- 1Institute of Marine Environment and Ecology, National Taiwan Ocean University, Keelung, Taiwan
- 2College of Ocean Science and Resource, National Taiwan Ocean University, Keelung, Taiwan
- 3General Education Center, National Taiwan Ocean University, Keelung, Taiwan
- 4Center of Excellence for the Oceans, National Taiwan Ocean University, Keelung, Taiwan
- 5Taiwan Ocean Genome Center, National Taiwan Ocean University, Keelung, Taiwan
Ciliates are an important ecological component in the microbial food web, but few studies have been conducted on the spatial distribution of small-sized planktonic ciliate communities in the East China Sea (ECS). Investigating ciliate communities using conventional morphological approaches is particularly difficult for the small, fragile, and naked species. Therefore, we applied DNA metabarcoding analysis to explore the spatial pattern of small-sized planktonic ciliate community structure within the surface, deep chlorophyll maximum (DCM), and bottom layers. Results showed the cosmopolitan species, Leegaardiella sp., was dominant and widespread in the ECS. The relative abundance of the mixotrophic family Tontonnidae decreased in the deeper layer. We characterized water masses of the ECS using environmental variables. In nano-sized ciliate communities, non-metric multidimensional scaling (NMDS) plots revealed a correlation with temperature, salinity, density, and depth. The circulation patterns were similar to cluster analysis results, suggesting that hydrographic conditions shaped small-sized ciliate community composition.
Introduction
Ciliates are highly diverse unicellular eukaryotes found in various aquatic environments. Planktonic ciliates, dominated by oligotrich, choreotrich, and tintinnid ciliates (Alveolata, Ciliophora, Spirotrichea), vary in size from tens to hundreds of micrometers (Lynn, 2008). In pelagic ecosystems, they are crucial components in microbial food webs, transferring energy to higher trophic levels while grazing on smaller plankton, such as pico- and nanoplankton (Azam et al., 1983; Pierce and Turner, 1992).
The East China Sea (ECS) is located in the Northwest Pacific Ocean, one of the world’s largest and most productive marginal seas. It is a system with complex hydrological dynamics resulting from the interaction of various water masses (Ichikawa and Beardsley, 2002; Lee and Chao, 2003; Yang et al., 2011; Zhu et al., 2022). In short, Yellow Sea Current flows from the north to the south, Changjiang diluted water (CDW) is along the coastal area, Kuroshio intrusion passes through the east of Taiwan from the south to the north, and Taiwan Current Warm Water (TCWW) flows from the south to the north (Isobe, 2008; Chen et al., 2009; Liu et al., 2021a, b; Kang and Na, 2022).
The Changjiang River generally discharges the highest in July or August, carrying large amounts of terrestrial nutrient inputs into the aquatic environments (Wu et al., 2019). A positive relationship between ciliate density and increased bacteria availability in the plume area has been reported (Chiang et al., 2003). Ciliate communities also transfer organic carbon to higher trophic levels by consuming phytoplankton and smaller plankton in the ECS (Suzuki and Miyabe, 2007; Choi et al., 2012).
Until now, more detailed surveys of tintinnid communities were studied than aloricate, oligotrich, and choreotrich ciliate communities, as their loricae were more accessible to be identified and stored by traditional taxonomic methods (Bachy et al., 2014; Dolan et al., 2009; Santoferrara and Alder, 2009; Dolan and Pierce, 2013; Dolan et al., 2013, 2016; Li et al., 2018). Moreover, aloricate ciliates are more abundant, especially in small sizes in the ECS, which increases the difficulties in traditional research under the microscope (Pitta and Giannakourou, 2000; Zheng et al., 2015; Yang et al., 2020). These small-sized ciliates represent an important grazer for picoplankton (Kim et al., 2021; Romano et al., 2021). An alternative way to survey the biodiversity and spatial distribution of ciliate communities is the metabarcoding approach, which can reveal more taxa than microscopic observations, particularly in small, fragile, and aloricate species (Bachy et al., 2013; Santoferrara et al., 2013; Gimmler et al., 2016; Santoferrara et al., 2018; Ganser et al., 2021). In this study, we characterized the species composition and distribution of small-sized planktonic ciliate communities using metabarcoding on vertical and horizontal scales. We also revealed the relationships between planktonic ciliates and environmental factors in this complex hydrological environment.
Materials and methods
Sample collection
Seawater samples were collected using Go-Flo bottles mounted on a conductivity, temperature, and depth (CTD) rosette (Sea-Bird 91 Electronics, Bellevue, WA, USA). A total of 30 stations with different depths were sampled in the ECS during a summer cruise in July 2019 (Figure 1). Hydrographic data were measured using a CTD profiler. The nutrient variables, including dissolved inorganic nitrate, phosphate, silicate, and chlorophyll a (Chl a) concentration, were measured according to the standard methods developed by Gong et al. (2003). The precision for the determination of nitrate, phosphate, silicate, and Chl a were ± 0.3 µM, ± 0.5 µM, ± 0.01 µM, and ±0.02 mg/m3, respectively.
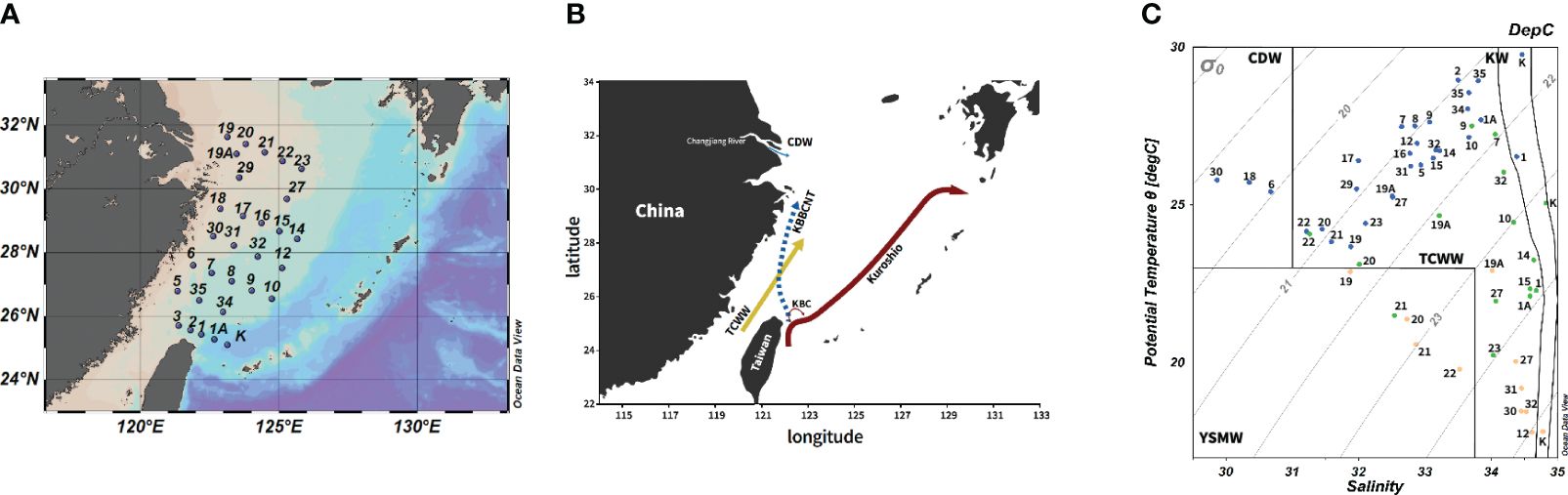
Figure 1 (A) Sampling sites from this cruise. (B) The schematic map of circulation in the ECS adapted from Yang et al. (2011). (C) The T-S diagram of ciliate samples from this cruise and water masses were defined by Gong et al. (1996). The blue, green, and orange circles represent water samples at the surface, DCM, and bottom layer, respectively. CDW, Changjiang diluted water; TCWW, Taiwan Current Warm Water; YSMW, Yellow Sea Mixed Water; KW, Kuroshio water. The schematic map shows the shelf current system of the ECS in summer. KBBCNT, Kuroshio Bottom Branch Current to the northeast of Taiwan; KBC, Kuroshio Branch Current; CDW, Changjiang diluted water; TCWW, Taiwan Current Warm Water; ECS, East China Sea; T-S, temperature–salinity; DCM, deep chlorophyll maximum.
For DNA samples, 10 L of three-layered seawater (surface, deep chlorophyll maximum (DCM), and bottom) were pre-filtered through a 200-μm nylon mesh, followed by filtering through a 20-μm nylon filter (90 mm diameter, 100 Millipore, Billerica, MA, USA), and then subsequently filtered through 3-μm and 0.2-μm pore size polycarbonate membranes (142 mm diameter, Millipore, USA) using a peristaltic pump. The filters were immediately preserved in liquid nitrogen and stored at −80°C until DNA extraction.
DNA extraction, amplification, and sequencing
DNA was extracted using the DNeasy PowerWater Kit (Qiagen, Valencia, CA, USA). The V4 region of rDNA was amplified using TAReuk454FWD1 (5′-CCA GCA SCY GCG GTA ATT CC-3′) as the forward primer and the modified TAReukREV3 (5′-ACT TTC GTT CTT GAT YRA-3′) as the reverse primer (Stoeck et al., 2010). PCR conditions were as described in Lin et al. (2022). Amplicons were sequenced on an Illumina MiSeq platform, generating 300-bp paired-end reads. Data have been deposited in the National Center for Biotechnology Information (NCBI) Sequence Read Archive (SRA) database under the project number PRJNA738614.
Sequence processing
The sequence primers were removed using cutadapt (Martin, 2011) and then underwent quality filtering and denoising using the standard pipeline of the DADA2 package (Callahan et al., 2016) under R software. Reads were filtered with the following parameters: truncLen and minLen = c (240, 180), truncQ = 2, and maxEE = c (2, 2). Taxonomic assignments of amplicon sequence variants (ASVs) were conducted using the Protist Ribosomal Reference Database (PR2) version 4.12.0 (Guillou et al., 2013). Planktonic ciliate amplicons were selected corresponding to the orders Tintinnida, Choreotrichida, and Strombidiida, among the division Ciliophora in PR2. Ciliate communities were subsampled with 250 reads for 100 times.
Data analysis and visualization
All statistical analyses were conducted using software R (Team, 2013). Alpha diversity indices (Shannon index H′ and richness) and the Bray–Curtis dissimilarities were calculated using the Vegan package (Oksanen et al., 2019). The non-metric multidimensional scaling (NMDS) plot was performed to visualize the patterns and identify potential environmental drivers for the ciliate community composition based on the log-transformed ASV abundances and scaled environmental variables. Venn diagram was made using the ggVennDiagram package (Gao et al., 2021). Ciliate communities were grouped by hierarchical cluster analysis using Ward’s minimum variance method and square-rooting Bray–Curtis distances with cluster package (Maechler et al., 2022). To understand the relationship between the environment and each cluster, differences in environmental variables among clusters were examined using Tukey’s honestly significant difference (HSD) post-hoc test, followed by an analysis of variance (ANOVA). Graphics were created using the ggplot2 package (Wickham, 2016). The plots of sampling stations, temperature–salinity (T-S) diagram, and vertical profiles of environment variables were generated in the Ocean Data View (Schlitzer, 2022). Small-sized planktonic ciliates (<20 µm) indicated sequences obtained from the nano-sized fraction. The pico-sized reads were counted for the presence of ASVs to reveal the distribution.
Results
Hydrographic conditions
During this cruise, seawater temperature and salinity ranged from 17.8°C to 29.7°C and 29.8 to 34.8, respectively. Four water masses observed in the study area followed the definition by Gong et al. (1996): TCWW, Yellow Sea Mixed Water (YSMW), CDW, and Kuroshio water (KW) (Figure 1). The YSMW influenced the bottom of the northern transect in the ECS, while the coastal surface area was influenced by the low-salinity CDW (S < 31; Supplementary Figure S1B). The KW intruded in northeastern Taiwan, involving a topographic upwelling at St. 1 (Supplementary Figure S1). The TCWW dominated the shelf area of most of the sampling sites (Figure 1C).
Overall diversity across three size fractions
We obtained a total of 15,980 ASVs represented by ~11,465,721 DNA reads. Alveolata contributed largely to total reads, particularly in nano-sized fraction (73.9%, data not shown). A total of 314 ASVs (21,336 reads) remained as planktonic ciliates across three size fractions after subsampling (19, 36, and 29 samples from micro-sized, nano-sized, and pico-sized fractions, respectively). A complete list of ciliate taxa from this dataset is reported in Supplementary Table 1. Overall, nano- and pico-sized fractions share more similarities than micro-sized where the Bray–Curtis distances were closer in the NMDS plot (Supplementary Figure S2D). The alpha diversity and species richness were lower in the micro-sized fraction when compared to both the nano- and pico-sized fractions (Supplementary Figure S2A). Tintinnida accounted for more than 50% of the ciliate relative abundance in the micro-sized fraction, in which Salpingella sp., Amphorellopsis acuta, and Stenosemella pacifica were found to be the major contributors (Supplementary Figure S3). Strombidiida dominated in two small-sized fractions, which were Strombidiida_G_XX_sp., Strombidiidae_H_X_sp., and Strombidiidae_G_X_sp. in the nano-sized fraction, and Strombidiida_G_XX_sp., Spirotontonia_sp., and Tontonnidae_B_X_sp. in pico-sized fractions (43.97% and 52.93%, respectively; Supplementary Figures S2B, S3). Leegaardiella sp. was the most abundant taxon across three size fractions in the ECS.
Nano-sized ciliate communities and relationship with environmental variables
Hierarchical cluster analysis based on the Bray–Curtis dissimilarities was performed on nano-sized ciliate communities. These clusters were subsequently organized into two groups, defined by a distance threshold of 1.7, resulting in one group containing three clusters and another with two clusters (Figure 2). In nano-sized samples, both cluster analysis and NMDS revealed a strong correlation with depth (Figures 2, 3). The first group, which included Clusters I, II, and IV, was influenced by the deeper water column. Cluster IV, which comprised St. 20 and St. 21 across all depths in the water mass of TCWW and YSMW, was dominated by Choreotrichida. The taxonomic compositions in Cluster I were mostly from deeper water samples in TCWW, with only one exception in YSMW. Although Clusters II and IV shared similar taxonomic compositions according to the analysis of Bray–Curtis dissimilarity, the former was mainly influenced by TCWW and KW (Figure 2), whereas the latter was mainly influenced by YSMW. The second group containing Clusters III and V was primarily composed of samples collected from shallow depths, particularly the surface layer. These shallow water samples were mostly from TCWW and positively correlated to temperature but significantly different in salinity (Supplementary Figure S4; Table 1). Clusters III and V, both from shallow water, were higher in temperature and oxygen concentration. The biological parameters indicated the potential prey (Synechococcus, photosynthetic picoeukaryotes, and bacteria), and only Synechococcus was higher in Cluster III (Supplementary Figure S4).
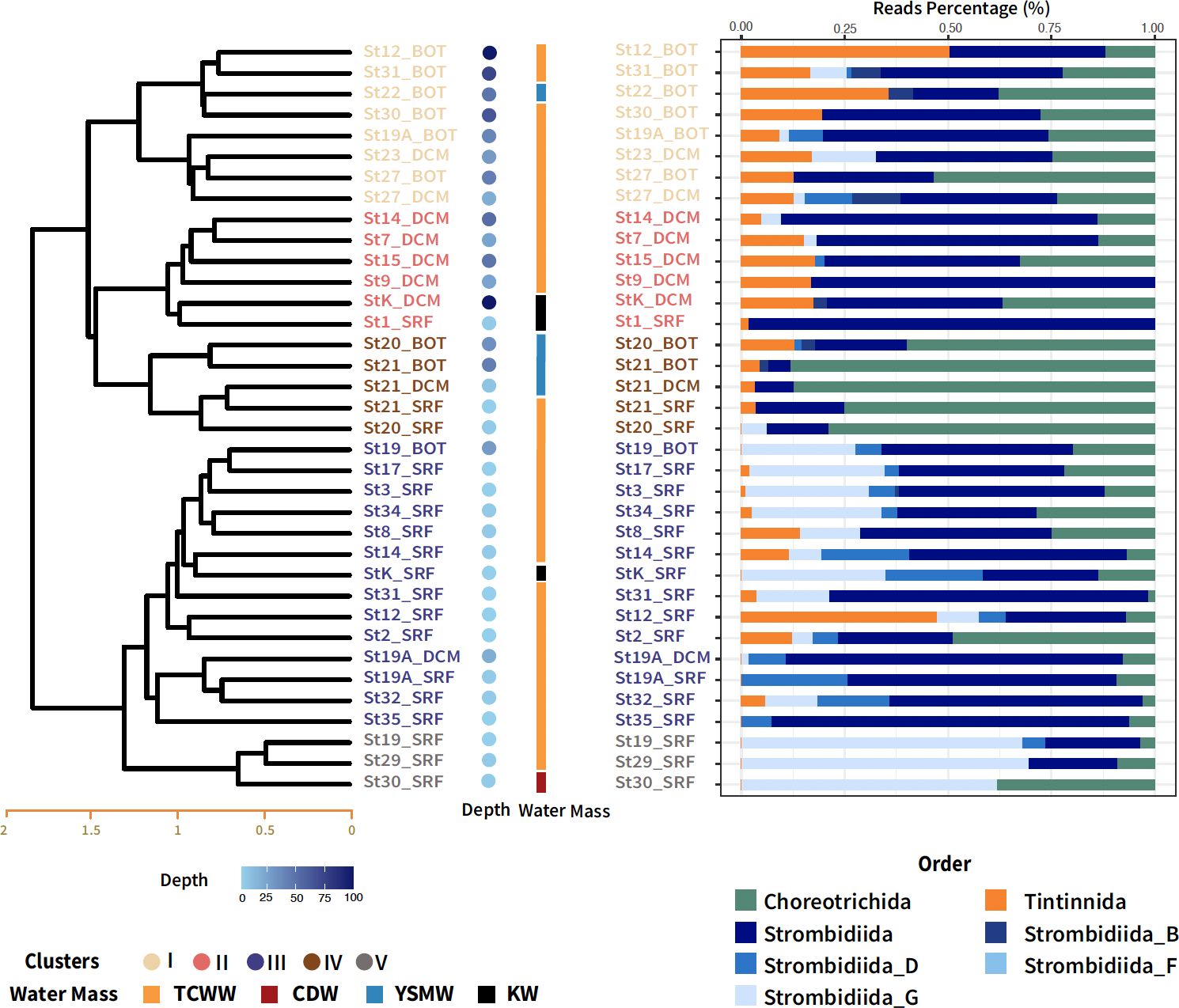
Figure 2 Cluster analysis based on Bray–Curtis dissimilarity distances among nano-sized ciliate communities in different depth layers. The right bar represents the water mass of each sample, where orange indicates the Taiwan Current Warm Water (TCWW), red indicates the Changjiang diluted water (CDW), black indicates the Kuroshio water (KW), and blue indicates the Yellow Sea Mixed Water (YSMW). The right panel is the relative abundance of taxonomic groups by classification order in the PR2 database.
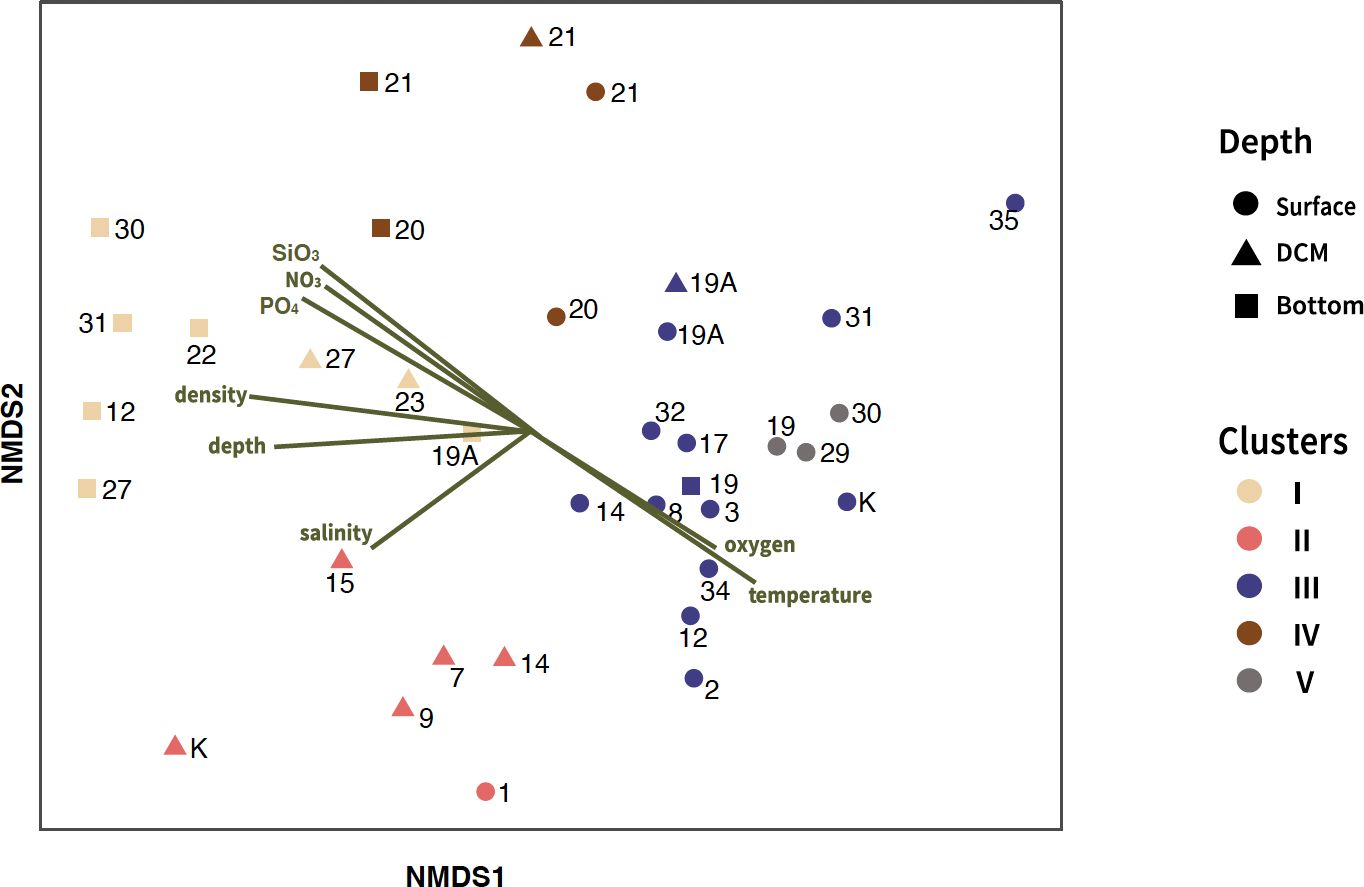
Figure 3 Non-metric multidimensional scaling (NMDS) plot using Bray–Curtis dissimilarity distances of nano-sized ciliate communities, overlaid with environmental vector fitting (p < 0.05). The plot indicates five different clusters of ciliate communities, each represented by a different color. The stress value for the plot was 0.17.
Distribution of small-sized ciliates
The top four taxa in nano-sized fraction, Leegaardiella sp., Strombidiida_G_XX_sp., Strombidiidae_G_X_sp., and Strombidiidae_H_X_sp., were widespread and across all three layers (Figures 4, 5). Lynnella semiglobulosa, Pelagostrobilidium minutum, Rimostrombidium venilia, Strombidium capitatum, Strombidium caudispina, and Strombidium triquetrum were only found in the surface layer (data not shown).
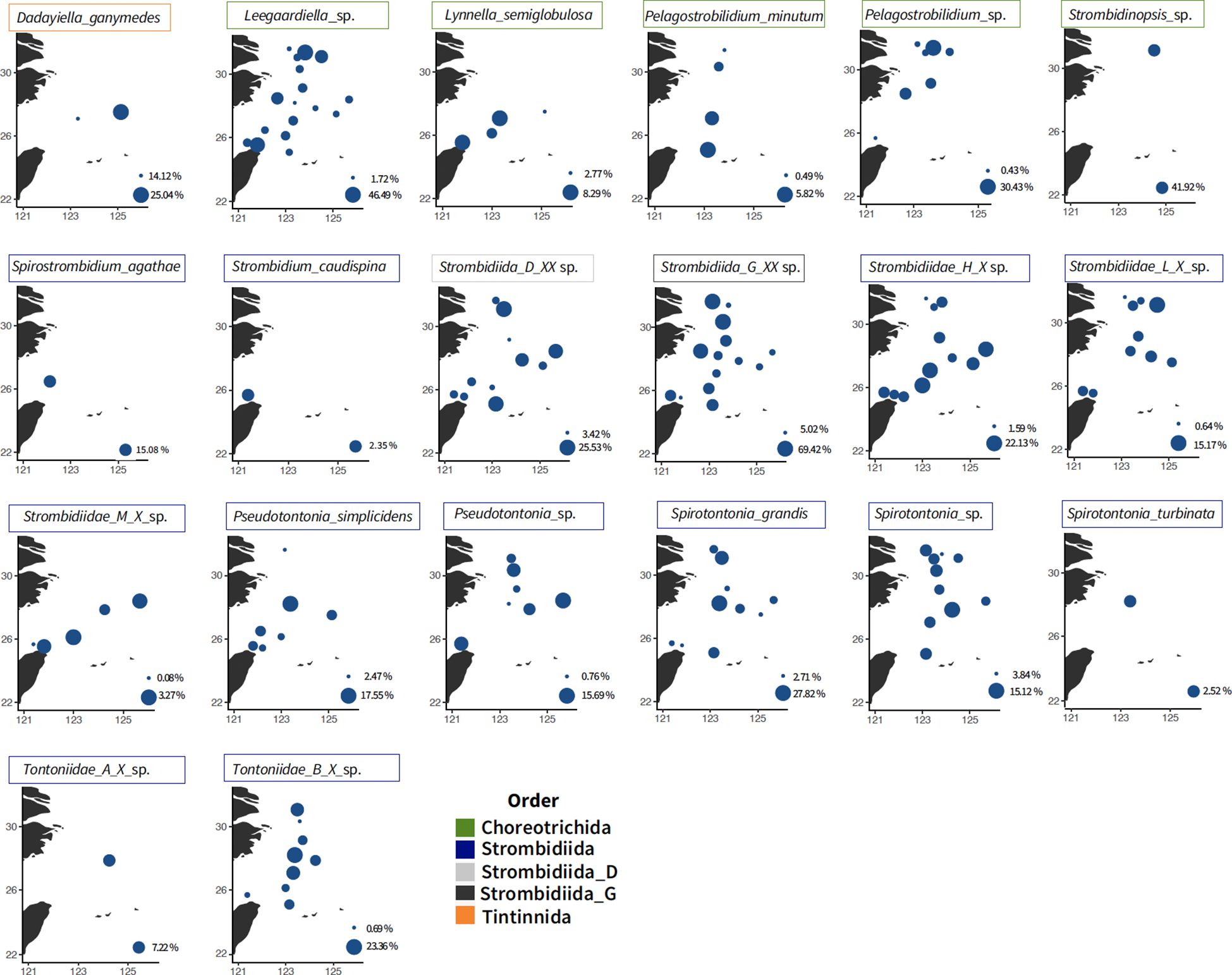
Figure 4 Distribution of the major taxa from nano-sized fraction at the surface. The colors represent the classification order. Bubble size corresponds to the percentage of reads relative to the total ciliate abundance in each station (absences are not shown), scaled from the minimum to the maximum in each plot.
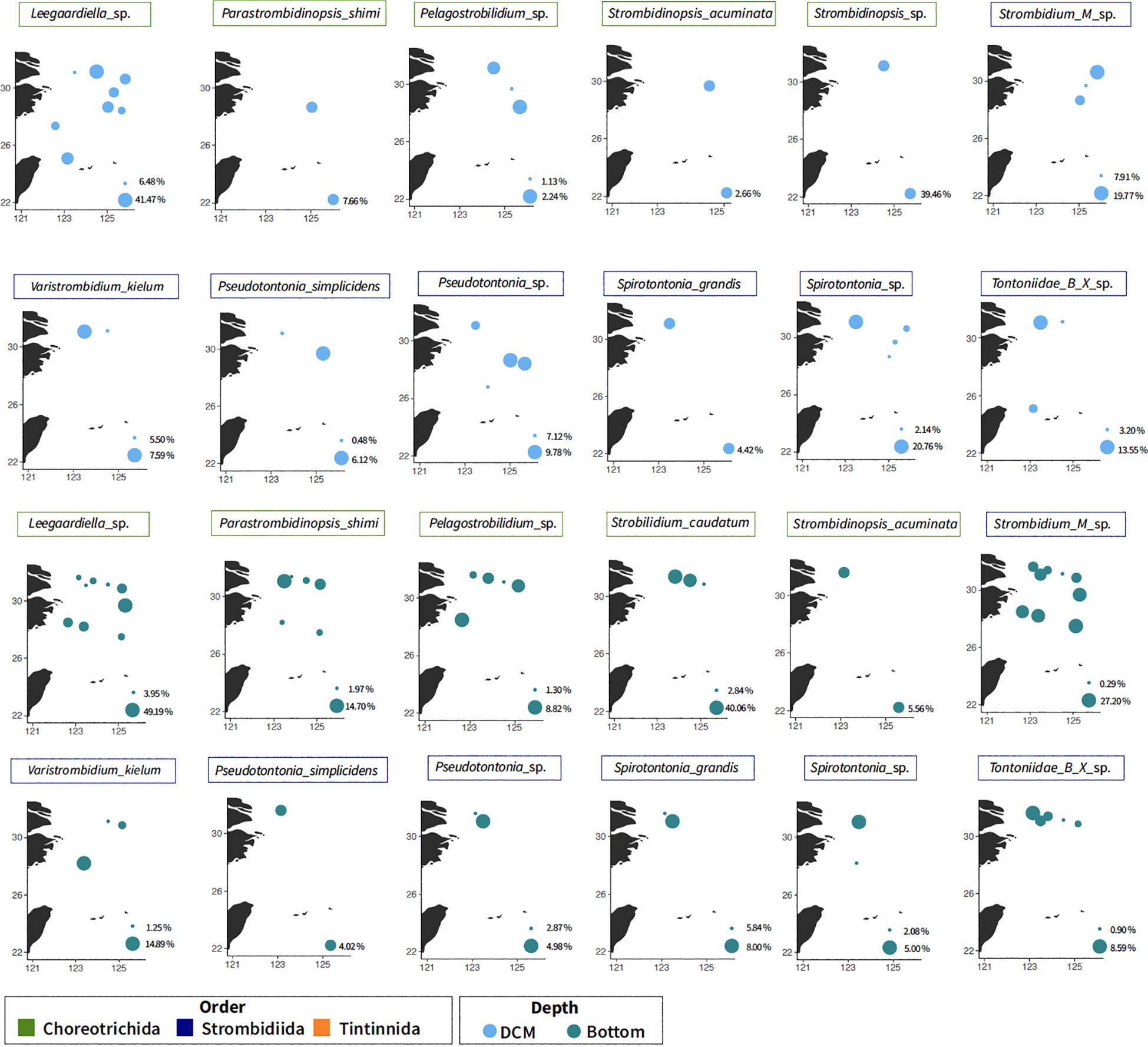
Figure 5 Distribution of the major taxa from nano-sized fraction at the DCM and bottom layer. The colors represent the classification order. Bubble size corresponds to the percentage of reads relative to the total ciliate abundance in each station (absences are not shown), scaled from the minimum to the maximum in each plot. DCM, deep chlorophyll maximum.
Multiple vertical distribution patterns were observed based on different annotated species. The presence of the mixotrophic family Tontonnidae, Pseudotontonia simplicidens, Pseudotontonia_sp., Spirotontonia grandis, Spirotontonia_sp., Spirotontonia turbinata, Tontonnidae_A_X_sp., and Tontonnidae_B_X_sp., decreased in the deeper layer. There was an opposite pattern with the Tontonnidae, with Tintinnidae_X_sp. and Parastrombidinopsis shimi in low abundance/absent in the surface layer (Figure 5). Strobilidium caudatum and Strombidium_M_sp. were found across three layers but on the north transect (Figure 4).
Discussion
Metabarcoding
Research on microbial community composition and diversity using metabarcoding has been more prevalent globally but relatively scarce in the ECS (Bik et al., 2012; De Vargas et al., 2015; Gimmler et al., 2016; Malviya et al., 2016; Noan et al., 2016; Cordier et al., 2022). Metabarcoding has the potential to reveal large-scale patterns in plankton diversity and community structure that were largely invisible in morphological analyses due to large numbers of undescribed species and the difficulty in characterizing the species present in the plankton community (Massana et al., 2006; Ichinomiya et al., 2016; Morard et al., 2018; Tragin and Vaulot, 2019). Studies focusing on aloricate ciliate communities often face challenges in the resolution of morphological characteristics due to differences in fixation methodologies, which results in fewer studies based on morphology, as staining requires specific expertise (Wang et al., 2013; Sun et al., 2019; Huang et al., 2021; Kim et al., 2021). Identification of tintinnids could be easier and more complete for tintinnid communities by their external loricae compared to aloricate ones (Dolan et al., 2012; Dolan and Pierce, 2013; Zhang et al., 2017; Romano et al., 2021; Wang et al., 2021b; Li et al., 2023; Mieczan et al., 2023) However, ciliate community investigations can be more accessible without elaborate staining procedures and subjective observation under microscopy once the standardized pipeline of molecular sequencing and data filtering was followed (Bachy et al., 2013; Santoferrara et al., 2020; Burki et al., 2021). Metabarcoding provides rigor standards comparing dissimilarities among plenty of communities. Small-sized aloricate ciliates (<20 µm) were up to 50% in planktonic ciliate communities in many regions, including the ECS by morphological data (Yang et al., 2020; Wang et al., 2023a, b). Thus, this study can improve the understanding of planktonic ciliate community structure, particularly for small and naked species.
Ciliate sequences in pico-sized fraction
To date, many surveys by metabarcoding have shown ciliate sequences in the pico-sized fraction (Romari and Vaulot, 2004; Wu et al., 2017; Xu et al., 2017; Grattepanche et al., 2022; Flegontova et al., 2023). Canals et al. (2020) indicated that these sequences provided useful information about ciliate communities where over 94% of Ciliophora abundance from the Tara Ocean expedition in each size fraction corresponded to operational taxonomic units (OTUs) detected in the three fractions. There are hypotheses to explain these sequences in the pico-sized fraction: a) cell breakage during sequential filtration or b) membrane flexibility of ciliates to pass the small pores on filters (Cheung et al., 2008). It is possible for ciliates with a minute oral diameter (i.e., 2–5 µm) to pass through 3 µm, but molecular data are lacking (Yang et al., 2020). Comparing reads in nano- and pico-sized fraction from the same sampling sites, only Strombidiida_G_XX_sp. differed significantly in read abundance (Supplementary Figure S5, p < 0.05). Our results supported ciliate sequences in the pico-sized fraction from the cell breakages during filtration.
Overall diversity across three size fractions
Leegaardiella sp., a cosmopolitan species reported by Agatha (2011), was dominant across three size fractions in this cruise (Supplementary Figure S4). Additionally, with morphological data, Leegaardiella ovalis was identified in the same region (Yang et al., 2020). However, more sequences from the database are needed to establish a connection between the two species. In the micro-sized fraction, tintinnid contributed to half of the relative abundance, which decreased as size decreased, which was similar to previous studies in both coastal and pelagic samples (Supplementary Figure S2B; Grattepanche et al., 2016b; Canals et al., 2020). Tintinnid loricae are larger (50–400 μm in length) than aloricate oligotrich and choreotrich ciliates, facilitating the retention of cells on 20-μm filters. Most tintinnid ciliates were reported with morphological records except S. pacifica, Tintinnopsis platensis, and Ascampbelliella acuta (Xu et al., 2013; Li et al., 2016; Feng et al., 2018; Li et al., 2018; Sun et al., 2019; Yang et al., 2021; Wang et al., 2021b; Yu et al., 2022; Li et al., 2023). In both nano-sized and pico-sized fractions, dominant taxa were similar but different in relative abundance (Supplementary Figure S3). P. minutum was absent in the micro-sized fraction, which corresponded to its morphological characteristics (Chen et al., 2017).
Horizontal and vertical distribution of small-sized ciliates
We focused more on ASVs, which were annotated with aloricate morphospecies (Figure 3). Dadayiella ganymedes was found in offshore stations in the ECS and recorded on a large scale in the western Pacific region (Li et al., 2021; Feng et al., 2022; Li et al., 2023). Strombidinopsis acuminata, S. caudatum, P. shimi, Strombidium_M_sp., and Varistrombidium kielum were found only on the north transect across different layers, which might be related to the Changjiang River discharge-induced aloricate ciliate abundance in the summer (Chiang et al., 2003; Tsai et al., 2011; Yu et al., 2016). The mixotrophic family Tontonnidae was found vertically and horizontally in the ECS, whereas several species were frequently recorded in adjacent areas (Yang et al., 2020, 2021). However, a common species, Loboea strobila, was not detected during this cruise (Sun et al., 2019; Huang et al., 2021).
Hydrographic conditions shape small-sized ciliate community composition
The hydrology of the ECS was intricate and influenced by factors such as the Changjiang River outflow, Taiwan Strait, and Kuroshio. In general, the clustering pattern in nano-sized communities was similar to the current circulation patterns in summer (Figure 1B; Yuan et al., 2008). Kuroshio Bottom Branch Current to the northeast of Taiwan (KBBCNT), which is bifurcated from Kuroshio subsurface water (KSSW), formed year-round upwelling off northeast Taiwan with its collision on the shelf (Liu et al., 1992; Wu et al., 2008; Kao et al., 2023). We observed upwelling in St. 1 and St. 1A by decreasing temperature (Supplementary Figure S1A; Chen et al., 2015). Then, KBBCNT flowed northwestward along the 60-m isobath, carrying high‐salinity and nutrient water and mixing with TCWW, toward the Changjiang River mouth (Yang et al., 2011). This circulation influenced Clusters I and II, in which Tintinnida and Strombidiida were dominant in both clusters (Figure 2). In addition, the TCWW flowed northward in the upper layer mixed with Kuroshio surface water (KSW) and CDW (Ichikawa and Beardsley, 2002). It might reflect the distribution as Cluster III.
The Changjiang River discharge carried a large amount of terrestrial nutrients in summer, which moved northeastward, subsequently mixing with saline ambient waters (Chen et al., 2009). However, only St. 30 in Cluster V was classified under CDW (Figure 1C). Considering the location of St. 19 and St. 29 and the highest Chl a concentration measured at the surface in St. 19 (3.39 mg/m3), which were possibly influenced by CDW and TCWW, resulting in the lowest salinity among all clusters (Supplementary Figure S4). The highest average Chl a value observed in Cluster V. Strombidiida_G_XX_sp. was dominant in Cluster V, indicating potential predation on phytoplankton (66%, Supplementary Table S3). Moreover, the two mixotrophic genera, Spirotontonia and Pseudotontonia, up to 12% in this cluster suggested the bottom-up environment. Cluster IV, which was from St. 20 and St. 21, was influenced by YSMW. Strombidinopsis sp. and S. caudatum were distinct and abundant species that implied different niches of YSMW, as in lower temperature and salinity (25% and 14%, Supplementary Table S3). However, different ASVs contributed to different clusters, which indicated the possibility of cryptic species of different niches (data not shown). The strong correlation between the ordination and depth (R2 = 0.68) and particularly density (R2 = 0.76), coupled with significant differences in temperature and salinity between clusters, implied that cluster dissimilarity was influenced by the currents (Table 1).
The definitions of the water masses are not consistent in the ECS (Gong et al., 2000; Ichikawa and Beardsley, 2002; Yang et al., 2011; Quan et al., 2013; Zhou et al., 2018). Surface water in the ECS is mainly mixed by the CDW, KSW, and TCWW, while the deeper layer is influenced by KSSW, Shelf Mixing Water (SMW), and TCWW by the Optimum Multiparameter analysis (Zhou et al., 2018). Ciliate community composition and diversity reported differ markedly with depth (Countway et al., 2010; Grattepanche et al., 2016a, b; Santoferrara et al., 2023; Tucker et al., 2017; Zhao et al., 2017; Wang et al., 2021a), water masses (Sun et al., 2020; Yang et al., 2020; Gu et al., 2021), and geographical position (inshore/offshore) (Grattepanche et al., 2015; Santoferrara et al., 2016; Grattepanche et al., 2016b). While Yang et al. (2020) indicated that planktonic ciliates were potentially reliable indicators of water masses in the ECS, this was not universally consistent, possibly due to methodological differences in definitions of water masses or ciliate analysis approaches (Zhang et al., 2015). The NMDS plot revealed a correlation with multiple environmental variables, particularly density (Figure 3, Table 1). Additionally, the distribution of clusters was similar to current circulation patterns, suggesting that currents influence community composition. Here, we presented evidence that the composition of nano-sized ciliate communities varied with the circulation of currents.
In summary, detailed planktonic ciliate community composition was reported by metabarcoding in the ECS. The specified vertical and horizontal distributions of major small-sized taxa were first revealed. We supported hydrographic conditions shaping community structure by the similarities of cluster distribution. Building a more comprehensive database of reference sequences in future studies can provide more information for ciliate communities.
Data availability statement
The datasets presented in this study can be found in online repositories. The names of the repository/repositories and accession number(s) can be found in the article/Supplementary Material.
Author contributions
W-TC: Writing – original draft, Writing – review & editing. Y-CL: Data curation, Formal analysis, Writing – review & editing. S-FT: Writing – review & editing. K-PC: Funding acquisition, Project administration, Supervision, Writing – review & editing.
Funding
The author(s) declare financial support was received for the research, authorship, and/or publication of this article. This research was supported by the Ministry of Science and Technology, Taiwan (110–2611-M-019–017 and 111–2611-M-019–014).
Acknowledgments
We thank the captain and crew of the R/V Ocean Researcher I for the sampling, G-CG lab for providing the environmental parameter data, Ching-Ting Huang for collecting the field samples, Yu-Ting Tseng for organizing the environmental parameter data, and Xin-Bei Chen for organizing the amplicon data.
Conflict of interest
The authors declare that the research was conducted in the absence of any commercial or financial relationships that could be construed as a potential conflict of interest.
Publisher’s note
All claims expressed in this article are solely those of the authors and do not necessarily represent those of their affiliated organizations, or those of the publisher, the editors and the reviewers. Any product that may be evaluated in this article, or claim that may be made by its manufacturer, is not guaranteed or endorsed by the publisher.
Supplementary material
The Supplementary Material for this article can be found online at: https://www.frontiersin.org/articles/10.3389/fmars.2024.1349707/full#supplementary-material
Supplementary Table 1 | List of the number of annotated ciliate species, the total number of reads, and the presence (+) or absence (-) across three size fractions.
Supplementary Table 2 | Nano-sized planktonic ciliate ASV table in each sample.
Supplementary Table 3 | Percentage of top ten nano-sized ciliate taxa in each cluster.
References
Agatha S. (2011). Global diversity of aloricate oligotrichea (Protista, ciliophora, spirotricha) in marine and brackish sea water. PloS One 6, e22466. doi: 10.1371/journal.pone.0022466
Azam F., Fenchel T., Field J. G., Gray J. S., Meyer-Reil L. A., Thingstad F. (1983). The ecological role of water-column microbes in the sea. Mar. Ecol. Prog. Ser. 10, 257–263. doi: 10.3354/meps010257
Bachy C., Dolan J. R., Lopez-Garcia P., Deschamps P., Moreira D. (2013). Accuracy of protist diversity assessments: morphology compared with cloning and direct pyrosequencing of 18S rRNA genes and ITS regions using the conspicuous tintinnid ciliates as a case study. ISME. J. 7, 244–255. doi: 10.1038/ismej.2012.106
Bachy C., Moreira D., Dolan J. R., López-García P. (2014). Seasonal dynamics of free-living tintinnid ciliate communities revealed by environmental sequences from the North-West Mediterranean Sea. FEMS Microbiol. Ecol. 87, 330–342. doi: 10.1111/1574-6941.12224
Bik H. M., Porazinska D. L., Creer S., Caporaso J. G., Knight R., Thomas W. K. (2012). Sequencing our way towards understanding global eukaryotic biodiversity. Trends Ecol. Evol. 27, 233–243. doi: 10.1016/j.tree.2011.11.010
Burki F., Sandin M. M., Jamy M. (2021). Diversity and ecology of protists revealed by metabarcoding. Curr. Biol. 31, R1267–R1280. doi: 10.1016/j.cub.2021.07.066
Callahan B. J., Mcmurdie P. J., Rosen M. J., Han A. W., Johnson A. J. A., Holmes S. P. (2016). DADA2: High-resolution sample inference from Illumina amplicon data. Nat. Methods 13, 581. doi: 10.1038/nmeth.3869
Canals O., Obiol A., Muhovic I., Vaqué D., Massana R. (2020). Ciliate diversity and distribution across horizontal and vertical scales in the open ocean. Mol. Ecol. 29, 2824–2839. doi: 10.1111/mec.15528
Chen P.-C., Chiang K.-P., Tsai S.-F. (2017). Pelagostrobilidium liui n. sp. (Ciliophora, Choreotrichida) from the Coastal Waters of Northeastern Taiwan and an Improved Description of Pelagostrobilidium minutum Liu et al. J. Eukaryotic. Microbiol. 64, 579–587. doi: 10.1111/jeu.12392
Chen C.-C., Hsu S.-C., Jan S., Gong G.-C. (2015). Episodic events imposed on the seasonal nutrient dynamics of an upwelling system off northeastern Taiwan. J. Mar. Syst. 141, 128–135. doi: 10.1016/j.jmarsys.2014.07.021
Chen C.-C., Shiah F.-K., Chiang K.-P., Gong G.-C., Kemp W. M. (2009). Effects of the Changjiang (Yangtze) River discharge on planktonic community respiration in the East China Sea. J. Geophys. Res.: Oceans. 114, C03005. doi: 10.1029/2008JC004891
Cheung M. K., Chu K. H., Li C. P., Kwan H. S., Wong C. K. (2008). Genetic diversity of picoeukaryotes in a semienclosed harbour in the subtropical western Pacific Ocean. Aquat. Microbial. Ecol. 53, 295–305. doi: 10.3354/ame01247
Chiang K. P., Lin C. Y., Lee C. H., Shiah F. K., Chang J. (2003). The coupling of oligotrich ciliate populations and hydrography in the East China Sea: spatial and temporal variations. Deep. Sea. Res. Part II.: Topical. Stud. Oceanography. 50, 1279–1293. doi: 10.1016/S0967-0645(03)00023-7
Choi K.-H., Yang E. J., Kim D., Kang H.-K., Noh J. H., Kim C.-H. (2012). The influence of coastal waters on distributions of heterotrophic protists in the northern East China Sea, and the impact of protist grazing on phytoplankton. J. Plankton. Res. 34, 886–904. doi: 10.1093/plankt/fbs046
Cordier T., Angeles I. B., Henry N., Lejzerowicz F., Berney C., Morard R., et al. (2022). Patterns of eukaryotic diversity from the surface to the deep-ocean sediment. Sci. Adv. 8, eabj9309. doi: 10.1126/sciadv.abj9309
Countway P. D., Vigil P. D., Schnetzer A., Moorthi S. D., Caron D. A. (2010). Seasonal analysis of protistan community structure and diversity at the USC Microbial Observatory (San Pedro Channel, North Pacific Ocean). Limnol. Oceanography. 55, 2381–2396. doi: 10.4319/lo.2010.55.6.2381
De Vargas C., Audic S., Henry N., Decelle J., Mahé F., Logares R., et al. (2015). Eukaryotic plankton diversity in the sunlit ocean. Science 348, 1261605. doi: 10.1126/science.1261605
Dolan J. R., Gimenez A., Cornet-Barthaux V., De Verneil A. (2016). Community structure of tintinnid ciliates of the microzooplankton in the south west pacific ocean: comparison of a high primary productivity with a typical oligotrophic site. J. Eukaryotic. Microbiol. 63, 813–822. doi: 10.1111/jeu.12328
Dolan J. R., Landry M. R., Ritchie M. E. (2013). The species-rich assemblages of tintinnids (marine planktonic protists) are structured by mouth size. ISME. J. 7, 1237–1243. doi: 10.1038/ismej.2013.23
Dolan J. R., Pierce R. W. (2013). “Diversity and distributions of Tintinnids,” in The biology and ecology of Tintinnid ciliates: models for marine plankton. Eds. Dolan J. R., Montagnes D. J., Agatha S., Coats D. W., Stoecker D. K. (Wiley-Blackwell, Hoboken).
Dolan J. R., Pierce R. W., Yang E. J., Kim S. Y. (2012). Southern ocean biogeography of tintinnid ciliates of the marine plankton. J. Eukaryotic. Microbiol. 59, 511–519. doi: 10.1111/j.1550-7408.2012.00646.x
Dolan J. R., Ritchie M. E., Tunin-Ley A., Pizay M.-D. (2009). Dynamics of core and occasional species in the marine plankton: tintinnid ciliates in the north-west Mediterranean Sea. J. Biogeogr. 36, 887–895. doi: 10.1111/j.1365-2699.2008.02046.x
Feng M., Lin S., Zhang W., Wang C., Liu H., Cheung S., et al. (2022). Micro-/meso-scale (Ciliophora: tintinnida) assemblages in three regions around the north pacific ocean. Front. Mar. Sci. 9, 863549. doi: 10.3389/fmars.2022.863549
Feng M., Wang C., Zhang W., Zhang G., Xu H., Zhao Y., et al. (2018). Annual variation of species richness and lorica oral diameter characteristics of tintinnids in a semi-enclosed bay of western Pacific. Estuarine. Coast. Shelf. Sci. 207, 164–174. doi: 10.1016/j.ecss.2018.04.003
Flegontova O., Flegontov P., Jachníková N., Lukeš J., Horák A. (2023). Water masses shape pico-nano eukaryotic communities of the Weddell Sea. Commun. Biol. 6, 64. doi: 10.1038/s42003-023-04452-7
Ganser M. H., Forster D., Liu W., Lin X., Stoeck T., Agatha S. (2021). Genetic diversity in marine planktonic ciliates (Alveolata, ciliophora) suggests distinct geographical patterns – data from chinese and european coastal waters. Front. Mar. Sci. 8, 395. doi: 10.3389/fmars.2021.643822
Gao C. H., Yu G., Cai P. (2021). ggVennDiagram: an intuitive, easy-to-use, and highly customizable R package to generate venn diagram. Front. Genet. 12, 706907. doi: 10.3389/fgene.2021.706907
Gimmler A., Korn R., De Vargas C., Audic S., Stoeck T. (2016). The Tara Oceans voyage reveals global diversity and distribution patterns of marine planktonic ciliates. Sci. Rep. 6, 33555. doi: 10.1038/srep33555
Gong G.-C., Lee Chen Y.-L., Liu K.-K. (1996). Chemical hydrography and chlorophyll a distribution in the East China Sea in summer: implications in nutrient dynamics. Continental. Shelf. Res. 16, 1561–1590. doi: 10.1016/0278-4343(96)00005-2
Gong G.-C., Shiah F.-K., Liu K.-K., Wen Y.-H., Ming-Hsin L. (2000). Spatial and temporal variation of chlorophyll a, primary productivity and chemical hydrography in the southern East China Sea. Continental. Shelf. Res. 20, 411–436. doi: 10.1016/S0278-4343(99)00079-5
Gong G.-C., Wen Y.-H., Wang B.-W., Liu G.-J. (2003). Seasonal variation of chlorophyll a concentration, primary production and environmental conditions in the subtropical East China Sea. Deep. Sea. Res. Part II.: Topical. Stud. Oceanography. 50, 1219–1236. doi: 10.1016/S0967-0645(03)00019-5
Grattepanche J. D., Jeffrey W. H., Gast R. J., Sanders R. W. (2022). Diversity of microbial eukaryotes along the west antarctic peninsula in austral spring. Front. Microbiol. 13, 844856. doi: 10.3389/fmicb.2022.844856
Grattepanche J.-D., Mcmanus G. B., Katz L. A. (2016a). Patchiness of Ciliate Communities Sampled at Varying Spatial Scales along the New England Shelf. PloS One 11, e0167659. doi: 10.1371/journal.pone.0167659
Grattepanche J. D., Santoferrara L. F., Mcmanus G. B., Katz L. A. (2015). Distinct assemblage of planktonic ciliates dominates both photic and deep waters on the New England shelf. Mar. Ecol. Prog. Ser. 526, 1–9. doi: 10.3354/meps11256
Grattepanche J.-D., Santoferrara L. F., Mcmanus G. B., Katz L. A. (2016b). Unexpected biodiversity of ciliates in marine samples from below the photic zone. Mol. Ecol. 25, 3987–4000. doi: 10.1111/mec.13745
Gu B., Wang Y., Xu J., Jiao N., Xu D. (2021). Water mass shapes the distribution patterns of planktonic ciliates (Alveolata, Ciliophora) in the subtropical Pearl River Estuary. Mar. pollut. Bull. 167, 112341. doi: 10.1016/j.marpolbul.2021.112341
Guillou L., Bachar D., Audic S., Bass D., Berney C., Bittner L., et al. (2013). The Protist Ribosomal Reference database (PR2): a catalog of unicellular eukaryote Small Sub-Unit rRNA sequences with curated taxonomy. Nucleic Acids Res 41 (Database issue), D597–D604. doi: 10.1093/nar/gks1160
Huang H., Yang J., Huang S., Gu B., Wang Y., Wang L., et al. (2021). Spatial distribution of planktonic ciliates in the western Pacific Ocean: along the transect from Shenzhen (China) to Pohnpei (Micronesia). Mar. Life Sci. Technol. 3, 103–115. doi: 10.1007/s42995-020-00075-7
Ichikawa H., Beardsley R. C. (2002). The current system in the yellow and east China seas. J. Oceanography. 58, 77–92. doi: 10.1023/A:1015876701363
Ichinomiya M., Dos Santos A. L., Gourvil P., Yoshikawa S., Kamiya M., Ohki K., et al. (2016). Diversity and oceanic distribution of the Parmales (Bolidophyceae), a picoplanktonic group closely related to diatoms. Isme. J. 10, 2419. doi: 10.1038/ismej.2016.38
Isobe A. (2008). Recent advances in ocean-circulation research on the Yellow Sea and East China Sea shelves. J. Oceanography. 64, 569–584. doi: 10.1007/s10872-008-0048-7
Oksanen J.B., Kindt F.G., Legendre R., Minchin P., P.R., G.L., Simpson R.B., et al. (2019). vegan: Community Ecology Package. R package version 2.5-6. Available online: https://cran.r-project.org/web/packages/vegan/index.html. Accessed 1 Sep 2019.
Kang J., Na H. (2022). Long-term variability of the kuroshio shelf intrusion and its relationship to upper-ocean current and temperature variability in the East China sea. Front. Mar. Sci. 9. doi: 10.3389/fmars.2022.812911
Kao K.-J., Huang W.-J., Chou W.-C., Gong G.-C., Weerathunga V. (2023). Factors controlling the sea surface partial pressure of carbon dioxide in upwelling regions: A case study of the Southern East China sea before and after typhoon maria. J. Geophys. Res.: Oceans. 128, e2022JC019195. doi: 10.1029/2022JC019195
Kim Y. O., Choi J., Kang H.-K., Noh J. H., Choi D. H., Oh K.-H. (2021). Spring distribution of ciliate plankton in the southeastern yellow sea in 2019. Ocean. Sci. J. 56, 69–77. doi: 10.1007/s12601-021-00004-4
Lee H.-J., Chao S.-Y. (2003). A climatological description of circulation in and around the East China Sea. Deep. Sea. Res. Part II.: Topical. Stud. Oceanography. 50, 1065–1084. doi: 10.1016/S0967-0645(03)00010-9
Li H., Wang C., Zhao L., Dong Y., Zhao Y., Zhang W. (2023). Variability of tintinnid ciliate communities with water masses in the western Pacific Ocean. J. Plankton. Res. 45, 509–522. doi: 10.1093/plankt/fbad011
Li H., Xuan J., Wang C., Chen Z., Grégori G., Zhao Y., et al. (2021). Summertime tintinnid community in the surface waters across the north pacific transition zone. Front. Microbiol. 12. doi: 10.3389/fmicb.2021.697801
Li H., Zhang W., Zhao Y., Zhao L., Dong Y., Wang C., et al. (2018). Tintinnid diversity in the tropical West Pacific Ocean. Acta Oceanol. Sin. 37, 218–228. doi: 10.1007/s13131-018-1148-x
Li H., Zhao Y., Chen X., Zhang W., Xu J., Li J., et al. (2016). Interaction between neritic and warm water tintinnids in surface waters of East China Sea. Deep. Sea. Res. Part II.: Topical. Stud. Oceanography. 124, 84–92. doi: 10.1016/j.dsr2.2015.06.008
Lin Y.-C., Chin C.-P., Yang J. W., Chiang K.-P., Hsieh C.-H., Gong G.-C., et al. (2022). How communities of marine stramenopiles varied with environmental and biological variables in the subtropical northwestern pacific ocean. Microbial. Ecol. 83, 916–928. doi: 10.1007/s00248-021-01788-7
Liu Z., Gan J., Hu J., Wu H., Cai Z., Deng Y. (2021a). Progress of studies on circulation dynamics in the east China sea: the kuroshio exchanges with the shelf currents. Front. Mar. Sci. 8. doi: 10.3389/fmars.2021.620910
Liu Z., Gan J., Wu H., Hu J., Cai Z., Deng Y. (2021b). Advances on coastal and estuarine circulations around the changjiang estuary in the recent decades, (2000–2020). Front. Mar. Sci. 8. doi: 10.3389/fmars.2021.615929
Liu K.-K., Gong G.-C., Shyu C.-Z., Pai S.-C., Wei C.-L., Chao S.-Y. (1992). Response of Kuroshio upwelling to the onset of the northeast monsoon in the sea north of Taiwan: Observations and a numerical simulation. J. Geophys. Res.: Oceans. 97, 12511–12526. doi: 10.1029/92JC01179
Lynn D. H. (2008). The Ciliated Protozoa. Characterization, Classification, and Guide to the Literature. 3rd edn (Dordrecht: Springer), 605pp.
Maechler M., Rousseeuw P., Struyf A., Hubert M., Hornik K. (2022). Cluster: Cluster Analysis Basics and Extensions. r package version 2.1.4 — for new features, see the 'Changelog' file (in the package source). https://CRAN.R-project.org/package=cluster
Malviya S., Scalco E., Audic S., Vincent F., Veluchamy A., Poulain J., et al. (2016). Insights into global diatom distribution and diversity in the world’s ocean. Proc. Natl. Acad. Sci. 113, E1516–E1525. doi: 10.1073/pnas.1509523113
Martin M. (2011). Cutadapt removes adapter sequences from high-throughput sequencing reads. EMBnet.journal 17 (1), 10–12. doi: 10.14806/ej.17.1.200
Massana R., Terrado R., Forn I., Lovejoy C., Pedrós-Alió C. (2006). Distribution and abundance of uncultured heterotrophic flagellates in the world oceans. Environ. Microbiol. 8, 1515–1522. doi: 10.1111/j.1462-2920.2006.01042.x
Mieczan T., Bartkowska A., Bronowicka-Mielniczuk U., Rudyk-Leuska N. (2023). The effect of peatland restoration on ciliate communities: long-term analyses. Water 15, 3793. doi: 10.3390/w15213793
Morard R., Garet-Delmas M.-J., Mahé F., Romac S., Poulain J., Kucera M., et al. (2018). Surface ocean metabarcoding confirms limited diversity in planktonic foraminifera but reveals unknown hyper-abundant lineages. Sci. Rep. 8, 2539. doi: 10.1038/s41598-018-20833-z
Noan L. B., Frédéric M., Stéphane A., Céline D., Marie-José G., Julie P., et al. (2016). Global patterns of pelagic dinoflagellate diversity across protist size classes unveiled by metabarcoding. Environ. Microbiol. 18, 609–626. doi: 10.1111/1462-2920.13039
Pierce R. W., Turner J. T. (1992). Ecology of planktonic ciliates in marine food webs. Rev. Aquat. Sci. 6, 139–181.
Pitta P., Giannakourou A. (2000). Planktonic ciliates in the oligotrophic Eastern Mediterranean: vertical, spatial distribution and mixotrophy. Mar. Ecol. Prog. Ser. 194, 269–282. doi: 10.3354/meps194269
Quan Q., Mao X., Yang X., Hu Y., Zhang H., Jiang W. (2013). Seasonal variations of several main water masses in the southern Yellow Sea and East China Sea in 2011. J. Ocean. Univ. China 12, 524–536. doi: 10.1007/s11802-013-2198-5
Romano F., Symiakaki K., Pitta P. (2021). Temporal variability of planktonic ciliates in a coastal oligotrophic environment: mixotrophy, size classes and vertical distribution. Front. Mar. Sci. 8, 113. doi: 10.3389/fmars.2021.641589
Romari K., Vaulot D. (2004). Composition and temporal variability of picoeukaryote communities at a coastal site of the English Channel from 18S rDNA sequences. Limnol. Oceanography. 49, 784–798. doi: 10.4319/lo.2004.49.3.0784
Santoferrara L., Alder V. (2009). Abundance trends and ecology of planktonic ciliates of the south-western Atlantic (35–63° S): a comparison between neritic and oceanic environments. J. Plankton. Res. 31, 837–851. doi: 10.1093/plankt/fbp033
Santoferrara L., Burki F., Filker S., Logares R., Dunthorn M., Mcmanus G. B. (2020). Perspectives from ten years of protist studies by high-throughput metabarcoding. J. Eukaryotic. Microbiol. 67, 612–622. doi: 10.1111/jeu.12813
Santoferrara L. F., Grattepanche J.-D., Katz L. A., Mcmanus G. B. (2016). Patterns and processes in microbial biogeography: do molecules and morphologies give the same answers[quest]. ISME. J. 10, 1779–1790. doi: 10.1038/ismej.2015.224
Santoferrara L. F., Mcmanus G. B., Alder V. A. (2013). Utility of genetic markers and morphology for species discrimination within the order tintinnida (Ciliophora, spirotrichea). Protist 164, 24–36. doi: 10.1016/j.protis.2011.12.002
Santoferrara L. F., Qureshi A., Sher A., Blanco-Bercial L. (2023). The photic-aphotic divide is a strong ecological and evolutionary force determining the distribution of ciliates (Alveolata, Ciliophora) in the ocean. J. Eukaryotic. Microbiol. n/a, e12976. doi: 10.1111/jeu.12976
Santoferrara L. F., Rubin E., Mcmanus G. B. (2018). Global and local DNA (meta)barcoding reveal new biogeography patterns in tintinnid ciliates. J. Plankton. Res. 40, 209–221. doi: 10.1093/plankt/fby011
Schlitzer R. (2022) Ocean data view. Available online at: http://odv.awi.de.
Stoeck T., Bass D., Nebel M., Christen R., Jones M. D. M., Breiner H.-W., et al. (2010). Multiple marker parallel tag environmental DNA sequencing reveals a highly complex eukaryotic community in marine anoxic water. Mol. Ecol. 19, 21–31. doi: 10.1111/j.1365-294X.2009.04480.x
Sun P., Huang L., Xu D., Warren A., Huang B., Wang Y., et al. (2019). Integrated space-time dataset reveals high diversity and distinct community structure of ciliates in mesopelagic waters of the Northern South China sea. Front. Microbiol. 10, 2178. doi: 10.3389/fmicb.2019.02178
Sun P., Wang Y., Laws E., Huang B. (2020). Water mass–driven spatial effects and environmental heterogeneity shape microeukaryote biogeography in a subtropical, hydrographically complex ocean system - A case study of ciliates. Sci. Total. Environ. 706, 135753. doi: 10.1016/j.scitotenv.2019.135753
Suzuki T., Miyabe C. (2007). Ecological balance between ciliate plankton and its prey candidates, pico- and nanoplankton, in the East China Sea. Hydrobiologia 586, 403–410. doi: 10.1007/s10750-007-0715-5
Team R. D. C. (2013). R: A Language and Environment for Statistical Computing. Vienna, Austria: R Foundation for Statistical Computing.
Tragin M., Vaulot D. (2019). Novel diversity within marine Mamiellophyceae (Chlorophyta) unveiled by metabarcoding. Sci. Rep. 9, 5190. doi: 10.1038/s41598-019-41680-6
Tsai A.-Y., Gong G.-C., Chiang K.-P., Chao C.-F., Guo H.-R. (2011). Long-term, (1998–2007) trends on the spatial distribution of heterotrophic ciliates in the East China Sea in summer: effect of the Three Gorges Dam construction. J. Oceanography. 67, 725–737. doi: 10.1007/s10872-011-0069-5
Tucker S. J., Mcmanus G. B., Katz L. A., Grattepanche J.-D. (2017). Distribution of abundant and active planktonic ciliates in coastal and slope waters off New England. Front. Microbiol. 8. doi: 10.3389/fmicb.2017.02178
Wang C., Dong Y., Denis M., Wei Y., Li H., Zheng S., et al. (2023a). Diel variations in planktonic ciliate community structure in the northern South China Sea and tropical Western Pacific. Sci. Rep. 13, 3843. doi: 10.1038/s41598-023-30973-6
Wang C., Li H., Dong Y., Zhao L., Gregori G., Zhao Y., et al. (2021a). Planktonic ciliate trait structure variation over Yap, Mariana, and Caroline seamounts in the tropical western Pacific Ocean. J. Oceanol. Limnol. 39, 1705–1717. doi: 10.1007/s00343-021-0476-4
Wang C., Wang X., Wei Y., Guo G., Li H., Wan A., et al. (2023b). Pelagic ciliate (Ciliophora) communities in the Southern Ocean: Bioindicator to water mass, habitat suitability classification and potential response to global warming. Prog. Oceanography. 216, 103081. doi: 10.1016/j.pocean.2023.103081
Wang C., Xu M., Xuan J., Li H., Zheng S., Zhao Y., et al. (2021b). Impact of the warm eddy on planktonic ciliate, with an emphasis on tintinnids as bioindicator species. Ecol. Indic. 133, 108441. doi: 10.1016/j.ecolind.2021.108441
Wang Y., Zhang W., Lin Y., Zheng L., Cao W., Yang J. (2013). Spatial pattern of the planktonic ciliate community and its relationship with the environment in spring in the northern Beibu Gulf, South China Sea. Oceanol. Hydrobiol. Stud. 42, 470–479. doi: 10.2478/s13545-013-0103-x
Wickham H. (2016). ggplot2: Elegant Graphics for Data Analysis (New York: Springer-Verlag). doi: 10.1007/978-3-319-24277-4
Wu G., Cao W., Wang F., Su X., Yan Y., Guan Q. (2019). Riverine nutrient fluxes and environmental effects on China’s estuaries. Sci. Total. Environ. 661, 130–137. doi: 10.1016/j.scitotenv.2019.01.120
Wu W., Logares R., Huang B., Hsieh C.-H. (2017). Abundant and rare picoeukaryotic sub-communities present contrasting patterns in the epipelagic waters of marginal seas in the northwestern Pacific Ocean. Environ. Microbiol. 19, 287–300. doi: 10.1111/1462-2920.13606
Wu C.-R., Lu H.-F., Chao S.-Y. (2008). A numerical study on the formation of upwelling off northeast Taiwan. J. Geophys. Res. (Oceans). 113, C08025. doi: 10.1029/2007JC004697
Xu Z., Song X., Wang M., Liu Q., Jiang Y., Shao H., et al. (2017). Community patterns and temporal variation of picoeukaryotes in response to changes in the Yellow Sea Warm Current. J. Oceanography. 73, 687–699. doi: 10.1007/s10872-017-0425-1
Xu D., Sun P., Warren A., Noh J. H., Choi D. L., Shin M. K., et al. (2013). Phylogenetic investigations on ten genera of tintinnid ciliates (Ciliophora: Spirotrichea: Tintinnida), based on small subunit ribosomal RNA gene sequences. J. Eukaryot. Microbiol. 60, 192–202. doi: 10.1111/jeu.12023
Yang J., Chen Z., Chen D., Xu D. (2021). Spatial distribution of the microzooplankton communities in the northern South China Sea: Insights into their function in microbial food webs. Mar. pollut. Bull. 162, 111898. doi: 10.1016/j.marpolbul.2020.111898
Yang J., Huang S., Fan W., Warren A., Jiao N., Xu D. (2020). Spatial distribution patterns of planktonic ciliate communities in the East China Sea: Potential indicators of water masses. Mar. pollut. Bull. 156, 111253. doi: 10.1016/j.marpolbul.2020.111253
Yang D., Yin B., Liu Z., Feng X. (2011). Numerical study of the ocean circulation on the East China Sea shelf and a Kuroshio bottom branch northeast of Taiwan in summer. J. Geophys. Res.: Oceans. 116, C05015. doi: 10.1029/2010JC006777
Yu Y., Zhang W., Feng M., Xu X. (2022). Seasonal variation of vegetative stages and cysts of planktonic ciliates in a temperate coastal bay. J. Plankton. Res. 44, 911–922. doi: 10.1093/plankt/fbac047
Yu Y., Zhang W., Feng M., Zhao Y., Zhang C., Zhou F., et al. (2016). Differences in the vertical distribution and response to freshwater discharge between aloricate ciliates and tintinnids in the East China Sea. J. Mar. Syst. 154, 103–109. doi: 10.1016/j.jmarsys.2015.02.005
Yuan D., Zhu J., Li C., Hu D. (2008). Cross-shelf circulation in the Yellow and East China Seas indicated by MODIS satellite observations. J. Mar. Syst. 70, 134–149. doi: 10.1016/j.jmarsys.2007.04.002
Zhang C., Sun J., Wang D., Song S., Zhang X., Munir S. (2017). Tintinnid community structure in the eastern equatorial Indian Ocean during the spring inter−monsoon period. Aquat. Biol. 26, 87–100. doi: 10.3354/ab00677
Zhang C., Zhang W., Ni X., Zhao Y., Huang L., Xiao T. (2015). Influence of different water masses on planktonic ciliate distribution on the East China Sea shelf. J. Mar. Syst. 141, 98–111. doi: 10.1016/j.jmarsys.2014.09.003
Zhao F., Filker S., Xu K., Huang P., Zheng S. (2017). Patterns and drivers of vertical distribution of the ciliate community from the surface to the abyssopelagic zone in the western pacific ocean. Front. Microbiol. 8. doi: 10.3389/fmicb.2017.02559
Zheng L., Chen B., Liu X., Huang B., Liu H., Song S. (2015). Seasonal variations in the effect of microzooplankton grazing on phytoplankton in the East China Sea. Continental. Shelf. Res. 111, 304–315. doi: 10.1016/j.csr.2015.08.010
Zhou P., Song X., Yuan Y., Cao X., Wang W., Chi L., et al. (2018). Water mass analysis of the east China sea and interannual variation of kuroshio subsurface water intrusion through an optimum multiparameter method. J. Geophys. Res.: Oceans. 123, 3723–3738. doi: 10.1029/2018JC013882
Keywords: planktonic ciliate, metabarcoding analysis, SSU rDNA, spatial distribution, East China Sea (ECS)
Citation: Chen W-T, Lin Y-C, Tsai S-F and Chiang K-P (2024) Diversity and distribution of small-sized planktonic ciliate communities in the East China Sea. Front. Mar. Sci. 11:1349707. doi: 10.3389/fmars.2024.1349707
Received: 05 December 2023; Accepted: 03 May 2024;
Published: 24 May 2024.
Edited by:
Stelios Katsanevakis, University of the Aegean, GreeceReviewed by:
Wuchang Zhang, Chinese Academy of Sciences (CAS), ChinaJiaxing Liu, Chinese Academy of Sciences (CAS), China
Copyright © 2024 Chen, Lin, Tsai and Chiang. This is an open-access article distributed under the terms of the Creative Commons Attribution License (CC BY). The use, distribution or reproduction in other forums is permitted, provided the original author(s) and the copyright owner(s) are credited and that the original publication in this journal is cited, in accordance with accepted academic practice. No use, distribution or reproduction is permitted which does not comply with these terms.
*Correspondence: Kuo-Ping Chiang, a3BjaGlhbmdAbWFpbC5udG91LmVkdS50dw==; Sheng-Fang Tsai, c3RzYWlAbWFpbC5udG91LmVkdS50dw==