- Faculty of Fisheries and Protection of Waters, South Bohemian Research Center of Aquaculture and Biodiversity of Hydrocenoses, Research Institute of Fish Culture and Hydrobiology, University of South Bohemia in České Budějovice, Vodňany, Czechia
Introduction: The successful cryopreservation of common carp sperm is crucial for its application in aquaculture and selective breeding programs. This study investigates the efficacy of cryopreserving sperm in large containers (5 mL) with a low dilution rate (1:1) in three different cryoprotective media and thawing in different conditions.
Methods: The developed method utilizes a low-ionic (hypotonic) cryoprotective medium, freezing with a controlled cooling rate, and high-temperature sperm thawing (60°C). The investigation employs a detailed spermatozoon motility assessment.
Results: Post-thaw motility of 32.3% ± 14% and initial curvilinear velocity of 89 ± 20 μm/s across 30 males were observed. Principal component analysis of sperm kinematic characteristics revealed distinct populations of sperm cells exhibiting varying responses to cryopreservation. The developed method achieved successful fertilization comparable to that of the non-frozen control group using sperm from a single cryotube (2.5 mL, approximately 50 * 109 spermatozoa) to fertilize 200 g of eggs (1:120,000 egg:spz).
Discussion: This novel approach demonstrates an effective cryopreservation protocol for common carp sperm in large-volume cryo-containers in combination with low-ionic cryomedia and high thawing temperature, providing methods well-suited for fisheries practices and selective breeding programs. Future studies of the biological properties of different sperm subpopulations in post-thaw sperm samples can contribute to a deeper understanding of sperm biology, improve cryopreservation techniques, and enhance the success rates of assisted reproductive technologies.
1 Introduction
Aquaculture is a rapidly growing industry that is vital to the global food supply. The Cyprinidae family, which includes various fish species, contributes significantly to global finfish aquaculture production, accounting for approximately 50% of the total production (FAO, 2022). Common carp (Cyprinus carpio) is one of the important species in this family, with its production alone accounting for 8.6% of the total fish production worldwide in 2020 (FAO, 2022). The long history of the domestication of common carp, which dates back to ancient China (Balon, 1995), has resulted in numerous common carp strains worldwide. European carp aquaculture has utilized crossbreeding strains to achieve sustainable production for the market demand (Janssen et al., 2017).
Maintaining these carp strains for breeding programs is crucial for the continued success of carp aquaculture. That is why live gene banks have been established, and cryobanking of common carp sperm is a component of this effort in several countries (Gorda et al., 1995; Flajšhans et al., 1999). Moreover, sperm cryopreservation in fisheries practice is generally considered a potentially helpful tool (Martínez-Páramo et al., 2017). However, nowadays, only a few examples of its production-scale application in salmon, but not carp, industry exist (https://www.hatcheryinternational.com/breeders-tout-cryopreservation-to-ensure-high-quality-genetics/). While the reasons for such scarce involvement of sperm cryopreservation into the entire strategy of aquaculture practice vary greatly according to specificity of aquaculture object, the need for reliable, practice-oriented protocols is one of them (Tiersch, 2008).
The development of an optimal sperm cryopreservation protocol involves the determination of the composition of the optimal cryoprotective medium, the dilution ratio of sperm by cryoprotective medium, freezing and thawing rates, and conditions for fertilization. Notably, cryoprotective medium composition, sperm dilution ratio, and freezing and thawing rates of cryopreservation protocol cannot be chosen without consideration of cryo-container shape, as the use of different types of containers for cryopreservation led to different surface-to-volume ratios in connection with the total temperature-energy capacity of samples. These changes can dramatically change the solution’s freezing conditions and ice formation profiles, necessitating the adjustment of the cryomedium content and freezing profile. Finally, the appropriate fisheries practice protocol is the specific combination of container shape and volume, cryoprotective medium composition, and freezing/thawing parameters. Developing these methods for carp sperm was started in the 1970s (Horton and Ott, 1976), but real success was achieved much later. Nowadays, it is clear that for fisheries practice, common carp sperm should be cryopreserved in containers of relatively big volume (4–10 mL) to allow fertilization of corresponding big amount of eggs (Urbányi and Horváth, 2008); e.g., for fertilization of 1 kg of eggs, 5–10 mL of good quality sperm is needed. To meet this need, long-term research activities of different scientific teams were conducted, leading to the conclusion that carp sperm cryopreservation in big volumes can be achieved by following quite different approaches. The first approach uses 4-mL straws, a relatively high sperm:cryoprotective medium dilution rate (1:9), conventional glucose-tris-methanol cryoprotective media, and a simple cooling device without freezing rate control (Urbányi and Horváth, 2008). Another approach utilizes 1.5-mL cryotubes, a low sperm dilution rate (1:1), a simple NaCl-glucose-methanol-ethylene glycol cryoprotective medium, and a freezing rate-controlling cooling device (Dokina et al., 2019). These methods allow the individual sample of sperm of 0.5 and 0.75 mL to be frozen. Both these approaches were appropriate for obtaining a post-thaw sperm fertilizing ability comparable with non-frozen control when one 4-mL straw and at least three 1.5-mL containers were needed to fertilize 100 g of eggs. That makes both methods quite similar in terms of the volume of cryopreserved samples required for practical application. The main point of concern regarding the first approach is the relatively ineffective use of storage space (as the cryopreserved sample contains just one-tenth part of the sperm) and the presence of sperm agglutination after thawing, which potentially can affect its fertilizing capacity (Bernáth et al., 2016). For the second approach, it is unclear if this cryopreservation protocol will be realizable for containers of bigger volume, which is required for the effective fertilization of big egg batches. In addition to the above issues, most existing methods rely on the application of a 40°C water bath for carp sperm thawing, with quite rare attempts to apply other temperatures (Yavas and Bozkurt, 2011). Using a higher thawing temperature may benefit sperm quality (Francis et al., 2013). Nowadays, it is not clear if outcomes of sperm cryopreservation in big volumes can be additionally improved by screening the thawing condition, which in turn can be also related to container type.
A bigger container may be beneficial for use in aquaculture due to the increasing number of eggs that can be fertilized by one sample. At the same time, using too big a volume can be counterproductive in decreasing the number of samples obtained from one male, and a larger amount of sperm can be misused. According to Kopeika, the application of cryo-containers larger than 5 mL should be avoided (Kopeika et al., 2007).
All the above-mentioned considerations underscore the need for future advancement in practice-oriented protocols for carp sperm cryopreservation. These protocols should be additionally evaluated, considering the natural heterogeneity of common carp sperm cryo-resistance. The current study aims to develop a common carp sperm cryopreservation approach that can be implemented in routine fisheries practices, using large and easily manipulable containers, a low sperm dilution rate, and specific thawing rates.
2 Materials and methods
2.1 Ethical statement
Manipulation with animals were performed according to the authorization for breeding and delivery of experimental animals (Reference number: 56665/2016-MZE-17214 and 64155/2020-MZE-18134) and the permission of the use of experimental animals (Reference number: 68763/2020-MZE-18134) issued to the Faculty of Fisheries and Protection of Waters, the University of South Bohemia, by the Ministry of Agriculture of the Czech Republic.
2.2 General experimental plan
Two consecutive experimental stages were performed during the study. In the initial stage, preliminary experiments were conducted to determine a suitable cryopreservation protocol for carp sperm cryopreservation in large-volume containers using a low sperm dilution rate, as assessed by qualitative sperm motility analysis. This part of the study included the exploration of three cryoprotective media and various thawing temperatures using sperm samples from five common carp males. Based on the results of this initial stage, a specific cryopreservation protocol was selected for further evaluation in the second stage. The second stage involved an expanded study using sperm samples from 30 common carp males, employing quantitative sperm motility analysis to test the chosen cryopreservation protocol. Finally, sperm samples from four males among the 30 were used to test sperm fertilizing ability, and the resulting hatching rate of embryos was obtained using cryopreserved sperm.
2.3 Sperm and egg collection
Experiments were performed during artificial propagation of common carp C. carpio in the natural spawning period (May–June) in 2021–2022 in Vodnany, Czech Republic (49.1479°N, 14.1751°E). Fish with an average total length of 45 ± 9 cm and an average weight of 4.2 ± 0.7 kg were collected from aquaculture ponds and transported to hatchery tanks with a recirculating water flow rate of 0.2 L/s, temperature adjusted to 20°C with pH of 7.9–8.0, and dissolved oxygen content of 8.7–8.9 mg/L. Males were injected with carp pituitary powder extract (product of Rybníkářství Pohořelice a.s., Pohořelice, Czech Republic www.rybnikarstvi-pohorelice.cz) at 1 mg/kg per 24 hours before sperm collection. Before gamete collection, fish were anesthetized with 2-fenoksyetanol diluted in hatchery water at a 0.3 mL/L concentration. Sperm was collected by an abdominal massage directly into a 250-mL plastic cell culture container, avoiding contamination with urine, mucus, feces, or water. Sperm samples from different males were stored individually on ice at 4°C, for no longer than 2 hours, before freezing. Samples with motility higher than 80% were used in the father investigation. Sperm concentration and semen volume ranged from 25–32 * 109 spz/mL and 7–25 mL, respectively. Females were injected with carp pituitary powder extract twice: at 0.3 mg/kg, 24 hours before egg collection, and 2.7 mg/kg, 12 hours after the first injection. Eggs were obtained by an abdominal massage directly into dry plastic bowls.
2.4 Sperm motility data acquisition
In the initial stage of experiments in the preliminary investigation, sperm motility percentage was visually assessed by microscopic inspection and scoring of sperm motility by the same examiner (Dzuba and Kopeika, 2002). In the second stage of the study, sperm motility was video-recorded for 60 s after motility activation in two repetitions for future examination of video records by computer-assisted sperm analysis (CASA). In both methods, sperm motility was activated in an ISAS SpermTrack-10 sperm counting chamber (PROISER, Valencia, Spain) using an activating medium (AM) consisting of 30 mMi Tris, 45 mM NaCl, and 5 mM KCl, pH 8.0 (Horvath et al., 2003), supplemented with 0.125% Pluronic F-127 (catalog number P2443, Sigma-Aldrich, St. Louis, MO, USA) to avoid sperm sticking to the glass slide. A microscope with optical negative phase-contrast, ×10 magnification (PROISER, Spain), and an IDS digital camera (IDS Imaging Development Systems GmbH, Obersulm, Germany) were used for sperm motility observation and video recording, respectively. The videos were analyzed using the CASA plugin for ImageJ (Wilson-Leedy and Ingermann, 2007; Purchase and Earle, 2012). The following parameters were evaluated: percent of motile cells (%), VCL (curvilinear velocity; µm/s), VAP (average path velocity; µm/s), VSL (straight-line velocity; µm/s), LIN (linearity of the path; VSL/VAP), WOB (oscillation of the track; VAP/VCL), and BCF (beat-cross frequency; Hz).
2.5 Sperm cryopreservation
Three cryoprotective media known to be appropriate for common carp sperm cryopreservation using low sperm dilution rate were checked in the first stage of the study. These media were as follows: 1) 110 mM NaCl, 240 mM KCl, 3.5 mM CaCl2, 1.5 mM MgCl2, and 4.3 mM NaHCO3 with 18% v/v dimethyl sulfoxide (DMSO) (Sotnikov et al., 2023), modified after Magyary et al. (1996); 2) 59 mM NaCl, 6.3 mM KCl, 0.68 mM CaCl2, 2.1 mM Mg2SO4, 27 mM NaHCO3, 3.4 mM sucrose, 69 mM d-mannitol, 118 mM Tris–HCl, pH 8.1, 16% ethylene glycol, and 12% egg yolk, modified after Kopeika et al. (2007); 3) 60 mM NaCl and 3 mM sucrose, with 5% v/v ethylene glycol and 22% v/v methanol (Dokina et al., 2019). Sperm samples were diluted 1:1 with one of these extenders and loaded into 4.5-mL cryotubes (Thermo Fisher Scientific, Shanghai, China). After 10 min of incubation on the surface of the ice (at approximately 4°C), cryotubes were frozen using a programmable freezer (PLANER Kryo 10 series III, Planer Limited, Sunbury-On-Thames, United Kingdom). Samples were cooled by a two-stage freezing regime. In the first stage, the chamber with samples was cooled at −2°C/min until the crystallization point was reached (detected by a temperature spike in the sample during freezing) and afterward with a cooling rate of −20°C/min down to −140°C, followed by sample transfer into liquid nitrogen. The temperature inside the samples was monitored using a Chromel and Alumel (type K) thermocouple with a diameter of 0.25 mm (Model 5SRTC-KK-K-30-72, Omega, Stamford, CT, USA), connected to a 2-Channel Thermocouple Data Logger (HH127 TC datalogger thermometer, Omega, Stamford, CT, USA) inserted into the center of the cryotubes. The temperature was recorded at 1-s intervals. During preliminary experiments, a water bath with a temperature in the range of 40°C–80°C with a 10°C increment was used to select the appropriate temperature and time of thawing. At the initial experimental stage, optimal thawing temperature was determined considering two factors: temperature in the center of the container recorded by the thermocouple and visual inspection of the presence of liquid phase in the sample (Kopeika et al., 2007) frozen using cryomedium (3). Cryotubes were actively stirred inside the water bath to determine the thawing time until the sample temperature in the center of the cryotubes reached 10°C. After thawing at the optimal thawing time, samples were removed from the water bath, actively shaken, and transferred to an ice-containing box before motility analysis or fertilization tests for up to 10 min.
2.6 Fertilization test
For fertilization trials, a mix of eggs from eight females was used. A 200-g mixed-egg batch was used for each repetition of the fertilization test. In the case of fresh sperm collected from the same males that were used for cryopreservation 1 year before, 2.25 mL was used for fertilization. In the case of cryopreserved samples, a whole cryotube, 4.5 mL (i.e., 2.25 mL of cryopreserved sperm), a half cryotube (1.125 mL of cryopreserved sperm), and 2 cryotubes (4.5 mL of cryopreserved sperm) were used. After mixing sperm with eggs, 50 mL of activation media (same as in 2.4) was added. After 2 min of mixing, the activation media were removed by pipetting, and after a period of controlled swelling in hatchery water, eggs were treated with tannic acid to avoid stickiness. After the treatment, the eggs were transferred to 2-L Kannengieter jars and incubated at 20°C. Aliquots of 150–200 eggs from each fertilization test were incubated separately in Petri dishes to monitor the development rate and hatching rates. The sperm/egg ratio ranged from 60,000 spz/egg in the case of adding 0.5 cryotubes to 240,000 spz/egg in the case of adding 2 cryotubes. After 5 days of incubation, the total number of hatched larvae was calculated by the volumetric method during the release of all hatched larvae into rearing tanks.
2.7 Statistical analysis
2.7.1 Sperm motility
At the second stage of the study, motility parameters were found by CASA from video records performed in duplicate of sperm samples from 30 males in two experimental conditions (native and cryopreserved in cryotubes of 4.5-mL volume); sperm motility traits were calculated with 1-s interval from 10 to 60 s post-activation. Altogether, data from 442,539 spermatozoa were obtained. All obtained CASA parameters were analyzed using two approaches. In the first multivariate exploratory approach, the general structure of CASA data was visualized using principal component (PC) analysis. Calculation of three PCs was performed for the presentation of averaged data of kinematic parameters and motility percent by Wafer plots. For this, different post-activation times were used, and computation was performed of two PCs for the presentation of kinematic parameters of each spermatozoon at 10 s with the representation of data centroids (the dot with averaged PC1–PC3 coordinates) and plotting 95% confidence (prediction) ellipses. In the second approach, oriented on quantifying differences in motility parameters, averaged by male and treatment motility percent, VCL and LIN were used to determine statistically significant differences among groups. As data have heterogeneous variations as determined by Levene’s test, a non-parametric repeated-measures ANOVA (Friedman’s test) was performed. In this analysis, as an independent factor, type of sperm (native and cryopreserved) was used. The possible individual variations of males were not considered as a research question, which was a limitation of the study. In the case of pair-wise comparisons, the Wilcoxon matched pairs test was used. All statistical analyses were performed using Statistica (data analysis software system, version 14.0.0.15, TIBCO Software Inc. (2020), http://tibco.com). Statistical hypotheses in the applied tests were rejected at p < 0.05. Data are presented as the median and 25% percentiles in the figures.
2.7.2 Development and hatching rates
The data on development and hatching rates for larvae hatched in Petri dishes and total number of larvae obtained during experiments were analyzed by a non-parametric Kruskal–Wallis ANOVA followed by multiple comparisons of mean ranks for all groups because of the small number of repetitions (n = 4).
3 Results
3.1 Experimental stage 1: determination of optimal cryomedium and thawing regime
Examples of typical thermograms at cooling and thawing of the samples at different temperatures are presented in Figure 1. The crystallization point of sperm suspended in the third cryoprotective medium was observed at −8°C to −9°C, with the ice formation process lasting 2.8–3 min (Figure 1A). During thawing, the appearance of the liquid phase preceded the complete melting of the sample. The time between the appearance of the liquid phase and the point where the temperature in the center of the container reached 0°C decreased gradually, ranging from 38 s at thawing at 40°C to 7 s at 70°C (Figure 1B). The optimal thawing times were determined to be 140 s, 120 s, 100 s, and 80 s at temperatures of 40°C, 50°C, 60°C, and 70°C, respectively (Figure 1B).
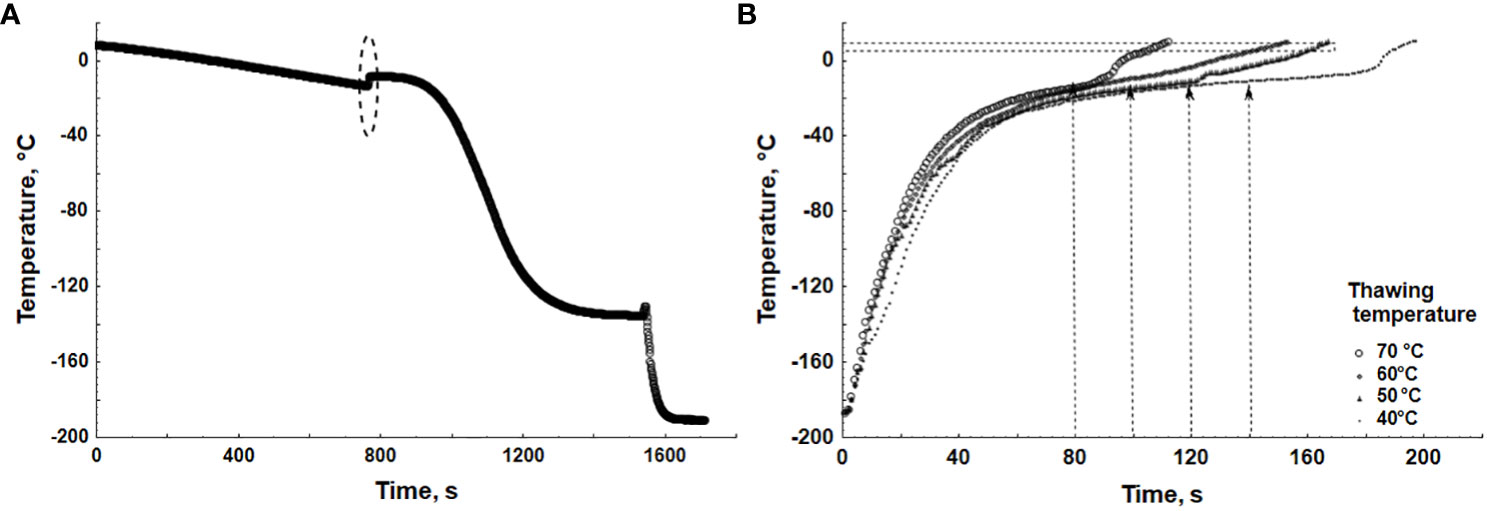
Figure 1 Thermograms at the sample’s cooling and thawing. (A) Freezing; the dotted region shows the area of supercooling and the start of ice formation at the freezing point; after this point, the cooling rate in the freezer was switched from −2°C/min to −20°C/min. (B) Thawing at water bath temperatures of 40°C–70°C; horizontal lines represent temperature ranges at which the appearance of the liquid phase in samples was observed; vertical lines represent thawing time corresponding to each thawing temperature.
The application of various cryoprotective media and thawing at 40°C for sperm samples obtained from five males revealed that the highest post-thaw motility percent was observed after the application of cryomedium “3” (Dokina et al., 2019) (Table 1). The application of increased thawing temperature led to an increase in the past-thaw percent of motile spermatozoa (Table 2). For the next step of experiments, thawing at 60°C was selected.
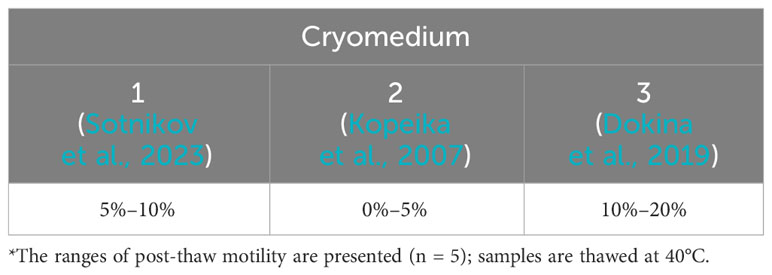
Table 1 Qualitative description of post-thaw sperm motility of carp sperm frozen in cryotubes of 4.5-mL volumes with different cryomedia*.
3.2 Experimental stage 2: quantitative sperm motility analysis and fertilization trial
3.2.1 Sperm motility analysis
The visualization of the general structure of CASA data obtained at different post-activation times of sperm samples by principal components 1–3 is presented in Figure 2, and the principal components’ loadings are shown in Table 3. Results of the PC analysis indicated that PC1 highly correlated with motility percent and all kinematic parameters except BCF and LIN. At the same time, PC2 positively correlated with VCL, VSL, and BCF and highly negatively correlated with LIN and STR. The PC3 is highly correlated with motility percent and ALH (see principal component loadings in Table 3). Eigenvalues for PC1–PC3 were in the range of 5.632–0.576. Percent of explained variances was calculated to be 70%, 15%, and 7% for PC1, PC2, and PC3, respectively, corresponding to a 93% cumulative proportion of variance explained.
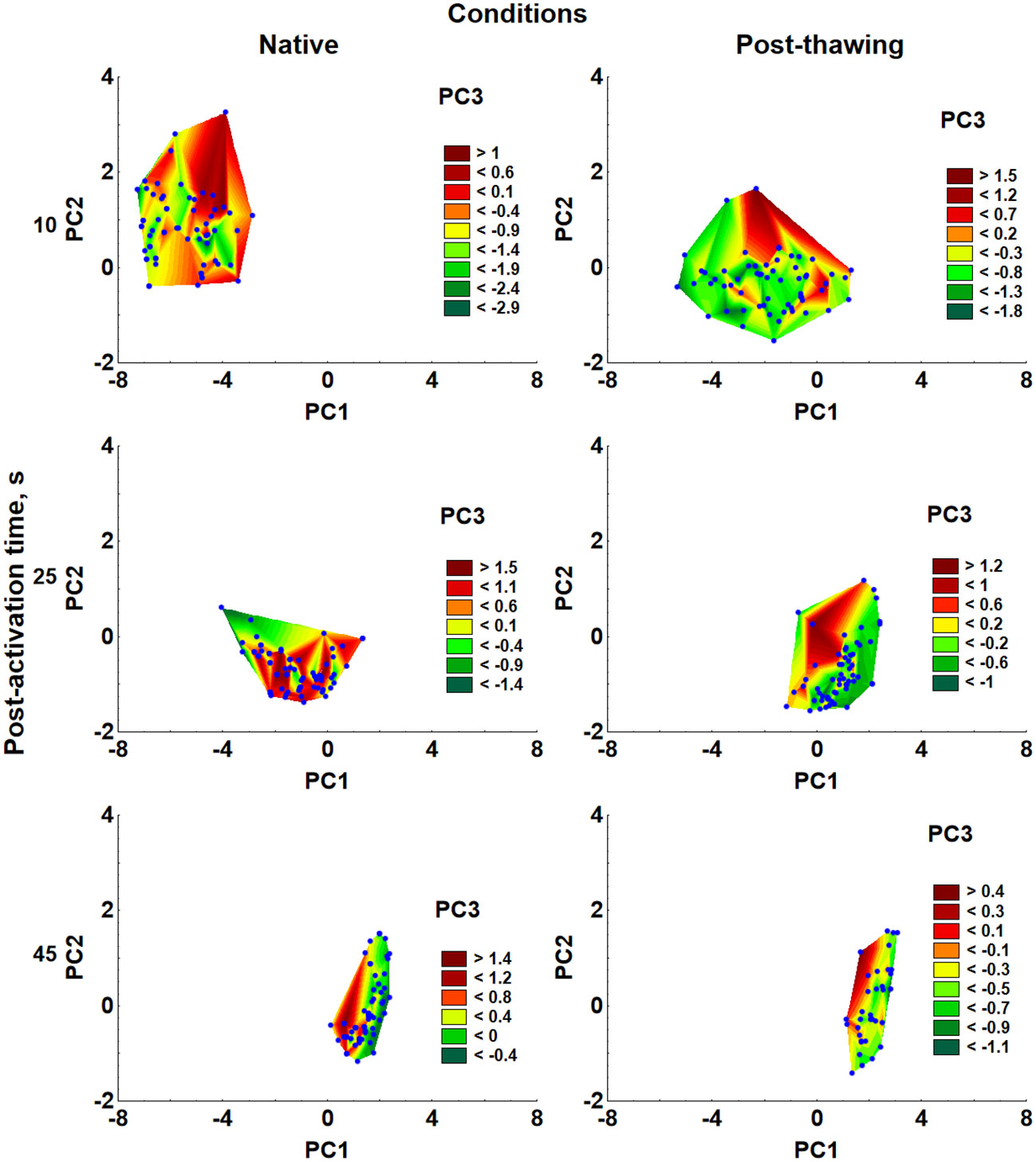
Figure 2 Wafer plots of three principal components (PC1, PC2, and PC3) were obtained after sperm kinematic parameter analysis in samples of sperm before (native) and after (post-thawing) cryopreservation at different post-activation times. Dots represent individual sperm samples in PC1–PC2 coordinates. Precise coordinates are presented in Table 3.
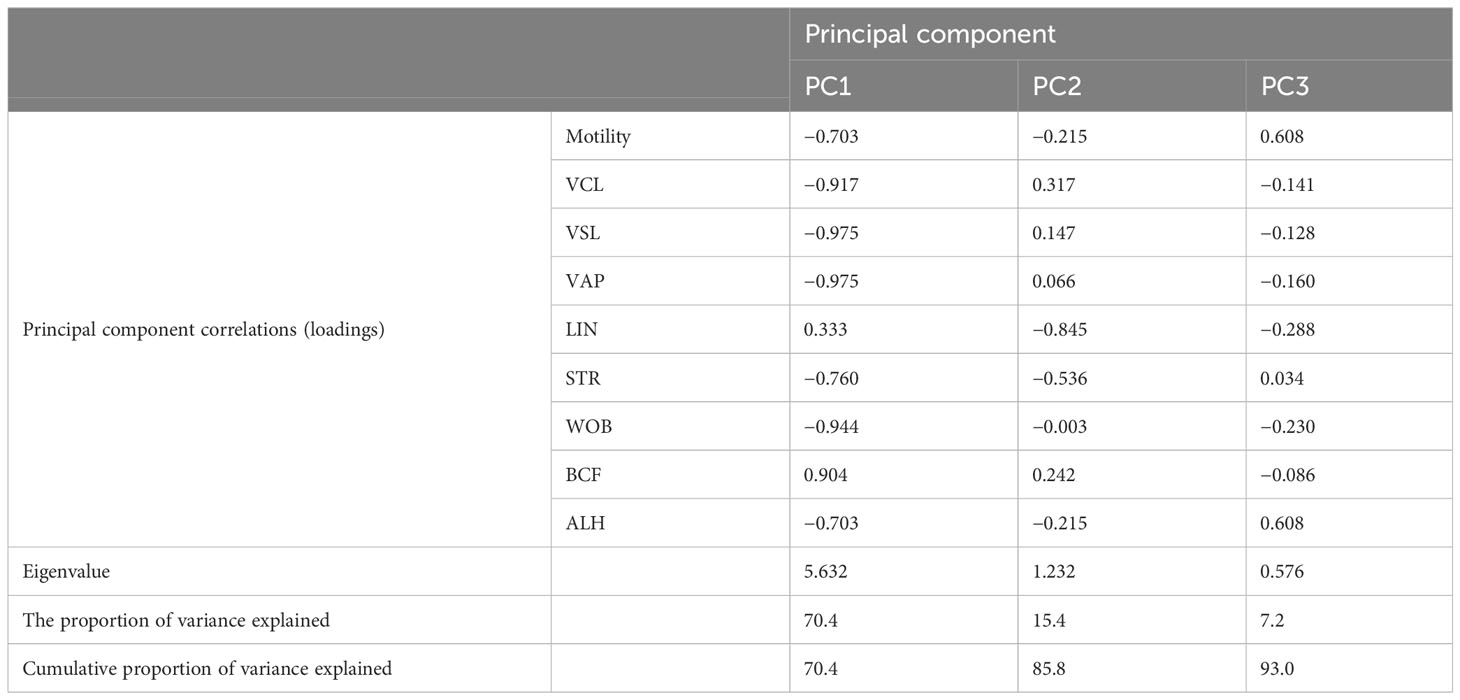
Table 3 Principal component loadings of the dataset of averaged sperm motility percent and kinematic parameters.
The obtained data in PC1–PC3 coordinates for each experimental group are presented as Wafer plots at 10 s, 25 s, and 40 s post-activation. The plots highlight the most remarkable differences in motility parameters at 10 s post-activation time and then diminishing by 40 s post-activation (see Figure 3). Notably, these changes are qualitatively linked to a general reduction in sperm kinematic parameters associated with sperm velocity (VCL, VAP, and VSL) and an increase in BCF and LIN, which are a result of both post-activation time and the impact of cryopreservation.
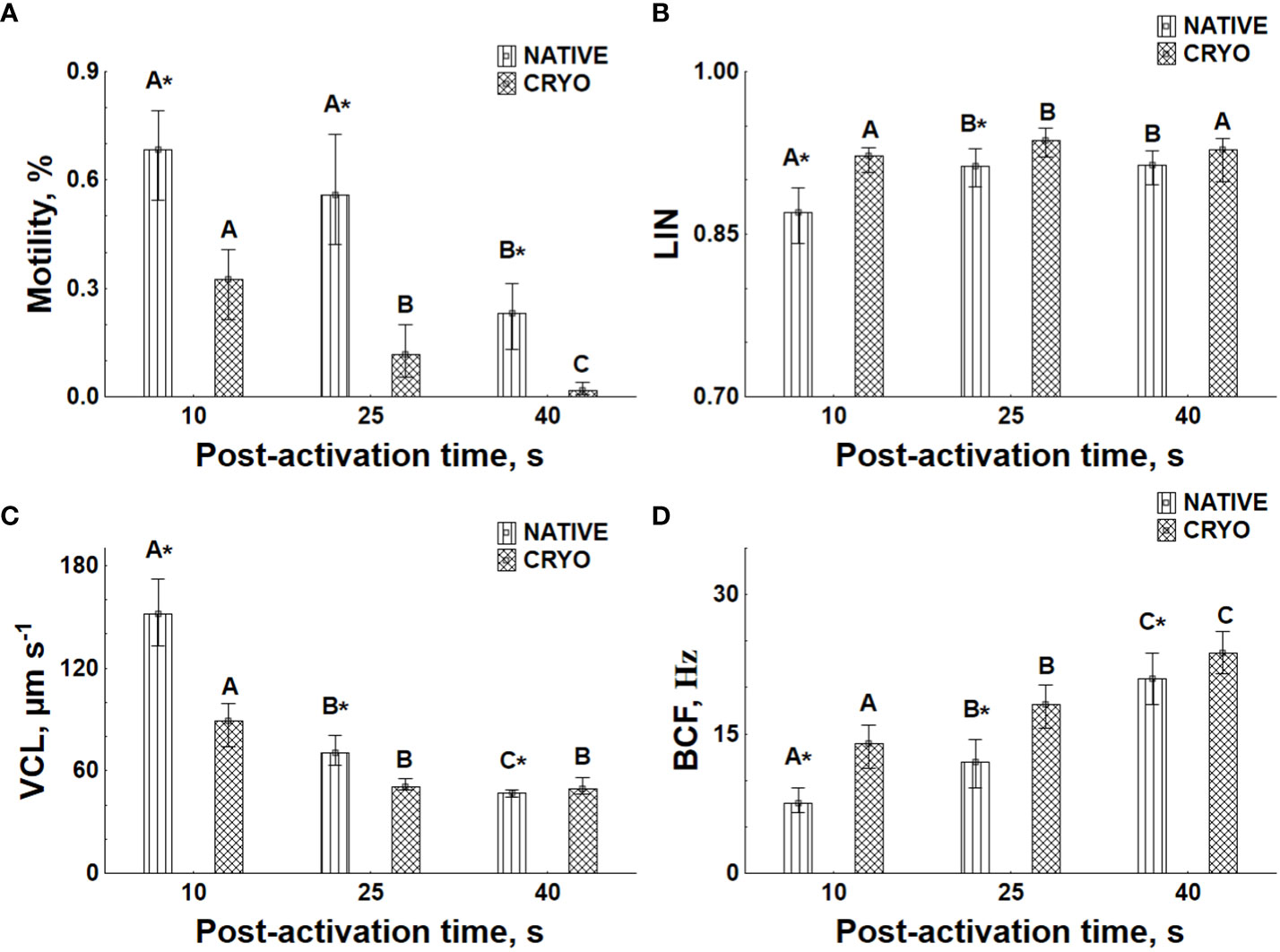
Figure 3 Motility parameters of sperm samples in different activation conditions. NATIVE, before cryopreservation; CRYO, after sperm cryopreservation and thawing. Sperm motility, curvilinear velocity (VCL), linearity (LIN), and beat-cross frequency (BCF) are presented in (A–D) correspondingly. Data are presented as median and 25th–75th percentiles (n = 30). Data with different uppercase letters are different among the same experimental group at different post-activation times (non-parametric repeated-measures ANOVA, p < 0.05). The asterisk indicates a significant difference between groups at the same post-activation time (Wilcoxon matched pairs test, p < 0.05).
To validate these qualitative observations, non-parametric repeated-measures ANOVA, followed by the Wilcoxon matched pairs test as a post hoc, revealed a significant decrease in motility and VCL during post-activation time (Figures 3A, B). In particular, a significant decrease in these parameters due to cryopreservation was observed at 10 s, 25 s, and 40 s post-activation, with the exception of VCL at 40 s. The opposite effects of post-activation time and cryopreservation on LIN and BCF were found (Figures 3C, D).
The principal component plot of kinematic parameters at 10 s post-activation time (Figure 3A) indicates the presence of a distinct sperm fraction in samples after cryopreservation, which does not differ from native spermatozoa. This specific fraction in cryopreserved samples is characterized by high-velocity parameters, i.e., VSL, VCL, and VSL (Figure 3B).
The data in PC1–PC2 coordinates for kinematic parameters of each spermatozoon from native and cryopreserved samples at 10 s post-activation are shown in Figure 4. Generally, remarkable differences in localization on PC plots of spermatozoa from distinct experimental groups are quite obvious. The PC1 coordinates of centroids of the post-thawing group exhibit a shift from positive to negative values relative to the control group. Considering PC loadings (Figure 4B), these data correspond to the differences in sperm motility parameters (see Figure 2; Table 3). Simultaneously, the presence of highly overlapping 95% confidence ellipses suggests the existence of a sperm subpopulation in the sperm samples after cryopreservation. This subpopulation has motility parameters quite similar to those of native sperm samples.
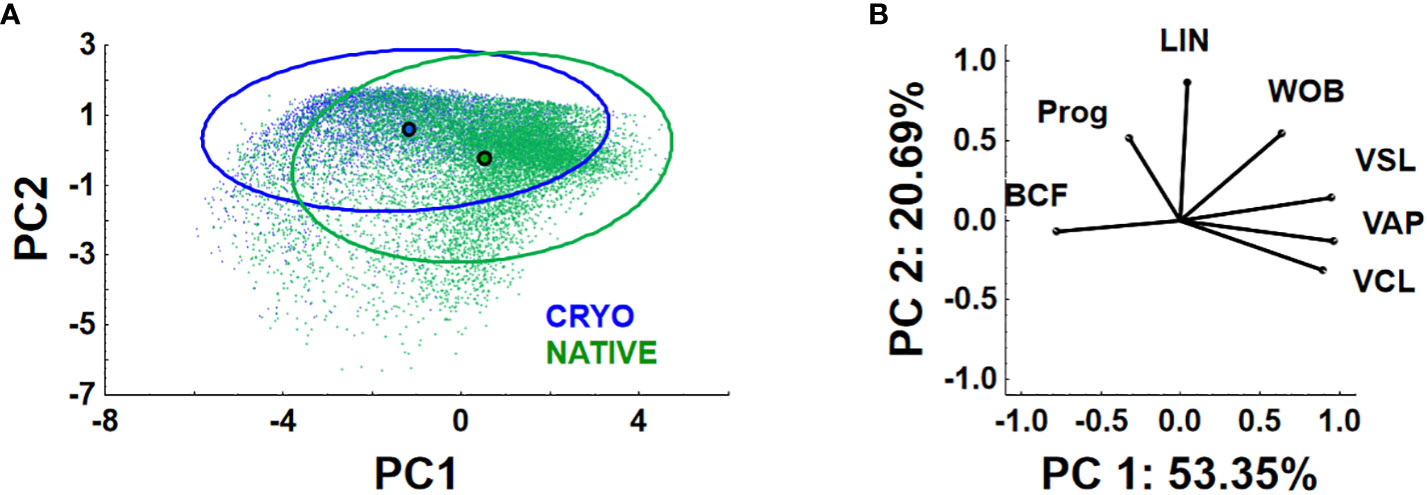
Figure 4 Results of principal component analysis of the entire dataset of sperm motility parameters (PC1 and PC2) obtained after sperm kinematic parameter analysis in samples of sperm before (native) and after (cryo) cryopreservation at 10 s post-activation time. VCL (curvilinear velocity), VAP (average path velocity), VSL (straight-line velocity), LIN (linearity of the path), WOB (oscillation of the track), and BCF (beat-cross frequency). Dots represent individual spermatozoons in PC1–PC2 coordinates, and circles are centroids of experimental groups (A). Ellipses are 95% confidence (prediction) ones. Principal component loadings are presented in panel (B).
3.2.2 Fertilization tests
The performed fertilization tests demonstrated a robust post-thaw sperm fertilizing ability, ranging from 77% to 84% in terms of development rate and with hatching rates between 61% and 69%. Total number of larvae in the range of 83–108 * 103 was obtained from 200 g of eggs. Notably, the only significant decrease in development rate and total number of larvae compared with control was observed when half of a sample from a cryotube was used to fertilize 200 g of eggs (Figure 5). However, the hatching rate was also significantly decreased in the case of the 0.5 cryotube and 2 cryotubes.
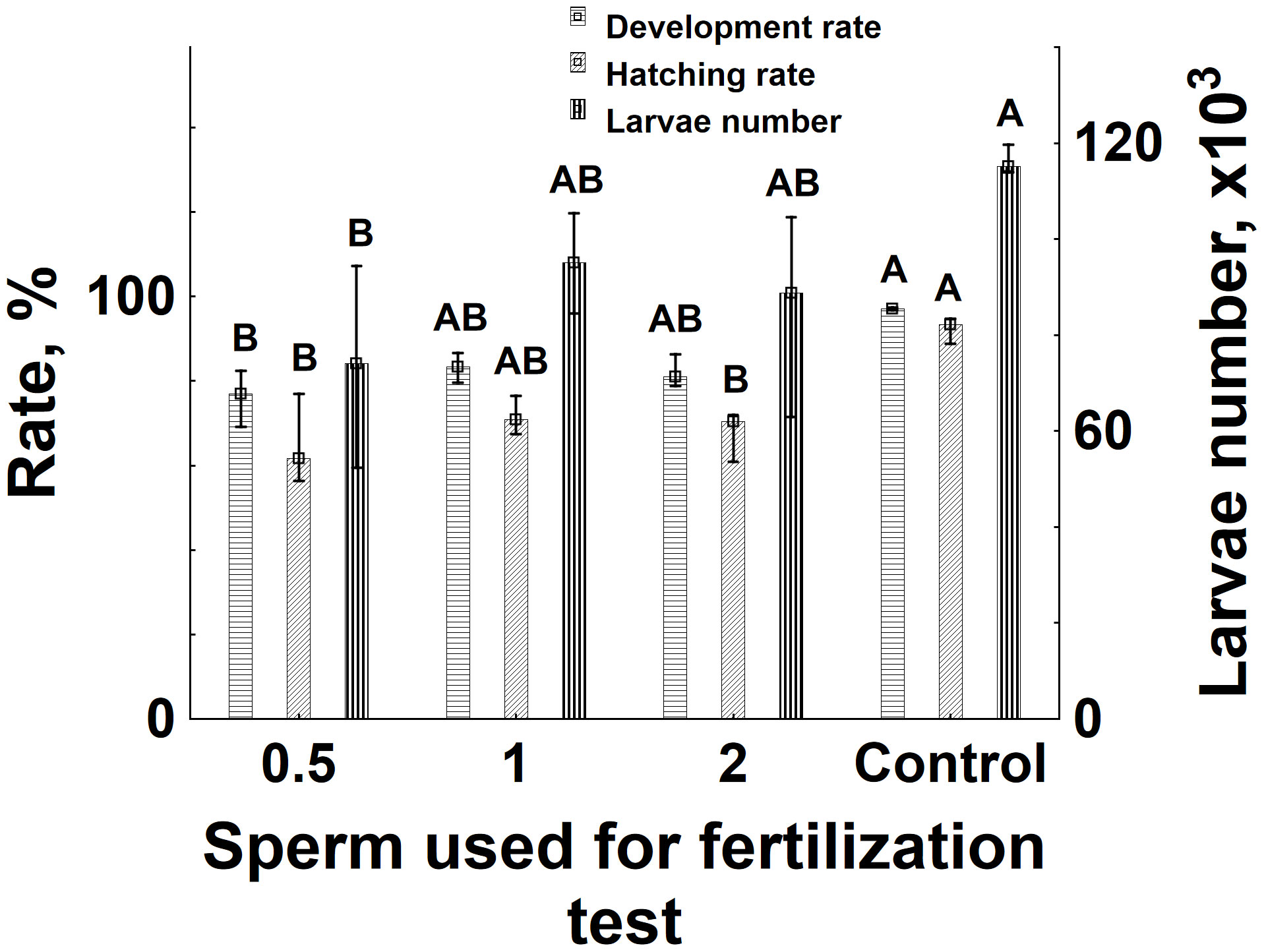
Figure 5 Sperm fertilizing ability before and after cryopreservation. Development and fertilization rates, along with the total number of larvae obtained during experiments, are presented. The indices of 0.5, 1, and 2 represent the number of cryotubes used to fertilize 200 g of eggs with cryopreserved sperm. In the control group, 2.25 mL of fresh sperm, equivalent to the sperm volume in a 4.5-mL cryotube, was used. Data labeled with different uppercase letters within the same group are significantly different (Kruskal–Wallis ANOVA followed by multiple comparisons of mean ranks for all groups, p < 0.05).
4 Discussion
The cryopreservation of common carp sperm has undergone extensive development over the years, and numerous different approaches have been explored for application in aquaculture methods (Urbányi and Horváth, 2008). Considering the specific properties of carp sperm, such as an ability of motility activation only in hypotonic conditions associated with spermatozoon swelling (Bondarenko et al., 2013) and notably high sperm density (Cejko et al., 2011), the key advancements in carp sperm cryopreservation can be summarized through the following descriptors. Usually, to prevent sperm motility activation, the isotonic or hypertonic cryomedia should be used (Aygül et al., 2022), and to avoid excessive sperm squeezing during freezing (Morris et al., 2012), a relatively high sperm dilution by cryoprotective medium has been frequently applied (Lahnsteiner et al., 2000). However, recently, several reports have challenged these conventions. First, as suggested by Dokina et al. (2019), a hypotonic carp sperm cryoprotective medium has been successively applied for carp sperm using a low dilution rate (1:1). Second, several studies have demonstrated that application of low sperm dilution rates is not a critically dangerous factor for successful common carp sperm cryopreservation (Pataki et al., 2022; Sotnikov et al., 2023). These findings provide a robust background for the current study.
A fundamental understanding of cryobiology determines the key prerequisites for successful cryopreservation of biological samples. Among these, the thawing rate of cryopreserved samples is crucial to prevent processes like ice recrystallization and avoid osmotic stress during thawing (Henry et al., 1993). However, contemporary knowledge associates the optimal thawing rate with factors such as cell type, freezing rate, and composition of cryoprotective medium (Baboo et al., 2019). Since the thawing rate within the sample strongly depends on external temperature and container shape, the overall cryopreservation success requires the appropriate thawing conditions for specific freezing methods. This critical task was, among others, solved in the current study, which provides, for the first time, the proper combination of cryoprotective medium and freezing and thawing rates appropriate for the cryopreservation of carp sperm in containers of large volume and low dilution rate. This combination distinguishes the described method from the methods already described for big sperm volume cryopreservation, which often involves high dilution rates (Horvath et al., 2007; Várkonyi et al., 2019; Bokor et al., 2023) or low dilution rates; the fertilizing ability was not assessed (Várkonyi et al., 2018).
The current study’s findings revealed a significant decrease in the percentage of motile cells and curvilinear velocity (VCL) during the cryopreservation of carp sperm, accompanied by an increase in LIN and BCF. Consistent with numerous prior investigations on common carp motility parameters, our results align with reported trends in motility percentage, VCL, and LIN (Boryshpolets et al., 2009; Dzyuba et al., 2010; Dzyuba et al., 2013; Bernáth et al., 2016; Boryshpolets et al., 2017; Marinović et al., 2021). However, the observed disparity in BCF outcomes contradicts data presented in several studies (Várkonyi et al., 2019; Marinović et al., 2021) and aligns with research conducted in non-cyprinid species (Gallego et al., 2017). Although the underlying reasons for these discrepancies remain challenging to elucidate due to the limited number of comparable examples, it is supposed that both cryopreservation and post-activation time may contribute to increased dribbling of spermatozoa during their motility, as suggested by the observed rise in BCF (Wilson-Leedy and Ingermann, 2007). The biological significance of this behavior needs further investigation. Agglutination of thawed samples was not detected when this protocol was used. Nevertheless, the preservation of high post-thaw sperm fertilizing ability implies that this alteration in motility may not be of critical importance. Future studies should investigate the biological implications of these observed changes in sperm motility.
In the present study, the identification of sperm subpopulations with similar motility characteristics in both fresh and cryopreserved samples represents a novel and significant finding. While analogous observations have been documented in a few studies (Gallego et al., 2017; Marinović et al., 2021), the general importance of this discovery for the future development of sperm cryopreservation technologies should be emphasized. The presence of sperm subpopulations in post-thaw carp sperm, mirroring the motility parameters of native sperm, is intriguing and holds promise for advancing our understanding of the relationships between sperm motility parameters and fertilizing ability.
The potential role of observed sperm populations in obtaining genetically uncompromised progeny using cryopreserved sperm remains unclear. Adverse effects of sperm cryopreservation have been reported in various fish species, such as brown trout and common carp. In brown trout, cryopreservation showed no significant impact on fertilization rates, growth, and survival during the embryo stage. Nevertheless, it led to a significantly reduced larval growth after hatching (Nusbaumer et al., 2019). Conversely, in common carp, significantly lower hatching and larval survival rates were observed in progeny obtained using cryopreserved sperm compared to those with non-frozen sperm, and unlike the example with brown trout, the growth and viability in larvae propagated from cryopreserved sperm were comparable to those from fresh sperm (Bokor et al., 2023). These discrepancies underscore the need for further studies to understand the risks of applying cryopreserved sperm in biodiversity conservation measures.
As we mentioned above, the possible individual variability of males was not assessed in this study because we were interested in the influence of cryopreservation on the general spermatozoon population response. However, future research oriented on the individual properties of males that can influence their cryo resistance must be investigated, including motility parameters and bioenergetic and membrane composition characteristics.
Worth mentioning is that one of the possible approaches to address the observed effects of cryopreservation is the application of post-thaw sperm fractionation, which was developed specifically for common carp, allowing the extraction of minimally damaged spermatozoa from samples after cryopreservation (Li et al., 2010). However, the current understanding of the approach does not help clarify if its application will effectively help eliminate the negative impact of sperm cryopreservation on the future performance of the obtained progeny.
5 Conclusions
The study introduces a novel cryopreservation protocol that merges the best practices from prior research and proves the reliability of a new type of cryovial and a higher thawing temperature. The results indicate that the high sperm fertilizing ability after using this protocol is comparable to native sperm. Furthermore, this new approach demonstrates a potential for easy scalability and can be widely adopted in aquaculture. The effectiveness of hypotonic cryoprotective media for different species should be further explored, especially taking into account the possible application of methods of targeted post-cryo extraction of “superior” spermatozoa from the whole sperm samples to mitigate potential negative effects of cryopreservation.
Data availability statement
The raw data supporting the conclusions of this article will be made available by the authors, without undue reservation.
Ethics statement
Manipulation with animals were performed according to the authorization for breeding and delivery of experimental animals (Reference number: 56665/2016-MZE-17214 and 64155/2020-MZE-18134) and the permission of the use of experimental animals (Reference number: 68763/2020-MZE-18134) issued to the Faculty of Fisheries and Protection of Waters, the University of South Bohemia by the Ministry of Agriculture of the Czech Republic. The study was conducted in accordance with the local legislation and institutional requirements.
Author contributions
AS: Conceptualization, Data curation, Formal analysis, Investigation, Software, Visualization, Writing – original draft, Writing – review & editing. MR: Methodology, Project administration, Resources, Writing – review & editing. DG: Project administration, Resources, Writing – review & editing. SB: Project administration, Supervision, Writing – review & editing, Funding acquisition. VKh: Investigation, Visualization, Writing – review & editing. VKa: Investigation, Methodology, Resources, Writing – review & editing. OL: Funding acquisition, Resources, Writing – review & editing. BD: Conceptualization, Formal analysis, Investigation, Methodology, Project administration, Supervision, Validation, Visualization, Writing – original draft, Writing – review & editing, Funding acquisition.
Funding
The author(s) declare financial support was received for the research, authorship, and/or publication of this article. The study was financially supported by the Czech Science Foundation (No. GACR 22-14069S), by the Ministry of Education, Youth and Sports of the Czech Republic – projects “CENAKVA” (LM2018099), by the project Biodiversity (CZ.02.1.01./0.0/0.0/16_025/0007370 Reproductive and genetic procedures for preserving fish biodiversity and aquaculture), and by the project NAZV (No. QK21010141).
Acknowledgments
Dr. Viktoriya Dzyuba is acknowledged for her help with editing the text of the manuscript.
Conflict of interest
The authors declare that the research was conducted in the absence of any commercial or financial relationships that could be construed as a potential conflict of interest.
Publisher’s note
All claims expressed in this article are solely those of the authors and do not necessarily represent those of their affiliated organizations, or those of the publisher, the editors and the reviewers. Any product that may be evaluated in this article, or claim that may be made by its manufacturer, is not guaranteed or endorsed by the publisher.
References
Aygül E., Güneş Y., Menekşe Didem D. (2022). “Cryopreservation Studies in Aquaculture from Past to Present: Scientific Techniques and Quality Controls for Commercial Applications,” in Cryopreservation. Ed. Marian Q. (Rijeka: IntechOpen).
Baboo J., Kilbride P., Delahaye M., Milne S., Fonseca F., Blanco M., et al. (2019). The impact of varying cooling and thawing rates on the quality of cryopreserved human peripheral blood T cells. Sci. Rep. 9 (1), 3417. doi: 10.1038/s41598-019-39957-x
Balon E. K. (1995). Origin and domestication of the wild carp, cyprinus-carpio - from roman gourmets to the swimming flowers. Aquaculture 129 (1-4), 3–48. doi: 10.1016/0044-8486(94)00227-F
Bernáth G., Żarski D., Kása E., Staszny Á., Várkonyi L., Kollár T., et al. (2016). Improvement of common carp (Cyprinus carpio) sperm cryopreservation using a programable freezer. Gen. Comp. Endocrinol. 237, 78–88. doi: 10.1016/j.ygcen.2016.08.013
Bokor Z., Láng Z. L., Várkonyi L., Fodor F., Nagy B., Csókás E., et al. (2023). The growth performance of pond-reared common carp (Cyprinus carpio) larvae propagated using cryopreserved sperm. Fish Physiol. Biochem. doi: 10.1007/s10695-023-01245-x
Bondarenko O., Dzyuba B., Cosson J., Yamaner G., Prokopchuk G., Psenicka M., et al. (2013). Volume changes during the motility period of fish spermatozoa: interspecies differences. Theriogenology 79 (5), 872–881. doi: 10.1016/j.theriogenology.2013.01.005
Boryshpolets S., Dzyuba B., Rodina M., Li P., Hulak M., Gela D., et al. (2009). Freeze-thawing as the factor of spontaneous activation of spermatozoa motility in common carp (Cyprinus carpio L.). Cryobiology 59 (3), 291–296. doi: 10.1016/j.cryobiol.2009.08.005
Boryshpolets S., Sochorova D., Rodina M., Linhart O., Dzyuba B. (2017). Cryopreservation of carp (Cyprinus carpio L.) sperm: impact of seeding and freezing rates on post-thaw outputs. Biopreservation Biobanking 15 (3), 234–240. doi: 10.1089/bio.2016.0065
Cejko B. I., Kowalski R. K., Kucharczyk D., Ńarski D., Targońska K., Glogowski J. (2011). Effect of time after hormonal stimulation on semen quality indicators of common carp, Cyprinus carpio (Actinopterygii: Cypriniformes: Cyprinidae). Acta Ichthyologica Piscatoria 41 (2), 75–80. doi: 10.3750/aip2011.41.2.01
Dokina O., Pronina N., Kovalev K., Milenko V., Tsvetkova L. (2019). Improved technology of carp sperm cryopreservation in a large-scale cryobank. Fisheries 5 (5), 97–105.
Dzuba B. B., Kopeika E. F. (2002). Relationship between the changes in cellular volume of fish spermatozoa and their cryoresistance. Cryo Lett. 23 (6), 353–360.
Dzyuba B., Boryshpolets S., Rodina M., Gela D., Linhart O. (2010). Spontaneous activation of spermatozoa motility by routine freeze-thawing in different fish species. J. Appl. Ichthyology 26 (5), 720–725. doi: 10.1111/j.1439-0426.2010.01553.x
Dzyuba B., Cosson J., Yamaner G., Bondarenko O., Rodina M., Gela D., et al. (2013). Hypotonic treatment prior to freezing improves cryoresistance of common carp (Cyprinus carpio L.) spermatozoa. Cryobiology 66 (2), 192–194. doi: 10.1016/j.cryobiol.2012.12.003
Flajšhans M., Linhart O., Šlechtová V., Šlechta V. (1999). Genetic resources of commercially important fish species in the Czech Republic: present state and future strategy. Aquaculture 173 (1), 471–483. doi: 10.1016/S0044-8486(98)00477-3
Francis T., Archana Devi C., Selvamagheswaran M. (2013) Cryopreservation of carp spermatozoa Indian J. Sci. Technology. 6, 4524–4530. doi: 10.17485/ijst/2013/v6i5.15
Gallego V., Cavalcante S. S., Fujimoto R. Y., Carneiro P. C. F., Azevedo H. C., Maria A. N. (2017). Fish sperm subpopulations: Changes after cryopreservation process and relationship with fertilization success in tambaqui (Colossoma macropomum). Theriogenology 87, 16–24. doi: 10.1016/j.theriogenology.2016.08.001
Gorda S., Bakos J., Liska J., Kakuk C. (1995). Live gene bank of common carp strains at the fish-culture-research-institute, szarvas. Aquaculture 129 (1-4), 199–202. doi: 10.1016/0044-8486(94)00248-M
Henry M. A., Noiles E. E., Gao D., Mazur P., Critser J. K. (1993). Cryopreservation of human spermatozoa. IV. The effects of cooling rate and warming rate on the maintenance of motility, plasma membrane integrity, and mitochondrial function. Fertility Sterility 60 (5), 911–918. doi: 10.1016/S0015-0282(16)56296-7
Horton H. F., Ott A. G. (1976). Cryopreservation of fish spermatozoa and ova. J. Fisheries Res. Board Canada 33 (4), 995–1000. doi: 10.1139/f76-126
Horvath A., Miskolczi E., Mihalffy S., Osz K., Szabo K., Urbanyi B. (2007). Cryopreservation of common carp (Cyprinus carpio) sperm in 1.2 and 5 ml straws and occurrence of haploids among larvae produced with cryopreserved sperm. Cryobiology 54 (3), 251–257. doi: 10.1016/j.cryobiol.2007.02.003
Horvath A., Miskolczi E., Urbanyi B. (2003). Cryopreservation of common carp sperm. Aquat. Living Resour. 16 (5), 457–460. doi: 10.1016/s0990-7440(03)00084-6
Janssen K., Chavanne H., Berentsen P., Komen H. (2017). Impact of selective breeding on European aquaculture. Aquaculture 472 (1-4), 8–16. doi: 10.1016/j.aquaculture.2016.03.012
Kopeika E., Kopeika J., Zhang T. (2007). “Cryopreservation of Fish Sperm,” in Cryopreservation and Freeze-Drying Protocols. Eds. Day J. G., Stacey G. N. (Totowa, NJ, USA: Humana Press).
Lahnsteiner F., Berger B., Horvath A., Urbanyi B., Weismann T. (2000). Cryopreservation of spermatozoa in cyprinid fishes. Theriogenology 54 (9), 1477–1498. doi: 10.1016/S0093-691X(00)00469-6
Li P., Dzyuba B., Hulak M., Rodina M., Boryshpolets S., Li Z. H., et al. (2010). Percoll gradient separation of cryopreserved common carp spermatozoa to obtain a fraction with higher motility, velocity and membrane integrity. Theriogenology 74 (8), 1356–1361. doi: 10.1016/j.theriogenology.2010.06.005
Magyary I., Urbányi B., Horváth L. (1996). Cryopreservation of common carp (Cyprinus carpio L.) sperm II. Optimal conditions for fertilization. J. Appl. Ichthyology 12 (2), 117–119. doi: 10.1111/j.1439-0426.1996.tb00073.x
Marinović Z., Šćekić I., Lujić J., Urbányi B., Horváth Á. (2021). The effects of cryopreservation and cold storage on sperm subpopulation structure of common carp (Cyprinus carpio L.). Cryobiology 99, 88–94. doi: 10.1016/j.cryobiol.2021.01.007
Martínez-Páramo S., Horváth Á., Labbé C., Zhang T., Robles V., Herráez P., et al. (2017). Cryobanking of aquatic species. Aquaculture 472, 156–177. doi: 10.1016/j.aquaculture.2016.05.042
Morris J. G., Acton E., Murray B. J., Fonseca F. (2012). Freezing injury: the special case of the sperm cell. Cryobiology 64 (2), 71–80. doi: 10.1016/j.cryobiol.2011.12.002
Nusbaumer D., Marques da Cunha L., Wedekind C. (2019). Sperm cryopreservation reduces offspring growth. Proc. R. Soc. B: Biol. Sci. 286 (1911), 20191644. doi: 10.1098/rspb.2019.1644
Pataki B., Horváth Á., Mészáros G., Kitanović N., Ács A., Hegyi Á., et al. (2022). Adjustment of common carp sperm concentration prior to cryopreservation: Does it matter? Aquaculture Rep. 24, 101109. doi: 10.1016/j.aqrep.2022.101109
Purchase C. F., Earle P. T. (2012). Modifications to the IMAGEJ computer assisted sperm analysis plugin greatly improve efficiency and fundamentally alter the scope of attainable data. J. Appl. Ichthyology 28 (6), 1013–1016. doi: 10.1111/jai.12070
Sotnikov A., Rodina M., Stechkina T., Benevente C. F., Gela D., Boryshpolets S., et al. (2023). High sperm concentration during cryopreservation decreases post-thaw motility percentage without compromising in vitro fertilization outcomes in common carp. Aquaculture 562. doi: 10.1016/j.aquaculture.2022.738746
Tiersch T. R. (2008). Strategies for commercialization of cryopreserved fish semen. Rev. Bras. Zootecnia 37, 15–19. doi: 10.1590/s1516-35982008001300003
Urbányi B., Horváth Á. (2008). “Cryopreservation of common carp sperm,” in Methods in Reproductive Aquaculture: Marine and Freshwater Species. Eds. Cabrita E., Robles V., Herraez P. (Boca Raton: CRC Press, Taylor & Francis Group), 351–356.
Várkonyi L., Bokor Z., Ferincz Á., Staszny Á., Fodor F., Szári Z., et al. (2018). The applicability of 10 ml cryotubes for sperm cryopreservation in a Hungarian carp landrace (Cyprinus carpio carpio morpha accuminatus). Acta Agraria Debreceniensis 75), 93–97. doi: 10.34101/actaagrar/75/1653
Várkonyi L., Bokor Z., Molnár J., Fodor F., Szári Z., Ferincz Á., et al. (2019). The comparison of two different extenders for the improvement of large-scale sperm cryopreservation in common carp (Cyprinus carpio). Reprod. Domest. Anim. 54 (3), 639–645. doi: 10.1111/rda.13383
Wilson-Leedy J. G., Ingermann R. L. (2007). Development of a novel CASA system based on open source software for characterization of zebrafish sperm motility parameters. Theriogenology 67 (3), 661–672. doi: 10.1016/j.theriogenology.2006.10.003
Keywords: sperm motility, computer-assisted sperm analysis, cryopreservation, Cypriniformes, artificial reproduction
Citation: Sotnikov A, Rodina M, Gela D, Boryshpolets S, Kholodnyy V, Kašpar V, Linhart O and Dzyuba B (2024) Optimizing aquaculture-scale common carp artificial reproduction: a novel approach to sperm cryopreservation using large-volume containers and elevated thawing temperatures. Front. Mar. Sci. 11:1342483. doi: 10.3389/fmars.2024.1342483
Received: 23 November 2023; Accepted: 05 January 2024;
Published: 26 January 2024.
Edited by:
Yusuf Bozkurt, Iskenderun Technical University, TürkiyeReviewed by:
Andrzej Ciereszko, Polish Academy of Sciences, PolandFaruk Aral, Independent researcher, Konya, Türkiye
Copyright © 2024 Sotnikov, Rodina, Gela, Boryshpolets, Kholodnyy, Kašpar, Linhart and Dzyuba. This is an open-access article distributed under the terms of the Creative Commons Attribution License (CC BY). The use, distribution or reproduction in other forums is permitted, provided the original author(s) and the copyright owner(s) are credited and that the original publication in this journal is cited, in accordance with accepted academic practice. No use, distribution or reproduction is permitted which does not comply with these terms.
*Correspondence: Anatolii Sotnikov, asotnikov@frov.jcu.cz