- 1Área de Paleontología, Departamento de Geología, Universidad de Salamanca, Salamanca, Spain
- 2British Antarctic Survey, Natural Environment Research Council, Cambridge, United Kingdom
- 3CEFREM, CNRS - Université de Perpignan Via Domitia, Perpignan, France
- 4GRC Geociències Marines, Departament de Dinàmica de la Terra i de l’Oceà, Universitat de Barcelona, Barcelona, Spain
Pteropods are a group of cosmopolitan holoplanktic gastropods that produce an aragonite shell and play an important role in both marine ecosystems and geochemical cycles. In addition to being affected by anthropogenic impacts that include warming and changes in carbonate system parameters, the Mediterranean Sea is considered to be understudied concerning pteropods dynamics and abundances. This work aims to document the modern spatial and temporal distributions of pteropods populations in the Northwestern and Central Mediterranean Sea (Gulf of Lions and Strait of Sicily), respectively. We present data from two sediment-trap records that cover the timeframe between early 1996 and early 2004 for the Gulf of Lions and late 2013 to late 2014 for the Sicily Strait. A total of 843 pteropod shells and 18 different species were identified. Limacina inflata, Creseis virgula and Creseis clava were the most abundant species in the Gulf of Lions, while in the Sicily Strait, C. conica replaced C. clava as the most abundant species. These taxons represented around 70% of the total individuals identified in both sites. Overall, our results suggest a greater pteropod abundance in the Gulf of Lions than in the Sicily Strait, most likely due to enhanced food conditions. In the Gulf of Lions, maximum fluxes occurred in autumn (32.5% of the annual pteropod fluxes registered in October), while in the Sicily Strait peak fluxes occurred in winter (30.5% of the annual pteropod fluxes registered in January). Comparison of temporal changes pteropod fluxes with satellite sea surface temperature (SST), and chlorophyll-a concentration suggest a possible positive effect of high algal accumulation and cool water conditions in the Strait of Sicily on the main pteropod groups. In turn, no clear relationships between pteropod groups, SST and chlorophyll-a were identified in the Gulf of Lions, highlighting the effect of salinity and carbonate system parameters. Overall, and despite the limitations associated with the use of sediment traps for pteropod population monitoring, the consistency of our results with the literature supports the use of sediment traps as useful tools for documenting the diversity and temporal distribution of pteropods.
1 Introduction
Pteropods are a group of holoplanktic opisthobranch gastropods found in all regions of the global ocean (Bednaršek et al., 2012a). Adapting their gastropod foot into a wing-type parapodia allows them to be active swimmers and have a pelagic life cycle (Lalli and Gilmer, 1989). Thecosome pteropods produce a shell made of aragonite, a calcium carbonate polymorph. From a biogeochemical point of view, as marine calcifiers, pteropods contribute between 10% and 42% of the total CaCO3 flux worldwide depending on the zone of the world’s ocean (Bednaršek et al., 2012b; Berner and Honjo, 1981). Recent work showed that their calcification process is a non-negligible contributor to the regulation of the Carbonate Counter Pump (CCP) strength (Manno et al., 2018). From an ecological perspective, pteropods represent an important food source for a wide variety of carnivorous zooplankton, different fishes such as salmon or mackerel and bigger predators like seabirds and whales (Lalli and Gilmer, 1989; Aydin et al., 2005; Hunt et al., 2010). Therefore, pteropods play a fundamental role in both the marine biogeochemical cycles and ecosystems.
The Mediterranean Sea (Figure 1A) is an elongated and semi-enclosed sea characterized by a negative hydrological balance (the evaporation exceeds the freshwater inputs). It has been described as both calcite and aragonite supersaturated (Ω>>1) (Álvarez et al., 2014) and is overall considered to be an oligotrophic to ultra-oligotrophic sea in its easternmost basins (Krom et al., 2005; Schroeder et al., 2010; Huertas et al., 2012). A study from Lazzari et al. (2014) stated that sea surface temperatures (SSTs) warming in the Mediterranean exceeds the global trend (1.5-2°C increase by 2100). pH is expected to vary according to the global average in the coming decades (a 0.3-0.4 unit decrease by the end of the century; Hassoun et al., 2015). Moreover, the fast turnover of its waters (Bethoux et al., 1999) and rapid anthropogenic CO2 penetration (Schneider et al., 2007; Hassoun et al., 2022) makes it a sensitive part of the global ocean to increasing atmospheric CO2 (Ziveri et al., 2012).
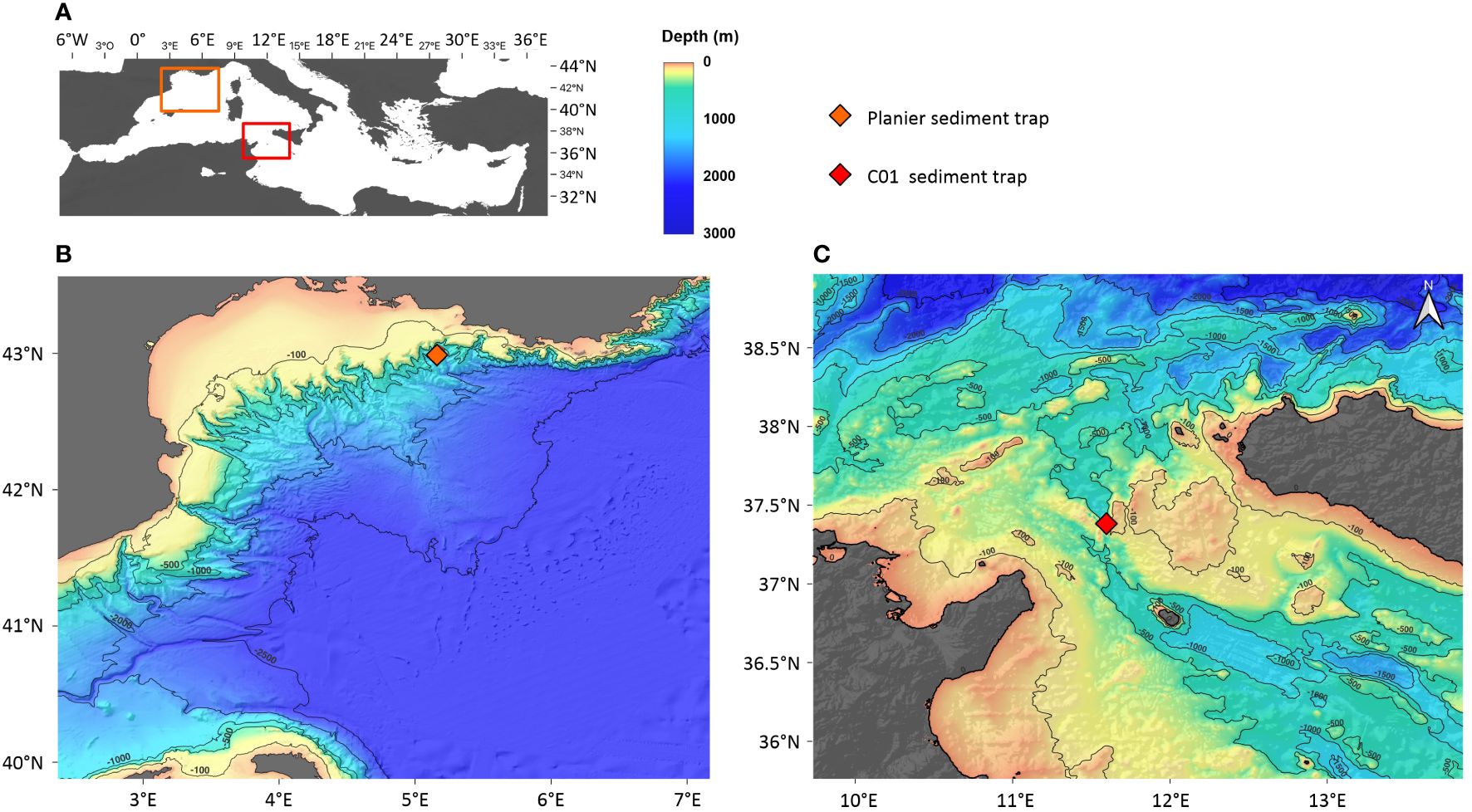
Figure 1 (A) Geographical situation of the Mediterranean Sea and the two studied sites (orange and red rectangles). (B) Geographical situation and bathymetry of the GoL and the location of the Planier sediment trap (orange diamond). (C) Geographical situation and bathymetry of the SS and the location of the C01 sediment trap (red diamond). The topographic model was downloaded from the GEBCO database.
The Gulf of Lions (GoL) is located in the northwestern part of the Mediterranean Sea (Figure 1B). In terms of nutrient distribution, the GoL can be considered an exception to the general oligotrophy of the Mediterranean Sea. During winter, strong northern winds induce dense water formation and the deep mixing of the upper water column both in the shelf and offshore, which enriches the surface layer with nutrients (Houpert et al., 2016; Kessouri et al., 2018; Many et al., 2021). Enhanced solar radiation during spring and summer causes stratification of the surface layer, leading to a nutrient depletion by phytoplankton that lasts until late summer, then autumn cooling brings down the stratification and sets a fall bloom (Heussner et al., 2006; Rigual-Hernández et al., 2013).
The Sicily Strait (SS) is located in the central part of the Mediterranean (Figure 1C). It is the sill that separates the western and eastern Mediterranean basins, which highlights its importance regarding the overall basin trophic regimes (Siokou-Frangou et al., 2010). The SS water column is affected by different water masses (Béranger et al., 2004) and its surface circulation presents a high seasonal variability. During winter and spring, Atlantic and western basin water masses dominate the surface circulation. During summer and fall, eastern basin waters dominate the surface circulation (Robinson et al., 1999; Lermusiaux and Robinson, 2001; Béranger et al., 2004) which results in an increased water mixing.
It has been described that pteropods abundance accounts for 1% to 6.6% of the Mediterranean zooplankton community (Fernández De Puelles et al., 2007; Siokou-Frangou et al., 2010; Granata et al., 2020). Few studies have focused on pteropod dynamics and their response to climate change in this part of the global ocean (Wall-Palmer et al., 2014; Manno et al., 2019; Johnson et al., 2023), therefore, it is generally accepted that there is a gap in pteropods research in the Mediterranean Sea (Hassoun et al., 2015; Bednaršek et al., 2016). However, the composition and temporal dynamics of pteropod populations in the northwestern and central Mediterranean Sea have never been documented. Therefore, this work aims to improve the current knowledge about the thecosome pteropods populations in recent years in both the GoL and the SS. For this purpose, we analyzed pteropods abundances and fluxes on an interannual and seasonal scale in samples collected by two moored sequential-sampling sediment traps deployed in the GoL and the SS (Figures 1B, C). Previous work in the Mediterranean (Hernández-Almeida et al., 2011; Rigual-Hernández et al., 2012; Avnaim-Katav et al., 2020) and across all major oceans [Southern Ocean: Manno et al. (2007); Howard et al. (2011); Roberts et al. (2014), North Atlantic: Rebotim et al. (2017), North and Central Pacific: Eguchi et al. (1999); Arabian Sea: Chernihovsky et al. (2018)] have used sediment traps in order analyze the zooplankton dynamics as well as the ecology and calcification on different time scales (Moy et al., 2009; Aldridge et al., 2012; Makabe et al., 2016). In that regard, sediment traps have been proven as a reliable tool to document the composition and seasonality of different zooplankton groups (Boltovskoy et al., 1996; Willis et al., 2008; Sampei et al., 2012; Matsuno et al., 2014). For pteropods phenology and population dynamics, net sampling or plankton tows are more widely used (Anglada-Ortiz et al., 2021, 2023; Beare et al., 2013; Loeb and Santora, 2013) than sediment traps (Oakes and Sessa, 2020); as these organisms might actively swim into the cups and can be considered an artefact generated by the presence of sediment traps that may bias total mass calculations (Buesseler et al., 2007). However, comparisons between net and sediment traps have demonstrated a close correlation between the assemblages collected by these two methods (Almogi-Labin et al., 1988; Makabe et al., 2016; Weldrick et al., 2021). In addition, previous work on pteropods collected from a sediment trap (Forbes et al., 1992) compared the pteropod’s seasonal distribution with the sea ice cover, allowing to document the pteropods populations in remote regions where regular net sampling is not possible. Then, the advantages of sediment traps are: (i) an efficient tool to provide insights into pteropod populations in open ocean environments over extended periods (Almogi-Labin et al., 1988; Grossman et al., 1986; Mohan et al., 2006; Roberts et al., 2014); (ii) they can provide datasets that document the seasonality due to their constant sampling, therefore they can register reliable data concerning annual fluxes (Jonkers et al., 2019). Overall, this represents an advantage compared to shipboard observations that only capture a snapshot of local conditions.
The collection intervals presented here cover eight years (March 1996 to January 2004) for the GoL and one year for the SS (November 2013 to October 2014). Overall, results indicate a higher pteropod abundance in the GoL, a similar assemblage composition and dominant species between the two sites yet a displaying distinct seasonal patterns. In addition, we compare the seasonal and interannual patterns of pteropod fluxes with chlorophyll-a and sea surface temperature satellite data and a suite of environmental parameters from the DYFAMED site, located in the Ligurian Sea, in an attempt to identify the drivers that affect the pteropod populations in the NW and Central Mediterranean.
2 Materials and methods
2.1 Sediment traps
Planier sediment trap (43.02°N, 5.18°E) is part of a series of mooring lines deployments in the GoL continental margin that started in 1993 from both French and European projects (PNEC, Euromarge-NB, MTP II-MATER, EUROSTRATAFORM) (see Heussner et al. (2006) for further details). Planier mooring site is still serviced annually as part of the MOOSE program (Mediterranean Ocean Observing System for the Environment) (Coppola et al., 2019). This sediment trap is located in the axis of the Planier Canyon (Figure 1A) at 530m water depth in a water column of ~1000m. Detailed mooring line equipment, design and preparation can be found in both Heussner et al. (1990, 2006). The sampling interval was every 14 days until early 1997 when it was set at 1 month. The mooring lines were maintained and serviced every 6 months (Heussner et al., 2006). Here, we present data from sediment trap samples collected by the Planier sediment between January 1996 and January 2004.
The C01 sediment trap was deployed in the mooring line maintained by ISMAR-CNR in the Sicily Channel (37.38°N, 11.59°E) thanks to the TransNational Access activity in the FP7 JERICO project (Mediterranean sediment Trap Observatory). This mooring line was equipped with a sediment trap located 413m deep in a water column of around 450m. Due to the vicinity of the trap to the seafloor, particular attention was paid to the identification of resuspended sediment, and although these particles were not frequent, we cannot completely rule out this phenomenon. The sampling period was 15 to 16 days from November 2013 to July 2014 and from September 2014 to October 2014, the timespan between July 2014 and September 2014 had a sampling period of 31 days. Here we present data from the full sampling period.
Both mooring lines sediment traps were a sequential-sampling Technicap PPS3/3 model, conical in shape with a 2.5 height/diameter ratio and equipped with 12 sampling cups. Before deployment and to limit the degradation of the material caught, sampling cups from both mooring lines were filled with a 5% formalin solution prepared with 40% formaldehyde mixed with 0.45 μm filtered seawater. The solution was then buffered with sodium borate to keep the pH stable and avoid the dissolution of carbonate. Only the samples from the C01 sediment trap were available for pH measurements, which showed results comprised between 7.7 and 7.9.
2.2 Sample processing
The buffered sediment trap samples of the Planier and C01 traps were divided into subsamples with a rotary splitting method (Heussner et al., 1990). Before the subsampling, zooplankton individuals bigger than 1 mm were wet-sieved through a 1mm mesh and manually removed.
Concerning the Planier sediment trap samples, aliquots were used, mainly 1/8 but also 1/3, 1/5, 1/10 and 1/16 on the base of the amount of material recovered in the original bottle. These samples were then wet-sieved with a phosphate-buffered solution (pH=7.5) through two mesh sizes (63 and 150 μm) and left to dry at ambient temperature. More details on sample preparation can be found in Rigual-Hernández et al. (2012).
On the other hand, C01 sediment trap samples consisted uniquely of 1/6 aliquots of the original bottle. Samples were wet-sieved according to the same size-fractions previously described and the same phosphate buffered solution was used.
A total of 165 samples from the Planier sediment trap and 19 from the C01 sediment trap were analyzed for characterization and quantification of pteropod assemblages. No further splits were applied and the whole available material was analyzed. Overall, pteropods were identified in 20 and 15 samples from Planier and C01 sediment traps respectively.
2.3 Pteropods picking and identification
We identified, counted and analyzed all the individuals as >63 μm, a fraction considered representative of the overall pteropod populations (Beccari et al., 2023). Most of the shells encountered were in a good enough preservation state to be identified to the species level (Figure 2), however, a few specimens appeared to be broken. Overall, no signs of partial dissolution were observed and the preservation state of the identified individuals was similar between the Planier and C01 sediment traps. This is in agreement with previous pteropod work with sediment trap samples (Oakes and Sessa, 2020). Identification was carried out following the taxonomic concepts of Bé and Gilmer (1977). Empty shells were differentiated from the shells including the soft body tissue. We theorize that empty shells were the product of successful attacks by their main predator Clione limacina (Böer et al., 2005), even though this organism has not been identified in our samples; while soft-body preserved individuals are either considered swimmers (Hamner et al., 1975) (organisms that actively swim onto the trap) or carcasses (a result of natural mortality) (Knauer et al., 1979; Michaels et al., 1990; Lischka et al., 2011).
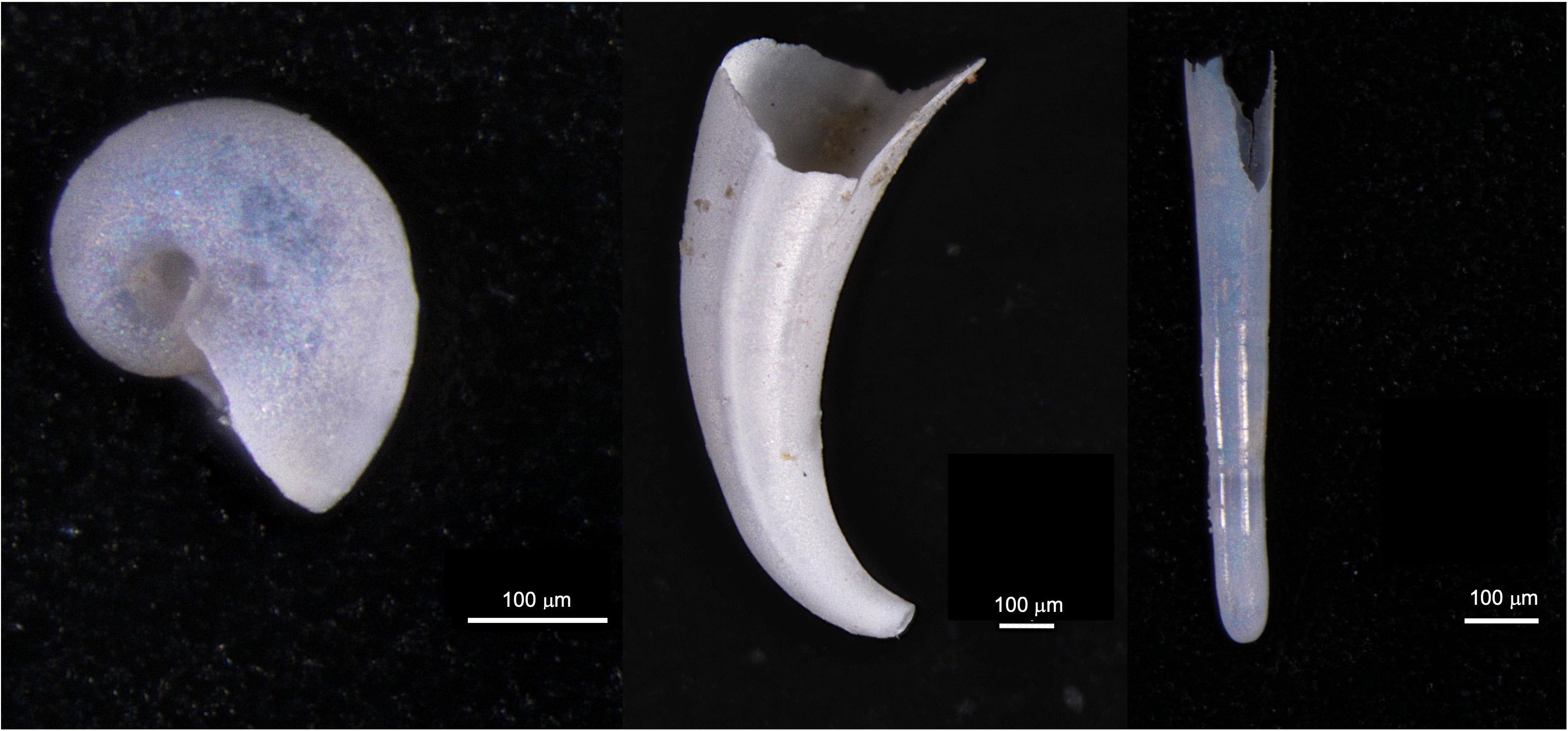
Figure 2 Photographs of the most abundant pteropod shells identified in this study: Limacina inflata, Creseis virgula and Creseis clava shells (from left to right) collected by the Planier sediment trap.
Pteropod fluxes were calculated according to the following formula:
Where “N” stands for the number of pteropods individuals, “Aliq.” is the aliquot of the sample analyzed, “D” represents the number of days the sediment trap cup stayed open and “0.1256” is the surface (in m2) of the PPS3/3 sediment trap model.
Finally, as stated previously, the C01 sediment trap is located around 30m from the sediment floor. In order to quantify the effect of resuspended material, the benthic foraminifera (unicellular protists that produce a calcareous shell and live in the close vicinity of the seabed sediment) were also counted (see Supplementary Data).
2.4 Environmental data
Satellite datasets were used for both sites concerning the chlorophyll-a, an indicator of algal biomass concentration, a possible food source for pteropods (Schiebel et al., 2001); and the SST, an indirect indicator of the water column stratification. Data was downloaded according to the maximum resolution available for both areas and using the same gridding around each area studied.
For the GoL site, chlorophyll-a data was obtained from SeaWiFS monthly derived concentrations from September 1997 to March 2004 (Rigual-Hernández et al., 2012). SST data was downloaded from the NOAA database with a 0.2x0.2° gridding around the mooring location. In addition, factors such as salinity and carbonate system parameters were retrieved from the DYFAMED database (Coppola et al., 2023), located in the Ligurian Sea and considered a representative site for the seasonal and interannual variability of the water column properties in the NW Mediterranean (Heussner et al., 2006). For the SS, the SST (°C) and chlorophyll-a (mg m-3) datasets were obtained from MODIS L3m satellite through NASA’s Giovanni program with an 8-day and 4 km resolution. Data was also retrieved on a 0.2x0.2° area around the mooring location. However, no in-situ measurements were available for neither the nutrients or the carbonate system parameters for the SS, making any comparison between the two studied sites unreliable in regard to the influence of environmental parameters others than the SST and the chlorophyll-a.
2.5 Statistical analysis
Concerning the statistical analyses, the descriptive analysis such as the mean, medians and standard deviation have been carried out with the Past4 program. The satellite and DYFAMED data described previously have been resampled to have uninterrupted reliable monthly environmental values. The resampling was carried out every 7 to 10 days with the QAnalySeries programme. Spearman correlation analyses (Table 1) were calculated with the Past4 program and a p< 0.05 was used to consider a correlation as significant. The environmental parameters considered in the correlation analyses were the SST, chlorophyll-a, but also carbonate system parameters downloaded from the DYFAMED database. Finally, a detrended canonical correspondence analysis (dCCA) was also used to estimate the influence the environmental variables on the pteropod populations. A CCA is a correspondence analysis of a species matrix where each site has given values for one or more environmental variables and it is considered an example of direct gradient analysis, where the gradient in environmental variables is known and the species abundances/fluxes are considered to be a response to this gradient (Nielsen, 2000).
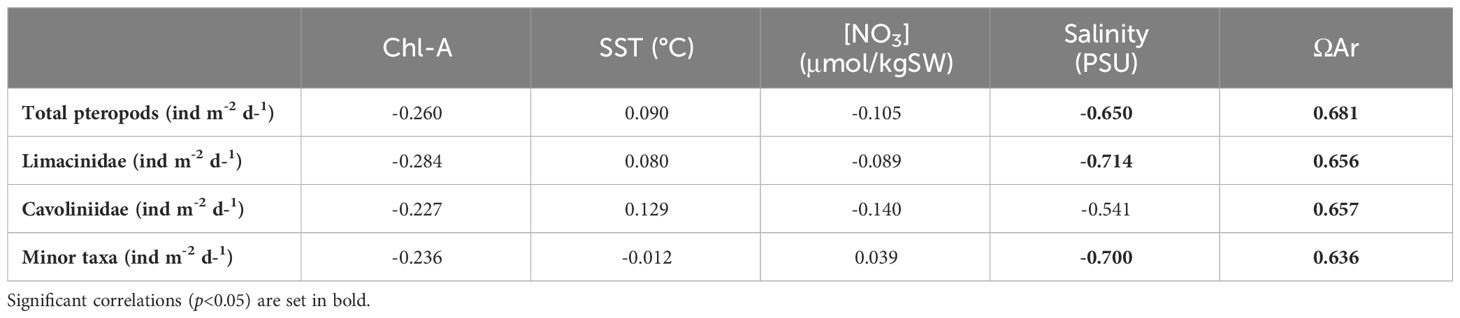
Table 1 Correlation matrix (see section 2.5) between the pteropod fluxes from the GoL and the satellite data (chlorophyll-a and SST) and the DYFAMED site data (nitrates concentration, salinity and ΩAr).
3 Results
3.1 Composition of pteropod assemblages collected by the traps
A total of 784 pteropod shells were counted from the GoL record and 59 from the SS.
18 different species were identified and grouped under three categories based on their morphology and ecology: Limacinidae (Limacina inflata (Heliconoides inflatus), L. bulimoides, L. trochiformis, L. retroversa and L. lesueurii), Cavoliniidae Creseis (Creseis virgula/Cavolinia inflexa, C. conica, C. convexa, C. clava and Clio cuspida) and Cavoliniidae and minor taxa (Styliola subula, Atlanta selvagensis, Peracles molusccensis, P. diversa, Firoloidea desmarestia and A. turritella/bruna). 17 and 12 species were identified in the GoL and SS records, respectively.
The most abundant species in both sites was L. inflata (Figure 3A), which represented 39.4% of the total pteropods from the GoL and 49.1% from the SS, respectively. C. virgula was the second most abundant taxon, representing 16% (Figure 3B) of the pteropods from the GoL and 11.9% from the SS (Figure 3C). The third most abundant taxon was C. clava in the Gulf of Lions, representing 13.5%. In the SS, C. conica was the third most abundant species, accounting for 11.9%. Other species included C. conica (6.9%), C. convexa (5%) and F. desmasrestia (4.9%) in the GoL; and L. trochiformis (8.5%) and C. clava (6.8%) in the SS. The remaining species abundances were below 5% in both sites.
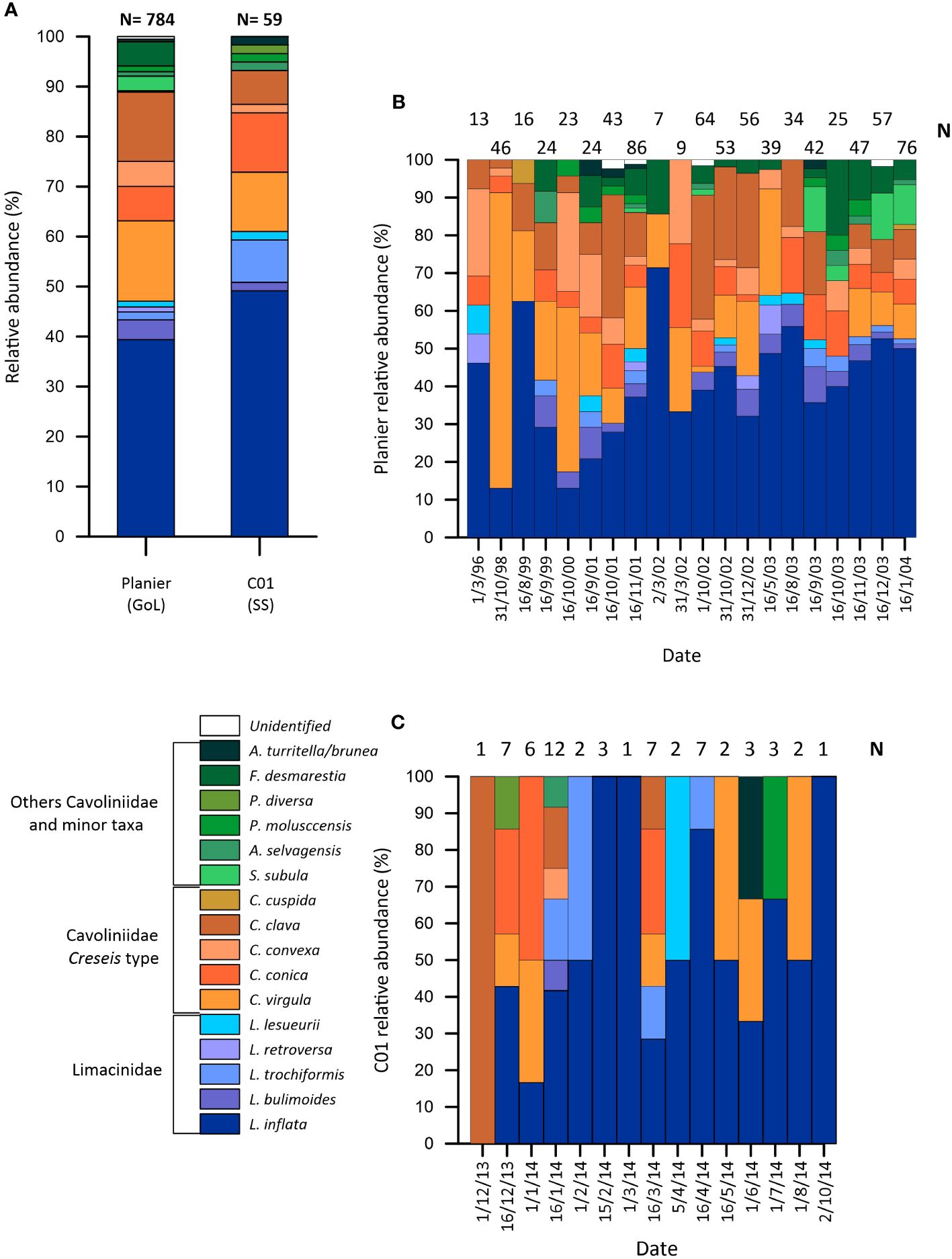
Figure 3 (A) Total relative abundance (%) of the different species identified across all samples, (B) relative abundance (%) for all the samples with pteropods from the GoL (Planier) and (C) relative abundance (%) from the SS (C01). Note that the period covered differs between the GoL and the SS [further details about the aliquots can be found in (Heussner et al., 2006; Rigual-Hernández et al., 2012)].
Limacinidae aggrupation was the most abundant, with 47.1% and 61% of the total pteropods in the GoL and the SS, respectively. Cavoliniidae Creseis type displayed 42.1% in the GoL and 32.2% in the SS. Finally, other Cavoliniidae and minor taxa reached 10.8% in the GoL and 6.8% in the SS.
3.2 Differences between empty and soft-body preserved specimens
As empty pteropod shells are considered the product of successful predation and soft-body preserved can either be considered carcasses or active swimmers, soft-body preserved and empty pteropod shells were differentiated (Figure 4).
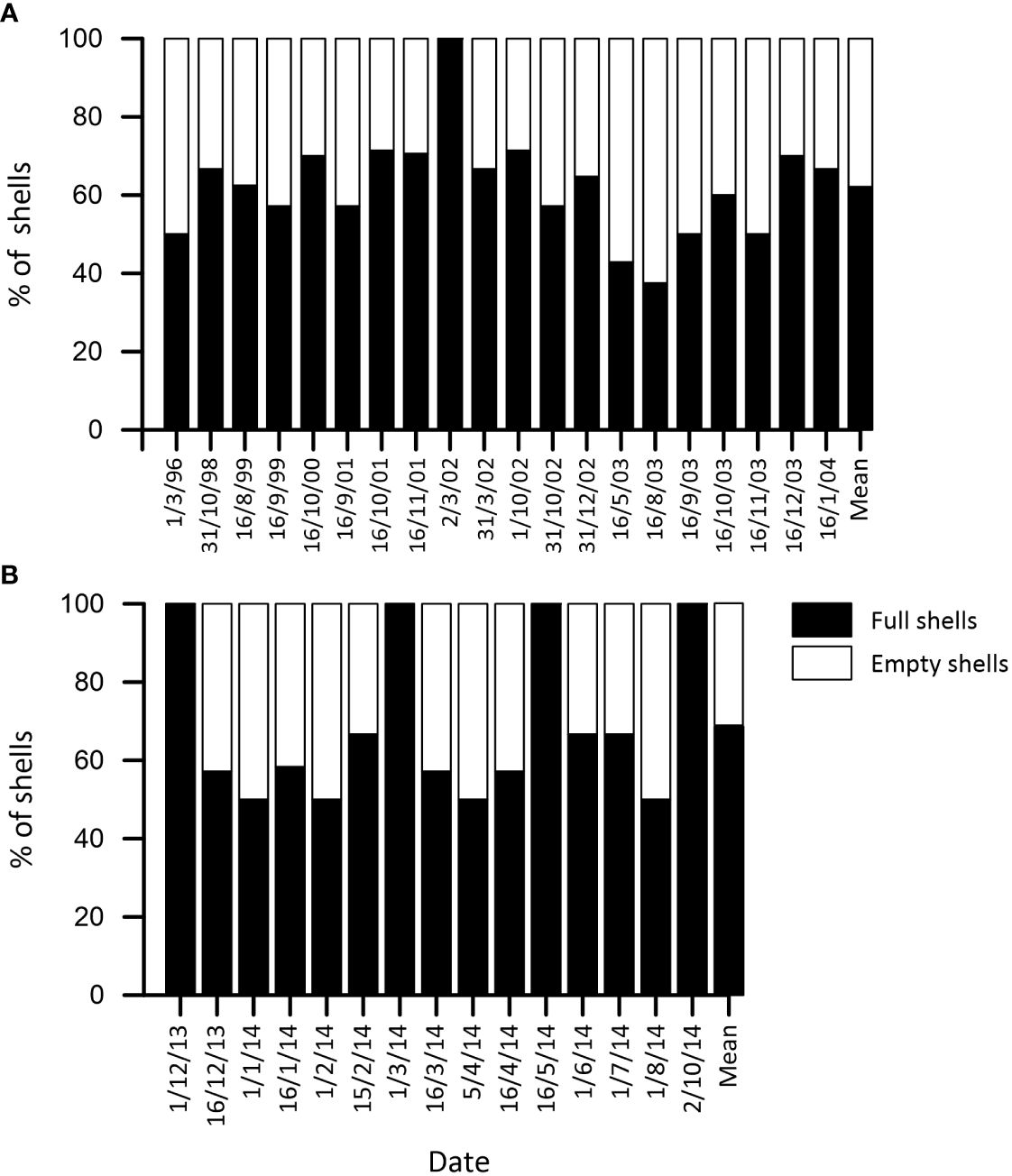
Figure 4 Comparison between soft-body preserved shells (full) and empty shells (%) in each sample in (A) the GoL and (B) the SS. The last column in each graphic represents the mean value from the corresponding studied site.
In the GoL, our results showed that empty shells represented between 0 and around 60%, with a mean value of 38% (Figure 4A), highlighting a dominance of soft-body preserved individuals. However, no clear seasonal or interannual pattern in the amount of empty shells has been detected here.
Concerning the SS, the empty shells accounted between 0 and 50% of all shells identified, while the mean value was 31% (Figure 4B. Overall, the soft-body preserved individuals dominated the assemblage here. Few samples displayed 100% soft-body preserved shells, however, those samples only presented 1 to 2 individuals (Figure 3C). No clear seasonal or interannual pattern in the proportion of empty shells was detected.
3.3 Magnitude, seasonality and composition of pteropod shell fluxes
The seasonality of the total and species pteropod fluxes collected by the traps differed between the GoL and the SS (Figure 3).
In the GoL, the average year derived from the eight-year record (Figure 5A) indicates that total pteropod fluxes (i.e. total flux is the sum of empty shell and shell including the soft body) exhibited maximum values between October and January and minima between May and August. In particular, October was the month with the highest fluxes (355 ind m-2 d-1, ± 152 ind m-2 d-1) followed by December (232 ind m-2 d-1, ± 9 ind m-2 d-1) and November (202 ind m-2 d-1, ± 115 ind m-2 d-1). This corresponded to 32.6%, 21.6% and 18.3% of the annual total pteropods flux, respectively. The seasonality of both soft-body preserved and empty flux followed a similar trend to that of total pteropod flux (Figure 5A). No pteropods were identified during February, April, June and July. During the months of higher fluxes, Cavoliniidae Creseis type aggrupation relative abundances were higher, however, Limacinidae aggrupation generally dominated the samples (Figure 5A). In terms of the annual flux-weighted shells fluxes collected by the trap composition, although the Limacinidae group was the most abundant, representing 47% (± 15%) of the assemblage, the Cavoliniidae Creseis type represented a similar 42% (± 18%) while the Minor taxa represented 15% (± 9%). Note that the average year higher and lower pteropod fluxes from the GoL did not display any coincidence with either chlorophyll-a or SST maximum values (Figure 5A).
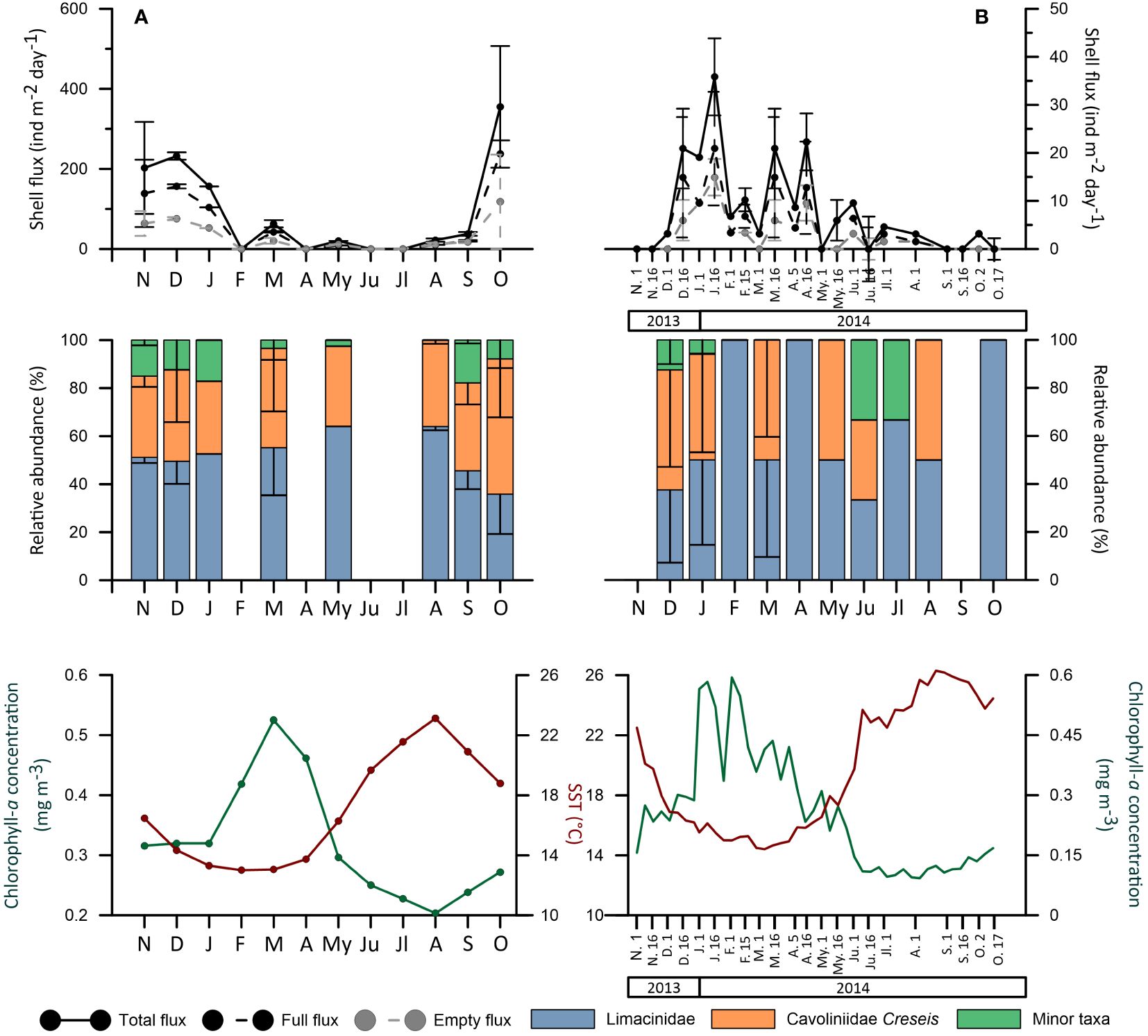
Figure 5 Seasonal total, soft-body preserved (full) and empty pteropod fluxes (ind m-2 d-1) patterns, monthly relative abundance (%) and standard deviation of the three pteropods groups and SST (°C) and chlorophyll-a (mg m-3) seasonal concentrations for (A) the GoL and (B) the SS. Note that the GoL data represents an average year while the SS data stands for the whole period of the study. For the SS fluxes, the months in which more than one flux value was available, the standard deviation was represented on the second date of the corresponding month (i.e. the standard deviation for December is shown on the 16th December).
In the SS, higher fluxes values were reached in the winter and spring periods of 2013, while the summer of 2014 displayed the lowest fluxes values (Figure 5B). Maximum fluxes were recorded between January and April and minima between August and November. The highest total shell fluxes were collected during January (55 ind m-2 d-1), April (31 ind m-2 d-1) and March (24 ind m-2 d-1). This translated into contributions of 30.5%, 15% and 13.5% of the seasonal total pteropods fluxes respectively. Both soft-body and empty shell fluxes followed a similar pattern to that of the total pteropod fluxes (Figure 5B). September and November were the only months in which no pteropods were found. In terms of species assemblage composition, the Limacinidae group dominated the populations representing 61% (± 28%) of the annual assemblage, followed by the Cavoliniidae Creseis type that accounted for a mean 32% (± 20%) and the minor taxa, which represented a mean 7% (± 12%). The only exception to this trend occurred in June and December when the three groups exhibited similar contributions to the total pteropod flux. Interestingly, higher pteropods were displayed coincidently with higher chlorophyll-a concentrations and cooler SST conditions (Figure 5B).
Note that, although the timeframe between the two studied sites differs and there is no overlap in the studied years, the total pteropod fluxes recorded in the GoL are significantly higher than the ones from the SS (Figures 5A, B), with the maximum values from each site being one order of magnitude greater in the GoL. Overall, the mean total pteropod fluxes for all the samples in which pteropods were counted were 54.5 ind m-2 d-2 for the GoL and 12.5 shells m-2 d-2 for the SS.
Finally, in the SS, higher pteropod fluxes were displayed coincidently with higher chlorophyll-a concentrations and cooler SST conditions (Figure 5B). In the GoL, higher pteropod fluxes were not specifically displayed during higher chlorophyll-a concentrations or either cool or warm SST, however, minimum pteropod fluxes and months in which no pteropods were identified (except February and April) coincided with lower chlorophyll-a values and increasing SST conditions (Figure 5A).
3.4 Interannual variability of pteropod fluxes in the Gulf of Lions
The eight-year-long sediment trap record of the Planier site generally shows annual maximum fluxes in either autumn or winter, while lower values are usually recorded during spring and summer. The maximum value was reached during autumn 2001: 182 shells m-2 d-1, although important variations in the magnitude and across years were identified. Relative maximum fluxes within the record were observed in the autumn and winter of 2001-2, 2002-3 and 2003-4 (Figure 6B). Between autumn 1998 and autumn 2001, no pteropods were found in the samples except for the summer and autumn periods of 1999 and 2000, when pulses of 9 to 11 shells m-2 d-1 fluxes were recorded. Note that higher pteropod fluxes were reached during periods when chlorophyll-a did not display any particular maximum or minimum concentration, however, generally, higher pteropod fluxes were observed during low SST conditions (Figure 6).
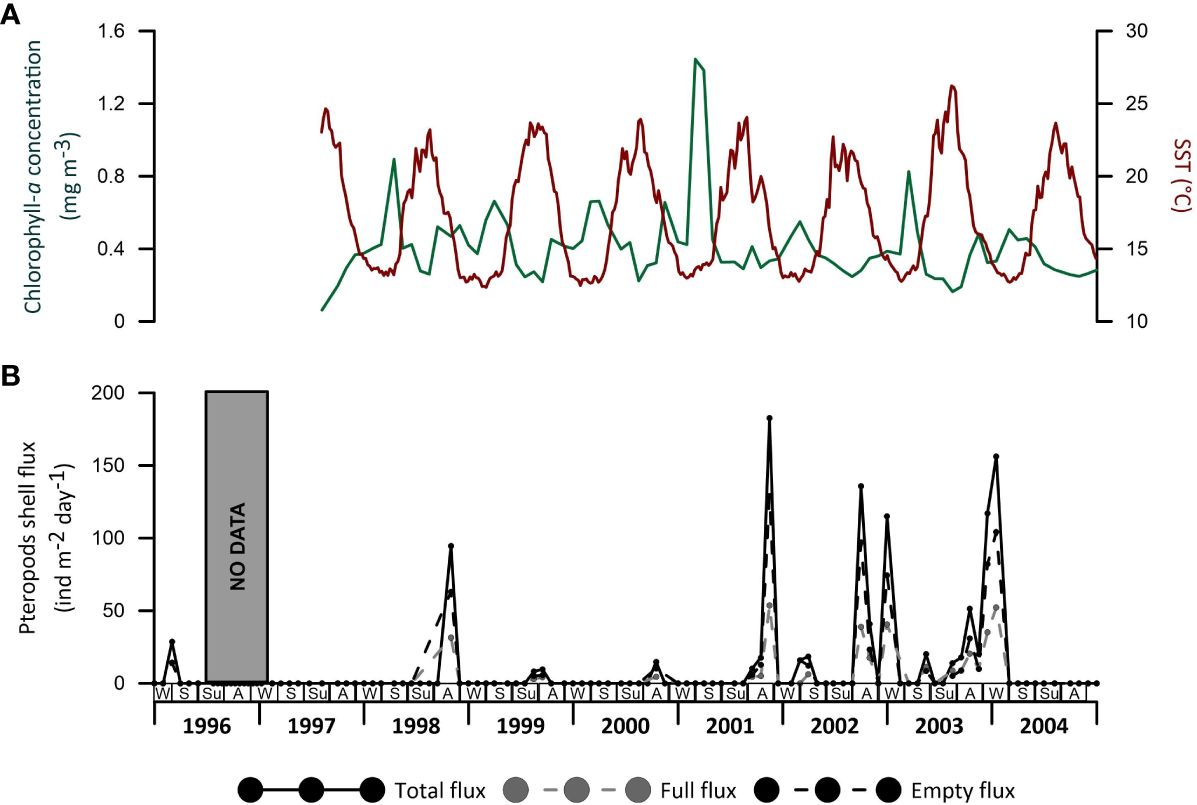
Figure 6 (A) Chlorophyll-a (mg m-3) and SST (°C) across the period studied in the GoL. (B) pteropods total, soft-body preserved (full) and empty fluxes (ind m-2 day-1) for the corresponding period.
In the GoL, cold and northern winds cool the water surface during winter, generating vertical mixing by dense shelf water cascading processes (Millot, 1990; Canals et al., 2006; Durrieu de Madron et al., 2013). This mixing recharges the surface waters with nutrients and, along with increased solar radiation, favours the spring productivity bloom (Figure 6). Increasing heat fluxes during summer cause the water column stratification and the consequent nutrient depletion, which lasts until late summer to autumn, when the cool conditions allow a secondary productivity bloom (Heussner et al., 2006; Rigual-Hernández et al., 2012).
4 Discussion
4.1 The value of sediment trap for monitoring pteropod dynamics in the Mediterranean Sea
In the Mediterranean Sea, pteropod data analyzed in sediment traps is scarce and the only study available did not focus on population dynamics but rather on the pteropod’s contribution to the carbon flux (Rutten et al., 2000). For this reason, we compare our results with previous work focused on pteropods distribution using zooplankton net (Bednaršek et al., 2012b; Rampal, 1975; Howes et al., 2015; Johnson et al., 2023). The results presented here showed that, in the GoL, the highest fluxes were recorded during autumn and the populations were dominated by L. inflata, C. virgula and C. clava (Figures 3B, 5A). In the SS, pteropod fluxes and abundance peaks were reached during spring, with L. inflata, C. virgula and C. conica being the dominant species (Figures 3C, 5B). The first work that investigated the pteropod’s distribution from a basin-wide perspective is the thesis manuscript of Rampal (1975), which showed that in the NW part of the basin, most of the pteropods appeared during the Autumn period, and the populations were mainly represented by L. inflata, C. virgula and C. clava. Despite the low number of individuals, Rampal (1975) also described that most of the pteropods were found during the winter and spring periods in the vicinity of the SS. Howes et al. (2015) work was carried out at Point B in the Ligurian Sea (43.68°N, 7.32°E) using a zooplankton time series that covered the years 1967 to 2003. This study showed that most pteropods appeared during autumn and the majority of the species identified were in agreement with our findings (i.e. S. subula, C. virgula, L. bulimoides), however, the dominant species were H. inflatus (L. inflata) for the Limacinidae type, and C. clava and C. inflexa for the Cavoliniidae type. Finally, Johnson et al. (2023) work focused on the spring distribution of pteropods and showed that the dominant species in both the western and central Mediterranean were H. inflatus (L. inflata), L. trochiformis, C. acicula (C. clava) and C. inflexa. Overall, the pteropod data presented here agrees with the previous observation carried out in the Mediterranean.
Note that no clear pattern was identified on a seasonal scale concerning the differences between empty and soft-body preserved shells (Figure 4) in addition to the fact that no studies in the vicinity of the studied zones here differentiated between these two categories, no comparison can be made about the intensity of the predation nor the amount of sinking pteropods captured by the sediment traps.
Finally, the distance of the C01 sediment trap to the seafloor is around 30m (see section 2.1). To estimate the effect of resuspended material entering into the sediment trap, we hereby approach the presence of benthic foraminifera. The most abundant species were Textularia spp. and Bulimina marginata (Béjard et al., 2024; under review). These species are considered infaunal, i.e. they live buried in the sediment and are considered abundant in continental slopes (Milker and Schmiedl, 2012; Balestra et al., 2017). Although these organisms have been identified constantly during the sampling (see Supplementary Data), they reached their maximum abundances during summer from early June to mid-September (Béjard et al., 2024; under review). On a seasonal scale, the deep circulation remains relatively stable in the Sicily Strait showing a dominance of the Levantine Intermediate Water (LIW), however, during summer, an upwelling settles in the Sicily Strait, allowing the LIW to reach shallower waters (Lermusiaux and Robinson, 2001; Béranger et al., 2004). During this period, the pteropod population showed a lower amount of specimens (Figure 3C) but the species identified and their seasonal distribution remained similar (Figures 3C, 5B). Therefore we consider that the C01 sediment trap collected resuspended material without substantially affecting the pteropod population distribution.
Then, although sediment traps are not widely used in the Mediterranean for documenting seasonal trends and composition (Rutten et al., 2000), the comparison with different datasets from surrounding regions allowed us to consider this pteropod data reliable.
4.2 Composition and seasonality of pteropod assemblages in the NW and central Mediterranean Sea
The three dominant species identified in the GoL (L. inflata, C. virgula and C. clava) accounted for around 70% of the total pteropods collected there. In the SS, the dominant species accounted for 75% of the total pteropods identified (Figure 3A).
Pteropod fluxes were one order of magnitude higher in the GoL. This difference in the magnitude of the pteropod fluxes found between the NW and the central Mediterranean agrees with recent work (Johnson et al., 2023). The latter study showed that the abundances of pteropods collected using a net during the spring were significantly more important in the western part of the basin than in the SS (although no station was located in the exact vicinity of the GoL).
L. inflata (Figure 2) contributed to 39 and 49% of the annual total pteropod fluxes identified in the GoL and the SS, respectively. It appeared mainly in autumn and winter (Figure 5A) in the GoL and during spring in the SS (Figure 5B), while it was very rare in summer in both sites (Figures 5A, B). This taxon is considered a cosmopolitan species and is one of the most common warm-water pteropods in the tropical and subtropical regions of all oceans (Burridge et al., 2017). It is also the best represented pteropod across the Mediterranean Sea (Batistic, 2004; Granata et al., 2020). Even though it has been theorized that it presents a high tolerance for low-productivity environments (Stubbings, 1936), it has also been shown that it presents an opportunistic behaviour and develops massively in upwelling regions, when nutrient and food conditions are favourable (Wormuth, 1985). As described previously, the GoL is considered one of the few non-oligotrophic regions in the Mediterranean due to the seasonal water mixing (Durrieu de Madron et al., 2005) that takes place in late winter, spring and late summer (Heussner et al., 2006) which allows bloom and increased productivity conditions. On the other hand, the SS is the sill that separates the eastern and western Mediterranean basins, therefore, it is a key point for the regional and basin-wide circulation regime. During summer, eastern basin waters dominate the circulation bringing warm and nutrient-depleted conditions, while during winter and spring, western basin waters (related to Atlantic waters) bring cool and nutrient and chlorophyll-enriched waters into the strait (Rinaldi et al., 2014; Jebri et al., 2017). The seasonal patterns suggested by the Planier and C01 traps are consistent with the observations of Rampal (1975) in the GoL and the vicinity of the SS. The latter author documented peak abundances of this species in autumn (Rampal, 1975) and attributed the decrease in the abundance during winter associated with its migration to the southern part of the Gulf. Moreover, the low fluxes of L. inflata in summer and spring during the nine-year record suggests that this species tends to avoid the regional extreme temperature conditions of the GoL. Therefore, a combination of favourable food and temperature conditions during the autumn upwelling could favour the L. inflata blooms, while the summer stratification and impoverished waters force it to migrate southward. Concerning the SS, our data showed that this taxon is more abundant during winter and spring, which is in accordance with the observations by Rampal (1975). This pattern coincides with the entrance of western cool and nutrient-enriched Atlantic waters in the strait. Overall, our data suggest that L. inflata could be associated with western basin waters in the SS, and appears preferably when food and SST conditions are favourable (similar to the GoL), which agrees with recent work (Johnson et al., 2023).
Creseis virgula (Figure 2) contributed to 17% of the annual pteropod fluxes in the GoL, while it represented 11.3% in the SS. This taxon appeared during autumn to early winter in the GoL and the SS, while its presence in spring and summer in both sites is low. C. virgula is considered a tropical to subtropical species widely distributed in the Atlantic Ocean (Burridge et al., 2017), however, few details concerning its life cycle have been studied. It tends to show some preference for deep waters and it has been described to display strong diel vertical migrations (Tarling et al., 2001; Granata et al., 2020). Rampal (1975) documented that during winter it migrates to the southern part of the NW Mediterranean basin. Based on all the above, we suggest that, in the northwestern Mediterranean, it tends to appear during a combination of enhanced food conditions and cool SST, while in summer and winter, it tends to migrate southward of the basin due to the lack of food. These observations also agree with our data from the SS, as this taxon is nearly absent during late spring and summer when eastern Mediterranean warm and oligotrophic wasters dominate the circulation in the strait. However, these results contrast with previous work that linked this taxon to warm SST in tropical to subtropical environments.
Creseis clava (Figure 2) represented 13.5% of the annual pteropod fluxes in the GoL, while, in the SS, it still represented 6.8% of the seasonal flux. It appears mainly in autumn (Figure 3) in the GoL, while it only appears in winter and spring in the SS, although its abundance was lower. Regarding its ecology, C. clava has been described as a cosmopolitan species (Wall-Palmer et al., 2014), however, studies that focus on its life cycle are scarce, therefore it is difficult to establish its ecological preferences. It tends to prefer warm waters (Albergoni, 1975), it has been reported as a typical upwelling taxon (Thiede and Jünger, 1992) and recent blooms of this species have been described in the South China Sea and linked to enhanced chlorophyll-a and lower salinity conditions (Dai et al., 2020). It has also been documented as abundant in subtropical gyres in the Atlantic Ocean (Burridge et al., 2017). Regarding the Mediterranean basin, Rampal (1975) described that this species appears to follow an “invasion” strategy larvae reach the adult age around mid-October and dominate the assemblages until the end of November when it nearly disappears (Rampal, 1975). Our data agrees well with the upwelling/invasion strategy, as its maximum fluxes were recorded during two months from October to late December, which coincided with favourable food conditions. It also agrees with the quick disappearance strategy, as it is nearly absent during late winter and summer. We suggest that the food scarcity and the cool SST could force it to migrate southward.
Finally, C. conica contributed to around 12% and a non-negligible 6.5% of the annual total pteropod fluxes from the SS and the GoL, respectively. In the SS, it appeared mainly during winter and early spring. Details about its life cycle are scarce, but it has been described as a subtropical taxon with a high tolerance for low-production environments. In the SS, maximum stocks of this species were observed during relatively cool SSTs.
Limacina inflata, C. virgula and C. clava tend to appear together and dominate the assemblages in the western Mediterranean, while, in the central Mediterranean, C. clava is replaced by C. conica. These observations agree with previous work carried out in the same regions (Rampal, 1975; Johnson et al., 2023). Here we propose that these trends are likely associated with the taxa-specific life cycle in addition to favourable environmental conditions. Our data showed that enhanced chlorophyll-a concentrations seem to affect positively all the dominant species in both the GoL and the SS on a seasonal scale. In contrast, the highest abundances of the latter species were observed during cool SST conditions, which are displayed coincidently with upwelling conditions in the GoL. This is an unexpected trend taking into account that the main three taxa are considered to be tropical to sub-tropical species. Therefore, here we theorise that, on a seasonal scale, food availability may play a major role in pteropod population dynamics in the NW and Central Mediterranean. In addition, the combined dominance of L. inflata and Creseis type taxons is also considered widespread in the Mediterranean (Rampal, 1975), especially in the easternmost part of the basin (Almogi-Labin et al., 1986; Violanti et al., 1991).
4.3 Environmental controls on the interannual distribution of pteropod fluxes in the NW Mediterranean
The interannual and geographical distributions of pteropods have been compared with a wide array of environmental parameters (Manno et al., 2007; Comeau et al., 2009; Ohman et al., 2009; Howard et al., 2011; Johnson et al., 2023, 2017; Roberts et al., 2014). Generally, their abundance is positively linked with enhanced food and nutrient supply, aragonite state saturation, and salinity. Conversely, high CO2 concentration, extreme (high and low) SSTs and acid pH conditions have proved to affect negatively the pteropod populations (Comeau et al., 2009; Lischka et al., 2011; Busch et al., 2014).
The SST is well documented as one of the main factors affecting the zooplankton distribution, however, their correlation with this parameter tends to be unpredictable and complex (Richardson, 2008). Previous work in the Mediterranean (Howes et al., 2015) and in the North Atlantic (Beaugrand et al., 2012) identified temperature as one of the main drivers of pteropods distributions, and, depending on the taxons, higher SSTs generally being linked to greater pteropods abundances. Surprisingly, the SST data displayed no clear correlation with the pteropods fluxes in the GoL (Figure 6 and Table 1), while in the SS (on a seasonal scale), higher fluxes were displayed during lower SSTs, highlighting a potential positive effect of cool conditions on pteropods fluxes (Figure 7). The seasonal amplitude of SST in the Mediterranean Sea is about 10°C, which are variations likely to be experienced by all of the pteropod population during their life cycle. Taking into account the rapid warming (an increase of 1.5-2°C by the end of the century) of the Mediterranean (Lazzari et al., 2014), recent SST trends effects on pteropod populations should be investigated in further studies. Based on all the above, we speculate that future trends regarding SST could affect the species diversity and accentuate the dominance of subtropical and tropical and low-productivity adapted taxons such as L. inflata, C. virgula or C. clava.
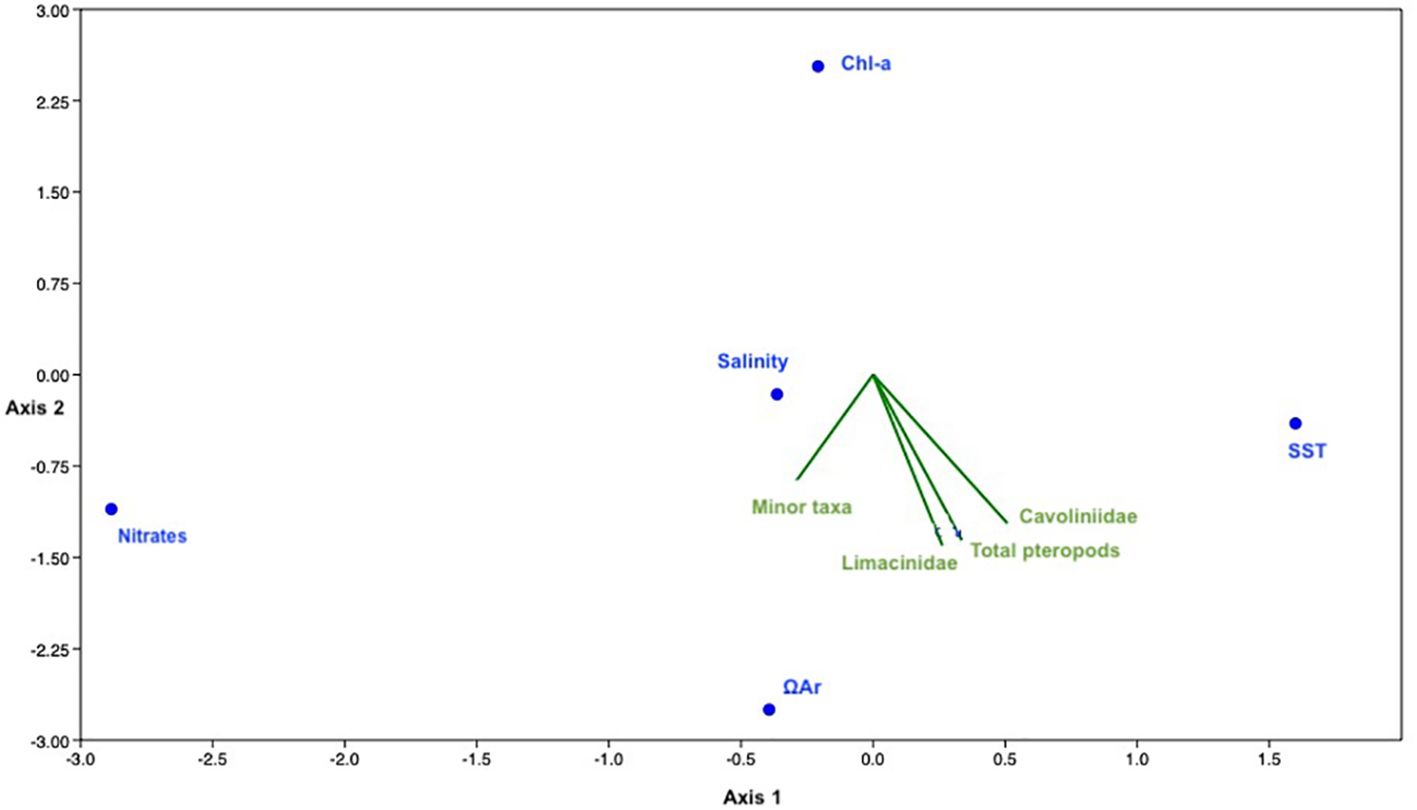
Figure 7 Detrended CCA for the 3 pteropod aggrupations fluxes, the satellite data [chlorophyll-a and SST (°C)] and the DYFAMED site data (nitrates concentration (μmol/kgSW), salinity (PSU) and ΩAr). The score and eigenvalues can be found in the Supplementary Data.
Therefore, as the previous two parameters did not display any significant correlation with the GoL pteropod (Figure 7), other parameters than the ones considered here affect the pteropod distribution in the GoL (Table 1).
Salinity data from the DYFAMED site displayed values between 38.2 and 38.5 PSU, with very small seasonal variations. These values are considered within a normally accepted range for pteropod communities (Han et al., 2022). In our study, salinity showed a negative correlation with all pteropod fluxes (Table 1). This is an unexpected trend as only very low salinities have been shown to negatively affect pteropods distributions. However, this data is in accordance with previous work in the Mediterranean (Johnson et al., 2023) that found C. clava (among other species) to be negatively correlated to salinity.
As the pteropod shell is made of aragonite, a metastable form of calcite (Mucci et al., 1989), variations in the aragonite saturation state (Ωar) can affect their calcification (Roger et al., 2012) and abundance (Mekkes et al., 2021). Numerous studies have positively linked the Ωar with pteropods distributions, abundances and shell thickness in different parts of the global ocean (Singh et al., 2005; Comeau et al., 2009; Roger et al., 2012; Bednaršek et al., 2014; Moya et al., 2016; Howes et al., 2017; Manno et al., 2019). Overall, the Mediterranean is considered to be supersaturated regarding aragonite (Álvarez et al., 2014), with values of 2.9-3.7 in the GoL, therefore, the positive and significant correlation (Table 1) between the pteropod fluxes and Ωar highlights the possible impact of this parameter on pteropod populations here (Figure 7).
In addition to the previously mentioned environmental parameters, biological behaviour can also affect pteropod distribution on interannual patterns. Factors outside of the scope of this study such as vertical migration linked to reproduction (Lalli and Gilmer, 1989; Willis et al., 2006) or trophic regimes can also affect substantially pteropods population dynamics (Maas et al., 2012).
Finally, although no clear pattern was identified when comparing empty and soft-body preserved shells (Figure 4), a change in the trophic regimes and the predation intensity could also play a role on an interannual scale in the GoL.
4.4 Soft-body and empty shells as a pteropod mortality indicator
Generally, soft-body preserved individuals are considered carcasses, while empty shells are considered dead individuals. Also, pteropods are involved in the carbon export from the surface to the deeper ocean. On the one hand, their shells contribute to the particulate inorganic carbon (PIC) flux, on the other hand, their soft-body/cytoplasm contributes to the particulate organic carbon (POC) flux (Heinze et al., 1991). These two transfer mechanisms result in a pteropod contribution to both the biological carbon pump that reduces the atmospheric CO2 due to the production of organic matter and its subsequent export to the deep ocean; and the carbonate counter pump that increases surface ocean CO2 and counteracts the carbon export to the deep ocean. Therefore, and as described previously, separating between soft-body preserved and empty pteropod shells is crucial and the biogeochemical importance of the aragonite produced by pteropods has been studied on both global and regional scales (Bednaršek et al., 2014; Manno et al., 2018, 2019; Tyrrell, 2007). In our study, soft-body preserved individuals were dominant in both studied sites, however, empty shells were non-negligible and accounted for a mean 38% of the total individuals in the GoL and 31% in the SS (Figure 4). As identifying the mortality cause of zooplankton populations is difficult, especially in this part of the ocean where similar data to compare our results is non-existent, we hereby speculate about the possible parameters that may affect the mortality of pteropods.
Firstly, the amount of empty shells can be discussed as the result of the environmental conditions of the water column. In the GoL, a higher amount of carcasses was identified during March 1996, May 2003 and August 2003 (Figure 4), which coincided with low concentrations of chlorophyll-a values) and relatively cool SST conditions (Figure 6). Our seasonal correlation data (see Supplementary Data) showed that empty carcasses are negatively correlated with the chlorophyll-a concentration (r= -0.77, p< 0.05). This can be interpreted as a negative effect of favourable food conditions on pteropod mortality. However, no such effect of SST on empty pteropods was observed. In addition, parameters discussed previously such as aragonite saturation state (2.9-3.7) also displayed a positive correlation with the empty shells, but also with the soft-body preserved individuals, similar to the environmental effect on the pteropod fluxes (Table 1). Note that, the link between carbonate state saturation, SST and food availability with the pteropod mortality has not been yet described in an open environment (Gazeau et al., 2013). In the SS, 4 samples showed a 50% of empty shells (Figure 4). Even though the time interval studied differed, a higher proportion of carcasses (Figure 4) were identified coincidently with higher chlorophyll-a concentrations (Figure 6) and cool SST conditions. Correlations with these two parameters (see Supplementary Data) showed that empty pteropods correlated positively and significantly with the chlorophyll-a (r= 0.58, p< 0.05) but negatively with the SST (r=-0.48, p> 0.05). Here we speculate that in the SS, as the favourable food conditions are associated with the cold Atlantic waters coming into the SS (Béranger et al., 2004), they play a negative role in the pteropod survival. This is not surprising as, in the Mediterranean, a greater diversity and overall pteropod abundance has been described in the eastern basins (Johnson et al., 2023).
Secondly, the proportion of empty shells can also be discussed as the result of trophic regimes such as successful attacks by their main predator, the previously mentioned gymnosomata C. limacine. Interestingly, no individuals of the latter species were identified in our samples, which, in this case, is in accordance with the soft-body preserved individuals dominance.
Overall, our results are in contrast with the ones from Zamelczyk et al. (2021) work, in which the empty shells dominated the pteropod assemblages. Although the latter study was carried out in the Barents Sea, in a seasonally ice-covered environment, our work agrees with the negative effect of cool SST and a positive effect of food availability on the pteropod survival.
5 Conclusion
The analyses of Planier and C01 sediment traps samples allowed us to document thecosome pteropod seasonal variability in both the Gulf of Lions (NW Mediterranean) and the Sicily Strait (Central Mediterranean) during the years 1996 to 2004 and 2013 to 2014 respectively.
A total of 843 pteropod individuals were counted and 18 different species were identified. L. inflata, C. virgula were the two most abundant taxa, while C. clava and C. conica were the third most abundant ones in the Gulf of Lions and the Sicily Strait, respectively. These species represented around 70% of the total individuals identified in both sites. Overall, the pteropods species were similar between the two studied sites. In the Gulf of Lions, maximum fluxes occurred while in the Sicily Strait peak fluxes occurred in winter. In both sites, summer was the period that showed minimum pteropods occurrences. Total pteropod fluxes were significantly higher in the Gulf of Lions than in the Sicily Strait: with mean seasonal values of 54.5 and 12.5 ind m-2 d-1 and the maximum values being one order of magnitude greater in the NW Mediterranean.
Although the use of sediment traps to document pteropod variability is not widely used, the comparison of our seasonal and interannual trends agrees with previous work in the Mediterranean. In the SS, higher pteropod fluxes were displayed during high chlorophyll-a and low SST conditions, showing the possible impact of favourable food conditions on pteropod distributions. In the GoL, the relationship with these parameters was not straightforward, highlighting the potential impact of parameters such as salinity and Ωar. Finally, the comparison between empty and soft-body preserved pteropod shells showed a dominance of the latter, highlighting the effect of factors such as the SST and the food availability on the pteropod population survival.
As pteropods represent an important component of marine ecosystems and biogeochemical cycles, documenting their distribution across seasonal and interannual scales is relevant. Our results call for increasing the monitoring of pteropod populations in the Mediterranean Sea to improve our understanding of the potential impact on these organisms of rapid environmental change.
Data availability statement
The original contributions presented in the study are included in the article/Supplementary Material, further inquiries can be directed to the corresponding author.
Ethics statement
The manuscript presents research on animals that do not require ethical approval for their study.
Author contributions
TB: Conceptualization, Formal analysis, Investigation, Methodology, Writing – original draft, Writing – review & editing. CM: Conceptualization, Data curation, Investigation, Methodology, Resources, Supervision, Validation, Writing – review & editing. FS: Funding acquisition, Project administration, Supervision, Validation, Writing – review & editing. JF: Funding acquisition, Project administration, Resources, Supervision, Validation, Writing – review & editing. JP: Data curation, Formal analysis, Software, Visualization, Writing – review & editing. XD: Resources, Supervision, Validation, Writing – review & editing. AS-V: Funding acquisition, Methodology, Resources, Supervision, Validation, Writing – review & editing. AR: Conceptualization, Data curation, Investigation, Methodology, Project administration, Supervision, Validation, Writing – review & editing.
Funding
The author(s) declare financial support was received for the research, authorship, and/or publication of this article. This research has been supported by the Ministerio de Ciencia e Innovación (grant nos. RTI2018-099489-B-100, PID2021-128322NB-100, and PRE2019-089091). This project has received funding from the project BASELINE (grant no. PID2021- 126495NB-741 C33) granted by the Spanish Ministry of Science, Innovation and Universities (Andrés S. Rigual-Hernández). This research has also received funding from the JERICO project under the FP7 contract agreement n&z.ousco; 262584 and supported by ISMAR, CNR. ASV acknowledges the financial support by the Catalan Government Grups de Recerca Consolidats grant (2021 SGR 01195).
Acknowledgments
The authors would like to thank Serge Heussner for the retrieval of the sediment trap collected within the French MOOSE program 20 (Mediterranean Ocean Observing System for the Environment) coordinated by CNRS-INSU and the Research Infrastructure ILICO (CNRS-IFREMER).
Conflict of interest
The authors declare that the research was conducted in the absence of any commercial or financial relationships that could be construed as a potential conflict of interest.
Publisher’s note
All claims expressed in this article are solely those of the authors and do not necessarily represent those of their affiliated organizations, or those of the publisher, the editors and the reviewers. Any product that may be evaluated in this article, or claim that may be made by its manufacturer, is not guaranteed or endorsed by the publisher.
Supplementary material
The Supplementary Material for this article can be found online at: https://www.frontiersin.org/articles/10.3389/fmars.2024.1346651/full#supplementary-material.
References
Albergoni A. (1975). Addensamento improvviso di Creseis acicula (Rang 1828) (Gastropoda, pteropoda) in una baia del Mare Ligure. Conchiglie 11, 233–236.
Aldridge D., Beer C. J., Purdie D. A. (2012). Calcification in the planktonic foraminifera Globigerina bulloides linked to phosphate concentrations in surface waters of the North Atlantic Ocean. Biogeosciences 9, 1725–1739. doi: 10.5194/bg-9-1725-2012
Almogi-Labin A., Hemleben Ch., Deuser W. G. (1988). Seasonal variation in the flux of euthecosomatous pteropods collected in a deep sediment trap in the Sargasso Sea. Deep Sea Res. Part A. Oceanographic Res. Papers 35, 441–464. doi: 10.1016/0198-0149(88)90020-9
Almogi-Labin A., Luz B., Duplessy J.-C. (1986). Quaternary paleo-oceanography, pteropod preservation and stable-isotope record of the Red Sea. Palaeogeography Palaeoclimatology Palaeoecol. 57, 195–211. doi: 10.1016/0031-0182(86)90013-1
Anglada-Ortiz G., Meilland J., Ziveri P., Chierici M., Fransson A., Jones E., et al. (2023). Seasonality of marine calcifiers in the northern Barents Sea: Spatiotemporal distribution of planktonic foraminifers and shelled pteropods and their contribution to carbon dynamics. Prog. Oceanography 218, 103121. doi: 10.1016/j.pocean.2023.103121
Anglada-Ortiz G., Zamelczyk K., Meilland J., Ziveri P., Chierici M., Fransson A., et al. (2021). Planktic foraminiferal and pteropod contributions to carbon dynamics in the Arctic ocean (North svalbard margin). Front. Mar. Sci. 8. doi: 10.3389/fmars.2021.661158
Avnaim-Katav S., Herut B., Rahav E., Katz T., Weinstein Y., Alkalay R., et al. (2020). Sediment trap and deep sea coretop sediments as tracers of recent changes in planktonic foraminifera assemblages in the southeastern ultra-oligotrophic Levantine Basin. Deep Sea Res. Part II: Topical Stud. Oceanography 171, 104669. doi: 10.1016/j.dsr2.2019.104669
Aydin K. Y., McFarlane G. A., King J. R., Megrey B. A., Myers K. W. (2005). Linking oceanic food webs to coastal production and growth rates of Pacific salmon (Oncorhynchus spp.), using models on three scales. Deep Sea Res. Part II: Topical Stud. Oceanography 52, 757–780. doi: 10.1016/j.dsr2.2004.12.017
Álvarez M., Sanleón-Bartolomé H., Tanhua T., Mintrop L., Luchetta A., Cantoni C., et al. (2014). The CO2 system in the Mediterranean Sea: a basin wide perspective. Ocean Sci. 10, 69–92. doi: 10.5194/os-10-69-2014
Balestra B., Grunert P., Ausin B., Hodell D., Flores J.-A., Alvarez-Zarikian C. A., et al. (2017). Coccolithophore and benthic foraminifera distribution patterns in the gulf of cadiz and western iberian margin during integrated ocean drilling program (IODP) expedition 339. J. Mar. Syst. 170, 50–67. doi: 10.1016/j.jmarsys.2017.01.005
Batistic M. (2004). Gelatinous invertebrate zooplankton of the South Adriatic: species composition and vertical distribution. J. Plankton Res. 26, 459–474. doi: 10.1093/plankt/fbh043
Bé A. W. H., Gilmer R. W. (1977). A zoogeographic and taxonomic review of euthecosomatous Pteropoda. Oceanic Micropaleontology 1, 733–808.
Beare D., McQuatters-Gollop A., van der Hammen T., Machiels M., Teoh S. J., Hall-Spencer J. M. (2013). Long-term trends in calcifying plankton and pH in the North Sea. PloS One 8, e61175. doi: 10.1371/journal.pone.0061175
Beaugrand G., McQuatters-Gollop A., Edwards M., Goberville E. (2012). Long-term responses of North Atlantic calcifying plankton to climate change. Nat. Clim Change 3, 263–267. doi: 10.1038/nclimate1753
Beccari V., Almogi-Labin A., Basso D., Panieri G., Makovsky Y., Hajdas I., et al. (2023). Late Holocene pteropod distribution across the base of the south-eastern Mediterranean margin: the importance of the > 63 µm fraction. J. Micropalaeontol. 42, 13–29. doi: 10.5194/jm-42-13-2023
Bednaršek N., Harvey C. J., Kaplan I. C., Feely R. A., Možina J. (2016). Pteropods on the edge: Cumulative effects of ocean acidification, warming, and deoxygenation. Prog. Oceanography 145, 1–24. doi: 10.1016/j.pocean.2016.04.002
Bednaršek N., Možina J., Vogt M., O’Brien C., Tarling G. A. (2012a). The global distribution of pteropods and their contribution to carbonate and carbon biomass in the modern ocean. Earth Syst. Sci. Data 4, 167–186. doi: 10.5194/essd-4-167-2012
Bednaršek N., Tarling G. A., Bakker D. C. E., Fielding S., Feely R. A. (2014). Dissolution dominating calcification process in polar pteropods close to the point of aragonite undersaturation. PloS One 9, e109183. doi: 10.1371/journal.pone.0109183
Bednaršek N., Tarling G. A., Fielding S., Bakker D. C. E. (2012b). Population dynamics and biogeochemical significance of Limacina helicina Antarctica in the Scotia Sea (Southern Ocean). Deep Sea Res. Part II: Topical Stud. Oceanography 59–60, 105–116. doi: 10.1016/j.dsr2.2011.08.003
Béjard T. M., Rigual-Hernández A. S., Tarruella J. P., Flores J. A., Sanchez Vidal A., Llamas Cano I., et al. (2024). Planktic foraminifera assemblage composition and flux dynamics inferred from an annual sediment trap record in the Central Mediterranean Sea. Biodiversity Ecosystem Function: Mar. doi: 10.5194/egusphere-2023-3101
Béranger K., Mortier L., Gasparini G.-P., Gervasio L., Astraldi M., Crépon M. (2004). The dynamics of the Sicily Strait: a comprehensive study from observations and models. Deep Sea Res. Part II: Topical Stud. Oceanography 51, 411–440. doi: 10.1016/j.dsr2.2003.08.004
Berner R. A., Honjo S. (1981). Pelagic sedimentation of aragonite: its geochemical significance. Science 211, 940–942. doi: 10.1126/science.211.4485.940
Bethoux J. P., Gentili B., Morin P., Nicolas E., Pierre C., Ruiz-Pino D. (1999). The Mediterranean Sea: a miniature ocean for climatic and environmental studies and a key for the climatic functioning of the North Atlantic. Prog. Oceanography 44, 131–146. doi: 10.1016/S0079-6611(99)00023-3
Böer M., Gannefors C., Kattner G., Graeve M., Hop H., Falk-Petersen S. (2005). The Arctic pteropod Clione limacina: seasonal lipid dynamics and life-strategy. Mar. Biol. 147, 707–717. doi: 10.1007/s00227-005-1607-8
Boltovskoy D., Oberhänsli H., Wefer G. (1996). Radiolarian assemblages in the eastern tropical atlantic: patterns in the plankton and in sediment trap samples. J. Mar. Syst. 8, 31–51. doi: 10.1016/0924-7963(95)00038-0
Buesseler K. O., Antia A. N., Chen M., Fowler S. W., Gardner W. D., Gustafsson O., et al. (2007). An assessment of the use of sediment traps for estimating upper ocean particle fluxes. J. Mar. Res. 65, 345–416. doi: 10.1357/002224007781567621
Burridge A. K., Goetze E., Wall-Palmer D., Le Double S. L., Huisman J., Peijnenburg K. T. C. A. (2017). Diversity and abundance of pteropods and heteropods along a latitudinal gradient across the Atlantic Ocean. Prog. Oceanography 158, 213–223. doi: 10.1016/j.pocean.2016.10.001
Busch D. S., Maher M., Thibodeau P., McElhany P. (2014). Shell condition and survival of puget sound pteropods are impaired by ocean acidification conditions. PloS One 9, e105884. doi: 10.1371/journal.pone.0105884
Canals M., Puig P., De Madron X. D., Heussner S., Palanques A., Fabres J. (2006). Flushing submarine canyons. Nature 444, 354–357. doi: 10.1038/nature05271
Chernihovsky N., Torfstein A., Almogi-Labin A. (2018). Seasonal flux patterns of planktonic foraminifera in a deep, oligotrophic, marginal sea: Sediment trap time series from the Gulf of Aqaba, northern Red Sea. Deep Sea Res. Part I: Oceanographic Res. Papers 140, 78–94. doi: 10.1016/j.dsr.2018.08.003
Comeau S., Gorsky G., Jeffree R., Teyssié J.-L., Gattuso J.-P. (2009). Impact of ocean acidification on a key Arctic pelagic mollusc (Limacina helicina). Biogeosciences 6, 1877–1882. doi: 10.5194/bg-6-1877-2009
Coppola L., Diamond Riquier E., Carval T., Irisson J.-O., Desnos C. (2023). Dyfamed observatory data. doi: 10.17882/43749
Coppola L., Raimbault P., Mortier L., Testor P. (2019). Monitoring the environment in the Northwestern Mediterranean Sea. Eos 100, 10. doi: 10.1029/2019EO125951
Dai M., Qi Z., Zeng L., Zhang S., Wang L., Qin X., et al. (2020). An unprecedented outbreak of pelagic molluscs Creseis acicula in Daya Bay, South China Sea. Authorea. doi: 10.22541/au.159809490.05151881
Durrieu de Madron X., Zervakis V., Theocharis A., Georgopoulos D. (2005). Comments on “Cascades of dense water around the world ocean.”. Prog. Oceanography 64, 83–90. doi: 10.1016/j.pocean.2004.08.004
Durrieu de Madron X., Houpert L., Puig P., Sanchez-Vidal A., Testor P., Bosse A., et al. (2013). Interaction of dense shelf water cascading and open-sea convection in the northwestern mediterranean during winter 2012: Shelf cascading and open-sea convection. Geophys. Res. Lett. 40, 1379–1385. doi: 10.1002/grl.50331
Eguchi N. O., Kawahata H., Taira A. (1999). Seasonal response of planktonic foraminifera to surface ocen condition: sediment trap results from the central north pacific ocean. J. Oceanography 55, 681–691. doi: 10.1023/A:1007812028703
Fernández De Puelles M. L., Alemany F., Jansá J. (2007). Zooplankton time-series in the Balearic Sea (Western Mediterranean): Variability during the decade 1994–2003. Prog. Oceanography 74, 329–354. doi: 10.1016/j.pocean.2007.04.009
Forbes J. R., Macdonald R. W., Carmack E. C., Iseki K., O’Brien M. C. (1992). Zooplankton Retained in Sequential Sediment Traps along the Beaufort Sea Shelf Break during Winter. Can. J. Fish. Aquat. Sci. 49, 663–670. doi: 10.1139/f92-075
Gazeau F., Parker L. M., Comeau S., Gattuso J.-P., O’Connor W. A., Martin S., et al. (2013). Impacts of ocean acidification on marine shelled molluscs. Mar. Biol. 160, 2207–2245. doi: 10.1007/s00227-013-2219-3
Granata A., Bergamasco A., Battaglia P., Milisenda G., Pansera M., Bonanzinga V., et al. (2020). Vertical distribution and diel migration of zooplankton and micronekton in Polcevera submarine canyon of the Ligurian mesopelagic zone (NW Mediterranean Sea). Prog. Oceanography 183, 102298. doi: 10.1016/j.pocean.2020.102298
Grossman E. L., Betzer P. R., Dudley W. C., Dunbar R. B. (1986). Stable isotopic variation in pteropods and atlantids from north pacific sediment traps. Mar. Micropaleontology 10, 9–22. doi: 10.1016/0377-8398(86)90022-8
Hamner W. M., Madin L. P., Alldredge A. L., Gilmer R. W., Hamner P. P. (1975). Underwater observations of gelatinous zooplankton: Sampling problems, feeding biology, and behavior1: Gelatinous zooplankton. Limnol. Oceanogr. 20, 907–917. doi: 10.4319/lo.1975.20.6.0907
Han T., Qi Z., Shi R., Liu Q., Dai M., Huang H. (2022). Effects of seawater temperature and salinity on physiological performances of swimming shelled pteropod creseis acicula during a bloom period. Front. Mar. Sci. 9. doi: 10.3389/fmars.2022.806848
Hassoun A. E. R., Bantelman A., Canu D., Comeau S., Galdies C., Gattuso J.-P., et al. (2022). Ocean acidification research in the Mediterranean Sea: Status, trends and next steps. Front. Mar. Sci. 9. doi: 10.3389/fmars.2022.892670
Hassoun A. E. R., Gemayel E., Krasakopoulou E., Goyet C., Abboud-Abi Saab M., Guglielmi V., et al. (2015). Acidification of the Mediterranean Sea from anthropogenic carbon penetration. Deep Sea Res. Part I: Oceanographic Res. Papers 102, 1–15. doi: 10.1016/j.dsr.2015.04.005
Heinze C., Maier-Reimer E., Winn K. (1991). Glacial pCO2 reduction by the world ocean: experiments with the hamburg carbon cycle model. Paleoceanography 6, 395–430. doi: 10.1029/91PA00489
Hernández-Almeida I., Bárcena M. A., Flores J. A., Sierro F. J., Sanchez-Vidal A., Calafat A. (2011). Microplankton response to environmental conditions in the Alboran Sea (Western Mediterranean): One year sediment trap record. Mar. Micropaleontology 78, 14–24. doi: 10.1016/j.marmicro.2010.09.005
Heussner S., Durrieu de Madron X., Calafat A., Canals M., Carbonne J., Delsaut N., et al. (2006). Spatial and temporal variability of downward particle fluxes on a continental slope: Lessons from an 8-yr experiment in the Gulf of Lions (NW Mediterranean). Mar. Geology 234, 63–92. doi: 10.1016/j.margeo.2006.09.003
Heussner S., Ratti C., Carbonne J. (1990). The PPS 3 time-series sediment trap and the trap sample processing techniques used during the ECOMARGE experiment. Continental Shelf Res. 10, 943–958. doi: 10.1016/0278-4343(90)90069-X
Houpert L., Durrieu de Madron X., Testor P., Bosse A., D’Ortenzio F., Bouin M. N., et al. (2016). Observations of open-ocean deep convection in the northwestern Mediterranean Sea: Seasonal and interannual variability of mixing and deep water masses for the 2007-2013 Period: DEEP CONVECTION OBS. NWMED 2007-2013. J. Geophys. Res. Oceans 121, 8139–8171. doi: 10.1002/jgrc.v121.11
Howard W. R., Roberts D., Moy A. D., Lindsay M. C. M., Hopcroft R. R., Trull T. W., et al. (2011). Distribution, abundance and seasonal flux of pteropods in the Sub-Antarctic Zone. Deep Sea Res. Part II: Topical Stud. Oceanography 58, 2293–2300. doi: 10.1016/j.dsr2.2011.05.031
Howes E. L., Eagle R. A., Gattuso J.-P., Bijma J. (2017). Comparison of mediterranean pteropod shell biometrics and ultrastructure from historical, (1910 and 1921) and present day, (2012) samples provides baseline for monitoring effects of global change. PloS One 12, e0167891. doi: 10.1371/journal.pone.0167891
Howes E., Stemmann L., Assailly C., Irisson J., Dima M., Bijma J., et al. (2015). Pteropod time series from the North Western Mediterranean, (1967-2003): impacts of pH and climate variability. Mar. Ecol. Prog. Ser. 531, 193–206. doi: 10.3354/meps11322
Huertas I. E., Ríos A. F., García-Lafuente J., Navarro G., Makaoui A., Sánchez-Román A., et al. (2012). Atlantic forcing of the Mediterranean oligotrophy: Atlantic forcing of the Mediterranean oligotrophy. Global Biogeochem. Cycles 26 (2). doi: 10.1029/2011GB004167
Hunt B., Strugnell J., Bednarsek N., Linse K., Nelson R. J., Pakhomov E., et al. (2010). Poles apart: the “Bipolar” Pteropod species limacina helicina is genetically distinct between the arctic and Antarctic Oceans. PloS One 5, e9835. doi: 10.1371/journal.pone.0009835
Jebri F., Zakardjian B., Birol F., Bouffard J., Jullion L., Sammari C. (2017). Interannual variations of surface currents and transports in the sicily channel derived from coastal altimetry. JGR Oceans 122, 8330–8353. doi: 10.1002/2017JC012836
Johnson R., Manno C., Ziveri P. (2023). Shelled pteropod abundance and distribution across the Mediterranean Sea during spring. Prog. Oceanography 210, 102930. doi: 10.1016/j.pocean.2022.102930
Jonkers L., Hillebrand H., Kucera M. (2019). Global change drives modern plankton communities away from the pre-industrial state. Nature 570, 372–375. doi: 10.1038/s41586-019-1230-3
Kessouri F., Ulses C., Estournel C., Marsaleix P., D’Ortenzio F., Severin T., et al. (2018). Vertical mixing effects on phytoplankton dynamics and organic carbon export in the Western Mediterranean Sea. JGR Oceans 123, 1647–1669. doi: 10.1002/2016JC012669
Knauer G. A., Martin J. H., Bruland K. W. (1979). Fluxes of particulate carbon, nitrogen, and phosphorus in the upper water column of the northeast Pacific. Deep Sea Res. Part A. Oceanographic Res. Papers 26, 97–108. doi: 10.1016/0198-0149(79)90089-X
Krom M. D., Woodward E. M. S., Herut B., Kress N., Carbo P., Mantoura R. F. C., et al. (2005). Nutrient cycling in the south east Levantine basin of the eastern Mediterranean: Results from a phosphorus starved system. Deep Sea Res. Part II: Topical Stud. Oceanography 52, 2879–2896. doi: 10.1016/j.dsr2.2005.08.009
Lalli C. M., Gilmer R. W. (1989). Pelagic snails: the biology of holoplanktonic gastropod mollusks. California: Stanford University Press. doi: 10.1515/9781503623088
Lazzari P., Mattia G., Solidoro C., Salon S., Crise A., Zavatarelli M., et al. (2014). The impacts of climate change and environmental management policies on the trophic regimes in the Mediterranean Sea: Scenario analyses. J. Mar. Syst. 135, 137–149. doi: 10.1016/j.jmarsys.2013.06.005
Lermusiaux P. F. J., Robinson A. R. (2001). Features of dominant mesoscale variability, circulation patterns and dynamics in the Strait of Sicily. Deep Sea Res. Part I: Oceanographic Res. Papers 48, 1953–1997. doi: 10.1016/S0967-0637(00)00114-X
Lischka S., Büdenbender J., Boxhammer T., Riebesell U. (2011). Impact of ocean acidification and elevated temperatures on early juveniles of the polar shelled pteropod Limacina helicina mortality, shell degradation, and shell growth. Biogeosciences 8, 919–932. doi: 10.5194/bg-8-919-2011
Loeb V. J., Santora J. A. (2013). Pteropods and climate off the Antarctic Peninsula. Prog. Oceanography 116, 31–48. doi: 10.1016/j.pocean.2013.05.030
Maas A. E., Wishner K. F., Seibel B. A. (2012). Metabolic suppression in thecosomatous pteropods as an effect of low temperature and hypoxia in the eastern tropical North Pacific. Mar. Biol. 159, 1955–1967. doi: 10.1007/s00227-012-1982-x
Makabe R., Hattori H., Sampei M., Darnis G., Fortier L., Sasaki H. (2016). Can sediment trap-collected zooplankton be used for ecological studies? Polar Biol. 39, 2335–2346. doi: 10.1007/s00300-016-1900-7
Manno C., Bednaršek N., Tarling G. A., Peck V. L., Comeau S., Adhikari D., et al. (2017). Shelled pteropods in peril: Assessing vulnerability in a high CO2 ocean. Earth-Science Rev. 169, 132–145. doi: 10.1016/j.earscirev.2017.04.005
Manno C., Giglio F., Stowasser G., Fielding S., Enderlein P., Tarling G. A. (2018). Threatened species drive the strength of the carbonate pump in the northern Scotia Sea. Nat. Commun. 9, 4592. doi: 10.1038/s41467-018-07088-y
Manno C., Rumolo P., Barra M., d’Albero S., Basilone G., Genovese S., et al. (2019). Condition of pteropod shells near a volcanic CO2 vent region. Mar. Environ. Res. 143, 39–48. doi: 10.1016/j.marenvres.2018.11.003
Manno C., Sandrini S., Tositti L., Accornero A. (2007). First stages of degradation of Limacina helicina shells observed above the aragonite chemical lysocline in Terra Nova Bay (Antarctica). J. Mar. Syst. 68, 91–102. doi: 10.1016/j.jmarsys.2006.11.002
Many G., Ulses C., Estournel C., Marsaleix P. (2021). Particulate organic carbon dynamics in the Gulf of Lion shelf (NW Mediterranean) using a coupled hydrodynamic–biogeochemical model. Biogeosciences 18, 5513–5538. doi: 10.5194/bg-18-5513-2021
Matsuno K., Yamaguchi A., Fujiwara A., Onodera J., Watanabe E., Imai I., et al. (2014). Seasonal changes in mesozooplankton swimmers collected by sediment trap moored at a single station on the Northwind Abyssal Plain in the western Arctic Ocean. J. Plankton Res. 36, 490–502. doi: 10.1093/plankt/fbt092
Mekkes L., Renema W., Bednaršek N., Alin S. R., Feely R. A., Huisman J., et al. (2021). Pteropods make thinner shells in the upwelling region of the California Current Ecosystem. Sci. Rep. 11, 1731. doi: 10.1038/s41598-021-81131-9
Michaels A. F., Silver M. W., Gowing M. M., Knauer G. A. (1990). Cryptic zooplankton “swimmers” in upper ocean sediment traps. Deep Sea Res. Part A. Oceanographic Res. Papers 37, 1285–1296. doi: 10.1016/0198-0149(90)90043-U
Milker Y., Schmiedl G. (2012). A taxonomic guide to modern benthic shelf foraminifera of the western mediterranean sea. Palaeontologia Electronica.. doi: 10.26879/271
Millot C. (1990). The gulf of lions’ hydrodynamics. Continental Shelf Res. 10, 885–894. doi: 10.1016/0278-4343(90)90065-T
Mohan R., Verma K., Mergulhao L. P., Sinha D. K., Shanvas S., Guptha M. V. S. (2006). Seasonal variation of pteropods from the western arabian sea sediment trap. Geo-Mar Lett. 26, 265–273. doi: 10.1007/s00367-006-0035-1
Moy A. D., Howard W. R., Bray S. G., Trull T. W. (2009). Reduced calcification in modern Southern Ocean planktonic foraminifera. Nat. Geosci 2, 276–280. doi: 10.1038/ngeo460
Moya A., Howes E. L., Lacoue-Labarthe T., Forêt S., Hanna B., Medina M., et al. (2016). Near-future pH conditions severely impact calcification, metabolism and the nervous system in the pteropod Heliconoides inflatus. Glob Change Biol. 22, 3888–3900. doi: 10.1111/gcb.13350
Mucci A., Canuel R., Zhong S. (1989). The solubility of calcite and aragonite in sulfate-free seawater and the seeded growth kinetics and composition of the precipitates at 25°C. Chem. Geology 74, 309–320. doi: 10.1016/0009-2541(89)90040-5
Nielsen S. N. (2000). Numerical Ecology. Legendre P. and Legendre L., second ed., Elsevier, Amsterdam, p. 853, 1998. Ecol. Model. 132, 303–304. doi: 10.1016/S0304-3800(00)00291-X
Oakes R. L., Sessa J. A. (2020). Determining how biotic and abiotic variables affect the shell condition and parameters of <i<Heliconoides inflatus</i< pteropods from a sediment trap in the Cariaco Basin. Biogeosciences 17, 1975–1990. doi: 10.5194/bg-17-1975-2020
Ohman M. D., Lavaniegos B. E., Townsend A. W. (2009). Multi-decadal variations in calcareous holozooplankton in the California Current System: Thecosome pteropods, heteropods, and foraminifera. Geophys. Res. Lett. 36, L18608. doi: 10.1029/2009GL039901
Rampal J. (1975). Les thécosomes (mollusques pélagiques). Université Aix-Marseille, Systématique et évolution - Écologies et biogéographie Mediterranéennes.
Rebotim A., Voelker A. H. L., Jonkers L., Waniek J. J., Meggers H., Schiebel R., et al. (2017). Factors controlling the depth habitat of planktonic foraminifera in the subtropical eastern North Atlantic. Biogeosciences 14, 827–859. doi: 10.5194/bg-14-827-2017
Richardson A. J. (2008). In hot water: zooplankton and climate change. ICES J. Mar. Sci. 65, 279–295. doi: 10.1093/icesjms/fsn028
Rigual-Hernández A. S., Bárcena M. A., Jordan R. W., Sierro F. J., Flores J. A., Meier K. J. S., et al. (2013). Diatom fluxes in the NW Mediterranean: evidence from a 12-year sediment trap record and surficial sediments. J. Plankton Res. 35, 1109–1125. doi: 10.1093/plankt/fbt055
Rigual-Hernández A. S., Sierro F. J., Bárcena M. A., Flores J. A., Heussner S. (2012). Seasonal and interannual changes of planktic foraminiferal fluxes in the Gulf of Lions (NW Mediterranean) and their implications for paleoceanographic studies: Two 12-year sediment trap records. Deep Sea Res. Part I: Oceanographic Res. Papers 66, 26–40. doi: 10.1016/j.dsr.2012.03.011
Rinaldi E., Buongiorno Nardelli B., Volpe G., Santoleri R. (2014). Chlorophyll distribution and variability in the Sicily Channel (Mediterranean Sea) as seen by remote sensing data. Continental Shelf Res. 77, 61–68. doi: 10.1016/j.csr.2014.01.010
Roberts D., Howard W. R., Roberts J. L., Bray S. G., Moy A. D., Trull T. W., et al. (2014). Diverse trends in shell weight of three Southern Ocean pteropod taxa collected with Polar Frontal Zone sediment traps from 1997 to 2007. Polar Biol. 37, 1445–1458. doi: 10.1007/s00300-014-1534-6
Robinson A. R., Sellschopp J., Warn-Varnas A., Leslie W. G., Lozano C. J., Haley P. J. Jr., et al. (1999). The atlantic ionian stream. J. Mar. Syst. 20, 129–156. doi: 10.1016/S0924-7963(98)00079-7
Roger L. M., Richardson A. J., McKinnon A. D., Knott B., Matear R., Scadding C. (2012). Comparison of the shell structure of two tropical Thecosomata (Creseis acicula and Diacavolinia longirostris) from 1963 to 2009: potential implications of declining aragonite saturation. ICES J. Mar. Sci. 69, 465–474. doi: 10.1093/icesjms/fsr171
Rutten A., De Lange G. J., Ziveri P., Thomson J., Van Santvoort P. J. M., Colley S., et al. (2000). Recent terrestrial and carbonate fluxes in the pelagic eastern Mediterranean; a comparison between sediment trap and surface sediment. Palaeogeography Palaeoclimatology Palaeoecol. 158, 197–213. doi: 10.1016/S0031-0182(00)00050-X
Sampei M., Sasaki H., Forest A., Fortier L. (2012). A substantial export flux of particulate organic carbon linked to sinking dead copepods during winter 2007-2008 in the Amundsen Gulf (southeastern Beaufort Sea, Arctic Ocean). Limnol. Oceanogr. 57, 90–96. doi: 10.4319/lo.2012.57.1.0090
Schiebel R., Waniek J., Bork M., Hemleben C. (2001). Planktic foraminiferal production stimulated by chlorophyll redistribution and entrainment of nutrients. Deep Sea Res. Part I: Oceanographic Res. Papers 48, 721–740. doi: 10.1016/S0967-0637(00)00065-0
Schneider A., Wallace D. W. R., Körtzinger A. (2007). Alkalinity of the mediterranean sea. Geophys. Res. Lett. 34, 2006GL028842. doi: 10.1029/2006GL028842
Schroeder K., Gasparini G. P., Borghini M., Cerrati G., Delfanti R. (2010). Biogeochemical tracers and fluxes in the Western Mediterranean Sea, spring 2005. J. Mar. Syst. 80, 8–24. doi: 10.1016/j.jmarsys.2009.08.002
Singh A. D., Nisha N. R., Joydas T. V. (2005). Distribution patterns of Recent pteropods in surface sediments of the western continental shelf of India. J. Micropalaeontol. 24, 39–54. doi: 10.1144/jm.24.1.39
Siokou-Frangou I., Christaki U., Mazzocchi M. G., Montresor M., Ribera d’Alcalá M., Vaqué D., et al. (2010). Plankton in the open Mediterranean Sea: a review. Biogeosciences 7, 1543–1586. doi: 10.5194/bg-7-1543-2010
Tarling G. A., Matthews J. B. L., David P., Guerin O., Buchholz F. (2001). The swarm dynamics of northern krill (Meganyctiphanes norvegica) and pteropods (Cavolinia inflexa) during vertical migration in the Ligurian Sea observed by an acoustic Doppler current profiler. Deep Sea Res. Part I: Oceanographic Res. Papers 48, 1671–1686. doi: 10.1016/S0967-0637(00)00105-9
Thiede J., Jünger B. (1992). Faunal and floral indicators of ocean coastal upwelling (NW African and Peruvian Continental Margins). SP 64, 47–76. doi: 10.1144/GSL.SP.1992.064.01.04
Tyrrell T. (2007). Calcium carbonate cycling in future oceans and its influence on future climates. J. Plankton Res. 30, 141–156. doi: 10.1093/plankt/fbm105
Violanti D., Grecchi G., Castradori D. (1991). Paleoenvironmen- tal interpretation of Core BAN88-11GC (Eastern Mediterranean, Pleistocene-Holocene), on the grounds of Foraminifera, Theco- soma and Calcareous Nannofossils. Il Quaternario 4, 13–19.
Wall-Palmer D., Smart C. W., Hart M. B., Leng M. J., Borghini M., Aliani S., et al. (2014). Late Pleistocene pteropods, heteropods and planktonic foraminifera from the Caribbean Sea, Mediterranean Sea and Indian Ocean. mpal 60, 557–558. doi: 10.47894/mpal.60.6.05
Weldrick C. K., Makabe R., Mizobata K., Moteki M., Odate T., Takao S., et al. (2021). The use of swimmers from sediment traps to measure summer community structure of Southern Ocean pteropods. Polar Biol. 44, 457–472. doi: 10.1007/s00300-021-02809-4
Willis K. J., Cottier F. R., Kwaśniewski S. (2008). Impact of warm water advection on the winter zooplankton community in an Arctic fjord. Polar Biol. 31, 475–481. doi: 10.1007/s00300-007-0373-0
Willis K., Cottier F., Kwasniewski S., Wold A., Falk-Petersen S. (2006). The influence of advection on zooplankton community composition in an Arctic fjord (Kongsfjorden, Svalbard). J. Mar. Syst. 61, 39–54. doi: 10.1016/j.jmarsys.2005.11.013
Wormuth J. H. (1985). The role of cold-core Gulf Stream rings in the temporal and spatial patterns of euthecosomatous pteropods. Deep Sea Res. Part A. Oceanographic Res. Papers 32, 773–788. doi: 10.1016/0198-0149(85)90114-1
Zamelczyk K., Fransson A., Chierici M., Jones E., Meilland J., Anglada-Ortiz G., et al. (2021). Distribution and abundances of planktic foraminifera and shelled pteropods during the polar night in the sea-ice covered Northern Barents Sea. Front. Mar. Sci. 8. doi: 10.3389/fmars.2021.644094
Keywords: pteropods, Limacina inflata, Heliconoides inflatus, seasonal variations, time series, Mediterranean Sea, sediment trap
Citation: Béjard TM, Manno C, Sierro FJ, Flores J-A, Tarruella JP, de Madron XD, Sanchez-Vidal A and Rigual-Hernández AS (2024) Biogeographical and seasonal distribution of pteropod populations in the western and central Mediterranean Sea inferred from sediment traps. Front. Mar. Sci. 11:1346651. doi: 10.3389/fmars.2024.1346651
Received: 29 November 2023; Accepted: 08 April 2024;
Published: 26 April 2024.
Edited by:
Stefano Aliani, National Research Council (CNR), ItalyReviewed by:
Ramadoss Dineshram, Council of Scientific and Industrial Research (CSIR), IndiaAlessandra Conversi, National Research Council (CNR), Italy
Copyright © 2024 Béjard, Manno, Sierro, Flores, Tarruella, de Madron, Sanchez-Vidal and Rigual-Hernández. This is an open-access article distributed under the terms of the Creative Commons Attribution License (CC BY). The use, distribution or reproduction in other forums is permitted, provided the original author(s) and the copyright owner(s) are credited and that the original publication in this journal is cited, in accordance with accepted academic practice. No use, distribution or reproduction is permitted which does not comply with these terms.
*Correspondence: Thibauld M. Béjard, dGhpYmF1bGQuYmVqYXJkQHVzYWwuZXM=