- Experimental Marine Ecology Laboratory, Department of Biological Sciences, National University of Singapore, Singapore, Singapore
Marine microplastics have become a pernicious global pollution issue. As field surveys to determine microplastic abundance in the marine environment and/or biota become more common, it is important to refine collection techniques to minimize contamination of samples. However, most contemporary sampling equipment is fabricated with plastic components. Microplastic contamination during sample collection can be minimized from the onset by employing techniques that are plastic-less or with minimal plastic-to-sample contact. Here we describe plastic-less equipment and techniques for field sampling of water, sediment, and organisms. Some of these are traditional designs that pre-date the plastic era and can be revived or repurposed for microplastic sampling. Others are self-developed and fabricated using materials such as metal, wood, silk, and cork. For relatively small costs in time and funds, it is possible to greatly reduce, or eliminate, plastics from the field sampling process.
1 Introduction
Since the start of mass-production in the 1950s plastic has been a pollutant in the marine environment (Barnes et al., 2009). In 2010, an estimated 275 million tonnes of plastic waste were produced across hundreds of coastal countries, of which 4.8 to 12.7 million tonnes were estimated to have entered the ocean (Jambeck et al., 2015). The number of plastics manufactured has continued to increase over the years (Geyer et al., 2017; Ostle et al., 2019) and has been predicted to reach a cumulative amount of 34,000 million tonnes in 2050 (Geyer et al., 2017). Microplastics, defined as plastics between 1 and 5,000 µm (GESAMP, 2019), are a subset of the growing mass of plastic pollution that is causing concern both among scientists and the general public. They have been found in a broad range of marine environments, from the deep sea (Woodall et al., 2014) to ocean gyres (Law et al., 2010; Eriksen et al., 2013) and coastal habitats (Jeyasanta et al., 2020; Zamprogno et al., 2021). Owing to their small size they are also widely accessible to, and have been found in, marine biota from mega- to microfauna (e.g. Thushari et al., 2017; Nelms et al., 2018; Sun et al., 2018; Maes et al., 2020; Xu et al., 2020) and can have various adverse effects on marine life, including negatively affecting energy budget and gamete quality (Sharifinia et al., 2020; Berlino et al., 2021).
Quantifying the risk of microplastics exposure in the environment and to associated organisms is an ongoing research priority (Omeyer et al., 2022). However, contemporary environment and biota sampling of microplastics is often conducted using plastic-derived equipment (in total or in part; e.g. Duursma, 1967). The use of plastic presents a contamination issue due to possible shedding or weathering of the material and introduction of these particles to the sample (Tsuchiya et al., 2019; Hung et al., 2021). Although this may be accounted for by including blanks during sample collection and processing (i.e., field blanks and procedural blanks), background contamination remains problematic, especially for samples with very low microplastic counts. Depending on the blank correction method used, high or highly variable background contamination could contribute to the underestimation of microplastic numbers in samples (Dawson et al., 2023). All plastics used in sampling equipment are a potential contamination source, hence their removal should be a priority for microplastic research.
The most certain way to eliminate or minimise contamination from equipment during sampling is by avoiding the use of plastic altogether. In this paper we identify various plastic-less approaches to collecting microplastics from both subtidal and intertidal environments. Some of these are older devices or traditional designs that pre-date the plastic era, whereas others are self-developed and fabricated using materials such as metal, wood, silk, cork, and glass (Table 1). Our goal is to collate as many options as possible into one document, including those that may be simple or obvious. We discuss the benefits and drawbacks of the designs and the materials used and, in some cases, provide fabrication instructions in the Supplementary Data. Many of the solutions are also suitable for sampling from freshwater habitats. We aim to show that, for relatively little effort and cost, it is possible to eliminate, or at least greatly reduce, plastics from the field sampling process. This work contributes to the ongoing efforts to reduce contamination in microplastic research (e.g. Tsuchiya et al., 2019; Hung et al., 2021). We do not make comparisons in contamination levels between plastic and plastic-less versions of the same equipment as this is a large exercise for even a single type of sampling device. The paper is organised into three fundamental categories of protocols: water, sediment, and organism sampling.
2 Sampling seawater
Approaches to seawater sampling depends on the desired microplastic size range (e.g. method of sampling or filtration can reduce or increase the sampling size range), sampling volume of interest (e.g. volume that is sufficient to overcome the variability of microplastic abundance in the environment; Covernton et al., 2019; Watkins et al., 2021), and the nature of the area to be sampled (e.g. some areas may not be accessible by boat). However, all methods can be grouped under the general categories of ‘container-based’, ‘pump-based’ and ‘net-based’ protocols.
2.1 Container-based
The most basic form of container-based water sampling is a bucket deployed from the side of a boat or jetty. This can be easily converted to plastic-less using an unpainted metal bucket and a natural fibre rope (e.g. hemp, jute and cotton). It is, however, very limited in its scope of use (e.g. surface water sampling only), and therefore may be most applicable to student exercises and citizen science studies.
Standard Van Dorn horizontal and vertical bottle water samplers are attached to the end of a rope and, again, can be deployed over the side of a boat or jetty. They enable the user to sample from a specified depth by remaining open until a messenger (a metal weight) is sent down the rope to trigger the closing mechanism. However, all horizontal and vertical samplers we identified on the market were made from plastic. Some of the old fashioned and/or discontinued oceanographic equipment, such as Nansen bottles (Figure 1A) are made entirely of metal (albeit originally painted), but these are expensive and/or hard to find. To conduct plastic-free water sampling at a specific depth with a desired sampling volume, it is feasible to build an all-metal Van Dorn bottle. We made ours out of a 500 mm long, 50 mm diameter stainless steel pipe, with hemispherical cork stoppers connected together with metal springs (Figure 1B, see S1.1 and Supplementary Figure S1 for details). The remote triggering system was made from stainless steel wire, metal spring clips, natural fibre string, and a brass messenger (Ormaza-González et al., 2022). The water volume collected was ultimately too small (1 L) for our needs, but the design could be scaled up.
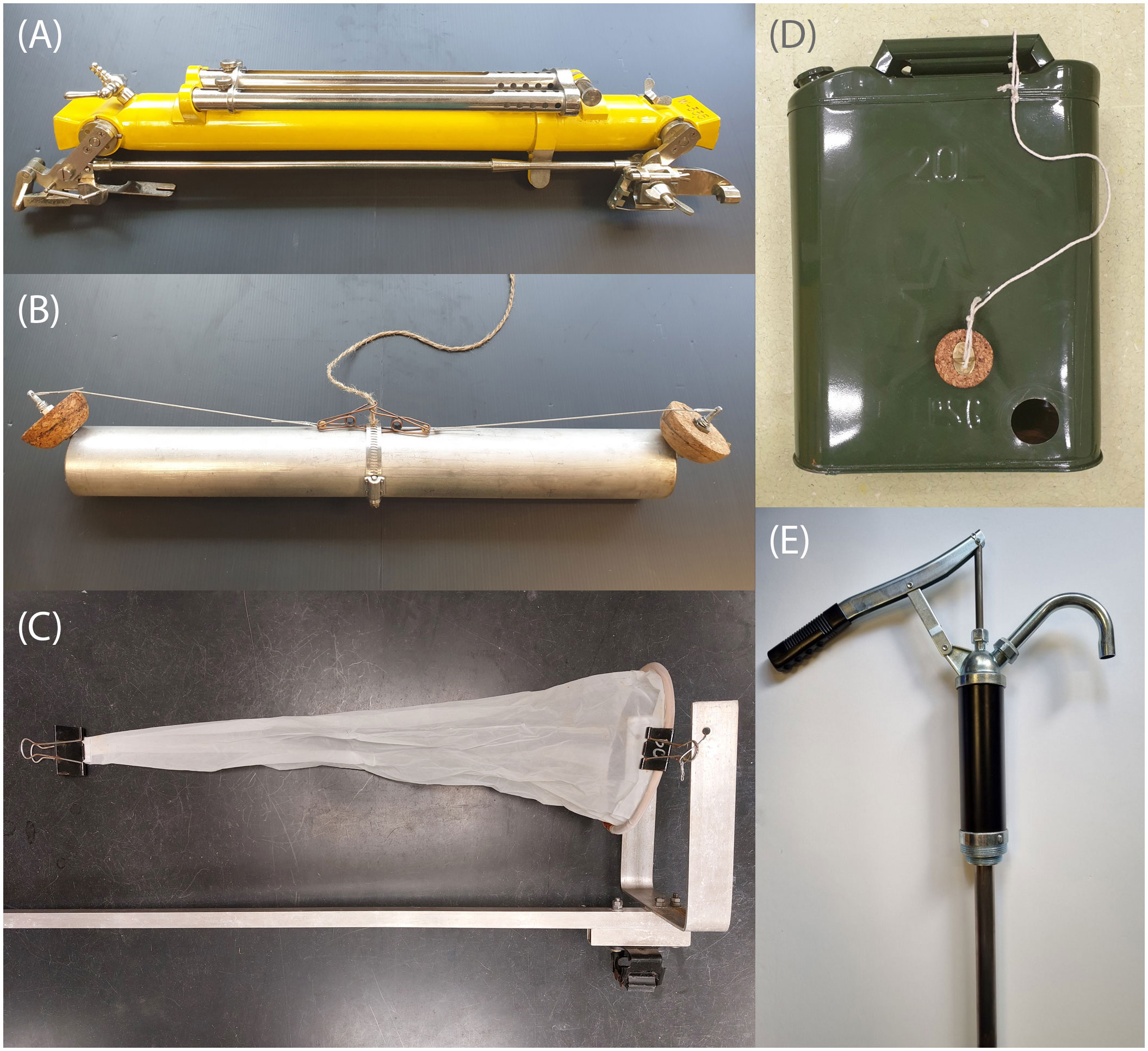
Figure 1 Examples of plastic-less water sampling devices including (A) an all-metal Nansen water sampling bottle, (B) a plastic-less Van Dorn horizontal water sampler, (C) a self-fabricated push-net (D) a simple water sampler based on a 20 L jerrycan, (E) a pump for water sampling. Fabrication instructions for the Van Dorn, plankton net frame and jerrycan designs can be found in the in Supplementary Data.
Collecting from specific depths is also possible through SCUBA diving (we will focus on SCUBA diving as surface-supply commercial diver services tend to be expensive). As a proof-of-concept we tested a sampler based on a 20 L steel jerrycan. We cut a 48 mm hole at the bottom of one side of the jerrycan and fashioned a cork stopper to fit it (Figure 1D, see S1.2 and Supplementary Figure S2 for details). When deploying in the field, a SCUBA diver descends with the jerrycan mouth uncapped and the hole uncorked, allowing water to flow through the can. When the desired depth is reached the jerrycan is purged with air from the SCUBA tank to ensure only water from that depth is collected, and then both openings are sealed. The diver then ascends with the water sampler secured to a lift bag (or, if below a boat, it could be on a rope so that personnel can lift it up and out of the water). One main advantage of having a SCUBA diver using such containers is that they can take them to locations that may otherwise be inaccessible. If advancing this design to real-world deployment, it would be necessary to strip the jerrycan of paint and replace the plastic gasket in the cap with one cut from cork sheet.
There are other factors to be considered when designing bespoke samplers, for example, does the design require large quantities of water to be transported from the study site to a laboratory? This is true for any container-based design, as well for the pump-based design described below. If the water is processed in situ, e.g. on the deployment boat or other platform, then the transport issue is resolved. However, processing samples on a boat (away from a laboratory-based environment) adds other challenges, such as creating a clean workspace.
2.2 Pump-based
Most electric-powered pump-based water collection systems require a plastic hose and a pump made with numerous plastic components. It is, however, possible to obtain a low-cost all-metal pumping system that collects water from down to at least 3.5 m depth (the max depth we tested). The design we used was hand operated (lever-style) stainless steel pump that we fitted with regular plumbing-type 15 mm diameter copper pipe (Figure 1E, see S1.3 and Supplementary Figure S3 for details). We used brass compression fittings because they can be loosened and retightened multiple times, facilitating dismantling and transport. The pump was mounted onto a wooden platform to stabilise it during the water collection. After connecting the sections needed for the desired sampling depth, the pipe was carefully lowered into the water and connected to the pump using another compression fitting. After first pumping to flush the system, we were able to achieve a water flow rate of approximately 9 L min-1.
2.3 Net-based
Plankton nets and variations such as manta net tows have substantial potential for marine microplastic sampling. They can filter large quantities of water in short periods of time and their deployment and procedures are well established based on a long history of use for sampling plankton. Plankton nets are simple in design and plastic can be reduced to zero with some effort. The net ring is generally made from metal, as is the front swivel and tow ring. If bridles/leaders are made from plastic these should be changed to metal wire, chain, or natural fibre string. A plastic net bucket can be replaced by a glass jar, or just removed and a metal clip (such as a paint-free metal bulldog clip) used to seal the cod (tail) end. A natural fibre towing line is needed. Plastic (usually nylon) plankton nets can be replaced with silk plankton nets, but these may need to be self-made. Mesh size can be based on the momme counts of the silk. It may be possible to buy a complete set with a silk, rather than nylon, net. However, it is important to note that, compared to nylon, silk is more likely to distort through linear extension and shrink in mesh size due to absorption of water (Heron, 1968), influencing the filtration efficiency and size accuracy of the net. Lastly, if removing the plastic from a commercially purchased plankton net means discarding most of its components, it may be more cost-effective to fabricate a full set. A summary of factors to consider during net design and fabrication can be found in S1.4.
Plankton nets can also be used by SCUBA divers for more targeted collections. We fabricated a metal frame for holding the net so it could be pushed by a diver over specific areas of coral reef. The design used a length of aluminium square section pipe with a U-shaped frame made from a piece of flat aluminium bar bent to shape attached to it at the leading end with stainless steel bolts (Figure 1C, see S1.4 and Supplementary Figures S4–S6 for details). On each side of the U-frame was a hole for a short chain or wire loop connected to a bulldog clip. This clip was then used for holding the plankton net ring (150 mm diameter). The chain/loop arrangement created a basic gimble effect (as opposed to clamping the ring directly onto the U-frame), allowing the net to follow the incoming current. The sampling distance was planned based on the intended sampling volume, following the simple calculation of a cylinder, i.e., the product of distance travelled and mouth area of the plankton net, as is the normal procedure for plankton sampling. To further account for any water current, we attached a water flow meter on the underside of the U-frame to ensure accurate calculation of the quantity of water passing though the plankton net.
Reusing plankton nets within a single sampling trip may not be feasible as removal of microplastics requires careful rinsing under controlled conditions. Treating each net as an independent sample by choking the net near the opening with wire or natural fibre string and storing in a metal container for processing in the laboratory is the most certain way to avoid contamination. However, this will increase costs as several plankton nets may be needed.
2.4 Summary
All three seawater sampling techniques can be executed without the use of plastics. De novo fabrication or modification of existing equipment is achievable with inexpensive materials. Only the jerrycan and push-net techniques allow for fine-scale spatial sampling as they can be deployed by SCUBA divers. Note that the high spatial variability in the abundance of microplastics in marine waters (Karlsson et al., 2020) means that generally multiple samples and/or large amounts of water need to be collected (Covernton et al., 2019; Watkins et al., 2021). While net-based techniques can sample high volumes of water, the size of microplastics captured is limited by the mesh size of the plankton net used (Covernton et al., 2019; Lindeque et al., 2020; Watkins et al., 2021). Furthermore, microfibres may pass through the net even when their length is longer than the mesh size (Covernton et al., 2019; Watkins et al., 2021). Container-based and pump-based sampling, on the other hand, capture the full range of microplastic sizes—but also generate bulky and heavy samples that, unless they can be processed immediately, need to be transported back to the laboratory where samples can be handled in a ‘clean’ environment.
3 Sampling marine sediment
There are two fundamental approaches to collecting settled (not suspended) sediment: ‘hand sampling’, i.e., gathering material in containers via swimming/snorkelling, SCUBA diving, or during low tide (e.g. Kreitsberg et al., 2021; Zamprogno et al., 2021), and ‘remote sampling’ using a grab or corer deployed from above, usually from a boat (e.g. Bakir et al., 2020; Jeyasanta et al., 2020).
3.1 Hand-sampling
Hand-sampling of sediment is straightforward, yet still presents a few challenges depending on the type of substrate, depth of sample, and quantities of material needed. For sampling coarse or consolidated sediments, a stainless steel trowel (non-painted with a wooden handle) can be used to dig up and transfer material into a glass jar with a metal screw lid or into a metal tin with a cork stopper may be sufficient. Fine sediment tends to be easily disturbed and difficult to trowel into a receptacle underwater. We explored syringe type devices for sucking up surface sediment, but these are difficult to make out of non-plastic materials, especially achieving a good plunger seal. Even pilot tests using regular 200 ml plastic syringes with the end partly cut off did not perform very well (poor sediment to water ratio). It may be preferable to collect the top layer of sediment using the receptacle itself. As all screw-top containers have circular mouths, it is problematic to achieve an even depth of scoop if positioned on their side and shovelled through the sediment. Furthermore, only a small amount of the sediment is collected in the container, while the rest of the sediment is simply moved forward. A square or rectangular container with a thin lip is more suitable, but sourcing one with a watertight lid can be difficult – especially if replication requires having many such containers. We fabricated ours using 100 mm × 25 mm aluminium extrusion (i.e. rectangular section pipe) — a cheap material that can be cut to the desired length (250 mm in our case). Both ends can be sealed with cork or wood stoppers that can be ordered to size or self-fabricated (Figure 2A, see S2.1 and Supplementary Figures S7, 8 for details). Note, when working underwater, it is impossible for the box to be completely packed with sediment as there will always be water collected too. For vertical sediment sampling, traditional coring techniques can be applied. A simple plastic-less sediment push-corer can be made from round stainless steel pipe (or aluminium, see Tsuchiya et al., 2019) and cork or wooden stopper. These may be available commercially (although generally not with non-plastic stopper), custom-made, or self-fabricated (Figure 2B, see S2.2 and Supplementary Figure S9 for details).
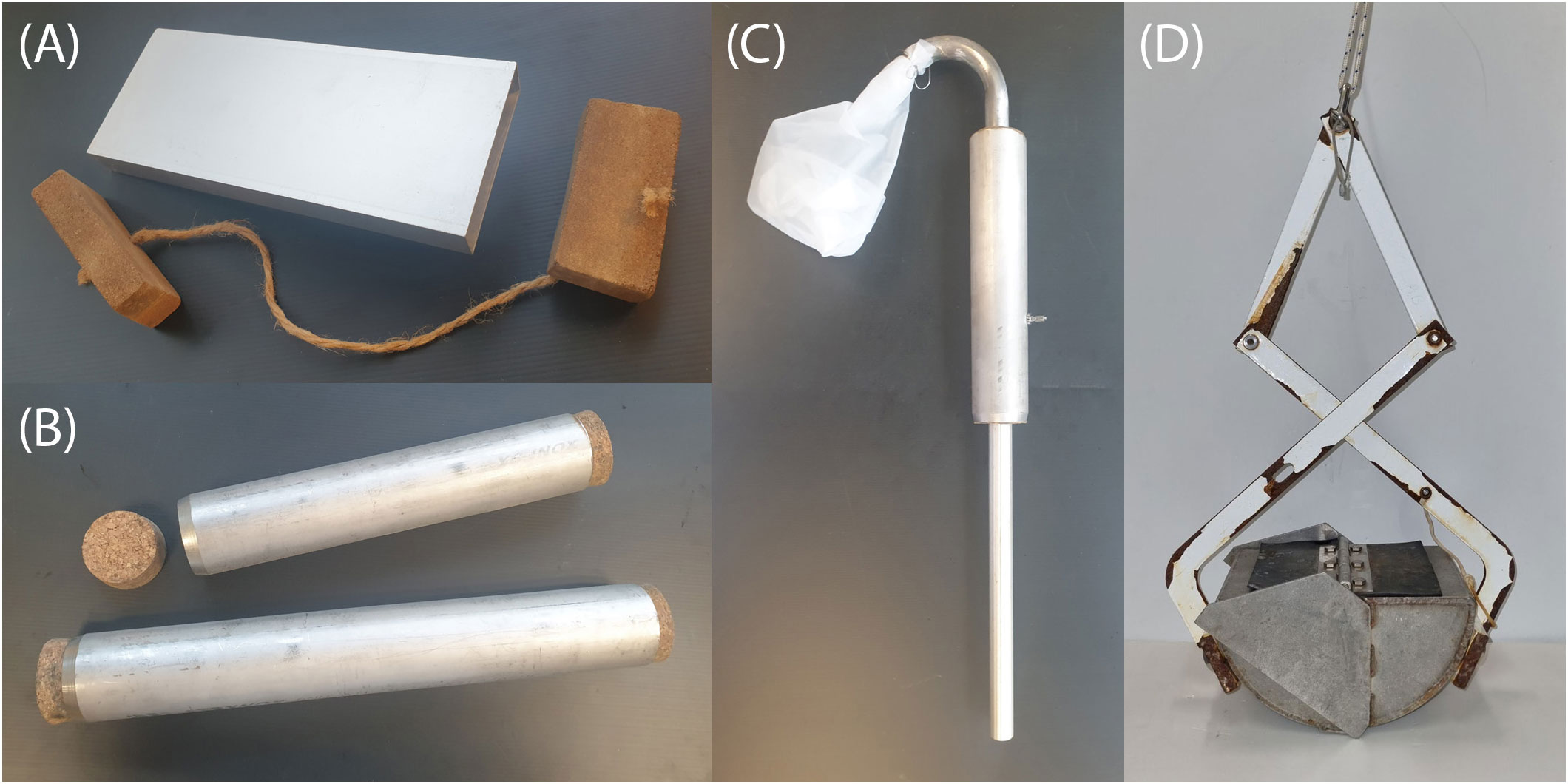
Figure 2 Examples of collection equipment including (A) a specially fabricated, plastic-less container for holding sediment samples, (B) specially fabricated, plastic-less push-corers, (C) a SCUBA-tank powered pooter device for sampling fine sediment, (D) a Ponar sediment grab with flaking paint.
For larger quantities of loose sediment, a pump approach can be considered. We have not employed pumps for sampling microplastics, but have used them previously for sampling sediment from coral reefs and micro-invertebrates from soft lake sediment. In Loke et al. (2010) we tested two designs: an air-lift pooter-type suction sampler that is used wholly underwater, and a powered water pump sampler mounted on a boat. These were largely made from plastic, but can be fabricated from metal – especially the pooter design as it does not require a hose leading to the surface, instead it has a mesh bag attached to the outlet of the device (Figure 2C, see S2.3 and Supplementary Figure S10 for details). Powered by a SCUBA tank, the pooter uses air rising in a tube to create suction strong enough to lift fine sediments. Loke et al. (2010) also tested a sampler that used a gasoline engine driven water pump on a boat to suck water and sediment which were then passed through a sieve. This design still requires a SCUBA diver to control the suction end of the device. The pump had plastic parts and the hose was made from plastic. Unlike the pump-based water sampling device described in section 2.2, we do not think it is feasible to replace the plastic hose with copper pipe for sediment sampling due to the flexibility needed. Both these pump systems disturb the substrate, and this can lead to loss of material and reduced underwater visibility.
3.2 Remote-sampling
Ekman, Van Veen, Ponar, and other bottom sand and silt type grabs have been used multiple times for plastics sampling (e.g. Jeyasanta et al., 2020; Zhang et al., 2020). They tend to be made wholly of metal but are sometimes painted (Figure 2D). If all paint is removed and they are lowered on a natural fibre rope, the equipment can be completely plastic free. Most of these devices ‘grab’ sediment automatically upon contact with the substrate or via a messenger. Although these types of grabs are reliable and robust, the seal is usually not perfect and therefore fine sediments, and potentially microplastics, can be lost. Remote corers can also be made from metal, and protocols for their use are well-established (see Tsuchiya et al., 2019). As grabs and corers are deployed from a vessel, it is often not possible to see the seafloor, making it difficult to achieve the fine spatial resolution sampling possible through hand sampling. Further, they do not perform very well in stony substrates. However, both grabs and corers are common techniques for sampling of sediment and have the substantial advantage of using standard equipment that makes comparison across studies more feasible.
3.3 Summary
There are a range of options that are readily available and/or can be fabricated easily to sample sediments using plastic-less materials. These can be applied to the collection of sediment at different spatial scales, habitats, and water depth. Hand-sampling methods are recommended for collecting sediment at finer spatial resolution as well as within less homogenous habitats, e.g. those with many rocks. Hand-sampling methods also allow more control over the depth of sediment collected. If larger quantities are required, the boat-based pump method is potentially more suitable, but it requires a flexible (plastic) pipe. Remote-sampling, on the other hand, does not require the deployment of divers, uses well-established equipment and techniques, and generally results in larger quantities of material collected.
4 Sampling marine organisms
The most suitable approach for sampling marine organisms very much depends on the species. Sampling of marine mammals, seabirds, and large fish such as sharks is beyond the scope of this paper. Other than organisms where the microplastics are attached to external surfaces, such as macroalgae and seagrass (Seng et al., 2020), the risk of contamination is lower compared to seawater and sediment sampling as the exterior of the organisms can be washed (e.g. Miller et al., 2023). The primary risk is the organism ingesting microplastics that originated from the sampling equipment.
4.1 Fishes
Traditional benthic cage-like traps to capture fishes and crustaceans have been used by artisanal fishermen in many parts of the worlds for decades or centuries (Figure 3A; S3.1, Supplementary Figure S11). While less commonly used in the contemporary era, traps made from rattan, bamboo, and/or metal are still fabricated and sold. It is important to use non-painted versions and replace any plastic components such as cable ties with wire or natural fibre string. While trapping is a common technique for capturing demersal fish, nets can be used to catch both demersal and pelagic fish depending on how the net is deployed. Small gill nets can be fabricated using cotton/hemp net with natural cork and metal weights attached to the top and bottom of the net respectively (Figure 3D; S3.1, Supplementary Figure S11). Note that these nets are easy for fish to see and may prevent some from being caught. Angling with metal hooks and natural fibre line is also very effective. If plastic fishing line needs to be used, a 30-50 cm length of fishing wire can be inserted between the hook and the plastic line to reduce the likelihood of contamination (Figure 3B; S3.1; Supplementary Figure S12). Unfortunately, using a metal wire also increases the visibility of the line to the fish and can reduce capture rate. To correct for any microplastic contamination in the bait used, we suggest having at least three bait samples from each batch purchased kept aside as controls, and to record the weight of the bait used to capture each specimen. Lastly, spear guns are potentially effective methods to capture fish and simple versions are relatively easy to make using wood, natural rubber, and metal rod. However, due to local regulations regarding owning them, we could not test their efficacy.
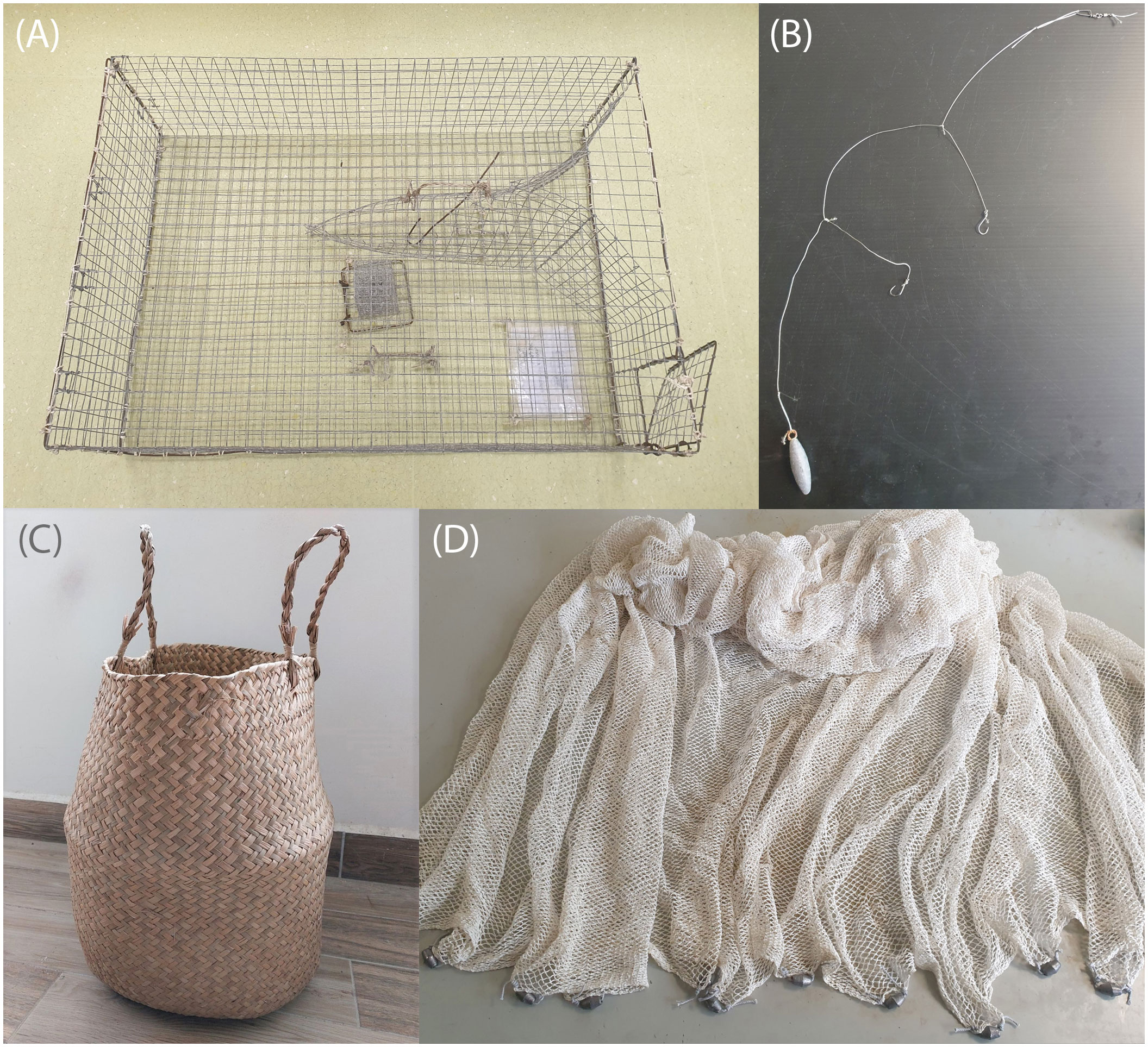
Figure 3 Plastic-less options for the collection of marine organisms such as (A) a traditional benthic cage-like trap to capture fish and crustaceans, (B) stainless steel wire leader line, hook, and lead sinker, (C) basket woven from seagrass to hold organisms collected by hand, (D) cotton net for catching fish.
4.2 Invertebrates
Most invertebrates can be collected by hand (optionally with cotton gloves for general field safety), forceps, or un-painted metal tongs (such as barbeque tongs). Infauna invertebrates can be filtered out of the sediment with a metal sieve or dug out using a paint-free metal spade or trowel. Grab samplers can also be used to collect sediment samples from which the invertebrates can be retrieved from. We placed smaller specimens into cotton draw string bags or metal strainer baskets with lids and larger motile invertebrates in rattan or seagrass baskets (Figure 3C; S3.2, Supplementary Figure S13). To prevent fast-moving organisms such as crustaceans from escaping, it is recommended to have a spring lid which opens only inwards or create an inward-pointing funnel. Some marine invertebrates such as sea cucumbers (holothurians) may evert their inner organs under stress. Hence, to avoid cross-contamination, these specimens should be stored in separate containers. Sessile invertebrates (e.g., corals and sponges) can be collected using paint-free hammers, chisels, and/or cutters. Some suspension- and filter-feeders may continue to take in water while divers return to shore or boat. To prevent this, we suggest storing them in pre-prepared aluminium foil bags underwater during collection, sealed by folding the opening multiple times over. Planktonic invertebrates can be sampled using the same or similar equipment as described in section 2.3 above.
4.3 Organisms where the microplastics are adhered to the surface
Recent work has described microplastic attached to the surfaces of seagrasses and macroalgae (e.g. Seng et al., 2020). It is important not to introduce further contamination to the surface of these organisms, and also eliminate or minimise contact with other samples (and sampling materials/containers) to minimize cross-contamination. Such specimens are also best inserted into single-use containers such as pre-prepared aluminium foil bags before placing them into a larger basket for transport.
4.4 Summary
Most sampling tools and materials for sampling marine organisms are affordable and often found in our daily lives, e.g., in the kitchen or garage. We found that handmade aluminium foil envelopes were good replacements for plastic bags as they are lightweight and flexible. Fish and crustacean traps can be fabricated or modified to be plastic free (e.g. replacing plastic cable ties with natural string). For sampling for carnivorous and omnivorous motile organisms, we suggest employing non-baiting techniques such as spearfishing (if permitted) and netting as baits are a potential source of microplastic contamination. Note that, in structurally complex habitats such as coral reefs, nets may become entangled and harm benthic species.
5 Discussion
To understand the abundance of microplastics in the environment and biota, the minimization of microplastic contamination to the sample is critical. A common treatment of field data is to subtract the mean abundance of microplastics in the control from the raw abundance of microplastics in the sample (e.g. Karlsson et al., 2017; Mizraji et al., 2017; Le Guen et al., 2020; Patterson et al., 2020; Patti et al., 2020; Oldenburg et al., 2021). However, a recent study by Dawson et al. (2023) compared the robustness of different data treatment methods employed among microplastic survey studies and recommended the use of limit of detection or quantification, whereby the final abundance of microplastics in the sample is determined by subtracting the mean abundance of microplastics in the control, plus 10× the standard deviation, from the raw microplastic abundance value in the sample. Such an approach is substantially more sensitive to contamination than current practices. As the recommended treatment of data becomes more conservative, it is even more crucial to reduce contamination in samples, especially those with potentially low microplastic load. As environmental microplastic sampling studies extend into more habitats, including mangrove forests, coral reefs, and seagrass meadows (e.g. Huang et al., 2020; Jeyasanta et al., 2020; Martin et al., 2020), and encompass more organisms of different feeding guilds and taxa (e.g. Bour et al., 2018; Driscoll et al., 2021; Covernton et al., 2022), a wider range of sampling techniques and devices is needed. Our objective is to help microplastic researchers achieve minimal, or zero, contamination emanating from their sampling equipment.
During the sampling process, contamination from water and air is still possible (e.g. de Vries et al., 2020; Huntington et al., 2020; Cunningham et al., 2022). Contamination of sediments from water can be accounted for by calculating the average volume of water collected per sediment sample and subtracting the average abundance of microplastics in the water from microplastic abundance in the sediment. There is some debate regarding whether organisms may be contaminated by microplastics from surrounding water. Conducting blanks for organisms is challenging due to the difficulty of mimicking the texture of the organism’s exterior and their behaviour under stress (e.g. increase stress may increase respiration rate and hence water intake rate). To control for airborne contamination, we suggest including a procedural blank whenever possible, i.e. performing the same sample processing procedure without collecting the sample (Cunningham et al., 2022). Ideally, this should be performed for water sampling too (Huntington et al., 2020). These approaches will account for most contamination sources.
We recommend microplastic studies adopt a hierarchy of control to reduce sample contamination. Firstly, any unnecessary plastic in the sampling devise or procedure should be eliminated. The next step is to replace essential plastic materials or parts with non-plastic materials. If this is not possible, then the amount of plastic used should be limited to that which is absolutely necessary. If studies have to proceed using equipment comprising plastic elements, we suggest using plastics in colours or types not commonly found among environmental samples. We recommend performing a scratch test on the plastic materials to observe their fragmented shape under the microscope. This will facilitate their removal during the sample sorting process.
Most the equipment presented here is inexpensive. Microplastic pollution is a global issue, and it is important to collect data from every region, however, access to funds is likely to vary and keeping costs low may make the difference between research proceeding or not. Numerous collection tools and devices were once made from non-plastic materials and, if these can be found, they can be repurposed. There are also many opportunities to design new techniques to suit both budget and sampling challenges. By combining the wide range of non-plastic materials available with some creativity (Table 1), contamination-free sampling equipment is an achievable goal.
Data availability statement
The original contributions presented in the study are included in the article/Supplementary Material. Further inquiries can be directed to the corresponding authors.
Author contributions
PT: Conceptualization, Methodology, Visualization, Writing – original draft, Writing – review & editing, Funding acquisition, Investigation, Supervision, Validation. CY: Conceptualization, Investigation, Methodology, Validation, Visualization, Writing – original draft, Writing – review & editing. SHF: Investigation, Methodology, Validation, Visualization, Writing – original draft, Writing – review & editing. LY: Investigation, Methodology, Validation, Visualization, Writing – review & editing. JL: Investigation, Methodology, Validation, Visualization, Writing – review & editing.
Funding
The author(s) declare financial support was received for the research, authorship, and/or publication of this article. This study was supported by the National Research Foundation, Prime Minister’s Office (Singapore) and the Natural Environment Research Council (United Kingdom) under the NRF-NERC-SEAP-2020 grant call ‘Understanding the Impact of Plastic Pollution on Marine Ecosystems in Southeast Asia (South East Asia Plastics [SEAP]). Author CY is supported by the NUSDeltares PhD Scholarship.
Acknowledgments
We thank the members of the Experimental Marine Ecology Laboratory, National University of Singapore, for their help in the field.
Conflict of interest
The authors declare that the research was conducted in the absence of any commercial or financial relationships that could be construed as a potential conflict of interest.
Publisher’s note
All claims expressed in this article are solely those of the authors and do not necessarily represent those of their affiliated organizations, or those of the publisher, the editors and the reviewers. Any product that may be evaluated in this article, or claim that may be made by its manufacturer, is not guaranteed or endorsed by the publisher.
Supplementary material
The Supplementary Material for this article can be found online at: https://www.frontiersin.org/articles/10.3389/fmars.2024.1345591/full#supplementary-material
References
Bakir A., Desender M., Wilkinson T., Van Hoytema N., Amos R., Airahui S., et al. (2020). Occurrence and abundance of meso and microplastics in sediment, surface waters, and marine biota from the South Pacific region. Mar. Pollut. Bull. 160, 111572. doi: 10.1016/j.marpolbul.2020.111572
Barnes D. K. A., Galgani F., Thompson R. C., Barlaz M. (2009). Accumulation and fragmentation of plastic debris in global environments. Philos. Trans. R. Soc Lond. Ser. B: Biol. Sci. 364 (1526), 1985–1998. doi: 10.1098/rstb.2008.0205
Berlino M., Mangano M., De Vittor C., Sarà G. (2021). Effects of microplastics on the functional traits of aquatic benthic organisms: A global-scale meta-analysis. Environ. Pollut. 285, 117174. doi: 10.1016/j.envpol.2021.117174
Bour A., Avio C. G., Gorbi S., Regoli F., Hylland K. (2018). Presence of microplastics in benthic and epibenthic organisms: Influence of habitat, feeding mode and trophic level. Environ. Pollut. 243, 1217–1225. doi: 10.1016/j.envpol.2018.09.115
Covernton G. A., Cox K. D., Fleming W. L., Buirs B. M., Davies H. L., Juanes F., et al. (2022). Large size (> 100-μm) microplastics are not biomagnifying in coastal marine food webs of British Columbia, Canada. Ecol. Appl. 32 (7), e2654. doi: 10.1002/eap.2654
Covernton G. A., Pearce C. M., Gurney-Smith H. J., Chastain S. G., Ross P. S., Dower J. F., et al. (2019). Size and shape matter: A preliminary analysis of microplastic sampling technique in seawater studies with implications for ecological risk assessment. Sci. Total Environ. 667, 124–132. doi: 10.1016/j.scitotenv.2019.02.346
Cunningham E. M., Rico Seijo N., Altieri K. E., Audh R. R., Burger J. M., Bornman T. G., et al. (2022). The transport and fate of microplastic fibres in the Antarctic: The role of multiple global processes. Front. Mar. Sci. 9. doi: 10.3389/fmars.2022.1056081
Dawson A. L., Santana M. F., Nelis J. L., Motti C. A. (2023). Taking control of microplastics data: A comparison of control and blank data correction methods. J. Hazard. Mater. 443, 130218. doi: 10.1016/j.jhazmat.2022.130218
de Vries A. N., Govoni D., Árnason S. H., Carlsson P. (2020). Microplastic ingestion by fish: Body size, condition factor and gut fullness are not related to the amount of plastics consumed. Mar. Pollut. Bull. 151, 110827. doi: 10.1016/j.marpolbul.2019.110827
Driscoll S. C., Glassic H. C., Guy C. S., Koel T. M. (2021). Presence of microplastics in the food web of the largest high-elevation lake in North America. Water 13 (3), 264. doi: 10.3390/w13030264
Duursma E. K. (1967). A simple, horizontally hauled large volume water-sampling bottle of Van Dorn type. Deep Sea Res. Oceanographic Abstracts 14 (1), 133–134. doi: 10.1016/0011-7471(67)90038-1
Eriksen M., Maximenko N., Thiel M., Cummins A., Lattin G., Wilson S., et al. (2013). Plastic Pollution in the South Pacific subtropical gyre. Mar. Pollut. Bull. 68 (1-2), 71–76. doi: 10.1016/j.marpolbul.2012.12.021
GESAMP (2019). “Guidelines for the monitoring and assessment of plastic litter in the ocean,” in IMO/FAO/UNESCO-IOC/UNIDO/WMO/IAEA/UN/UNEP/UNDP/ISA Joint Group of Experts on the Scientific Aspects of Marine Environmental Protection, vol. 130 . Eds. Kershaw P. J., Turr A., Galgani F. (GESAMP Reports and Studies).
Geyer R., Jambeck J. R., Law K. L. (2017). Production, use, and fate of all plastics ever made. Sci. Adv. 3 (7), e1700782. doi: 10.1126/sciadv.1700782
Heron A. C. (1968). “Plankton gauze,” in Zooplankton sampling. Eds. Tranter D. J., Fraser J. H. (France: United Nations Educational, Scientific and Cultural Organization), 18–24.
Huang Y., Xiao X., Xu C., Perianen Y. D., Hu J., Holmer M. (2020). Seagrass beds acting as a trap of microplastics - Emerging hotspot in the coastal region? Environ. Pollut. 257, 113450. doi: 10.1016/j.envpol.2019.113450
Hung C., Klasios N., Zhu X., Sedlak M., Sutton R., Rochman C. M. (2021). Methods matter: Methods for sampling microplastic and other anthropogenic particles and their implications for monitoring and ecological risk assessment. Integr. Environ. Assess. Manage. 17, 282–291. doi: 10.1002/ieam.4325
Huntington A., Corcoran P. L., Jantunen L., Thaysen C., Bernstein S., Stern G. A., et al. (2020). A first assessment of microplastics and other anthropogenic particles in Hudson Bay and the surrounding eastern Canadian Arctic waters of Nunavut. Facets 5 (1), 432–454. doi: 10.1139/facets-2019-0042
Jambeck J. R., Geyer R., Wilcox C., Siegler T. R., Perryman M., Andrady A., et al. (2015). Plastic waste inputs from land into the ocean. Science 347 (6223), 768–771. doi: 10.1126/science.1260352
Jeyasanta K. I., Patterson J., Grimsditch G., Edward J. K. P. (2020). Occurrence and characteristics of microplastics in the coral reef, sea grass and near shore habitats of Rameswaram Island, India. Mar. Pollut. Bull. 160, 111674. doi: 10.1016/j.marpolbul.2020.111674
Karlsson T. M., Karrman A., Rotander A., Hassellov M. (2020). Comparison between manta trawl and in situ pump filtration methods, and guidance for visual identification of microplastics in surface waters. Environ. Sci. Pollut. Res. 27 (5), 5559–5571. doi: 10.1007/s11356-019-07274-5
Karlsson T. M., Vethaak A. D., Almroth B. C., Ariese F., van Velzen M., Hassellov M., et al. (2017). Screening for microplastics in sediment, water, marine invertebrates and fish: Method development and microplastic accumulation. Mar. Pollut. Bull. 122 (1-2), 403–408. doi: 10.1016/j.marpolbul.2017.06.081
Kreitsberg R., Raudna-Kristoffersen M., Heinlaan M., Ward R., Visnapuu M., Kisand V., et al. (2021). Seagrass beds reveal high abundance of microplastic in sediments: A case study in the Baltic Sea. Mar. Pollut. Bull. 168, 112417. doi: 10.1016/j.marpolbul.2021.112417
Law K. L., Morét-Ferguson S., Maximenko N. A., Proskurowski G., Peacock E. E., Hafner J., et al. (2010). Plastic accumulation in the North Atlantic subtropical gyre. Science 329 (5996), 1185–1188. doi: 10.1126/science.1192321
Le Guen C., Suaria G., Sherley R. B., Ryan P. G., Aliani S., Boehme L., et al. (2020). Microplastic study reveals the presence of natural and synthetic fibres in the diet of King Penguins (Aptenodytes patagonicus) foraging from South Georgia. Environ. Int. 134, 105303. doi: 10.1016/j.envint.2019.105303
Lindeque P. K., Cole M., Coppock R. L., Lewis C. N., Miller R. Z., Watts A. J. R., et al. (2020). Are we underestimating microplastic abundance in the marine environment? A comparison of microplastic capture with nets of different mesh-size. Environ. Pollut. 265, 114721. doi: 10.1016/j.envpol.2020.114721
Loke L. H. L., Clews E., Low E. W., Belle C. C., Todd P. A., Eikaas H. S., et al. (2010). Methods for sampling benthic macroinvertebrates in tropical lentic systems. Aquat. Biol. 10 (2), 119–130. doi: 10.3354/ab00274
Maes T., van Diemen de Jel J., Vethaak A. D., Desender M., Bendall V. A., van Velzen M., et al. (2020). You are what you eat, microplastics in Porbeagle sharks from the North East Atlantic: Method development and analysis in spiral valve content and tissue. Front. Mar. Sci. 7. doi: 10.3389/fmars.2020.00273
Martin C., Baalkhuyur F., Valluzzi L., Saderne V., Cusack M., Almahasheer H., et al. (2020). Exponential increase of plastic burial in mangrove sediments as a major plastic sink. Sci. Adv. 6 (44), eaaz5593. doi: 10.1126/sciadv.aaz5593
Miller M. E., Motti C. A., Hamann M., Kroon F. J. (2023). Assessment of microplastic bioconcentration, bioaccumulation and biomagnification in a simple coral reef food web. Sci. Total Environ. 858, 159615. doi: 10.1016/j.scitotenv.2022.159615
Mizraji R., Ahrendt C., Perez-Venegas D., Vargas J., Pulgar J., Aldana M., et al. (2017). Is the feeding type related with the content of microplastics in intertidal fish gut? Mar. Pollut. Bull. 116 (1-2), 498–500. doi: 10.1016/j.marpolbul.2017.01.008
Nelms S. E., Galloway T. S., Godley B. J., Jarvis D. S., Lindeque P. K. (2018). Investigating microplastic trophic transfer in marine top predators. Environ. Pollut. 238, 999–1007. doi: 10.1016/j.envpol.2018.02.016
Oldenburg K. S., Urban-Rich J., Castillo K. D., Baumann J. H. (2021). Microfiber abundance associated with coral tissue varies geographically on the Belize Mesoamerican Barrier Reef System. Mar. Pollut. Bull. 163, 111938. doi: 10.1016/j.marpolbul.2020.111938
Ormaza-González F. I., Caiza-Quinga R., Cárdenas-Condoy J., Intriago-Basurto A., Piguave-Tarira E. J., Ocaña–Balcázar K. D., et al. (2022). Sampling bottles for shallow estuarine waters, constructed using inexpensive recyclable materials. Estuar. Coast. Shelf Sci. 275, 107965. doi: 10.1016/j.ecss.2022.107965
Omeyer L. C., Duncan E. M., Aiemsomboon K., Beaumont N., Bureekul S., Cao B., et al. (2022). Priorities to inform research on marine plastic Pollution in Southeast Asia. Sci. Total Environ. 841, 156704. doi: 10.1016/j.scitotenv.2022.156704
Ostle C., Thompson R. C., Broughton D., Gregory L., Wootton M., Johns D. G. (2019). The rise in ocean plastics evidenced from a 60-year time series. Nat. Commun. 10 (1), 1622. doi: 10.1038/s41467-019-09506-1
Patterson J., Jeyasanta K. I., Sathish N., Edward J. K. P., Booth A. M. (2020). Microplastic and heavy metal distributions in an Indian coral reef ecosystem. Sci. Total Environ. 744, 140706. doi: 10.1016/j.scitotenv.2020.140706
Patti T. B., Fobert E. K., Reeves S. E., Burke da Silva K. (2020). Spatial distribution of microplastics around an inhabited coral island in the Maldives, Indian Ocean. Sci. Total Environ. 748, 141263. doi: 10.1016/j.scitotenv.2020.141263
Seng N., Lai S., Fong J., Saleh M. F., Cheng C., Cheok Z. Y., et al. (2020). Early evidence of microplastics on seagrass and macroalgae. Mar. Freshw. Res. 71 (8), 922–924. doi: 10.1071/mf19177
Sharifinia M., Bahmanbeigloo Z. A., Keshavarzifard M., Khanjani M. H., Lyons B. P. (2020). Microplastic Pollution as a grand challenge in marine research: A closer look at their adverse impacts on the immune and reproductive systems. Ecotoxicol. Environ. Saf. 204, 111109. doi: 10.1016/j.ecoenv.2020.111109
Sun X., Liu T., Zhu M., Liang J., Zhao Y., Zhang B. (2018). Retention and characteristics of microplastics in natural zooplankton taxa from the East China Sea. Sci. Total Environ. 640-641, 232–242. doi: 10.1016/j.scitotenv.2018.05.308
Thushari G. G. N., Senevirathna J. D. M., Yakupitiyage A., Chavanich S. (2017). Effects of microplastics on sessile invertebrates in the eastern coast of Thailand: An approach to coastal zone conservation. Mar. Pollut. Bull. 124 (1), 349–355. doi: 10.1016/j.marpolbul.2017.06.010
Tsuchiya M., Nomaki H., Kitahashi T., Nakajima R., Fujikura K. (2019). Sediment sampling with a core sampler equipped with aluminum tubes and an onboard processing protocol to avoid plastic contamination. MethodsX 6, 2662–2668. doi: 10.1016/j.mex.2019.10.027
Watkins L., Sullivan P. J., Walter M. T. (2021). What you net depends on if you grab: A meta-analysis of sampling method's impact on measured aquatic microplastic concentration. Environ. Sci. Technol. 55 (19), 12930–12942. doi: 10.1021/acs.est.1c03019
Woodall L. C., Sanchez-Vidal A., Canals M., Paterson G. L., Coppock R., Sleight V., et al. (2014). The deep sea is a major sink for microplastic debris. R. Soc Open Sci. 1 (4), 140317. doi: 10.1098/rsos.140317
Xu X., Wong C. Y., Tam N. F. Y., Lo H. S., Cheung S. G. (2020). Microplastics in invertebrates on soft shores in Hong Kong: Influence of habitat, taxa and feeding mode. Sci. Total Environ. 715, 136999. doi: 10.1016/j.scitotenv.2020.136999
Zamprogno G. C., Canicali F. B., Cozer C. D. R., Otegui M. B. P., Graceli J. B., Costa M. B. D. (2021). Spatial distribution of microplastics in the superficial sediment of a mangrove in Southeast Brazil: A comparison between fringe and basin. Sci. Total Environ. 784, 146963. doi: 10.1016/j.scitotenv.2021.146963
Keywords: contamination, equipment, method, monitoring, plastic pollution, quality assurance, technique
Citation: Todd PA, Yong CLX, Foo SH, Ying LSM and Ledet J (2024) Plastic-less equipment for sampling marine microplastics. Front. Mar. Sci. 11:1345591. doi: 10.3389/fmars.2024.1345591
Received: 28 November 2023; Accepted: 22 January 2024;
Published: 13 February 2024.
Edited by:
Marina F. M. Santana, Australian Institute of Marine Science (AIMS), AustraliaReviewed by:
Mohamed Mohsen, Jimei University, ChinaAndrea Augusto Sfriso, University of Ferrara, Italy
Copyright © 2024 Todd, Yong, Foo, Ying and Ledet. This is an open-access article distributed under the terms of the Creative Commons Attribution License (CC BY). The use, distribution or reproduction in other forums is permitted, provided the original author(s) and the copyright owner(s) are credited and that the original publication in this journal is cited, in accordance with accepted academic practice. No use, distribution or reproduction is permitted which does not comply with these terms.
*Correspondence: Peter A. Todd, ZGJzcGF0QG51cy5lZHUuc2c=; Clara Lei Xin Yong, eW9uZy5seEB1Lm51cy5lZHU=
†These authors have contributed equally to this work