- 1Bodega Marine Laboratory, University of California, Davis, Bodega Bay, CA, United States
- 2Coastal and Marine Science Institute, University of California, Davis, Davis, CA, United States
- 3Department of Animal Science, University of California, Davis, Davis, CA, United States
Changes in climate conditions can have cascading repercussions for organismal performance and survival, altering the productivity of aquatic systems. While numerous studies have examined the direct effects of temperature on species fitness, fewer studies have analyzed how individual impacts may translate to downstream primary consumers. Temperature-induced changes in the nutritional quality of seaweed may cause critical shifts in energy and nutrient availability for these consumers. A multifaceted experiment was conducted to assess the indirect impacts of California’s coastal climate conditions on abalone growth. This experiment fed juvenile red abalone (Haliotis rufescens) of two different size classes in ambient water conditions with the seaweed dulse (Devaleraea mollis), conditioned at 13°C, 15°C, and 17°C for 92 days. Nutritional analyses revealed significantly higher protein and nitrogen content in the 17°C dulse culture than in the 15°C and 13°C cultures, and no significant differences in carbohydrates. Furthermore, analyses on body weight ratio and condition factor indicate that juvenile red abalone of smaller size display significantly better growth and condition when fed dulse cultured at higher temperatures. Differences between size classes suggest that temperature changes, and subsequent shifts in the nutritional composition of dulse, may have greater impacts on smaller, younger red abalone.
Introduction
Changing ocean conditions, particularly ocean warming, have a significant impact on algal primary producers, which are vital to maintaining healthy ocean ecosystems and play a crucial role in the food web (Gao et al., 2012). Ocean warming events, mainly attributed to anthropogenic greenhouse gas concentrations, are becoming more frequent and extended in duration (Cheng et al., 2022). In the Northeastern Pacific Ocean, elevated ocean temperatures have been linked to the emergence of various marine diseases (Burge et al., 2014), species range shifts (Sanford et al., 2019; Geppi and Riera, 2022), and species mortality events (De Wit et al., 2014; Harvell et al., 2019).
Seaweed primary producers respond to rising ocean temperatures through a series of physiological adaptations that encompass shifts in enzymatic activity, photosynthesis rates, respiration, and nutrient uptake (Bui et al., 2018; Chen et al., 2018; Roleda and Hurd, 2019). In response to prolonged thermal stress, seaweeds employ a range of strategies, including increased production of reactive oxygen species, increased starch storage (Cian et al., 2015; Stiger-Pouvreau et al., 2016), and upregulation of heat shock proteins (Smolina et al., 2016). At the extremes, when thermal conditions in seaweeds surpass critical thresholds, reproductive failures become more prevalent, ultimately resulting in regional extinctions and range contractions (Bond et al., 2015; Cai et al., 2021).
Temperature-induced changes in primary producer populations can also disrupt consumer productivity (Brown et al., 2004; Nakamura and Oka, 2019) and apex predator populations at the highest trophic levels (Whitney, 2015; McPherson et al., 2021; Rogers-Bennett et al., 2021). Recognizing the potential indirect repercussions of climate change across trophic scales is crucial given that ecosystems consist of diverse interacting species. Ocean warming, among other factors, is affecting the performance of organisms directly and indirectly through the food web (Shurin et al., 2012). Additionally, altered ocean temperatures influence coastal habitats, affecting the recruitment of larval invertebrates and subsequently the community dynamics (Hawkins et al., 2008; Kavousi et al., 2022; Rogers-Bennett and Catton, 2022). Rising temperatures notably impact invertebrate larvae, resulting in observed declines in gastropod survival (Davis et al., 2013), reductions in bivalve larvae size and survival (Talmage and Gobler, 2011), and elevated mortality rates in echinoderm larvae and embryos (Ross et al., 2016).
Within these ecological interactions, abalone (Haliotis spp.) emerge as an important herbivorous grazer in diverse coastal ecosystems. Abalone exhibit varying temperature preferences, which differ both between species and throughout their developmental stages (Steinarsson and Imsland, 2003). Temperature plays a significant role in shaping the physiological processes of many marine invertebrates, such as these marine gastropods, leading to heightened metabolic rates as temperatures increase, ultimately reaching critical thermal maximums for growth, reproduction, and survival (Frederich and Pörtner, 2000; Rogers-Bennett et al., 2010). Abalone farming, which is practiced globally, heavily relies on coastal waters for culture systems, necessitating monitoring and management to address shifts in abalone metabolic rates due to ocean temperature changes (Somero, 2002).
Abalone, including the iconic red abalone (H. rufescens), play vital roles in coastal California ecosystems (Tenger and Dayton, 2000), and have been a significant marine aquaculture product for decades (Mundy et al., 2023). However, as ocean temperatures continue to rise, abalone are facing increased vulnerability to diseases like withering foot syndrome, caused by Candidatus Xenohaliotis californiensis (Moore et al., 2002). This debilitating syndrome leads to starvation, anorexia, weakness, and foot atrophy, resulting in alarmingly high mortality rates that have hastened the decline of abalone populations, both in the wild and captivity (Di et al., 2016; Crosson and Friedman, 2018; Frederick et al., 2022a). Furthermore, abalone species along the California coastline, now considered endangered or critically endangered, exhibit several detrimental effects as ocean temperatures continue to rise (Rogers-Bennett et al., 2010; Frederick et al., 2022b).
While a large body of literature examines the direct effects of temperature on individual species’ fitness, few studies have analyzed the subsequent consequences for downstream consumers. Specifically, a shift in the nutritional value of primary producers may initiate bottom-up changes across the food web. The relationship between seaweed and abalone represents a critical consumer-resource interaction within the marine food web. Therefore, we asked if climate-induced variation in seaweed nutritional composition can influence red abalone as a primary consumer. We utilized Devaleraea mollis [formerly called Palmaria mollis (Skriptsova and Kalita, 2020)], a red seaweed commonly consumed by abalone with established culture methods (Evans and Langdon, 2000), to examine the indirect effects of temperature on juvenile red abalone.
Materials and methods
Juvenile red abalone of two size classes (Figure 1A) were fed dulse (D. mollis) grown at three different seawater temperatures (13°C, 15°C, 17°C) from August-November 2022 at the University of California Davis Bodega Marine Laboratory (BML) in Bodega Bay, California, USA. Dulse and red abalone were independently cultured to enable individual temperature manipulation of dulse while maintaining the abalone at ambient ocean temperatures.
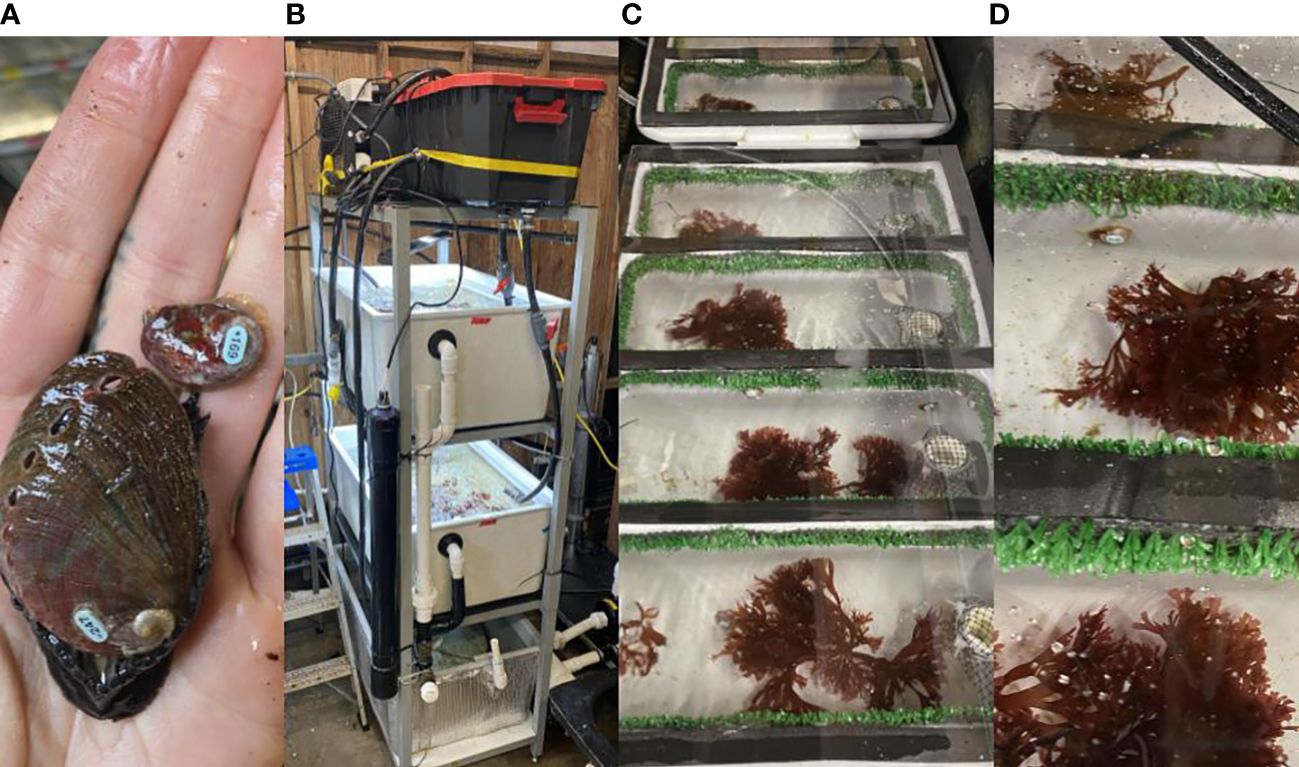
Figure 1 From left to right (A) displays the two juvenile red abalone (Haliotis rufescens) size classes, (B) displays the recirculating tank system for one temperature treatment of the dulse seaweed (Devaleraea mollis), (C) displays the replicated troughs and (D) portrays the dulse diet in the red abalone troughs.
Seaweed
Three sets of identical dulse cultures were composed of three vertically stacked (two culture tanks and one sump), insulated fiberglass tanks, each totaling 184 liters in volume. Cultures were supplied with sand-filtered raw seawater (~30 uM) sourced from the BML with 35% seawater replacement weekly. Total water volume for each independent culture system equated to a 560 L recirculating aquaculture system (Figure 1B). Culture system seawater was pumped (EcoPlus Eco 1056 Water Pump, 1000 GPH, Hawthorne, BC, CAN) and disinfected by passing the seawater through a low-pressure UV sterilizer (Pentair Smart UV 40 Watts, Minneapolis, MN, USA), and subsequently chilled using an in-line water chiller (Delta Star ¼ HP, Aqualogic, San Diego, CA, USA) prior to entering the culture tanks. Lighting was provided by two LEDs (Aqueon OptiBright LED Light, 850 Lumens, 21 µmol/s, Franklin, WI, USA) and operated by an electromechanical timer set for a 12:12 hour photoperiod. Culture tanks were configured with drip line aeration provided by a regenerative blower in T-formations (Dig Poly Drip Tubing 1/2” OD, Vista, CA, USA) to promote turbidity and fragmentation.
Experimental dulse cultures were maintained at 13°C, 15°C, and 17°C to simulate conditions reflective of California ocean warming. The 13°C culture served as a control, representing the approximate highest monthly average temperature in the Bodega Bay region during a typical year (NOAA, 2024). Temperature measurements were recorded at six-hour intervals for 92 days using a submerged temperature logger (HOBO Tidbits v2, Onset, Bourne, MA, USA) for a total of 368 measurements. Water quality parameters, including pH, ammonia, nitrites, and nitrates, were measured weekly with a commercial colorimetric kit (API Saltwater Master Test Kit, Los Angeles, CA, USA). Dulse culture tanks were scrubbed clean by brush to remove of epiphytic growth every 21 days during water changes.
Prior to use in the feeding trial, dulse batches underwent a cultivation period of at least 21 days for acclimation (top tank). Subsequently, the acclimated dulse was rotated to a secondary feed-out tank (middle tank) in the same temperature system, which provided tank space for a new batch of dulse. Dulse was fed to abalone ad libitum from the feed-out tank three times per week. Stocking density changed within batches due to dulse growth, and across batches to accommodate increases in abalone consumption (500-1200 g/184 L tank). Throughout the study, five batches of dulse were cultivated in each culture system.
Dulse tissue samples (3.5 g wet weight) were collected from the acclimation and feed-out tanks three times per week, dried in a 60°C oven, and stored in an airtight container for later analyses. Given the substantial volume of collected samples (~270 total samples) and financial logistics, analyses were performed on a subset of samples within each batch and temperature regime every seven days for 42 days, from acclimation through feed-out. These data further validated the nutritional results reported from a preliminary study (Mata, 2023). All nutrient analyses were conducted at the UC Davis Analytical Laboratory, Davis, CA. Protein was measured by thermal conductivity and infrared (IR) detection (Association of Official Analytical Collaboration (AOAC) Method 972.43). Total crude protein (AOAC Official Method 990.03) in dulse was calculated from the nitrogen content with the protein factor of 6.25. Carbohydrates were analyzed for free sugars glucose, fructose, and sucrose by high-performance liquid chromatography (HPLC) with mass selective detection. Total glucose was determined by enzymatic hydrolyzation at 55°C with an aminoglycoside for 12 hours and then analyzed by HPLC with mass selective detection. Total non-structural carbohydrates (TNC) were determined from the total glucose, fructose, and sucrose. Starch was calculated by subtracting the free glucose multiplied by 0.9 from the total glucose (Smith, 1969).
Abalone
Abalone culture troughs (Figure 1C) were fabricated using modified flow-through cutlery trays (Rubbermaid®, dimensions 21.5” x 11.5” x 3.75”), emulating conventional abalone farming methods. Each trough was filled with 3.7 L of seawater, delivering BML filtered and aerated seawater at an approximate rate of 0.5 L/min. Seawater entered the abalone troughs through a ⅛-inch diameter hose and manifold system and exited via a ½-inch PVC equipped with a 200 µm mesh. Each trough was lined at the water surface with AstroTurf® and sealed with clear acrylic lids to prevent abalone escapement. Ambient seawater temperatures averaged 10.4°C ± 3.2 during the study period (NOAA, 2024).
Juvenile red abalone were sourced from The Cultured Abalone Farm (Goleta, CA, USA). Two distinct size classes of juvenile abalone were utilized in this study, as smaller individuals exhibit proportionally greater growth rates (Tarbath et al., 2000; Proudfoot et al., 2008). The large abalone size class (43.4 ± 3.7 mm) were surplus from an earlier pilot study, and all 63 individuals had been maintained for over one year under ambient seawater conditions. The small abalone size class (15.8 ± 1.6 mm) included 300 individuals and encompassed the smallest recommended abalone for overnight shipment to BML. Upon arrival, the small abalone were slowly acclimated to ambient water temperatures for six hours before the initiation of the experiment. All abalone were tagged with Floy Tags (FTF-69 size ⅛”x ¼”, Seattle, WA), attached using CorAffix adhesive, and were allocated into experimental troughs using a random number generator. Maximum shell length and total weight data for the abalone were recorded on the initial and final day of the experimental period (92 days).
Diet treatments were allocated to troughs (Figure 1D) using a random number generator. For the small size abalone there were 8 replicates (n=8 troughs per treatment) with each trough containing 12 or 13 abalone. For the large size abalone, there were 7 replicates (n=7 troughs per treatment) with each trough containing 3 abalone (refer to Supplementary Figure 1). Abalone troughs were fed preweighed allotments of dulse according to the assigned temperature treatment, ad libitum, three times per week. Prior to each feeding, any remaining dulse was manually collected. To measure consumption, each trough was siphoned into a fine mesh netting (200 µm) in a 2 L container and remaining dulse was weighed after excess seawater was removed by centrifugation. To ensure ad libitum feeding, the quantity of dulse supplied per trough was progressively increased throughout the experiment to accommodate rising consumption rates.
Calculations
Consumption was quantified by weighing the residual dulse (to the nearest 0.01 g) in each trough after siphoning and centrifugation and then deducted from the initial feed quantity. Consumption was calculated using the following formula:
Here, consumption (C) was quantified using the initial feed quantity (F), the weight of the mesh and residual dulse together (Wf), and the weight of the mesh alone (Wi). Notably, dulse remained in troughs for approximately 48 hours before consumption measures and thus represents a rate between feeding dates.
Weights were recorded (to the nearest 0.01 g) using a digital scale after placing the abalone on a paper towel to remove excess water. Maximum shell lengths were measured according to established methods (Gareth et al., 2017; Hamilton et al., 2022), with calipers (to the nearest 0.1 mm). The following equation was used to calculate body weight ratio:
Here, the body weight ratio (BWR) was calculated using weight (W) and shell length (SL).
The condition factor is an index that signifies the correlation between the weight (g) of an abalone per unit of shell length (mm), offering insights into the relative condition of an abalone in comparison to others of similar size. The calculation of the condition factor was derived from Britz et al. (1996) using the following equation:
Here, the condition factor (CF) was calculated using weight (W), shell length (SL), and predetermined constants.
Statistical analysis
Data visualization and statistical analyses were conducted using R Studio (version 4.3.2). A linear mixed effects regression (LMER) was used for all statistical testing. The significance for all analyses were set to P<0.05. Data normality analysis (“performance” package; Lüdecke et al., 2021) was used to meet the assumptions of parametric statistical models. To investigate these effects, LMER models were tested for significance using an ANOVA with one fixed factor, and one random effect to determine the significance of treatment (“lme4” package; Bates et al., 2015). Body weight ratio and condition factor in the small size class were further analyzed along with protein and nitrogen levels in the dulse samples with pairwise comparisons to determine the differences between treatments (“emmeans” package; Lenth R., 2024). Sizes classes were treated as distinct populations due to differences in animal husbandry between the two groups before experimentation. Condition factor and body weight ratio models included trough as a random effect to control for variation despite the identical design. Initial measurements were also included as predictor variables in the final measurement’s models. Similarly, the nutritional analysis LMER examined the temperature effects while accounting for the variation across different sampling days and batches that were analyzed. Dulse consumption was analyzed using a LMER with trough and week as random variables. Differences in consumption between treatment groups were evaluated with a one-way ANOVA.
Results
Seaweed
All three temperatures of the dulse cultures were statistically different from each other throughout the experiment ANOVA (P<0.0001) with the average temperatures recorded as 13°C (12.7°C ± 0.43), 15°C (14.9°C ± 0.48), and 17°C (16.7°C ± 0.92). Water quality (pH, ammonia, nitrite, and nitrate) remained consistent throughout the experiment.
The dulse protein percentages were 15.6% ± 2.30, 16.1% ± 2.34, and 17.4% ± 2.77 for the 13°C, 15°C, and 17°C cultures respectively. There were significant differences in the one-way ANOVA of the LMER model (F2, 39.39 = 6.32, P<0.01). Protein levels in the 17°C were 1.8% higher than the 13°C culture (P<0.005, df=39.4, SE ± 0.50, Tukey’s HSD) and 1.3% higher than the 15°C culture (P<0.05, df=39.4, SE ± 0.5). Differences were not significant between the 13°C to 15°C cultures (P=0.68, df=39.4, SE ± 0.5). Average nitrogen levels were 2.51%, 2.57%, and 2.78% in the 13°C, 15°C, and 17°C cultures respectively. There were significant differences in the one-way ANOVA of the LMER model (F2, 39.39 = 6.31, P<0.01). Total nitrogen levels in the 17°C were 0.68% higher than the 13°C culture (P<0.005, df=39.4, SE ± 0.08, Tukey’s HSD) and 0.21% higher than the 15°C culture (P<0.05, df=39.4, SE ± 0.08). Differences were not significant between the 13°C to 15°C cultures (P=0.70, df=39.4, SE ± 0.08) (Figure 2).
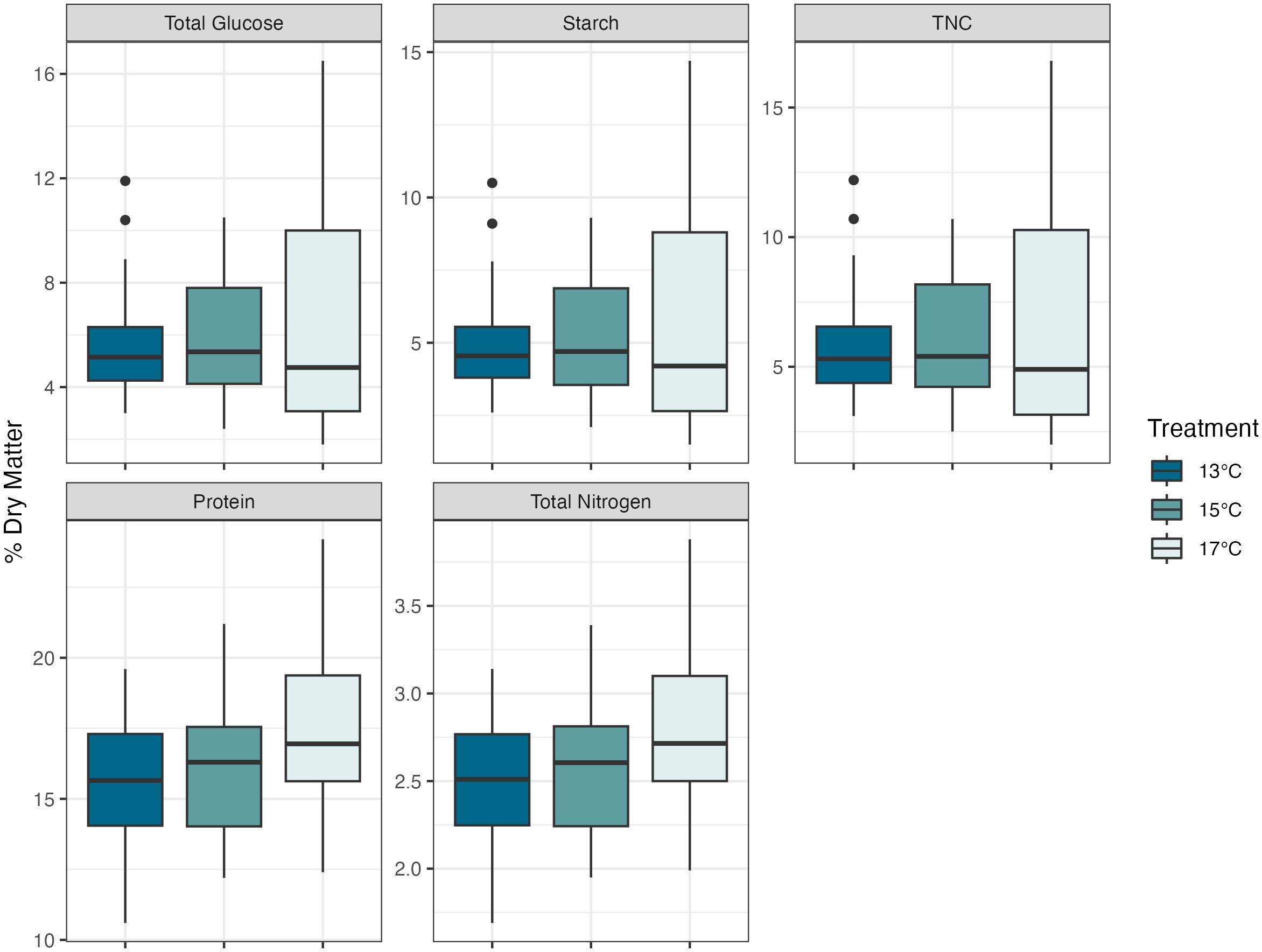
Figure 2 Nutritional composition of dulse (Devaleraea mollis) by temperature treatment. Protein and total nitrogen had significant differences, between the 13°C -17°C and 15°C -17°C. The boxes represent the interquartile range, with median lines and the whiskers extending represent the upper and lower quartile range with the closed circles representing the outliers.
Total glucose, TNC, and starch did not differ throughout treatment groups (P=0.52, P=0.56, and P=0.56, respectively). All three measurements were integrated into LMER models that accounted for variation across different sampling days and batches and analyzed with a one-way ANOVA. Many free glucose, fructose, and sucrose values returned from the nutritional analysis were below the detection level and could not be determined for these values.
Abalone
Over the experiment, there were no significant differences in consumption found in ANOVAs between the small or large abalone size classes and temperature treatment groups (P=0.57 and P=0.41, respectively) (Figure 3).
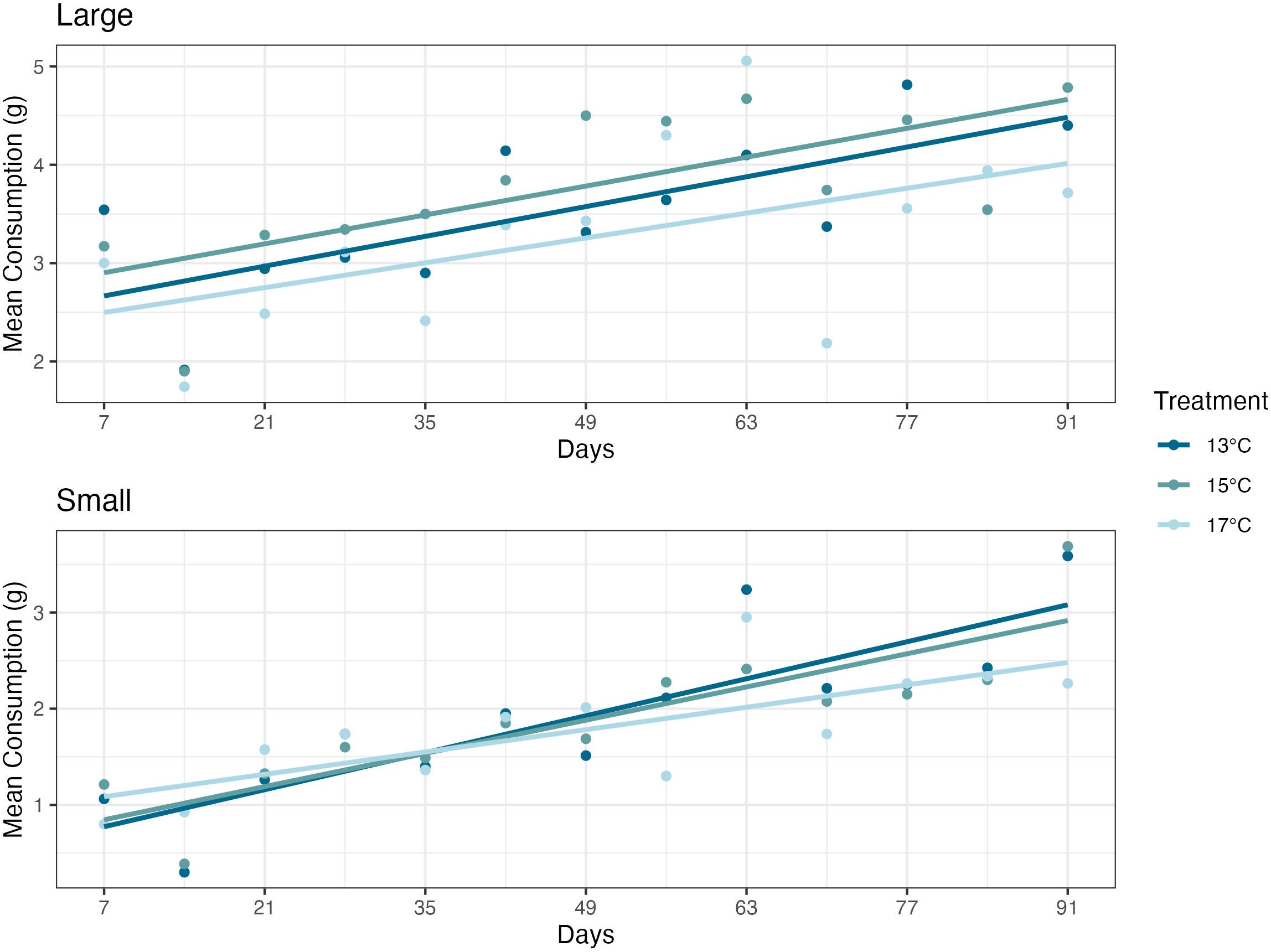
Figure 3 Mean feed consumption values, measured once weekly, for the duration of the experiment. Abalone (Haliotis rufescens) consumption had no significant differences between the 13°C, 15°C, or 17°C dulse (Devaleraea mollis) temperature treatment groups. Each circle represents the mean dulse consumption, over a 48-hour period, per treatment group in a given week.
Abalone survival was 99% from the acclimation period through the end of the experiment for both abalone size classes. During experimentation, the small abalone size class had one mortality in the 17°C treatment group. One trough underwent a plumbing failure, resulting in three large abalone mortalities from the 13°C treatment group. This replicate was removed from all analyses. The remaining large abalone size class exhibited 100% mean survival across all diets.
There were no significant differences in the ANOVA or the LMER on individual shell length change or weight change measurements between treatment groups in the small (P=0.07 and P=0.22 respectively) and large abalone (P=0.28 and P=0.55 respectively). There were no significant differences in CF in the small abalone size class at the onset of the experiment (P=0.86) (Figure 4). Significant differences in final CF were found in the small abalone size class via a one-way ANOVA of the LMER (F2, 20.95 = 5.33, P<0.05). A Tukey’s post hoc all pairwise comparisons test showed a significant difference in the 13°C cultured dulse diet had a lower CF when compared to those fed with 15°C and 17°C cultured dulse, with differences in CF between the 13°C - 15°C (P<0.05, df=20.9, SE ± 0.07) and 13°C - 17°C treatment groups (P<0.05, df=21, SE ± 0.07) (Figure 4). The initial or final CF calculations showed no significant differences between treatment groups in the large abalone size class (P=0.86 and P=0.22, respectively) (Figure 4).
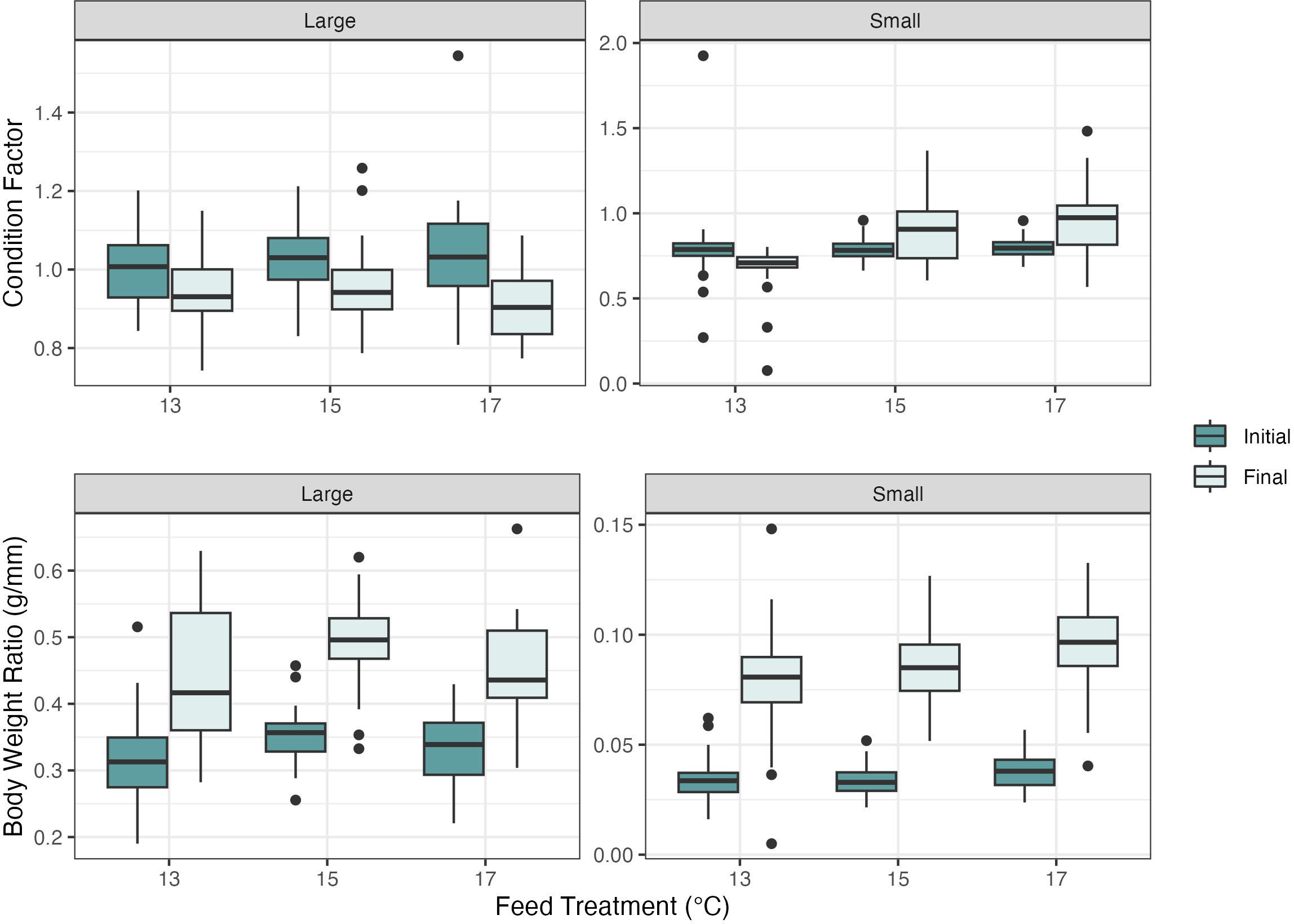
Figure 4 Red abalone (Haliotis rufescens) condition factor and body weight ratio measurements for the large and small size class with initial and final measurements. No significant differences were found for the large abalone size class. The small abalone size class had significant differences in the condition factor and body weight ratio between the 13°C -17°C and 15°C -17°C. The boxes represent the interquartile range, with median lines and the whiskers extending represent the upper and lower quartile range with the closed circles representing the outliers.
There were significant differences in the initial BWR between treatment groups using a one-way ANOVA of the LMER (F2, 19.38 = 4.81, P<0.05). As a result, the initial BWR measurements were used as predictor variables in the final BWR LMER model. A Tukey’s post hoc all pairwise comparisons test showed a significant difference in the initial body weight ratio between the abalone fed the 13°C -17°C dulse (P<0.05, df=19.1, SE ± 0.01) and the 15°C -17°C dulse (P<0.05, df=19.2, SE ± 0.01). There were no differences between 13°C -15°C (P=0.98). A one-way ANOVA of the final BWR LMER model revealed differences between small abalone treatment groups (F2, 19.76 = 7.05, P<0.005). A Tukey’s post hoc all pairwise comparisons test showed a significant difference in the body weight ratio between the abalone fed the 13°C -17°C dulse (P<0.005, df=19.9, SE ± 0.01) and the 13°C -15°C dulse (P<0.05, df=19.2, SE ± 0.01). There were no differences between 15°C -17°C (P=0.282) (Table 1). The initial and final BWR calculations showed no significant difference between treatment groups in the large abalone size class (P=0.30 and P=0.24, respectively) (Figure 2).
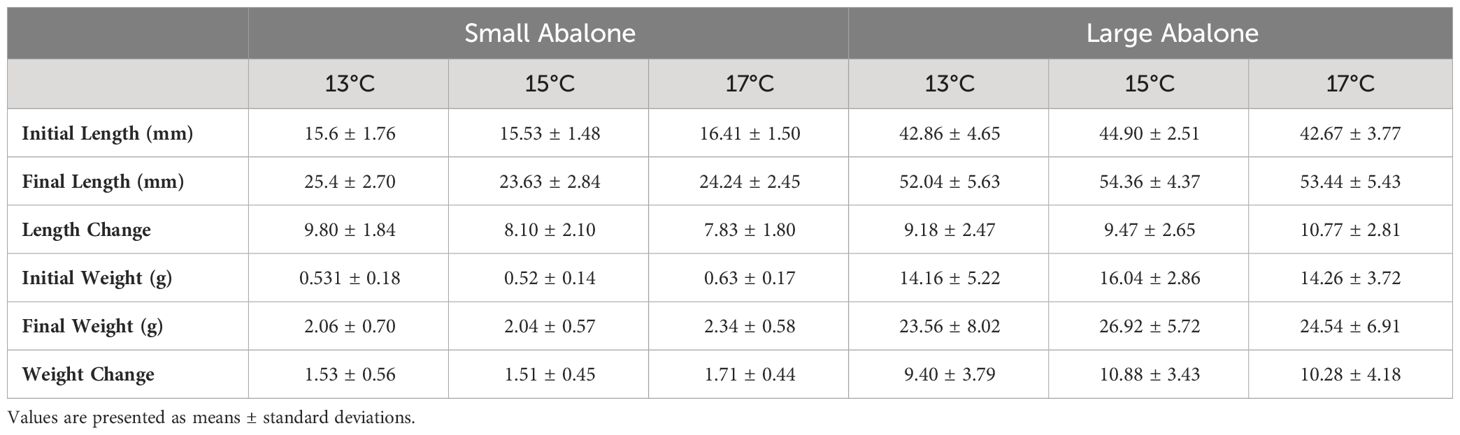
Table 1 Initial and final measurements for small and large cultured red abalone (Haliotis rufescens) size classes fed dulse (Devaleraea mollis) cultured at three temperatures.
Discussion
Direct effects of temperature on dulse nutrition
This study adds to the expanding body of literature investigating the influence of ocean warming, alongside other abiotic factors, on the nutritional quality of primary producers. Previous research has reported both negligible (Simonson et al., 2015) and altered biochemical compositions of seaweed when exposed to increased temperatures. Our findings align with studies demonstrating an elevation in protein (Mata, 2023) and nitrogen (Wilson et al., 2015) content under warming conditions. Nevertheless, a subset of studies presents conflicting outcomes, indicating a reduction in nitrogen or protein content in response to warming (Gao et al., 2018; Toth et al., 2020; Lowman et al., 2021). Considering that the structural components of carbohydrates are crucial for seaweed structural integrity and exhibit greater resilience to temperature variations (Costa et al., 2022; Tagliapietra et al., 2022), our carbohydrate findings align with anticipated trends. Seaweed consists of three distinct phyla (Chlorophyta, Rhodophyta, and Phaeophyceae) with deep phylogenetic splits (Raven and Hurd, 2012), potentially explaining the variation of responses documented in literature. Indeed, the effects of ocean warming on nutritional composition appear to exhibit species-specific patterns and may be influenced by the environment (e.g. nutrient abundance, light, acidity).
The temperatures selected for dulse cultivation were modeled off recent California ocean warming conditions. In 2014, record highs of 17.4°C were recorded off the California coast (Rogers-Bennett and Catton, 2019), thus informing the upper bound of our culture treatments. Given that dulse is an intertidal species, it naturally endures exposure to a multitude of temperature fluctuations and desiccation (Skriptsova et al., 2023). Nonetheless, prolonged or intensified thermal stress may compromise both the performance and nutritional quality of the dulse. In the current study, dulse cultivated at higher temperatures exhibited higher protein content, yet no discernible change in carbohydrates was observed. Typically, higher temperatures increase metabolic rates in seaweeds until a threshold is reached where normal functioning is disrupted (Wahl et al., 2020). The optimal thermotolerance range for cellular function in dulse is 12°C to 15°C (Stévant et al., 2023), indicating that our 17°C culture may have induced an up-regulation of protein synthesis (Zhang et al., 2022). Further analyses are needed to determine if the higher protein content in our dulse was due to faster metabolic processes or an increase in heat shock proteins (HSPs) resulting from thermal stress. A recent study in another red alga, Neopyropia yezoensis (Class Rhydophyta), revealed a specific NysHSP gene that supports cellular function when exposed to abiotic stress (Uji et al., 2018). Such findings are likely conserved throughout the Rhydophyta class and may act as a unique genetic marker that could be used to identify HSPs in future dulse studies.
Indirect effects of temperature on juvenile abalone growth
As part of a broader conservation effort to mitigate the impact of climate change on endangered abalone populations (National Marine Fisheries Service, 2008, 2020), we sought to investigate the direct and indirect effects of temperature on dulse and juvenile abalone physiology respectively. Our results contribute to a comprehensive exploration of the effects of ocean warming on abalone physiology, including adult reproductive health, with consequences for hatchery management and juvenile abalone recruitment (Boles et al., 2022, 2023). Concurrent efforts by Neylan et al. (2023), revealed slower growth, delays in fecundity, and decreased gonad tissue thickness on adult abalone when exposed to elevated ocean acidification conditions at 14°C. Since consumption was not measured in Neylan et al. (2023), it is conceivable that nutrition may have indirectly influenced measured endpoints, potentially through various mechanisms such as changes in feeding behavior or the impact of ocean acidification on digestive physiology. In this study, our primary goal was to assess how temperature-induced variations in seaweed nutrition affects the growth of juvenile abalone under ambient conditions. This provides insights into the dietary facet of their response to rising ocean temperatures, preceding future studies where seaweed and abalone cultures are linked.
Our findings suggest that changes in dulse nutritional composition may exert size-dependent effects on juvenile abalone. While the small abalone size class exhibited significant differences in both body weight ratio and condition factor between treatment groups, indicating that they benefited from the improved nutritional composition of dulse, no such benefits were observed in the large abalone size class. Digestive physiology and dietary requirements of juvenile abalone shift with age and between species (Bansemer et al., 2016; Frederick et al., 2022b), offering a potential explanation for the varied results observed among our abalone size classes. This variability may be attributed to the sigmoidal growth in red abalone, as larger abalone may grow at proportionally slower rates (Tarbath et al., 2000; Proudfoot et al., 2008). The consumption of dulse within each abalone size class was similar across temperature regimes indicating that the palatability of different dulse cultures did not influence subsequent growth results. Further analyses with an extended experimental duration would be necessary to definitively assert whether temperature can indirectly influence the growth of larger red abalone sizes. Considering that red abalone are the largest abalone species globally, these findings may also not necessarily apply to smaller abalone species. However, these results underscore the importance of considering abalone size when evaluating the potential effects of temperature on dulse nutritional composition.
An understanding of the effects of temperature-induced variations in seaweed dietary components on juvenile abalone growth may prove beneficial for hatcheries, as juvenile red abalone appears to derive indirect benefits from dulse cultured at higher temperatures. Protein-rich feed, resulting from warmer culture temperatures, was positively correlated with small abalone growth. This result is reflected in a suite of abalone diet studies (Britz and Hecht, 1997; Ma et al., 2020; Ma et al., 2022), which suggest that protein availability is crucial for optimal growth. However, it is unlikely that protein density alone drove the differences in abalone growth metrics observed between treatment groups. Unexamined micronutrients such as trace elements, vitamins, and minerals may be associated with increased protein content and could contribute to increased abalone growth (Demetropoulos and Langdon, 2004). While commercial aquaculture often relies on formulated diets with set parameters for micro- and macro-nutrients to maximize production (Ganguly et al., 2013; Willer and Aldridge, 2019; Jin et al., 2023), numerous diet studies have indicated that abalone preferentially consume seaweed over formulated feeds (Mulvaney et al., 2013; Bansemer et al., 2014). This knowledge has practical significance for conservation aquaculture and commercial abalone farms, as culturists can strategically adjust dulse culture temperatures to optimize juvenile growth by enhancing protein richness.
While the results of this controlled study suggest that temperature-induced changes in the nutritional composition of a primary producer can infer benefits to subsequent consumers, it is important to acknowledge that our experimental conditions do not replicate the environmental challenges that seaweed or wild abalone face seasonally or during broader climate fluctuations. Our results may help inform abalone conservation management and farming practices by offering a strategy to increase the protein content of seaweed diets through temperature manipulation and consequently enhancing juvenile abalone growth. When coupled with the direct effects of climate stressors, including temperature (Vilchis et al., 2005; Rogers-Bennett et al., 2010), ocean acidification (Swezey et al., 2020; Neylan et al., 2023), and deoxygenation (Nam et al., 2020), further research is necessary to confirm the implications of these findings for abalone conservation management.
Data availability statement
The raw data supporting the conclusions of this article will be made available by the authors, without undue reservation.
Ethics statement
The manuscript presents research on animals that do not require ethical approval for their study.
Author contributions
NRR: Data curation, Investigation, Software, Visualization, Writing – original draft, Writing – review & editing, Project administration. SBB: Investigation, Software, Writing – review & editing, Project administration. SEB: Project administration, Writing – review & editing, Funding acquisition, Supervision. JAG: Conceptualization, Writing – review & editing, Funding acquisition, Supervision.
Funding
The author(s) declare financial support was received for the research, authorship, and/or publication of this article. This research was funded by the United States Navy, Commander Pacific Fleet N62473-19-2-0023 and N62473-22-2-0007. Additionally, this work was supported by the UC-Davis Sustainable Oceans program, a United States National Science Foundation Research Traineeship (NSF DGE - 1734999).
Acknowledgments
We thank R. Patton and K. Weihrauch for their assistance in abalone husbandry and experimentations. We also thank the White Abalone Captive Breeding Program, specifically A. Frederick and N. Frank, for their assistance in Dulse culture as well as the BML Aquatic Resources Group for additional monitoring and life support systems. JAG utilized ChatGPT 3.5 for editorial purposes (https://chat.openai.com/).
Conflict of interest
The authors declare that the research was conducted in the absence of any commercial or financial relationships that could be construed as a potential conflict of interest.
Publisher’s note
All claims expressed in this article are solely those of the authors and do not necessarily represent those of their affiliated organizations, or those of the publisher, the editors and the reviewers. Any product that may be evaluated in this article, or claim that may be made by its manufacturer, is not guaranteed or endorsed by the publisher.
Supplementary material
The Supplementary Material for this article can be found online at: https://www.frontiersin.org/articles/10.3389/fmars.2024.1336793/full#supplementary-material
References
AOAC Official Method 990.03, Protein (Crude) in Animal Feed, Combustion Method, in Official Methods of Analysis of AOAC International, 18th edition Revision 1, 2006. Chapter 4, pp. 30–31, AOAC International, Gaithersburg, MD.
AOAC Official Method 972.43, Microchemical Determination of Carbon, Hydrogen, and Nitrogen, Automated Method, in Official Methods of Analysis of AOAC International, 18th edition, Revision 1, 2006. Chapter 12, pp. 5–6, AOAC International, Gaithersburg, MD.
Bansemer M. S., Qin J. G., Harris J. O., Howarth G. S., Stone D. A. J. (2014). Nutritional requirements and use of macroalgae as ingredients in abalone feed. Rev. Aquacult. 8, 121–135. doi: 10.1111/raq.12085
Bansemer M. S., Qin J. G., Harris J. O., Schaefer E. N., Wang H., Mercer G. J., et al. (2016). Age-dependent response of digestive enzyme activities to dietary protein level and water temperature in greenlip abalone (Haliotis laevigata). Aquaculture 451, 451–456. doi: 10.1016/j.aquaculture.2015.10.013
Bates D., Maechler M., Bolker B., Walker S. (2015). Fitting Linear Mixed-Effects Models using lme4. J. Stat. Softw. 67, 1–48. doi: 10.18637/jss.v067.i01
Boles S. E., Neylan I. P., Rogers-Bennett L., Gross J. A. (2022). Evaluation of gonad reproductive condition using non-invasive ultrasonography in red abalone (Haliotis rufescens). Front. Mar. Sci. 9. doi: 10.3389/fmars.2022.784481
Boles S. E., Rogers-Bennett L., Bragg W. K., Bredvik-Curran J., Graham S., Gross J. A. (2023). Determination of gonad reproductive state using non-lethal ultrasonography in endangered black (Haliotis cracherodii) and white abalone (H. sorenseni). Front. Mar. Sci. 10. doi: 10.3389/fmars.2023.1134844
Bond N. A., Cronin M. F., Freeland H., Mantua N. (2015). Causes and impacts of the 2014 warm anomaly in the NE Pacific. Geophys. Res. 42, 3414–3420. doi: 10.1002/2015GL063306
Britz P., Hecht T. (1997). Effect of dietary protein and energy level on growth and body composition of South African abalone, Haliotis midae. Aquaculture 156, 195–210. doi: 10.1016/S0044-8486(97)00090-2
Britz P. J., Hecht T., Mangold S. (1996). The suitability of selected protein sources for inclusion in formulated diets for the South African abalone, Haliotis midae. Aquaculture 140, 63–73. doi: 10.1016/0044-8486(95)01197-8
Brown J. H., Gillooly J. F., Allen A. P., Savage V. M., West G. B. (2004). Toward a metabolic theory of ecology. Ecology 85, 1771–1789. doi: 10.1890/03-9000
Bui H., Luu T. Q., Fotedar R. (2018). Effects of temperature and pH on the growth of Sargassum linearifolium and S. podacanthum in potassium-fortified inland saline water. Am. J. Appl. Sci. 15, 186–197. doi: 10.3844/ajassp.2018.186.197
Burge C. A., Mark Eakin C., Friedman C. S., Froelich B., Hershberger P. K., Hofmann E. E., et al. (2014). Climate change influences on marine infectious diseases: implications for management and society. Annu. Rev. Mar. Sci. 6, 249–277. doi: 10.1146/annurev-marine-010213-135029
Cai J., Lovatelli A., Aguilar-Manjarrez J., Cornish L., Dabbadie L., Desrochers A., et al. (2021). Seaweeds and microalgae: An overview for unlocking their potential in global aquaculture development. FAO Fish. Aquacult. Circ. 1229. (Rome: FAO). doi: 10.4060/cb5670en
Chen B., Zou D., Du H., Ji Z. (2018). Carbon and nitrogen accumulation in the economic seaweed Gracilaria lemaneiformis affected by ocean acidification and increasing temperature. Aquaculture 482, 176–182. doi: 10.1016/j.aquaculture.2017.09.042
Cheng S. H., Costedoat S., Sterling E. J., Chamberlain C. J., Jagadish A., Lichtenthal P. R., et al. (2022). What evidence exists on the links between natural climate solutions and climate change mitigation outcomes in subtropical and tropical terrestrial regions? A systematic map protocol. Environ. Evidence 11. doi: 10.1186/s13750-022-00268-w
Cian R. E., Drago S. R., De Medina F. S., Martínez-Augustin O. (2015). Proteins and carbohydrates from red seaweeds: evidence for beneficial effects on gut function and microbiota. Mar. Drugs 13, 8. doi: 10.3390/md13085358
Costa M., Pio L., Bule P., Cardoso V., Duarte M., Alfaia C. (2022). Recalcitrant cell wall of Ulva lactuca seaweed is degraded by a single ulvan lyase from family 25 of polysaccharide lyases. Anim. Nutr. 9, 184–192. doi: 10.1016/j.aninu.2022.01.004
Crosson L. M., Friedman C. S. (2018). Withering syndrome susceptibility of northeastern Pacific abalones: A complex relationship with phylogeny and thermal experience. J. Invertebr. Pathol. 151, 91–101. doi: 10.1016/j.jip.2017.11.005
Davis A. R., Coleman D., Broad A., Byrne M., Dworjanyn S. A., Przeslawski R. (2013). Complex responses of intertidal molluscan embryos to a warming and acidifying ocean in the presence of UV radiation. PloS One 8, E55939. doi: 10.1371/journal.pone.0055939
Demetropoulos C. L., Langdon C. J. (2004). Enhanced production of Pacific dulse (Palmaria mollis) for co-culture with abalone in a land-based system: Nitrogen, phosphorus, and trace metal nutrition. Aquaculture 235, 433–455. doi: 10.1016/j.aquaculture.2003.09.010
De Wit P., Rogers-Bennett L., Kudela R. M., Palumbi S. (2014). Forensic genomics as a novel tool for indentifying the causes of mass mortality events. Nat. Commun. 5, e3652. doi: 10.1038/ncomms4652
Di G., Kong X., Zhu G., Liu S., Zhang C., Ke C. (2016). Pathology and physiology of Haliotis diversicolor with withering syndrome. Aquaculture 453, 1–9. doi: 10.1016/j.aquaculture.2015.11.030
Evans F., Langdon C. J. (2000). Co-culture of dulse Palmaria mollis and red abalone Haliotis rufescens under limited flow conditions. Aquaculture 185, 137–158. doi: 10.1016/S0044-8486(99)00342-7
Frederich M., Pörtner H. O. (2000). Oxygen limitation of thermal tolerance defined by cardiac and ventilatory performance in spider crab, Maja squinado. Am. J. Physiol. Regul. Integr. Comp. Physiol. 279, R1531–R1538. doi: 10.1152/ajpregu.2000.279.5.R1531
Frederick A. R., Heras J., Friedman C. S., German D. P. (2022a). Withering syndrome induced gene expression changes and a de-novo transcriptome for the Pinto abalone, Haliotis kamtschatkana. Comp. Biochem. Physiol. Part D: Genomics Proteomics 41, 100930. doi: 10.1016/j.cbd.2021.100930
Frederick A. R., Wehrle B. A., Lee A. M., Catabay C. C., Rankins D. R., Clements K. D., et al. (2022b). The abalone digestive system may be able to keep pace with moderate heat stress. Comparative Biochemistry and Physiology - Part A. Adapt. Nutrient Suppl. Organs that Fuel Fire Life Special Issue. 270, 111230. doi: 10.1016/j.cbpa.2022.111230
Ganguly S., Dora K. C., Sarkar S., Chowdhury S. (2013). Supplementation of prebiotics in fish feed: a review. Rev. Fish Biol. Fish. 23, 195–199. doi: 10.1007/s11160-012-9291-5
Gao G., Clare A. S., Chatzidimitriou E., Rose C., Caldwell G. (2018). Effects of ocean warming and acidification, combined with nutrient enrichment, on chemical composition and functional properties of Ulva rigida. Food Chem. 258, 71–78. doi: 10.1016/j.foodchem.2018.03.040
Gao K., Helbling E., Häder D., Hutchins D. (2012). Responses of marine primary producers to interactions between ocean acidification, solar radiation, and warming. Mar. Ecol. Prog. Ser. 470, 167–189. doi: 10.3354/meps10043
Gareth F. D., Arnoldus C. V., Rhode C., Brink D. (2017). Heritability of growth traits in South African Abalone (Haliotis midae) using the “internal reference” method. Aquaculture 468, 451–457. doi: 10.1016/j.aquaculture.2016.10.042
Geppi E., Riera R. (2022). Responses of intertidal seaweeds to warming: A 38-year time series shows differences of sizes. Estuarine Coast. And Shelf Sci. 270, 107841. doi: 10.1016/j.ecss.2022.107841
Hamilton S. L., Elliott M., deVries M. S., Adelaars J., Rintoul M. D., Graham M. H. (2022). Integrated multi-trophic aquaculture mitigates the effects of ocean acidification: Seaweeds raise system pH and improve growth of juvenile abalone. Aquaculture 560, 738571–738571. doi: 10.1016/j.aquaculture.2022.738571
Harvell C. D., Montecino-Latorre D., Cadlwell J. M., Burt J. M., Keller A., Heron S. F., et al. (2019). Disease epidemic and a marine heat wave are associated with the continental-scale collapse of a pivotal predator (Pycnopodia helianthoides). Sci. Advance. 5, 7042. doi: 10.1126/sciadv.aau7042
Hawkins S., Moore P., Burrows M., Poloczanska E., Mieszkowska N., Herbert R., et al. (2008). Complex interactions in a rapidly changing world: Responses of rocky shore communities to recent climate change. Climate Res. 37, 123–133. doi: 10.3354/cr00768
Jin S., Kong Q., John C., Wang Z., Zhang T., Li X., et al. (2023). Natural biota’s contribution to cultured aquatic animals’ Growth in aquaculture cannot be ignored. Aquacult. Res. 2023, 2646607. doi: 10.1155/2023/2646607
Kavousi J., Roussel S., Martin S., Gaillard F., Badou A., Di Poi C., et al. (2022). Combined effects of ocean warming and acidification on the larval stages of the European abalone Haliotis tuberculata. Mar. pollut. Bull. 175, 113131. doi: 10.1016/j.marpolbul.2021.113131
Lenth R. V. (2024). Estimated Marginal Means, aka Least-Squares Means [R package emmeans version 1.10.0]. Available online at: https://cran.r-project.org/web/packages/emmeans/index.html.
Lowman H. E., Emery K. A., Dugan J. E., Miller R. J. (2021). Nutritional quality of giant kelp declines due to warming ocean temperatures. Oikos 2022. doi: 10.1111/oik.08619
Lüdecke D., Shachar M. S., Patil I., Waggoner P., Makowski D. (2021). performance: an R package for assessment, comparison and testing of statistical models. J. Open Source Softw. 6, 3139. doi: 10.21105/joss.03139
Ma S., Guo Y., Sun L., Fan W., Liu Y., Liu D., et al. (2020). Over high or low dietary protein levels depressed the growth, TOR signaling, apoptosis, immune, and anti-stress of abalone Haliotis discus hannai. Fish shell. Immunol. 106, 241–251. doi: 10.1016/j.fsi.2020.08.004
Ma Y., Zou W., Ai C., You W., Liu S., Luo X., et al. (2022). Evaluation of optimal dietary protein levels for juvenile hybrid abalone under three temperatures: growth performance, body composition, biochemical responses, and antioxidant capacity. Aquacult. Nutr. 2022, 7008746. doi: 10.1155/2022/7008746
Mata R. (2023). “Effects of Climate Change on the Growth and Chemical Composition of Primary Producers and its Impacts on Coastal Aquaculture.” ProQuest Dissertations Publishing.
McPherson M. L., Finger D. J. I., Houskeeper H. F., Bell T. W., Carr M. H., Rogers-Bennett L., et al. (2021). Large-scale shift in the structure of a kelp forest ecosystem co-occurs with an epizootic and marine heatwave. Commun. Biol. 4, 1–9.
Moore J., Finley C., Robbins T., Friedman C. (2002). Withering syndrome and restoration of southern California abalone populations. California Cooper. Ocean. Fish. Investig. Rep. 43.
Mulvaney W., Winberg P., Adams L. (2013). Comparison of macroalgal (Ulva and Grateloupia spp.) and formulated terrestrial feed on the growth and condition of juvenile abalone. J. Appl. Phycol. 25, 815–824. doi: 10.1007/s10811-013-9998-2
Mundy C., Haddon M., McAllister J. (2023). Assessment of commercial abalone fisheries. Developments in Aquaculture and Fisheries Science. 42, 291–330. doi: 10.1016/B978-0-12-814938-6.00009-9
Nakamura Y., Oka A. (2019). CMIP5 model analysis of future changes in ocean net primary production focusing on differences among individual oceans and models. J. Oceanogr. 75, 1–22. doi: 10.1007/s10872-019-00513-w
Nam S., Haque M., Lee J., Park H., Rhee J. (2020). Prolonged exposure to hypoxia inhibits the growth of Pacific abalone by modulating innate immunity and oxidative status. Aquat. Toxicol. 227, 105596. doi: 10.1016/j.aquatox.2020.105596
Neylan I. P., Swezey D. S., Boles S. E., Gross J. A., Sih A., Stachowicz J. J. (2023). Within- and transgenerational stress legacy effects of ocean acidification on red abalone (Haliotis rufescens) growth and survival. Global Change Biol. 30. doi: 10.1111/gcb.17048
NOAA. (2024). National Data Buoy Center. Available at: https://www.ndbc.noaa.gov/station_page.php?station=46013.
Proudfoot L., Kaehler S., McQuaid C. D. (2008). Using growth band autofluorescence to investigate large-scale variation in growth of the abalone Haliotis midae. Mar. Biol. 153, 789–796. doi: 10.1007/s00227-007-0848-0
Raven J. A., Hurd C. L. (2012). Ecophysiology of photosynthesis in macroalgae. Photosynthesis Res. 113, 105–125. doi: 10.1007/s11120-012-9768-z
Rogers-Bennett L., Catton C. (2019). Marine heat waves and multiple stressors tip bull kelp forest to sea urchin barrens. Sci. Rep. 9. doi: 10.1038/s41598-019-51114-y
Rogers-Bennett L., Catton C. (2022). Marine heat waves and multiple stressors tip bull kelp forest to sea urchin barrens. Sci. Rep. 9. doi: 10.1038/s41598-019-51114-y
Rogers-Bennett L., Dondanville R. F., Moore J. D., Vilchis L. I. (2010). Response of red abalone reproduction to warm water, starvation, and disease stressors: implications of ocean warming. J. Shellf. Res. 29, 599–611. doi: 10.2983/035.029.0308
Rogers-Bennett L., Klmat R., Catton C. A. (2021). Survivors of climate-driven abalone mass mortality exhibit declines in health and reproduction following kelp forest collapse. Front. Mar. Sci.: Global Change Future Oceans. 8, 725134.
Roleda M. Y., Hurd C. L. (2019). Seaweed nutrient physiology: Application of concepts to aquaculture and bioremediation. Phycologia 58, 552–562. doi: 10.1080/00318884.2019.1622920
Ross P., Parker L., Byrne M. (2016). Transgenerational responses of molluscs and echinoderms to changing ocean conditions. ICES J. Mar. Sci. 73, 537–549. doi: 10.1093/icesjms/fsv254
Sanford E., Sones J. L., García-Reyes M., Goddard J. H. R., Largier J. L. (2019). Widespread shifts in the coastal biota of northern California during the 2014–2016 marine heatwaves. Sci. Rep. 9, 4216. doi: 10.1038/s41598-019-40784-3
Shurin J. B., Clasen J. L., Greig H. S., Kratina P., Thompson P. L. (2012). Warming shifts top-down and bottom-up control of pond food web structure and function. Philos. Trans. R. Soc. B. 367, 3008–3017. doi: 10.1098/rstb.2012.0243
Simonson E., Metaxas A., Scheibling R. E. (2015). Kelp in hot water: II. Effects of warming seawater temperature on kelp quality as a food source and settlement substrate. Mar. Ecol. Prog. Ser. 537, 105–119. doi: 10.3354/meps11421
Skriptsova A. V., Kalita T. L. (2020). A re-evaluation of palmaria (Palmariaceae, rhodophyta) in the north-west pacific. Eur. J. Phycol. 55, 266–274. doi: 10.1080/09670262.2020.1714081
Skriptsova A. V., Shibneva S., Semenchenko A. A. (2023). Morphological and molecular investigations shed light on diversity and distribution of Palmariaceae in the Fsmith Northwestern Pacific. Eur. J. Phycol. 58, 438–456. doi: 10.1080/09670262.2023.2169767
Smith D. (1969). Removing and Analyzing Total Nonstructural Carbohydrates from Plant Tissue. College of Agriculture and Life Sciences. Wisconsin Agricultural Experiment Station Research. 41, 1–13.
Smolina I., Kollias S., Jueterbock A., Coyer J. A., Hoarau G. (2016). Variation in thermal stress response in two populations of the brown seaweed, Fucus distichus, from the Arctic and subarctic intertidal. Royale Soc. Open Sci. 3, 150429. doi: 10.1098/rsos.150429
Somero G. N. (2002). Thermal physiology and vertical zonation of intertidal animals: optima, limits, and costs of living. Integr. Comp. Biol. 42, 780–789. doi: 10.1093/icb/42.4.780
Steinarsson A., Imsland A. K. (2003). Size dependent variation in optimum growth temperature of red abalone (Haliotis rufescens). Aquaculture 224, 353–362. doi: 10.1016/S0044-8486(03)00241-2
Stévant P., Schmedes P., Le Gall L., Wegeberg S., Dumay J., Rebours C. (2023). Concise review of the red macroalga dulse. Palmaria palmata. J. Of Appl. Phycol. 35, 523–550. doi: 10.1007/s10811-022-02899-5
Stiger-Pouvreau V., Bourgougnon N., Deslandes E. (2016). Carbohydrates from seaweeds. In Seaweed Health Dis. Prev. (Elsevier), 223–274. doi: 10.1016/B978-0-12-802772-1.00008-7
Swezey D., Boles S., Aquilino K., Stott H., Bush D., Whitehead A., et al. (2020). Evolved differences in energy metabolism and growth dictate the impacts of ocean acidification on abalone aquaculture. Proc. Natl. Acad. Sci. United States America 117, 26513–26519. doi: 10.1073/pnas.2006910117
Tagliapietra B. L., Borges L. A., Ferreira N. L. B., Clerici M. T. P. S. (2022). Seaweed as a potential new source for starch, produced in the sea: A short review. Starch 75, 2200130. doi: 10.1002/star.202200130
Talmage S. C., Gobler C. J. (2011). Effects of elevated temperature and carbon dioxide on the growth and survival of larvae and juveniles of three species of northwest Atlantic bivalves. PloS One 6, e26941. doi: 10.1371/journal.pone.0026941
Tarbath D., Hodgson K., Karlov T., Haddon M. (2000). Tasmanian abalone fishery (Tasmanian Aquaculture and Fisheries Institute Technical Report i-103), Hobart, Australia.
Tenger M. J., Dayton P. K. (2000). Ecosystem effects of fishing in kelp forest communities. J. Mar. Sci. 57, 579–589. doi: 10.1006/jmsc.2000.0715
Toth G. B., Harrysson H., Wahlström N., Olsson J., Oerbekke A., Steinhagen S., et al. (2020). Effects of irradiance, temperature, nutrients, and pCO2 on the growth and biochemical composition of cultivated Ulva fenestrata. J. Appl. Phycol. 32, 3243–3254. doi: 10.1007/s10811-020-02155-8
Uji T., Gondaira Y., Fukuda S., Mizuta H., Saga N. (2018). Characterization and expression profiles of small heat shock proteins in the marine red alga Pyropia yezoensis. Cell Stress Chaperones 24, 223–233. doi: 10.1007/s12192-018-00959-9
United States, National Marine Fisheries Service, West Coast Region, Protected Resources Division (2008). Final White Abalone Recovery Plan (Haliotis sorenseni).
United States, National Marine Fisheries Service, West Coast Region, Protected Resources Division (2020). Final Endangered Species Act Recovery Plan for Black Abalone (Haliotis cracherodii).
Vilchis L. I., Tegner M. J., Moore J. D., Friedman C. S., Riser K. L., Robbins T. T., et al. (2005). Ocean warming effects on growth, reproduction, and survivorship of southern California abalone. Ecol. Appl. 15, 469–480. doi: 10.1890/03-5326
Wahl M., Werner F. J., Buchholz B., Raddatz S., Graiff A., Matthiessen B., et al. (2020). Season affects the strength and direction of the interactive impacts of ocean warming and biotic stress in a coastal seaweed ecosystem. Limnology Oceanogr. 65, 807–827. doi: 10.1002/lno.11350
Whitney F. A. (2015). Anomalous winter winds decrease 2014 transition zone productivity in the NE Pacific. Geophysical Res. Lett. 42, 428–431. doi: 10.1002/2014GL062634
Willer D. F., Aldridge D. (2019). Microencapsulated diets to improve growth and survivorship in juvenile European flat oysters (Ostrea edulis). Aquaculture. doi: 10.1016/j.aquaculture.2019.02.072
Wilson K. L., Kay L. M., Schmidt A. L., Lotze H. K. (2015). Effects of increasing water temperatures on survival and growth of ecologically and economically important seaweeds in Atlantic Canada: implications for climate change. Mar. Biol. 162, 2431–2444. doi: 10.1007/s00227-015-2769-7
Keywords: red abalone, seaweed, aquaculture, nutrition, ocean warming, climate change
Citation: Rizzo NR, Beckert SB, Boles SE and Gross JA (2024) Temperature-induced variations in dulse (Devaleraea mollis) nutrition provide indirect benefits on juvenile red abalone (Haliotis rufescens) Growth. Front. Mar. Sci. 11:1336793. doi: 10.3389/fmars.2024.1336793
Received: 11 November 2023; Accepted: 11 March 2024;
Published: 26 March 2024.
Edited by:
Sm Sharifuzzaman, University of Chittagong, BangladeshReviewed by:
Marc Baeta, University of Barcelona, SpainFabiola Lafarga, Center for Scientific Research and Higher Education in Ensenada (CICESE), Mexico
Copyright © 2024 Rizzo, Beckert, Boles and Gross. This is an open-access article distributed under the terms of the Creative Commons Attribution License (CC BY). The use, distribution or reproduction in other forums is permitted, provided the original author(s) and the copyright owner(s) are credited and that the original publication in this journal is cited, in accordance with accepted academic practice. No use, distribution or reproduction is permitted which does not comply with these terms.
*Correspondence: Jackson A. Gross, jagross@ucdavis.edu
†These authors have contributed equally to this work and share first authorship