- Department of Ecology and Hydrology and Regional Campus of International Excellence “Mare Nostrum”, University of Murcia, Murcia, Spain
The study of jellyfish blooms has gained attention in the recent decades because of the importance of forecasting and anticipating them and avoiding their interference with human activities. However, after thirty years of scientific effort (monitoring systems, empirical laboratory and field studies, modeling, etc.), the occurrence of blooms remains unpredictable, and their consequences unavoidable. Climate change, eutrophication, overfishing, coastal construction, and species translocation have been suggested as stressors that increase them, but robust evidence to support these claims is limited. The widespread belief that jellyfish blooms are “increasing in number” has been challenged in recent years. Among the gelatinous zooplankton, the bloom forming species are concentrated in the class Scyphozoa, and the number of species with at least one recorded bloom has increased during the last decade. The analyses of long-term time series show seasonality in the dynamic of each blooming jellyfish species population, but the blooms vary in intensity and there are years of an unexplained absence of jellyfish. In this review, we focus on the current state of knowledge, uncertainties and gaps in the critical points that can strongly influence the intensity of the bloom or even lead to the absence of the medusa population. These points include ephyrae, planulae and scyphistoma natural, predatory or fishing mortality, the molecular pathway of strobilation, benthic population dynamics, planula settlement and ephyra to medusa transition success. Some of these points account for certain empirical laboratory evidence under controlled conditions, and are difficult to be studied on the field, but the different sources of non-typically recorded variability need to be addressed to improve our understanding of jellyfish population dynamics.
1 Introduction
The massive proliferations of jellyfishes, commonly referred to as jellyfish blooms, are usually described in negative terms because of the interference with human activities that results from their appearance. Those interferences include competing with commercial fish species both through predation on fish larvae and through the direct competition for food, clogging fishing nets, damaging catches, stinging fishermen and swimmers or clogging water intake systems in coastal power plants, among others (Purcell et al., 2007; Richardson et al., 2009; Purcell et al., 2013). The socio-economic impacts of the jellyfish blooms are often neglected in scientific studies (Bosch-Belmar et al., 2020), but some examples can be found. In the case of fisheries, catches can be reduced by up to 25.3%, the catch value can be reduced by up to 33.7%, and the economic loss, in Korean fisheries alone, can exceed US$ 200 million in a single jellyfish season (Kim et al., 2012). For the tourism sector in the United Kingdom, avoiding the areas where jellyfish are present can result in an economic loss of more than 12000 US$ per day for a 10 km2 tourist area (Kennerley et al., 2022), while in Spain, the average visitor is willing to pay an additional 3.20 € per beach visit if it guarantees a lower risk of encountering jellyfish (Nunes et al., 2015).
However, despite the socio-economic impacts derived from the appearance of jellyfish blooms, the ecosystem services they provide should not be ignored. In eutrophication processes, jellyfish have been linked to the maintenance of water quality through top-down control of the food web (Pérez-Ruzafa et al., 2002; Fernández-Alías et al., 2022; Fernández-Alías et al., 2023), and their use as food has led to the emergence of a fishing and aquaculture industry focused on these organisms (Hsieh et al., 2001; Omori and Nakano, 2001; Purcell et al., 2007; Nishikawa et al., 2015; Khong et al., 2016; Leone et al., 2019). In addition, jellyfish are being studied in search of biomolecules with potential applications in pharmacology and medicine (Zimmer, 2005; Sugahara et al., 2006; De Rinaldis et al., 2021; De Domenico et al., 2023; Sudirman et al., 2023), and as the most energetically efficient swimmers, jellyfish biomechanics are a subject of study in the development of remotely operated swimming vehicles (Gemmell et al., 2013; Gemmell et al., 2015; Costello et al., 2021; Gemmell et al., 2021).
The disturbances and ecological services provided by jellyfish blooms have attracted the attention of the scientific community with increasing interest since the last decade of the twentieth century (Pitt et al., 2018). Along with the scientific community, the general public have also been engaged to participate in the monitoring of jellyfish blooms through citizen science campaigns (Marambio et al., 2021; Dobson et al., 2023). During the years in which this topic has been studied, the perception of a general increase in the frequency, intensity, and extent of jellyfish blooms has become established in the scientific community, and a whole conceptual framework of how ocean degradation favors the occurrence of jellyfish blooms has been developed (Arai, 2001; Purcell, 2005; Purcell et al., 2007; Richardson et al., 2009; Purcell, 2012). Among the ocean degradation factors cited as drivers of jellyfish blooms, overfishing, climate change, species translocation, eutrophication, and habitat modification are commonly highlighted as part of an eventual regression of the ocean state to Cambrian-like assemblages dominated by short-lived organisms such as jellyfish (Figure 1) (Pauly et al., 1998; Parsons and Lalli, 2002; Pauly et al., 2008; Richardson et al., 2009).
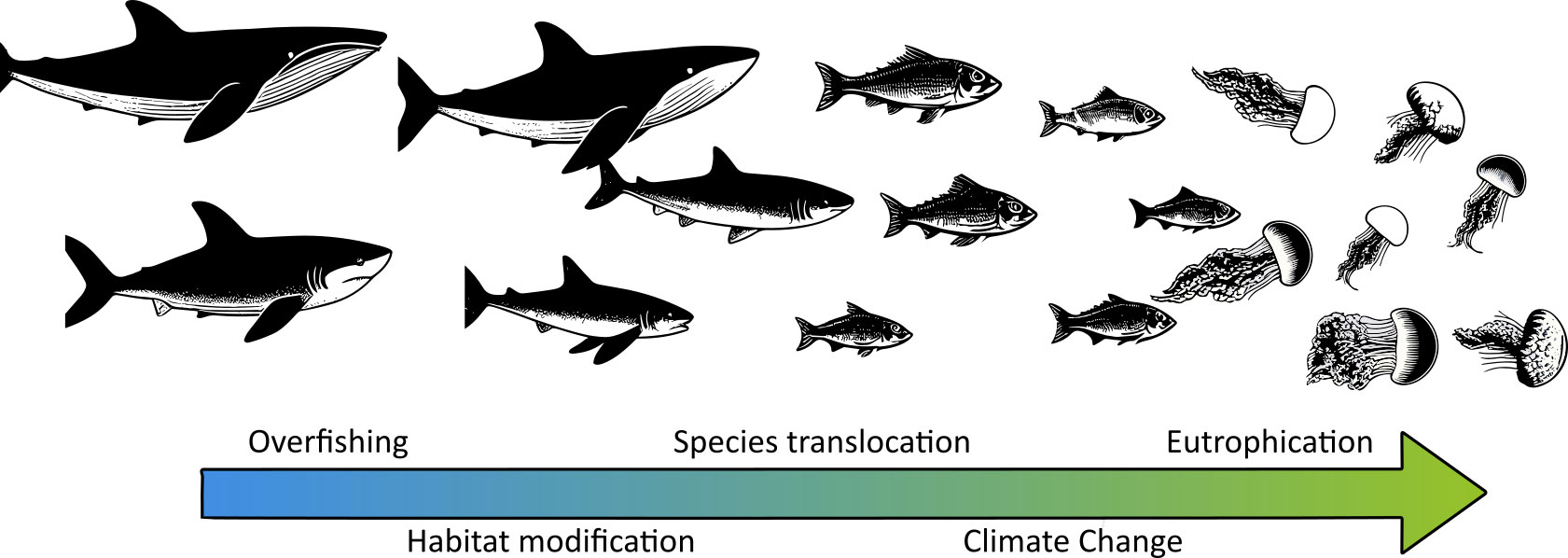
Figure 1 Conceptual framework of the change in the key-species controlling marine food webs in a regression to Cambrian-like assemblages because of ocean degradation (based on Pauly et al., 2008).
The same authors who contributed to the development of the conceptual framework also noted its limitations, highlighting the paucity of reliable baseline data and the short length of the time-series those assumptions were made on (Purcell et al., 2007; Pauly et al., 2008; Richardson et al., 2009). The low availability of data forced scientists attempting to analyze these assumptions to gather all the organisms referred to as jellyfish (Brotz et al., 2012) into a single and generic ‘gelatinous zooplankton’ group (Condon et al., 2013), despite their taxonomic, genetic and life cycle differences (Hamner and Dawson, 2009; Khalturin et al., 2019). In addition, research efforts have not been equally distributed among all components of the gelatinous zooplankton, with an overrepresentation of the scyphozoan Aurelia spp. Lamarck, 1816 and the ctenophore Mnemiopsis leidyi A. Agassiz, 1865 (Pitt et al., 2018). It should also be noted that bloom-forming species are not evenly distributed throughout the gelatinous zooplankton but are concentrated in the class Scyphozoa (Hamner and Dawson, 2009), with special incidence in the larger species dwelling in temperate, shallow waters (Fernández-Alías et al., 2021). The importance of this class is not limited to a higher number of bloom forming species, as they are also responsible for most of the disturbances in fisheries and aquaculture (Bosch-Belmar et al., 2020), but also collect the highest number of edible species (Brotz et al., 2017).
Despite the controversies and limitations, the conceptual framework is generally accepted by the scientific community, and the number of published papers on the topic increases every year, contributing to the dissemination of the idea of an increase in jellyfish blooms in some areas (Sanz-Martín et al., 2016; Pitt et al., 2018). Considering only the class Scyphozoa, where most of the blooms are concentrated, an increase in the percentage of bloom forming species has been detected in the last decade (Fernández-Alías et al., 2021). However, this increase does not necessarily mean that jellyfish populations are increasing worldwide (Brotz et al., 2012), as it is associated with a higher interest in the topic and more sampling efforts (Pitt et al., 2018).
Of all the variables analyzed within the conceptual framework, temperature is the one that stands out the most (Fernández-Alías et al., 2021), probably reflecting the seasonality that most species exhibit during their life cycle. In the class Scyphozoa, this variable has been shown to control the life cycle, modifying the asexual reproduction rate of the polyp stage, acting as a strobilation trigger and regulating the transition from ephyra to medusa (Prieto et al., 2010; Fuentes et al., 2011; Fuchs et al., 2014; Brekhman et al., 2015; Fernández-Alías et al., 2023). The effect of temperature can also be observed in short-term studies for most ecosystems and species (Fernández-Alías et al., 2020; Gueroun et al., 2020; Leoni et al., 2021a), but becomes blurred when longer time series are considered (van Walraven et al., 2015; Stone et al., 2019). This suggests that temperature is not an exclusive requirement for bloom development (Fernández-Alías et al., 2021). At the same time, the scyphozoan jellyfish species do not seem to have the thermal control on their phenology modified in the context of climate change (Fernández-Alías et al., 2023), but an increased period of suitable temperatures could extend the duration of the scyphozoan blooms (Ruiz et al., 2012; Edelist et al., 2020; Leoni et al., 2021b). In any case, both situations raise more questions and challenges about the interannual variability of bloom intensity in relation to thermal oscillations.
After more than 30 years of combined scientific research (monitoring systems, empirical laboratory and field studies, modeling, etc.), we are still unable to answer the main question asked by managers, tourists and other stakeholders: “Will there be jellyfish next season?”. There are several socio-economic implications of our inability to answer this demand, but one of the clearest examples is the cannonball jellyfish fishery in Mexico, where the national government promoted the activity and businesses developed in anticipation of a long-term productive industry, only to eventually experience a collapse in catches due to interannual variability or fisheries mismanagement (López-Martínez and Álvarez-Tello, 2013; Girón-Nava et al., 2015; Brotz et al., 2021). Improving the quality of jellyfish bloom predictions using traditional approaches, when not a single bloom has been correctly predicted in more than three decades of research, seems unlikely. Therefore, we have focused this review both on less commonly addressed sources of variability that affect the viability of scyphozoan blooms (e.g. predation on larval stages, parasitism, or interspecific competition), and on sources of variability that are commonly referred to as promoters of jellyfish blooms, but which can also induce physiological stress when they are not optimal (e.g. temperature, food availability or salinity).
This work builds on the systematic review by Fernández-Alías et al. (2021) in which all scyphozoan genera were individually used as keywords in the Web of Science and Scopus search engines to identify the bloom forming genera and species. The search algorithm was modified in the genera Cyanea, Chrysaora, Pelagia to Genus AND Jellyfish to eliminate papers from unrelated scientific fields and further modified to Genus AND Jellyfish AND (Bloom OR “Life cycle”) in the case of Aurelia due to its use as a model species. The original search was replicated to include the publications on blooming genera from January 2021 to December 2023. Previously selected papers on blooming species were re-analyzed with the recent publications using the following selection criteria of i) reference to scyphozoan species, ii) analysis of aspects of the jellyfish ecology at any developmental stage, iii) description of possible facilitators or perturbations to the regular development of the life cycle. The review was supplemented by additional searches in Google Scholar under combinations of the term “Jellyfish” with “Predation”, “Disease”, “Parasitism”, “Fisheries”, “Mortality” and “Stress” to include thesis and other ‘gray literature’ items. No filter was applied in terms of number of citations or year of publication. Since the aim of the review was to construct a conceptual model of the factors that control the biological cycle of scyphozoans and to discuss the sources of variability that influence the difficulty of predicting the events of massive proliferations of these organisms, and not to perform meta-analyses, no attempt was made to quantify the retrieved publications, selected or excluded papers.
2 Control of the ‘never-ending jellyfish joyride’
The proposed conceptual framework for a jellyfish-dominated ocean depicted a series of interrelated mechanisms that further enhanced this jellyfish dominance over other marine species, based on how their own biological characteristics seemed to overcome any possible control over populations (Richardson et al., 2009). However, reports of long-term monitoring of jellyfish populations usually reveal the existence of years of unexplained absence of jellyfish. In the Mar Menor coastal lagoon (Spain), an ecosystem affected by eutrophication over the last 30 years, habitat modification, global warming, and fishing (Pérez-Ruzafa et al., 2019; Fernández-Alías et al., 2022), populations of Cotylorhiza tuberculata (Macri, 1778) and Rhizostoma pulmo (Macri, 1778) unexpectedly collapsed after two decades of benefiting from the factors described in the conceptual framework (Fernández-Alías et al., 2022; Fernández-Alías et al., 2023). In the Dutch Wadden Sea (Netherlands), another monitored eutrophic ecosystem, the different scyphozoan jellyfish species showed different patterns of abundance over the years, alternating between years of unexplained absence or sparse abundance of some individual species and bloom years (van Walraven et al., 2015).
Scyphozoan jellyfish species generally have a metagenic life cycle, alternating between benthic and pelagic phases (Hamner and Dawson, 2009). During the same, there are several stages where a numerical expansion of the population can occur: a single medusa can carry thousands to millions of planulae (Kikinger, 1992; Lucas, 1996), the polyp population can double to five times its initial number within a month under the appropriate food-temperature conditions (Lucas et al., 2012; Wang et al., 2015), and each strobila can release from 1 to 30 ephyrae (Di Camillo et al., 2010; Fuentes et al., 2011; Lucas et al., 2012). Under the most optimal conditions without any kind of mortality, neither natural nor predatory, a single medusa can yield from a minimum of 2×103 (≈1 medusa × 1000 planulae/medusa × 2 polyps/planula × 1 ephyra/polyp) to a maximum of 1.5×108 medusae (≈1 medusa × 106 planulae/medusa × 5 polyps/planula × 30 ephyrae/polyp) within a single year (Figure 2, black arrows). Consequently, the large interannual variation in the abundance of the medusa phase of the scyphozoan jellyfish populations can only be explained by the existence of complex interactions that regulate, either as enhancers (Figure 2, green arrows) or inhibitors (Figure 2, red arrows), the dynamics of the different scyphozoan jellyfish populations during the different stages of the life cycle.
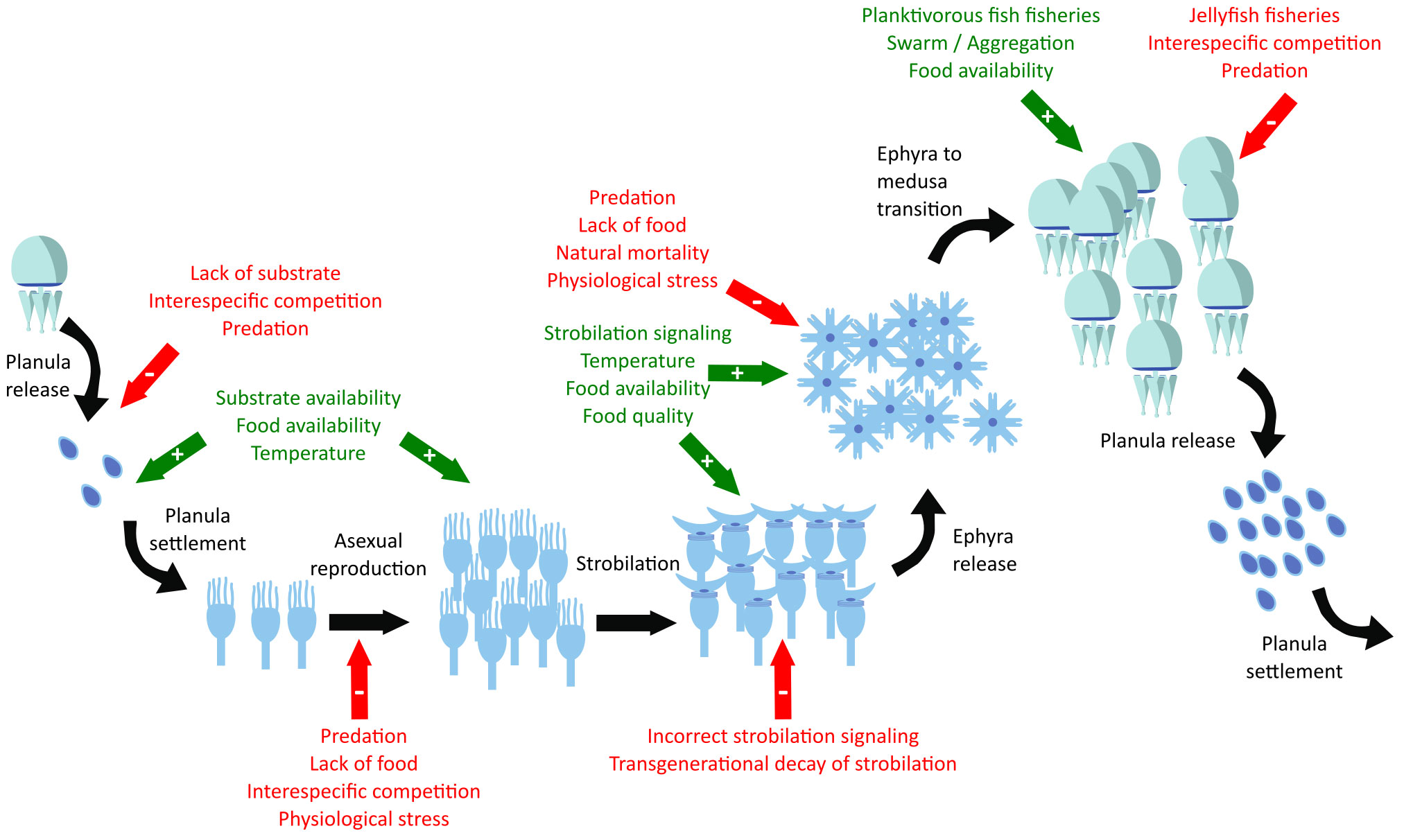
Figure 2 Scyphozoan life cycle (black arrows), enhancer factors (green arrows with plus sign), and limiting or inhibiting factors (red arrows with minus sign).
2.1 Planula stage
Scyphozoan planulae can settle on a variety of natural and artificial hard substrates, including shells, concrete, plastic, glass, wood, rope, seagrass, macroalgae, rocks, etc., with different efficiencies depending on the type and orientation of the substrate and the different scyphozoan species (Miyake et al., 2002; Holst and Jarms, 2007; Malej et al., 2012; Marques et al., 2015; Franco, 2016; Gambill et al., 2018; van Walraven et al., 2020). There is an environmental control by factors such as temperature, salinity or light on the settlement and excystment of the planulae, providing an efficiency of the process, with the absence of competition for the substrate or predation, which decreases from a maximum of ~ 60% under the most suitable conditions to a minimum of 0% in a progression towards less suitable conditions (Prieto et al., 2010; Franco, 2016; Gambill et al., 2018; Feng et al., 2021; Gueroun et al., 2021; Holst et al., 2023). Even under the preferred environmental conditions and in the absence of competitors and predators, the settlement needs to occur within the first few days after planula release to prevent a significant decline in its effectiveness (Gambill et al., 2018). However, spatial competition and predation cannot be ignored when attempting to build predictive models of jellyfish blooms, yet both topics remain largely understudied. Boughton et al. (2023) conducted an in-situ fouling settlement experiment on PVC panels testing the settlement efficiency of Aurelia aurita (Linnaeus, 1758) planulae against potential competitors, showing high competition for the space and a relative increase in planula settlement efficiency after removal of potential competitors. Predation on scyphozoan planulae, which is rarely addressed, can occur at least by ctenophores, ascidians, and bivalves at rates of 10 – 25, 20 and 40 planulae × ind-1 × h-1, respectively (Javidpour et al., 2009; Kuplik et al., 2015), as well as by scyphozoan polyps (Gröndahl, 1988a; Gröndahl, 1988b). In this line, Franco (2016) linked the absence of planula settlement on live oyster shells to the filtering capacity of oysters. In contrast, Marques et al. (2015) linked the absence of polyps attached to oyster shells in the Thau lagoon (France) to the oyster farming method rather than to the oyster predation on planulae, while Malej et al. (2012) do provide images of polyps over oyster shells. However, in the latter example it is not certain whether the planulae have settled directly over the shell or whether the presence of polyps is due to a colonization by asexual reproduction and polyp motility (Feng et al., 2017; Zang et al., 2023).
2.2 Polyp stage
After the planula settlement, the polyps emerge in a process called excystment and develop tentacles to acquire their typical morphology in a process that can be negatively affected by inadequate temperature-salinity conditions or by the lack of food (Holst et al., 2023). Newly formed polyps can colonize the substrate through 7 different modes of asexual reproduction (Schiariti et al., 2014; Wang F. et al., 2023). The different scyphozoan jellyfish species can exhibit a mono-mode asexual reproductive strategy (e.g. Phyllorhiza punctata von Lendenfeld, 1884; Schiariti et al., 2014), multi-mode asexual reproductive strategy (Aurelia spp.; Schiariti et al., 2014; Wang F. et al., 2023), or a preferential and a secondary strategy as it found in R. pulmo (Fuentes et al., 2011; Schiariti et al., 2014). Under the adequate food and temperature conditions, each polyp can produce 8 to 20 buds from which new polyps can develop (Purcell et al., 2012), but the overall efficiency in the absence of competitors or predators is typically limited to a two to fivefold increase in the initial number of polyps (Lucas et al., 2012; Wang et al., 2015). In general, species with multi-mode asexual reproductive strategies exhibit higher asexual reproduction rates (Schiariti et al., 2014).
Scyphozoan polyps generally exhibit a wide thermal and salinity tolerance, but the asexual reproduction rates can decline rapidly when the optimal conditions are not met and eventual mass mortality can occur when the upper or lower tolerance limits are exceeded (Purcell et al., 1999; Prieto et al., 2010; Purcell et al., 2012; Treible and Condon, 2019). As a result, the evolution of the different scyphozoan species has led to higher asexual reproduction rates of polyps at temperatures when their planulae are present in the water column (Prieto et al., 2010; Purcell et al., 2012; Schiariti et al., 2014; Treible and Condon, 2019). Scyphozoan polyps can also withstand starvation conditions, but surprisingly, when the food scarcity conditions are coupled with the optimal temperatures for the asexual reproduction, the survival is significantly reduced, likely due to increased metabolic demands (Zang et al., 2023).
In nature, the scyphozoan polyps face interspecific competition for food and space, predation, synergies with other organisms derived from the construction of their hard structures, and physiological stress associated with environmental conditions (Feng et al., 2017; Marques et al., 2019; Feng et al., 2021; Zang et al., 2023). The organisms that efficiently compete for the space and reduce substrate availability for scyphozoan polyps by killing and displacing them, have a slimy or soft surface that cannot be used as a substrate for polyp development (Zang et al., 2023). This first group mainly includes sponges and ascidians, but there are some exceptions, as Aurelia sp. has been shown to be able to grow on certain solitary ascidians (Miyake et al., 2002; Feng et al., 2017; Rekstad et al., 2021). A second group of organisms are those with a spiny or multibranched surface. This group includes species that never provide a suitable substrate for settlement and others that may eventually provide a suitable substrate, as happens when the mud tubes of amphipods become stiffer (Miyake et al., 2002). The third group includes bivalves, the calcareous tubes of polychaetes, balanoids, and, in general, organisms that build hard structures and increase the available substrate for polyps to attach to (Miyake et al., 2002; Rekstad et al., 2021; Zang et al., 2023). Scyphozoan polyp predators include crustaceans, nudibranchs, and gastropods with consumption rates greater than 300 polyps × ind-1 × day-1 (Arai, 2005; Takao et al., 2014; Feng et al., 2017; Tang et al., 2021). Intraguild predation among scyphozoan polyps has also been observed in laboratory studies co-culturing multiple species (Tang et al., 2020). The overall balance between polyp asexual reproduction, competition, predation, and physiological stress may benefit the scyphozoan polyps during the initial stages of colonization of a bare substrate (Feng et al., 2021; Boughton et al., 2023), but it is reversed within months, leading to severe reductions in scyphozoan polyp densities or even the disappearance of polyp colonies of certain species (Hernroth and Gröndahl, 1983; Feng et al., 2017; Feng et al., 2021).
2.3 Strobila and post-strobila stages
The strobilation process involves the reabsorption of polyp tentacles and the differentiation of segments that will eventually produce ephyrae at the oral end of the scyphistoma (Schiariti et al., 2008; Fuentes et al., 2011). Triggering of the process occurs following physical or chemical signals (Holst, 2012; Lucas et al., 2012; Helm, 2018). The molecular mechanism of strobilation is not fully understood, but the candidate hormones that induce the process exhibit strong temperature regulation during the polyp stage (Fuchs et al., 2014; Brekhman et al., 2015; Ge et al., 2022) and show structural similarities to certain compounds used in aquaria to chemically trigger the process (Spangenberg, 1965; Helm, 2018). Temperature must act in concert with other factors to induce strobilation: in tropical ecosystems with low thermal oscillations, strobilation can be signaled by salinity shifts (Lucas et al., 2012; Helm, 2018), zooxanthellate bearing species must host their symbionts and be exposed to both light and temperature appropriate conditions (Kikinger, 1992; Prieto et al., 2010; Schiariti et al., 2014), and there is a food requirement for polyps to strobilate (Wang et al., 2015; Zang et al., 2023). In non-zooxanthellate species, where the light and symbionts are not required, the microbiota organisms still play a key role in the strobilation, as the process is inhibited by a downregulation of the genetic pathway in their absence (Jensen et al., 2023).
The seasonal appearance of the medusa phase in most of the scyphozoan species is indicative of a temperature regulated strobilation process (Holst, 2012; Fernández-Alías et al., 2021), but there are reports of ephyra appearing at temperatures unsuitable for their posterior development (Fernández-Alías et al., 2023). Incorrect strobilation signaling can be problematic for the completion of the life cycle as it is a stressful process for the scyphozoan polyps. In most species, the calix diameter of the polyp shrinks after ephyra release (Feng et al., 2017), and the remnant is not always able to recover. In C. tuberculata, the polyp population completely disappears shortly after the strobilation (Kikinger, 1992; Prieto et al., 2010), and the polyps of Cyanea nozakii Kishinouye, 1891, Rhopilema esculentum Kishinouye, 1891, and Nemopilema nomurai Kishinouye, 1922 were unable to recover their original size and eventually disappeared in the field experiment by Feng et al. (2017). In contrast, R. pulmo has been reported to regrow the tentacles within two weeks after strobilation (Fuentes et al., 2011), and Rhopilema nomadica Galil, Spanier and Ferguson, 1990 can undergo multiple strobilation events while reproducing asexually (Lotan et al., 1992). As a result, some scyphozoan polyp cultures can be maintained in an aquarium for years (Lucas et al., 2012), but their ability to reproduce asexually may decline with successive generations (Chi et al., 2022). However, Holst (2012) observed that Cyanea capillata (Linnaeus, 1758) produced more ephyrae per polyp in older polyps, but the latter development to the medusa phase was not recorded and the overall effect on jellyfish population dynamics remains unknown.
2.4 Ephyra stage
The ephyra stage is not typically reported to limit the magnitude of the jellyfish blooms, but there is evidence that ephyra peaks followed by a massive mortality reduce the abundance of the medusa phase and even lead to an absolute absence of the same (Fernández-Alías et al., 2023). This occurs when a strobilation event occurs outside the appropriate temperature range for the development of the ephyrae, as there are upper and lower thresholds for the transition from ephyra to medusa (Astorga et al., 2012; Fernández-Alías et al., 2023).
The transition from ephyra to medusa involves a somatic growth, fusion of the rhopaliar lappets by the extension of the umbrella, the development of a gastric system, oral arms, and tentacles, and can last from 10 to 150 days, depending on the species and the environmental conditions (Holst et al., 2007; Fuentes et al., 2011; Astorga et al., 2012; Fernández-Alías et al., 2020; Gueroun et al., 2020; Fernández-Alías et al., 2023). During this period, ephyrae are exposed to natural mortality and intraguild predation by scyphozoan polyps, ephyrae, and medusae (Carrizo et al., 2016; Avian et al., 2021; Stoltenberg et al., 2021; Wang P. et al., 2023). To maintain the growth rate of the ephyra and increase the likelihood of completing the transition from ephyra to medusa, food requirements must be matched in quantity and quality (Chambel et al., 2016; Miranda et al., 2016; Ballesteros et al., 2022).
Mortality at this stage is rarely addressed, but it is likely to influence the development of the bloom. In the most conspicuous blooms of C. tuberculata, where ephyra abundance was also assessed, mortality at this stage was less than 5%, but the average mortality of C. tuberculata ephyra is around 70% and greatly affects the intensity of the bloom (Fernández-Alías et al., 2023). In the case of the moon jellyfish, Aurelia spp., the minimum recorded mortality at the ephyra stage is over 70%, the average mortality is over 90% and the maximum mortality is over 99% (Ishii et al., 2004; Fernández-Alías et al., 2023). The most likely factors involved in ephyrae mortality are physiological stress or predation (Carrizo et al., 2016; Stoltenberg et al., 2021; Fernández-Alías et al., 2023), but the absence of microbiota results in deformed ephyra after strobilation (Jensen et al., 2023), whose correct development is unlikely.
2.5 Medusa phase
The first observations in the water column of some individuals in their medusa phase can be a sign of an outshore bloom whose individuals have not yet been drifted ashore by winds or tides (Zavodnik, 1987; Keesing et al., 2016), an early bloom warning or an unsuccessful inshore jellyfish bloom (Fernández-Alías et al., 2023). The importance of the medusa phase goes beyond the ecosystem services and disturbances resulting from the bloom that occurs, as they can carry from thousands to millions of planulae (Kikinger, 1992; Lucas, 1996). The underlying biological reason for the occurrence of swarms or aggregations of medusae during the bloom events is not fully understood, but it has been argued that the reproductive success and the protection from predators could be enhanced by these behaviors (Hamner and Dawson, 2009; Fernández-Alías et al., 2021).
In this line, the scyphozoan jellyfish have traditionally been considered as a trophic dead end, but their trophic role has probably been underestimated as their absence in stomach contents can be explained by their extremely high digestion rate (Ates, 1988; Arai et al., 2003; Hays et al., 2018). Recent analyses, including increased data collection of stomach contents, direct observations, stable isotope analyses, DNA metabarcoding, and animal-borne cameras have significantly increased our knowledge of gelatinous zooplankton predation (Hays et al., 2018). The wide variety of organisms that predate on scyphozoan medusae include sea anemones, corals, starfish, brittle stars, sea cucumbers, balanoids, amphipods, decapods, fishes, flying seabirds, penguins, turtles, and other scyphozoan jellyfish (Ates, 1988; Arai, 2005; Titelman et al., 2007; Heaslip et al., 2012; Thiebot et al., 2016; Ates, 2017; McInnes et al., 2017; Thiebot et al., 2017; Hays et al., 2018; Wang P. et al., 2023).
The interest in studying the link between scyphozoan jellyfish and predators is partly driven by the parasites that the scyphozoan medusae harbor as intermediate hosts before infecting commercially exploited fish (Browne, 2014; Kondo et al., 2016; Motta et al., 2023). This developmental stage can harbor parasites with single host life cycles, such as amphipods or anemones, and parasites with multiple host life cycles, such as digeneans and cestodes (Arai, 2005; D’Ambra and Graham, 2009; Diaz Briz et al., 2012; Browne, 2014). The high prevalence of parasites in scyphozoan medusae suggests that a fundamental part of the parasite life cycle may occur in jellyfish (Diaz Briz et al., 2012), providing benefits such as protection, feeding or transport (Sal Moyano et al., 2012; Gonçalves et al., 2022), but the effect of parasites on the scyphozoan life cycle is poorly understood (D’Ambra and Graham, 2009). This infection may be important for life cycle completion, as somatic growth, gonad size, and egg production of scyphozoan medusae may be reduced by parasitism (Chiaverano et al., 2015).
The final factor influencing the medusa phase of the scyphozoan jellyfish life cycle is fishing, which can promote or disrupt the biological strategies of scyphozoan species, depending on the species targeted by the fishery (Lynam et al., 2006; Brotz, 2016). Overfishing of filter-feeding fish has promoted a shift in the system toward jellyfish dominance in some locations (Lynam et al., 2006), but this has not occurred when an alternative stock of filter-feeding fish could replace the original species (Schwartzloze et al., 1999). Similarly, filter-feeding fishes may benefit from the collapse of a predatory fish fishery (Mullon et al., 2005). In these cases, there is no increase in jellyfish abundance (Richardson et al., 2009), but they are indicative of the complexity of the system. This can be further illustrated by the case of salmon fisheries on the Pacific coast of North America, where the population of the sea nettle Chrysaora fuscescens Brandt, 1835 showed a complex pattern of zooplanktonic relationships that affected its abundance as well as the fisheries for the targeted salmon species (Ruzicka et al., 2016).
Scyphozoan jellyfish are also targeted species, and the impact of their fisheries on population dynamics must also be discussed. Indeed, jellyfish fisheries have become increasingly important worldwide, with more than 20 countries reporting their activity and average annual jellyfish landings exceeding 7.5×108 kg (Brotz, 2016; Brotz et al., 2017), but the biological parameterization of the species necessary to develop sustainable fishing programs has only been carried out for certain species and locations (Palomares and Pauly, 2008; Brotz, 2016; Fernández-Alías et al., 2020; López-Martínez et al., 2020; Leoni et al., 2021a; Behera et al., 2022). Consequently, the overfishing has been identified as the main cause of the collapse of the jellyfish fisheries of R. esculentum in China (Dong et al., 2014) and of Stomolophus meleagris Agassiz, 1860 in Mexico (Brotz et al., 2021). However, it is important to consider that jellyfish populations fluctuate naturally, and this is one of the main challenges in establishing long-term, productive jellyfish fisheries. For example, to maintain the economic productivity of the R. esculentum fishery in China, a stock enhancement program was developed to maintain and increase the catches in anticipation of a population collapse due to overfishing (Dong et al., 2009). In contrast, the collapse of the C. tuberculata population in the Mar Menor coastal lagoon (Spain) occurred after the jellyfish removal program was suspended (Fernández-Alías et al., 2022; Fernández-Alías et al., 2023), suggesting that overfishing is not the only cause of population collapse.
2.6 Non-metagenic life cycle
The scyphozoan species do not require a metagenic life cycle to bloom (Hamner and Dawson, 2009). The most relevant holopelagic jellyfish of the class Scyphozoa capable massive proliferation Pelagia noctiluca (Forsskål, 1775) and Periphylla periphylla (Péron and Lesueur, 1810). The set of factors affecting their life cycle is likely to be similar to that described previously in this review, but their lack of a benthic stage justifies the inclusion of an additional section to discuss the transitions from planula to ephyra (P. noctiluca) and from fertilized egg to medusa (P. periphylla).
The mauve stinger, P. noctiluca, is one of the most conspicuous scyphozoan species in the western Mediterranean (Canepa et al., 2014). This species lacks a benthic phase, and the planula larva transforms directly into an ephyra (Canepa et al., 2014; Ramondenc et al., 2019; Ballesteros et al., 2021). The medusa stage can survive for more than one year (Lilley et al., 2014), shows different reproduction peaks during the same (Milisenda et al., 2018), and shows a heterogeneous bloom pattern and spatial variability across the Mediterranean Sea (Marambio et al., 2021; Pastor-Prieto et al., 2021, Bellido et al., 2020). The population dynamics seems to follow a regular seasonality (Benedetti-Cecchi et al., 2015) with the spawning period adapting to the most suitable temperature frame for the transition from planula to ephyra (Rosa et al., 2013; Milisenda et al., 2018). This seasonality is likely to be a product of evolutionary selection, as the larval survival rate through the planula to ephyra transition decreases from 50% to approximately 12% when the temperature requirements are not met (Rosa et al., 2013). However, during the medusa spawning period, when the gonads of P. noctiluca reach their largest size (Milisenda et al., 2018), certain fish species have been reported to selectively feed on these organs, which are more nutritious than the somatic tissues (Milisenda et al., 2014), but the impact of this selective foraging over the species dynamics requires further exploration. Finally, during the ephyra stage, as is the case for the species with a metagenic life cycle, food requirements must to be matched in quantity and quality for its proper development to the medusa phase (Ballesteros et al., 2022).
P. periphylla is one of the scyphozoan blooming species that deviates the most from the metagenic life cycle and from the ‘boom and boost’ appearance of the medusae. In this species the medusae appear after direct transformation from the fertilized egg, thus, lacking not only the benthic phase, but also the planula and ephyra stages (Jarms et al., 1999). Moreover, the medusa stage has a low growth rate, and a lifespan of several years (Jarms et al., 1999; Båmstedt, 2023). The number of oocytes carried by a female P. periphylla increases with the medusa size and can be as high as 1000 oocytes per female in a 12 cm diameter, 9 year old female (Båmstedt et al., 2020; Båmstedt, 2023). This species has only been found in significant numbers in fjords, where there appears to be no predation or parasitism on the medusae, thus contributing to their longevity (Fosså, 1992). Within the fjords, P. periphylla medusae make vertical migrations to the surface during the night for reproductive purposes, allowing the fertilized eggs to sink to depths that prevent their predation by visual predators (Båmstedt et al., 2020), but leaving them vulnerable to the deep-water renewal (Båmstedt, 2023). Despite this strategy, and analogous to the planula settlement or the ephyra to medusa transition, the mortality in the fertilized egg to medusa transition exceeds 90% and can be almost complete in most cases (Båmstedt, 2023).
2.7 Combined effect of the control points over the life cycle
The predictability of jellyfish blooms is extremely limited because the “never-ending jellyfish joyride” is in fact strongly influenced by non-typically measured external conditions (Table 1). The magnitude of the link between planula settlement, polyp development, and medusae population size at any temporal and spatial scale is unexplored (Gibbons et al., 2016), but from our review it can be inferred that the blockage of the life cycle in any of the stages by any of the aforementioned factors can limit the occurrence of future blooms, while, on the other hand, the success of the different developmental stages may increase the number of individuals by orders of magnitude from tens, asexually in the benthic phase, to millions, sexually in the pelagic phase (Figure 2; Table 1). This means that the collapse of a scyphozoan population can be followed by a sudden explosive recovery of the same through the survival of sparse medusae from the previous season or through the reception of a few new individuals that restore the population. Examples of the same are the recovery of the population of Mastigias sp. Agassiz, 1862 in the Jellyfish Lake (Palau) (Martin et al., 2006) or the recovery of the population of C. tuberculata in the Mar Menor (Spain) (Fernández-Alías and Pérez-Ruzafa, 2023). In the case of Mastigias sp., the collapse of the population was attributed to a warming of the waters beyond its tolerance limit, and the recovery of the same to a decrease in water temperature, both physical events driven by El Niño/Southern Oscillation (ENSO) (Dawson et al., 2001; Martin et al., 2006). For C. tuberculata, the control of the population is part of a complex top-down and bottom-up equilibrium in which phytoplankton could prevent the light from reaching the substrate, thus inhibiting the strobilation, while C. tuberculata predates on the phytoplankton and the symbiont zooxanthellae compete with the same for nutrient uptake (Pérez-Ruzafa et al., 2002; Fernández-Alías et al., 2020; Fernández-Alías et al., 2022; Fernández-Alías and Pérez-Ruzafa, 2023; Fernández-Alías et al., 2023).

Table 1 Effect and magnitude of the different factors modulating the biological processes of the developmental stages during the scyphozoan life cycle.
3 Main challenges and future research directions
The small size and fragility of the larval stages (planula, polyp and ephyra) of scyphozoan species has limited the number of field studies on them, as it is difficult to find them and monitor their interactions with biotic and abiotic factors (Feng et al., 2017; Marques et al., 2019; Fernández-Alías et al., 2020; van Walraven et al., 2020; Leoni et al., 2021a; Boughton et al., 2023; Fernández-Alías et al., 2023; Zang et al., 2023). However, far from being discouraging, it should be noted that since the proposal of the conceptual framework for a general increase of jellyfish in the ocean (Arai, 2001; Purcell, 2005; Purcell et al., 2007; Richardson et al., 2009; Purcell, 2012), our knowledge of the factors that contribute to modulate the intensity of proliferations has greatly increased (Table 1).
Nevertheless, we are still far from developing truly useful predictive modeling tools, given the limited number of species for which each individual factor has been studied (Table 1) and the asymmetric response of different scyphozoan species to biotic and abiotic factors (Purcell et al., 1999; Schiariti et al., 2014; Feng et al., 2017; Fernández-Alías et al., 2021; Fernández-Alías et al., 2023). This likely limits the reliability of multispecies analyses across broad spatial and temporal scales (Brotz et al., 2012; Condon et al., 2013), but reinforces the need to build models that are site- and species-specific (Fernández-Alías et al., 2021). In this line, we propose a future research path that addresses the main knowledge gaps and sources of variability in jellyfish abundance to improve our understanding of the complex interactions (Figure 3).
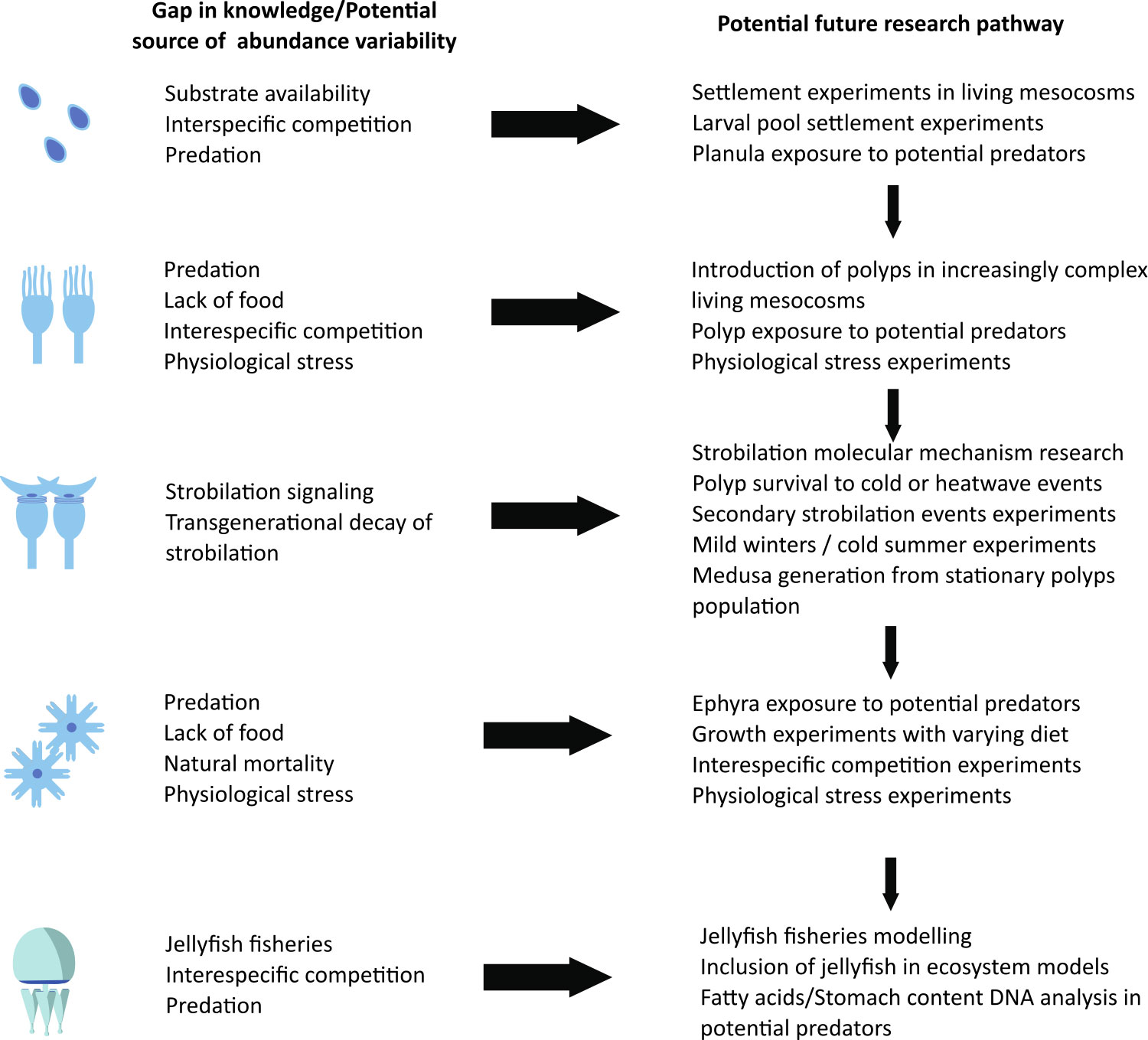
Figure 3 Main potential sources of abundance variability and gaps in knowledge along the potential research pathway to improve our forecast on scyphozoan jellyfish blooms.
3.1 Planula stage
The planula stage is affected by physiological stress, interspecific competition for the substrate, and predation. However, even if suboptimal environmental conditions for planula settlement reduce the success of this biological process (Table 1), in nature planulae are typically released under optimal settlement conditions (Prieto et al., 2010; Rosa et al., 2013; Franco, 2016; Boughton et al., 2023). Therefore, we believe that planula settlement is more likely to be constrained by substrate availability and predation. We propose a potential research pathway to increase our knowledge of planula settlement efficiency in nature: testing the effects of substrate competition with both other potential settlers and previous colonizers, and calculating the effect of predation on planulae over the initial polyp population density.
Planulae are known to settle efficiently on bare substrate (Prieto et al., 2010; Franco, 2016; Boughton et al., 2023; Holst et al., 2023), but the competition for hard substrate in the ocean is one of the clearest examples of competition in nature (Dial and Roughgarden, 1998; Connolly and Roughgarden, 1999). This substrate is typically dominated by species with an adult benthic phase, but the experimental designs of planula settlement have mostly been conducted on non-living substrates. The only examples we are aware of are a settlement experiment of C. tuberculata’s planulae on living oyster’s shells with 0% settlement success (Franco, 2016), and a second in which C. nozakii’s planulae were uncapable of settling on plates heavily colonized with biofouling organisms (Feng et al., 2021). Therefore, we suggest conducting planula settlement experiments in living mesocosms with bare and colonized substrate.
Marine species with a benthic adult phase have pulses of reproductive activity when the larvae are released into the water column before resettling to complete the life cycle (e.g. Gittings et al., 1992; Cárdenas and Aranda, 2000), and planulae compete with the larval pool present in the water column for the space in which to settle (Boughton et al., 2023). However, the observation of this competition is based on a single scyphozoan species and ecosystem (Boughton et al., 2023), and a quantification of the magnitude of the competition is lacking (Table 1). This highlights the need for settlement experiments with a larval pool of potential colonizers rather than just the scyphozoan planulae.
Finally, even though there is some evidence for the presence of planulae’s predators (Javidpour et al., 2009; Kuplik et al., 2015), the effect of planula removal by naturally present predators on population dynamics is unknown, indicating the need for further research on planula exposure to potential predators.
3.2 Polyp stage
The polyp stage faces a similar set of factors as the planula stage (Table 1), but contrary to what happened to the planulae, the perennial presence of polyps in most scyphozoan life cycle strategies (Fernández-Alías et al., 2021) implies that the role of physiological stress is higher in this stage. In fact, the further away the polyp population is from its optimal conditions, the lower the asexual reproduction rate and the higher the polyp mortality rate (Prieto et al., 2010; Wang F. et al., 2023). Therefore, when constructing polyp density models, it is important to consider where the thresholds of tolerance are and how long the benthic population is exposed to these inadequate conditions. Our information on these thresholds is limited to a few species, and yet it is sufficient to determine that they are species specific (Purcell et al., 1999; Schiariti et al., 2014). Thus, it is a prerequisite to know the thresholds, the decline in asexual reproduction, and the survival of the modeled species under inadequate conditions.
Most studies of polyp population dynamics have been conducted in the absence of competitors, synergistic organisms, or predators (Prieto et al., 2010; Purcell et al., 2012; Schiariti et al., 2014; Treible and Condon, 2019). These interactions should not be neglected, as their overall balance may be detrimental to the scyphozoan polyp populations (Feng et al., 2017; Zang et al., 2023). To fully understand polyp dynamics, it is necessary to increase the biological complexity of the mesocosms in which the physiological stress experiments are conducted.
3.3 Strobila and post-strobila stages
Strobilation is a stressful process for polyp populations (Feng et al., 2017), and their posterior recovery is not guaranteed in some species, while others have better chances (Prieto et al., 2010; Fuentes et al., 2011; Feng et al., 2017). In this context, it remains unclear why certain conditions, which could be cold or heat waves, trigger the strobilation process, when their return to normal water temperatures could affect the correct development of ephyrae into medusae (Fernández-Alías et al., 2023). It has also been shown that the strobilation process and the ephyra production are affected by the minimum sea temperature reached during the winter season, the length of the winter season, the water warming speed and the food regime (Feng et al., 2015a; Feng et al., 2015b; Loveridge et al., 2021; Zang et al., 2022), factors that should be further studied to increase our bloom predicting tools.
A better understanding of the process could be achieved with a complete description of the molecular mechanism of strobilation (Helm et al., 2018), but for now our knowledge of the molecular pathway is limited to certain aspects and to the genus Aurelia (Fuchs et al., 2014; Brekhman et al., 2015; Khalturin et al., 2019; Jensen et al., 2023). In fact, we are not aware of the presence of the gene CL390, the most overexpressed gene in the strobilation process (Fuchs et al., 2014; Brekhman et al., 2015), in any other scyphozoan species outside this genus. In the nucleotide database (GenBank) of the National Center for Biotechnology Information (https://www.ncbi.nlm.nih.gov/, accessed on 30 November 2023) there are only 4 DNA sequences of the gene translated into this protein, all of them belonging to Aurelia, and launching a Basic Local Alignment Search Tool (BLAST) with the most permissive parameters does not provide any additional sequence from a scyphozoan species (Fernández-Alías, A., unpublished result). Of the four available sequences, only two are supported by a publication, the one sequenced in Fuchs et al. (2014) and the one from Brekhman et al. (2015). Moreover, their transcribed proteins have only 68% identity (Brekhman et al., 2015), and their expression is induced by opposite temperature changes, a decrease in the case of the Fuchs et al. (2014) gene and an increase in the case of the Brekhman et al. (2015) gene. Therefore, there is a need for more transcriptomic analysis throughout the life cycle of the different scyphozoan species.
Finally, polyp populations have been reported to decrease their asexual reproduction rate within successive generations, but this may be associated with a higher ephyra production per polyp (Chi et al., 2022; Holst et al., 2023), and the overall balance of long-lived polyp populations in ephyra production remains unclear. In addition, medusae developed from long-lived polyp cultures in the aquarium may show abnormal pulsation or even absence of pulsation (Fernández-Alías, pers. obs.), suggesting a need for refreshment of benthic populations.
3.4 Ephyra stage
It takes between 10 and 150 days for the ephyrae to reach the medusa stage, with an average success rate of less than 30% (Ishii et al., 2004; Kawahara et al., 2006; Fuentes et al., 2011; Astorga et al., 2012; Fernández-Alías et al., 2020; Gueroun et al., 2020; Fernández-Alías et al., 2023). Predation and physiological stress have been identified as the two most important factors affecting mortality at the ephyra stage (Table 1), but evidence is limited. The extremely fast digestion rate of the medusa phase has made it difficult to detect them as prey by stomach content analysis (Arai et al., 2003), and given their smaller size, the lack of detection of the ephyra phase in predator stomachs should be more acute. In fact, all the collected evidence on predation of ephyrae is based on their direct exposure to predators in aquarium experiments (Table 1), and the list of potential predators is likely to be much more extensive than those observed so far. The composition of the planktonic community available for the ephyrae to prey on is likely to influence proper development to the medusa stage (Chambel et al., 2016; Miranda et al., 2016; Ballesteros et al., 2022), suggesting the need to monitor the plankton assemblages along the ephyrae and medusae and conduct experiments to determine the most appropriate diet for the ephyrae. Finally, the disappearance of ephyrae when temperature conditions were inadequate has been observed in long-term studies (Fernández-Alías et al., 2023), but they did not discriminate whether temperature was the primary cause of mortality or whether it was starvation or the long-term exposure to potential predators without reaching the medusa phase. Thus, physiological stress experiments need to be conducted to determine the resilience of the ephyrae to inadequate conditions for their development.
3.5 Medusa phase
Once a bloom of a species occurs, interest usually shifts to the socioeconomic impacts of the massive proliferation and how to mitigate them (Bosch-Belmar et al., 2020), and less attention is paid to the implications of its development for subsequent seasons. In fact, attention to future blooms seems to have gained interest only since the development of jellyfish fisheries threatened by interannual variability in medusae abundance (Brotz et al., 2017), and yet not many efforts have been made to calculate the biological parameters required to implement sustainable fishing programs (Palomares and Pauly, 2008; Brotz, 2016; Fernández-Alías et al., 2020; López-Martínez et al., 2020; Leoni et al., 2021a; Behera et al., 2022). Traditionally considered a trophic “dead end” (Hays et al., 2018), medusae are not typically included in ecosystem management models (Pauly et al., 2008), and larval stages are even less likely to be included in these models, despite their potential to influence adult abundance (Table 1). The inclusion of jellyfish in ecological models is particularly important in small, sheltered ecosystems, since the smaller the ecosystem, the greater the importance of jellyfish as keystone species (Pauly et al., 2008), to the point where they can act as top pelagic predators and as a buffer against dystrophic crises in eutrophicated coastal lagoons (Pérez-Ruzafa et al., 2002; Fernández-Alías et al., 2022; Fernández-Alías et al., 2023). In this line, it is also important to consider that the trophic role of scyphozoan jellyfish changes between species, within species between locations, and throughout their ontogeny (Holst et al., 2007; Marques et al., 2020; Wang et al., 2020; Leoni et al., 2022). For the correct implementation of these models, we need to put more effort in the parameterization of jellyfish growth and in the determination of trophic links with upper and lower levels.
4 Conclusions
The scyphozoan life cycle has the potential to numerically increase the number of individuals in the order of several million during a single generation, but the contour conditions can block the life cycle and cause a collapse of the population. The complex interactions between the different developmental stages of the life cycle and the set of biotic and abiotic factors are the main causes of the interannual variability in the abundance of the medusa phase, the most conspicuous stage of the life cycle. During the last decade we have increased our knowledge of the controlling factors that modulate the densities of the different developmental stages, but we are still far from being able to provide reliable predictions of the scyphozoan blooms. Therefore, it is important to distinguish between what it is known and what remains a gap in knowledge to effectively direct the future research on this topic.
Temperature seems to be the most important factor involved in the correct development of the life cycle, triggering processes and favoring the transition between phases when it is optimal, and causing mass mortality or abnormal development when it is not. The second important factor is the availability of food, the quality and quantity of which modulate the asexual reproduction, the strobilation process and the somatic growth. In general, the factors regulating the transition between the different stages of the life cycle would be what allows the species to anticipate adequate conditions for the development and growth of the individuals. In this context, food availability, productivity, and temperature act in concert with salinity, being it a key factor in many tropical and sheltered habitats, prone to host scyphozoan blooms, where salinity fluctuates due to storms, flash floods, and evapotranspiration, affecting the life cycle and the species distribution. In the same way, salinity may play a key role in the Mediterranean Sea under temperature rising scenarios. Despite their importance, these factors are reported to act asymmetrically between the different scyphozoan species, and here appears one of the most important limitations in our ability to predict massive proliferations: the knowledge we have generated is species-specific and cannot be extrapolated to the whole class. Moreover, for most of the species the magnitude of the effect of these factors in some (or in all) of the biological processes that take place during the life cycle is unknown.
A second gap in our knowledge is the interspecific interactions between the scyphozoan species and the other faunistic groups. As a scientific community, we have reported the existence of numerous relationships between scyphozoan jellyfish and the other groups, including predator-prey interactions, both in the benthic and the pelagic stages, competition for the substrate in the planula settlement and polyp dynamics, symbiosis, commensalism, and parasitism. However, the description of the relationships is still in its infancy, not yet quantified and most likely we still underestimate the number of predators and competitors for the different stages.
To improve our forecasting ability, our research should focus on determining the parameters needed to implement ecosystem-based models. This includes determining the environmental tolerance limit for each species and stage, parameterizing how the growth, asexual reproduction, strobilation and mortality rates vary under inadequate conditions, and determining the interspecific and trophic relationships by progressively increasing the complexity of the design of the mesocosms in which the experiments are conducted.
Author contributions
AF-A: Conceptualization, Formal analysis, Visualization, Writing – original draft, Writing – review & editing. CM: Funding acquisition, Project administration, Writing – review & editing. APR: Conceptualization, Funding acquisition, Project administration, Supervision, Visualization, Writing – review & editing.
Funding
The author(s) declare financial support was received for the research, authorship, and/or publication of this article. This study has been supported by the project Monitoring the Ecological State of the Mar Menor funded by the General Directorate of the Mar Menor of the Community of the Region of Murcia. AF-A was supported by Fundación Séneca, Región de Murcia (Spain), grant number 21449/FPI/20. This work has also benefited from funding from the different projects on “Monitoring and predictive analysis of the ecological state evolution of the Mar Menor lagoon ecosystem and prevention of impacts (2016–2023)” financed by the General Directorate of the Mar Menor of the Autonomous Community of the Region of Murcia.
Acknowledgments
This study was conducted as part of the Ph.D. dissertation of AF-A. We extend our gratitude totwo reviewers whose comments improved the quality of the manuscript.
Conflict of interest
The authors declare that the research was conducted in the absence of any commercial or financial relationships that could be construed as a potential conflict of interest.
The author(s) declared that they were an editorial board member of Frontiers, at the time of submission. This had no impact on the peer review process and the final decision.
Publisher’s note
All claims expressed in this article are solely those of the authors and do not necessarily represent those of their affiliated organizations, or those of the publisher, the editors and the reviewers. Any product that may be evaluated in this article, or claim that may be made by its manufacturer, is not guaranteed or endorsed by the publisher.
References
Arai M. N. (2001). Pelagic coelenterates and eutrophication: A review. Hydrobiologia 451, 69–87. doi: 10.1023/A:1011840123140
Arai M. N. (2005). Predation on pelagic coelenterates: a review. J. Mar. Biol. Assoc. United Kingdom 85 (3), 523–536. doi: 10.1017/S0025315405011458
Arai M. N., Welch D. W., Dunsmuir A. L., Jacobs M. C., Ladouceur A. R. (2003). Digestion of pelagic Ctenophora and Cnidaria by fish. Can. J. Fisheries Aquat. Sci. 60 (7), 825–829. doi: 10.1139/f03-071
Astorga D., Ruiz J., Prieto L. (2012). Ecological aspects of early life stages of Cotylorhiza tuberculata (Scyphozoa: Rhizostomae) affecting its pelagic population success. Hydrobiologia 690, 141–155. doi: 10.1007/s10750-012-1036-x
Ates R. M. (2017). Benthic scavengers and predators of jellyfish, material for a review. Plankton Benthos Res. 12 (1), 71–77. doi: 10.3800/pbr.12.71
Avian M., Motta G., Prodan M., Tordoni E., Macaluso V., Beran A., et al. (2021). Asexual reproduction and strobilation of Sanderia malayensis (Scyphozoa, Pelagiidae) in relation to temperature: experimental evidence and implications. Diversity 13 (2), 37. doi: 10.3390/d13020037
Ballesteros A., Östman C., Santín A., Marambio M., Narda M., Gili J. M. (2021). Cnidome and morphological features of Pelagia noctiluca (Cnidaria: Scyphozoa) throughout the different life cycle stages. Front. Mar. Sci. 8. doi: 10.3389/fmars.2021.714503
Ballesteros A., Paez D., Santín A., García A., Martin Y., Alonso E., et al. (2022). Successful culture of Pelagia noctiluca (Cnidaria: Scyphozoa) over time: a continuous supply of the holoplanktonic jellyfish for research and industrial applications. Front. Mar. Sci. 9. doi: 10.3389/fmars.2022.911383
Båmstedt U. (2023). Life history traits of the deep-water medusa Periphylla periphylla as revealed through failure and recovery of recruitment. Estuar. Coast. Shelf Sci. 282, 108205. doi: 10.1016/j.ecss.2022.108205
Båmstedt U., Sötje I., Tiemann H., Martinussen M. B. (2020). Fecundity and early life of the deep-water jellyfish Periphylla periphylla. J. Plankton Res. 42 (1), 87–101. doi: 10.1093/plankt/fbz076
Bayha K. M., Graham W. M., Higgins J. E. III, Fletcher H. A. (2012). Predation potential of the jellyfish Drymonema larsoni Bayha & Dawson (Scyphozoa: Drymonematidae) on the moon jellyfish Aurelia sp. in the northern Gulf of Mexico. Hydrobiologia 690, 189–197. doi: 10.1007/s10750-012-1038-8
Behera P. R., Jishnudev M. A., Ghosh S., Saravanan R. (2022). Biometry and size distribution of Crambionella annandalei and Chrysaora spp. along the coast of Andhra Pradesh, India. Aquat. Ecol. 56, 227–237. doi: 10.1007/s10452-021-09911-z
Bellido J. J., Baez J. C., Souviron-Priego L., Ferri-Yanez F., Salas C., López J. A., et al. (2020). Atmospheric indices allow anticipating the incidence of jellyfish coastal swarms. Mediterr. Mar. Sci. 21 (2), 289–297. doi: 10.12681/mms.20983
Benedetti-Cecchi L., Canepa A., Fuentes V., Tamburello L., Purcell J. E., Piraino S., et al. (2015). Deterministic factors overwhelm stochastic environmental fluctuations as drivers of jellyfish outbreaks. PloS One 10 (10), e0141060. doi: 10.1371/journal.pone.0141060
Bosch-Belmar M., Milisenda G., Basso L., Doyle T. K., Leone A., Piraino S. (2020). Jellyfish impacts on marine aquaculture and fisheries. Rev. Fisheries Sci. Aquac. 29 (2), 242–259. doi: 10.1080/23308249.2020.1806201
Boughton J., Hirst A. G., Lucas C. H., Spencer M. (2023). Negative and positive interspecific interactions involving jellyfish polyps in marine sessile communities. PeerJ 11, e14846. doi: 10.7717/peerj.14846
Brekhman V., Malik A., Haas B., Sher N., Lotan T. (2015). Transcriptome profiling of the dynamic life cycle of the scypohozoan jellyfish Aurelia aurita. BMC Genomics 16 (1), 74. doi: 10.1186/s12864-015-1320-z
Brotz L. (2016). Jellyfish fisheries of the world. PhD Thesis (Vancouver, BC, Canada: University of British Columbia).
Brotz L., Cheung W. W. L., Kleisner K., Pakhomov E., Pauly D. (2012). Increasing jellyfish populations: trends in Large Marine Ecosystems. Hydrobiologia 690, 3–20. doi: 10.1007/s10750-012-1039-7
Brotz L., Cisneros-Montemayor A. M., Cisneros-Mata M. Á. (2021). The race for jellyfish: Winners and losers in Mexico’s Gulf of California. Mar. Policy 134, 104775. doi: 10.1016/j.marpol.2021.104775
Brotz L., Schiariti A., López-Martínez J., Álvarez-Tello J., Peggy Hsieh Y. H., Jones R. P., et al. (2017). Jellyfish fisheries in the Americas: origin, state of the art, and perspectives on new fishing grounds. Rev. Fish Biol. Fisheries 27, 1–29. doi: 10.1007/s11160-016-9445-y
Browne J. G. (2014). Parasites of jellyfish in eastern Australia. PhD Thesis (Brisbane, Australia: Griffith University).
Canepa A., Fuentes V., Sabatés A., Piraino S., Boero F., Gili J. M. (2014). “Pelagia noctiluca in the mediterranean sea,” in Jellyfish Blooms. Eds. Pitt K. A., Lucas C. H. (Netherlands: Springer), 237–266. doi: 10.1007/978-94-007-7015-7
Cárdenas E. B., Aranda D. A. (2000). A review of reproductive patterns of bivalve mollusks from Mexico. Bull. Mar. Sci. 66 (1), 13–27.
Carrizo S. S., Schiariti A., Nagata R. M., Morandini A. C. (2016). Preliminary observations on ephyra predation by Lychnorhiza lucerna medusa (Scyphozoa; Rhizostomeae). Der Zoologische Garten 85 (1-2), 74–83. doi: 10.1016/j.zoolgart.2015.09.011
Chambel J., Araújo T., Mendes C., Miranda F., Câncio L., Maranhão P., et al. (2016). “New marine ornamental species: the potential of Moon jellyfish Aurelia aurita,” in Frontiers in Marine Science Conference Abstract: IMMR| International Meeting on Marine Research. Peniche, Portuga: Frontiers In Marine Science. doi: 10.3389/conf.FMARS.2016.04.00047
Chi X., Zhang F., Sun S. (2022). Transgenerational effects and temperature variation alter life history traits of the moon jellyfish. Front. Mar. Sci. 9. doi: 10.3389/fmars.2022.913654
Chiaverano L. M., Graham W. M., Costello J. H. (2015). Parasites alter behavior, reproductive output, and growth patterns of Aurelia medusae in a marine lake. Mar. Ecol. Prog. Ser. 540, 87–98. doi: 10.3354/meps11513
Ciriaco S., Faresi L., Segarich M. (2021). Observations on the feeding of Drymonema dalmatinum in the gulf of Trieste. Diversity 13 (4), 163. doi: 10.3390/d13040163
Condon R. H., Duarte C. M., Pitt K. A., Robinson K. L., Lucas C. H., Sutherland K. R., et al. (2013). Recurrent jellyfish blooms are a consequence of global oscillations. Proc. Natl. Acad. Sci. 110 (3), 1000–1005. doi: 10.1073/pnas.1210920110
Connolly S. R., Roughgarden J. (1999). Theory of marine communities: competition, predation, and recruitment-dependent interaction strength. Ecol. Monogr. 69 (3), 277–296. doi: 10.1890/0012-9615(1999)069[0277:TOMCCP]2.0.CO;2
Costello J. H., Colin S. P., Dabiri J. O., Gemmell B. J., Lucas K. N., Sutherland K. R. (2021). The hydrodynamics of jellyfish swimming. Annu. Rev. Mar. Sci. 13, 375–396. doi: 10.1146/annurev-marine-031120-091442
D’Ambra I., Graham W. M. (2009). Early developmental sequence of an anthozoan parasite of the jellyfish Aurelia sp. 5 in an isolated marine lake (Mljet, Croatia). Annales: Series Historia Naturalis. 19 (2), 59–64.
Dawson M. N., Martin L. E., Penland L. K. (2001). Jellyfish swarms, tourists, and the Christ-child. Hydrobiologia 451, 131–144. doi: 10.1023/A:1011868925383
De Domenico S., De Rinaldis G., Mammone M., Bosch-Belmar M., Piraino S., Leone A. (2023). The zooxanthellate jellyfish holobiont Cassiopea andromeda, a source of soluble bioactive compounds. Mar. Drugs 21 (5), 272. doi: 10.3390/md21050272
De Rinaldis G., Leone A., De Domenico S., Bosch-Belmar M., Slizyte R., Milisenda G., et al. (2021). Biochemical characterization of Cassiopea andromeda (Forsskål 1775), another red sea jellyfish in the western mediterranean sea. Mar. Drugs 19 (9), 498. doi: 10.3390/md19090498
Dial R., Roughgarden J. (1998). Theory of marine communities: the intermediate disturbance hypothesis. Ecology 79 (4), 1412–1424. doi: 10.1890/0012-9658(1998)079[1412:TOMCTI]2.0.CO;2
Diaz Briz L. M., Martorelli S. R., Genzano G. N., Mianzan H. W. (2012). Parasitism (Trematoda, Digenea) in medusae from the southwestern Atlantic Ocean: medusa hosts, parasite prevalences, and ecological implications. Hydrobiologia 690 (1), 215–26. doi: 10.1007/s10750-012-1040-1
Di Camillo C. G., Betti F., Bo M., Martinelli M., Puce S., Bavestrello G. (2010). Contribution to the understanding of seasonal cycle of Aurelia aurita (Cnidaria: Scyphozoa) scyphopolyps in the northern Adriatic Sea. J. Mar. Biol. Assoc. United Kingdom 90 (6), 1105–1110. doi: 10.1017/S0025315409000848
Dobson J. Y., Fonfría E. S., Palacios R., Blasco E., Bordehore C. (2023). Citizen science effectively monitors biogeographical and phenological patterns of jellyfish. Ocean Coast. Manage. 242, 106668. doi: 10.1016/j.ocecoaman.2023.106668
Dong J., Jiang L. X., Tan K. F., Liu H. Y., Purcell J. E., Li P. J., et al. (2009). Stock enhancement of the edible jellyfish (Rhopilema esculentum Kishinouye) in Liaodong Bay, China: A review. Hydrobiologia 616, 113–118. doi: 10.1007/s10750-008-9592-9
Dong Z., Liu D., Keesing J. K. (2014). “Contrasting trends in populations of Rhopilema esculentum and Aurelia aurita in Chinese waters,” in Jellyfish blooms. Ed. Pitt K.A. and Lucas C. (Dordrecht: Springer). doi: 10.1007/978-94-007-7015-7_9
Dong Z., Wang L., Liu Q., Sun T. (2018). Effects of salinity and temperature on the recruitment of Aurelia coerulea planulae. Mar. Biol. Res. 14 (5), 454–461. doi: 10.1080/17451000.2018.1459725
Edelist D., Guy-Haim T., Kuplik Z., Zuckerman N., Nemoy P., Angel D. L. (2020). Phenological shift in swarming patterns of Rhopilema nomadica in the Eastern Mediterranean Sea. J. Plankton Res. 42 (2), 211–219. doi: 10.1093/plankt/fbaa008
Enrique-Navarro A., Huertas I. E., León Cobo M. J., Prieto L. (2021). Impact of ocean warming and ocean acidification on asexual reproduction and statolith formation of the symbiotic jellyfish Cotylorhiza tuberculata. PloS One 16 (8), e0254983. doi: 10.1371/journal.pone.0254983
Feng S., Lin J., Sun S., Zhang F., Li C., Xian W. (2020). Combined effects of seasonal warming and hyposalinity on strobilation of Nemopilema nomurai polyps. J. Exp. Mar. Biol. Ecol. 524, 151316. doi: 10.1016/j.jembe.2020.151316
Feng S., Lin J., Uye S. I., Sun S., Zhang F. (2021). Effects of salinity, light intensity and biofouling on planula settlement and subsequent development to polyps in Cyanea nozakii (Cnidaria: Scyphozoa). J. Exp. Mar. Biol. Ecol. 542, 151608. doi: 10.1016/j.jembe.2021.151608
Feng S., Wang S. W., Zhang G. T., Sun S., Zhang F. (2017). Selective suppression of in situ proliferation of scyphozoan polyps by biofouling. Mar. pollut. Bull. 114 (2), 1046–1056. doi: 10.1016/j.marpolbul.2016.10.062
Feng S., Zhang F., Sun S., Wang S., Li C. (2015a). Effects of duration at low temperature on asexual reproduction in polyps of the scyphozoan Nemopilema nomurai (Scyphozoa: Rhizostomeae). Hydrobiologia 754, 97–111. doi: 10.1007/s10750-015-2173-9
Feng S., Zhang G. T., Sun S., Zhang F., Wang S. W., Liu M. T. (2015b). Effects of temperature regime and food supply on asexual reproduction in Cyanea nozakii and Nemopilema nomurai. Hydrobiologia 754, 201–214. doi: 10.1007/s10750-015-2279-0
Fernández-Alías A., Marcos C., Perez-Ruzafa A. (2021). Larger scyphozoan species dwelling in temperate, shallow waters show higher blooming potential. Mar. pollut. Bull. 173, 113100. doi: 10.1016/j.marpolbul.2021.113100
Fernández-Alías A., Marcos C., Quispe J. I., Sabah S., Pérez-Ruzafa A. (2020). Population dynamics and growth in three scyphozoan jellyfishes, and their relationship with environmental conditions in a coastal lagoon. Estuar. Coast. Shelf Sci. 243, 106901. doi: 10.1016/j.ecss.2020.106901
Fernández-Alías A., Molinero J. C., Quispe-Becerra J. I., Bonnet D., Marcos C., Pérez-Ruzafa A. (2023). Phenology of scyphozoan jellyfish species in a eutrophication and climate change context. Mar. pollut. Bull. 194, 115286. doi: 10.1016/j.marpolbul.2023.115286
Fernández-Alías A., Montaño-Barroso T., Conde-Caño M. R., ManChado-Pérez S., López-Galindo C., Quispe-Becerra J. I., et al. (2022). Nutrient overload promotes the transition from top-down to bottom-up control and triggers dystrophic crises in a Mediterranean coastal lagoon. Sci. Total Environ. 846, 157388. doi: 10.1016/j.scitotenv.2022.157388
Fernández-Alías A., Pérez-Ruzafa A. (2023) Estado de las poblaciones de medusas en el Mar Menor (Actualización 6 de julio 2023). Report of the “Ecología y Ordenación de Ecosistemas Marinos Costeros” Research Group (Department of Ecology and Hydrology, University of Murcia, Spain). Available at: https://canalmarmenor.carm.es/wp-content/uploads/Estado-poblaciones-de-Medusas_06-Julio-2023.pdf (Accessed 30 November 2023).
Fosså J. H. (1992). Mass occurrence of Periphylla periphylla (Scyphozoa, Coronatae) in a Norwegian fjord. Sarsia 77 (3-4), 237–251. doi: 10.1080/00364827.1992.10413509
Franco I. (2016). “20 años de seguimiento e investigación sobre las poblaciones de medusas en el Mar Menor: Experimentos de laboratorio,” in Mar Menor: una laguna singular y sensible. Evaluación científica de su estado. Eds. León V. M., Bellido J. M. (Madrid: Instituto Español de Oceanografía, Ministerio de Economía y Competitividad), 133–156.
Fu Z., Xing Y., Gu Z., Liu C., Chen S. (2019). Effects of temperature, salinity and dissolved oxygen on excystment of podocysts in the edible jellyfish Rhopilema esculentum Kishinouye 1891. Indian J. Fish 66 (4), 69–77. doi: 10.21077/ijf.2019.66.4.91762-09
Fuchs B., Wang W., Graspeuntner S., Li Y., Insua S., Herbst E., et al. (2014). Regulation of polyp-to-jellyfish transition in Aurelia aurita. Curr. Biol. 24, 263–273. doi: 10.1016/j.cub.2013.12.003
Fuentes V., Straehler-Pohl I., Atienza D., Franco I., Tilves U., Gentile M., et al. (2011). Life cycle of the jellyfish Rhizostoma pulmo (Scyphozoa: Rhizostomeae) and its distribution, seasonality and inter-annual variability along the Catalan coast and the Mar Menor (Spain, NW Mediterranean). Mar. Biol. 158 (10), 2247–2266. doi: 10.1007/s00227-011-1730-7
Gambill M., McNaughton S. L., Kreus M., Peck M. A. (2018). Temperature-dependent settlement of planula larvae of two scyphozoan jellyfish from the North Sea. Estuar. Coast. Shelf Sci. 201, 64–71. doi: 10.1016/j.ecss.2016.08.042
Ge J., Chen X., Liu C., Tan J., Bian L., Chen L., et al. (2022). Metabolomics provide insights into the endogenous mechanism of strobilation in the scyphozoan jellyfish Rhopilema esculentum. J. Oceanol. Limnol. 40 (1), 226–234. doi: 10.1007/s00343-021-0252-5
Gemmell B. J., Colin S. P., Costello J. H., Dabiri J. O. (2015). Suction-based propulsion as a basis for efficient animal swimming. Nat. Commun. 6 (1), 8790. doi: 10.1038/ncomms9790
Gemmell B. J., Costello J. H., Colin S. P., Stewart C. J., Dabiri J. O., Tafti D., et al. (2013). Passive energy recapture in jellyfish contributes to propulsive advantage over other metazoans. Proc. Natl. Acad. Sci. United States America 110 (44), 17904–17909. doi: 10.1073/pnas.1306983110
Gemmell B. J., Du Clos K. T., Colin S. P., Sutherland K. R., Costello J. H. (2021). The most efficient metazoan swimmer creates a ‘virtual wall’to enhance performance. Proc. R. Soc. B 288 (1942), 20202494. doi: 10.1098/rspb.2020.2494
Gibbons M. J., Boero F., Brotz L. (2016). We should not assume that fishing jellyfish will solve our jellyfish problem. ICES J. Mar. Sci. 73 (4), 1012–1018. doi: 10.1093/icesjms/fsv255
Girón-Nava A., López-Sagástegui C., Aburto-Oropeza O. (2015). On the conditions of the 2012 cannonball jellyfish (Stomolophus meleagris) bloom in Golfo de Santa Clara: a fishery opportunity? Fisheries Manage. Ecol. 22 (3), 261–264. doi: 10.1111/fme.12115
Gittings S. R., Boland G. S., Deslarzes K. J., Combs C. L., Holland B. S., Bright T. J. (1992). Mass spawning and reproductive viability of reef corals at the East Flower Garden Bank, northwest Gulf of Mexico. Bull. Mar. Sci. 51 (3), 420–428.
Gonçalves G. R. L., Wolf M. R., Antunes M., Amorim F. W., Negreiros-Fransozo M. L., Leão Castilho A. (2022). Ontogenetic niche specialization of the spider crab Libinia ferreirae associated with the medusa Lychnorhiza lucerna. Curr. Zool. 68 (5), 549–559. doi: 10.1093/cz/zoab095
Gröndahl F. (1988a). A comparative ecological study on the scyphozoans Aurelia aurita, Cyanea capillata and C. lamarckii in the Gullmar Fjord, western Sweden 1982 to 1986. Mar. Biol. 97 (4), 541–550. doi: 10.1007/BF00391050
Gröndahl F. (1988b). Interactions between polyps of Aurelia aurita and planktonic larvae of scyphozoans: An experimental study. Mar. Ecol. Prog. Series. Oldendorf 45 (1), 87–93. doi: 10.3354/meps045087
Gueroun S. K. M., Molinero J. C., Piraino S., Dali Yahia M. N. (2020). Population dynamics and predatory impact of the alien jellyfish Aurelia solida (Cnidaria, Scyphozoa) in the Bizerte Lagoon (southwestern Mediterranean Sea). Mediterr. Mar. Sci. 21 (1), 22–35. doi: 10.12681/mms.17358
Gueroun S. K. M., Torres T. M., Dos Santos A., Vasco-Rodrigues N., Canning-Clode J., Andrade C. (2021). Catostylus tagi (Class: Scyphozoa, Order: Discomedusae, Suborder: Rhizostomida, Family: Catostylidae) life cycle and first insight into its ecology. PeerJ 9, e12056. doi: 10.7717/peerj.12056
Hamner W. M., Dawson M. N. (2009). A review and synthesis on the systematics and evolution of jellyfish blooms: Advantageous aggregations and adaptive assemblages. Hydrobiologia 616, 161–191. doi: 10.1007/s10750-008-9620-9
Hays G. C., Doyle T. K., Houghton J. D. (2018). A paradigm shift in the trophic importance of jellyfish? Trends Ecol. Evol. 33 (11), 874–884. doi: 10.1016/j.tree.2018.09.001
Heaslip S. G., Iverson S. J., Bowen W. D., James M. C. (2012). Jellyfish support high energy intake of leatherback sea turtles (Dermochelys coriacea): video evidence from animal-borne cameras. PloS One 7 (3), e33259. doi: 10.1371/journal.pone.0033259
Helm R. R. (2018). Evolution and development of scyphozoan jellyfish. Biol. Rev. 93 (2), 1228–1250. doi: 10.1111/brv.12393
Hernroth L., Gröndahl F. (1983). On the biology of Aurelia aurita (L.) 1. Release and growth of Aurelia aurita (L.) ephyra in the Gullmar Fjord, Western Sweden 1982 – 83. Ophelia 22 (2), 189–199. doi: 10.1080/00785326.1983.10426595
Holst S. (2012). “Effects of climate warming on strobilation and ephyra production of North Sea scyphozoan jellyfish,” in Jellyfish Blooms IV. Interactions with humans and fisheries. Eds. Purcell J., Mianzan H., Frost J. R. (Springer: Dordrecht), 127–140. doi: 10.1007/s10750-012-1043-y
Holst S., Jarms G. (2007). Substrate choice and settlement preferences of planula larvae of five Scyphozoa (Cnidaria) from German Bight, North Sea. Mar. Biol. 151, 863–871. doi: 10.1007/s00227-006-0530-y
Holst S., Kaiser L. R., Sötje I. (2023). Planula settlement and polyp morphogenesis in two bloom forming jellyfish species of the genus Cyanea Péron and Lesueur 1810 and effects of abiotic factors on planulocysts. Mar. Biol. 171 (1), 1–21. doi: 10.1007/s00227-023-04315-z
Holst S., Sötje I., Tiemann H., Jarms G. (2007). Life cycle of the rhizostome jellyfish Rhizostoma octopus (L.)(Scyphozoa, Rhizostomeae), with studies on cnidocysts and statoliths. Mar. Biol. 151, 1695–1710. doi: 10.1007/s00227-006-0594-8
Hsieh Y. P., Leong F. M., Rudloe J. (2001). “Jellyfish as food,” in Jellyfish Blooms: Ecological and Societal Importance. Eds. Purcell J. E., Graham W. M., Dumont H. J. (Dordrecht: Springer), 11–17.
Ishii H., Kojima S., Tanaka Y. (2004). Survivorship and production of Aurelia aurita ephyra in the innermost part of Tokyo Bay, Japan. Plankton Biol. Ecol. 51 (1), 26–35.
Jarms G., Båmstedt U., Tiemann H., Martinussen M. B., Fosså J. H., Høisœter T. (1999). The holopelagic life cycle of the deep-sea medusa Periphylla periphylla (Scyphozoa, Coronatae). Sarsia 84 (1), 55–65. doi: 10.1080/00364827.1999.10420451
Javidpour J., Molinero J. C., Lehmann A., Hansen T., Sommer U. (2009). Annual assessment of the predation of Mnemiopsis leidyi in a new invaded environment, the Kiel Fjord (Western Baltic Sea): a matter of concern? J. Plankton Res. 31 (7), 729–738. doi: 10.1093/plankt/fbp021
Jensen N., Weiland-Bräuer N., Joel S., Chibani C. M., Schmitz R. A. (2023). The life cycle of Aurelia aurita depends on the presence of a microbiome in polyps prior to onset of strobilation. Microbiol. Spectr. 11 (4), e00262–e00223. doi: 10.1128/spectrum.00262-23
Kawahara M., Uye S. I., Ohtsu K., Iizumi H. (2006). Unusual population explosion of the giant jellyfish Nemopilema nomurai (Scyphozoa: Rhizostomeae) in East Asian waters. Mar. Ecol. Prog. Ser. 307, 161–173. doi: 10.3354/meps307161
Keesing J. K., Gershwin L. A., Trew T., Strzelecki J., Bearham D., Liu D., et al. (2016). Role of winds and tides in timing of beach strandings, occurrence, and significance of swarms of the jellyfish Crambione mastigophora (Mass 1903) (Scyphozoa: Rhizostomeae: Catostylidae) in north-western Australia. Hydrobiologia 768 (1), 19–36. doi: 10.1007/s10750-015-2525-5
Kennerley A., Wood L. E., Luisetti T., Ferrini S., Lorenzoni I. (2022). Economic impacts of jellyfish blooms on coastal recreation in a UK coastal town and potential management options. Ocean Coast. Manage. 227, 106284. doi: 10.1016/j.ocecoaman.2022.106284
Khalturin K., Shinzato C., Khalturina M., Hamada M., Fujie M., Koyanagi R., et al. (2019). Medusozoan genomes inform the evolution of the jellyfish body plan. Nat. Ecol. Evol. 3 (5), 811–822. doi: 10.1038/s41559-019-0853-y
Khong N. M. H., Yusoff F. M., Jamilah B., Basri M., Maznah I., Chan K. W., et al. (2016). Nutritional composition and total collagen content of three commercially important edible jellyfish. Food Chem. 196, 953–960. doi: 10.1016/j.foodchem.2015.09.094
Kikinger R. (1992). Cotylorhiza tuberculata (Cnidaria: Scyphozoa) - life history of a stationary population. Mar. Ecol. 13 (4), 333–362. doi: 10.1111/j.1439-0485.1992.tb00359.x
Kim D. H., Seo J. N., Yoon W. D., Suh Y. S. (2012). Estimating the economic damage caused by jellyfish to fisheries in Korea. Fisheries Sci. 78, 1147–1152. doi: 10.1007/s12562-012-0533-1
Kondo Y., Ohtsuka S., Hirabayashi T., Okada S., Ogawa N. O., Ohkouchi N., et al. (2016). Seasonal changes in infection with trematode species utilizing jellyfish as hosts: evidence of transmission to definitive host fish via medusivory. Parasite 23, 16. doi: 10.1051/parasite/2016016
Kremer P., Costello J., Kremer J., Canino M. (1990). Significance of photosynthetic endosymbionts to the carbon budget of the Scyphomedusa Linuche unguiculata. Limnol. Oceanogr. 35 (3), 609–624. doi: 10.4319/lo.1990.35.3.0609
Kuplik Z., Kerem D., Angel D. L. (2015). Regulation of Cyanea capillata populations by predation on their planulae. J. Plankton Res. 37 (5), 1068–1073. doi: 10.1093/plankt/fbv064
Leone A., Lecci R. M., Milisenda G., Piraino S. (2019). Mediterranean jellyfish as novel food: Effects of thermal processing on antioxidant, phenolic, and protein contents. Eur. Food Res. Technol. 245, 1611–1627. doi: 10.1007/s00217-019-03248-6
Leoni V., Bonnet D., Ramírez-Romero E., Molinero J. C. (2021b). Biogeography and phenology of the jellyfish Rhizostoma pulmo (Cnidaria: Scyphozoa) in southern European seas. Global Ecol. Biogeogr. 30 (3), 622–639. doi: 10.1111/geb.13241
Leoni V., Molinero J. C., Crochemore S., Meffre M., Bonnet D. (2022). Ontogenetic dietary shifts of the medusa Rhizostoma pulmo (Cnidaria: Scyphozoa). Hydrobiologia 849 (13), 2933–2948. doi: 10.1007/s10750-022-04903-y
Leoni V., Molinero J. C., Meffre M., Bonnet D. (2021a). Variability of growth rates and thermohaline niches of Rhizostoma pulmo’s pelagic stages (Cnidaria: Scyphozoa). Mar. Biol. 168 (7), 1–19. doi: 10.1007/s00227-021-03914-y
Lilley M. K. S., Ferraris M., Elineau A., Berline L., Cuvilliers P., Gilletta L., et al. (2014). Culture and growth of the jellyfish Pelagia noctiluca in the laboratory. Mar. Ecol. Prog. Ser. 510, 265–273. doi: 10.3354/meps10854
López-Martínez J., Álvarez-Tello J. (2013). The jellyfish fishery in Mexico. Agric. Sci. 4 (6A), 57–61. doi: 10.4236/as.2013.46A009
López-Martínez J., Arzola-Sotelo E. A., Nevárez-Martínez M. O., Alvarez-Tello F. J., Morales-Bojórquez E. (2020). Modeling growth on the cannonball jellyfish Stomolophus meleagris based on a multi-model inference approach. Hydrobiologia 847 (6), 1399–1422. doi: 10.1007/s10750-020-04182-5
Lotan A., Ben-Hillel R., Loya Y. (1992). Life cycle of Rhopilema nomadica: a new immigrant scyphomedusan in the Mediterranean. Mar. Biol. 112, 237–242. doi: 10.1007/BF00702467
Loveridge A., Lucas C. H., Pitt K. A. (2021). Shorter, warmer winters may inhibit production of ephyrae in a population of the moon jellyfish Aurelia aurita. Hydrobiologia 848 (3), 739–749. doi: 10.1007/s10750-020-04483-9
Lucas C. H. (1996). Population dynamics of Aurelia aurita (Scyphozoa) from an isolated brackish lake, with particular reference to sexual reproduction. J. Plankton Res. 18 (6), 987–1007. doi: 10.1093/plankt/18.6.987
Lucas C. H., Graham W. M., Widmer C. (2012). Jellyfish life histories: role of polyps in forming and maintaining scyphomedusa populations. Adv. Mar. Biol. 63, 133–196. doi: 10.1016/B978-0-12-394282-1.00003-X
Lynam C. P., Gibbons M. J., Axelsen B. E., Sparks C. A. J., Coetzee J., Heywood B. G., et al. (2006). Jellyfish overtake fish in a heavily fished ecosystem. Curr. Biol. 16 (13), 492–493. doi: 10.1007/s00227-006-0429-7
Malej A., Kogovšek T., Ramšak A., Catenacci L. (2012). Blooms and population dynamics of moon jellyfish in the northern Adriatic. Cahiers Biol. Mar. 53 (3), 337–342.
Mammone M., Bosch-Belmar M., Milisenda G., Castriota L., Sinopoli M., Allegra A., et al. (2023). Reproductive cycle and gonadal output of the Lessepsian jellyfish Cassiopea andromeda in NW Sicily (Central Mediterranean Sea). PloS One 18 (2), e0281787. doi: 10.1371/journal.pone.0281787
Marambio M., Canepa A., Lòpez L., Gauci A. A., Gueroun S. K., Zampardi S., et al. (2021). Unfolding jellyfish bloom dynamics along the Mediterranean basin by transnational citizen science initiatives. Diversity 13 (6), 274. doi: 10.3390/d13060274
Marques R., Bonnet D., Carre C., Roques C., Darnaude A. M. (2020). Trophic ecology of a blooming jellyfish (Aurelia coerulea) in a Mediterranean coastal lagoon. Limnol. Oceanogr. 66 (1), 141–157. doi: 10.1002/lno.11593
Marques R., Cantou M., Soriano S., Molinero J. C., Bonnet D. (2015). Mapping distribution and habitats of Aurelia sp. polyps in Thau lagoon, north-western Mediterranean Sea (France). Mar. Biol. 162, 1441–1449. doi: 10.1007/s00227-015-2680-2
Marques R., Darnaude A. M., Schiariti A., Tremblay Y., Molinero J. C., Soriano S., et al. (2019). Dynamics and asexual reproduction of the jellyfish Aurelia coerulea benthic life stage in the Thau lagoon (northwestern Mediterranean). Mar. Biol. 166, 1–14. doi: 10.1007/s00227-019-3522-4
Martin L. E., Dawson M. N., Bell L. J., Colin P. L. (2006). Marine lake ecosystem dynamics illustrate ENSO variation in the tropical western Pacific. Biology Letters 2, 144–147. doi: 10.1098/rsbl.2005.0382
McInnes J. C., Alderman R., Lea M. A., Raymond B., Deagle B. E., Phillips R. A., et al. (2017). High occurrence of jellyfish predation by black-browed and Campbell albatross identified by DNA metabarcoding. Mol. Ecol. 26 (18), 4831–4845. doi: 10.1111/mec.14245
Milisenda G., Martinez-Quintana A., Fuentes V. L., Bosch-Belmar M., Aglieri G., Boero F., et al. (2018). Reproductive and bloom patterns of Pelagia noctiluca in the Strait of Messina, Italy. Estuar. Coast. Shelf Sci. 201, 29–39. doi: 10.1016/j.ecss.2016.01.002
Milisenda G., Rosa S., Fuentes V. L., Boero F., Guglielmo L., Purcell J. E., et al. (2014). Jellyfish as prey: frequency of predation and selective foraging of Boops boops (Vertebrata, Actinopterygii) on the mauve stinger Pelagia noctiluca (Cnidaria, Scyphozoa). PloS One 9 (4), e94600. doi: 10.1371/journal.pone.0094600
Miranda F. S., Chambel J., Almeida C., Pires D., Duarte I., Esteves L., et al. (2016). “Effect of different diets on growth and survival of the white-spotted jellyfish, Phyllorhiza punctata,” in Front. Mar. Sci. Conference Abstract: IMMR | International Meeting on Marine Research 2016 Peniche, Portuga: Frontiers In Marine Science. doi: 10.3389/conf.FMARS.2016.04.00042
Miyake H., Honda S., Nishikawa J., Yusoff F. M. (2021). Life cycle of edible jellyfish Acromitus hardenbergi Stiasny 1934 (Scyphozoa: Rhizostomeae) inhabiting a brackish-water environment. Animals 11 (7), 2138. doi: 10.3390/ani11072138
Miyake H., Terazaki M., Kakinuma Y. (2002). On the polyps of the common jellyfish Aurelia aurita in Kagoshima Bay. J. Oceanogr. 58, 451–459. doi: 10.1023/A:1021628314041
Motta G., Caffara M., Fioravanti M. L., Bottaro M., Avian M., Terlizzi A., et al. (2023). Parasitic infection in the scyphozoan Rhizostoma pulmo (Macri 1778). Sci. Rep. 13 (1), 5549. doi: 10.1038/s41598-023-31693-7
Muffett K. M., Aulgur J., Miglietta M. P. (2022). Impacts of light and food availability on early development of Cassiopea medusae. Front. Mar. Sci. 8. doi: 10.3389/fmars.2021.783876
Mullon C., Fréon P., Cury P. (2005). The dynamics of collapse in world fisheries. Fish Fisheries 6 (2), 111–120. doi: 10.1111/j.1467-2979.2005.00181.x
Nishikawa J., Ohtsuka S., Mulyadi M., Mujiono N., Lindsay D. J., Miyamoto H., et al. (2015). A new species of the commercially harvested jellyfish Crambionella (Scyphozoa) from central Java, Indonesia with remarks on the fisheries. J. Mar. Biol. Assoc. United Kingdom 95 (3), 471–481. doi: 10.1017/S002531541400157X
Nunes P. A., Loureiro M. L., Piñol L., Sastre S., Voltaire L., Canepa A. (2015). Analyzing beach recreationists’ preferences for the reduction of jellyfish blooms: Economic results from a stated-choice experiment in Catalonia, Spain. PloS One 10 (6), e0126681. doi: 10.1371/journal.pone.0126681
Omori M., Nakano E. (2001). Jellyfish fisheries in southeast Asia. Hydrobiologia 451, 19–26. doi: 10.1023/A:1011879821323
Palomares M. L. D., Pauly D. (2008). “The growth of jellyfishes,” in Jellyfish Blooms: Causes, Consequences, and Recent Advances. Developments in Hydrobiology, vol. vol 206 . Ed. Pitt K.A. and Purcell J. E. (Dordrecht: Springer), 11–21. doi: 10.1007/s10750-008-9582-y
Parsons T. R., Lalli C. M. (2002). Jellyfish population explosions: revisiting a hypothesis of possible causes. La mer 40 (3), 111–121.
Pascual M., Fuentes V., Canepa A., Atienza D., Gili J. M., Purcell J. E. (2015). Temperature effects on asexual reproduction of the scyphozoan Aurelia aurita sl: differences between exotic (Baltic and Red seas) and native (Mediterranean Sea) populations. Mar. Ecol. 36 (4), 994–1002. doi: 10.1111/maec.12196
Pastor-Prieto M., Bahamon N., Sabatés A., Canepa A., Gili J. M., Carreton M., et al. (2021). Spatial heterogeneity of Pelagia noctiluca ephyra linked to water masses in the Western Mediterranean. PloS One 16 (4), e0249756. doi: 10.1371/journal.pone.0249756
Pauly D., Christensen V., Dalsgaard J., Froese R., Torres F. Jr. (1998). Fishing down marine food webs. Science 279 (5352), 860–863. doi: 10.1126/science.279.5352.860
Pauly D., Graham W., Libralato S., Morissette L., Palomares M. L. D. (2008). Jellyfish in ecosystems, online databases, and ecosystem models. Hydrobiologia 616 (1), 67–85. doi: 10.1007/s10750-008-9583-x
Pérez-Ruzafa A., Campillo S., Fernández-Palacios J. M., Garcia-Lacunza A., Garcia-Oliva M., Ibañez H., et al. (2019). Long-term dynamic in nutrients, chlorophyll a, and water quality parameters in a coastal lagoon during a process of eutrophication for decades, a sudden break and a relatively rapid recovery. Front. Mar. Sci. 6. doi: 10.3389/fmars.2019.00026
Pérez-Ruzafa A., Gilabert J., Gutiérrez J. M., Fernández A. I., Marcos C., Sabah S. (2002). Evidence of a planktonic food web response to changes in nutrient input dynamics in the Mar Menor coastal lagoon, Spain. Hydrobiologia 475/476, 359–369. doi: 10.1023/A:1020343510060
Pitt K. A., Lucas C. H., Condon R. H., Duarte C. M., Stewart-Koster B. (2018). Claims that anthropogenic stressors facilitate jellyfish blooms have been amplified beyond the available evidence: A systematic review. Front. Mar. Sci. 5. doi: 10.3389/fmars.2018.00451
Prieto L., Astorga D., Navarro G., Ruiz J. (2010). Environmental control of phase transition and polyp survival of a massive-outbreaker jellyfish. PloS One 5 (11), e13793. doi: 10.1371/journal.pone.0013793
Purcell J. E. (2005). Climate effects on formation of jellyfish and ctenophore blooms: a review. J. Mar. Biol. Assoc. U.K. 85, 461–476. doi: 10.1017/S0025315405011409
Purcell J. E. (2012). Jellyfish and ctenophore blooms coincide with human proliferations and environmental perturbations. Annu. Rev. Mar. Sci. 4 (1), 209–235. doi: 10.1146/annurev-marine-120709-142751
Purcell J. E., Atienza D., Fuentes V., Olariaga A., Tilves U., Colahan C., et al. (2012). “Temperature effects on asexual reproduction rates of scyphozoan species from the northwest Mediterranean Sea,” in Jellyfish Blooms IV. Eds. Purcell J., Mianzan H., Frost J. R. (Dordrecht: Springer), 169–180. doi: 10.1007/s10750-012-1047-7
Purcell J. E., Baxter E. J., Fuentes V. L. (2013). “Jellyfish as products and problems of aquaculture,” in Advances in Aquaculture Hatchery Technology. Eds. Allan G., Burnell G. (Cambridge, United Kingdom: Woodhead Publishing Series in Food Science, Technology and Nutrition), 404–430. doi: 10.1533/9780857097460.2.404
Purcell J. E., Uye S. I., Lo W. T. (2007). Anthropogenic causes of jellyfish blooms and their direct consequences for humans: a review. Mar. Ecol. Prog. Ser. 350, 153–174. doi: 10.3354/meps07093
Purcell J. E., White J. R., Nemazie D. A., Wright D. A. (1999). Temperature, salinity and food effects on asexual reproduction and abundance of the scyphozoan Chrysaora quinquecirrha. Mar. Ecol. Prog. Ser. 180, 187–196. doi: 10.3354/meps180187
Ramondenc S., Ferrieux M., Collet S., Benedetti F., Guidi L., Lombard F. (2019). From egg to maturity: a closed system for complete life cycle studies of the holopelagic jellyfish Pelagia noctiluca. J. Plankton Res. 41 (3), 207–217. doi: 10.1093/plankt/fbz013
Rato L. D., Pinto C., Duarte I. M., Leandro S. M., Marques S. C. (2021). Euryhalinity and thermal tolerance of Phyllorhiza punctata (Scyphozoa) scyphostomae: life history and physiological trade-offs. Mar. Biol. 168 (11), 158. doi: 10.1007/s00227-021-03969-x
Rekstad M. E., Majaneva S., Borgersen Å. L., Aberle N. (2021). Occurrence and habitat characteristics of Aurelia sp. polyps in a high-latitude fjord. Front. Mar. Sci. 8. doi: 10.3389/fmars.2021.684634
Richardson A. J., Bakun A., Hays G. C., Gibbons M. J. (2009). The jellyfish joyride: causes, consequences and management responses to a more gelatinous future. Trends Ecol. Evol. 24 (6), 312–322. doi: 10.1016/j.tree.2009.01.010
Rosa S., Pansera M., Granata A., Guglielmo L. (2013). Interannual variability, growth, reproduction and feeding of Pelagia noctiluca (Cnidaria: Scyphozoa) in the Straits of Messina (Central Mediterranean Sea): Linkages with temperature and diet. J. Mar. Syst. 111, 97–107. doi: 10.1016/j.jmarsys.2012.10.001
Ruiz J., Prieto L., Astorga D. (2012). A model for temperature control of jellyfish (Cotylorhiza tuberculata) outbreaks: A causal analysis in a Mediterranean coastal lagoon. Ecol. Model. 233, 59–69. doi: 10.1016/j.ecolmodel.2012.03.019
Ruzicka J. J., Daly E. A., Brodeur R. D. (2016). Evidence that summer jellyfish blooms impact Pacific Northwest salmon production. Ecosphere 7 (4), 1–22. doi: 10.1002/ecs2.1324
Sal Moyano M. P., Schiariti A., Giberto D. A., Diaz Briz L., Gavio M. A., Mianzan H. W. (2012). The symbiotic relationship between Lychnorhiza lucerna (Scyphozoa, Rhizostomeae) and Libinia spinosa (Decapoda, Epialtidae) in the Río de la Plata (Argentina–Uruguay). Mar. Biol. 159, 1933–1941. doi: 10.1007/s00227-012-1980-z
Sanz-Martín M., Pitt K. A., Condon R. H., Lucas C. H., Novaes de Santana C., Duarte C. M. (2016). Flawed citation practices facilitate the unsubstantiated perception of a global trend toward increased jellyfish blooms. Global Ecol. Biogeogr. 25 (9), 1039–1049. doi: 10.1111/geb.12474
Schäfer S., Gueroun S. K., Andrade C., Canning-Clode J. (2021). Combined effects of temperature and salinity on polyps and ephyrae of Aurelia solida (Cnidaria: Scyphozoa). Diversity 13 (11), 573. doi: 10.3390/d13110573
Schiariti A., Kawahara M., Uye S., Mianzan H. W. (2008). Life cycle of the jellyfish Lychnorhiza lucerna (Scyphozoa: Rhizostomeae). Mar. Biol. 156 (1), 1–12. doi: 10.1007/s00227-008-1050-8
Schiariti A., Morandini A. C., Jarms G., von Glehn Paes R., Franke S., Mianzan H. (2014). Asexual reproduction strategies and blooming potential in Scyphozoa. Mar. Ecololy Prog. Ser. 510, 241–253. doi: 10.3354/meps10798
Schwartzloze R. A., Alheit J., Bakun A., Baumgartner T. R., Cloete R., Crawford R. J. M., et al. (1999). Worldwide large-scale fluctuations of sardine and anchovy populations. South Afr. J. Mar. Sci. 21, 289–347. doi: 10.2989/025776199784125962
Spangenberg D. B. (1965). Cultivation of the life stages of Aurelia aurita under controlled conditions. J. Exp. Zool. 159 (3), 303–318. doi: 10.1002/JEZ.1401590303
Stampar S. N., Silveira F. L. D., Morandini A. C. (2008). Food resources influencing the asexual reproductive cycle of coronate Scyphozoa. Cahiers Biol. Mar. 49 (3), 247.
Stoltenberg I., Dierking J., Müller-Navarra D. C., Javidpour J. (2021). Review of jellyfish trophic interactions in the Baltic Sea. Mar. Biol. Res. 17 (4), 311–326. doi: 10.1080/17451000.2021.1964532
Stone J. P., Steinberg D. K., Fabrizio M. C. (2019). Long-term changes in gelatinous zooplankton in Chesapeake Bay, USA: environmental controls and interspecific interactions. Estuar. Coasts 42 (2), 513–527. doi: 10.1007/s12237-018-0459-7
Sudirman S., Chen C. Y., Chen C. K., Felim J., Kuo H. P., Kong Z. L. (2023). Fermented jellyfish (Rhopilema esculentum) collagen enhances antioxidant activity and cartilage protection on surgically induced osteoarthritis in obese rats. Front. Pharmacol. 14. doi: 10.3389/fphar.2023.1117893
Sugahara T., Ueno M., Goto Y., Shiraishi R., Doi M., Akiyama K., et al. (2006). Immunostimulation effect of jellyfish collagen. Biosci. Biotechnol. Biochem. 70 (9), 2131–2137. doi: 10.1271/bbb.60076
Takao M., Okawachi H., Uye S. I. (2014). Natural predators of polyps of Aurelia aurita s.l. (Cnidaria: Scyphozoa: Semaeostomeae) and their predations rates. Plankton Benthos Res. 9 (2), 105–113. doi: 10.3800/pbr.9.105
Takao M., Uye S. I. (2018). Effects of low salinity on the physiological ecology of planulae and polyps of scyphozoans in the East Asian Marginal Seas: potential impacts of monsoon rainfall on medusa population size. Hydrobiologia 815, 165–176. doi: 10.1007/s10750-018-3558-3
Tang C., Sun S., Zhang F. (2020). Intraguild predation by polyps of three scyphozoan jellyfish: Nemopilema nomurai, Aurelia coerulea, and Rhopilema esculentum. J. Oceanol. Limnol. 38 (6), 1755–1761. doi: 10.1007/s00343-019-9079-8
Tang C., Sun S., Zhang F. (2021). Natural predators of polyps of three scyphozoans: Nemopilema nomurai, Aurelia coerulea, and Rhopilema esculentum. J. Oceanol. Limnol. 39 (2), 598–608. doi: 10.1007/s00343-020-0284-2
Thiebot J. B., Arnould J. P. Y., Gómez-Laich A., Ito K., Kato A., Mattern T., et al. (2017). Jellyfish and other gelata as food for four penguin species–insights from predator-borne videos. Front. Ecol. Environ. 15 (8), 437–441. doi: 10.1002/fee.1529
Thiebot J. B., Ito K., Raclot T., Poupart T., Kato A., Ropert-Coudert Y., et al. (2016). On the significance of Antarctic jellyfish as food for Adélie penguins, as revealed by video loggers. Mar. Biol. 163, 1–8. doi: 10.1007/s00227-016-2890-2
Titelman J., Gandon L., Goarant A., Nilsen T. (2007). Intraguild predatory interactions between the jellyfish Cyanea capillata and Aurelia aurita. Mar. Biol. 152, 745–756. doi: 10.1007/s00227-007-0721-1
Treible L. M., Condon R. H. (2019). Temperature-driven asexual reproduction and strobilation in three scyphozoan jellyfish polyps. J. Exp. Mar. Biol. Ecol. 520, 151204. doi: 10.1016/j.jembe.2019.151204
van Walraven L., Langenberg V., Dapper R., Witte J. I., Zuur A. F., Van Derveer H. (2015). Long-Term patterns in 50 years of scyphomedusae catches in the western Dutch Wadden Sea in relation to climate change and eutrophication. J. Plankton Res. 37 (1), 151–167. doi: 10.1093/plankt/fbu088
van Walraven L., van Bleijswijk J., van der Veer H. W. (2020). Here are the polyps: in situ observations of jellyfish polyps and podocysts on bivalve shells. PeerJ 8, e9260. doi: 10.7717/peerj.9260
Wang N., Li C. (2015). The effect of temperature and food supply on the growth and ontogeny of Aurelia sp. 1 ephyra. Hydrobiologia 754 (1), 157–167. doi: 10.1007/s10750-014-1981-7
Wang F., Schiariti A., Xu S., Ma Y., Sun T., Wang L., et al. (2023). Asexual reproduction strategies in the moon jellyfish Aurelia (Cnidaria: Scyphozoa). Front. Ecol. Evol. 10. doi: 10.3389/fevo.2022.1071518
Wang Y. T., Sun S. (2015). Population dynamics of Aurelia sp.1 ephyra and medusae in Jiaozhou Bay, China. Hydrobiologia 754 (1), 147–155. doi: 10.1007/s10750-014-2021-3
Wang P., Zhang F., Liu M., Sun S., Xian H. (2020). Isotopic evidence for size-based dietary shifts in the jellyfish Cyanea nozakii in the northern East China Sea. J. Plankton Res. 42 (6), 689–701. doi: 10.1093/plankt/fbaa042
Wang P., Zhang F., Sun S., Lü S. (2023). Experimental clearance rate and intraguild predation of jellyfish Cyanea nozakii. J. Oceanol. Limnol., 1–13. doi: 10.1007/s00343-023-2321-4
Wang Y. T., Zheng S., Sun S., Zhang F. (2015). Effect of temperature and food type on asexual reproduction in Aurelia sp.1 polyps. Hydrobiologia 754 (1), 169–178. doi: 10.1007/s10750-014-2020-4
Zang W., Zhang F., Chi X., Sun S. (2022). Relationship between asexual reproduction of Aurelia coerulea polyps and jellyfish blooms under the influence of temperature dynamics in winter and spring. Front. Mar. Sci. 9. doi: 10.3389/fmars.2022.888656
Zang W., Zhang F., Sun Y., Xu Z., Sun S. (2023). Benthic ecosystem determines jellyfish blooms by controlling the polyp colony development. Mar. pollut. Bull. 193, 115232. doi: 10.1016/j.marpolbul.2023.115232
Zavodnik D. (1987). Spatial aggregations of the swarming jellyfish Pelagia noctiluca (Scyphozoa). Mar. Biol. 94, 265–269. doi: 10.1007/BF00392939
Keywords: jellyfish proliferation, life cycle, modeling, predictability, Scyphozoa
Citation: Fernández-Alías A, Marcos C and Pérez-Ruzafa A (2024) The unpredictability of scyphozoan jellyfish blooms. Front. Mar. Sci. 11:1349956. doi: 10.3389/fmars.2024.1349956
Received: 05 December 2023; Accepted: 15 January 2024;
Published: 02 February 2024.
Edited by:
Angel Borja, Marine Research Division, SpainReviewed by:
Antonio Canepa, University of Burgos, SpainMar Bosch Belmar, University of Palermo, Italy
Copyright © 2024 Fernández-Alías, Marcos and Pérez-Ruzafa. This is an open-access article distributed under the terms of the Creative Commons Attribution License (CC BY). The use, distribution or reproduction in other forums is permitted, provided the original author(s) and the copyright owner(s) are credited and that the original publication in this journal is cited, in accordance with accepted academic practice. No use, distribution or reproduction is permitted which does not comply with these terms.
*Correspondence: Alfredo Fernández-Alías, alfredo.fernandez@um.es