- 1Tropical & Subtropical Research Center, Korea Institute of Ocean Science & Technology, Jeju, Republic of Korea
- 2Jeju Research Institute, Korea Institute of Ocean Science & Technology, Jeju, Republic of Korea
- 3Office of the President, Korea Institute of Ocean Science & Technology, Busan, Republic of Korea
Aim of study: Marine climatic transition zones are boundary areas of major climate zones, here the boundary between the subtropical and temperate zones. They present areas containing high abundance of organisms living at the limit of their physiological tolerance. These marginal populations are particularly sensitive to changes in their environment. As such, marine climatic transition zones are excellent natural playgrounds for climate change-related hypothesis testing, especially with respect to marine habitat response to ocean warming. The marginal biogenic habitats around Jeju Island, South Korea, which lies within the temperate transition zone, have gradually changed from macroalgal-dominated to hard coral-dominated habitats. Understanding the specific abiotic environmental factors that influence the distribution of the marginal populations in temperate transition zones (i.e., species at their occurrence limit) is crucial to predicting and managing temperate zone habitat changes caused by climate change. This study aims to identify the specific abiotic environmental factors that contribute to explaining the current spatial distribution of the declining temperate and expanding subtropical foundation species in Jeju waters.
Methods: Coverage and composition of sessile benthic communities were determined by photo-quadrat analysis at two depths (10 m and 15 m) at three sites along the island’s south, east, and north coasts in May and November 2022. Divergences in community composition between sites were characterized in light of ten quantitative environmental parameters.
Results: Our results show that sessile foundation communities vary significantly at different sites around the island. While the south is defined by high-latitude hard corals, predominately Alveopora japonica, the east is defined by the temperate canopy-forming macroalga Ecklonia cava, and the north is characterized by coralline algae. Winter sea surface temperature, water transparency, nutrient concentration, and water movement were statistically the most impactful environmental factors determining which foundation species constitute each distinct benthic community.
Conclusion: This study provides valuable baseline information on the impacts of abiotic environmental factors on marine sessile communities in a temperate transition zone.
1 Introduction
Marine climatic transition areas are geographically located between two separated climate zones, but they display a combination of characteristics from both. This leads to a high environmental variability that promotes the co-occurrence of different marine communities (Ferro and Morrone, 2014; Shimabukuro et al., 2023). These communities are considered marginal communities, describing the fact that they experience environmental conditions near their physiological limit, which makes them particularly vulnerable to changes in environmental conditions, biotic interactions, and local extinctions (Kawecki, 2008; Wernberg et al., 2016; Agostini et al., 2018; Soares, 2020). Jeju Island, the southernmost region of South Korea (33°23.75’ N, 126°33.42’ E), is a temperate transition zone, as it is part of the Temperate Northern Pacific ecoregion, but exhibits subtropical characteristics due to the Kuroshio Current, a massive, subtropical current that brings warm, oligotrophic waters to the region (Sakamoto et al., 2005; Spalding et al., 2007; Wu et al., 2012). In fact, Jeju Island lies between two climatic zones with alternating influence depending on the season: the “polar dry” in winter and “tropical/subtropical moist” (due to a short monsoon) in summer (Shimabukuro et al., 2023). Additionally, Jeju Island is frequently affected by typhoons (Park et al., 2006; Lee K.-T. et al., 2023). Over the past half a century, the coastal waters of Jeju, and the Korean mainland in general, have recorded a rise in annual sea surface temperature (SST) by +1.23°C, 2.5 times the global average (Belkin, 2009; Wu et al., 2012; Hobday and Pecl, 2014 ; Han and Lee, 2020; Tang et al., 2020). Takatsuki et al. (2007) reported an increase in Jeju winter SST by 2.1°C per century from the early 20th century until 2007, while Kim T. et al. (2022) reported a rise in Jeju winter SST of 3.6°C over the last 36 years (compared to just 0.7°C in summer). This trend is associated with an increase in heat wave- and typhoon-frequency and -intensity (Oouchi et al., 2006; Park et al., 2006; Lee S. et al., 2023), highlighting Jeju Island’s significant position as a climate change hotspot (Hobday and Pecl, 2014). All these circumstances lead to a large portion of Jeju’s marine life being highly susceptible to environmental changes, especially benthos with limited mobility and dispersal abilities, such as sessile species.
Although biotic interactions, anthropogenic pressures, and general environmental conditions all determine benthic marine community composition, including species distribution and diversity dynamics (Sousa, 1984; Hillebrand, 2004; Campbell et al., 2014; Pinsky et al., 2020), water temperature is largely accepted as a primary factor in structuring species distribution (Sunday et al., 2012; Tanaka et al., 2012; Ribas-Deulofeu et al., 2023). Therefore, marine climatic transition zones are expected to undergo severe changes in their biogenic habitats as a consequence of global ocean warming. Changes in marine community composition, detrimental to native temperate species, such as increasing numbers of species with tropical affinities and species of turf-forming algae, have already been observed in high-latitude areas worldwide, for example, in the Atlantic Ocean along the Portuguese continental coast (de Azevedo et al., 2023), the west Pacific Ocean and East China Sea along the Japanese coast (Yamano et al., 2011), and the Indian Ocean along the western Australian coast (Wernberg et al., 2016). This settlement of subtropical and tropical species in temperate ecosystems also raises concerns about the management of marine systems, as it may be too difficult to return to previous states (Hobbs et al., 2006; Beger et al., 2014; Makino et al., 2014; Pecl et al., 2017). Moreover, prominent changes in foundation species, such as a kelp species, can have cascading consequences for the entire community (Vergés et al., 2014) by altering biotic interactions and modifying the habitat, hence the goods and services the ecosystem provides (Kang, 2010; Pecl et al., 2017; Aguilar et al., 2022). Until the late 1980s, Jeju Island’s benthic ecosystem was dominated by temperate kelp forests, especially the native species Ecklonia cava (Kang, 2010). This foundation species plays a critical role for associated organisms, providing shelter, food, and nursery grounds (Steneck et al., 2002; Filbee-Dexter and Wernberg, 2018). The fishing industry in South Korea, especially on Jeju Island, relies heavily on kelp forest habitats to sustain economically valuable species (Kang, 2010, 2011; Hwang et al., 2013). Previous changes in canopy-forming algae coverage have reportedly decreased commercial animal species catches (Serisawa et al., 2004; Kang, 2010). Currently, the E. cava population around Jeju Island is declining and being replaced by a variety of species, especially crustose coralline algae (CCA), leading to the formation of a habitat type known as “barren grounds” (Kim, 2006; Kang, 2010). This phenomenon, called “getnoguem” in Korea, is similar to “isoyake” in Japan (Fujita, 2010; Lee et al., 2022). Barren grounds are characterized by large areas of bare rock, extensively covered by CCA, and exhibiting low biodiversity (Serisawa et al., 2004; Kang, 2010; Uribe et al., 2015). Around Jeju Island, barren ground coverage has increased by nearly 11% over a 5-year period between 1998 and 2003, at which point it was covering 4541 hectares of coastal seafloor (Kim, 2006).
This study aims to investigate the distribution of marine benthic communities in Jeju waters with regard to local environmental conditions. As such, focus was laid on dominant sessile macro-foundation species, such as canopy-forming algae (characterizing kelp forests), coralline and turf-forming algae (characterizing barren grounds), hard corals (characterizing high-latitude coral habitats), soft corals (characterizing soft coral beds), and sponges and bryozoans (characterizing sessile invertebrate macrofauna habitats). To determine how site-specific local stressors and hydrographic parameters may influence marginal marine communities, three sites from different parts of the island were chosen to ensure an extensive range of biotic and abiotic data. At each site, sessile communities at two specific water depths were analyzed to determine potential depth-related changes in species coverage and composition, as depth is directly correlated with light and nutrient availability and, as such, impacts the vertical zonation of many marine benthic species, especially photosynthetic organisms and organisms sensitive to nutrient flux and chlorophyll content, respectively (Kang and Kim, 2012; Muir et al., 2015). This study provides valuable insights into the primary abiotic environmental factors that can structure coastal benthic communities on an annual scale in a marine climatic transition zone.
2 Materials and methods
2.1 Study site
This study was conducted at three sites around Jeju Island: Sinheung (SH) in the northeast (33°33.5173’ N, 126°39.1891’ E), Seongsan (SS) in the east (33°27.2356’ N, 126°56.5033’ E), and Bomok (BM) in the south (33°14.2995’ N, 126°35.3935’ E) (Figure 1B). Globally, Jeju Island is surrounded by the Yellow Sea in the east, the East/Japan Sea in the west, and the East China Sea in the south (Figure 1). Jeju Island’s marine environment is strongly influenced by the Kuroshio Current (Figure 1A), which is characterized by high temperature, high salinity, and low dissolved oxygen values (Park, 1986; Choe, 1988). Specifically, the Tsushima Warm Current (TWC; Figure 1A), a warm and saline surface branch of the Kuroshio Current, which originates at 31°N and flows northward reaching the southern coast of Jeju Island, has a strong influence during summer (Lie et al., 1998; Hur et al., 1999; Cha and Moon, 2020). The majority of the TWC passes southeast of the island, flowing eastward up to the Tsushima Strait, while a minor branch flows westward and turns clockwise in the Jeju Strait as Jeju Warm Current (Teague et al., 2003; Cha and Moon, 2020) (Figure 1A). However, coastal circulation is primarily driven by tidal currents, particularly in the Jeju Strait, north of the island (Cha and Moon, 2020). These regional impacts are measurable, leading to distinct environmental conditions between the north and the south of the island, with a conspicuous thermal north/south gradient expressed by average annual water temperatures of 18.8 ± 4.5°C and 20.0 ± 4.6°C, respectively (average SST from 2004 to 2022; Korea Marine Environment Management Corporation, http://data.kma.go.kr/, accessed on 08 March 2023). Despite these local differences, environmental conditions present a strong seasonality. In winter, the coastal waters are mainly influenced by strong and cold Siberian winds, cooling down the water column (Hur et al., 1999). In summer, the fresh discharge of the China-based Changjiang River combined with the summer monsoon accentuates the stratification of Jeju waters by reducing sea surface salinity (Park, 1986; Hur et al., 1999) (Figure 1A). Despite the natural differences in water mass-driven conditions, each site is, in varying degrees, affected by locality-specific anthropogenic stressors. The northern SH site is located within proximity of three fish farms (distance of 890 m to 1331 m), while the eastern SS site is far from any obvious artificial effluents. The southern BM site is just 350 m from a sewage treatment plant.
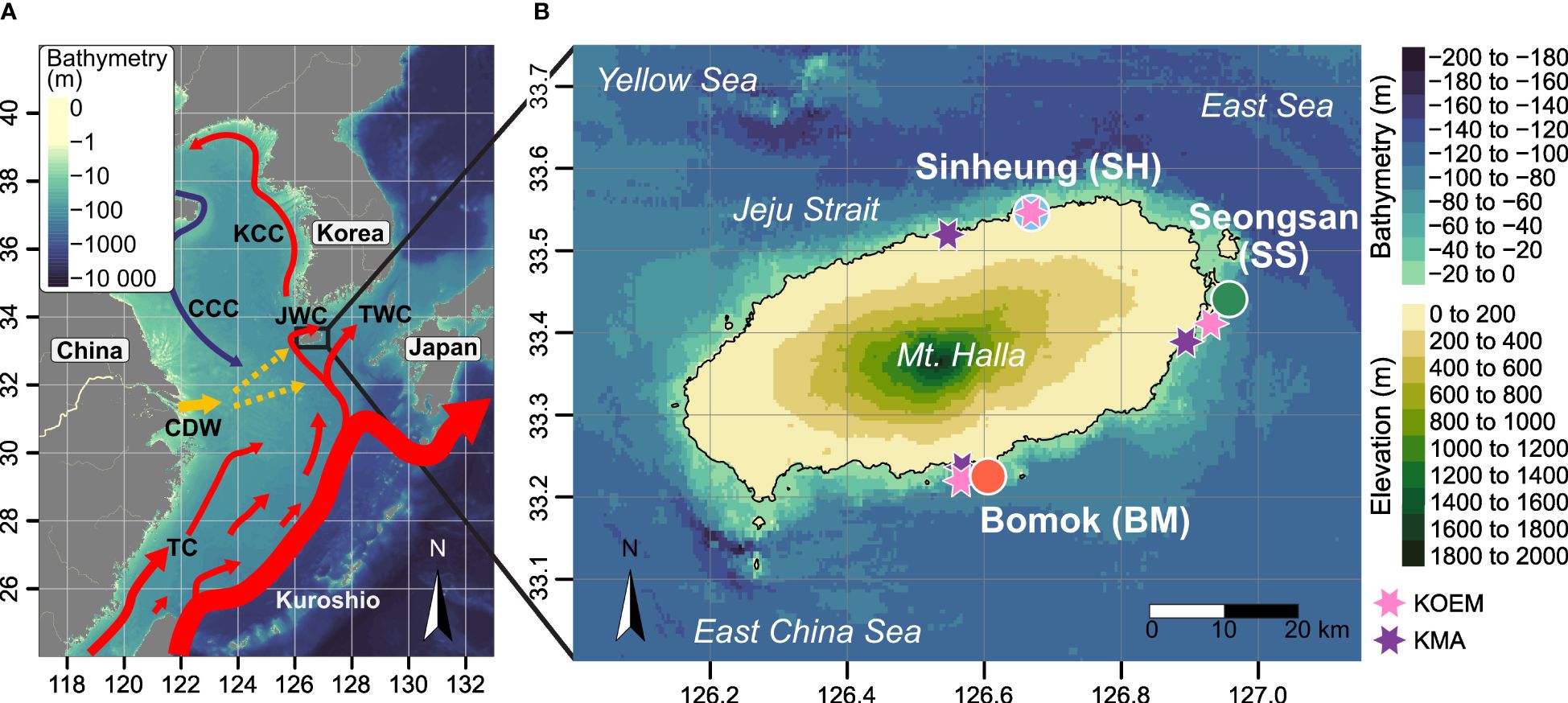
Figure 1 (A) Map of the study region and the main currents (based on Park et al., 2017; Figure 4A). CDW, Changjiang Discharge Water; CCC, Chinese Coastal Current; JWC, Jeju Warm Current; KCC, Korean Coastal Current; TC, Taiwan Warm Current; TWC, Tsushima Warm Current. (B) Map of study sites on Jeju Island, South Korea. Dots represent sites with permanent 100-m line transects at 10 m and 15 m depth. Purple stars are KMA stations (wind speed and direction data), and pink stars are KOEM stations (all other environmental data). Bathymetry and elevation data are from NOAA. Coastline coordinates are from GADM website (accessed on 17 November 2023).
2.2 Environmental variables
All abiotic environmental variables applied in this study were extracted from publicly available websites. Hydrographic variables are available from the Korea Marine Environment Management Corporation website (KOEM, n.d.) , while meteorological variables are available from the website of the Korean Meteorological Administration (KMA, n.d.). Data from 2004 (beginning of systematic record at the sites) to 2022 were used. Hydrographic variables (KOEM data) were collected quarterly (i.e., February, May, August, November; one data point each) by boat survey from coastal surface waters at each site (1.5–2.5 km from the coastline) (Figure 1B). KOEM parameters were measured using a CTD-rosette sampler followed by laboratory analyses (Korea Marine Environment Management Corporation, 2019). KMA variables were recorded hourly at each site from ground atmospheric observatories (Synopsis Weather Observations - ASOS program). Consequently, for meteorological variables, the monthly average for February, May, August, and November, respectively, was computed to also gain one data point for each month. Recording locations closest to the coordinates of our respective sampling site were chosen (indicated by stars on the map; max. distance to study site: 10 km; Figure 1B).
A total of 10 variables were chosen for statistical analyses, 9 hydrographic variables and 1 meteorological variable: Chlorophyll a (Chla; μg L-1), Dissolved Inorganic Nitrogen (DIN; μg L-1), Dissolved Inorganic Phosphate (DIP; μg L-1), Dissolved Oxygen (DO; mg L-1), pH, Salinity (PSU), Suspended Organic Matter (SOM; mg L-1), Sea Surface Temperature (SST; °C), Transparency (m) (from KOEM); and Wind Speed (WS; m s-1) (from KMA). An additional 4 variables were considered for discussion purposes: Nitrite (NO2-; μg L-1), Nitrate (NO3-; μg L-1), Ammonia (NH4+; μg L-1) (from KOEM); and Wind Direction (WD; degrees) (from KMA). Nutrient concentration-related variables (DIN, DIP, NO2-, NO3-, NH4+) were converted to μmol L-1 based on the corresponding molar masses to facilitate comparisons to the literature. Wind speed and direction were used as proxies for water movement and surface currents (Charlier, 2005; Skirving et al., 2006).
2.3 Benthic community
Two surveys were carried out by scuba diving in May and November 2022, respectively. These months were chosen to avoid extreme summer and winter environmental values, in order to reduce seasonal variations in the data set and obtain a representative picture of benthic communities by site. Indeed, May and November show temperatures close to the annual average (Supplementary Table 1), and this study focuses on dominant foundation macro-species at each site, all of which have a perennial life cycle and an overall annual stable coverage (Haroun et al., 1989; Vieira et al., 2016). At each site, permanent 100-m line transects were installed at 10 m and 15 m depth, respectively, parallel to the coastline following each isobath. The 10 m and 15 m depths were chosen according to the vertical distribution of dominant benthic species around Jeju Island (Supplementary Table 2) and to avoid intertidal and sand bottom zones, respectively. The transects were installed in a “zigzag” fashion to cover a wider area (Supplementary Figure 1). Along the transects, 100 photos (1 x 1 m) were taken at each site and depth using an underwater camera (Sony ILCE-7RM3A with underwater housing; maximum resolution of 7952 × 5304 pixels). The “zigzag”-scheme ensures that the one photo taken at every meter of the respective permanent transect does not overlap with the next. The camera was mounted on a tetrapod quadrat to maintain a constant distance from the substrate and ensure comparable pictures. At the SH-10m site, data are missing for the November 2022 survey because typhoon Hinnamnor destroyed the permanent line transect at that depth in September 2022.
Dark, blurred, and poorly framed images were excluded from the photo stack (n = 12 in May, and n = 8 in November). From the remaining photos, 30 images (i.e., replicates) were randomly selected using the “base::sample” function in R from each “site-depth-month” group (e.g., BM-10m-May; hereafter called “group”) for image annotation. The PhotoQuad® software (version 1.4; Trygonis and Sini, 2012) was used to identify and quantify benthic organisms and bare substrate in each group following our benthic community categories (Supplementary Table 3). The relative percentage cover of the benthic community in each photo-quadrat was estimated using the software’s stratified random points count tool (Figure 2). On each image, a point was randomly placed on each of the 100 grid cells (i.e., 100 points), and the underlying benthic organism and substrate type (i.e., sand, gravel and rock) was identified to the lowest possible taxonomic level (Supplementary Table 3; except for coralline and turf algae) based on visual information, such as texture, color, and shape. Taxonomic identification was mainly based on fieldwork taxonomic books of Jeju and Japanese coasts (Masuda et al., 1986; Sugihara et al., 2014; Kim M.-S. et al., 2022). Indeterminate annotations and motile benthic organisms, such as asteroids and other echinoderms, polychaetes, holothurians, bivalves, and gastropods were removed before statistical analyses. Sand, gravel, and rock annotations were grouped in the “Substrate” category, which was included in the analysis as the only non-biological component because of its essential role in habitat characterization. Based on the overall species percentage distribution in our data set, a species or non-biological component was described as “major” when its coverage was greater than 2.5% in a group. “Dominance” was defined as a coverage over 25% by a single species or category in a group (e.g., BM-10m-May), following Reimer et al. (2021). All remaining species (cover < 2.5%) were merged into the “Other” category. Coverages were reduced to 100% at the group level. As the dense kelp cover obstructs the vision of underlying species, this photo-quadrat method cannot be used to study species-specific coverages and biodiversity in the presence of canopy-forming algae, and percentage cover data have been interpreted cautiously. All species categories used to classify benthic communities in the image annotations are listed in Supplementary Table 3.
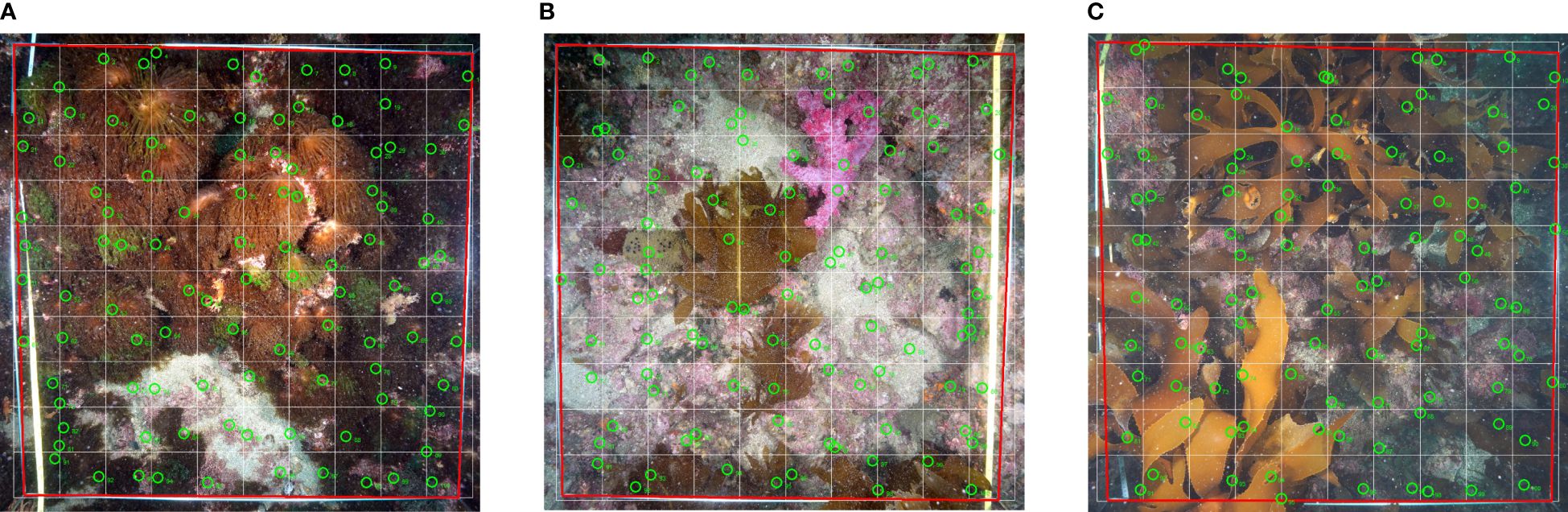
Figure 2 Examples of photo-quadrat annotations on PhotoQuad® of (A) Bomok site at 15 m, (B) Sinheung site at 15 m, and (C) Seongsan site at 15 m, in May. The stratified random points count method (100 points per image) was used to assess the relative percentage cover (%) of benthic communities.
2.4 Statistical analyses
All statistical analyses were performed using R (version 4.3.0).
2.4.1 Environmental variables
Statistical analyses were carried out for each month independently (i.e., February, May, August, and November, corresponding to four time series of 18 points each) to eliminate seasonal variability in the results. Each monthly time series was then detrended using linear regression to remove the inter-annual trend, thus obtaining each site’s “average” environmental conditions and avoiding masking spatial variability. The median of each monthly time series was summed with the regression’s residuals (Supplementary Figure 2). The resulting data (hereafter called “environmental data”) were used in further statistical analyses.
A Principal Component Analysis (PCA) was performed on the ten standardized abiotic variables selected to assess spatial divergences in environmental conditions. The PCA distance biplot (i.e., scaling 1) was used to visualize the most impactful environmental variables contributing to the spatial data ordination. Furthermore, to test the effect of space (i.e., site) and time (i.e., year) on environmental conditions, a non-parametric Multivariate Analysis of Variance (PERMANOVA; vegan::adonis2) was carried out (Anderson, 2001). A Kruskal-Wallis test (KW) was performed on each variable to specifically determine which environmental parameters vary according to the factor “site” (Kruskal and Wallis, 1952). When the test was significant, a Dunn’s post-hoc test with a Bonferroni correction for multiple comparisons was performed (Dunn, 1964).
2.4.2 Benthic community
Multivariate analyses were conducted at the species level. The percentage cover matrix of the benthic community was transformed using Hellinger’s method to reduce the weight of rare and absent species (Legendre and Gallagher, 2001).
To investigate which spatiotemporal factor (i.e., “site”, “depth”, and “month”) statistically influences benthic communities the most, partial Redundancy Analysis (pRDA) was carried out (Legendre and Legendre, 2012). The pRDA searches for the variability in the community matrix explained by a single, main explanatory factor (i.e., the constraining factor) by removing the variability induced by additional explanatory variables (i.e., the conditioning factors). In this study, the factors “site” (i.e., BM, SH, SS), “month” (i.e., May, November), and “depth” (i.e., 10 m, 15 m) were each, in turn, considered as the main explanatory factor, resulting in three pRDA models. The RDAx axes indicate the proportion of variation explained by the pRDA model, while PCx axes indicate the proportion of variation in the residuals (which is not explained by any model). After each pRDA, a permutation test (nperm = 999) was used to test for the analysis’ significance and each RDA axis’ significance. In addition, the effect of each spatiotemporal factor without interaction (i.e., “site”, “depth”, and “month”, respectively) on the percentage cover of the benthic community was tested using PERMANOVA. The test was performed using the Hellinger distances of the percentage cover matrix, with 9999 permutations. For the factor “site”, as it contains three levels, as soon as a significant difference between benthic communities was detected, a post-hoc, pairwise PERMANOVA test (pairwiseAdonis::pairwise.adonis) with Bonferroni significance correction was conducted to identify which site was responsible for the difference. The role of biotic interactions in shaping benthic communities was excluded from the scope of this paper.
3 Results
3.1 Environmental variables
To determine and illustrate the weight of each abiotic variable in setting the environmental conditions around Jeju Island, PERMANOVA, KW tests, and PCA were applied. Environmental variables clearly clustered according to sites, with distinct seasonal differences, largest between November and February (Table 1A; Figure 3). In November, clustering appeared along the primary PCA axis only, while in February, clustering appeared along primary (PC1: 20.9% of the variance) and secondary (PC2: 17.4% of the variance) axes, representing 38.3% of the total February variance (Figures 3A, D). The first principal component in the November graph (PC1: 29.4% of variance) was related to salinity, SST, transparency, DIN, DIP, and WS. In the February graph, the first principal component was related to SST and DIP, and the second principal component was related to WS and transparency. The driving environmental variables of the site variability, therefore, are season-dependent, consisting of temperature (SST), phosphorus levels (DIP), WS, and transparency in February, and salinity, SST, phosphorus and nitrogen levels (DIP and DIN), transparency, and WS in November. Although no distinct site-specific clustering was discernible in May and August in the PCA, the PERMANOVA, KW, and Dunn tests indicated significant differences (Figures 3B, C, 4; Table 1): in August, DO was lower at SH (mean ± SD = 7.3 ± 0.7 mg L-1) than at BM (7.6 ± 0.6 mg L-1) and SS (7.7 ± 0.7 mg L-1), while in May, the DIN concentration was lower at SS (median = 0.7 μM) than at BM (1.1 μM) and SH (1.0 μM) (Supplementary Table 1). WS was always significantly lower at BM, especially in November and February (Figure 4E). The wind directions also differed between sites and months (Supplementary Figure 3). In February and November, strong north-westerly winds dominated at SS and SH (February mean ± SD = 3.5 ± 0.1 m s-1 and 3.7 ± 0.1 m s-1, respectively), with maximal gusts of up to 4.2 m s-1 and 4.3 m s-1, respectively (Supplementary Figures 3B, C). In contrast, BM was subject to light winds, mainly from the north (max. 3.2 m s-1; mean ± SD = 2.4 ± 0.1 m s-1) (Supplementary Figure 3A). In May and August, the wind regime shifted. BM experienced winds from the southwest and the northeast, while wind directions at SS and SH were similar and highly variable (Supplementary Figure 3).
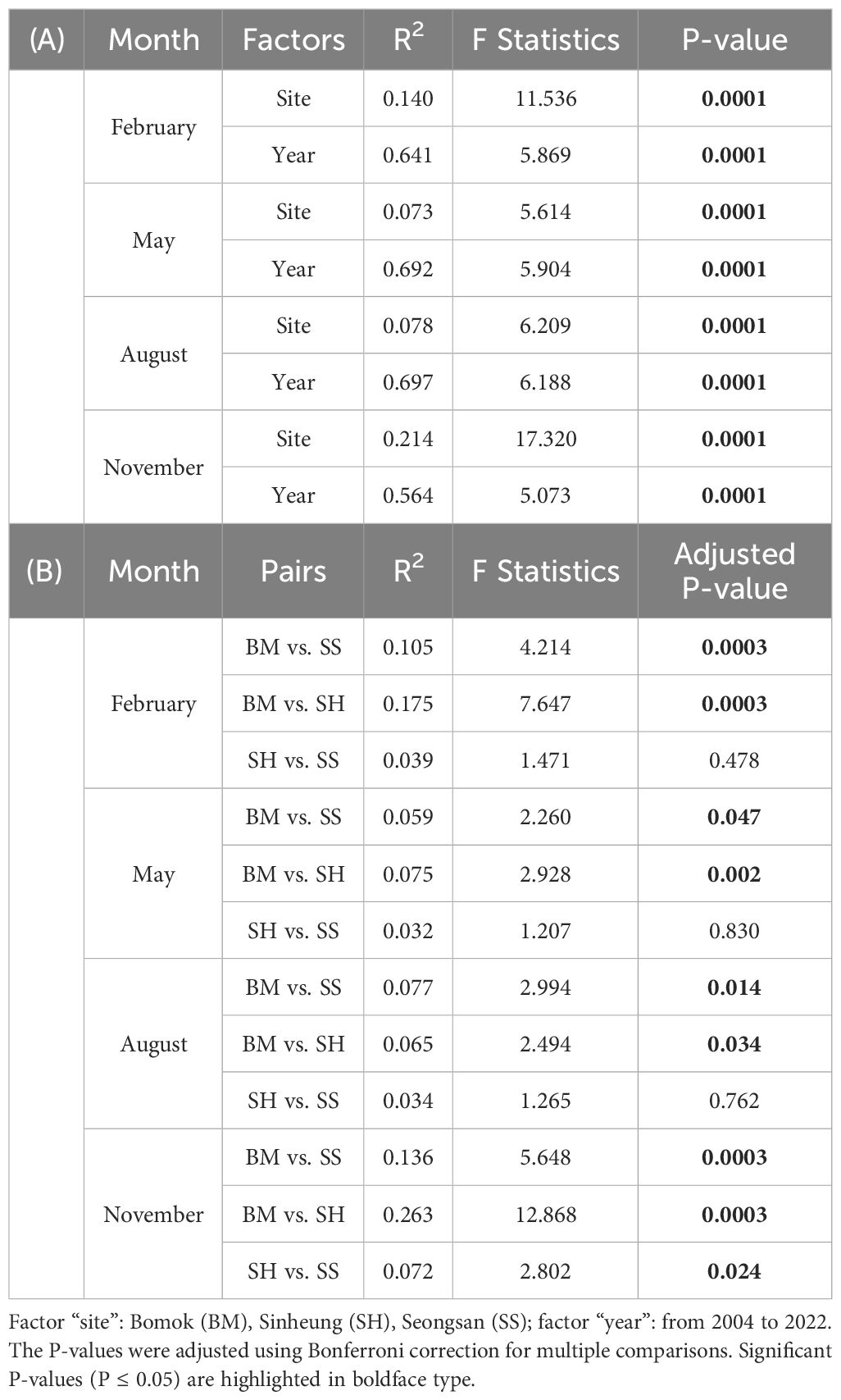
Table 1 (A) PERMANOVA test results from Euclidean distance matrix of the 10 standardized environmental data. (B) Pairwise PERMANOVA test results for the factor “site”.
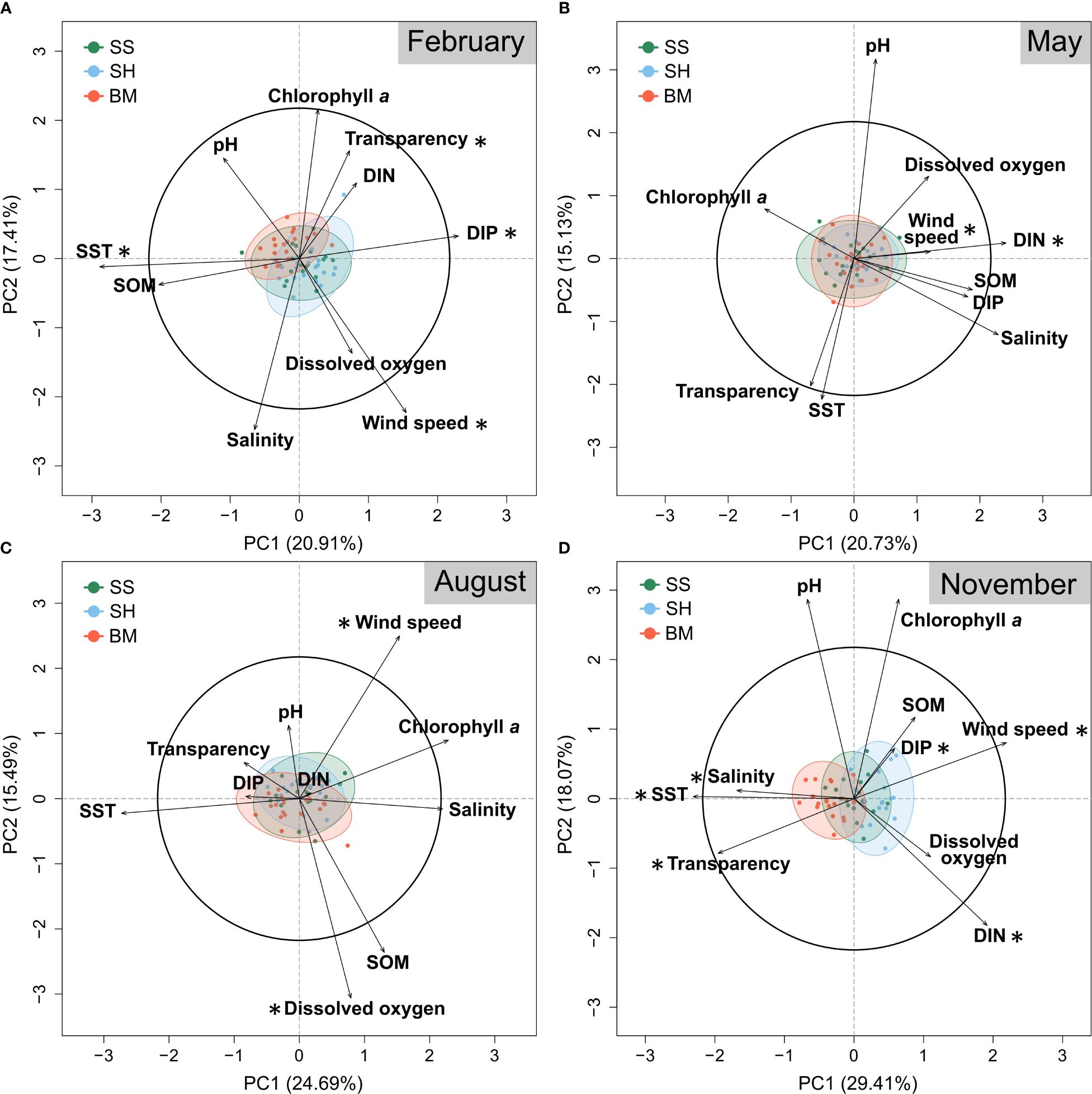
Figure 3 Principal Coordinate Analysis (PCA) biplot – scaling 1 – of the standardized environmental data from 2004 to 2022 of (A) February, (B) May, (C) August, and (D) November. Each dot represents a year. Red: Bomok (BM); Blue: Sinheung (SH); and Green: Seongsan (SS). The ellipses are the square-root chi-squared density distributions of objects with quantile = 0.95. The black asterisks indicate significantly different variables according to sites using the non-parametric Kruskal-Wallis test. The black circle is the equilibrium circle of descriptors. DIN, Dissolved Inorganic Nitrogen; DIP, Dissolved Inorganic Phosphate; SOM, Suspended Organic Matter; SST, Surface Seawater Temperature.
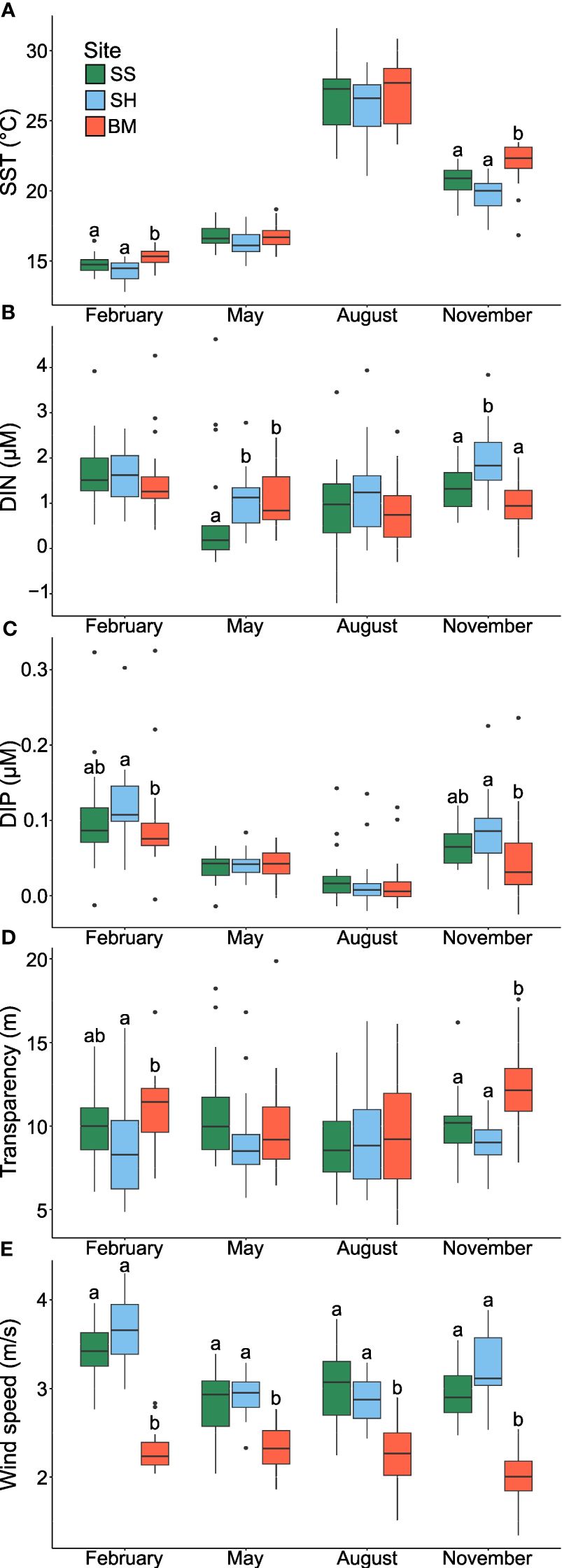
Figure 4 Boxplots by month and site of (A) Surface Seawater Temperature (SST, °C), (B) Dissolved Inorganic Nitrogen (DIN; µM), (C) Dissolved Inorganic Phosphate (DIP; µM), (D) transparency (m), and (E) wind speed (ms-1). Red: Bomok (BM); Blue: Sinheung (SH); and Green: Seongsan (SS). Letters indicate the results of the non-parametric pairwise Dunn test in cases where the Kruskal-Wallis test was significant.
Regardless of season (i.e., months), BM was consistently distinct from SS and SH (Table 1B). Specifically, November and February DIN and DIP concentrations were significantly lower at BM (November mean ± SD = 1.2 ± 0.8 μM and 0.07 ± 0.06 μM, respectively) than at SH (November mean ± SD = 1.8 ± 0.9 μM and 0.09 ± 0.05 μM, respectively) (see also Figure 4 and Supplementary Table 1). November and February transparency and SST were significantly higher at BM (November mean ± SD = 12.6 ± 2.7 m and 21.5 ± 1.7°C, respectively) than at SS (November mean ± SD = 10.4 ± 2.2 m and 20.5 ± 1.2°C, respectively) and SH (November mean ± SD = 8.8 ± 1.5 m and 19.5 ± 1.3°C, respectively). In contrast, SS and SH exhibited generally similar environmental conditions (Table 1B). However, although no statistically significant trend in nutrient concentration-related measurements (i.e., DIP, DIN, NO2-, NO3-, and NH4+) could be established, it is noteworthy that the mean November concentrations were consistently highest at SH (Supplementary Table 1).
3.2 Benthic community
Forty-six benthic taxa and one non-biological component were identified, 16 of which were considered major (percentage cover > 2.5%) (Supplementary Tables 3, 4). All three sites were dominated (percentage cover > 25%) by a single species or category (Figure 5). Generally, the wide range of species coverage percentages (Supplementary Table 5) combined with very high standard deviations (when compared to the mean percentage cover of each species by group; Supplementary Table 4) mirror a patchy distribution of benthic species. Sixteen species were present at all three sites in variable percentages, including coralline algae, turf-forming algae, and the encrusting rhodophytes Peyssonnelia sp. and Hildenbrandia sp. that had high coverages at all three sites (Supplementary Tables 4, 6). However, regardless of depth and season, coralline algae species strongly dominated at SH, with CCA showing coverages between 7.5% and 62.0% per image and geniculate coralline algae between 0% and 51.0%. At SS and BM, respectively, CCA coverage ranges of 0–48.3% and 4.0–57.0%, and geniculate coralline algae coverage ranges of 0–35.4% and 0–42.9% were recorded (Supplementary Table 5). Interestingly, SH had the lowest coverage of bare substrate (mean ± SD = 2.5 ± 3.5%) compared to SS and BM (5.6 ± 8.5% and 5.3 ± 7.6%, respectively). SH also showed highest coverage percentages in recorded bryozoans (max. 6.5%) and sponges (max. 12.5%), as well as sponge diversity (7 species) (Supplementary Tables 4, 5). At BM, only 4 Porifera species (max. 1.1%), and at SS, only 3 Porifera species (max. 6.7%) were recorded (Supplementary Tables 4, 5). Additionally, highest diversity in soft and fire corals was recorded from SH, including one species of sea anemone (Heteractis sp., max. 2.2%), one species of thick-polyp-bearing soft coral (Dendronephthya gigantea, max. 36.1%), and two species of fire corals (Solanderia sp., max. 1.1%; Aglaophenia pluma, max. 6.7%) (Supplementary Table 5). At SS, although environmentally most similar to SH, no soft corals were recorded. At BM, two species of sea anemone were recorded (Entacmaea sp., max. 10.3%; Heteractis sp., max. 4.1%) (Supplementary Table 5). Scleractinian hard corals were present at all three sites, although the highest diversity (4 species) and, by large, the highest coverage percentage (sum by site = 54.7 ± 25.1%) were recorded from BM, followed by SS (3 species; sum by site = 0.5 ± 1.9%), and SH (2 species; sum by site = 0.06 ± 0.5%) (Supplementary Table 4). At BM at both depths, Alveopora japonica dominated by over 60% in May and 30% in November (Figure 5B; Supplementary Table 4). Recently, it has been suggested that there are three cryptic species of Alveopora japonica corresponding to their Japanese, Korean and Taiwanese distributions respectively (Kang et al., 2020). At SS at both depths, canopy-forming brown algae (Ecklonia cava and Sargassum spp.) dominated by over 65% in May and 23% in November. At SH at both depths, coralline algae (geniculate coralline algae and CCA) dominated by over 40% in May and 66% in November (only 15 m depth data available). These differences in dominance are highlighted by multivariate analyses, showing a clear difference in benthic percentage cover between sites (Figure 6A; Tables 2, 3).
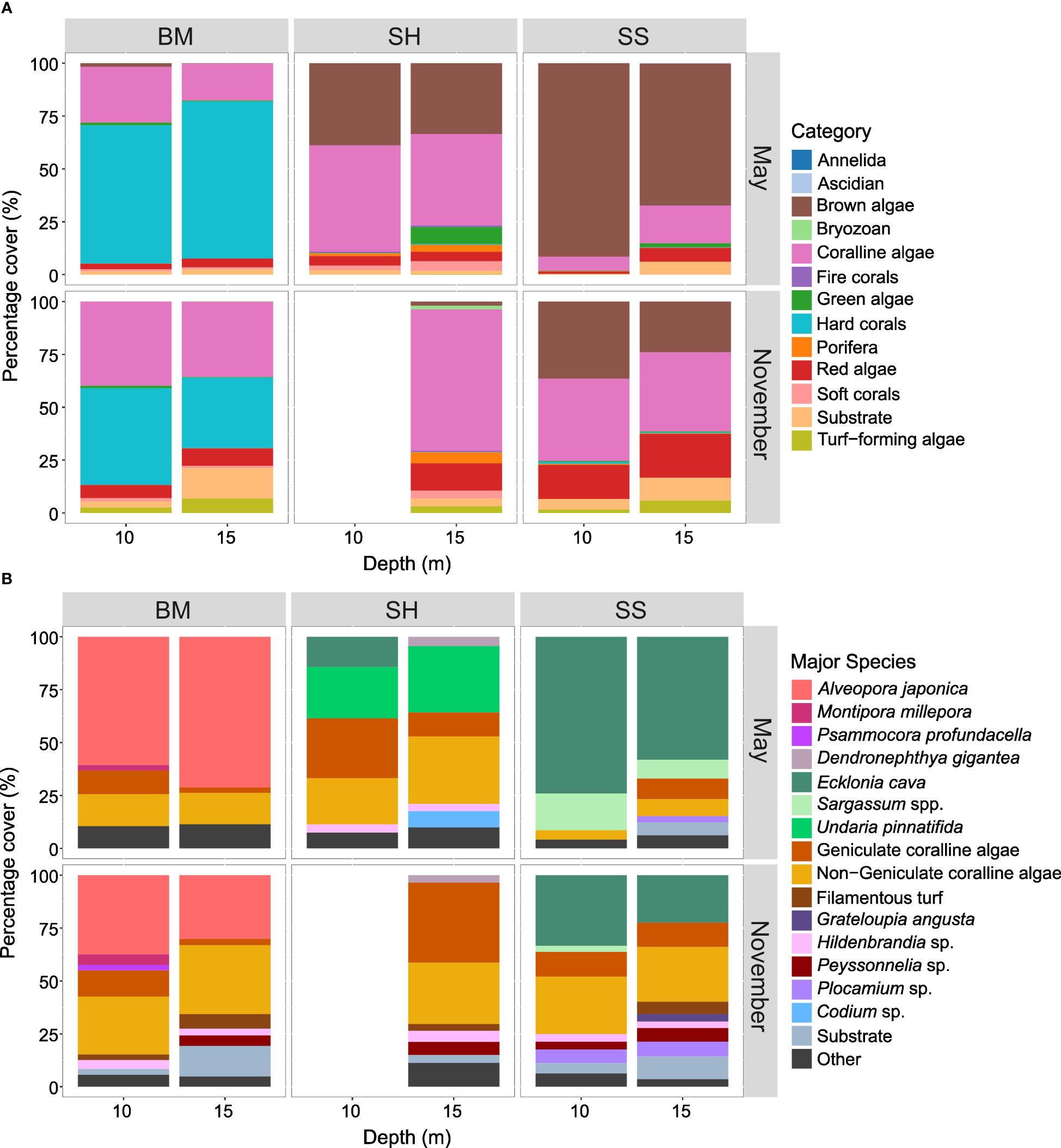
Figure 5 Percentage cover (%) of (A) categories and (B) major species/substrate for each site and depth in May and November 2022. BM, Bomok; SH, Sinheung; SS, Seongsan. In (B), species with a percentage cover < 2.5% in each column are summed in the group “Other”. The data are missing at the SH site at 10 m in November.
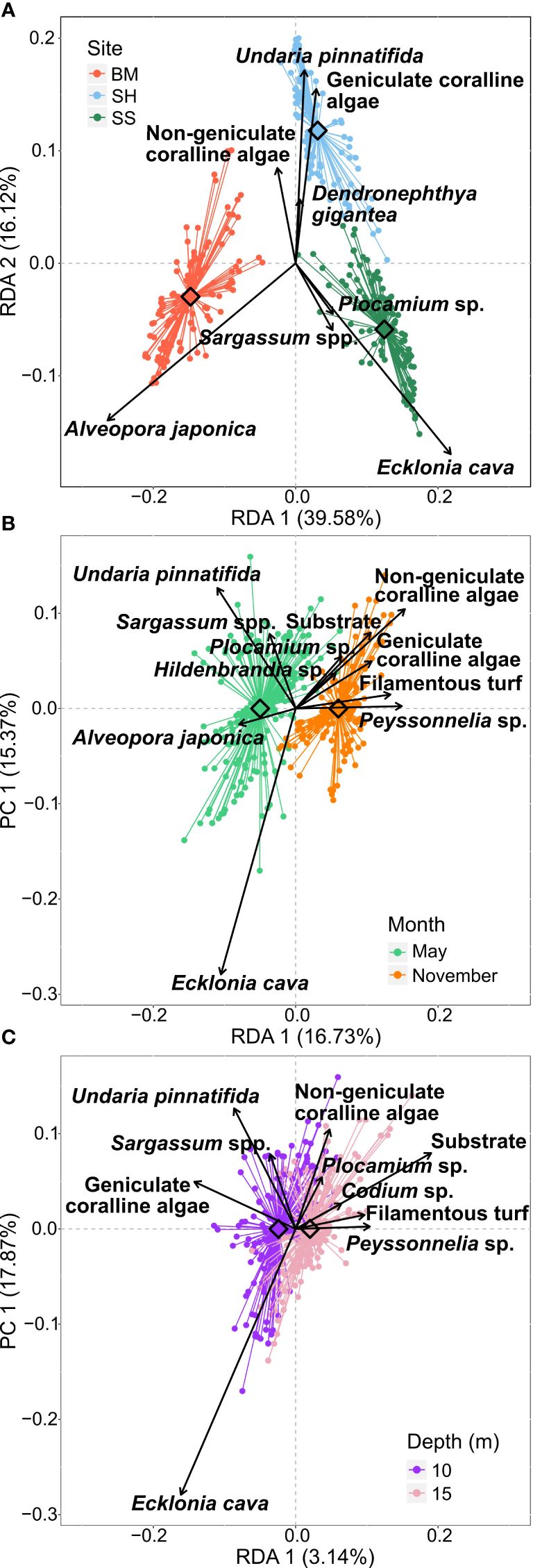
Figure 6 Partial Redundancy Analysis (pRDA) of Hellinger transformed percentage cover data. The constraining factor is (A) “site”, (B) “month”, and (C) “depth”. For each graphic, the influence of the two other factors was removed (conditioning factors). The diamond is the centroid of each group. Each dot represents a photo-quadrat. In (A) – Red: Bomok (BM); Blue: Sinheung (SH); and Green: Seongsan (SS). In (B) – Light green: May 2022; and Orange: November 2022. In (C) – Purple: 10 m depth; and Pink: 15 m depth. Table 2 reports the pRDA models’ details and the permutation test results.
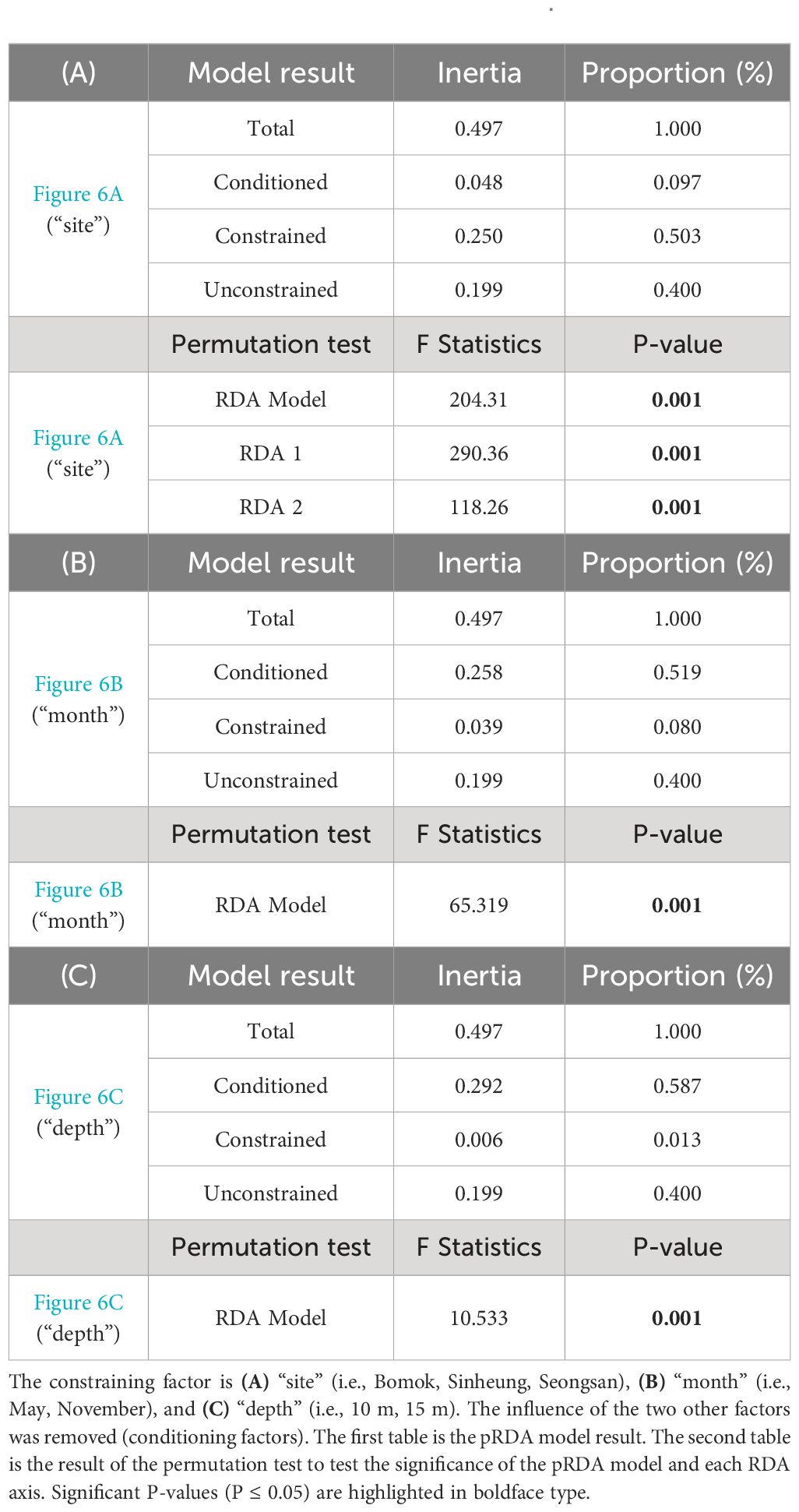
Table 2 Complementary pRDA results of Figure 6.
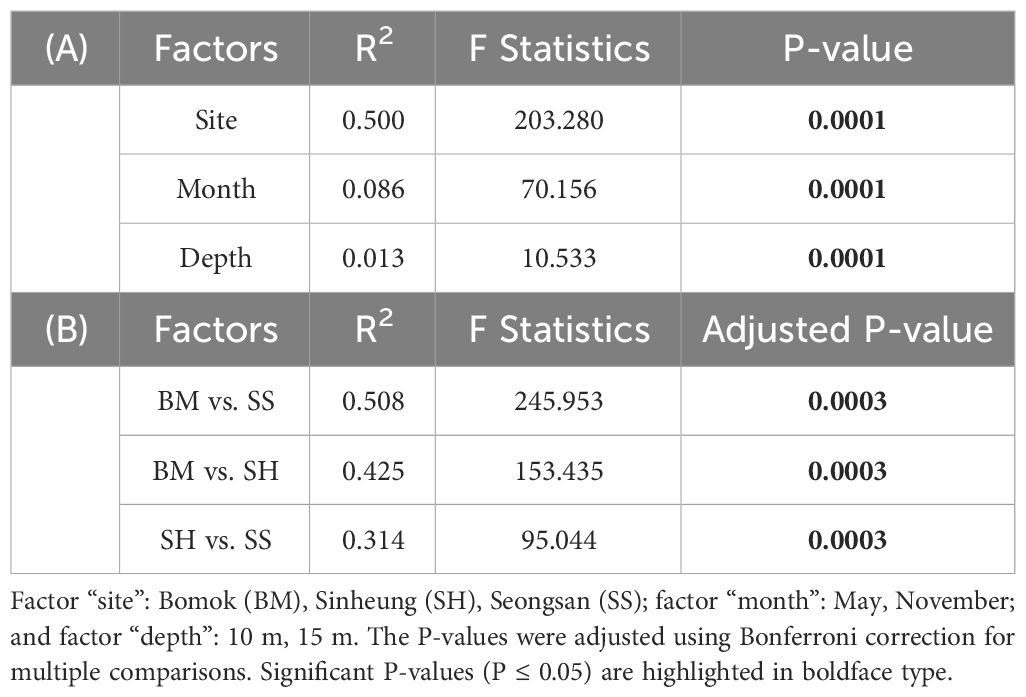
Table 3 (A) PERMANOVA test results from Euclidean distance matrix of Hellinger transformed percentage cover data of all species. (B) Pairwise PERMANOVA test results for the factor “site”.
The pRDA results showed that the greatest proportion of variance in the benthic community cover was explained by the factor “site” (50.3%), followed by “month” (8.0%) and “depth” (1.3%), while 40.0% of the variance remained unexplained by these factors (Figure 6; Table 2). All three pRDA models were significant (Table 2; p-value = 0.001). However, the variability explained by the pRDA model (RDA axis) was far greater than that of the residuals (PC axis) for “site” only, where the first two canonical axes were significant (Table 2A; RDA1 = 39.6%, RDA2 = 16.1%). The inter-site variability observed in the benthic community was explained primarily by the species E. cava, Plocamium sp., and Sargassum spp. (i.e., macroalgae species) which were representative of SS, opposed on the first axis to A. japonica (i.e., scleractinian hard coral) representing BM. Undaria pinnatifida, coralline algae, and D. gigantea (i.e., macroalgae and soft coral species, respectively) represented SH on the second axis (Figure 6A). The PERMANOVA test showed an F-statistic and R2 of more than two times higher for sites than for months and depths, although all three factors significantly influenced the benthic community (Table 3A). The benthic communities of BM and SS were the most distinct, followed by BM and SH, then SH and SS (Table 3B; Figure 6A).
The benthic compositions of BM and SS exhibited differences between May and November (Figure 5; Table 3), mainly characterized by a decrease in the percentage cover of the dominant taxa (i.e., E. cava and A. japonica), an increase in coralline algae, and the emergence of filamentous turf, Hildenbrandia sp. and Peyssonnelia sp. as major species. At SS, the rhodophytic macroalgae Grateloupia angusta and Plocamium sp. had higher coverages in November. At SH, the canopy-forming algae E. cava and U. pinnatifida and the chlorophyte Codium sp. were present at non-negligible coverages only in May (mean ± SD between depths = 8.2 ± 12.8%, 27.8 ± 21.4%, and 3.9 ± 6.4%, respectively) (Figure 5B; Supplementary Table 4). At all three sites, the coverage of substrate was constantly higher in November than in May. These observations are supported by the pRDA “month” model (RDA1 = 16.7%; Figure 6B), where A. japonica, E. cava, U. pinnatifida, and Sargassum spp. were indicative of May, while substrate, coralline and turf algae, Hildenbrandia sp., Plocamium sp., and Peyssonnelia sp. were linked to November. Note that SH data from November at 10 m depth are missing.
There were slight differences in benthic percentage cover between depths (Figures 5, 6C; Table 3). Indeed, the variation explained by the factor “depth” was five times lower than the proportion of variance in the residuals (PC1 = 17.9%; RDA1 = 3.1%). Regardless of months and sites, only macroalgae species and substrate were responsible for the variability observed across depths. For instance, U. pinnatifida, Sargassum spp., E. cava, and geniculate coralline algae were representative of the 10 m depth, while Plocamium sp., Peyssonnelia sp., Codium sp., filamentous turf and CCA converged to 15 m depth (Figure 6C). Specifically, at BM, at 10 m, geniculate coralline algae, hard coral species Montipora millepora and Psammocora albopicta, sea anemone Entacmeae sp., and chlorophyte species Cladophora wrightiana had higher coverage than at 15 m, contrary to rhodophyte Peyssonnelia sp. and sea anemone Heteractis sp. (Supplementary Table 4). At SS, the coverages of canopy-forming brown algae were higher at 10 m than at 15 m, contrary to the trends recorded for rhodophytes G. angusta, Peyssonnelia sp., and Plocamium sp., as well as the substrate category. At SH, the soft coral D. gigantea, CCA, Porifera, turf-forming algae, and rhodophytes Peyssonnelia sp. and G. angusta were more abundant at 15 m depth, contrary to kelp species E. cava.
4 Discussion
This study quantified abiotic environmental variations at three sites around Jeju Island and investigated accompanying community dynamics of sessile benthos. Hard corals dominated in the TWC-influenced, low-energy (i.e., less windy), southern BM; canopy-forming brown algae dominated in less TWC-influenced, high-energy, eastern SS; and coralline algae dominated in shallow, strongly tide-influenced, more eutrophic, northern SH.
First, it was found that the level of environmental distinctness between sites varied with season, being strongest in November and February, respectively, while being weakest in May and August. Consequently, the distinct environmental setting at each site was subject to seasonal variations. The TWC, a branch of the warm, saline, and oligotrophic Kuroshio Current, shows seasonal variability, intensifying in summer (i.e., reaching further north than in winter), with a current velocity that can vary by 20 cm s-1 between July and January (Takikawa and Yoon, 2005; Cha and Moon, 2020). Additionally, Changjiang River discharge (termed Changjiang Diluted Water, CDW) from the Chinese mainland arrives at Jeju Island in the southwest. Then, driven by southerly summer winds, the CDW encompasses the whole island, peaking in July and August (Park, 1986; Kim et al., 2009; Park et al., 2011; Kwon et al., 2018). Therefore, during summer, environmental heterogeneity around the island is further reduced as CDW adds fresh, warm, oligotrophic water to the TWC-dominated coastal surface waters. Moreover, increased precipitation during the summer monsoon season further homogenizes surface water properties around the island (Kwon et al., 2020). In winter, however, the weakened TWC only reaches the south of Jeju Island, increasing the SST by up to 2°C at BM compared to SS and SH. Cha and Moon (2020) even reported a winter temperature gradient of 6°C between the northwest (12°C) and the southeast (18°C), compared to just 3°C in summer (25°C and 28°C, respectively). Additionally, in winter, the east and north are subject to strong northerly wind bursts, impacting currents, surface mixing layer, SST, nutrient supply and turbidity, while Mt. Halla, a nearly 2000 m high volcano situated near the center of the island, acts as a wind-breaker, sheltering the south from these strong, northerly winter gusts (Jacobs et al., 2000; Nishihara and Terada, 2010; Cho et al., 2013). Hence, environmental conditions between sites were most similar in summer and most different in winter. Considering that in our benthic community data, the factor “month” explained only a comparably low proportion of variability in sessile community coverages (pRDA = 8.0% between May and November), these environmental winter differences may play a key role in structuring benthic community around the island. However, a more in-depth study, including high time-resolution data to detect seasonal patterns, is necessary to confirm this assumption.
Second, the recorded site-specific sessile benthic compositions around Jeju Island were consistent with the observed local environmental conditions and topography. It was found that all three sites showed a distinct sessile benthic composition. Each site was dominated by one foundation species or category, which reflected local abiotic conditions. While all our study sites showed the presence of hermatypic scleractinian coral species, BM was characterized by highest coverage and species diversity, including A. japonica, M. millepora, P. profundacella, and P. albopicta. Generally, the southern coastal marine area is steep and deep, while winds are milder (see Figure 1 bathymetry). In addition, the tidal energy contribution is low (< 30%), while the majority of prevailing environmental conditions are determined by the specific water properties of the TWC (i.e., saline, warm, oligotrophic) passing Jeju Island (Hur et al., 1999; Cha and Moon, 2020). Such hydrographic and topographic features may promote a comparably oligotrophic environment. Although the nutrient concentration at BM may show a localized peak due to the presence of a sewage treatment plant near the sampling site, environmental data from the exact sampling site are currently lacking. In addition, the consistent supply of oligotrophic TWC waters may soften direct impacts of the sewage treatment plant. As high nutrient concentrations can benefit corals’ competitors and hinder coral physiological processes (Harriott and Banks, 2002; Manzello et al., 2014; Kwon et al., 2020), more oligotrophic conditions could favor the thriving of hard coral species at BM. Moreover, this low-energy habitat and the influence of the TWC lead to significantly higher winter SST and transparency readings. At BM, the mean SST and transparency in February were over 15°C and 10 m, respectively, contrary to SH and SS. Subtropical hard coral richness and abundance are more sensitive to minimum temperatures and short-term thermal variability (Sommer et al., 2018), which is in line with Jeju’s hard coral thermal optimums (see Supplementary Table 2). For instance, temperatures between 10°C and 15°C can induce A. japonica bleaching and reduction of its basal metabolism, although without being lethal (Higuchi et al., 2020; Keshavmurthy et al., 2021). Recenthly, it was showed that three cryptic species of Alveopora japonica corresponding to Japonese, Korean and Taiwan distributions existed (Kang et al. 2020). Moreover, McIlroy et al. (2019) reported that 80% of the maximum productivity of five subtropical coral species, including one Montipora species, was maintained at temperatures between 15.7°C and 32°C, with their optimal temperatures always being greater than 20°C, which fall within the annual temperature range of BM. Additionally, photosynthetic symbionts-carrying hard corals thrive better in high transparency waters where there is more sunlight (De’ath and Fabricius, 2010; Muir et al., 2015), while the reduction in suspended particles can promote coral recruitment by decreasing the abrasion effect (Gilmour, 1999; Gove et al., 2015). However, habitats dominated by A. japonica (percentage cover > 25%) have been identified at Biyangdo, northwest of Jeju Island (Denis et al., 2014). Although the environmental conditions at this site are unknown, they are likely to differ from those at BM, and additional controlling factors could explain this hard coral outbreak (see Kwon et al., 2020). The low coverage of canopy-forming algae and other non-encrusting macroalgae species at BM (< 2.5%) can favor the growth of hermatypic scleractinian corals by decreasing competition stress, abrasion and dislodgment (Coyer et al., 1993; Miller and Hay, 1996; Tuckett et al., 2017; De Vargas Ribeiro et al., 2022). Interestingly, a few decades ago, the perennial canopy-forming brown alga E. cava thrived at BM (Chung et al., 1998; Ribas-Deulofeu et al., 2023). Meanwhile, in our study, E. cava was not found at the BM site, while it was present at the SH site and dominated the SS site. Tuckett et al. (2017) reported a shift in ecosystems in Western Australia from temperate canopy-forming algae to hard coral species with warm water affinities. They attributed this shift to a competitive release caused by kelp decline resulting from heatwaves and global warming. Indeed, temperate kelps, such as E. cava, are heat-sensitive (Wernberg et al., 2011, 2016) (Supplementary Table 2) and, like hard corals, may be limited by water temperature in winter. The optimal water temperature for E. cava growth during winter is 15–18°C, while water temperatures above 20°C in summer can inhibit growth, although promoting reproductive potential (Yokohama et al., 1987; Kim et al., 2016). At SS, mean SST in February and May were nearly 15°C and 17°C, respectively, lying within the range of E. cava’s optimal temperature. Moreover, the high-energy environment caused by strong winter winds could contribute to the persistence of kelp forests at SS. Water motion is key in promoting and aiding kelp forest growth by dislodging grazers (Denny and Gaylord, 1996) and increasing nutrient availability and uptake (Hurd et al., 1996; Nishihara and Terada, 2010). E. cava is well-equipped to handle these physical disturbances due to its finger-like holdfasts and large thalli, which help to attach itself firmly to the substrate (Kang et al., 2011; Kang and Kim, 2012; Lee K.-T. et al., 2023). Therefore, climate change-related events, such as the intensification of the KWC (i.e., increased SST and oligotrophy) (Wu et al., 2012; Kim et al., 2022a), could promote the expansion of high-latitude hard coral communities along the southern coastline towards east and west, while driving a decline in eastern temperate kelp forests. On the north of the island, the coastal marine area is flat and shallow, the wind speed is high, and tidal energy is prevalent (> 90%) in setting local environmental conditions (Cha and Moon, 2020). Results showed that DIN and DIP concentrations were significantly higher at SH than at BM, and generally, all nutrient concentration-related variables were consistently highest at SH, especially the November measurements. This may be due to a combination of reasons, such as topography hindering quick dilution, increased input through aquaculture wastewater, and inefficient biological uptake by marine organisms. Indeed, nutrient availability in Jeju’s coastal waters is strongly related to land-based, anthropogenic activities, such as land-operated fish farms which are present in the vicinity of SH. Wastewater from land-operated fish farms accounts for up to 95% of the seawater ammonium (Kwon et al., 2022), increasing coastal DIN and DIP concentrations (Choi et al., 2021), and generally contributes significantly to the nitrogen budget in the coastal waters of Jeju (Koh et al., 2013; Kim et al., 2022b). Sea water enriched in ammonium is preferred by coralline algae, which dominate SH (Nguyen et al., 2020; Kwon et al., 2022). Moreover, geniculate coralline algae have lower nitrogen amounts in their tissue than brown algae (e.g., E. cava, U. pinnatifida), hence incorporating less total nitrogen (Choi et al., 2021). Although, throughout the year, SH showed similar environmental conditions to SS, SH was not dominated by E. cava. Canopy-forming algae populations of U. pinnatifida and E. cava were present at SH but showed much lower coverage percentages than in SS and were only recorded in May. High nutrient concentrations due to sewage effluents can inhibit the germination and photo efficiency of brown algae (Doblin and Clayton, 1995; Scherner et al., 2012), but this substantial difference in benthic composition compared to the abiotic environmental similarity of these sites suggests that other factors may hold a key role in shaping these benthic ecosystems. Overgrazing by sea urchins and other invertebrates is known to cause decrease in kelp forests (Kang, 2010; Bekkby et al., 2015; Ling et al., 2015), and a high abundance of such grazers at SH compared to SS could explain the low coverage of canopy-forming algae despite the apparent favorable environmental conditions. Such information is not reported in Jeju’s literature, and personal observations did not attest to high abundance of grazers. Still, additional ecological factors, such as intensity and frequency of extreme weather-related events (Smale and Wernberg, 2013) and biotic interactions (Negri et al., 2001; Tebben et al., 2015), can all hold essential roles in shaping benthic communities, and additional study including these parameters is warranted. Additionally, DO measurements were significantly lowest at SH, especially in August, which may be caused, in parts, by the elevated nutrient levels, as increased nitrogen and phosphorous content lowers the amount of dissolved oxygen in the water (Dodds, 2006). The overall lower water quality at SH mirrored in the high coverage percentages and diversity of Bryozoa, Porifera, and soft corals. Sponges and bryozoans have been found in large species numbers and percentage cover on hard bottoms of highly disturbed environments, and especially bryozoans are reported to thrive in low light conditions, while soft corals can tolerate high eutrophication and sedimentation environments (Corriero et al., 2007; Baum et al., 2016; Evseeva and Dvoretsky, 2023). In contrast to the southern coast, the benthic community composition in the northern coastal waters may be more influenced by topography and additional localized anthropogenic stressors rather than natural abiotic parameters. Similarly, the impact of global warming on the community composition of the northern barren grounds may be comparably low, as CCA communities are less sensitive to rising SST (Krieger et al., 2023).
Third, water depth did not much influence any of the observed community dynamics, although depth is directly correlated with light and nutrient availability and, as such, impacts the vertical zonation of marine benthic species (Kang and Kim, 2012; Muir et al., 2015). Generally, photosynthetic species, such as canopy-forming brown algae and hermatypic scleractinian species, always showed higher coverages at the shallower depth. At BM, the hard corals M. millepora, P. profundacella, and P. albopicta, and geniculate coralline algae were more abundant at 10 m depth. As light is a major limiting factor in high-latitude ecosystems, particularly in winter, hard corals carrying photosynthetic symbionts living above 30° latitude tend to thrive best in shallow areas that receive more sunlight (Muir et al., 2015). Interestingly, there was no depth-related trend in the coverage of A. japonica. This may be because the reported depth range of this species around Jeju Island is much wider than those of M. millepora and P. profundacella (5–25 m and < 10 m, respectively; Sugihara et al., 2014). Geniculate coralline algae usually dominate at shallower depths (1–10 m depth; Kang et al., 2023) because of their higher tolerance to wave action. Similarly, the photosynthetic brown algae E. cava and Sargassum spp. showed decreasing coverages with depth at SH and SS. These species exhibited maximum abundances at depths between 6 m and 10 m in Jeju waters, explained by their higher light demand and lower tolerance to strong physical forces (Kang et al., 2023). On the contrary, encrusting red algae (e.g., CCA and Peyssonnelia sp.) and G. angusta have increasing coverages with depth due to their lower demand for light (Kang et al., 2023). At SH, the soft coral D. gigantea was more abundant at 15 m depth (coverage ≈ 4% in May and November), which may be explained by its non-photosynthetic lifestyle, i.e., not requiring sunlight for energy acquisition, and its generally high stress-tolerance to sedimentation, turbidity, and eutrophication (De’ath and Fabricius, 2010; Powell et al., 2014; Baum et al., 2016; Lalas et al., 2023). These traits enable D. gigantea to thrive in poorer quality waters while avoiding increased competition at shallower depths. Likewise, the sampling months accounted for a minor part of the variability observed in the benthic community. For instance, the decrease in canopy-forming algae and high-latitude hard corals at SS and BM, respectively, can be attributed mainly to, in the absence of other extreme events, destruction by super typhoon Hinnamnor, which reached Jeju Island in September 2022. Lee K.-T. et al. (2023) reported a 42% loss of E. cava at 10 m and 34% at 15 m in September 2022 compared to May 2022 at SS, which is coherent with our results. This decline in canopy density has led to an artificial increase in the percentage cover of underlying species in November, such as rhodophytes Plocamium sp. and G. angusta. Similarly, the seasonal decline of A. japonica at BM by roughly half its coverage from May (61% at 10 m; 71% at 15 m) to November (37% at 10 m; 30% at 15 m) was also likely caused by typhoon Hinnamnor. Numerous dead and bleached A. japonica were observed on photo-quadrats in September 2022, right after Hinnamnor had hit. At the same time, the increase at all three sites and two depths of turf-forming algae, substrate, red algae, and coralline algae may have been promoted by space, light, and resources availability, as well as species-specific invasion capacity of a newly disturbed area (Sousa, 1984; Airoldi, 1998; Kim T. et al., 2022). Life history traits can also contribute to this observed monthly divergence (Darling et al., 2012). Whereas the dominant E. cava and A. japonica have a perennial life cycle and are known to have an overall annual stable coverage (Haroun et al., 1989; Vieira et al., 2016), U. pinnatifida is an annual species living as macroscopic, diploid sporophyte from January to July, and the rest of the year, as microscopic, haploid gametophyte (Schiel and Thompson, 2012). In its microscopic stage, its presence cannot be identified by image analysis, which can explain its absence in the November results. This study investigated the current spatial variability in Jeju’s benthic communities and local environmental conditions, and seasonal variability of these marginal ecosystems needs further assessment.
5 Conclusion
Marine climatic transition zones are emerging as geographic hot spots of scientific interest in climate warming-related ocean changes. Increasing temperatures threaten the survival of the heat-sensitive temperate species living at the limit of their physiological tolerance while driving the arrival and expansion of species with warmer climate affinities. Therefore, benthic habitats of marine climatic transition areas are subject to extensive faunal turnover, driven by an interplay of extinction, outbreak, and migration events.
This study greatly improved the understanding of Jeju benthic community dynamics on an annual scale and demonstrated the importance of considering environmental parameters in understanding benthic community distribution in climatic transition zones. It clearly suggests that a combination of hydrographic, meteorological, and topographic parameters structures coastal benthic communities in the temperate transition zone of Jeju Island. Statistically, winter water temperature, nutrient concentration, water motion (derived from wind speed and direction), and transparency were the most significant in setting the particular environmental conditions around the island. These local environmental conditions were congruent with the distribution, composition, and coverage of sessile foundation species.
Although the underlying drivers of Jeju benthic communities need to be further investigated, our study suggests that site divergence in winter environmental conditions may play a significant role. Higher spatio-temporal resolution abiotic and biotic data are needed in future studies to improve our understanding of marginal benthic community dynamics. Moreover, experimental research is necessary in the mostly overlooked, temperate transition zone of Jeju to investigate the controlling factors of these marginal communities further. Having such information will help clarify the role of ecological variables in driving biogenic habitat shifts, assessing how each affects sessile communities, including, among others, effects on the physiology of foundation species, antagonistic and synergistic relationships, and food web interactions.
Data availability statement
The original contributions presented in the study are included in the article/Supplementary Material. Further inquiries can be directed to the corresponding author.
Author contributions
GP: Conceptualization, Data curation, Formal analysis, Investigation, Methodology, Software, Visualization, Writing – original draft, Writing – review & editing. AJ: Conceptualization, Validation, Visualization, Writing – review & editing. K-TL: Data curation, Investigation, Methodology, Writing – review & editing. LP: Formal analysis, Investigation, Visualization, Writing – review & editing. H-SY: Investigation, Project administration, Writing – review & editing. YS: Investigation, Validation, Writing – review & editing. H-SP: Funding acquisition, Project administration, Resources, Writing – review & editing. D-HK: Funding acquisition, Project administration, Resources, Writing – review & editing. TK: Conceptualization, Data curation, Investigation, Methodology, Supervision, Validation, Visualization, Writing – review & editing.
Funding
The author(s) declare financial support was received for the research, authorship, and/or publication of this article. This research was supported by the Korea Institute of Ocean Science and Technology (KIOST), grant numbers PEA0205, PEA0206 and PEA0211. AJ was financially supported by the Brain Pool Program through NRF funded by the Ministry of Science and ICT (reference code: 2019H1D3A1A01070922).
Acknowledgments
We would like to express our appreciation to Taeho Kim and other administrative and technical support from the Jeju Marine Research Center, KIOST. We would like to express our sincere gratitude to the editor, Prof. Stelios Katsanevakis, and the reviewers, Dr. Francisco Arenas and Dr. Roberto Uribe Alzamora, for their valuable and constructive comments that helped to improve the article.
Conflict of interest
The authors declare that the research was conducted in the absence of any commercial or financial relationships that could be construed as a potential conflict of interest.
Publisher’s note
All claims expressed in this article are solely those of the authors and do not necessarily represent those of their affiliated organizations, or those of the publisher, the editors and the reviewers. Any product that may be evaluated in this article, or claim that may be made by its manufacturer, is not guaranteed or endorsed by the publisher.
Supplementary material
The Supplementary Material for this article can be found online at: https://www.frontiersin.org/articles/10.3389/fmars.2024.1345518/full#supplementary-material
References
Agostini S., Harvey B. P., Wada S., Kon K., Milazzo M., Inaba K., et al. (2018). Ocean acidification drives community shifts towards simplified non-calcified habitats in a subtropical–temperate transition zone. Sci. Rep. 8, 11354. doi: 10.1038/s41598-018-29251-7
Aguilar S., Moore P. J., Uribe R. A. (2022). Habitat formed by the invasive macroalga Caulerpa filiformis (Suhr) Hering (Caulerpales, Chlorophyta) alters benthic macroinvertebrate assemblages in Peru. Biol. Invasions 24, 3305–3319. doi: 10.1007/s10530-022-02847-x
Airoldi L. (1998). Roles of disturbance, sediment stress, and substratum retention on spatial dominance in algal turf. Ecology 79, 2759–2770. doi: 10.1890/0012-9658(1998)079[2759:RODSSA]2.0.CO;2
Anderson M. J. (2001). A new method for non-parametric multivariate analysis of variance. Austral Ecol. 26, 32–46. doi: 10.1111/j.1442-9993.2001.01070.pp.x
Baum G., Januar I., Ferse S. C. A., Wild C., Kunzmann A. (2016). Abundance and physiology of dominant soft corals linked to water quality in Jakarta Bay, Indonesia. PeerJ 4, e2625. doi: 10.7717/peerj.2625
Beger M., Sommer B., Harrison P. L., Smith S. D. A., Pandolfi J. M. (2014). Conserving potential coral reef refuges at high latitudes. Divers. Distrib. 20, 245–257. doi: 10.1111/ddi.12140
Bekkby T., Angeltveit G., Gundersen H., Tveiten L., Norderhaug K. M. (2015). Red sea urchins (Echinus esculentus) and water flow influence epiphytic macroalgae density. Mar. Biol. Res. 11, 375–384. doi: 10.1080/17451000.2014.943239
Belkin I. M. (2009). Rapid warming of large marine ecosystems. Prog. Oceanogr. 81, 207–213. doi: 10.1016/j.pocean.2009.04.011
Campbell A. H., Marzinelli E. M., Vergés A., Coleman M. A., Steinberg P. D. (2014). Towards restoration of missing underwater forests. PloS One 9, e84106. doi: 10.1371/journal.pone.0084106
Cha S.-C., Moon J.-H. (2020). Current systems in the adjacent Seas of Jeju Island using a high-resolution regional ocean circulation model. Ocean Polar Res. 42, 211–223. doi: 10.4217/OPR.2020.42.3.211
Charlier R. H. (2005). Encyclopedia of coastal science. J. Coast. Res. 214, 312–313. doi: 10.2112/1551-5036(2005)21[866:BR]2.0.CO;2
Cho Y.-K., Seo G.-H., Kim C.-S., Choi B.-J., Shaha D. C. (2013). Role of wind stress in causing maximum transport through the Korea Strait in autumn. J. Mar. Syst. 115–116, 33–39. doi: 10.1016/j.jmarsys.2013.02.002
Choe K.-L. (1988). “On Ostracod biofacies and five new genera in Korean Seas,” in Developments in Palaeontology and Stratigraphy Evolutionary biology of ostracoda: its fundamentals and applications. Eds. Hanai T., Ikeya N., Ishizaki K. (Tokyo, Japan: Elsevier and Kodansha), 121–133. doi: 10.1016/S0920-5446(08)70177-8
Choi S. K., Kim T. H., Kang Y. H., Kim S., Kim T.-H., Kim J. K., et al. (2021). Changes in the dynamics and nutrient budget of a macroalgal community exposed to land-based fish farm discharge off Jeju Island, Korea. Sustainability 13, 11793. doi: 10.3390/su132111793
Chung H., Cho K. W., Chung K. H., Kim J. H., Shin J., Seo Y., et al. (1998). Ecological characteristics of algal whitening in coastal zone of Seogwipo area, Cheju Island. Algae 13, 361–374.
Corriero G., Longo C., Mercurio M., Marchini A., Occhipinti-Ambrogi A. (2007). Porifera and Bryozoa on artificial hard bottoms in the Venice Lagoon: Spatial distribution and temporal changes in the northern basin. Ital. J. Zool. 74, 21–29. doi: 10.1080/11250000601084100
Coyer J. A., Ambrose R. F., Engle J. M., Carroll J. C. (1993). Interactions between corals and algae on a temperate zone rocky reef: Mediation by sea urchins. J. Exp. Mar. Biol. Ecol. 167, 21–37. doi: 10.1016/0022-0981(93)90181-M
Darling E. S., Alvarez-Filip L., Oliver T. A., McClanahan T. R., Côté I. M. (2012). Evaluating life-history strategies of reef corals from species traits. Ecol. Lett. 15, 1378–1386. doi: 10.1111/j.1461-0248.2012.01861.x
De’ath G., Fabricius K. (2010). Water quality as a regional driver of coral biodiversity and macroalgae on the Great Barrier Reef. Ecol. Appl. 20, 840–850. doi: 10.1890/08-2023.1
de Azevedo J., Franco J. N., Vale C. G., Lemos M. F. L., Arenas F. (2023). Rapid tropicalization evidence of subtidal seaweed assemblages along a coastal transitional zone. Sci. Rep. 13, 11720. doi: 10.1038/s41598-023-38514-x
Denis V., Ribas-Deulofeu L., Loubeyres M., De Palmas S., Hwang S.-J., Woo S., et al. (2014). Recruitment of the subtropical coral Alveopora japonica in the temperate waters of Jeju Island, South Korea. Bull. Mar. Sci. 91, 85–96. doi: 10.5343/bms.2014.1032
Denny M., Gaylord B. (1996). Why the urchin lost its spines: Hydrodynamic forces and survivorship in three Echinoids. J. Exp. Biol. 199, 717–729. doi: 10.1242/jeb.199.3.717
De Vargas Ribeiro F., Pessarrodona A., Tucket C., Mulders Y., Pereira R. C., Wernberg T. (2022). Shield wall: Kelps are the last stand against corals in tropicalized reefs. Funct. Ecol. 36, 2445–2455. doi: 10.1111/1365-2435.14141
Doblin M. A., Clayton M. N. (1995). Effects of secondarily-treated sewage effluent on the early life-history stages of two species of brown macroalgae: Hormosira banksii and Durvillaea potatorum. Mar. Biol. 122, 689–698. doi: 10.1007/BF00350691
Dodds W. K. (2006). Nutrients and the “dead zone”: The link between nutrient ratios and dissolved oxygen in the northern Gulf of Mexico. Front. Ecol. Environ. 4, 211–217. doi: 10.1890/1540-9295(2006)004[0211:NATDZT]2.0.CO;2
Dunn O. J. (1964). Multiple comparisons using rank sums. Technometrics 6, 241–252. doi: 10.1080/00401706.1964.10490181
Evseeva O., Dvoretsky A. G. (2023). Shallow-water Bryozoan communities in a glacier fjord of west Svalbard, Norway: Species composition and effects of environmental factors. Biology 12, 185. doi: 10.3390/biology12020185
Ferro I., Morrone J. J. (2014). Biogeographical transition zones: A search for conceptual synthesis. Biol. J. Linn. Soc 113, 1–12. doi: 10.1111/bij.2014.113.issue-1
Filbee-Dexter K., Wernberg T. (2018). Rise of turfs: A new battlefront for globally declining kelp forests. BioScience 68, 64–76. doi: 10.1093/biosci/bix147
Fujita D. (2010). Current status and problems of isoyake in Japan. Bull. Fish. Res. Agency 32, 33–42.
Gilmour J. (1999). Experimental investigation into the effects of suspended sediment on fertilisation, larval survival and settlement in a scleractinian coral. Mar. Biol. 135, 451–462. doi: 10.1007/s002270050645
Gove J., Williams G., McManus M., Clark S., Ehses J., Wedding L. (2015). Coral reef benthic regimes exhibit non-linear threshold responses to natural physical drivers. Mar. Ecol. Prog. Ser. 522, 33–48. doi: 10.3354/meps11118
Han I.-S., Lee J.-S. (2020). Change the annual amplitude of sea surface temperature due to climate change in a recent decade around the Korean Peninsula. J. Korean Soc Mar. Environ. Saf. 26, 233–241. doi: 10.7837/kosomes.2020.26.3.233
Haroun R. J., Yokohama Y., Aruga Y. (1989). Annual growth cycle of the brown alga Ecklonia cava in central Japan. Sci. Mar. 53, 349–356.
Harriott V., Banks S. (2002). Latitudinal variation in coral communities in eastern Australia: A qualitative biophysical model of factors regulating coral reefs. Coral Reefs 21, 83–94. doi: 10.1007/s00338-001-0201-x
Higuchi T., Yuyama I., Agostini S. (2020). “Physiology of winter coral bleaching in temperate zone,” in Evolution of marine coastal ecosystems under the pressure of global changes. Eds. Ceccaldi H.-J., Hénocque Y., Komatsu T., Prouzet P., Sautour B., Yoshida J. (Springer International Publishing, Cham), 147–162. doi: 10.1007/978-3-030-43484-7_10
Hillebrand H. (2004). On the generality of the latitudinal diversity gradient. Am. Nat. 163, 192–211. doi: 10.1086/381004
Hobbs R. J., Arico S., Aronson J., Baron J. S., Bridgewater P., Cramer V. A., et al. (2006). Novel ecosystems: Theoretical and management aspects of the new ecological world order. Glob. Ecol. Biogeogr. 15, 1–7. doi: 10.1111/j.1466-822X.2006.00212.x
Hobday A. J., Pecl G. T. (2014). Identification of global marine hotspots: Sentinels for change and vanguards for adaptation action. Rev. Fish Biol. Fish. 24, 415–425. doi: 10.1007/s11160-013-9326-6
Hur H. B., Jacobs G. A., Teague W. J. (1999). Monthly variations of water masses in the Yellow and East China Seas, November 6, 1998. J. Oceanogr. 55, 171–184. doi: 10.1023/A:1007885828278
Hurd C. L., Harrison P. J., Druehl L. D. (1996). Effect of seawater velocity on inorganic nitrogen uptake by morphologically distinct forms of Macrocystis integrifolia from wave-sheltered and exposed sites. Mar. Biol. 126, 205–214. doi: 10.1007/BF00347445
Hwang E. K., Gong Y. G., Hwang I.-K., Park E.-J., Park C. S. (2013). Cultivation of the two perennial brown algae Ecklonia cava and Ecklonia stolonifera for abalone feeds in Korea. J. Appl. Phycol. 25, 825–829. doi: 10.1007/s10811-012-9941-y
Jacobs G. A., Hur H. B., Riedlinger S. K. (2000). Yellow and East China Seas response to winds and currents. J. Geophys. Res. Oceans 105, 21947–21968. doi: 10.1029/2000JC900093
Kang R.-S. (2010). A review of destruction of seaweed habitats along the coast of the Korean Peninsula and its consequences. Bull. Fish Res. Agen 32, 25–31.
Kang S.-K. (2011). Economic analysis of the seaweed forest creation project: The case of Jeju Woodo Seokwang-ri. J. Fish. Bus. Adm. 42, 37–55.
Kang J.-C., Choi H.-G., Kim M.-S. (2011). Macroalgal species composition and seasonal variation in biomass on Udo, Jeju Island, Korea. ALGAE 26, 333–342. doi: 10.4490/algae.2011.26.4.333
Kang J. H., Jang J. E., Kim J. H., Kim S., Keshavmurthy S., Agostini S., et al. (2020). The origin of the subtropical coral Alveopora japonica (Scleractinia: Acroporidae) in high-latitude environments. Front. Ecol. Evol. 8, 12. doi: 10.3389/fevo.2020.00012
Kang J. C., Kim M. S. (2012). Seasonal variation in depth-stratified macroalgal assemblage patterns on Marado, Jeju Island, Korea. ALGAE 27, 269–281. doi: 10.4490/algae.2012.27.4.269
Kang J. C., Lee H. W., Ko Y. W., Kim M. S. (2023). Phase shift in vertical distribution of macroalgal assemblages on Udo Islet, Jeju, Korea, after a decade of revisiting. Ocean Sci. J. 58, 24. doi: 10.1007/s12601-023-00119-w
Kawecki T. J. (2008). Adaptation to marginal habitats. Annu. Rev. Ecol. Evol. Syst. 39, 321–342. doi: 10.1146/annurev.ecolsys.38.091206.095622
Keshavmurthy S., Beals M., Hsieh H. J., Choi K.-S., Chen C. A. (2021). Physiological plasticity of corals to temperature stress in marginal coral communities. Sci. Total Environ. 758, 143628. doi: 10.1016/j.scitotenv.2020.143628
Kim D.-K. (2006). A study on the restoration of marine forests using artificial reef in the barren grounds along the coast of Jeju. [PhD thesis]. Jeju National University, Jeju-si, South Korea.
Kim S., Kang Y. H., Kim T.-H., Park S. R. (2016). Recovery pattern and seasonal dynamics of kelp species, Ecklonia cava population formed following the large-scale disturbance. J. Korean Soc Oceanogr. 21, 103–111. doi: 10.7850/JKSO.2016.21.3.103
Kim M.-S., Kang J. C., Kim B., Yang M. Y., Lee H. W. (2022). Seaweed diversity in Jeju Udo (Jeju, Republic of Korea: Jeju National University Institute of Basic Science).
Kim J., Kim G., Cho H., Nam S., Hwang J., Park S., et al. (2022a). Decline in the nutrient inventories of the upper subtropical northwest Pacific Ocean. Geophys. Res. Lett. 49, e2021GL093968. doi: 10.1029/2021GL093968
Kim J., Kim Y., Park S. E., Kim T.-H., Kim B.-G., Kang D.-J., et al. (2022b). Impact of aquaculture on distribution of dissolved organic matter in coastal Jeju Island, Korea, based on absorption and fluorescence spectroscopy. Environ. Sci. pollut. Res. 29, 553–563. doi: 10.1007/s11356-021-15553-3
Kim T., Kim T., Yang H.-S., Choi S. K., Son Y. B., Kang D.-H. (2022). Alveopora japonica conquering temperate reefs despite massive coral bleaching. Diversity 14, 86. doi: 10.3390/d14020086
Kim H.-C., Yamaguchi H., Yoo S., Zhu J., Okamura K., Kiyomoto Y., et al. (2009). Distribution of Changjiang Diluted Water detected by satellite chlorophyll-a and its interannual variation during 1998–2007. J. Oceanogr. 65, 129–135. doi: 10.1007/s10872-009-0013-0
Koh H.-J., Park S.-E., Cha H.-K., Chang D.-S., Koo J.-H. (2013). Coastal eutrophication caused by effluent from aquaculture ponds in Jeju. J. Korean Soc Mar. Environ. Saf. 19, 315–326. doi: 10.7837/kosomes.2013.19.4.315
Korea Marine Environment Management Corporation. (2019). Protocol of national marine ecosystem monitoring program. 3rd edition (Busan, Republic of Korea: Ministry of Oceans and Fisheries/Korea Marine Environment Management Corporation). Available at: https://www.meis.go.kr/mes/data/1/board.do.
Korea Marine Environment Management Corporation. Available online at: https://www.meis.go.kr/mei/observe/port.do (Accessed March 8, 2023).
Korean Meteorological Administration. Available online at: https://data.kma.go.kr/cmmn/main.do (Accessed March 28, 2023).
Krieger E. C., Taise A., Nelson W. A., Grand J., Le Ru E., Davy S. K., et al. (2023). Tolerance of coralline algae to ocean warming and marine heatwaves. PloS Clim. 2, e0000092. doi: 10.1371/journal.pclm.0000092
Kruskal W. H., Wallis W. A. (1952). Use of ranks in one-criterion variance analysis. J. Am. Stat. Assoc. 47, 583–621. doi: 10.1080/01621459.1952.10483441
Kwon H. K., Kim G., Hwang J., Lim W. A., Park J. W., Kim T.-H. (2018). Significant and conservative long-range transport of dissolved organic nutrients in the Changjiang Diluted Water. Sci. Rep. 8, 12768. doi: 10.1038/s41598-018-31105-1
Kwon H. K., Kim G., Kim T.-H., Park S.-E., Lee W. C. (2022). Quantification of groundwater versus fish-farm sources of nutrients in the coastal water off Jeju Island, Korea, using fluorescent dissolved organic matter as a tracer. J. Sea Res. 188, 102270. doi: 10.1016/j.seares.2022.102270
Kwon H. K., Seo J., Cho H.-M., Kim G. (2020). Tracing different freshwater sources for nutrients and dissolved organic matter in coastal waters off Jeju Island using Radon. Estuaries Coasts 43, 487–495. doi: 10.1007/s12237-018-0471-y
Lalas J. A. A., Manzano G. G., Desabelle L. A. B., Baria-Rodriguez M. V. (2023). Spatial variation in the benthic community structure of a coral reef system in the central Philippines: Highlighting hard coral, octocoral, and sponge assemblages. Reg. Stud. Mar. Sci. 61, 102859. doi: 10.1016/j.rsma.2023.102859
Lee K.-T., Lee H.-M., Subramaniam T., Yang H.-S., Park S. R., Kang C.-K., et al. (2022). Dominance of the scleractinian coral Alveopora japonica in the barren subtidal hard bottom of high-latitude Jeju Island off the south coast of Korea assessed by high-resolution underwater images. PloS One 17, e0275244. doi: 10.1371/journal.pone.0275244
Lee S., Park M. S., Kwon M., Park Y. G., Kim Y. H., Choi N. (2023). Rapidly changing East Asian marine heatwaves under a warming climate. J. Geophys. Res. Oceans 128, e2023JC019761. doi: 10.1029/2023JC019761
Lee K.-T., Perrois G., Yang H.-S., Kim T., Choi S. K., Kang D.-H., et al. (2023). Impact of super typhoon ‘Hinnamnor’ on density of kelp forest and associated benthic communities in Jeju Island, Republic of Korea. J. Mar. Sci. Eng. 11, 1035. doi: 10.3390/jmse11051035
Legendre P., Gallagher E. D. (2001). Ecologically meaningful transformations for ordination of species data. Oecologia 129, 271–280. doi: 10.1007/s004420100716
Legendre P., Legendre L. (2012). “Chapter 11: canonical analysis,” in Numerical ecology (Elsevier, Amsterdam, The Netherlands), 625–710. doi: 10.1016/B978-0-444-53868-0.50011-3
Lie H.-J., Cho C.-H., Lee J.-H., Niiler P., Hu J.-H. (1998). Separation of the Kuroshio water and its penetration onto the continental shelf west of Kyushu. J. Geophys. Res. Oceans 103, 2963–2976. doi: 10.1029/97JC03288
Ling S. D., Scheibling R. E., Rassweiler A., Johnson C. R., Shears N., Connell S. D., et al. (2015). Global regime shift dynamics of catastrophic sea urchin overgrazing. Philos. Trans. R. Soc B Biol. Sci. 370, 20130269. doi: 10.1098/rstb.2013.0269
Makino A., Yamano H., Beger M., Klein C. J., Yara Y., Possingham H. P. (2014). Spatio-temporal marine conservation planning to support high-latitude coral range expansion under climate change. Divers. Distrib. 20, 859–871. doi: 10.1111/ddi.12184
Manzello D. P., Enochs I. C., Bruckner A., Renaud P. G., Kolodziej G., Budd D. A., et al. (2014). Galápagos coral reef persistence after ENSO warming across an acidification gradient. Geophys. Res. Lett. 41, 9001–9008. doi: 10.1002/2014GL062501
Masuda H., Hayashi K., Nakamura K., Kobayashi Y. (1986). Field encyclopedia: coastal animals. 1st (Tokyo, Japan: Tokai University Press).
McIlroy S. E., Thompson P. D., Yuan F. L., Bonebrake T. C., Baker D. M. (2019). Subtropical thermal variation supports persistence of corals but limits productivity of coral reefs. Proc. R. Soc B Biol. Sci. 286, 20190882. doi: 10.1098/rspb.2019.0882
Miller M. W., Hay M. E. (1996). Coral-seaweed-grazer-nutrient interactions on temperate reefs. Ecol. Monogr. 66, 323–344. doi: 10.2307/2963521
Muir P. R., Wallace C. C., Done T., Aguirre J. D. (2015). Limited scope for latitudinal extension of reef corals. Science 348, 1135–1138. doi: 10.1126/science.1259911
Negri A., Webster N., Hill R., Heyward A. (2001). Metamorphosis of broadcast spawning corals in response to bacteria isolated from crustose algae. Mar. Ecol. Prog. Ser. 223, 121–131. doi: 10.3354/meps223121
Nguyen H. T. T., Pritchard D. W., Hepburn C. D. (2020). Nitrogen and phosphorus ecophysiology of coralline algae. J. Appl. Phycol. 32, 2583–2597. doi: 10.1007/s10811-019-02019-w
Nishihara G. N., Terada R. (2010). Species richness of marine macrophytes is correlated to a wave exposure gradient. Phycol. Res. 58, 280–292. doi: 10.1111/pre.2010.58.issue-4
Oouchi K., Yoshimura J., Yoshimura H., Mizuta R., Kusunoki S., Noda A. (2006). Tropical cyclone climatology in a global-warming climate as simulated in a 20 km-mesh global atmospheric model: Frequency and wind intensity analyses. J. Meteorol. Soc Jpn. Ser. II 84, 259–276. doi: 10.2151/jmsj.84.259
Park Y.-H. (1986). Water characteristics and movements of the Yellow Sea Warm Current in summer. Prog. Oceanogr. 17, 243–254. doi: 10.1016/0079-6611(86)90047-9
Park T., Jang C. J., Jungclaus J. H., Haak H., Park W., Sang Oh I. (2011). Effects of the Changjiang River discharge on sea surface warming in the Yellow and East China Seas in summer. Cont. Shelf Res. 31, 15–22. doi: 10.1016/j.csr.2010.10.012
Park J.-K., Kim B.-S., Jung W.-S., Kim E.-B., Lee D.-G. (2006). Change in statistical characteristics of typhoon affecting the Korean Peninsula. Atmosphere 16, 1–17.
Park K.-A., Park J.-E., Choi B.-J., Lee S.-H., Shin H.-R., Lee S.-R., et al. (2017). Schematic maps of ocean currents in the Yellow Sea and the East China Sea for science textbooks based on scientific knowledge from oceanic measurements. Sea J. Korean Soc Oceanogr. 22, 151–171. doi: 10.7850/JKSO.2017.22.4.151
Pecl G. T., Araújo M. B., Bell J. D., Blanchard J., Bonebrake T. C., Chen I.-C., et al. (2017). Biodiversity redistribution under climate change: Impacts on ecosystems and human well-being. Science 355, eaai9214. doi: 10.1126/science.aai9214
Pinsky M. L., Selden R. L., Kitchel Z. J. (2020). Climate-driven shifts in marine species ranges: Scaling from organisms to communities. Annu. Rev. Mar. Sci. 12, 153–179. doi: 10.1146/annurev-marine-010419-010916
Powell A., Smith D. J., Hepburn L. J., Jones T., Berman J., Jompa J., et al. (2014). Reduced diversity and high sponge abundance on a sedimented Indo-Pacific reef system: Implications for future changes in environmental quality. PloS One 9, e85253. doi: 10.1371/journal.pone.0085253
Reimer J. D., Wee H. B., López C., Beger M., Cruz I. C. (2021). “Widespread Zoanthus and Palythoa dominance, barrens, and phase shifts in shallow water subtropical and tropical marine ecosystems,” in Oceanography and marine biology (CRC Press, Boca Raton), 533–557. doi: 10.1201/9781003138846-7
Ribas-Deulofeu L., Loubeyres M., Denis V., De Palmas S., Hwang S.-J., Woo S., et al. (2023). Jeju Island: A sentinel for tracking ocean warming impacts on high-latitude benthic communities. Coral Reefs 42, 1097–1112. doi: 10.1007/s00338-023-02400-9
Sakamoto T. T., Hasumi H., Ishii M., Emori S., Suzuki T., Nishimura T., et al. (2005). Responses of the Kuroshio and the Kuroshio Extension to global warming in a high-resolution climate model. Geophys. Res. Lett. 32, L14617. doi: 10.1029/2005GL023384
Scherner F., Bonomi Barufi J., Horta P. A. (2012). Photosynthetic response of two seaweed species along an urban pollution gradient: Evidence of selection of pollution-tolerant species. Mar. pollut. Bull. 64, 2380–2390. doi: 10.1016/j.marpolbul.2012.08.012
Schiel D. R., Thompson G. A. (2012). Demography and population biology of the invasive kelp Undaria pinnatifida on shallow reefs in southern New Zealand. J. Exp. Mar. Biol. Ecol. 434–435, 25–33. doi: 10.1016/j.jembe.2012.07.023
Serisawa Y., Imoto Z., Ishikawa T., Ohno M. (2004). Decline of the Ecklonia cava population associated with increased seawater temperatures in Tosa Bay, southern Japan. Fish. Sci. 70, 189–191. doi: 10.1111/j.0919-9268.2004.00788.x
Shimabukuro R., Tomita T., Fukui K. (2023). Update of global maps of Alisov’s climate classification. Prog. Earth Planet. Sci. 10, 19. doi: 10.1186/s40645-023-00547-1
Skirving W., Heron M., Heron S. (2006). “The hydrodynamics of a bleaching event: Implications for management and monitoring,” in Coastal and estuarine studies. Eds. Phinney J. T., Hoegh-Guldberg O., Kleypas J., Skirving W., Strong A. (American Geophysical Union, Washington, D. C), 145–161. doi: 10.1029/61CE09
Smale D. A., Wernberg T. (2013). Extreme climatic event drives range contraction of a habitat-forming species. Proc. R. Soc B Biol. Sci. 280, 20122829. doi: 10.1098/rspb.2012.2829
Soares M. D. O. (2020). Marginal reef paradox: A possible refuge from environmental changes? Ocean Coast. Manage. 185, 105063. doi: 10.1016/j.ocecoaman.2019.105063
Sommer B., Beger M., Harrison P. L., Babcock R. C., Pandolfi J. M. (2018). Differential response to abiotic stress controls species distributions at biogeographic transition zones. Ecography 41, 478–490. doi: 10.1111/ecog.02986
Sousa W. P. (1984). The role of disturbance in natural communities. Annu. Rev. Ecol. Syst. 15, 353–391. doi: 10.1146/annurev.es.15.110184.002033
Spalding M. D., Fox H. E., Allen G. R., Davidson N., Ferdaña Z. A., Finlayson M., et al. (2007). Marine ecoregions of the world: A bioregionalization of coastal and shelf areas. BioScience 57, 573–583. doi: 10.1641/B570707
Steneck R. S., Graham M. H., Bourque B. J., Corbett D., Erlandson J. M., Estes J. A., et al. (2002). Kelp forest ecosystems: Biodiversity, stability, resilience and future. Environ. Conserv. 29, 436–459. doi: 10.1017/S0376892902000322
Sugihara K., Yamano H., Choi K.-S., Hyeong K. (2014). “Zooxanthellate scleractinian corals of Jeju Island, Republic of Korea,” in Integrative observations and assessments ecological research monographs. Eds. Nakano S.-I., Yahara T., Nakashizuka T. (Springer Japan, Tokyo), 111–130. doi: 10.1007/978-4-431-54783-9_6
Sunday J. M., Bates A. E., Dulvy N. K. (2012). Thermal tolerance and the global redistribution of animals. Nat. Clim. Change 2, 686–690. doi: 10.1038/nclimate1539
Takatsuki Y., Kuragano T., Shiga T., Bungi Y., Inoue H., Fujiwara H., et al. (2007). Long-term trends in sea surface temperature adjacent to Japan. Sokko Jiho 74, S33–S87.
Takikawa T., Yoon J.-H. (2005). Volume transport through the Tsushima Straits estimated from sea level difference. J. Oceanogr. 61, 699–708. doi: 10.1007/s10872-005-0077-4
Tanaka K., Taino S., Haraguchi H., Prendergast G., Hiraoka M. (2012). Warming off southwestern Japan linked to distributional shifts of subtidal canopy-forming seaweeds. Ecol. Evol. 2, 2854–2865. doi: 10.1002/ece3.391
Tang Y., Huangfu J., Huang R., Chen W. (2020). Surface warming reacceleration in offshore China and its interdecadal effects on the East Asia–Pacific climate. Sci. Rep. 10, 14811. doi: 10.1038/s41598-020-71862-6
Teague W. J., Jacobs G. A., Ko D. S., Tang T. Y., Chang K.-I., Suk M.-S. (2003). Connectivity of the Taiwan, Cheju, and Korea straits. Cont. Shelf Res. 23, 63–77. doi: 10.1016/S0278-4343(02)00150-4
Tebben J., Motti C. A., Siboni N., Tapiolas D. M., Negri A. P., Schupp P. J., et al. (2015). Chemical mediation of coral larval settlement by crustose coralline algae. Sci. Rep. 5, 10803. doi: 10.1038/srep10803
Trygonis V., Sini M. (2012). photoQuad: A dedicated seabed image processing software, and a comparative error analysis of four photoquadrat methods. J. Exp. Mar. Biol. Ecol. 424–425, 99–108. doi: 10.1016/j.jembe.2012.04.018
Tuckett C. A., De Bettignies T., Fromont J., Wernberg T. (2017). Expansion of corals on temperate reefs: Direct and indirect effects of marine heatwaves. Coral Reefs 36, 947–956. doi: 10.1007/s00338-017-1586-5
Uribe R. A., Ortiz M., Macaya E. C., Pacheco A. S. (2015). Successional patterns of hard-bottom macrobenthic communities at kelp bed (Lessonia trabeculata) and barren ground sublittoral systems. J. Exp. Mar. Biol. Ecol. 472, 180–188. doi: 10.1016/j.jembe.2015.08.002
Vergés A., Steinberg P. D., Hay M. E., Poore A. G. B., Campbell A. H., Ballesteros E., et al. (2014). The tropicalization of temperate marine ecosystems: Climate-mediated changes in herbivory and community phase shifts. Proc. R. Soc B Biol. Sci. 281, 20140846. doi: 10.1098/rspb.2014.0846
Vieira C., Keshavmurthy S., Ju S.-J., Hyeong K., Seo I., Kang C.-K., et al. (2016). Population dynamics of a high-latitude coral Alveopora japonica Eguchi from Jeju Island, off the southern coast of Korea. Mar. Freshw. Res. 67, 594. doi: 10.1071/MF14330
Wernberg T., Bennett S., Babcock R. C., De Bettignies T., Cure K., Depczynski M., et al. (2016). Climate-driven regime shift of a temperate marine ecosystem. Science 353, 169–172. doi: 10.1126/science.aad8745
Wernberg T., Russell B. D., Moore P. J., Ling S. D., Smale D. A., Campbell A., et al. (2011). Impacts of climate change in a global hotspot for temperate marine biodiversity and ocean warming. J. Exp. Mar. Biol. Ecol. 400, 7–16. doi: 10.1016/j.jembe.2011.02.021
Wu L., Cai W., Zhang L., Nakamura H., Timmermann A., Joyce T., et al. (2012). Enhanced warming over the global subtropical western boundary currents. Nat. Clim. Change 2, 161–166. doi: 10.1038/nclimate1353
Yamano H., Sugihara K., Nomura K. (2011). Rapid poleward range expansion of tropical reef corals in response to rising sea surface temperatures. Geophys. Res. Lett. 38, L04601. doi: 10.1029/2010GL046474
Keywords: barren grounds, climatic transition area, environmental control, foundation species, high-latitude hard corals, kelp forest, marginal populations, ocean warming
Citation: Perrois G, Jöst AB, Lee K-T, Pons LMT, Yang H-S, Son YB, Park H-S, Kang D-H and Kim T (2024) Environmental impact on marginal coastal benthic communities within the Jeju Island, South Korea temperate transition zone. Front. Mar. Sci. 11:1345518. doi: 10.3389/fmars.2024.1345518
Received: 28 November 2023; Accepted: 05 March 2024;
Published: 08 April 2024.
Edited by:
Stelios Katsanevakis, University of the Aegean, GreeceReviewed by:
Francisco Arenas, University of Porto, PortugalRoberto Uribe Alzamora, Institute of the Sea of Peru (IMARPE), Peru
Copyright © 2024 Perrois, Jöst, Lee, Pons, Yang, Son, Park, Kang and Kim. This is an open-access article distributed under the terms of the Creative Commons Attribution License (CC BY). The use, distribution or reproduction in other forums is permitted, provided the original author(s) and the copyright owner(s) are credited and that the original publication in this journal is cited, in accordance with accepted academic practice. No use, distribution or reproduction is permitted which does not comply with these terms.
*Correspondence: Taihun Kim, tk2020@kiost.ac.kr