- 1Distant Water Fisheries Resources Division, National Institute of Fisheries Science, Busan, Republic of Korea
- 2Large Pelagics Research Center, Gloucester, MA, United States
- 3Cetacean Research Institute, National Institute of Fisheries Science, Ulsan, Republic of Korea
- 4Coastal Water Fisheries Resources Division, National Institute of Fishers Science, Busan, Republic of Korea
Introduction: To better understand Patagonian toothfish (Dissostichus eleginoides) movement and habitat in the Southwest Atlantic, fifty popup satellite archival tags (PSATs) were deployed off Davis Bank on North Scotia Ridge between 2019 and 2020 on individuals ranging from 97-139 cm total length.
Methods: PSATs (18 Lotek Wireless PSATFLEX and 32 Wildlife Computers MiniPAT) were programmed to detach after completing 1 to 16-month missions recording pressure (depth) and water temperature.
Results: Six tags failed to report, and among the remaining 44 reporting tags, 34 reported on schedule, up to 487 days at sea – the longest electronic tag deployment for this species to date. Although the majority of PSATs reported within 50 km from the release sites, confirming high site fidelity, 12% of tags reported more than 200 km away, showing connectivity to Shag Rocks and South Georgia in the Southern Ocean. Toothfish moved across the Antarctic Polar Front through/to areas with no fishing activities, and hence, explained the absence of any previous conventional tag recapture. A 1-month transit to the Falkland/Malvinas Plateau Basin also revealed that toothfish can attain a surprisingly high movement rate of 33 km day-1.
Discussion: Fishery independent examples of toothfish presence and their movement capabilities are inviting us to broaden our examination on how toothfish move around their Scotia Arc habitats and link up different regional aggregation sites in the South Atlantic.
1 Introduction
Patagonian toothfish (Dissostichus eleginoides) is a notothenioid fish, and has a circum-sub-Antarctic distribution. Off the South American continent, they are found on the continental shelves and slopes along Chile and Argentina, around the sub-Antarctic islands of the Southern Ocean. D. eleginoides is long-lived, reaching over 33-54 years old (Horn, 2002), and can attain over 2.3 m total length (TL) and weight over 200 kg (Collins et al., 2010). Given its rich oil content and white flesh, toothfish are particularly sought after by chefs and seafood consumers worldwide, fetching high prices, and thus represent an important species for the commercial fisheries in the South Atlantic and around the Southern Ocean (CCAMLR Secretariat, 2016).
Patagonian toothfish is primarily targeted by bottom longliners using the trotline and Spanish set systems1, and can also be caught as a bycatch in the finfish and squid trawl fisheries north of the Commission for the Conservation of Antarctic Marine Living Resources (CCAMLR) Convention Area (Skeljo et al., 2022; Collins et al., 2010). This study explored the high-sea fishing grounds of the Korean bottom longline fleets in FAO Area 41 between 40°S and 55°S, which consist of a northern ground in Subarea 3.1 (north of 48°S) and a southern ground in Subarea 3.2 (Park et al., 2021). The fleets operate year-round over a latitudinally broad area, but a longitudinally narrow area outside of coastal states’ exclusive economic zones (EEZs) on the Patagonian Continental Shelf, and the North Scotia Ridge (Figure 1A). Among these fishing areas, Davis Bank is of particular interest as it has been consistently associated with high catch (Park et al., 2021) and sits at the southeastern extent of the fishing grounds (Figure 1A). It is situated about 160 km to the east of Burdwood Bank, another high catch location and a known toothfish spawning site (Laptikhovsky et al., 2006). These two banks are separated by a deep trough of > 3000 m bottom depth. Davis Bank features steep drop-offs on its southern edge. Together with Tierra del Fuego, Burdwood Bank, Aurora Bank, Shag Rocks, South Georgia, and South Sandwich Islands, Davis Bank make up the northern part of the Scotia Arc (Figure 1A, Dalziel et al., 2013). This island arc system separates the Southern Ocean from the Atlantic and Pacific Oceans, and interacts with the eastward-flowing Antarctic Circumpolar Current to form complex circulation patterns (Figure 1B), providing a platitude of productive habitats for many species to flourish (Dalziel et al., 2013). Apart from its toothfish availability, Davis Bank is located just to the east of the Antarctic Polar Front (Artana et al., 2016; Frey et al., 2021), which purportedly acts as a biogeographic barrier that structures toothfish populations (Ashford et al., 2008; Canales-Aguirre et al., 2018), and provides an isolation mechanism for potential speciation (Arkhipkin et al., 2022).
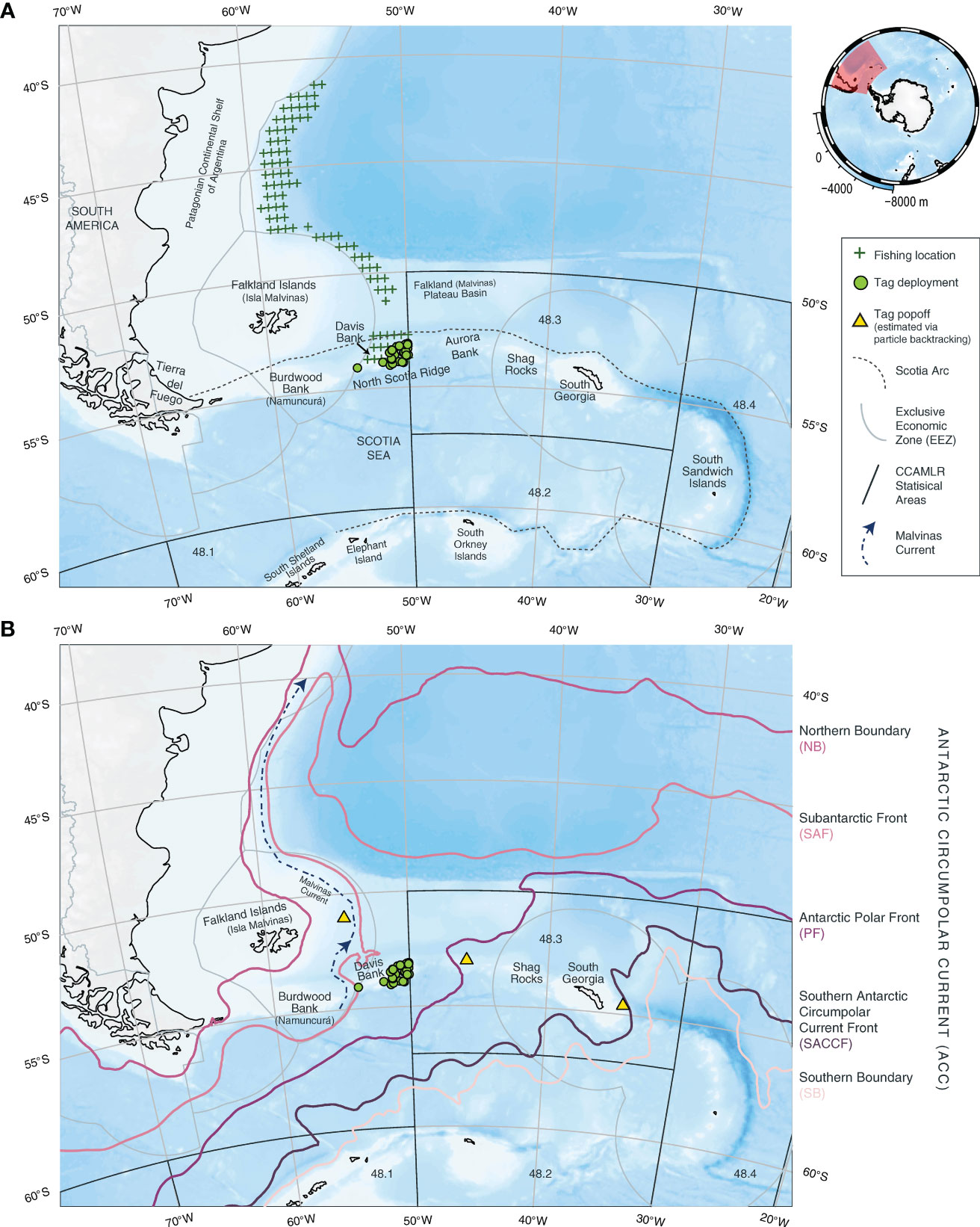
Figure 1 An overview of Patagonian toothfish popup satellite archival tag, or PSAT, deployments (green circles) on Davis Bank in relation to exclusive economic zones (EEZs, gray polygons) and Commission for the Conservation of Antarctic Marine Living Resources (CCAMLR) Convention Area (black polygons). (A) Physical and fisheries settings: Davis Bank is part of the group of islands and submarine ridges forming the Scotia Arc (dashed line). Gridded (0.5° x 0.5°) locations of the Korean longline fleet targeting toothfish (Park et al., 2021) are indicated by green crosses (+). Notice the relatively narrow longitudinal range of the fishing operations. (B) Antarctic Circumpolar Current (ACC) in the Scotia Sea: climatological mean locations of the ACC fronts are derived by satellite altimetry in Park et al. (2019) and made available in Park and Durand (2019). The fronts include the Northern Boundary (magenta line), Subantarctic Front (pink line), Antarctic Polar Front (purple line), Southern Antarctic Circumpolar Current Front (dark purple line) and Southern Boundary (light pink line). Location of the Malvinas Current (navy blue dotted line) is approximated from Frey et al. (2021). Estimated PSAT popoff positions from three toothfish that dispersed over 300 km from Davis Bank are shown with yellow triangles. Refer to the main text for the estimation methods. Spatial extents of the maps in (A, B) are represented by a red polygon in the mini-map (top right) centered on Antarctica. Bathymetry is obtained from the GEBCO 2014 gridded data set (version 20150318) through the R package, SOmap (australianantarcticdivision.github.io/SOmap).
Biology and ecology of toothfish have been studied extensively in the Southern Ocean by nation-state research authorities, often in support of or complement to CCAMLR research activities. For example, just off the Falkland Islands/Isla Malvinas (FLK) alone, north of the CCAMLR Convention Area, over 4400 conventional tags were deployed off the fishing grounds within its EEZ (Lee et al., 2022), and two electronic tagging studies had released fish north/northeast of the FLK, and on Burdwood Bank (Brown et al., 2013; Farrugia, 2018). Tagging, be it conventional or electronic, has shown most Patagonian toothfish tend to remain within a 50-km radius from their release sites (Brown et al., 2013; Farrugia, 2018; Lee et al., 2022; Troccoli et al., 2023). Recapture distances of > 200 km are observed in less than 10% of tagged fish, even though adults can undertake journeys in excess of 5000 km (CCAMLR Secretariat, 2017; Grilly et al., 2022). Earlier studies have therefore concluded that Patagonian toothfish exhibit year-round site fidelity (Collins et al., 2010; Lee et al., 2022) and extended periods of limited displacement (up to 8 years, Troccoli et al., 2023), implicating specific life history choices adopted by Patagonian toothfish, such as the maximization of energy savings through reduced migratory dispersals (Collins et al., 2010; Lee et al., 2022). Restricted movements appear to contradict results obtained from the recent applications of whole genome sequencing, which show some levels of regional connectivity and genetic homogeneity (Canales-Aguirre et al., 2018; Touma et al., 2019). The mechanisms behind population connectivity, other than the dispersal that occurred during a prolonged, pelagic drifting phase in egg and larval stages (Ashford et al., 2008), remain elusive. Ontogenetic change in toothfish bathymetric range is well established (Ashford et al., 2007; Collins et al., 2010; Lee et al., 2021). Juveniles first become benthopelagic and migrate into deeper waters as they grow, and as adults, toothfish occupy depths mostly below 1000 m. It is unclear if ontogenesis would also apply to horizontal movement, or even more broadly, habitat utilization that varies over space (location and/depth) and time. The large geographic scope of Patagonian toothfish around the Southern Ocean, and that differences in size composition of juvenile and adults in different areas (e.g., Ashford et al., 2007) suggest ontogenetic migration could be plausible.
To address this knowledge gap, we therefore utilized newer-generation popup satellite archival tag (PSAT), equipped with better data acquisition and reporting technologies, to capture fishery-independent movement and behavior of Patagonian toothfish. PSAT tagging is preferred given its potential to study toothfish in areas or time of year with no/low fishing effort or closed to fishing activities (Collins et al., 2021). We prioritized the tagging of longline-caught toothfish over 100 cm TL, as the mean sizes at capture on Davis Bank were 97 cm and 95 cm TL for female and male, respectively (Kim, E. J., unpublished data). A larger body size makes these fish better suited to bear the energetic costs of towing a PSAT, thereby increasing the chance of post-release survival and improving data recovery. Moreover, ≥ 90% of both sexes reach sexual maturity by 100 cm TL (Laptikhovsky et al., 2006). Mature adult toothfish might migrate in search of potential spawning sites, and thus, contribute to population mixing.
2 Materials and methods
2.1 Satellite tags
Wildlife Computers MiniPAT (n = 32) and Lotek Wireless PSATFLEX (n = 18) were rigged via an attachment assembly consisted of a 2-cm (length) “umbrella” plastic dart anchor2,3, stainless steel crimps and an 11.4-cm (4.5-inch) tether made with 110-lb test monofilament (Galuardi and Lutcavage, 2012). Metal anchors and attachment wires were avoided to minimize potential damage to the tagged animals.
Per manufacturer specifications, Wildlife Computers MiniPAT has a pressure rating of 2000 m, and records depth to 1700 m (± 0.5 m resolution), temperature from -40 to 60°C (± 0.05°C resolution), and light from 5 x 10-12 to 5 x 10-2 W cm-2 at 440 nm wavelength. MiniPAT has a total length and width of 118 x 38 mm, with a weight of 61 g. MiniPAT sensors sample at various frequencies, however measurements are only written into a tag’s memory archive every 3 seconds to 1 minute, depending on the user-programmed duration of a mission. Archival records can be downloaded from the memory after physically recovering a tag. Lotek Wireless PSATFLEX also has a pressure rating of 2000 m, and records depth to 2000 m (± 1 m resolution), temperature from -10 to 45°C (± 0.05°C resolution), and light levels for geolocation (sensitivity is not specified in manufacturer references). The tag’s total length and width measures 131 x 42 mm, with a weight of 87 g. Upon physical tag recovery, 1-minute archival measurements can be retrieved.
Given previous successful deployments of PSATs on toothfish species in the literature (e.g., Brown et al., 2013), the prospect of operating in a pressure environment that slightly exceeds stated specifications was not a practical concern. Technical discussions with manufacturer engineers also supported this assessment.
2.2 Experimental design
The propensity of adult toothfish staying at depths below 800 m means no or little ambient light is available for the use of light-based geolocation to position PSATs. We therefore utilized a PSAT to provide a fishery-independent location when the tag ended its mission and detached. To avoid tagged specimens from being prematurely recaptured by the Korean fleet, tag deployments were scheduled towards the end of their fishing activities on Davis Bank (i.e., January). This time of the year also provided more stable weather conditions that were conducive to fishing and tag deployments. PSATs were configured to detach from tagged animals after mission durations of 1 month (n = 7), 2 months (n = 6), 3 months (n = 10), 5 months (n = 3), 6 months (n = 4), 9 months (n = 4), 12 months (n = 13) and 16 months (n = 3). Missions of up to 9 months were scheduled to provide various observation periods after the Korean fleet had left the southern fishing ground. These durations also enveloped the known spawning period on Burdwood Bank between April and September (Laptikhovsky et al., 2006). A full-year or 16-month mission would enable the capturing of any seasonal or inter-annual movement pattern. Almost half of the tags were set for 1 to 3-month missions to observe potential short-term movement, e.g., the departure from Davis Bank after foraging there. These short deployments could also provide practical feedback to help refine tag configurations and field operations. Upon detachment, PSAT was configured to initiate data transmission sequence as soon as it reached the sea surface. This minimized the amount of time that a tag was drifting with surface currents before it was able to establish a link with an orbiting Argos satellite.
Given various mission durations used, PSATs were programmed to transmit to satellites sensor data at a common resolution to facilitate data analysis: time series at 5-minute interval and daily summaries. Depth data were always transmitted, but temperature data were on a duty cycle. This tradeoff was made because below the thermocline, temperature fluctuate less. Summarized data products (e.g., time-at-depth histograms) were transmitted for selected tags while balancing their mission durations and the number of satellite messages required. Detailed settings for each tag are out of scope for characterizing toothfish movement here, but we invite any interested readers to reach out so that we can share and discuss further our programming logic.
2.3 Field operations
PSATs were deployed on toothfish off Davis Bank by scientific observers on board of the Korean commercial longliner, F/V No. 8 Meridian in 2019 and 2020 (Table 1). Safe fish handling and conventional tagging protocols detailed in the CCAMLR tagging handbook (CCAMLR Secretariat, 2014) were followed. During haul back, toothfish (~ 100 cm TL) assessed in excellent condition (e.g., lively, injury free and without scars) were carefully brought onboard with a conical net and unhooked (Figure 2A). Total length of a specimen was measured to the nearest 0.1 cm. A designated, trained observer carefully, aiming for the base of the pterygiophores, inserted a PSAT and two conventional T-bar tags (Supplementary Table 1) around the region between the first and second dorsal fin (Figure 2B). With all tags placed and secured, the tagged fish was kept in a stainless steel holding tank for about five minutes (Figure 2C), during which the observer checked if the PSAT was being towed properly (e.g., positively buoyant and floating away from the body) and monitored the specimen’s condition (Supplementary Video 1). Video and still recording of the fish’s swimming and behavior were also obtained. At the end of the recovery period, the fish was delicately and promptly released head first into the water (Figure 2D).
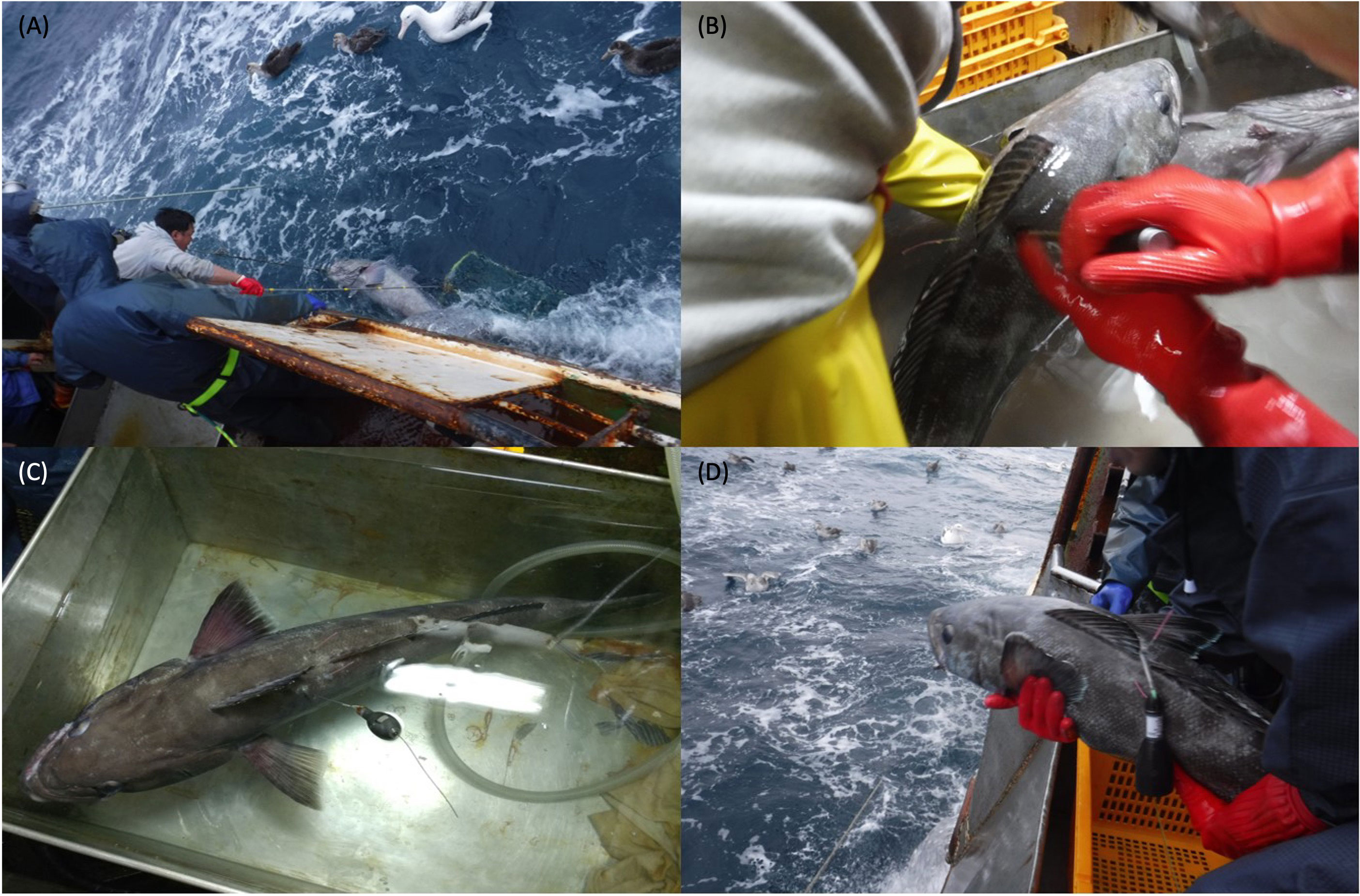
Figure 2 Tagging of Patagonian toothfish. (A) A healthy toothfish was carried on the boat carefully using a conical net; (B) fish was tagged with one PSAT and two T-bar tags; (C) tagged fish was held inside a recovery tank, and (D) tagged fish delicately released head first into the water.
2.4 Data recovery
Once a PSAT reached the end of its programmed mission, an electric current was passed to the release pin to trigger the popoff process. For MiniPAT, the current is passed at 20:00 UTC after the mission is completed. The metal release pin usually corrodes (referred to as “pin burn”) and dissolves within one day, allowing the positively buoyant tag to separate from the attachment assembly and begin its ascent. Once at the sea surface, a PSAT attempted to communicate with any of the orbiting Argos satellites. Upon establishing a successful satellite link, a first reporting location was registered on the Argos system, and the tag proceeded to transmit its data. Received messages were downloaded through the web daily. Consolidated messages were parsed and decoded by manufacturer’s software into human-readable output files. Each dataset was then visually inspected to determine a tag’s fate, such as premature shedding or the occurrence of a post-release mortality event that is deducible from abnormally high daily maximum temperatures, changes in light levels or other anomalies in the sensor data.
2.5 Data analysis
Dispersal distance was calculated using the fish release and first Argos satellite reporting positions via the R-package geosphere (github.com/rspatial/geosphere). This minimum distance calculation does not account for any drift that could have occurred while a floating PSAT was attempting a satellite fix. In the absence of any geolocation capabilities, horizontal movement can only be estimated. This is done using a least-cost path calculation function “lc.path” in the R-package marmap (github.com/ericpante/marmap) using the release and reporting positions as inputs. The estimation is based on the original work by van Etten and Hijmans (2010) with bathymetric constraints placed between 450 and 2000-m habitats, following the same methods described in Lee et al. (2022).
For PSATs that reported > 300 km away from their initial release site, we corrected for the distance that a tag had drifted prior to its first successful satellite link (Table 2). Particle backtracking was conducted with OpenDrift (opendrift.github.io; Dagestad et al., 2018) and forced by the HYCOM NCODA GOFS 1/12° Analysis (GLBy0.08) (hycom.org/data/glby0pt08). OpenDrift’s standard ocean drift model (current + 2% wind drift) was applied, releasing 10000 particles around a 1500-m radius from the first reporting location. This radius was selected to buffer around the accuracies (location class 1 to 3) of the Argos system (Irvine et al., 2020). Drift duration varied by individual tag, ranging from hours to days (Table 2). For computational efficiency, we set backtracking time step to every 30 minutes for drift duration < 33 hr, and to daily for drift duration > 33 hr. The centroid of the final positions of the released particles was taken as the estimated tag popoff position. Least-cost path was recalculated using the release and estimated popoff positions.
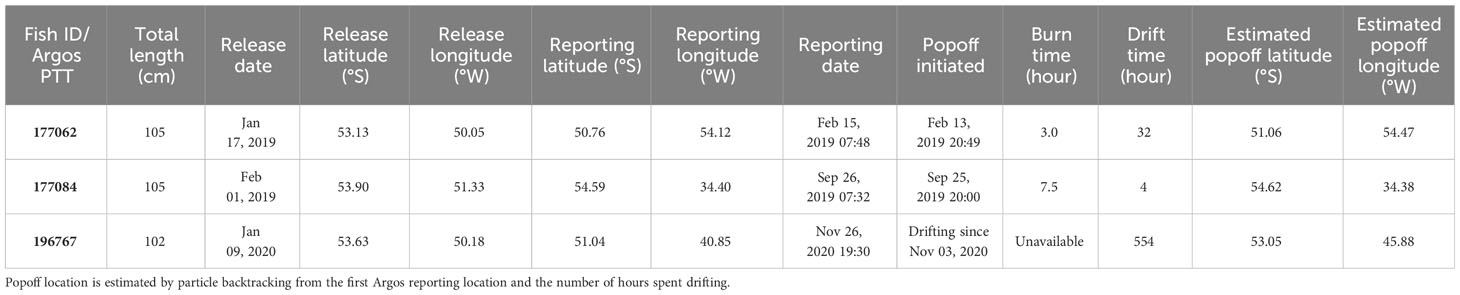
Table 2 Satellite tag deployment and reporting metadata for selected Patagonian toothfish that dispersed a minimum distance of > 300 km.
3 Results
Tagged toothfish ranged 97 to 139 cm TL, with a mean of 114 ± 12 cm (Table 1). Even though the sex of specimens were not identified, their sizes suggested that they were adults (Laptikhovsky et al., 2006).
3.1 PSAT performance
Among the PSATs deployed, 34 (68%) completed their designated mission and reported on time, 10 detached prematurely and reported early (20%), and 6 failed to report (12%). MiniPAT and PSATFLEX each contributed three cases of non-reporting. The majority (39%) of reporting tags were deployed on the 100-110 cm individuals. We found no significant relationships among any combinations of tag performance with fish length, tag model or release year/month. PSATs stayed attached for 10-487 days, with a mean duration of 168 days. This summary statistic does not account for the different programmed mission durations. Irrespective of experimental design, this study’s overall reporting rate of 88% is higher than those seen in Brown et al. (2013); (73%) and in Farrugia (2018); (73%).
3.2 Survivorship
Among the 44 reporting PSATs, archived sensor data showed no evidence of tag ingestion, predatory interaction or post-release mortality i.e., tags remained attached to live fish until popoff. Even for the two shortest deployments (< 10 d), both specimens returned to depths below 1600 m and made numerous small depth changes (< 60 m) for over a week before their tag anchors got loose and the PSATs worked their way out of the fish. Scientific observer also noted that fish 177069 (103 cm TL) was appeared to be poor condition in the holding tank before release, but this individual survived and was monitored for 102 days until the scheduled tag popoff.
Three physical recoveries also confirmed the longer-term survival of tagged toothfish. Fish 177081 was recaptured with its MiniPAT still attached, on a Chilean longline on July 31, 2019 at 53.26°S 50.34°W. Fish 177071, only carrying T-bar tags at the time of recapture, was caught by F/V Kingstar at 53.36°S 50.36°W on 10th October 2021, 880 days after its PSAT popped off. Fish 196770, also carrying only T-bar tags, was recaptured by F/V Kingstar at 53.42°S 50.96°W on 19th October 2022, 278 days after its PSAT had popped off.
3.3 Horizontal movement
Satellite data retrieval provided 42 pairs of reliable release and reporting locations for minimum distance calculation (Table 1). A total of 29 fish (69%) were found within 50 km of the release locations when their tags reported. Eight fish (19%) were found within 200 km of the release locations, and five fish (12%) dispersed over 200 km. The longest dispersal was recorded on a 97-cm specimen (fish 196768), with its tag popped off after 201 days at liberty on a known toothfish fishing ground in the Southeast Atlantic (Kim and Kim, 2008). Archived sensor data did not support the scenario that the tag/fish was being transported by a fishing vessel at any given time. Given our current focus on fish movement around the Scotia Arc, horizontal and vertical data for fish 196768 will be presented in details by a later study.
Least cost paths showed an extensive use of Davis Bank by all sizes of toothfish (Figures 3–6), with tagged specimens concentrated around < 1000-m bottom depth. Fish appeared to aggregate on the eastern and southern side of Davis Bank, proximal to the deep trough in which the Antarctic Polar Front flows through the relatively narrow (~ 180 km wide) Shag Rocks Passage, forming eddies and meanders that sit on Davis Bank (Artana et al., 2016). Toothfish that carried a tag for at least 12 months were found within 15 km from where they were first released on Davis Bank, implicating strong site fidelity for and/extended residency at this release location. The largest specimen, a 139-cm TL fish (177081) was recaptured about 264 km northeast from its initial release site on western Davis Bank, after 193 days at liberty (Figure 6).
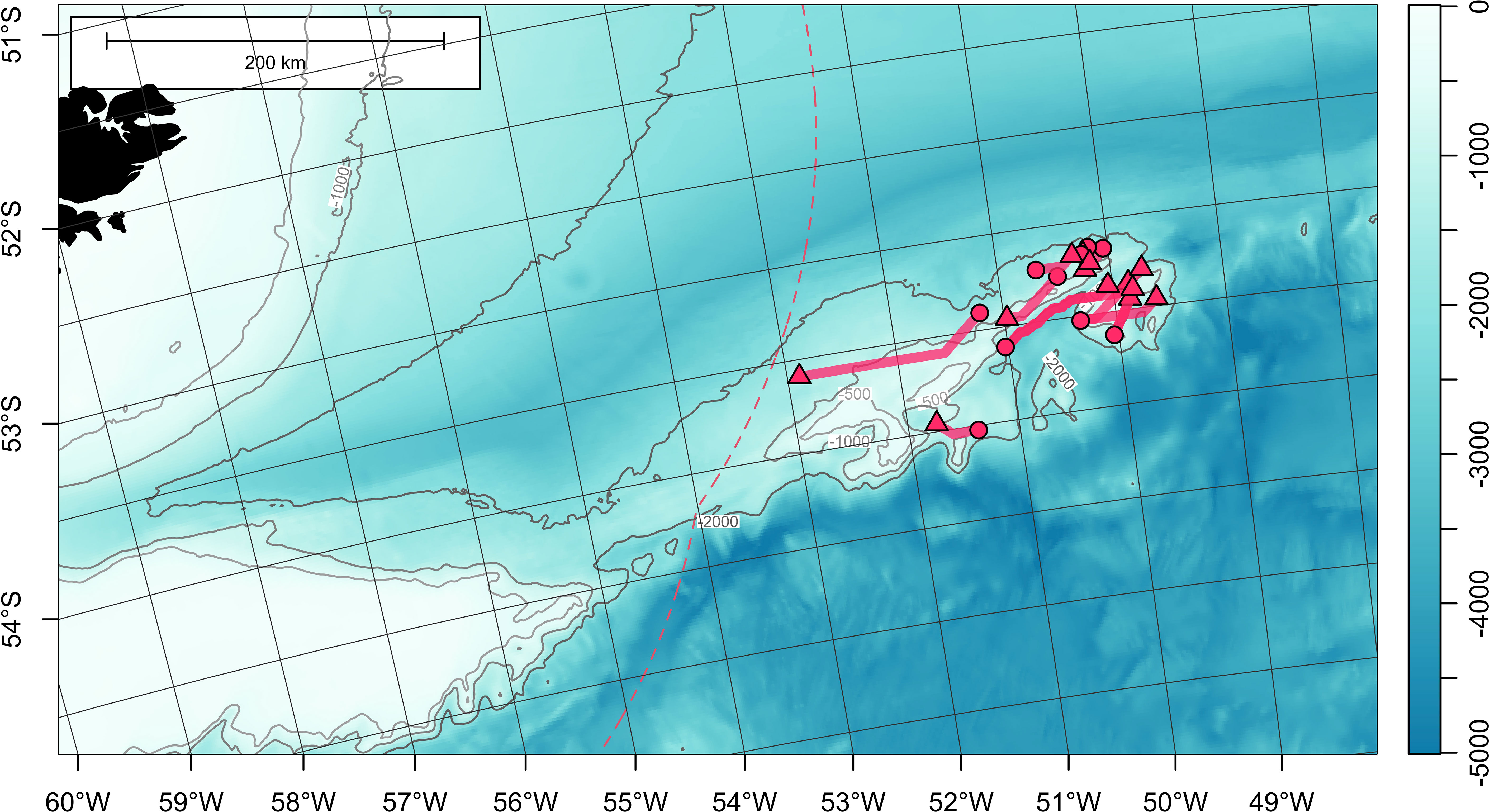
Figure 3 Least-cost paths for 13 satellite-tagged Patagonian toothfish measuring 100-109 cm total length, around Davis Bank, on the North Scotia Ridge. Tag deployment and popoff locations are indicated with circles and triangles, respectively. Bathymetry (in meters) is obtained from ETOPO 2022 (15-arcseconds) product through NOAA National Centers for Environmental Information (ncei.noaa.gov/maps/grid-extract). Bathymetry is plotted as a false-color overlay, and also contoured at 500, 1000 and 2000 m as gray lines. Color scale of the bathymetry differs from Figure 1 in order to enhance the visibility of topographic features. Exclusive economic zones (EEZs) are outlined with red dashed lines.
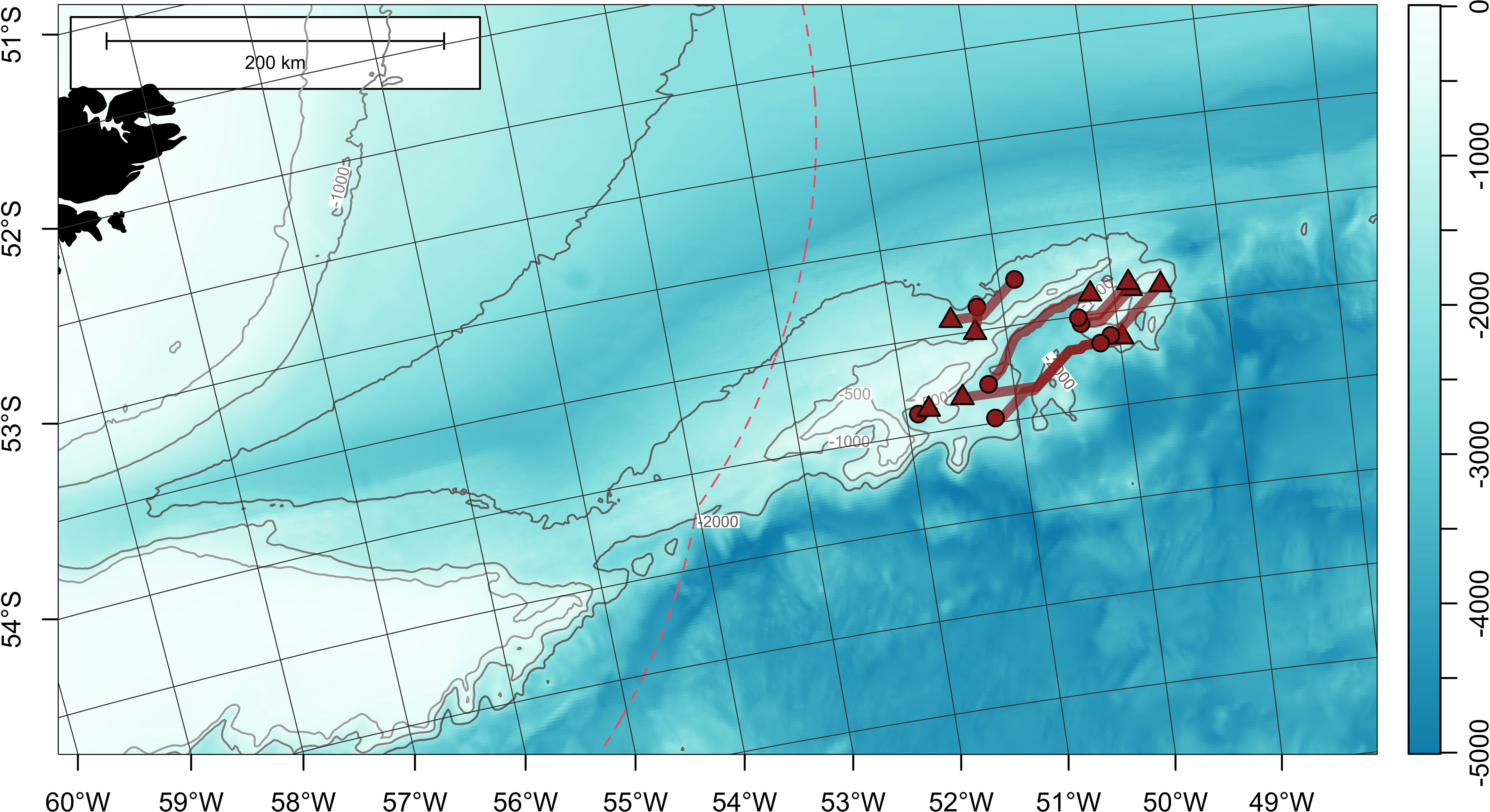
Figure 4 Least-cost paths for 9 satellite-tagged Patagonian toothfish measuring 110-119 cm total length, around Davis Bank, on the North Scotia Ridge. Tag deployment and popoff locations are indicated with circles and triangles, respectively. Bathymetry (in meters) is obtained from ETOPO 2022 (15-arcseconds) product through NOAA National Centers for Environmental Information (ncei.noaa.gov/maps/grid-extract). Bathymetry is plotted as a false-color overlay, and also contoured at 500, 1000 and 2000 m as gray lines. Color scale of the bathymetry differs from Figure 1 in order to enhance the visibility of topographic features. Exclusive economic zones (EEZs) are outlined with red dashed lines.
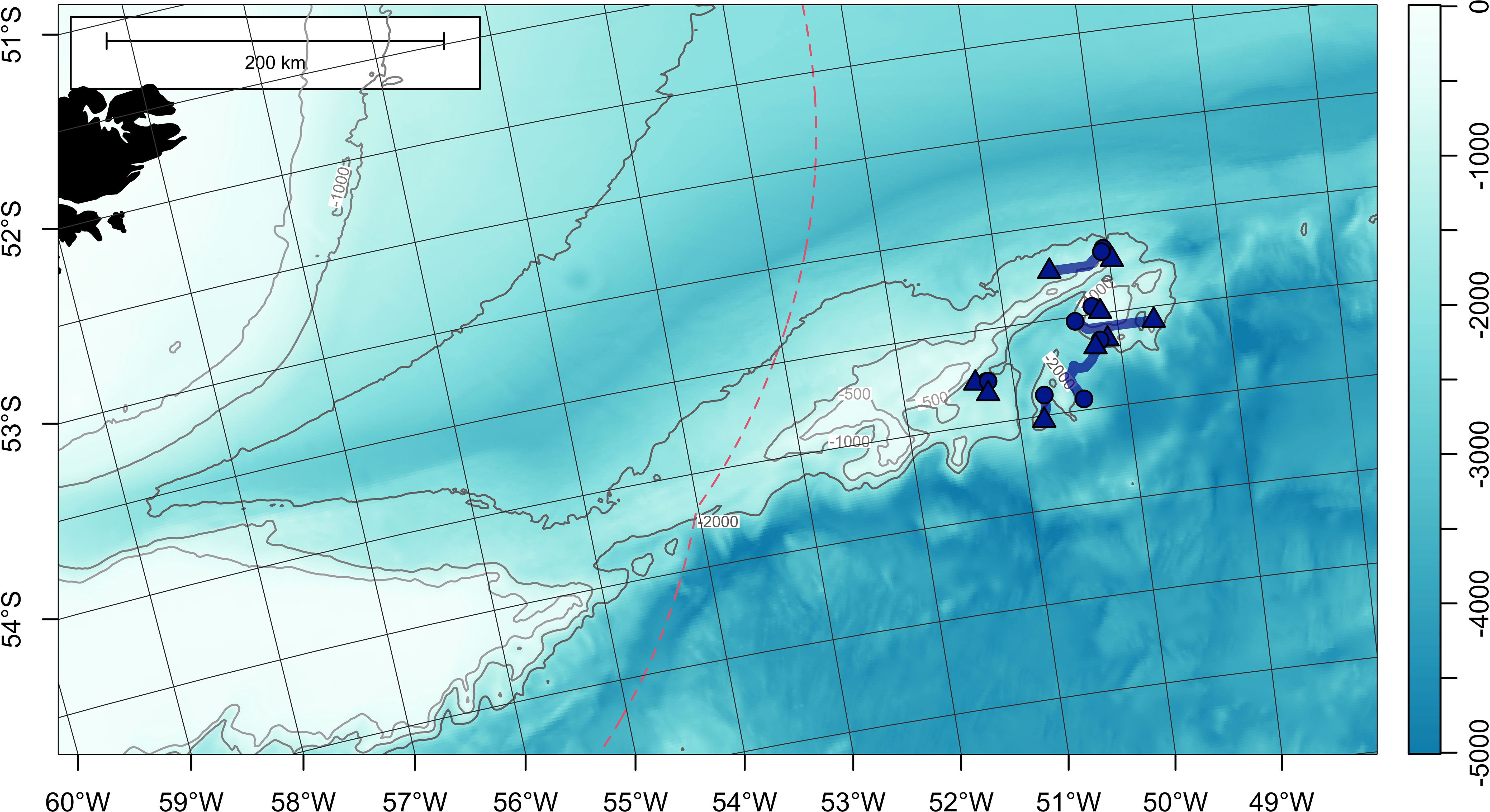
Figure 5 Least-cost paths for 9 satellite-tagged Patagonian toothfish measuring 120-129 cm total length, around Davis Bank, on the North Scotia Ridge. Tag deployment and popoff locations are indicated with circles and triangles, respectively. Bathymetry (in meters) is obtained from ETOPO 2022 (15-arcseconds) product through NOAA National Centers for Environmental Information (ncei.noaa.gov/maps/grid-extract). Bathymetry is plotted as a false-color overlay, and also contoured at 500, 1000 and 2000 m as grey lines. Color scale of the bathymetry differs from Figure 1 in order to enhance the visibility of topographic features. Exclusive economic zones (EEZs) are outlined with red dashed lines.
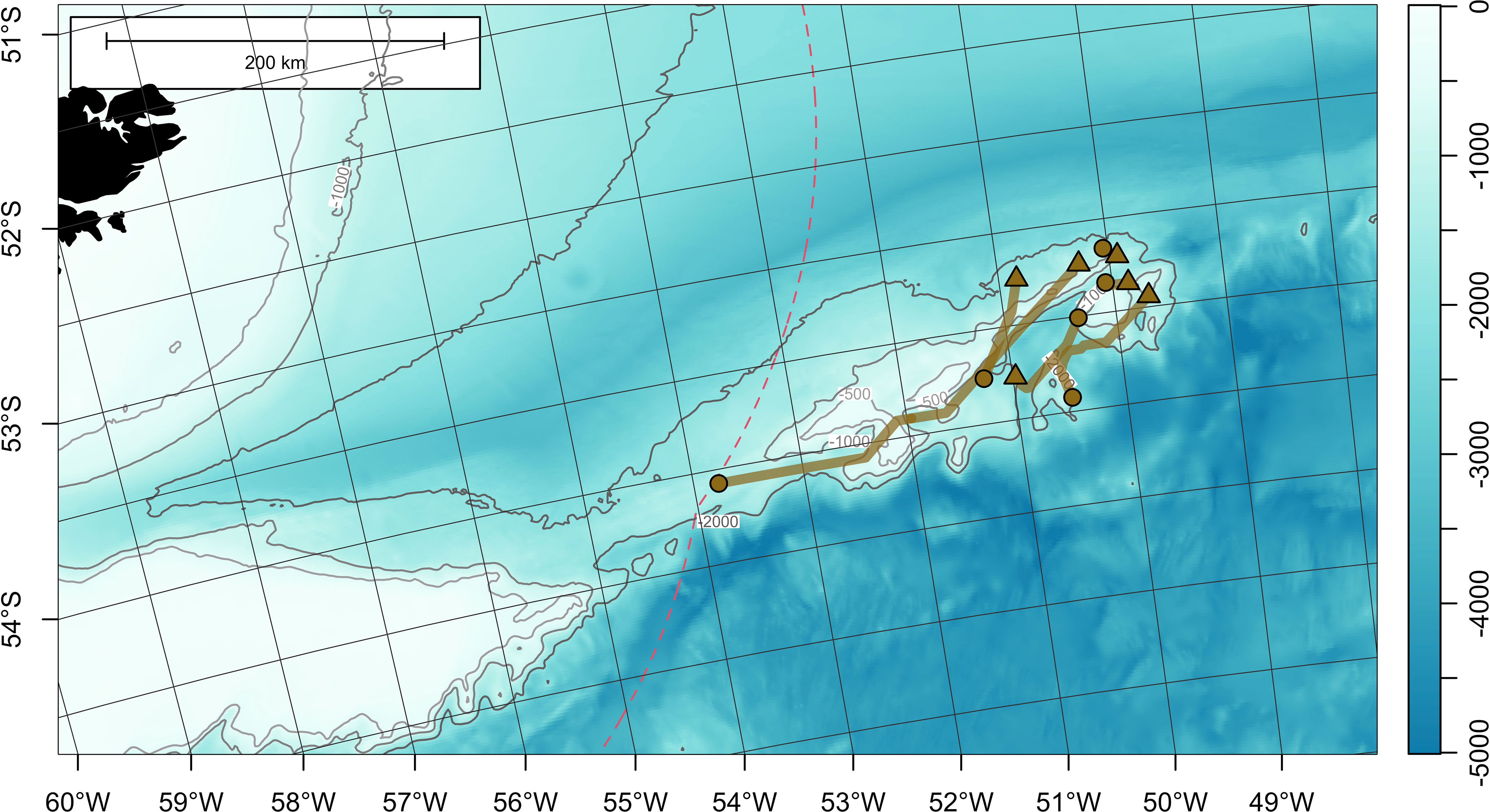
Figure 6 Least-cost paths for 6 satellite-tagged Patagonian toothfish measuring 130-139 cm total length, around Davis Bank, on the North Scotia Ridge. Tag deployment and popoff locations are indicated with circles and triangles, respectively. Bathymetry (in meters) is obtained from ETOPO 2022 (15-arcseconds) product through NOAA National Centers for Environmental Information (ncei.noaa.gov/maps/grid-extract). Bathymetry is plotted as a false-color overlay, and also contoured at 500, 1000 and 2000 m as gray lines. Color scale of the bathymetry differs from Figure 1 in order to enhance the visibility of topographic features. Exclusive economic zones (EEZs) are outlined with red dashed lines.
3.4 Long dispersals
Cases for toothfish dispersing > 300 km were few but they were all completed within the same calendar year (Table 2; Figure 7; Figure 1B). Fish 177062 (105 cm TL) moved west from its release location on Davis Bank, and went through the north of Burdwood Bank/Namuncurá before reaching the Falkland/Malvinas Plateau Basin, at the edge of the Malvinas Current (Figure 7). This PSATFLEX registered a first reporting location with the Argos satellite system on February 15 07:48 a.m. UTC. After a pin burn time of 3 hours, the tag was drifting on the surface for a maximum of 32 hours, putting it 42 km away from its initial popoff position. Fish 177062 covered a least-cost path distance of 929 km in 28 days at liberty, translating to a daily movement rate of 33.2 km day-1.
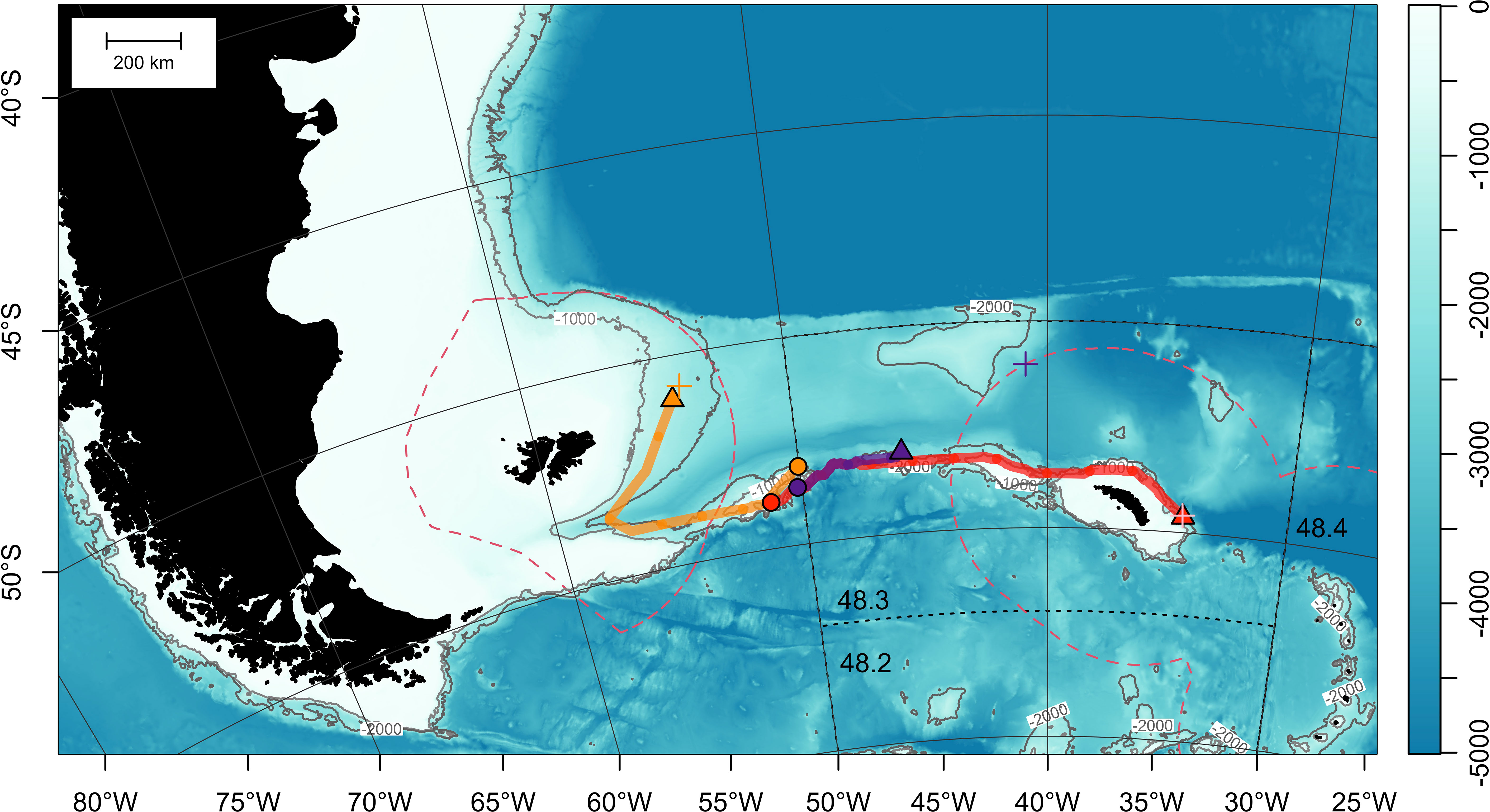
Figure 7 Least-cost paths for 3 satellite-tagged Patagonian toothfish that dispersed over 300 km (see Table 2 for metadata). Tag deployment and estimated popoff locations are indicated with circles and triangles, respectively. First Argos reporting locations are represented by crosses (+). Bathymetry (in meters) is obtained from ETOPO 2022 (15-arcseconds) product through NOAA National Centers for Environmental Information (ncei.noaa.gov/maps/grid-extract). Bathymetry is plotted as a false-color overlay, and also contoured at 1000 and 2000 m as gray lines. Color scale of the bathymetry differs from Figure 1 in order to enhance the visibility of topographic features. Exclusive economic zones (EEZs) are outlined with red dashed lines. Commission for the Conservation of Antarctic Marine Living Resources (CCAMLR) Convention Area (Subareas 48.2, 48.3 and 48.4) is marked by black dotted lines.
Fish 177084 (105 cm TL) undertook an eastward 1231-km journey along the North Scotia Ridge towards Shag Rocks and South Georgia (Figure 7). After 237 days at sea, this MiniPAT reported on September 26 07:32 a.m. UTC to the east of South Georgia off the 1000-m bathymetric contour, in a region with very slow surface current (Smith et al., 2010). Tag release sequence was initiated on September 25 20:00 p.m. UTC. After 7.5 hours, the release pin was burnt through, releasing the tag. On the sea surface, the tag drifted only 4 km before linking up with Argos satellites. Based on the least-cost path, movement rate was 5.2 km day-1.
The MiniPAT on fish 196767 (102 cm TL) detached on November 3, after 299 days at sea. However, this tag only registered a first reporting position on the Argos system on November 26. The cause for this delay is not obvious or deducible from the transmitted tag diagnostics, but it could be possible that biofouling reduced the buoyancy of the PSAT and hampered its ascent to the sea surface. Particle backtracking provided an estimate of the tag initial popoff location at 53.05°S 45.88°W, west of Shag Rocks (Table 2; Supplementary Figure 1; Supplementary Video 2). Given the extended drift period, no daily movement rate was estimated.
3.5 Evidence for location-specific depth behavior
Based on by the presence of fish 177084 off South Georgia in September 2019, we reviewed the ambient temperatures that the specimen experienced and depths it occupied to approximate the timing of its first arrival. Maximum temperatures experienced, all recorded at depths below 500 m, were under 1.7°C since July 14 till tag popoff (Figure 8A). Earlier in the time series, maximum and minimum temperatures began to drop from late April to early May (Figure 8A), during when the fish stayed predominantly below 800 m (Figure 8B). Minimum temperature first fell below 2°C in mid-May, and remained low through mid-June (Figure 8A). During this period, the fish occupied a bimodal depth distribution above 800 m (Figure 8B). A shift to occupying depths below 800 m resumed after mid-June and maintained until the tag detached and popped off (Figure 8B). Moving across longitudinally from Burdwood Bank to South Georgia at depths of 0-1000 m, water masses of ≤ 2°C can only be found after crossing the Antarctic Polar Front (Figure 1B), in waters east of 47°W, or an area referred to as the Shag Rocks Passage (Brandon et al., 2004; Smith et al., 2010). This implied that fish 177084 might have gone past the Antarctic Polar Front at a date much earlier than September, and possibly, as early as May. Taking this altogether, distinct phases of temperature experienced and changes in depth occupancy over the course of fish 177084’s journey towards South Georgia provide the first observations for D. eleginoides that vertical behaviors varied over place and time.
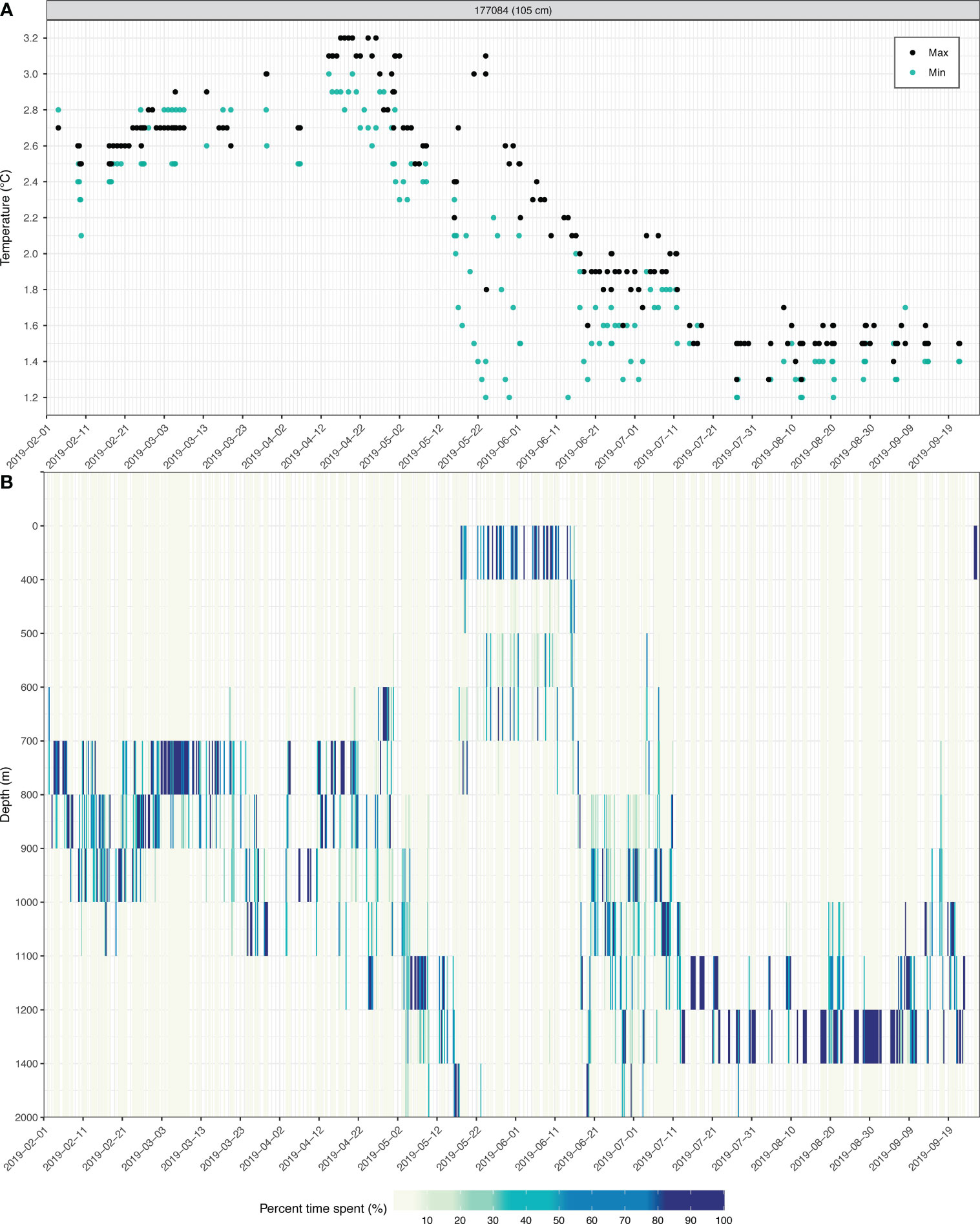
Figure 8 Transmitted underwater data logged by fish 177084 PSAT sensors throughout its time at liberty, summarized at 4-hour intervals. (A) Minimum (turquoise dots) and maximum (black dots) ambient temperature recorded. (B) Time-spent at depth intervals expressed at percentage (0-100%) of the 4-hour period (false color). Note not all acquired data can be transmitted and recovered by orbiting satellites, resulting in gaps along the x-axis.
4 Discussion
Current understanding on toothfish movement has been rooted in the concepts of site fidelity (e.g., Troccoli et al., 2023) and home range relocation (e.g., Lee et al., 2022). Data returned from our PSATs have revealed that Patagonian toothfish might move in more complex ways than previously thought. Similar to studies releasing thousands of conventional tags (CCAMLR Secretariat, 2017; Burch et al., 2019; Lee et al., 2022; Troccoli et al., 2023), dispersals > 300 km were observed in about 10% of our handful of electronic tags. The main distinction here is that, with satellite tagging, it is possible to observe toothfish in places and/times of year that are typically not sampled by the fisheries. This contrasts with conventional tagging in which tag recaptures are, by nature, incidental, and thus, are highly dependent on the timing, location and effort of survey and fishing activities. With PSATs, we showed that toothfish took only 1-9 months to disperse over 300 km from Davis Bank, and attained movement rates previously unobserved from conventional tag recaptures.
4.1 Movement connectivity
Dispersals from Davis Bank include: (1) northward movement to fishing grounds located northeast and east of the Falkland Islands/Isla Malvinas, (2) western movement towards Burdwood Bank, and (3) eastern movement towards South Georgia, also a known spawning location (Bamford et al., 2024). Most importantly, our tagged toothfish, all between 102-105 cm TL (n = 3), completed their long-distance dispersals within 10 months (Figure 7). This contrasts with Lee et al. (2022) observing that only fish > 120 cm TL undertook long dispersals. Based on an average recapture time of 2 years, Lee et al. (2022) concluded that large-scale, annual migrations undertaken by toothfish on a seasonal basis were unlikely, and long-distance movements are a result of home-range relocation. Our current findings contradict with this view as long dispersals can occur as short as within 1 month (fish 177062). The concept of “one-way” relocation requires further examination, as it relies on the assumption that adult toothfish possess limited mobility due to its white-muscle dominated physiology (Collins et al., 2010), and consequently, would not be capable of returning to where they have previously dispersed from through sustained swimming.
Dispersal patterns identified in this study can be integrated with those observed in other tagging studies (Brown et al., 2013; Lee et al., 2022; Troccoli et al., 2023) to form a more informed picture of regional connectivity. For instance, a tagged toothfish traveled 1009 km from north of the Falkland/Malvinas Slope to North Scotia Ridge (Lee et al., 2022), close to our PSAT release locations on Davis Bank. Coupling that example with fish 177062’s dispersal to the Falkland/Malvinas Plateau Basin, two-way exchanges between these sites become plausible. This connection could be extended by fish 177084’s eastward transit to South Georgia, linking toothfish hotspots along the northern section of the Scotia Arc. Connectivity patterns such as this one may yet to be better defined. Genetic techniques have detected putative migrants from other South American locations, including migrants from northern Peru detected in FLK samples, and southern Peru migrants found among South Georgia specimens (Canales-Aguirre et al., 2018). Moving in the opposite direction, putative migrants from South Georgia were found in specimens collected from FLK and southern Chile (Canales-Aguirre et al., 2018). Broad-scale connectivity has also been suggested for toothfish across the Kerguelen Plateau and with those at Crozet and Marion and Prince Edward Islands, deduced from a small number of migrants (37 out of 9417 recaptured fish) moving against the Antarctic Circumpolar Current (Burch et al., 2019). As the analyses of genetic markers, chemical signatures, morphological features and parasitic load patterns continue to provide new clues on population structure (e.g., Arkhipkin et al., 2022), fishery-independent tagging studies could show how movements of individuals are accomplished and result in the genetic connectivity patterns observed.
The route that individual toothfish took in long-distance dispersals indicates that it may become pelagic for a period to maneuver over deep water. Fish 177084’s eventual arrival at waters near South Georgia must have pointed that during part of its journey, it moved away from the bottom and traveled in the water column, for example, through the Shag Rocks Passage where bottom depths exceeded 3000 m (Olivé Abelló et al., 2021). Moreover, the rapid movement rate of fish 177062 suggests that, off the eastern Burdwood Bank, a toothfish may utilize the Subantarctic Front that offshoots into the Malvinas Current to travel north (Figure 1B). There, subsurface currents at 400-600 m can reach up to 0.1 ms-1 (Frey et al., 2021), and can provide an energy-efficient way to travel. Applications of magnetometers in electronic tags (e.g., Parker et al., 2014) may assist in characterizing toothfish navigational capabilities and physiological adaptations (e.g., visual physiology; Kiss, 2008) for their deep-sea niches.
4.2 Experimental design and future directions
Movements of marine fishes have traditionally been and continued to be inferred from conventional tag recaptures. The low cost of conventional tags (< $2 USD) has allowed that large sample sizes are routinely achieved, and tag recaptures be readily incorporated into stock assessment and simulation models used in fisheries management. In particular, the hundreds of thousands of conventional tags released on Patagonian toothfish during CCAMLR activities (CCAMLR 2017) provides a robust and growing data source to help improve stock definitions and delineate exchanges among management units. PSATs, as a matter of financial realities, will never reach the scale and statistical power provided by conventional tags, no matter how advances in technology may drive down the cost. Therefore, it is crucial that researchers design experiments to maximize the benefits of utilizing various, often complementary, tag types.
PSATs, with its unique advantage of being fishery independent, can detect toothfish presence in other locations around the vast Southern Ocean that are difficult to monitor through fisheries interactions. A lesson learnt, albeit painfully from our own mistake, is the need to collect light curves used for geolocation, in case of a toothfish spending a substantial amount of time above 400 m. PSAT deployments can also be extended to other life stages to profile movement dynamics more representatively. For instance, tagging sub-adults or juveniles at 70-90 cm TL is a priority, as they are frequently observed in the longline catch compositions within CCAMLR4 and in conventional tag recaptures off South Georgia (Earl and Readdy, 2022).
In this study, mission duration, or “popup date”, was configured to track toothfish movement across temporal scales, reduce incidental recapture of PSATs, and span known spawning periods. This approach is not new; in fact, Lutcavage et al. (1999) synchronized all PSATs to report on June 1, at the assumed peak of spawning for Atlantic bluefin tuna, in order to map the distribution of mature adults. Similarly, PSATs can be scheduled to provide spatial information on toothfish at a time of interest or in a data-poor situation, such as during the minor spawning period off South Georgia (Bamford et al., 2024). Decoupled from fishery recapture, PSATs offer temporal and practical flexibility that researchers can creatively exploit in their investigation of toothfish ecology.
Cooperative research with industry partners should also be encouraged. Apart from delivering concrete results in this study, the iterative planning process and field implementation provided a platform for our stakeholders to exchange ideas and develop solutions collaboratively. It also allowed fishers to better understand the logic behind research objectives and approaches, which oftentimes are obscure to them. In turn, fishers shared their valuable knowledge in at-sea operations and animal handling, something that should not be overlooked and can contribute to higher fish survival and data recovery. To address the myriad of challenges presented by climate change, stakeholders must work together in constructive and innovative ways to deliver the best, up-to-date scientific information.
4.3 Implications for fisheries management and conservation
With PSAT popping off South Georgia, we showed that toothfish dispersal links up areas outside and inside of the CCAMLR Convention Area. Marine fish’s apparent disregard for management boundaries, despite posing a headache to fisheries managers, is not uncommon (e.g., Lam et al., 2022). Recognizing this biological phenomenon can lead to an increased and much needed appreciation of regional connectivity (Moon et al., 2017), and a move towards integrated management and conservation across jurisdictions (Murphy et al., 2021). Coordination among all managed fisheries has already been recommended for a conspecific, Antarctic toothfish (D. mawsoni), as necessitated by the high genetic connectivity across their circumpolar distribution (Maschette et al., 2023). In the management of Dissostichus spp., CCAMLR is well positioned to leverage its knowledge, expertise and cooperation among its contracting parties to foster partnerships and improve regional governance5. This is especially important as the dynamics of the Southern Ocean are rapidly changing, and the Antarctic ecosystem may not be as isolated as previously thought (Fraser et al., 2018).
At a basic science level, how Patagonian toothfish are able to distribute across a broad latitudinal range (~ 50°), evident from D. eleginoides fisheries spanning the Southern Ocean, coastal South America to the equatorial Pacific (off Ecuador, Zambrano et al., 2023), remains unsolved. Novel connectivity mechanisms across the Antarctic Polar Front are yet to be explored, as invertebrates (e.g., brittle star, Galaska et al., 2017) and plants (e.g., kelp, Fraser et al., 2017) have managed a similar feat. As a start, we postulate that adult toothfish are capable of utilizing underwater currents to assist with their dispersal. A greater understanding on toothfish movement and navigation will widen our perspectives and enhance the biological realism of our fisheries and ecosystem models.
4.4 Concluding remarks
Satellite tagging of Patagonian toothfish in the Southwest Atlantic has offered new insights into fish movement, challenging us to consider that toothfish might not be as sedentary as currently assumed. While we only confirmed long dispersals in a handful of individuals, the short amount of time (< 1 year) that they used to cover large distances implies brief dispersal events could be missed by conventional tag recaptures and analyses of genetic signatures. Our understanding of Patagonian toothfish life history and ecology is likely to expand with the exciting fishery-independent clues on location, depth and temperature habitat that have not been made available in large volume until now.
Data availability statement
The original contributions presented in the study are included in the article/Supplementary Material. Further inquiries can be directed to the corresponding author.
Ethics statement
Ethical approval was not required for the study involving animals in accordance with the local legislation and institutional requirements because External tagging of toothfish is a standard procedure for commercial toothfish fisheries operating in and around CCAMLR areas in support of stock assessment practices. No special approval was required.
Author contributions
EK: Conceptualization, Funding acquisition, Methodology, Data curation, Investigation, Project administration, Supervision, Validation, Writing – review & editing, Writing – original draft. CL: Conceptualization, Funding acquisition, Data curation, Formal analysis, Investigation, Methodology, Software, Visualization, Writing – original draft, Writing – review & editing. GP: Data curation, Project administration, Resources, Writing – review & editing. JL: Conceptualization, Funding acquisition, Methodology, Project administration, Resources, Writing – review & editing.
Funding
The author(s) declare financial support was received for the research, authorship, and/or publication of this article. This work is supported by the National Institute of Fisheries Science, South Korea through award number NIFS R2024003. The authors declare that this study received funding from the Jeongil Corporation. The funder was not involved in the study design, collection, analysis, interpretation of data, the writing of this article or the decision to submit it for publication.
Acknowledgments
We acknowledge the Jeongil Corporation as a fantastic cooperative research partner, and in particular, we thank Kunwoong Ji, the captain and crew of F/V No. 8 Meridian. Their open-mindedness and field expertise were instrumental to the successful execution of this work. We also want to express our special appreciation for four scientific observers who were immensely effective in the field and exceptionally meticulous with their work: HeeChul Choi, Deukkyun Ahn, Jeongyun Park, and Bokyun Choi. We thank Globalpesca SpA in recovering a PSAT and reporting recapture metadata from the Chilean fleet. Last but not least, we acknowledge Drs. Doo Nam Kim, Jeong Seok Park and Sukyung Kang for their enduring leadership and unwavering support in the project.
Conflict of interest
The authors declare that the research was conducted in the absence of any commercial or financial relationships that could be construed as a potential conflict of interest.
Publisher’s note
All claims expressed in this article are solely those of the authors and do not necessarily represent those of their affiliated organizations, or those of the publisher, the editors and the reviewers. Any product that may be evaluated in this article, or claim that may be made by its manufacturer, is not guaranteed or endorsed by the publisher.
Supplementary material
The Supplementary Material for this article can be found online at: https://www.frontiersin.org/articles/10.3389/fmars.2024.1334339/full#supplementary-material
Footnotes
- ^ https://www.ccamlr.org/en/publications/fishing-gear-library
- ^ https://static.wildlifecomputers.com/mds/Towed-Tag-Anchor-Suite.pdf
- ^ https://www.hinchinbrookei.com/product-page/anchors
- ^ https://fisheryreports.ccamlr.org/
- ^ https://www.ccamlr.org/en/organisation/second-ccamlr-performance-review
References
Arkhipkin A. I., Brickle P., Lee B., Shaw P. W., McKeown N. J. (2022). Taxonomic re-appraisal for toothfish (Dissostichus: Notothenioidea) across the Antarctic Polar Front using genomic and morphological studies. J. Fish Biol. 100 (5), 1158–1170. doi: 10.1111/jfb.15013
Artana C., Ferrari R., Koenig Z., Saraceno M., Piola A. R., Provost C. (2016). Malvinas Current variability from Argo floats and satellite altimetry. J. Geophys. Res. Oceans 121, 4854–4872. doi: 10.1002/2016JC011889
Ashford J. R., Arkhipkin A. I., Jones C. M. (2007). Otolith chemistry reflects frontal systems in the Antarctic Circumpolar Current. Mar. Ecol. Prog. Ser. 351, 249–260. doi: 10.3354/meps07153
Ashford J. R., Jones C. M., Hofmann E. E., Everson I., Moreno C. A., Duhamel G., et al. (2008). Otolith chemistry indicates population structuring by the Antarctic Circumpolar Current. Can. J. Fish. Aquat. Sci. 65, 135–146. doi: 10.1139/f07-158
Bamford C. C. G., Hollyman P. R., Abreu J., Darby C., Collins M. A. (2024). Spatial, temporal, and demographic variability in patagonian toothfish (Dissostichus eleginoides) spawning from twenty-five years of fishery data at South Georgia. Deep Sea Res. Part I Oceanogr. Res. Pers. 203 (2024), 104199. doi: 10.1016/j.dsr.2023.104199
Brandon M. A., Naganobu M., Demer D. A., Chernyshkov P., Trathan P. N., Thorpe S. E., et al. (2004). Physical oceanography in the Scotia Sea during the CCAMLR 2000 survey, austral summer 2000. Deep Sea Res. Part II Top. Stud. Oceanogr. 51 (12-13), 1301–1321. doi: 10.1016/j.dsr2.2004.06.006
Brown J., Brickle P., Scott B. E. (2013). Investigation the movements and behaviour of Patagonian toothfish (Dissostichus eleginoides Smitt 1898) around the Falkland Islands using satellite linked archival tags. J. Exp. Mar. Biol. Ecol. 443, 65–74. doi: 10.1016/j.jembe.2013.02.029
Burch P., Péron C., Potts J., Ziegler P., Welsford D., Dell J., et al. (2019). “Estimating Patagonian toothfish (Dissostichus eleginoides) movement on the Kerguelen Plateau: reflections on 20 years of tagging at Heard island and McDonald islands,” in The kerguelen plateau: marine ecosystem and fisheries (Kingston: The Department of the Environment and Energy, Australian Antarctic Division), 237–245. Proceedings of the Second Symposium.
Canales-Aguirre C. B., Ferrada-Fuentes S., Galleguillos R., Oyarzun F. X., Hernández C. E. (2018). Population genetic structure of Patagonian toothfish (Dissostichus eleginoides) in the Southeast Pacific and Southwest Atlantic Ocean. PeerJ 6, e4173. doi: 10.7717/peerj.4173
CCAMLR Secretariat (2014) Toothfish and skates tagging methods. Available at: https://www.ccamlr.org/node/85702.
CCAMLR Secretariat (2016). The value of marine resources harvested in the CCAMLR Convention Area – an assessment of GVP, Document CCAMLR-XXXV/10. Hobart, Australia.
CCAMLR Secretariat (2017). Long-distance movements of Patagonian (Dissostichus eleginoides) and Antarctic toothfish (D. mawsoni) from fishery-based mark recapture data, Document CCAMLR-WG-FSA-17/06. Hobart, Australia.
Collins M. A., Brickle P., Brown J., Belchier M. (2010). “The Patagonian toothfish: biology, ecology and fishery,” in Advances in marine biology 58. Ed. Lesser M. (Burlington: Academic Press), 227–300.
Collins M. A., Hollyman P. R., Clark J., Söffker M., Yates O., Phillips R. A. (2021). Mitigating the impact of longline fisheries on seabirds: Lessons learned from the South Georgia Patagonian toothfish fishery (CCAMLR Subarea 48.3). Mar. Pol. 131, 104618. doi: 10.1016/j.marpol.2021.104618
Dagestad K.-F., Röhrs J., Breivik Ø., Ådlandsvik B. (2018). OpenDrift v1.0: a generic framework for trajectory modelling. Geosci. Model. Dev. 11, 1405–1420. doi: 10.5194/gmd-11-1405-2018
Dalziel I. W. D., Lawver L. A., Norton I. O., Gahagan L. M. (2013). The scotia arc: genesis, evolution, global significance. Annu. Rev. Earth. Planet. Sci. 41 (1), 767–793. doi: 10.1146/annurev-earth-050212-124155
Earl T., Readdy L. (2022). Assessment of Patagonian toothfish (Dissostichus eleginoides) in Subarea 48.3. Document WG-FSA-2022/57 Rev. 1. Hobart, Australia.
Farrugia T. J. (2018). Tagging report PSAT Patagonian toothfish (Dissostichus eleginoides) (Falkland Islands: Falkland Islands Government Directorate of Natural Resources Fisheries Department Stanley), 11.
Fraser C. I., Kay G. M., Plessis M. D., Ryan P. G. (2017). Breaking down the barrier: dispersal across the Antarctic Polar Front. Ecography 40, 235–237. doi: 10.1111/ecog.02449
Fraser C. I., Morrison A. K., Hogg A. M., Macaya E. C., van Sebille E., Ryan P. G., et al. (2018). Antarctica’s ecological isolation will be broken by storm-driven dispersal and warming. Nat. Clim Change 8, 704–708. doi: 10.1038/s41558-018-0209-7
Frey D. I., Piola A. R., Krechik V. A., Fofanov D. V., Morozov E. G., Silvestrova K. P., et al. (2021). Direct measurements of the Malvinas Current velocity structure. J. Geophys. Res.: Oceans 126, e2020JC016727. doi: 10.1029/2020JC016727
Galaska M. P., Sands C. J., Santos S. R., Mahon A. R., Halanych K. M. (2017). Crossing the divide: admixture across the Antarctic Polar Front revealed by the brittle star Astrotoma agassizii. Biol. Bull. 232 (3), 198–211. doi: 10.1086/693460
Galuardi B., Lutcavage M. (2012). Dispersal routes and habitat utilization of juvenile Atlantic bluefin tuna, Thunnus thynnus, tracked with mini PSAT and archival tags. PloS One 7 (5), e37829. doi: 10.1371/journal.pone.0037829
Grilly E., Reid K., Thanassekos S. (2022). Long-distance movements of Antarctic toothfish (Dissostichus mawsoni) as inferred from tag-recapture data. J. Fish Biol. 100 (5), 1150–1157. doi: 10.1111/jfb.14941
Horn P. L. (2002). Age and growth of Patagonian toothfish (Dissostichus eleginoides) and Antarctic toothfish (D. mawsoni) in waters from the New Zealand subantarctic to the Ross Sea, Antarctica. Fish. Res. 56 (3), 275–287. doi: 10.1016/S0165-7836(01)00325-3
Irvine L. M., Winsor M. H., Follett T. M. (2020). An at-sea assessment of Argos location accuracy for three species of large whales, and the effect of deep-diving behavior on location error. Anim. Biotelemetry 8 (20), 10.1186. doi: 10.1186/s40317-020-00207-x
Kim D. N., Kim Y. S. (2008). Distribution of Patagonian toothfish (Dissostichus eleginoides) by bottom longliner in the southeastern Atlantic Ocean. Bulletin of the Korean society of Fisheries Technology. Korean Soc. Fisheries Ocean Technol. 44, 304–311. doi: 10.3796/ksft.2008.44.4.304
Kiss A. J. (2008). “The antarctic toothfish: A new model system for eye lens biology,” in Animal models in eye research. Ed. Tsonis P. A. (Academic Press), 48–56. doi: 10.1016/B978-0-12-374169-1.00015-1
Lam C. H., Tam C., Lutcavage M. E. (2022). Connectivity of striped marlin from the Central North Pacific Ocean. Front. Mar. Sci. 9. doi: 10.3389/fmars.2022.879463
Laptikhovsky V., Arkhipkin A., Brickle P. (2006). Distribution and reproduction of the Patagonian toothfish Dissostichus eleginoides Smitt around the Falkland Islands. J. Fish Biol. 68, 849–861. doi: 10.1111/j.0022-1112.2006.00973.x
Lee B., Arkhipkin A., Randhawa H. S. (2021). Environmental drivers of Patagonian toothfish (Dissostichus eleginoides) spatial-temporal patterns during an ontogenetic migration on the Patagonian Shelf. Estuar. Coast. Shelf Sci. 259, 107473. doi: 10.1016/j.ecss.2021.107473
Lee B., Skeljo F., Randhawa H. S., Arkhipkin A. (2022). Deep-sea movement patterns of the Patagonian toothfish Dissostichus eleginoides Smitt in the Southwest Atlantic. Mar. Freshw. Res. 73 (6), 833–845. doi: 10.1071/MF21338
Lutcavage M. E., Brill R. W., Skomal G. B., Chase B. C., Howey P. W. (1999). Results of pop-up satellite tagging of spawning size class fish in the Gulf of Maine: do North Atlantic bluefin tuna spawn in the mid-Atlantic? Can. J. Fish Aquat. Sci. 56 (2), 173–177. doi: 10.1139/f99-016
Maschette D., Wotherspoon S., Polanowski A., Deagle B., Welsford D., Ziegler P. (2023). Circumpolar sampling reveals high genetic connectivity of Antarctic toothfish across their spatial distribution. Rev. Fish. Biol. Fisheries 33, 295–310. doi: 10.1007/s11160-023-09756-9
Moon K. L., Chown S. L., Fraser C. I. (2017). Reconsidering connectivity in the sub-Antarctic. Biol. Rev. 92, 2164–2181. doi: 10.1111/brv.12327
Murphy E. J., Johnston N. M., Hofmann E. E., Phillips R. A., Jackson J. A., Constable A. J., et al. (2021). Global Connectivity of Southern Ocean Ecosystems. Front. Ecol. Evol. 9, 624451. doi: 10.3389/fevo.2021.624451
Olivé Abelló A., Pelegrí J. L., Machín F. J., Vallès-Casanova I. (2021). The transfer of Antarctic circumpolar waters to the western South Atlantic Ocean. J. Geophys. Res: Oceans 126, e2020JC017025. doi: 10.1029/2020JC017025
Park K. J., Choi S., An D. (2021). Distribution of fishing grounds of Korean bottom longline and annual change of CPUE of the Patagonian toothfish Dissostichus eleginoides in Southwest Atlantic. Korean J. Aquat. Sci. 54 (1), 129–134. doi: 10.5657/KFAS.2021.0129
Park Y. H., Durand I. (2019). Altimetry-derived antarctic circumpolar current fronts. SEANOE. doi: 10.17882/59800
Park Y. H., Park T., Kim T. W., Lee S. H., Hong C. S., Lee J. H., et al. (2019). Observations of the Antarctic Circumpolar Current over the Udintsev Fracture Zone, the narrowest choke point in the Southern Ocean. J. Geophys. Res. Oceans 124, 4511–4528. doi: 10.1029/2019JC015024
Parker S. J., Webber D. N., Arnold. R. (2014). Deployment and recovery of an archival tag on an Antarctic toothfish in the Ross Sea, Document CCAMLR-WG-FSA-14/64. Hobart, Australia.
Skeljo F., Lee B., Winter A. (2022). Stock assessment report - Patagonian toothfish (Dissostichus eleginoides) (Falkland Islands: Fisheries Department Directorate of Natural Resources Falkland Islands Government Stanley), 47.
Smith I. J., Stevens D. P., Heywood K. J., Meredith M. P. (2010). The flow of the Antarctic Circumpolar Current over the North Scotia Ridge. Deep Sea Res. Part I Oceanogr. Res. Pap. 57 (1), 14–28. doi: 10.1016/j.dsr.2009.10.010
Touma J., García K. K., Bravo S., Leiva F., Moya J., Vargas-Chacoff L., et al. (2019). De novo assembly and characterization of Patagonian toothfish transcriptome and develop of EST-SSR markers for population genetics. Front. Mar. Sci. 6. doi: 10.3389/fmars.2019.00720
Troccoli G. H., Martínez P. A., Di Marco E. J., Waessle J. A., Wöhler O. C. (2023). Migratory patterns of Patagonian toothfish (Dissostichus eleginoides) in the southwestern Atlantic Ocean. Mar. Fish. Sci. 36 (3), 245–265. doi: 10.47193/mafis.3632023010907
van Etten J., Hijmans R. J. (2010). A geospatial modelling approach integrating archaeobotany and genetics to trace the origin and dispersal of domesticated plants. PloS One 5 (8), e12060. doi: 10.1371/journal.pone.0012060
Keywords: dispersal, migration, stock structure, Southwest Atlantic, connectivity, site fidelity, mixing, PSAT
Citation: Kim E, Lam CH, Park GJ and Lee JH (2024) Satellite tagging confirms long distance movement and fast dispersal of Patagonian toothfish (Dissostichus eleginoides) in the Southwest Atlantic. Front. Mar. Sci. 11:1334339. doi: 10.3389/fmars.2024.1334339
Received: 07 November 2023; Accepted: 10 January 2024;
Published: 26 January 2024.
Edited by:
Steven Parker, Commission for the Conservation of Antarctic Marine Living Resources (CCAMLR), AustraliaReviewed by:
Christopher Jones, National Oceanic and Atmospheric Administration, United StatesJin-Hyoung Kim, Korea Polar Research Institute, Republic of Korea
Copyright © 2024 Kim, Lam, Park and Lee. This is an open-access article distributed under the terms of the Creative Commons Attribution License (CC BY). The use, distribution or reproduction in other forums is permitted, provided the original author(s) and the copyright owner(s) are credited and that the original publication in this journal is cited, in accordance with accepted academic practice. No use, distribution or reproduction is permitted which does not comply with these terms.
*Correspondence: Eunjung Kim, eunjungkim@korea.kr
†These authors have contributed equally to this work and share first authorship