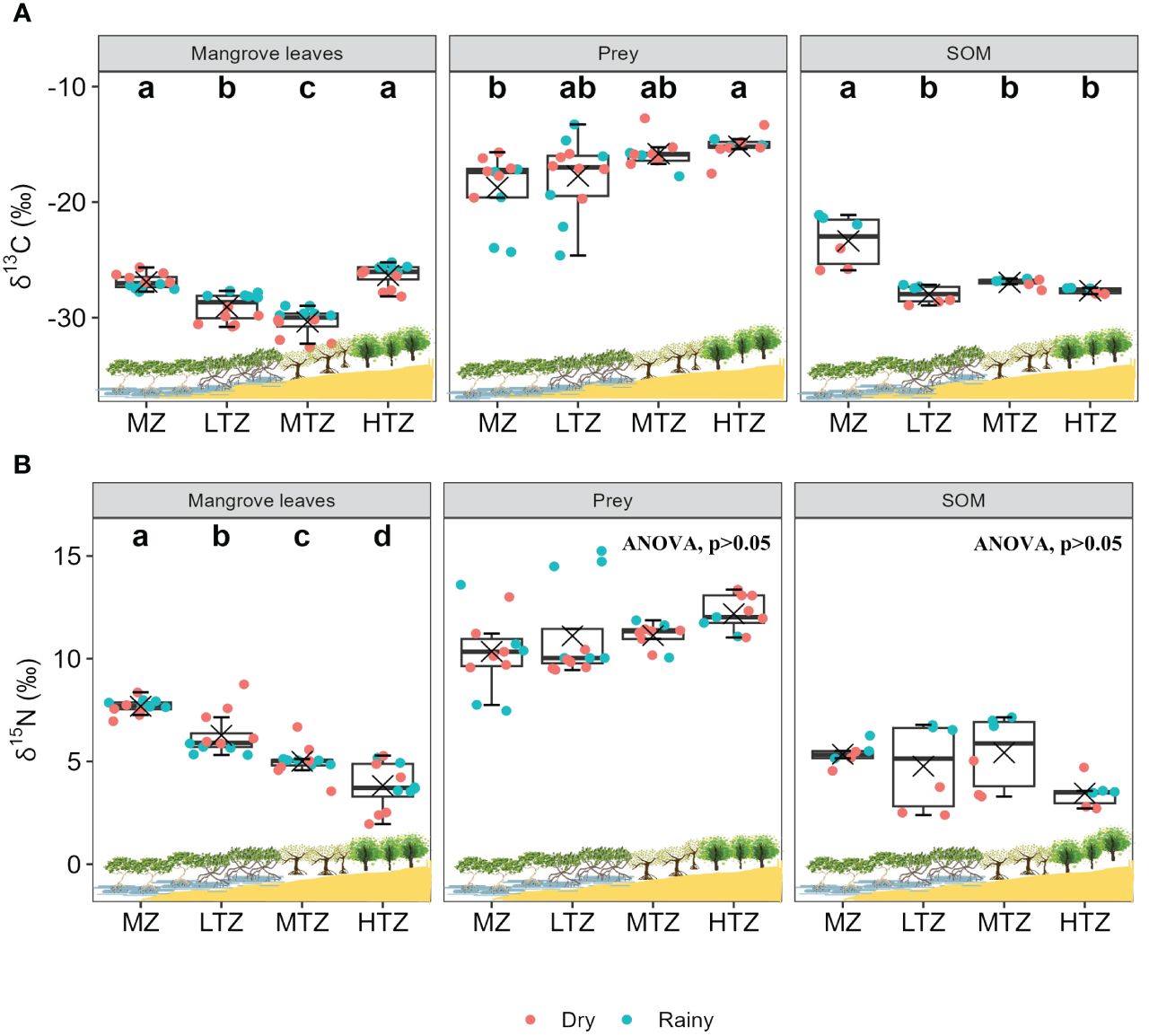
Figure 4 Variations in dry and rainy season combinations of data of (A) δ13C and (B) δ15N values of potential food resources of leaf-eating crabs, including leaf litter of dominant mangrove species (Avicennia marina [MZ], Rhizophora stylosa [LTZ], Bruguiera sexangula [MTZ], and Ceriops tagal [HTZ]), prey (animal tissues), and sediment organic matter (SOM), at different intertidal zones (MZ, margin zone; LTZ, low intertidal zone; MTZ, middle intertidal zone; HTZ, high intertidal zone) of a mangrove forest in Dongzhaigang Bay, Hainan Island, China. Different lowercase letters indicate significant differences in isotope values of a food resource among intertidal zones (post hoc tests, p< 0.05). Boxes extend from the 25th to 75th percentile, with the black horizontal line indicating the median and the saltire inside boxes indicating the mean. The whiskers extending from boxes represent the highest and lowest values.
Food contribution to leaf-eating crab’s diets
The relative contributions of different sources to the diets of leaf-eating crabs as determined by the SIMMR (Figure 5). The highest contribution of mangrove leaves to diets was in the MZ and the lowest was in the HTZ. Mangrove leaves were the primary source of food for leaf-eating crabs in the MZ, especially during the dry season, when leaves accounted for 75.2% of their diet (Figure 5). In the LTZ, the diet mainly consisted of mangrove leaves (48.4% in the rainy season; 30.7% in the dry season) and SOM (41.7% in the rainy season; 39.8% in the dry season). Similarly, in the MTZ, the diet primarily comprised mangrove leaves (63.7% in the rainy season; 43.8% in the dry season) and SOM (24.5% in the rainy season; 28.0% in the dry season). In contrast, the HTZ exhibited the lowest consumption of mangrove leaves (35.6% in the rainy season; 29.7% in the dry season), with prey (24.7% in the rainy season; 41.7% in the dry season) and SOM (39.7% in the rainy season; 28.6% in the dry season) constituting the major sources of crab food. Furthermore, reliance on prey in the crab diet was higher in the dry season compared to the rainy season across all tidal zones.
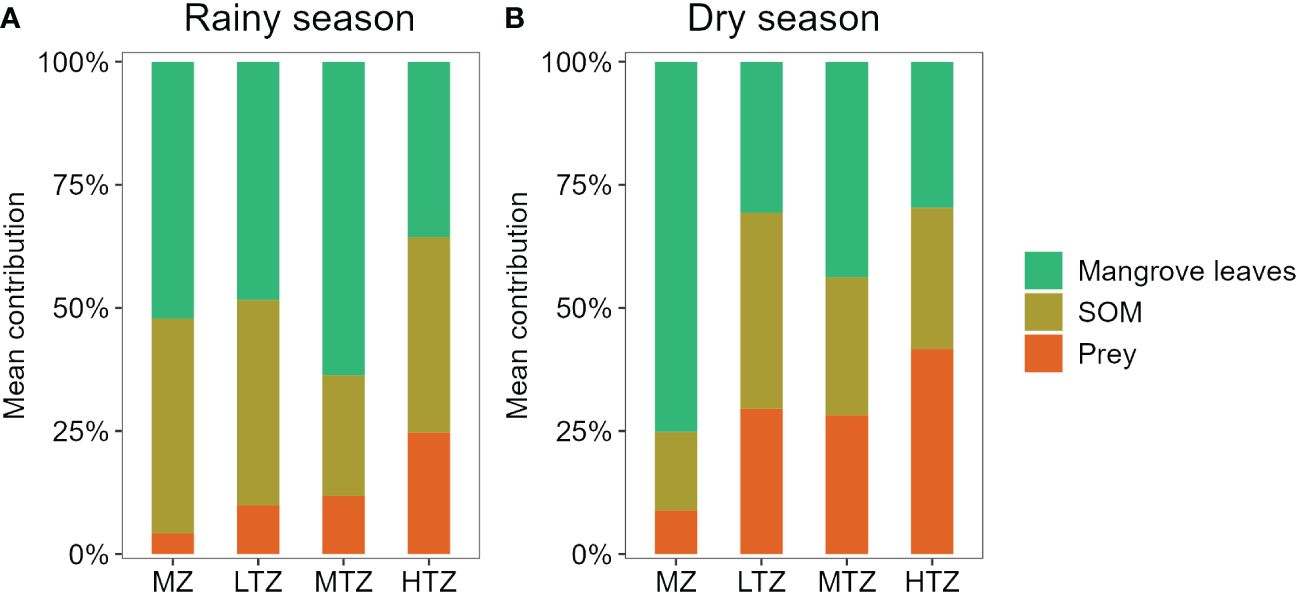
Figure 5 Contributions of different food sources to diets of leaf-eating crabs at different intertidal zones (MZ, margin zone; LTZ, low intertidal zone; MTZ, middle intertidal zone; HTZ, high intertidal zone) of a mangrove forest in Dongzhaigang Bay, Hainan Island, China. SOM, sediment organic matter. (A) Rainy season; (B) Dry season.
Relations between carbon to nitrogen ratio and food contribution of mangrove leaves
The linear regression between C/N ratios of mangrove leaves and the proportion of leaves that contributed to diets of leaf-feeding crabs across rainy and dry seasons at different intertidal zones indicated a significantly negative relationship (Figure 6; regression equation: y = 71.7 – 0.598x, R2 = 0.49, p = 0.032). The 95% confidence interval for the regression coefficient was from –1.125 to –0.072. In the MZ, mangrove leaves had the lowest C/N ratios of 17.84 ± 0.95 and 21.02 ± 1.53 in wet and dry seasons, respectively, and the highest contributions to diet of 52.2% and 75.2% in wet and dry seasons, respectively (Table 1). In the MTZ, mangrove leaves with a C/N ratio of 29.05 ± 0.36 contributed 63.7% to diets in the wet season and those with ratio of 29.67 ± 1.30 contributed 43.8% in the dry season (Table 1). In the LTZ, mangrove leaves with a C/N ratio of 44.83 ± 0.22 contributed 48.4% to diets in the rainy season and those with a ratio of 44.17 ± 3.02 contributed 30.7% in the dry season (Table 1). In the HTZ, mangrove leaves had the highest C/N ratios, with values of 70.37 ± 2.21 in the rainy season and 68.37 ± 0.72 in the dry season. They contributed the least to crab diets, accounting for 35.6% and 29.7% in the rainy and dry seasons, respectively.
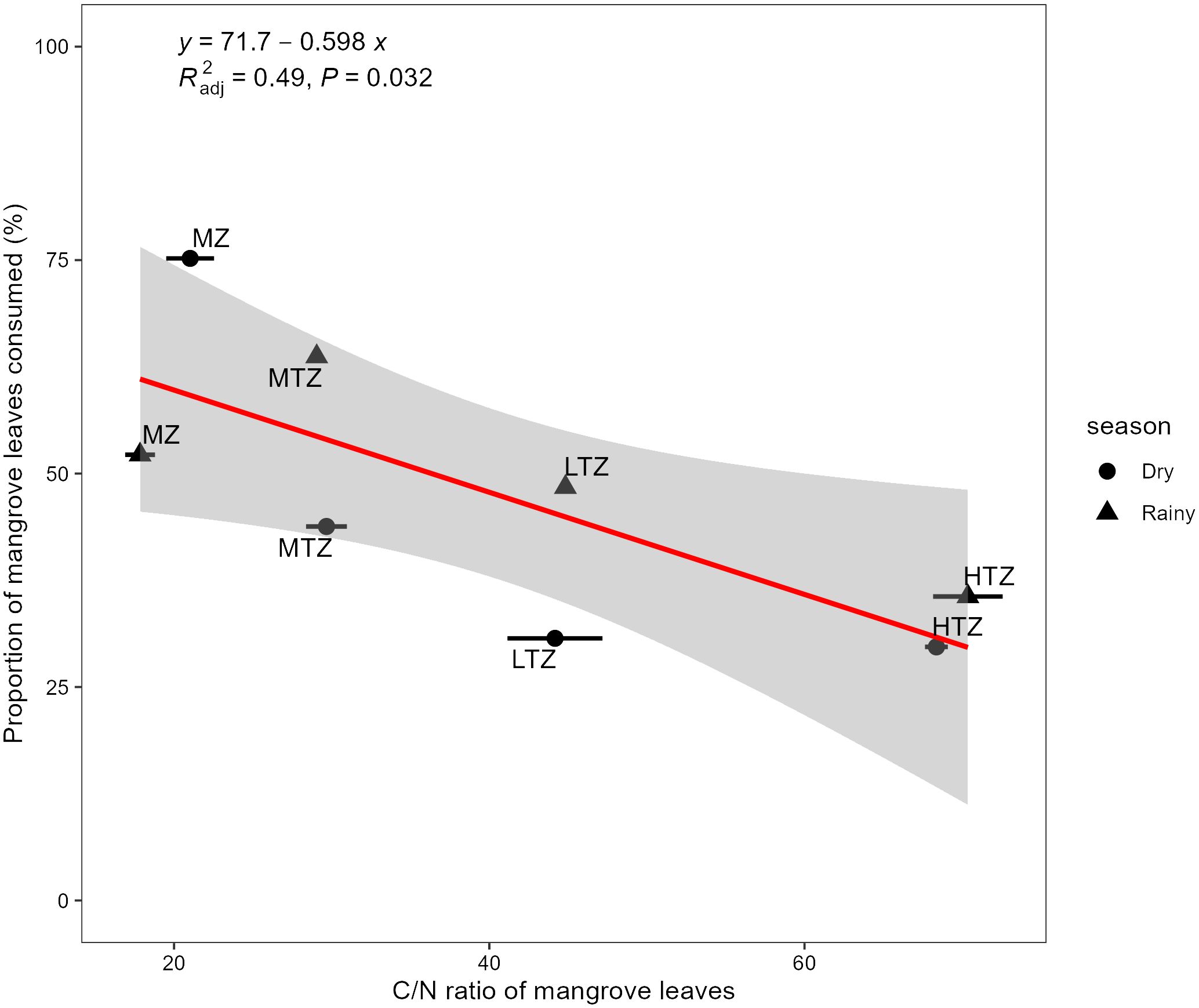
Figure 6 Linear regression between mangrove leaf carbon/nitrogen (C/N) ratio (mean ± SD) and the proportion ingested by leaf-eating crabs (mean ± SD, n = 6) across rainy and dry seasons at different intertidal zones (MZ, margin zone; LTZ, low intertidal zone; MTZ, middle intertidal zone; HTZ, high intertidal zone) of a mangrove forest in Dongzhaigang Bay, Hainan Island, China. R2adj is the coefficient of determination after correcting for degrees of freedom, and the shaded area indicates confidence intervals.When the SD is not depicted, it indicates that the SD is smaller than the data points being presented.
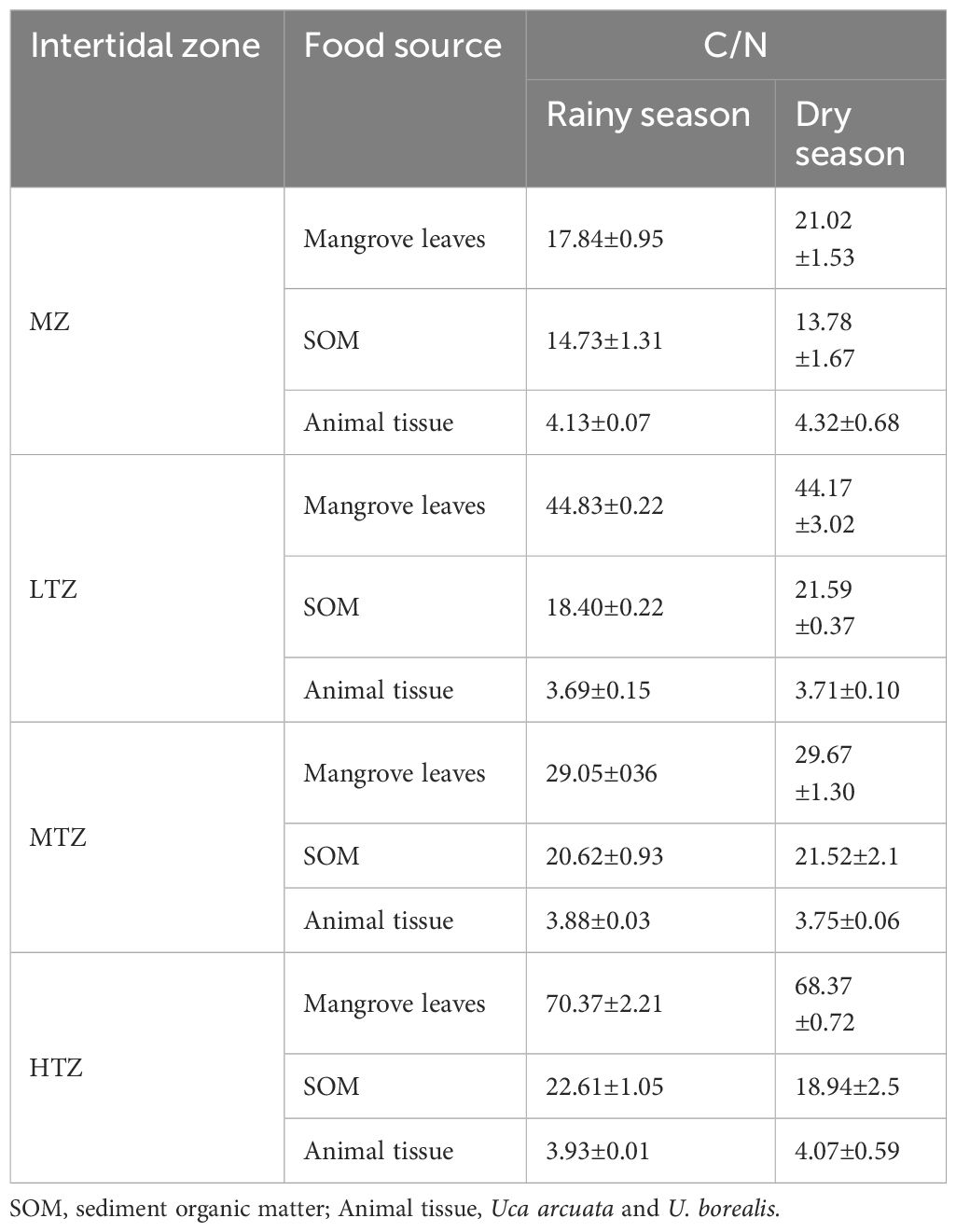
Table 1 Carbon to nitrogen ratio (C/N) of leaf-eating crab food sources in rainy and dry seasons at different intertidal zones (MZ, margin zone; LTZ, low intertidal zone; MTZ, middle intertidal zone; HTZ, high intertidal zone) of a mangrove forest in Dongzhaigang Bay, Hainan Island, China.
Discussion
Stable isotope signatures of leaf-eating crabs and potential crab food sources
The δ13C signatures of leaf-feeding crabs in MZ (mean = −23.9‰) are consistent with those reported previously in the eastern Pearl River estuary, China (mean = −24.2‰; Lee, 2000). Furthermore, in all four zones, δ13C signatures of leaf-eating crabs were relatively higher compared with those of mangrove leaves, indicating that leaf-eating crabs did not feed exclusively on mangrove litter. Compared with other leaf-eating crabs of the same genus in previous studies (Lee, 2000; Bouillon et al., 2004), the δ15N values of P. continentale were higher. Their study revealed that the food sources of leaf-eating crabs included mangrove leaves, sediments, particulate organic matter (POM), and microalgae, but did not include animal tissues. Our findings indicated that animal tissues had higher δ15N values than mangrove leaves. Therefore, leaf-eating crabs supplementing their diet with animal tissues may have had higher δ15N values than same-genus leaf-eating crabs in other areas. The reliance of Sesarmidae on animal tissues in mangrove ecosystems has been reported previously (Thongtham et al., 2008; Kristensen et al., 2017).
The δ13C signatures of mangrove leaves from different mangrove tidal zones (MZ, –26.9‰; LTZ, –29.1‰; MTZ, –30.4‰; and HTZ, –26.4‰; Figure 4A) are within the range of –35‰ to –22‰ reported for mangrove leaves worldwide (Bouillon et al., 2008; Medina-Contreras et al., 2024). In this research, the δ13C values of SOM were elevated by 1.2‰ to 5.9‰ in comparison to mangrove leaves. This result differs from the observed increase in δ13C values of SOM compared to mangrove leaves in other mangrove ecosystems. In the Godavari mangrove ecosystem in India, Bouillon et al. (2002) found that carbon isotope composition was enriched by 6‰–8‰ in mangrove sediments compared with mangrove vegetation. Bouillon et al. (2002) found that the Godavari mangrove forest had significant inputs of suspended matter from nearby mangrove creeks and adjacent bays, whereas there was no suspended matter replenishment from other ecosystems in our study area.
The prey (U. arcuata and U. borealis) exhibited higher δ13C values compared with the leaf-eating crabs (P. continentale). This result was consistent with findings from the same genus observed in Kenya and India (Bouillon et al., 2004), where Uca spp. exhibited higher δ13C values compared to Sesarmidae. Furthermore, the δ15N values of the prey were higher than those of the leaf-eating crabs, which was in line with the results reported by Kristensen et al. (2017) in the pristine mangrove forests of Pak Meng in southern Thailand. U. arcuata and U. borealis are omnivorous crabs that primarily consume benthic microalgae and zooplankton (Rianta et al., 2018). The higher δ13C and δ15N values of these crabs may be attributed to differences in their food sources compared with the leaf-feeding crab P. continentale. Further analysis of the food sources of prey in different tidal zones is necessary to determine their contribution to the diet of leaf-feeding crabs. Despite stable isotope evidence indicating that leaf-eating crabs frequently use C from sources other than mangrove leaves, they can consume and remove a substantial portion of overall mangrove litterfall (Schories et al., 2003; Nordhaus et al., 2006). As a result, leaf-eating crabs can provide an important link between energy flow and nutrient cycling.
Relationship between the C/N ratio and food contribution of mangrove leaves
In this study, we detected a significant negative relation between the C/N ratio of mangrove leaves and the contribution of leaves to crab diets. This result is comparable with that of Sandoval et al. (2022), who found that Aratus pisonii had the lowest intake rate of mangrove leaves with a high C/N ratio (155 ± 23) and the highest intake rate of those with a low C/N ratio (51 ± 18). Similarly, Chen and Ye (2008) found that leaf-eating crabs increased feeding rates on leaves with low C/N ratios. Typically, the ingestion of litterfall and vegetal organic matter varies according to the palatability of leaves (Robertson and Daniel, 1989; Steinke et al., 1993; Christofoletti et al., 2013). Christofoletti et al. (2013) concluded that leaf-eating crabs prefer leaves with relatively low polyphenol contents. Furthermore, structural components decrease and palatability increase of mangrove leaves after 20 h of soaking in estuarine water (Erickson et al., 2004; Nordhaus et al., 2011). Hirano et al. (2023) found that herbivory rates in mangroves were moderately affected by the concentration of condensed tannins in leaves and decreased from seaward to landward. This result was consistent with our finding that leaf-feeding crabs were influenced by the C/N ratio in leaves and decreased from low to high tidal zone. Therefore, in the field, leaf-eating crabs may prefer leached, brown leaves with a lower C/N ratio. However, tidal flooding is rare in the HTZ, and complete flooding only occurs during astronomical high tides. Additionally, because the rate of plant litter decomposition decreases as the C/N ratio increases (Enriquez et al., 1993; Almahasheer et al., 2016), relatively recalcitrant mangrove litter may accumulate in the nutrient-limited HTZ.
The environment of mangrove ecosystems is highly complex and variable, and therefore, the consumption of diverse C sources by leaf-eating crabs is expected to vary significantly owing to factors such as geomorphology, tidal amplitude, vegetation type, and biotic influences (Kon et al., 2007; Bouillon et al., 2008).
Food contribution to diets of leaf-eating crabs among mangrove zones
Food contributions to leaf-eating crab diets were different between wet and dry seasons, and this variation might be influenced by tidal cycles (Delong et al., 2001), seasonal abundance of benthic primary producers (Kang et al., 2006), and dietary preferences (Davenport et al., 2011; Rossi et al., 2015). In the field, we observed fewer litter inputs in the HTZ compared with other zones. The decrease in inputs could be a contributing factor to the reduced proportion of mangrove leaves in diets in the HTZ. The highest densities of P. continentale were in the LTZ and MTZ, which might be related to the relatively lower C/N ratio of mangrove leaves in the MTZ and the higher density of prey tissue in the LTZ and MTZ. The lower level of tidal scour disturbance in LTZ and MTZ, compared with the MZ which is frequently scoured by the tide, may have been another influencing factor. Therefore, the low and mid-tide zones are the optimum habitats for the leaf-eating crab P. continentale.
Sediment organic matter consistently contributed more than 20% to the diet of leaf-feeding crabs across zones and seasons, except in the MZ during the dry season (Figure 5). Similarly, Kawaida et al. (2019) determined that SOM contributed 23.1% to diets of leaf-eating crabs (P. continentale) in the spring and 23.3% in the summer. However, sediment is not generally regarded as an important food source, because crabs only assimilate associated refractory detritus and bacteria to a small extent (Thongtham and Kristensen, 2005; Nerot et al., 2009). Sediment organic matter in mangrove estuaries originates from various sources, such as phytoplankton, benthic microalgae, macroalgae, and mangrove detritus (Bouillon and Boschker, 2006). Therefore, the composition of the top 0–2 cm of surface sediment collected for analysis in this study, which includes organic matter from phytoplankton, microphytobenthos, and other components, might be one reason why SOM contributed more than 20% to the diet of leaf-eating crabs.
Further analysis of the chemical of SOM is necessary to determine its contributions to diets of leaf-feeding crabs (Chen et al., 2016) and to understand differences in the contribution at different tidal levels. The components of SOM, such as microalgae and plankton, should be individually sampled to determine their dietary contributions.
Conclusion
This study is the first to analyze the variations in food sources of leaf-eating crabs in different tidal zones. Mangrove leaves were the primary C source for leaf-eating crabs in the forest margin area, and leaves and sediment organic matter were the primary C sources in low and mid tidal zones. By contrast, mangrove leaves had the lowest contributions to the diets of leaf-eating crabs in the high tide zone. With increasing tidal levels, the primary food sources for leaf-eating crabs switched from mangrove leaves and sediment organic matter to sediment organic matter and animal tissues. Thus, the dietary habits of leaf-feeding crabs in mangrove ecosystems were affected by tidal-level zoning.
Data availability statement
The original contributions presented in the study are included in the article/Supplementary Material. Further inquiries can be directed to the corresponding author.
Ethics statement
The manuscript presents research on animals that do not require ethical approval for their study.
Author contributions
XL: Writing – original draft, Data curation, Formal analysis, Methodology. XG: Formal analysis, Methodology, Writing – review & editing. LZ: Data curation, Formal analysis, Writing – review & editing. JZ: Data curation, Writing – original draft. WW: Data curation, Funding acquisition, Methodology, Writing – original draft. MW: Formal analysis, Funding acquisition, Methodology, Writing – original draft.
Funding
The authors declare financial support was received for the research, authorship, and/or publication of this article. This research was funded by National Natural Science Foundation of China (NSFC) (Grant No. 42076161, 42176169, 31670490).
Acknowledgments
We thank the staff of Hainan’s Dongzhaigang National Nature Reserve and the local community for their understanding and support.
Conflict of interest
The authors declare that the research was conducted in the absence of any commercial or financial relationships that could be construed as a potential conflict of interest.
Publisher’s note
All claims expressed in this article are solely those of the authors and do not necessarily represent those of their affiliated organizations, or those of the publisher, the editors and the reviewers. Any product that may be evaluated in this article, or claim that may be made by its manufacturer, is not guaranteed or endorsed by the publisher.
Supplementary material
The Supplementary Material for this article can be found online at: https://www.frontiersin.org/articles/10.3389/fmars.2024.1351183/full#supplementary-material
Supplementary Figure 1 | Variations in rainy season of (A) δ13C and (B) δ15N values of potential food resources of leaf-eating crabs, including leaf litter of dominant mangrove species (Avicennia marina [MZ], Rhizophora stylosa [LTZ], Bruguiera sexangula [MTZ], and Ceriops tagal [HTZ]), prey (animal tissues), and sediment organic matter (SOM), at different intertidal zones (MZ, margin zone; LTZ, low intertidal zone; MTZ, middle intertidal zone; HTZ, high intertidal zone) of a mangrove forest in Dongzhaigang Bay, Hainan Island, China. Different lowercase letters indicate significant differences in isotope values of a food resource among intertidal zones (post hoc tests, p< 0.05). Boxes extend from the 25th to 75th percentile, with the black horizontal line indicating the median and the saltire inside boxes indicating the mean. The whiskers extending from boxes represent the highest and lowest values.
Supplementary Figure 2 | Variations in dry season of (A) δ13C and (B) δ15N values of potential food resources of leaf-eating crabs, including leaf litter of dominant mangrove species (Avicennia marina [MZ], Rhizophora stylosa [LTZ], Bruguiera sexangula [MTZ], and Ceriops tagal [HTZ]), prey (animal tissues), and sediment organic matter (SOM), at different intertidal zones (MZ, margin zone; LTZ, low intertidal zone; MTZ, middle intertidal zone; HTZ, high intertidal zone) of a mangrove forest in Dongzhaigang Bay, Hainan Island, China. Different lowercase letters indicate significant differences in isotope values of a food resource among intertidal zones (post hoc tests, p< 0.05). Boxes extend from the 25th to 75th percentile, with the black horizontal line indicating the median and the saltire inside boxes indicating the mean. The whiskers extending from boxes represent the highest and lowest values.
Supplementary Table 1 | Results of the one-way analysis of variance (ANOVA) for the stable isotope values of leaf-eating crabs in different tidal zones.
Supplementary Table 2 | P-values and t-values of Tukey HSD post-hoc tests for stable isotope values of leaf-eating crabs among different tidal zones. (MZ, margin zone; LTZ, low intertidal zone; MTZ, middle intertidal zone; HTZ, high intertidal zone) of a mangrove forest in Dongzhaigang Bay, Hainan Island, China.
Supplementary Table 3 | P-values of Student’s t-test for δ13C and δ15N of leaf-eating crabs and their food sources in the dry and rainy seasons. (MZ, margin zone; LTZ, low intertidal zone; MTZ, middle intertidal zone; HTZ, high intertidal zone) of a mangrove forest in Dongzhaigang Bay, Hainan Island, China.
References
Almahasheer H., Duarte C. M., Irigoien X. (2016). Nutrient limitation in Central Red Sea mangroves. Front. Mar. Sci. 3. doi: 10.3389/fmars.2016.00271
Araújo J. M. C., Otero X. L., Marques A. G. B., Nóbrega G. N., Silva J. R. F., Ferreira T. O. (2012). Selective geochemistry of iron in mangrove soils in a semiarid tropical climate: effects of the burrowing activity of the crabs Ucides cordatus and Uca maracoani. Geo-Mar Lett. 32, 289–300. doi: 10.1007/s00367-011-0268-5
Basyuni M., Bimantara Y., Cuc N. T. K., Balke T., Vovides A. G. (2022). Macrozoobenthic community assemblage as key indicator for mangrove restoration success in North Sumatra and Aceh, Indonesia. Restor. Ecol. 30, e13614. doi: 10.1111/rec.13614
Bouillon S., Boschker H. T. S. (2006). Bacterial carbon sources in coastal sediments: a cross-system analysis based on stable isotope data of biomarkers. Biogeosciences 3, 175–185. doi: 10.5194/bg-3-175-2006
Bouillon S., Connolly R. M., Lee S. Y. (2008). Organic matter exchange and cycling in mangrove ecosystems: Recent insights from stable isotope studies. J. Sea Res. 59, 44–58. doi: 10.1016/j.seares.2007.05.001
Bouillon S., Koedam N., Raman A. V., Dehairs F. (2002). Primary producers sustaining macro-invertebrate communities in intertidal mangrove forests. Oecologia 130, 441–448. doi: 10.1007/s004420100814
Bouillon S., Moens T., Overmeer I., Koedam N., Dehairs F. (2004). Resource utilization patterns of epifauna from mangrove forests with contrasting inputs of local versus imported organic matter. Mar. Ecol. Prog. Ser. 278, 77–88. doi: 10.3354/meps278077
Bui T. H. H., Lee S. Y. (2014). Does ‘you are what you eat’ apply to mangrove grapsid crabs? PloS One 9, e89074. doi: 10.1371/journal.pone.0089074
Caut S., Angulo E., Courchamp F. (2009). Variation in discrimination factors (Δ15N and Δ13C): the effect of diet isotopic values and applications for diet reconstruction. J. Appl. Ecol. 46, 443–453. doi: 10.1111/j.1365-2664.2009.01620.x
Chen G.-C., Azkab M. H., Chmura G. L., Chen S. Y., Sastrosuwondo P., Ma Z. Y., et al. (2017). Mangroves as a major source of soil carbon storage in adjacent seagrass meadows. Sci. Rep. 7, 42406. doi: 10.1038/srep42406
Chen G.-C., Lu C. Y., Li Y., Chen B., Hu. Q. F., Ye Y. (2016). Effects of foraging leaf litter of Aegiceras corniculatum (Ericales, Myrsinaceae) by Parasesarma plicatum (Brachyura, Sesarmidae) crabs on properties of mangrove sediment: a laboratory experiment. Hydrobiologia 763, 125–133. doi: 10.1007/s10750-015-2367-1
Chen G.-C., Ye Y. (2008). Leaf consumption by Sesarma plicata in a mangrove forest at Jiulongjiang Estuary, China. Mar. Biol. 154, 997–1007. doi: 10.1007/s00227-008-0990-3
Christofoletti A. R., Hattori Y. G., Pinheiro A. A. M. (2013). Food selection by a mangrove crab: temporal changes in fasted animals. Hydrobiologia 702, 63–72. doi: 10.1007/s10750-012-1307-6
Davenport J., Exgeta-Balić D., Peharda M., Skejić S., Ninčević-Gladan Ž., Matijević S. (2011). Size-differential feeding in Pinna nobilis L. (Mollusca: Bivalvia): exploitation of detritus, phytoplankton and zooplankton. Estuar. Coast. Shelf Sci. 92, 246–254. doi: 10.1016/j.ecss.2010.12.033
Delong M. D., Thorp J. H., Greenwood K. S., Miller M. C. (2001). Responses of consumers and food resources to a high magnitude, unpredicted flood in the upper Mississippi River basin. Regul. River 17, 217–234. doi: 10.1002/rrr.614
Donázar-Aramendía I., Sánchez-Moyano J. E., García-Asencio I., Miró J. M., Megina C., García-Gómez J. C. (2019). Human pressures on two estuaries of the Iberian Peninsula are reflected in food web structure. Sci. Rep. 9, 11495. doi: 10.1038/s41598-019-47793-2
Duke N. C., Ball M. C., Ellison J. C. (1998). Factors influencing biodiversity and distributional gradients in mangrove. Global Ecol. Biogeogr. Lett. 7, 27–47. doi: 10.2307/2997695
Enriquez S., Duarte C. M., Sand-Jensen K. (1993). Patterns in decomposition rates among photosynthetic organisms: the importance of detritus C:N:P content. Oecologia 94, 457–471. doi: 10.1007/BF00566960
Erickson A. A., Bell S. S., Dawes C. J. (2004). Does mangrove leaf chemistry help explain crab herbivory patterns? Biotropica 36, 333–343. doi: 10.1111/j.1744-7429.2004.tb00325.x
Feng J. X., Huang Q., Chen H., Guo J. M., Lin G. H. (2017). Restoration of native mangrove wetlands can reverse diet shifts of benthic macrofauna caused by invasive cordgrass. J. Appl. Ecol. 55, 905–916. doi: 10.1111/1365-2664.12987
Gao X. D., Chen X. J., Li Y. K. (2018). A review on the methods used in aquatic food web research: Development and applications. J. Fishery Sci. China 25, 1347–1360. doi: 10.3724/SP.J.1118.2018.17334
Gao X. Q., Lee S. Y. (2022). Feeding strategies of mangrove leaf-eating crabs for meeting their nitrogen needs on a low-nutrient diet. Front. Mar. Sci. 9. doi: 10.3389/fmars.2022.872272
Gelman A., Carlin J. B., Stern H. S., Dunson D. B., Vehtari A., Rubin D. B. (2013). “Chapter 7. Evaluating, comparing, and expanding models,” in Bayesian data analysis (CRC Press, Boca Raton, FL), 165–196. doi: 10.1201/b16018
Hirano Y., Marui M., Tachikake S., Kato T., Imai N. (2023). Relationship between herbivory and leaf traits in mangroves on Iriomote Island, southern Japan. J. For. Res., 29, 204–213. doi: 10.1080/13416979.2023.2279003
Kang C. K., Lee Y. W., Choy E. J., Shin J. K., Seo I. S., Hong J. S. (2006). Microphytobenthos seasonality determines growth and reproduction in intertidal bivalves. Mar. Ecol. Prog. Ser. 315, 113–127. doi: 10.3354/meps315113
Kawaida S., Nanjo K., Ohtsuchi N., Kohno H., Sano M. (2019). Cellulose digestion abilities determine the food utilization of mangrove estuarine crabs. Estuar. Coast. Shelf Sci. 222, 43–52. doi: 10.1016/j.ecss.2019.04.004
Kon K., Kurokura H., Hayashizaki K. (2007). Role of microhabitats in food webs of benthic communities in a mangrove forest. Mar. Ecol. Prog. Ser. 340, 55–62. doi: 10.3354/meps340055
Kristensen E., Holmer M., Banta G. T., Jensen M. H., Hansen K. (1995). Carbon, nitrogen and sulfur cycling in sediment of the Ao Nam Bor mangrove forest, Phuket, Thailand: a review. Phuket Mar. Biol. Cent. Res. Bull. 60, 37–64.
Kristensen E. (2008). Mangrove crabs as ecosystem engineers; with emphasis on sediment processes. J. Sea Res. 59, 30–43. doi: 10.1016/j.seares.2007.05.004
Kristensen D. K., Kristensen E., Mangion P. (2010). Food partitioning of leaf-eating mangrove crabs (Sesarminae): Experimental and stable isotope (13C and 15N) evidence. Estuar. Coast. Shelf Sci. 87, 583–590. doi: 10.1016/j.ecss.2010.02.016
Kristensen E., Lee S. Y., Mangion P., Quintana C. O., Valdemarsen T. (2017). Trophic discrimination of stable isotopes and potential food source partitioning by leaf-eating crabs in mangrove environments. Limnol. Oceanogr. 62, 2097–2112. doi: 10.1002/lno.10553
Kruitwagen G., Nagelkerken I., Lugendo B. R., Mgaya Y. D., Bonga S. E. W. (2010). Importance of different carbon sources for macroinvertebrates and fishes of an interlinked mangrove-mudflat ecosystem (Tanzania). Estuar. Coast. Shelf Sci. 88, 464–472. doi: 10.1016/j.ecss.2010.05.002
Lee S. Y. (1997). Potential trophic importance of the faecal material of the mangrove sesarmine crab Sesarma massa. Mar. Ecol. Prog. Ser. 159, 275–284. doi: 10.3354/meps159275
Lee S. Y. (2000). Carbon dynamics of Deep Bay, eastern Pearl River estuary, China. II: Trophic relationship based on carbon- and nitrogen-stable isotopes. Mar. Ecol. Prog. Ser. 205, 1–10. doi: 10.3354/meps205001
Lee S. Y. (2008). Mangrove macrobenthos: Assemblages, services, and linkages. J. Sea Res. 59, 16–29. doi: 10.1016/j.seares.2007.05.002
MacKenzie R. A., Cormier N., Demopoulos A. W. (2020). Estimating the value of mangrove leaf litter in sesarmid crab diets: the importance of fractionation factors. Bull. Mar. Sci. 96, 501–520. doi: 10.5343/bms.2019.0026
McCutchan J. H., Lewis W. M., Kendall C., McGrath C. C. (2003). Variation in trophic shift for stable isotope ratios of carbon, nitrogen, and sulfur. Oikos 102, 378–390. doi: 10.1034/j.1600-0706.2003.12098.x
Medina-Contreras D., Arenas F., Cantera-Kintz J., Sánchez A., Lázarus J.-F. (2022). Carbon sources supporting macrobenthic crustaceans in tropical eastern pacific mangroves. Food Webs 30. doi: 10.1016/j.fooweb.2022.e00219
Medina-Contreras D., Arenas F., Sánchez A. (2024). “Mangrove Food Web Structure and Isotopic Niche,” in Treatise on estuarine and coastal science, 2nd edition. Eds. Baird D., Elliott M. (Elsevier, London).
Mieczan T., Michał N., Adamczuk M., Bielańska-Grajner I. (2015). Stable isotope analyses revealed high seasonal dynamics in the food web structure of a peatbog. Int. Rev. Hydrobiol 100, 141–150. doi: 10.1002/iroh.201501788
Moore J. W., Semmens B. X. (2008). Incorporating uncertainty and prior information into stable isotope mixing models. Ecol. Lett. 11, 470–480. doi: 10.1111/j.1461-0248.2008.01163.x
Nagelkerken I., Blaber S. J. M., Bouillon S., Green P., Haywood M., Kirton L. G., et al. (2008). The habitat function of mangroves for terrestrial and marine fauna: A review. Aquat. Bot. 89, 155–185. doi: 10.1016/j.aquabot.2007.12.007
Nerot C., Meziane T., Provost-Govrich A., Rybarczyk H., Lee S. Y. (2009). Role of grapsid crabs, Parasesarma erythrodactyla, in entry of mangrove leaves into an estuarine food web: a mesocosm study. Mar. Biol. 156, 2343–2352. doi: 10.1007/s00227-009-1262-6
Nordhaus I., Salewski T., Jennerjahn T. C. (2011). Food preferences of mangrove crabs related to leaf nitrogen compounds in the Segara Anakan Lagoon, Java, Indonesia. J. Sea Res. 65, 414–426. doi: 10.1016/j.seares.2011.03.006
Nordhaus I., Wolff M., Diele K. (2006). Litter processing and population food intake of the mangrove crab Ucides cordatus in a high intertidal forest in northern Brazil. Estuar. Coast. Shelf Sci. 67, 239–250. doi: 10.1016/j.ecss.2005.11.022
Oakes J. M., Connolly R. M., Revill A. T. (2010). Isotope enrichment in mangrove forests separates microphytobenthos and detritus as carbon sources for animals. Limnol. Oceanogr. 55, 393–402. doi: 10.4319/lo.2010.55.1.0393
Parnell A. C., Inger R. (2016) A Stable Isotope Mixingmodel. R Package Version 0.3. Available online at: https://CRAN.R-project.org/package=simmr (Accessed 01 Sep 2021).
Parnell A. C., Inger R., Bearhop S., Jackson A. L. (2010). Source partitioning using stable isotopes: coping with too much variation. PloS One 5, e9672. doi: 10.1371/journal.pone.0009672
Parnell A. C., Phillips D. L., Bearhop S., Semmens B. X., Ward E. J., Moore J. W., et al. (2013). Bayesian stable isotope mixing models. Environmetrics 24, 387–399. doi: 10.1002/env.2221
Pereira T. M., Nóbrega G. N., Ferreira T. O., Ogawa C. Y., de Camargo P. B., Feitosa Silva J. R., et al. (2019). Does food partitioning vary in leaf-eating crabs in response to source quality? Mar. Environ. Res. 144, 72–83. doi: 10.1016/j.marenvres.2018.12.005
Phillips D. L., Inger R., Bearhop S., Jackson A. L., Moore J. W., Parnell A. C., et al. (2014). Best practices for use of stable isotope mixing models in food-web studies. Can. J. Zool. 92, 823–835. doi: 10.1139/cjz-2014-0127
Preciado I., Cartes J. E., Punzón A., Frutos I., López-López L., Serrano A. (2017). Food web functioning of the benthopelagic community in a deep-sea seamount based on diet and stable isotope analyses. Deep-Sea Res. Part II 137, 56–68. doi: 10.1016/j.dsr2.2016.07.013
R Core Team (2017). R: A language and environment for statistical computing (Austria: R Found. Stat. Comput. Vienna). Available at: https://www.R-project.org/.
Rianta P., Ernawati W., Chen G., Chen S. (2018). Diversity and abundance of mangrove fiddle crabs, genus Uca (Decapoda, Ocypodidae) at a mangrove in Kema, North Sulawesi, Indonesia. Acta Oceanologica Sin. 37, 92–96. doi: 10.1007/s13131-018-1336-8
Robertson A. I. (1986). Leaf-burying crabs: their influence on energy flow and export from mixed mangrove forests (Rhizophora spp.) in northeastern Australia. J. Exp. Mar. Biol. Ecol. 102, 237–248. doi: 10.1016/0022-0981(86)90179-6
Robertson A. I., Daniel P. A. (1989). The influence of crabs on litter processing in high intertidal mangrove forests in tropical Australia. Oecologia 78, 191–198. doi: 10.1007/BF00377155
Rossi F., Baeta A., Marques J. C. (2015). Stable isotopes reveal habitat-related diet shifts in facultative deposit-feeders. J. Sea Res. 95, 172–179. doi: 10.1016/j.seares.2014.07.004
Ryba S. A., Burgess R. M. (2002). Effects of sample preparation on the measurement of organic carbon, hydrogen, nitrogen, sulfur, and oxygen concentrations in marine sediments. Chemosphere 48, 139–147. doi: 10.1016/S0045-6535(02)00027-9
Sandoval L. A., Mancera-Pineda J. E., Delgado-Huertas A., Blanco-Libreros J. F., Leal-Flórez J. (2022). Leaf consumption and experimental discrimination of stable isotopes between mangrove leaves and the tree-climbing crab (Brachyura: Sesarmidae: Aratus pisonii). Estuar. Coast. Shelf Sci. 274. doi: 10.1016/j.ecss.2022.107906
Schories D., Barletta-Bergan A., Barletta M., Krumme U., Mehlig U., Rademaker V. (2003). The keystone role of leaf-removing crabs in mangrove forests of North Brazil. Wetl Ecol. Manag 11, 243–255. doi: 10.1023/A:1025011431984
Shang X., Huang H., Mei K., Xia F., Chen Z., Yang Y., et al. (2020). Riverine nitrate source apportionment using dual stable isotopes in a drinking water source watershed of southeast China. Sci. Total Environ. 724, 137975. doi: 10.1016/j.scitotenv.2020.137975
Steinke T. D., Rajh A., Holland A. J. (1993). The feeding behaviour of the red mangrove crab Sesarma meinerti De Man 1887(Crustacea: Decapoda: Grapsidae) and its effect on the degradation of mangrove leaf litter. South Afr. J. Mar. Sci. 13, 151–160. doi: 10.2989/025776193784287455
Stock B. C., Jackson A. L., Ward E. J., Parnell A. C., Phillips D. L., Semmens B. X. (2018). Analyzing mixing systems using a new generation of Bayesian tracer mixing models. PeerJ 6, e5096. doi: 10.7717/peerj.5096
Swindells K. L., Robyn J. M., William D. B., Nicholas W. H., Richard K. F. U. (2017). Habitat configuration alters herbivory across the tropical seascape. Front. Mar. Sci. 4. doi: 10.3389/fmars.2017.00048
Thongtham N., Kristensen E. (2005). Carbon and nitrogen balance of leaf-eating sesarmid crabs (Neoepisesarma versicolor) offered different food sources. Estuar. Coast. Shelf Sci. 65, 213–222. doi: 10.1016/j.ecss.2005.05.014
Thongtham N., Kristensen E., Puangprasan S.-Y. (2008). Leaf removal by sesarmid crabs in Bangrong mangrove forest, Phuket, Thailand; with emphasis on the feeding ecology of Neoepisesarma versicolor. Estuar. Coast. Shelf Sci. 80, 573–580. doi: 10.1016/j.ecss.2008.09.017
Tue N. T., Hamaoka H., Sogabe A., Quy T. D., Nhuan M. T., Omori K. (2012). Food sources of macro-invertebrates in an important mangrove ecosystem of Vietnam determined by dual stable isotope signatures. J. Sea Res. 72, 14–21. doi: 10.1016/j.seares.2012.05.006
Van Hieu P., Hoang Ha N. T., Viet Dung L., Omori K. (2020). Carbon sources supporting macro-invertebrate communities in restored mangrove forests from Hau Loc, Thanh Hoa, Vietnam. J. Mar. Sci. Eng. 8, 651. doi: 10.3390/jmse8090651
Ward E. J., Semmens B. X., Schindler D. E. (2010). Including source uncertainty and prior information in the analysis of stable isotope mixing models. Environ. Sci. Technol. 44, 4645–4650. doi: 10.1021/es100053v
Wickham H. (2016). ggplot2: Elegant Graphics for Data Analysis (Version 4.0.1) (New York: Springer-Verlag). Available at: https://cran.r-project.org/web/packages/ggplot2/index.html. doi: 10.1007/978-3-319-24277-4
Wolcott D., O’Connor N. (1992). Herbivory in crabs: adaptations and ecological considerations. Integr. Comp. Biol. 32, 370–381. doi: 10.1093/icb/32.3.370
Xie T., Wang A., Li S., Cui B., Bai J., Shao D. (2022). Crab contributions as an ecosystem engineer to sediment turnover in the Yellow River Delta. Front. Mar. Sci. 9. doi: 10.3389/fmars.2022.1019176
Xiong Y., Liu X., Guan W., Liao B., Chen Y., Li M., et al. (2017). Fine root functional group based estimates of fine root production and turnover rate in natural mangrove forests. Plant Soil 413, 83–95. doi: 10.1007/s11104-016-3082-z
Keywords: food sources, Bayesian mixing models, stable isotopes, mangrove tidal zones, leaf-eating crabs, mangrove leaf C/N ratio
Citation: Lu X, Gu X, Zhang L, Zhang J, Wang W and Wang M (2024) Tidal zone effects on the diet composition of leaf-eating crabs in natural mangrove communities: a stable isotope analysis. Front. Mar. Sci. 11:1351183. doi: 10.3389/fmars.2024.1351183
Received: 06 December 2023; Accepted: 10 May 2024;
Published: 23 May 2024.
Edited by:
Pedro Morais, University of Algarve, PortugalReviewed by:
Inés G. Viana, Spanish Institute of Oceanography, SpainAlberto Sánchez-González, National Polytechnic Institute (IPN), Mexico
Copyright © 2024 Lu, Gu, Zhang, Zhang, Wang and Wang. This is an open-access article distributed under the terms of the Creative Commons Attribution License (CC BY). The use, distribution or reproduction in other forums is permitted, provided the original author(s) and the copyright owner(s) are credited and that the original publication in this journal is cited, in accordance with accepted academic practice. No use, distribution or reproduction is permitted which does not comply with these terms.
*Correspondence: Mao Wang, d2FuZ21hb0B4bXUuZWR1LmNu