- Department of Life Sciences, Texas A&M University-Corpus Christi, Corpus Christi, TX, United States
Anthropogenic activity in coastal areas can damage marine habitats and alter marine mammal behavior and habitat use. Understanding behavioral associations with diverse habitat features in industrialized coastal areas is crucial for marine mammal conservation management. A shore-based digital theodolite was used to assess the behavioral states and habitat use of common bottlenose dolphins (Tursiops truncatus) off Port Aransas, Texas across seasons. The relationship between behavioral states and environmental and geographical variables were analyzed. Behavioral hotspots were identified. Dolphins frequently foraged (46%), indicating the area is an important feeding ground. Dolphins also traveled (25%) and milled (22%), with less frequent occurrences of socializing (6%) and resting (1% of observations). Season, time of day, and distance to shoreline were significant predictors of foraging and traveling behavioral states. Dolphins engaged in all behavioral states closer to seawall shorelines than to mangroves and natural seagrass beds, suggesting that hard shoreline features may influence daily activity patterns. Despite daily anthropogenic operations, bottlenose dolphins use features of the industrialized area (i.e., deeply dredged channels, human-engineered seawalls) to engage in a variety of behaviors. Monitoring of dolphin behavioral states and habitat use in active ship channels are needed to assess changes from baseline data from increasing coastal development and vessel activities.
1 Introduction
Understanding how marine mammals utilize different habitats in coastal environments is critical for informing management and conservation practices (Ingram and Rogan, 2002; Di Sciara et al., 2016). Many marine habitats continuously experience anthropogenic modifications with the potential to alter marine mammal behavior as human populations increase along the coast (Caldwell, 2016; Wells and Scott, 2018). Marine mammals, including dolphins, whales, and manatees may use urban areas with high anthropogenic activities regardless of frequent disturbances (Gubbins, 2002; Chilvers et al., 2005; Kraus and Rolland, 2009; Brown et al., 2019; Cloyed et al., 2022). Habitat preferences may be influenced by temporal factors (e.g., season, time of day), tidal characteristics (current speed, tidal state), and bathymetric features (e.g., water depth, slope; distances to shore), in addition to oceanographic features (e.g., temperature, salinity), prey distribution, predation pressure, and anthropogenic activities (Shane et al., 1986; Heithaus, 2001; Heithaus and Dill, 2002; Hastie et al., 2004; Karczmarski et al., 2005; Bejder et al., 2006; Saj and Sicotte, 2007; van Ginkel et al., 2018; Pearson, 2019). The effects of environmental, geographic, and biotic factors are not necessarily mutually exclusive and can have cumulative and cascading impacts. Common bottlenose dolphins (Tursiops truncatus; herein referred to as “bottlenose dolphins”) often inhabit coastal areas and may travel long distances within inshore and offshore environments, while others demonstrate high site fidelity to small areas and move short distances between habitats (Toth et al., 2011; Wells and Scott, 2018). Therefore, bottlenose dolphins may engage in a variety of behavioral states in geographically small areas with distinct habitats.
Temporal factors and tidal characteristics can contribute to variations in dolphin behavior occurrences in coastal areas. Seasonal movement in the prey species of bottlenose dolphins may alter foraging locations and tactics (Shane et al., 1986). In the dredged Galveston Ship Channel, Texas, bottlenose dolphins were more likely to forage in the morning and rest and socialize at mid-day during the summer (Piwetz, 2019). In Argentina, bottlenose dolphins rested mainly in the morning, while foraging and socializing (e.g., mate, play, body contact) increased in the afternoon (Würsig and Würsig, 1979). Bottlenose dolphins in the Sado Estuary, Portugal, milled (moved non-directionally) and traveled in the early afternoon (Harzen, 2002). In Aransas Pass, Texas, bottlenose dolphins oriented against strong tidal currents, often ebb tides, which may be a foraging tactic (Shane, 1977, 1980).
Geographic coastal features (e.g., channels, passes, open bays) and changes in bathymetry may influence dolphin behavioral states. Along the Texas coast, bottlenose dolphins favored dredged ship channels and narrow passes over open bay environments (Leatherwood and Reeves, 1983; Ronje et al., 2020). Bottlenose dolphin distribution patterns in dredged channels may reflect enhanced foraging opportunities in areas with steep seabed gradients (Allen et al., 2001; Hastie et al., 2004) or natural bottlenecks for traveling fish species (Henderson and Würsig, 2007). Some bottlenose dolphins chase/herd fish and disturb bottom sediments (Connor et al., 2019; Wells, 2019), which can be enhanced by natural structures (e.g., sand, mangroves, seagrass beds) and human-made obstacles (e.g., bridges, seawalls, fishing nets) (Rossbach, 1999; Torres and Read, 2009; Cantor et al., 2018). For example, bottlenose dolphins milled around rocky shoreline structures when not searching for prey near natural hard outcroppings (Würsig and Würsig, 1979). Bottlenose dolphins frequently foraged and traveled in the turbulent, deep, dredged channels (14 m deep) near Aransas Pass (Shane, 1977; Leatherwood and Reeves, 1983; Shane et al., 1986). Deep channels and passes provide dolphins with more three-dimensional space and maneuverability to dive and avoid potentially harmful coastal anthropogenic activities at the water’s surface compared to shallow bays (Hastie et al., 2004). However, bottlenose dolphins in Texas waters often socialized in open bays away from vessel activity (Shane, 1977; Shane et al., 1986). Resting dolphins appear to have reduced awareness of their surroundings and may take refuge in habitats they have deemed safe or close to shore, potentially to avoid predators in deep offshore water (Würsig and Würsig, 1979; Heithaus, 2001; Lammers, 2019).
The Corpus Christi Ship Channel (CCSC) is the main ship channel leading from the Gulf of Mexico to the inshore Port of Corpus Christi, Texas. The CCSC has undergone extensive infrastructure development and oil exportation growth over the last 40 years. A national oil export ban was lifted in 2015, which stimulated increased factory development, large-scale dredging projects, and high amounts of vessel activity in the area to accommodate port expansions (U.S. Coast Guard, 2019). Previous studies on bottlenose dolphin movement and distribution in the CCSC are dated to the 1980’s, prior to the expansive escalation in port growth (Shane, 1977, 1980; Leatherwood and Reeves, 1983). The objective of this study was to determine if bottlenose dolphin behavioral states vary with environmental and geographic factors in the CCSC-Aransas Pass confluence area. Understanding bottlenose dolphin behavior and habitat associations can aid in improving conservation of species and habitats in understudied industrialized and urbanized developing coastal ports.
2 Materials and methods
2.1 Study area
The Corpus Christi Ship Channel (CCSC, 14.6 m deep), Aransas Channel (AC, 4.3 m deep), and Lydia Ann Channel (LAC, 7.6 m deep) converge in the CCSC-Aransas Pass confluence area along the South Texas coast between Harbor Island, San José Island, and Mustang Island before extending into the Gulf of Mexico through Aransas Pass (27°50’42.1”N 97°03’29.1”W; Figure 1). All three dredged channels are composed of mud and flake (U.S. Coast Guard, 2019) and are utilized for vessel travel between the Gulf of Mexico and protected inshore Corpus Christi Bay. The CCSC and confluence area near the town of Port Aransas are characterized by human-engineered shorelines of granite blocks and concrete seawalls that provide protection from flooding and waves created by vessel movement. Natural shorelines (e.g., rocks, sand) and coastal habitats (e.g., mangroves, seagrass beds) are located along the AC and LAC bordering undeveloped environments (Figure 1). The CCSC-Aransas Pass confluence area experiences a diurnal tide with a 0.42 m range and current speeds averaging approximately 2 kn (NOAA National Ocean Service, 2022). During ebb and flood tides, surface observations of strong currents from each channel converge, contributing to nutrient mixing.
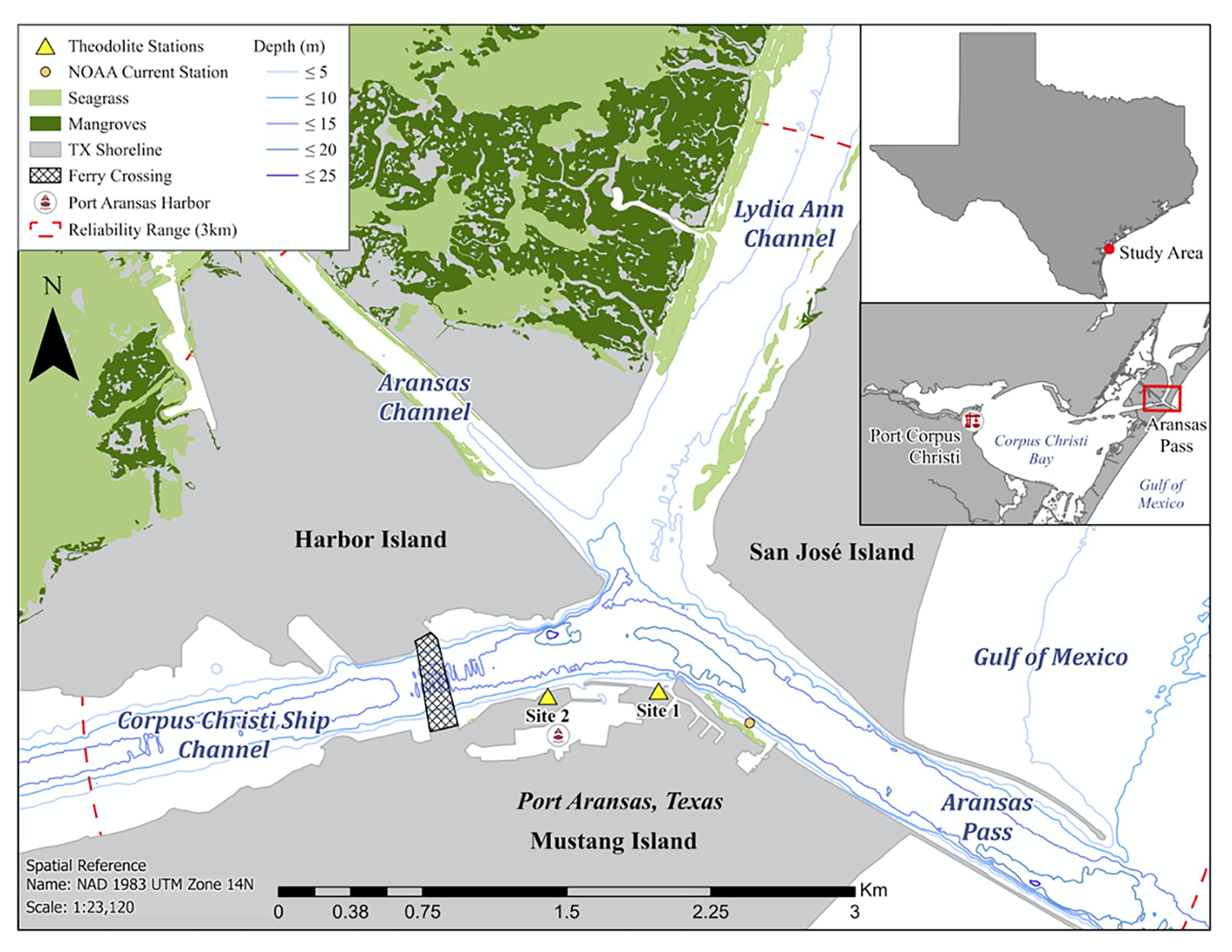
Figure 1 Theodolite stations (site 1: 27°50.4867’N, 97°3.4750’W; site 2: 27°50.4767’N, 97°3.8267’W) and marine habitat types in the CCSC-Aransas Pass, Texas area.
2.2 Sampling method
Bottlenose dolphin geographic positions and behavioral states were recorded during daylight hours in 6-hour intervals using non-invasive techniques from two elevated land-based theodolite stations along the CCSC in Port Aransas between June 2021 and September 2022. A digital theodolite (Sokkia Model DT5/DT5S, 30x magnification) with a known position and height above sea level and two reference positions were used to calculate a Global Positioning System (GPS) coordinate of a surfacing dolphin (Harzen, 2002). The theodolite was positioned on a level surface with an eyepiece height positioned 24.3 – 24.7 m (Site 1) and 7 – 8 m (Site 2) above sea level (Figure 1). Sampling was prioritized at Site 1 due to its elevated height and proximity to the convergence area. The geographic positions of individual dolphins were recorded by aligning the theodolite monocular crosshair on the focal dolphin at the waterline when it surfaced. A dolphin group included adults with or without calves (< 2/3 adult size and echelon swimming position; Shane, 1990) and was defined as individuals within approximately 100 m of each other engaged in similar simultaneous behaviors (Azzellino et al., 2008). The mean dolphin group size ± S.D. is reported. The position of the middle individual was recorded when the dolphin group was close together, while the approximate center of the group was recorded when the group was spread out. A Dell laptop (Inspiron 3179) with Mysticetus software (version 2021.22) was connected to the theodolite to convert theodolite x and y angle measurements into Global Positioning System (GPS) coordinates in real-time and store observed dolphin behavioral states. Sampling consisted of one theodolite operator/observer and one computer operator/observer. The theodolite operator remained the same individual to eliminate observer bias.
Dolphin behavioral states (forage, mill, rest, socialize, travel; Table 1) were initially observed using binoculars (Bushnell, 12x50 mm; Lakwar, 10x50 mm). Systematic scans of the entire survey area were conducted for approximately 20 minutes every hour and at the beginning and end of each field day. When dolphins were observed during scans, the behavioral states and locations of dolphins were recorded. When behavioral states were indiscernible or several occurred simultaneously, the behavioral state was categorized as “other” and excluded from the analyses. Each dolphin position was recorded until individuals/groups moved beyond the theodolite’s reliable visibility range (> 3 km; Sagnol et al., 2014; Figure 1) or environmental conditions (e.g., Beaufort state > 3, fog, sunset, rain) restricted visibility.
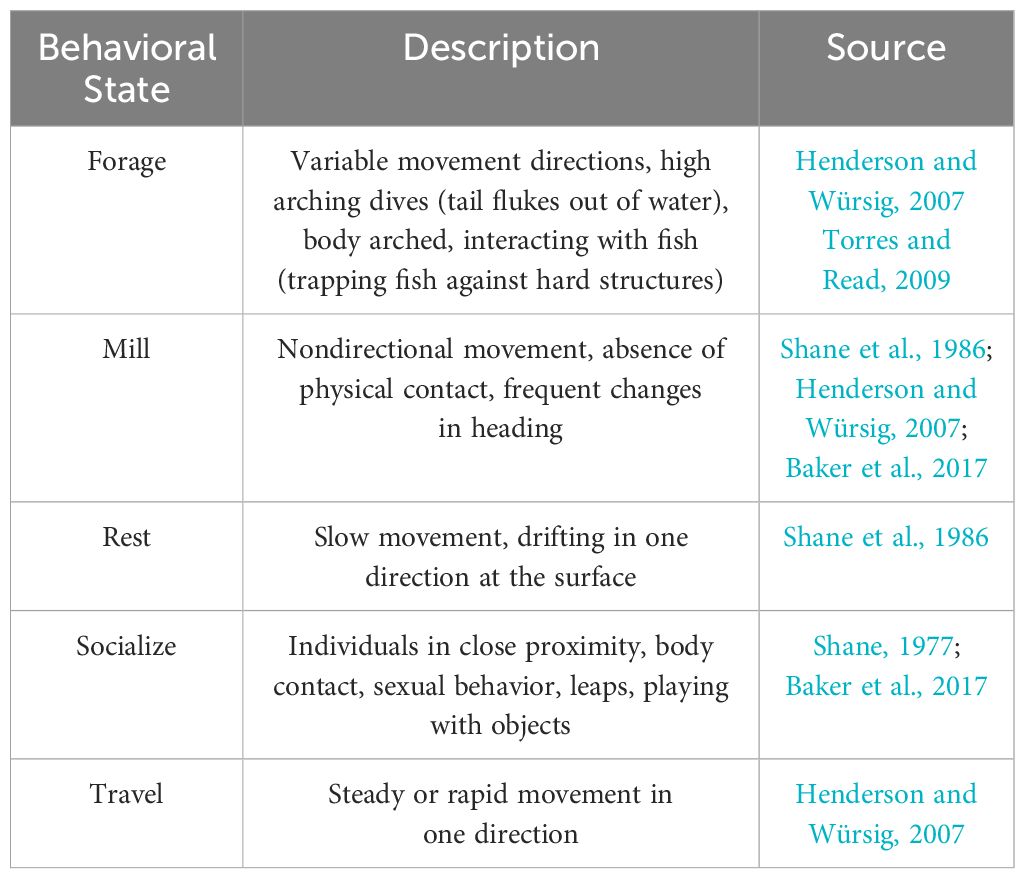
Table 1 Behavioral state definitions of common bottlenose dolphins adapted from published literature.
2.3 Analyses
A multinomial logistic regression (MLR) using the “nnet” package of RStudio statistical software (version 2022.07.2) was used to assess the relationship between dolphin behavioral state and environmental and geographic features (Koper et al., 2015; Hua et al., 2021; Félix et al., 2022; McNulty, n.d). Environmental factors included season, time of day, current speed, tidal state, and water depth, while geographic factors included shortest distances to shorelines, mangroves, and seagrass beds. Seasons were categorized as Summer (June – August), Fall (September – November), Winter (December – February), and Spring (March – May). Sampling was divided into Morning (0800 – 1059 hrs), Mid-day (1100 – 1359 hrs), Early Afternoon (1400 – 1659 hrs), and Late Afternoon (1700 – 2000 hrs). A National Oceanic Atmospheric Administration (NOAA) measurement station (cc0301, submerged 3.9 m) in the CCSC (Figure 1) automatically recorded daily current speed (knots) and direction (degrees true) in 6-minute intervals; data were obtained post hoc from the NOAA Center for Operational Oceanographic Products and Services (NOAA National Ocean Service, 2022). The measurement station typically displayed an approximate flood tide of 307 degrees in the CCSC-Aransas Pass confluence area. Tidal state ranges were established using current direction and an azimuth circle and were categorized as flood (262.25 – 351.75 degrees), slack (172.75 – 262.25 degrees and 351.75 – 83.25 degrees), and ebb (83.25 – 172.75 degrees) tides. The shortest distance between each dolphin group to the closest bathymetric line (water depth) and the closest shoreline were calculated using the Generate Near Table tool in ArcGIS Pro (version 2.8.3, ESRI- Environmental Systems Research Institute, Inc). The Euclidean Distance tool was used to find the shortest distance from any given dolphin group to mangrove substrates and seagrass beds, followed by the Cost Path as Polyline tool to account for realistic paths around land. Coastal marsh, emergent marsh, and oyster beds present in the area were not analyzed due to their inaccessibility to dolphins in the study area. Shapefile data from the CCSC-Aransas Pass confluence area were obtained from NOAA, Texas Parks and Wildlife Department, and Texas Department of Transportation online repositories.
Multinomial logistic regression models require defined reference groups for each categorical variable (Hua et al., 2021; McNulty, 2022). The travel behavioral state was used as the behavior reference group as it had a large sample size and consisted of uniform movement unlike the other behavioral states. Summer and mid-day were used as temporal reference groups as most collection effort occurred in these periods. Slack tide was used as the tidal reference group as it consisted of the weakest or no currents. Surface depth (0 m) was used as the depth reference group. Collinearity between explanatory variables was assessed with an augmented pairs plot. Explanatory variables were stepwise removed from MLR models based on Akaike Information Criterion (AIC) and models were compared to the fully saturated model (Shukla, 2020). The best-fitting model had the lowest AIC and deviance (Pipis, 2020; Shukla, 2020). A Pearson’s Chi-squared test was used to assess the goodness of fit of the expected results with the observed data in the final MLR model. A Nagelkerke’s Pseudo-R-square test determined how well the model explained the data. Log-likelihood ratio tests used the RStudio “lmtest” package to determine the statistical significance of categorical explanatory variables on behavioral state in the final MLR model. A one-way analysis of variance (ANOVA) followed by a Tukey post-hoc test with Shaffer adjustment using the “multcomp” package in RStudio was performed on significant numerical explanatory variables.
Hot spot analyses of dolphin behavioral states were conducted in ArcGIS Pro to determine spatial patterns and habitat-specific behavioral states. Optimized hot spot analysis uses the Getis-Ord Gi* statistic to calculate statistically significant areas where features with high values are surrounded by other high values (hot spots identified by low p-values and high z-scores) or low values surrounded by other low values (cold spots identified by low p-values and low z-scores). The False Discovery Rate (FDR) correction method was applied to correct for spatial dependence and multiple testing when calculating confidence intervals. Behavioral states with a minimum of 70 observations were used in the hot spot analyses (ESRI, n.d.). Kernel density analyses were used in ArcGIS Pro when behavioral states were observed less than 70 times (Koper et al., 2015). The kernel density analyses calculated the number of sightings of each behavioral state per square kilometer with a manually set search radius of 150 m and an output raster cell size of 15 m.
3 Results
Data were collected on 63 days, totaling 287.8 hours, from theodolite stations in Port Aransas between June 2021 and September 2022. Data were collected across seasons and time of day, with most effort during summer (Table 2) and at mid-day (Table 3). A total of 1,189 dolphin geographic positions were collected and used in various analyses: 1,120 positions were used in spatial analyses and 898 positions were used in statistical analyses. Dolphin group sizes ranged from 1 – 20 adults (mean = 3.06 ± 2.21) with 0 – 4 calves present (mean = 0.08 ± 0.33), however, it was not possible to individually identify dolphins observed from elevated theodolite stations. As theodolite scanning did not allow for identification of individuals, it is possible that the same dolphin occurred in more than one group. The predominant behavioral state of dolphin groups was foraging (n = 516 positions, 46% of observations) followed by traveling (n = 277 positions, 25% of observations), milling (n = 244 positions, 22% of observations), socializing (n = 70 positions, 6% of observations), and resting (n = 13 positions, 1% of observations).
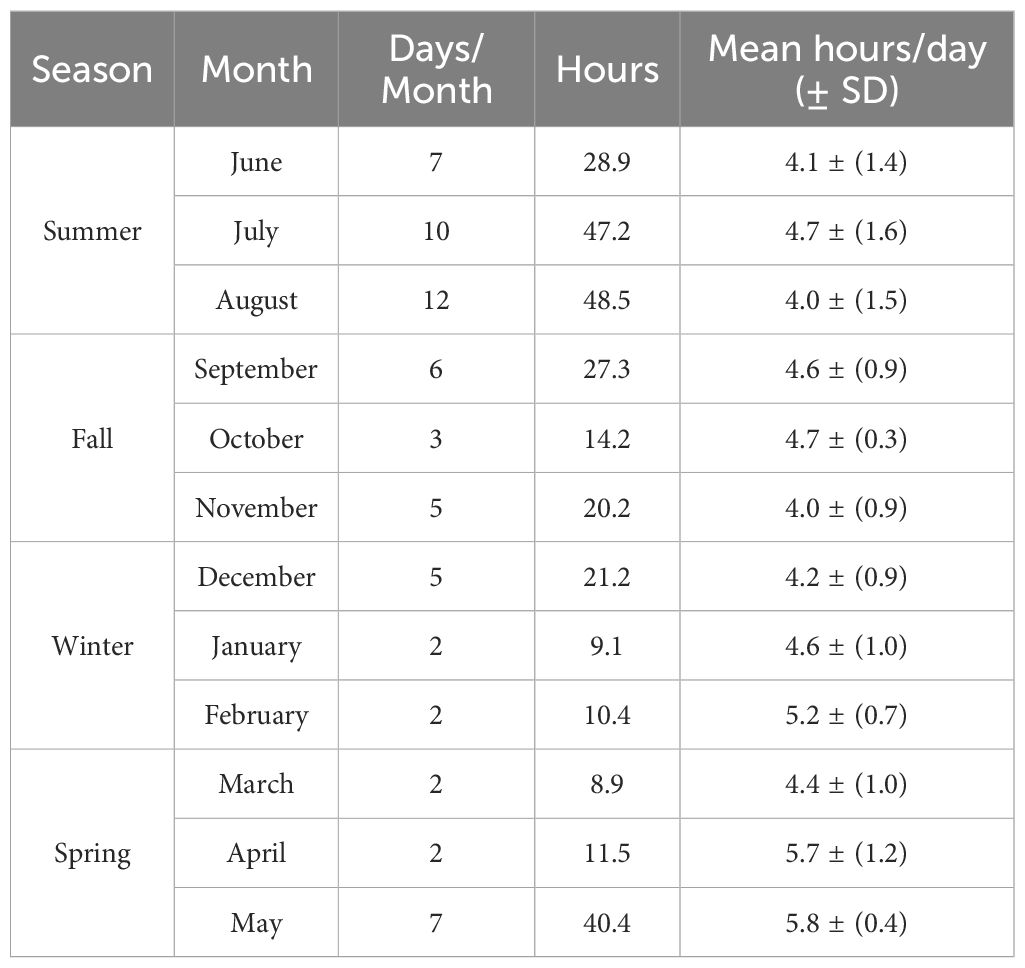
Table 2 Days (n = 63) and hours (n = 287.8) of observation of common bottlenose dolphins in the CCSC-Aransas Pass, Texas area per month from June 2021 to September 2022.
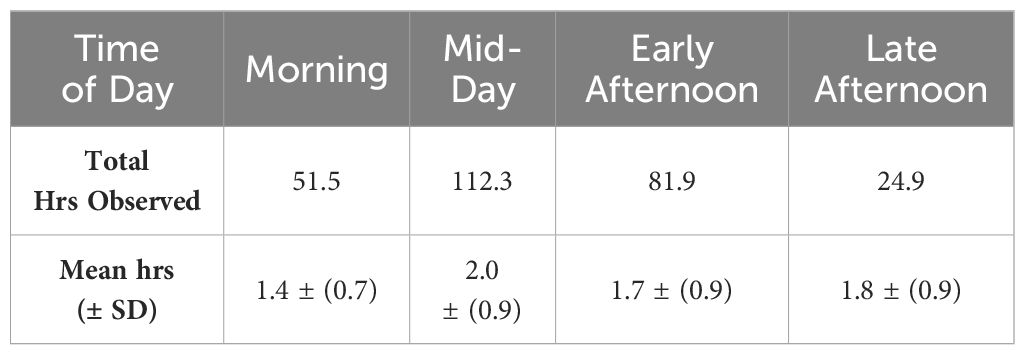
Table 3 Number of hours (n = 287.8) of observations of common bottlenose dolphins per time period in the CCSC-Aransas Pass, Texas area from June 2021 to September 2022.
The fully saturated MLR model overpredicted the three most common behavioral states (forage, travel, mill) and masked the least common behavioral sates (social, rest). The final MLR model included the three most common behavioral states and excluded the least common behavioral states (Table 4). Tidal state, current speed, water depth, shortest distance to mangroves, and shortest distance to seagrass beds were excluded from the final model based on AIC values. The best fitting model for behavioral state (n = 834 positions, AIC = 1,711.744, deviance = 1,679.744) included the significant explanatory variables of season, time of day, and shortest distance to shoreline (X22 = 19.00, p < 0.001; Table 5). The Nagelkerke pseudo-R-square indicated a weak goodness of fit (9%) between explanatory variables and behavioral states. Weak model fit might suggest that other factors, such as biotic variables like group size, may be important contributors to dolphin behavior and should be addressed in future studies. Frequencies of behavioral state occurrences indicate statistical trends of occurrences expected by chance.
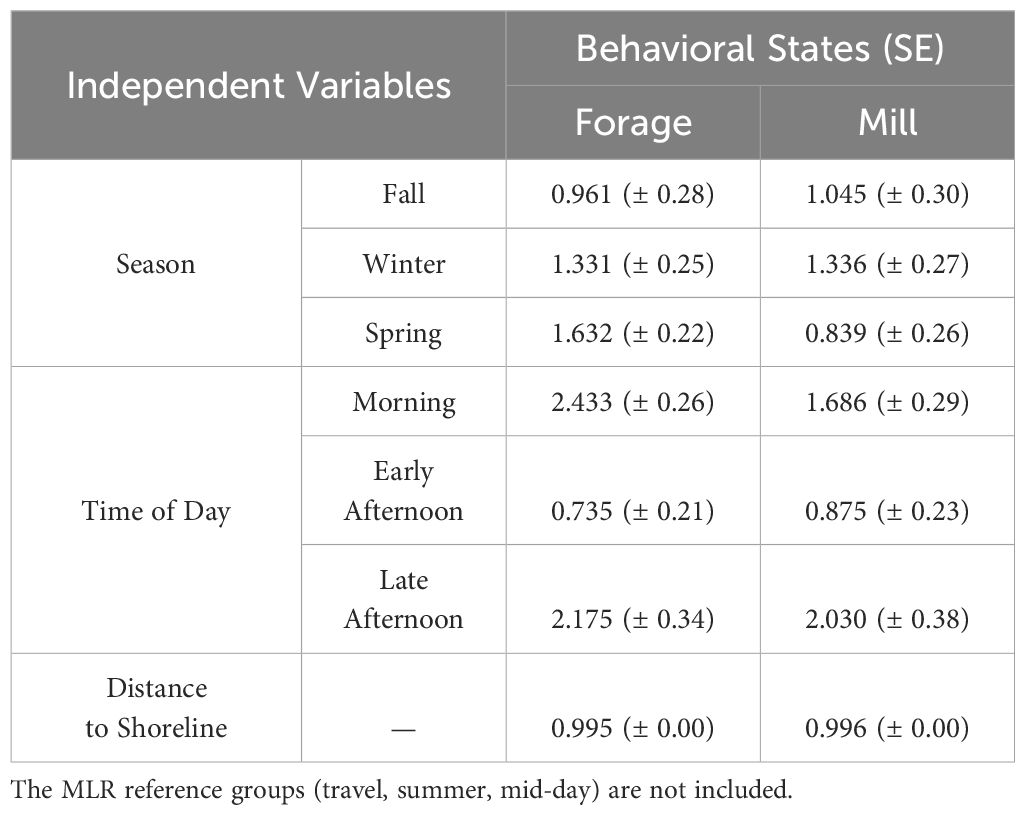
Table 4 Exponentiated coefficients (± SE) of the final multinomial logistic regression (MLR) assessing relationship between common bottlenose dolphin behavioral states (forage, travel, mill) and significant explanatory variables (n = 834).
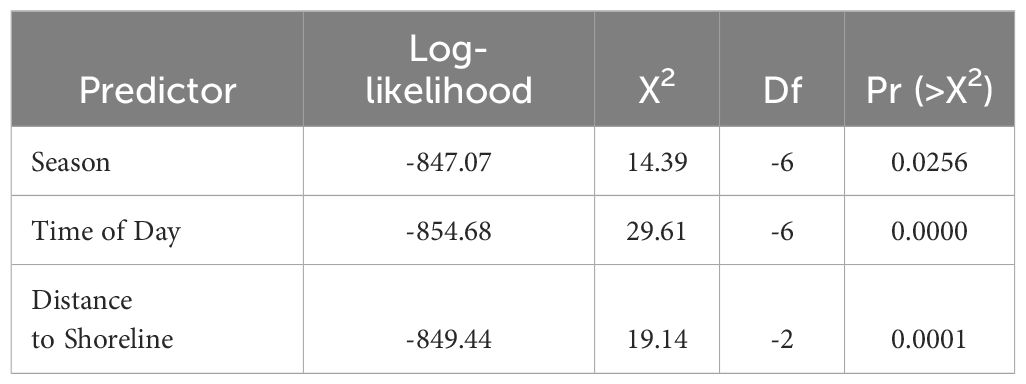
Table 5 Log-likelihood ratios (a = 0.05) of significant explanatory variables included in the best fitting final MLR model (n = 834).
3.1 Environmental patterns
The behavioral states of bottlenose dolphins were significantly related to season (X26 = 14.39, p < 0.05; Table 5). Behavioral states were also significantly related to time of day (X26 = 29.61, p < 0.001; Table 5). Foraging was frequently observed during spring and winter months in the morning and late afternoon, and less frequently observed during the summer and fall in the early afternoon (Figures 2, 3; Supplementary Tables S1, S2). Dolphins frequently traveled during the summer and fall in the early afternoon and infrequently during the spring and winter months in the morning (Figures 2, 3; Supplementary Tables S1, S2). Dolphins milled frequently during the summer and winter in the late afternoon and infrequently during the spring. Season and time of day were weak explanatory variables for milling as observed values were close to predicted values (Figures 2, 3; Supplementary Tables S1, S2).
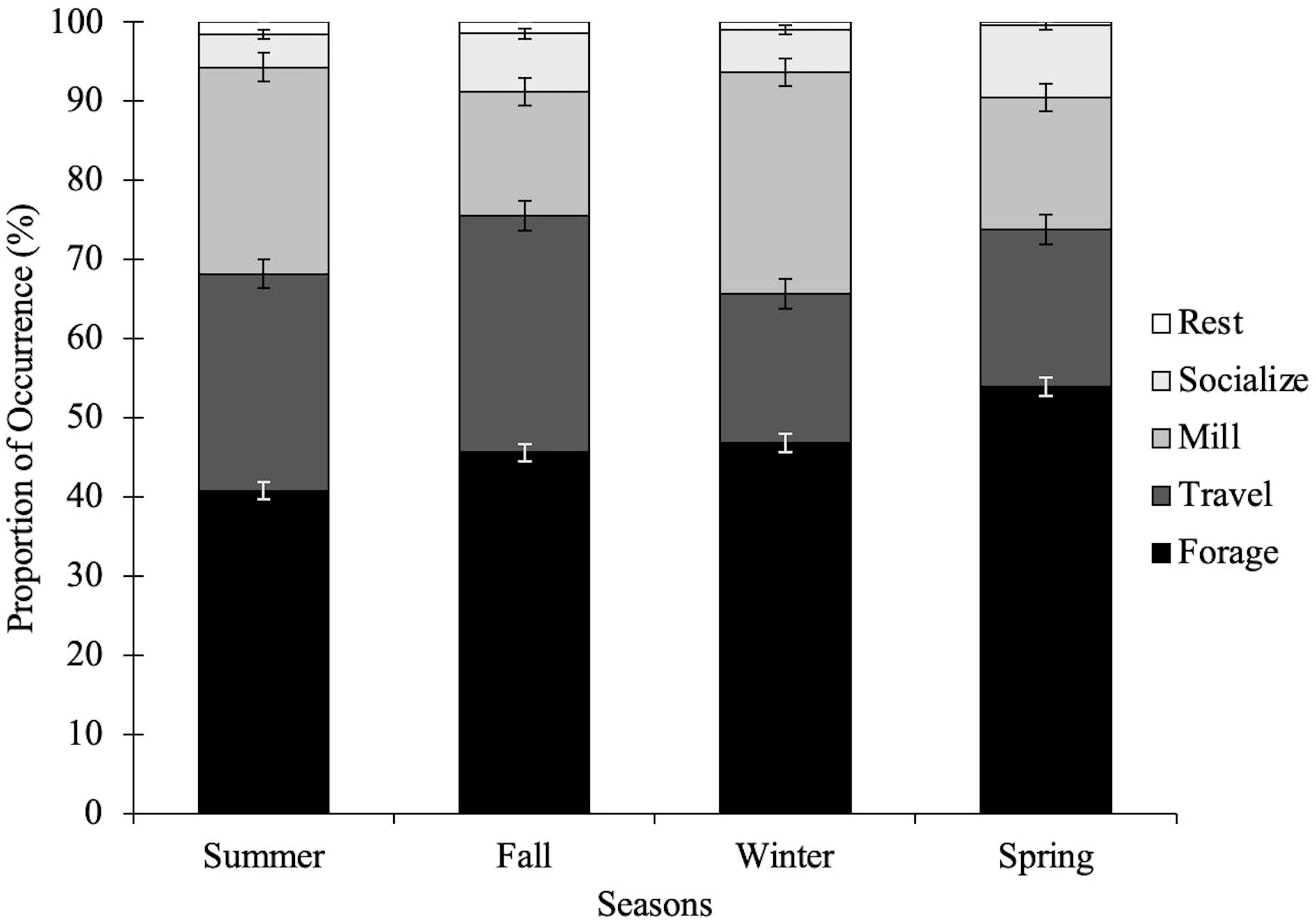
Figure 2 Proportion of behavioral state occurrences of common bottlenose dolphins in the CCSC-Aransas Pass, Texas area from June 2021 to September 2022 based on season. Summer (June-August, n = 387), Fall (September-November, n = 274), Winter (December-February, n = 207), Spring (March-May, n = 252).
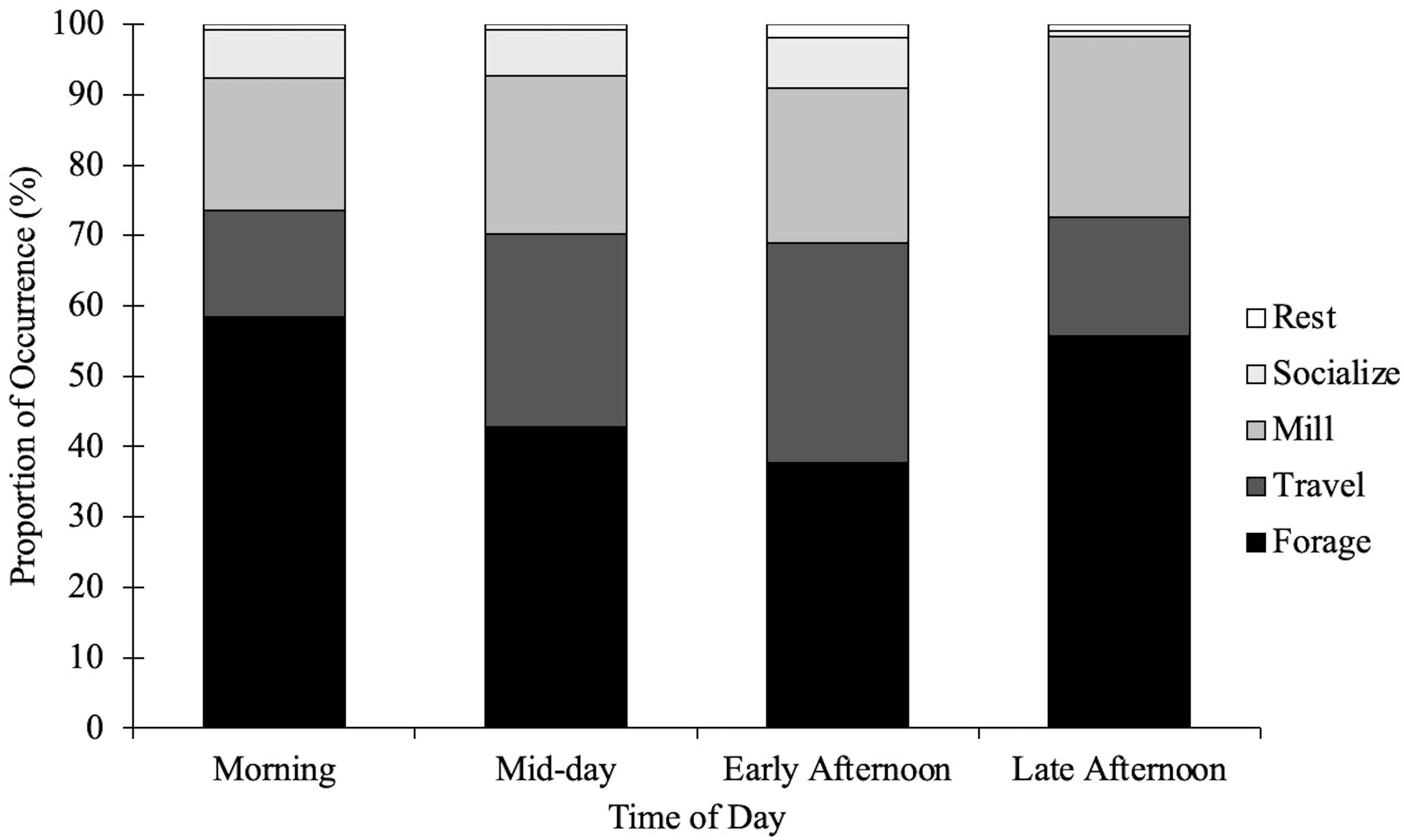
Figure 3 Proportion of behavioral state occurrences of common bottlenose dolphins in the CCSC-Aransas Pass, Texas area from June 2021 to September 2022 based on time of day. Morning (0800–1059 hrs, n = 264), Mid-day (1100–1359 hrs, n = 369), Early Afternoon (1400–1659, n = 374), Late Afternoon (1700–2000 hrs, n = 113).
3.2 Geographic patterns
On average, bottlenose dolphins engaged in all behavioral states closer to the shoreline than to mangroves and seagrass beds (Table 6). The distance of bottlenose dolphins to the shoreline was a significant predictor of behavioral state in the MLR model (X22 = 19.14, p < 0.001; Table 5). Specific behavioral states occurred at significantly different distances from the shoreline (F(2, 831) = 11.23, p < 0.001), with foraging (t = -4.69, p < 0.001) and milling (t = -3.28, p = 0.001) occurring significantly closer to the shoreline than traveling. When bottlenose dolphins foraged and milled, there was no significant difference in the distance to the shoreline (t = 0.968, p > 0.05).
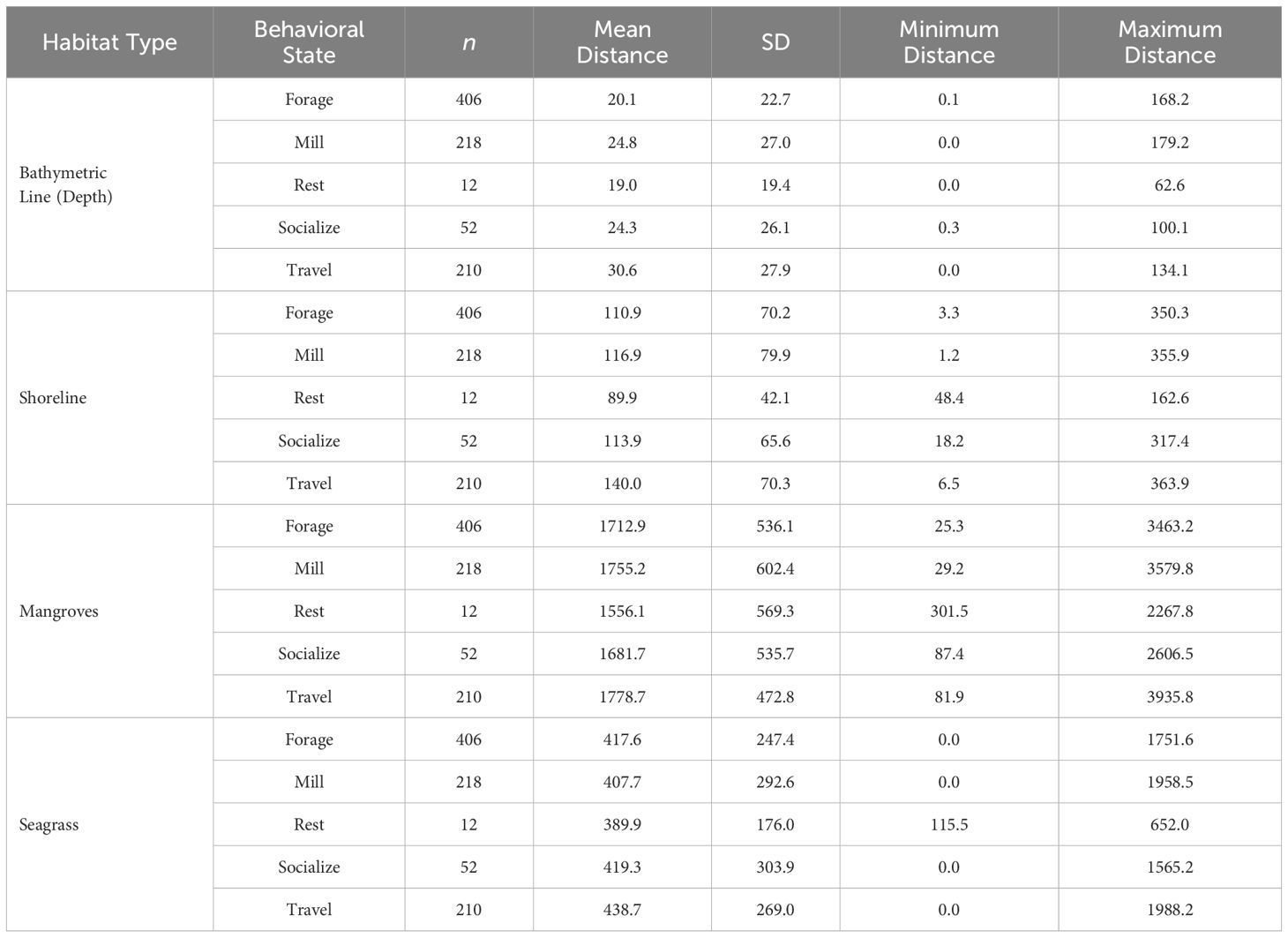
Table 6 Descriptive statistics for the distances (m) to the closest bathymetric line (m) and habitat type for common bottlenose dolphins in each behavioral state in the CCSC-Aransas Pass, Texas area from June 2021 to September 2022 (n = 898).
Foraging occurred throughout the study area with hot spots along the seawall of the CCSC under Site 1 and near the ferry crossing in the middle of the CCSC (Figure 4A). No foraging cold spots were identified (Supplementary Table S3). Traveling occurred throughout the study area with hot spots across the confluence area of the AC, LAC, and CCSC, as well as at the entrance to the Port Aransas boat harbor (Figure 4B). Traveling cold spots were in the middle of the CCSC toward the mouth of the Aransas Pass jetty along San José Island and in the middle of the LAC (Figure 4B; Supplementary Table S3). Milling occurred throughout the study area with a hot spot across the confluence area of the AC, LAC, and CCSC and extending into the Port Aransas harbor (Figure 4C; Supplementary Table S3). There was no cold spot for milling (Figure 4C; Supplementary Table S3). The densest occurrences of socializing per kilometer were at the periphery of the CCSC near Site 1 and off the Harbor Island point in the middle of the channel confluence area (Figure 5A). Resting had the densest occurrence per kilometer particularly along Harbor Island, along the seawall near Site 1, and along the periphery of the CCSC in Aransas Pass near the opening of the AC (Figure 5B).
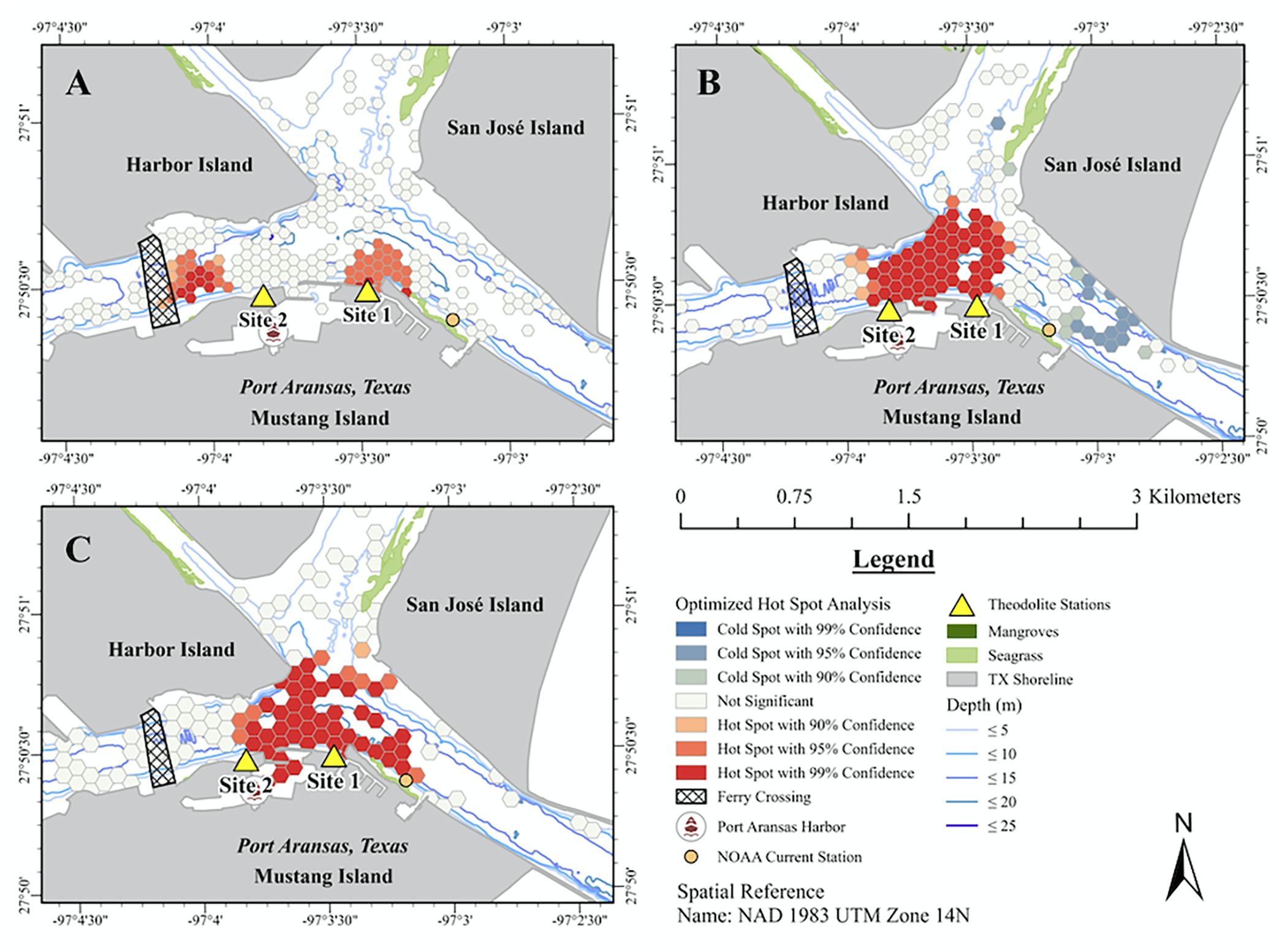
Figure 4 Hot spot analyses of common bottlenose dolphin behavioral states in the CCSC-Aransas Pass, Texas area between June 2021 to September 2022. (A) foraging, n = 516; (B) traveling, n = 277; (C) milling, n = 244.
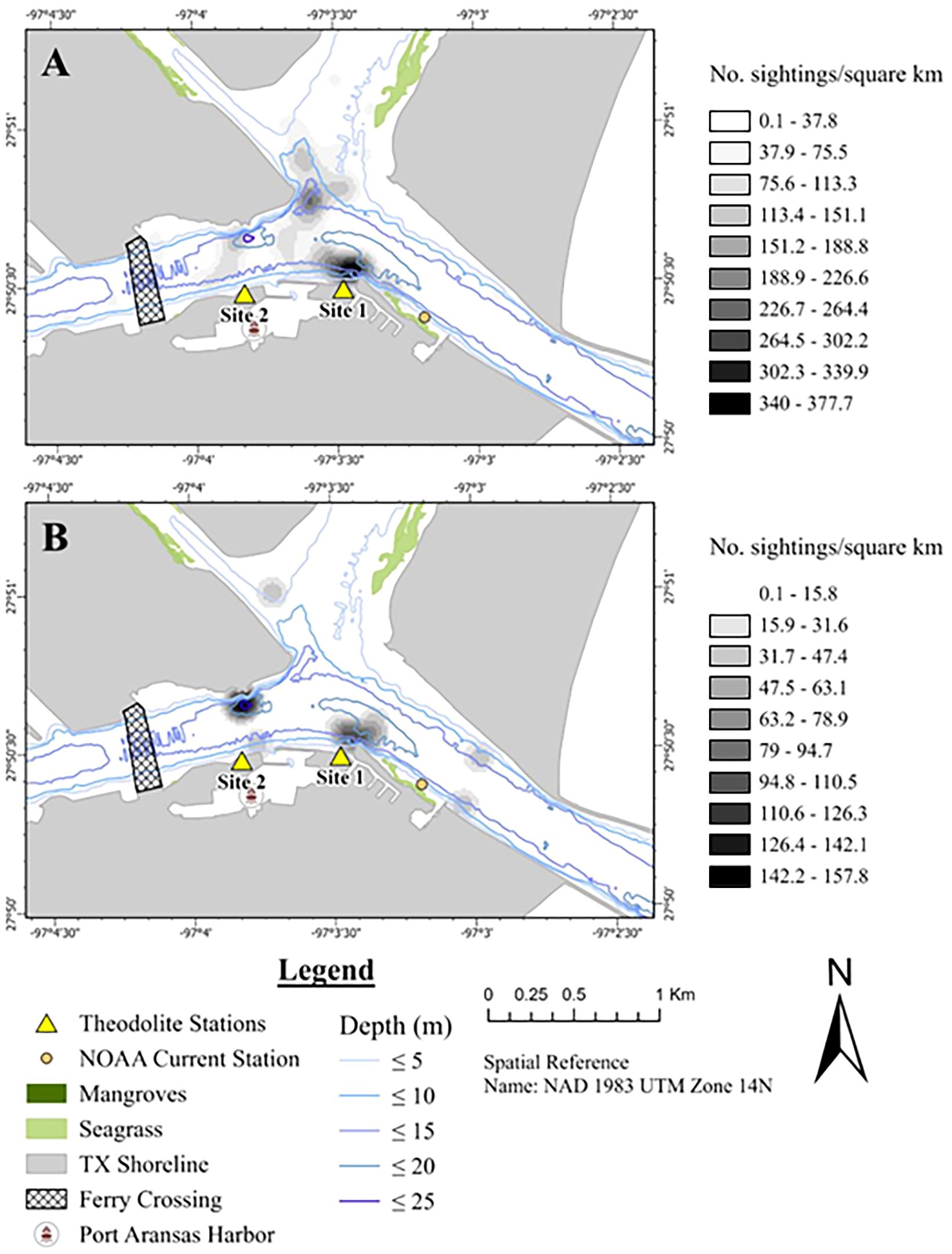
Figure 5 Kernel density map of common bottlenose dolphin behavioral states in the CCSC-Aransas Pass, Texas area between June 2021 to September 2022. (A) socializing, n = 70; (B) resting, n = 13. Density analysis represents the number of sightings per square kilometer with radius of 150 m and an output raster cell size of 15 m.
4 Discussion
The behavioral states of dolphins varied with environmental and geographic parameters in a highly industrialized active seaport. The CCSC-Aransas Pass confluence area was predominately used by bottlenose dolphins to forage, travel, and mill and appears to be a foraging hot spot (46% of observations) that is rarely used for resting during daylight hours (1% of observations). The CCSC-Aransas Pass confluence area seems to serve as a natural bottleneck for prey species moving between the Gulf of Mexico and Corpus Christi Bay (Stokes, 1977; Gilbert, 1986; Bushon, 2006; Payne, 2011), which may attract and sustain dolphins despite overlap with extensive anthropogenic threats. Bottlenose dolphins along the Texas coast favor dredged channels and passes (Shane, 1977; Leatherwood and Reeves, 1983; Henderson and Würsig, 2007; Ronje et al., 2020; Mills et al., 2023), which provide a three-dimensional space to dive and avoid vessel activity at the surface. High vessel traffic and potential predation from sharks may contribute to the limited occurrence of dolphins resting in the CCSC-Aransas Pass confluence area. Dolphins may reduce resting behavior and remain active to avoid predators and protect their calves, as large, migratory sharks (e.g., tiger shark: Galeocerdo cuvier; scalloped hammerhead: Sphyrna lewini; bigeye thresher: Alopias superciliosus; bull shark: Carcharhinus leucas) have been found to move between offshore waters and inshore nursery areas through channels like Aransas Pass along the Gulf of Mexico (Froeschke et al., 2010; Gibson et al., 2019; Ajemian et al., 2020; Anderson et al., 2022). Reduced resting may result in energy depletion with adverse potential effects on dolphins (Lusseau, 2003; Visser et al., 2011; Bas et al., 2015). However, research efforts in nearby bays may provide insights into dolphin resting hotspots and facilitate evaluation of the sustainability of bottlenose dolphins in the area.
The high occurrences of dolphin foraging in the morning and late afternoons in the spring but not the summer are consistent with other findings in Texas waters (Henderson and Würsig, 2007; Piwetz, 2019). Dolphins may mill between bouts of foraging, as milling also occurred frequently in the late afternoon, although our data indicate that milling patterns do not seem to be driven by temporal variables as time of day was a weak explanatory variable for milling patterns. Temporal patterns of foraging align with the spawning and migration of prey species in the CCSC; common prey of bottlenose dolphins in the region include Southern flounder (Paralichthys lethostigma) and Gulf flounder (P. albigutta) that spawn in the Gulf of Mexico from September to April and return to bays during January and February (Stokes, 1977; Gilbert, 1986; Bushon, 2006; Payne, 2011). Flounder movement through the CCSC peak between November and February (Stokes, 1977). As dolphins traveled most during the summer and fall when flounder abundance is historically low in the CCSC (Stokes, 1977; Bushon, 2006; Nañez-James et al., 2009), dolphins may be transiting to other areas for foraging opportunities; bottlenose dolphin abundance in the CCSC-Aransas Pass confluence area is historically low during the summer (Shane, 1980). Early afternoon traveling has also been observed in a different management stock of bottlenose dolphins along the Texas coast (Piwetz, 2019). Dolphins in the CCSC-Aransas Pass confluence area appear to transition from traveling in the early afternoon to foraging during late afternoon as previously observed (Shane, 1977), potentially reflecting understudied temporal patterns of prey abundance in the area.
Hard shoreline structures appear to influence dolphin behavioral states as foraging, traveling, and milling occurred closer to human-made shorelines (e.g., seawalls, granite blocks) than natural habitats (e.g., mangroves, seagrass beds), the latter of which had little influence on dolphin behavioral states. Dolphins may use human-made physical structures as barriers to herd and trap prey, which is supported by visual observations of dolphins chasing fish along seawalls during the course of this research (Rossbach, 1999; Torres and Read, 2009; Cantor et al., 2018). Dolphin preferences for foraging in dredged channels may reflect increased prey abundance in channels and passes (Shane, 1977; Leatherwood and Reeves, 1983; Shane et al., 1986; Allen et al., 2001; Félix et al., 2022). Flounder abundance was historically high in Aransas Pass with no vegetation along the shoreline (Stokes, 1977). As dolphins traveled farther from the shoreline compared to when foraging or milling, dolphins may transit across channels to utilize hard structures close to the shoreline for prey capture. Dolphin foraging in the CCSC-Aransas Pass confluence overlapped extensively with areas of recreational fishing. Dolphins may utilize habitats regardless of marine noise and high vessel activity due to prey availability (Piwetz et al., 2021). Pile driving, welding, and crane operation occurred along some hard shorelines during the study period, likely producing amplified underwater noise known to disrupt the communication, behavior, and distribution of some populations of bottlenose dolphins (Todd et al., 2015; Piwetz et al., 2021). Bottlenose dolphins also foraged frequently near the local ferry crossing, congruent with findings for a different management stock of bottlenose dolphins in Texas (Rivard et al., 2016). The mixing of water and nutrients from ferry and vessel operations may increase prey abundance.
Traveling and milling hot spots overlapped with areas of frequent vessel traffic in the CCSC-Aransas Pass confluence area and at the entrance of the Port Aransas boat harbor. Dolphins may use the confluence area to travel from deep channel environments to shallower vegetated habitats connected to Aransas-Copano Bay where Southern flounder densities are high (Nañez-James et al., 2009). Although dredged channels connecting bays to the Gulf of Mexico may serve as travel corridors for bottlenose dolphins in Texas waters (Henderson and Würsig, 2007), they are not consistently used for this function (Piwetz, 2019) and were a cold spot for dolphin travel in this study. Dolphins may mill in the CCSC-Aransas Pass confluence area when prey abundance is low or when other behaviors cease, similar to findings in San Luis Pass, Texas (Henderson and Würsig, 2007). Bottlenose dolphins traveled and milled in the entrance of the Port Aransas boat harbor recurrently. As vessels frequent the harbor, there is an increased collision risk to milling dolphins.
Bottlenose dolphins socialized and rested along hard shoreline structures that experience strong surface currents which vary with tidal state. Most socializing and resting occurred at the peripheral confluence of channels, where dolphin groups may aggregate before transiting; these peripheral areas had low vessel traffic and reduced likelihood of vessel strikes compared to the middle of the channels. As vessel traffic, especially from dolphin-watching ecotours, can interrupt dolphin resting in coastal areas, dolphins may selectively engage in vulnerable behavioral states in areas of low vessel traffic (Lusseau, 2004; Arcangeli and Crosti, 2009; Bas et al., 2015). Research is warranted to explore the interactions between and effects of anthropogenic disturbances on bottlenose dolphins in the highly industrialized CCSC-Aransas Pass confluence area (e.g., stress levels, acoustic masking, reproductive success) to determine if dolphins are habituated or desensitized to anthropogenic activity. Dolphins may have varying tolerance levels to vessel traffic (Bejder et al., 2009), as bottlenose dolphins rested infrequently in the CCSC-Aransas Pass confluence area. The costs of tolerating anthropogenic disturbances may be lower than the energetic efforts to relocate (Bas et al., 2015). Continued monitoring of long-term impacts of port growth and industry development on bottlenose dolphins in the area is strongly encouraged for species conservation management. Updated data on fish movement and seasonal abundance patterns may provide further insight into why dolphins remain in an area of such high risk.
5 Conclusions
This study provides insight into the influences of environmental and geographic factors on dolphin daily activities in one of the busiest vessel ports in the United States. The CCSC-Aransas Pass confluence area remains a key foraging hot spot for bottlenose dolphins despite anthropogenic threats, suggesting abundant prey particularly along hard-structured seawalls where dolphins foraged in close proximity to fishing vessels. Marine megafauna inhabiting coastal environments often overlap with anthropogenic operations in industrialized areas (Chilvers et al., 2005; Bain et al., 2006; Pirotta et al., 2013; Todd et al., 2015; Marley et al., 2017; Cloyed et al., 2019; Piwetz, 2019; Mills et al., 2023). Increased port growth and vessel operations in coastal areas may pose threats to marine species and the habitats they occupy. As marine megafauna remain in industrialized areas, interactions between species and anthropogenic activities (e.g., tourism, recreational boating, commercial shipping and fishing, marine construction) may increase and can cause behavioral disruptions, shifts in habitat use, and collisions resulting in injury or death (Bejder et al., 2006; Culloch et al., 2016; Cloyed et al., 2019; Piwetz, 2019). Knowledge of the temporal and spatial patterns of marine megafauna relative to various behavioral states can aid in informing management decisions, providing proper operating procedures around marine species, and ultimately species protection.
Data availability statement
The raw data supporting the conclusions of this article will be made available by the authors, without undue reservation.
Ethics statement
The requirement of ethical approval was waived by Texas A&M University- Corpus Christi IACUC because it was a non-invasive shore-based observational study. The studies were conducted in accordance with the local legislation and institutional requirements.
Author contributions
EM: Conceptualization, Formal analysis, Funding acquisition, Investigation, Methodology, Writing – original draft, Writing – review & editing. SP: Formal analysis, Methodology, Supervision, Writing – review & editing. DO: Conceptualization, Funding acquisition, Supervision, Writing – review & editing.
Funding
The author(s) declare financial support was received for the research, authorship, and/or publication of this article. Funding for this study was provided by Texas Parks & Wildlife (State Wildlife Grant, CA-0000891), Texas A&M University-Corpus Christi (Center for Coastal Studies-Hans and Pat Suter Endowment Scholarship), the Southwestern Association of Naturalists (Howard McCarley Student Research Award), and the Texas Sea Grant College Program (Institutional Grant NA18OAR4170088) from the National Sea Grant Office, National Oceanic and Atmospheric Administration, U.S.A. Department of Commerce.
Acknowledgments
We thank all field data collection assistants: Thaddeus Mills, Jacquline Rich, Allison Wilkins, Peter Marra, Audra Clute, Makayla Guinn, Jennifer Gilmore, Tina Shield, Samantha Huron, Brianna Hurst, Madeleine Deel, Caitlynn Partin, Emily Cano, Austin Willson, Anya Ocampos, Kayla Torres. We thank Dave Steckler for setting up and complementary use of Mysticetus software, Roy Roberts and Blair Sterba-Boatwright for statistical assistance, and Shawn McCracken for spatial analysis advice and manuscript edits. We are grateful to the manager and staff at field station one. We also acknowledge NOAA Benthic Atlas, NOAA Digital Coast, Texas Parks & Wildlife, and Texas Department of Transportation for the availability of benthic habitats, the Texas shoreline, and bathymetry shapefile data used in GIS analysis.
Conflict of interest
The authors declare that the research was conducted in the absence of any commercial or financial relationships that could be construed as a potential conflict of interest.
Publisher’s note
All claims expressed in this article are solely those of the authors and do not necessarily represent those of their affiliated organizations, or those of the publisher, the editors and the reviewers. Any product that may be evaluated in this article, or claim that may be made by its manufacturer, is not guaranteed or endorsed by the publisher.
Supplementary material
The Supplementary Material for this article can be found online at: https://www.frontiersin.org/articles/10.3389/fmars.2024.1334252/full#supplementary-material
References
Ajemian M. J., Drymon J. M., Hammerschlag N., Wells R. J. D., Street G., Falterman B., et al. (2020). Movement patterns and habitat use of tiger sharks (Galeocerdo cuvier) across ontogeny in the Gulf of Mexico. PloS One 15, 1–24. doi: 10.1371/journal.pone.0234868
Allen M., Read A., Gaudet J., Sayigh L. (2001). Fine-scale habitat selection of foraging bottlenose dolphins Tursiops truncatus near Clearwater, Florida. Mar. Ecol. Prog. Ser. 222, 253–264. doi: 10.3354/meps222253
Anderson T., Meese E. N., Drymon J. M., Stunz G. W., Falterman B., Menjivar E., et al. (2022). Diel vertical habitat use observations of a scalloped hammerhead and a bigeye thresher in the Northern Gulf of Mexico. Fishes 7, 148. doi: 10.3390/fishes7040148
Arcangeli A., Crosti R. (2009). The short-term impact of dolphin-watching on the behavior of bottlenose dolphins (Tursiops truncatus) in western Australia. J. Mar. Anim. Their Ecol. 2, 3–9.
Azzellino A., Gaspari S., Airoldi S., Nani B. (2008). Habitat use and preferences of cetaceans along the continental slope and the adjacent pelagic waters in the western Ligurian Sea. Deep-Sea Res. Part I-Oceanographic Res. Papers 55, 296–323. doi: 10.1016/j.dsr.2007.11.006
Bain D. E., Williams R., Smith J. C., Lusseau D. (2006). Effects of vessels on behavior of Southern Resident killer whales (Orcinus spp.) 2003–2005. NMFS Contract AB133F05SE3965. (Available from D. E. Bain, Friday Harbor Laboratories, Univ. Washington, 620 University Road, Friday Harbor, WA 98250.).
Baker I., O’Brien J., McHugh K., Berrow S. (2017). An ethogram for bottlenose dolphins (Tursiops truncatus) in the Shannon Estuary, Ireland. Aquat. Mammals 43, 594–613. doi: 10.1578/AM.43.6.2017.594
Bas A. A., Öztürk A. A., Öztürk B. (2015). Selection of critical habitats for bottlenose dolphins (Tursiops truncatus) based on behavioral data, in relation to marine traffic in the Istanbul Strait, Turkey. Mar. Mammal Sci. 31, 979–997. doi: 10.1111/mms.12202
Bejder L., Samuels A., Whitehead H., Finn H., Allen S. (2009). Impact assessment research: use and misuse of habituation, sensitization, and tolerance in describing wildlife responses to anthropogenic stimuli. Mar. Ecol. Prog. Ser. 395, 177–185. doi: 10.3354/meps07979
Bejder L., Samuels A., Whitehead H., Gales N., Mann J., Connor R., et al. (2006). Decline in relative abundance of bottlenose dolphins exposed to long-term disturbance. Conserv. Biol. 20, 1791–1798. doi: 10.1111/j.1523-1739.2006.00540.x
Brown D. M., Sieswerda P. L., Parsons E. C. M. (2019). Potential encounters between humpback whales (Megaptera novaeangliae) and vessels in the New York bight apex, USA. Mar. Policy 106, 103527. doi: 10.1016/j.marpol.2019.103527
Bushon A. (2006). Recruitment, spatial distribution, and fine-scale movement patterns of estuarine dependent species through tidal inlets in Texas. (Corpus Christi, Texas: Doctoral dissertation, Texas A&M University-Corpus Christi).
Caldwell M. (2016). Historical evidence of Tursiops truncatus exhibiting habitat preference and seasonal fidelity in Northeast Florida. Aquat. Mammals 42, 89–103. doi: 10.1578/AM.42.1.2016.89
Cantor M., Simoães-Lopes P. C., Daura-Jorge F. G. (2018). Spatial consequences for dolphins specialized in foraging with fishermen. Anim. Behav. 139, 19–27. doi: 10.1016/j.anbehav.2018.03.002
Chilvers B. L., Lawler I. R., Macknight F., Marsh H., Noad M., Paterson R. (2005). Moreton Bay, Queensland, Australia: An example of the co-existence of significant marine mammal populations and large-scale coastal development. Biol. Conserv. 122, 559–571. doi: 10.1016/j.biocon.2004.08.013
Cloyed C. S., Hieb E. E., Collins M. K., DaCosta K. P., Carmichael R. H. (2019). Linking use of ship channels by West Indian Manatees (Trichechus manatus) to seasonal migration and habitat use. Front. Mar. Sci. 6. doi: 10.3389/fmars.2019.00318
Cloyed C. S., Hieb E. E., DaCosta K. P., Ross M., Carmichael R. H. (2022). Habitat selection and abundance of West Indian manatees Trichechus manatus at the margins of their expanding range. Mar. Ecol. Prog. Ser. 696, 151–167. doi: 10.3354/meps14116
Connor R. C., Sakai M., Morisaka T., Allen S. J. (2019). “The Indo-Pacific bottlenose dolphin (Tursiops aduncus),” in Ethology and behavioral ecology of odontocetes. Ed. Würsig B. (Springer Nature, Switzerland), 345–368. doi: 10.1007/978-3-030-16663-2_16
Culloch R. M., Anderwald P., Brandecker A., Haberlin D., McGovern B., Pinfield R., et al. (2016). Effect of construction-related activities and vessel traffic on marine mammals. Mar. Ecol. Prog. Ser. 549, 231–242. doi: 10.3354/meps11686
Di Sciara G. N., Hoyt E., Reeves R., Ardron J., Marsh H., Vongraven D., et al. (2016). Place-based approaches to marine mammal conservation: Place-based approaches to marine mammal conservation. Aquat. Conservation: Mar. Freshw. Ecosyst. 26, 85–100. doi: 10.1002/aqc.2642
ESRI- Environmental Systems Research Institute, Inc Optimized hot spot analysis (Spatial statistics). Available online at: https://pro.arcgis.com/en/pro-app/latest/tool-reference/spatial-statistics/optimized-hot-spot-analysis.htm.
Félix F., Fernández J. E., Paladines A., Centeno R., Romero J., Burneo S. F. (2022). Habitat use of the common bottlenose dolphin (Tursiops truncatus) in the Gulf of Guayaquil, Ecuador: Management needs for a threatened population. Ocean Coast. Manage. 223, 1–12. doi: 10.1016/j.ocecoaman.2022.106174
Froeschke J. T., Stunz G. W., Sterba-Boatwright B., Wildhaber M. L. (2010). An empirical test of the ‘shark nursery area concept’ in Texas bays using a long-term fisheries-independent data set. Aquat. Biol. 11, 65–76. doi: 10.3354/ab00290
Gibson K. J., Streich M. K., Topping T. S., Stunz G. W. (2019). Utility of citizen science data: A case study in land-based shark fishing. PloS One 14, e0226782. doi: 10.1371/journal.pone.0226782
Gilbert C. R. (1986). Species profiles: Life histories and environmental requirements of coastal fishes and invertebrates (South Florida)-southern, gulf, and summer flounders (Gainesville, Florida: U.S. Army Corps of Engineers).
Gubbins C. (2002). Use of home ranges by resident bottlenose dolphins (Tursiops truncatus) in a South Carolina estuary. J. Mammalogy 83, 178–187. doi: 10.1644/1545-1542(2002)083<0178:UOHRBR>2.0.CO;2
Harzen S. E. (2002). Use of an electronic theodolite in the study of movement of the bottlenose dolphin (Tursiops truncatus) in the Sado Estuary, Portugal. Aquat. Mammals 28, 251–260.
Hastie G. D., Wilson B., Wilson L. J., Parsons K. M., Thompson P. M. (2004). Functional mechanisms underlying cetacean distribution patterns: Hotspots for bottlenose dolphins are linked to foraging. Mar. Biol. 144, 397–403. doi: 10.1007/s00227-003-1195-4
Heithaus M. R. (2001). Predator–prey and competitive interactions between sharks (order Selachii) and dolphins (suborder Odontoceti): A review. J. Zoology 253, 53–68. doi: 10.1017/S0952836901000061
Heithaus M. R., Dill L. M. (2002). Food availability and tiger shark predation risk influence bottlenose dolphin habitat use. Ecology 83, 480–491. doi: 10.1890/0012-9658(2002)083[0480:FAATSP]2.0.CO;2
Henderson E. E., Würsig B. (2007). Behavior patterns of bottlenose dolphins in San Luis Pass, Texas. Gulf Mexico Sci. 25, 153–161. doi: 10.18785/goms.2502.06
Hua C., Choi Y., Shi. Q. (2021). Companion to BER 643: Advanced regression methods (Bookdown). Available at: https://bookdown.org/chua/ber642_advanced_regression/multinomial-logistic-regression.html.
Ingram S., Rogan E. (2002). Identifying critical areas and habitat preferences of bottlenose dolphins Tursiops truncatus. Mar. Ecol. Prog. Ser. 244, 247–255. doi: 10.3354/meps244247
Karczmarski L., Würsig B., Gailey G., Larson K. W., Vanderlip C. (2005). Spinner dolphins in a remote Hawaiian atoll: Social grouping and population structure. Behav. Ecol. 16, 675–685. doi: 10.1093/beheco/ari028
Koper R. P., Karczmarski L., du Preez D. R., Plön S. (2015). ). Sixteen years later: Occurrence, group size, and habitat use of humpback dolphins (Sousa plumbea) in Algoa Bay, South Africa. Mar. Mammal Sci. 32, 490–507. doi: 10.1111/mms.12279
Kraus S. D., Rolland R. M. (2009). The urban whale: North Atlantic right whales at the crossroads (Cambridge, Massachusetts: Harvard University Press).
Lammers M. O. (2019). “Spinner dolphins of islands and atolls,” in Ethology and behavioral ecology of odontocetes. Ed. Würsig B. (Springer Nature, Switzerland), 369–385. doi: 10.1007/978-3-030-16663-2_17
Leatherwood S., Reeves R. R. (1983). Abundance of bottlenose dolphins in Corpus Christi Bay and coastal Southern Texas. Contributions Mar. Sci. 26, 179–199.
Lusseau D. (2003). Effects of tour boats on the behavior of bottlenose dolphins: Using Markov chains to model anthropogenic impacts. Conserv. Biol. 17, 1785–1793. doi: 10.1111/j.1523-1739.2003.00054.x
Lusseau D. (2004). The hidden cost of tourism: detecting long-term effects of tourism using behavioral information. Ecol. Soc. 9, 1–10. doi: 10.5751/ES-00662-0902r01
Marley S. A., Kent C. P. S., Erbe C. (2017). Occupancy of bottlenose dolphins (Tursiops aduncus) in relation to vessel traffic, dredging, and environmental variables within a highly urbanized estuary. Hydrobiologia 792, 243–263. doi: 10.1007/s10750-016-3061-7
McNulty K. (2022). Handbook of regression modeling in people analytics. (Bookdown). Available at: https://peopleanalytics-regression-book.org/multinomial-logistic-regression-for-nominal-category-outcomes.html.
Mills E. M. M., Piwetz S., Orbach D. N. (2023). Vessels disturb bottlenose dolphin behavior and movement in an active ship channel. Animals 13, 3441. doi: 10.3390/ani13223441
Nañez-James S. E., Stunz G. W., Holt S. A. (2009). Habitat use patterns of newly settled southern flounder (Paralichthys lethostigma) in Aransas-Copano Bay, Texas. Estuaries Coasts 32, 350–359. doi: 10.1007/sl2237-008-9107-y
NOAA National Ocean Service. (2022). “Tides and Currents [Data set],” in Center for operational oceanographic products and services (CO-OPS). Available at: https://tidesandcurrents.noaa.gov/map/index.html.
Payne L. M. (2011). Evaluation of large-scale movement patterns of spotted seatrout (Cynoscion nebulosus) using acoustic telemetry. (Corpus Christi, Texas: Master’s Thesis, Texas A&M University- Corpus Christi, Corpus Christi, Texas).
Pearson H. C. (2019). “Dusky dolphins of continental shelves and deep canyons,” in Ethology and behavioral ecology of odontocetes. Ed. Würsig B. (Springer Nature, Switzerland), 387–411. doi: 10.1007/978-3-030-16663-218
Pipis G. (2020). Contingency tables in R. (R-Bloggers). Available at: https://www.r-bloggers.com/2020/12/contingency-tables-in-r/.
Pirotta E., Laesser B. E., Hardaker A., Riddoch N., Marcoux M., Lusseau D. (2013). Dredging displaces bottlenose dolphins from an urbanized foraging patch. Mar. pollut. Bull. 74, 396–402. doi: 10.1016/j.marpolbul.2013.06.020
Piwetz S. (2019). Common bottlenose dolphin (Tursiops truncatus) behavior in an active narrow seaport. PloS One 14, e0211971. doi: 10.1371/journal.pone.0211971
Piwetz S., Jefferson T. A., Würsig B. (2021). Effects of coastal construction on Indo-Pacific humpback dolphin (Sousa chinensis) behavior and habitat-use off Hong Kong. Front. Mar. Sci. 8. doi: 10.3389/fmars.2021.572535
Rivard A. E., Gelwick F. P., von Zharen W. (2016). Behavioral patterns of common bottlenose dolphins (Tursiops truncatus) within the Galveston-Port Bolivar ferry lane. Southeastern Nat. 15, 742–759. doi: 10.1656/058.015.0418
Ronje E. I., Whitehead H. R., Barry K., Piwetz S., Struve J., Lecours V., et al. (2020). Abundance and occurrence of common bottlenose dolphins (Tursiops truncatus) in three estuaries of the northwestern Gulf of Mexico. Gulf Caribbean Res. 31, 18–34. doi: 10.18785/gcr.3101.09
Rossbach K. A. (1999). Cooperative feeding among bottlenose dolphins (Tursiops truncatus) near Grand Bahamas Island, Bahamas. Aquat. Mammals 25, 163–167.
Sagnol O., Reitsma F., Richter C., Field L. H. (2014). Correcting positional error in shore-based theodolite measurements of animals at sea. J. Mar. Biol. 2014, 1–8. doi: 10.1155/2014/267917
Saj T. L., Sicotte P. (2007). Predicting the competitive regime of female Colobus vellerosus from the distribution of food resources. Int. J. Primatology 28, 315–336. doi: 10.1007/s10764-007-9124-x
Shane S. H. (1977). The population biology of the Atlantic bottlenose dolphin, Tursiops truncatus, in the Aransas Pass area of Texas (Galveston, Texas: Master’s Thesis, Texas A&M University- Galveston).
Shane S. H. (1980). Occurrence, movements, and distribution of bottlenose dolphin, Tursiops truncatus, in southern Texas. Fishery Bull. 78, 593–601.
Shane S. H. (1990). Behavior and ecology of the bottlenose dolphin at Sanibel Island, Florida. In Leatherwood S., Reeves R. R. (Eds.), The Bottlenose Dolphin (San Diego: Academic Press), 245–265
Shane S. H., Wells R. S., Würsig B. (1986). Ecology, behavior and social organization of the bottlenose dolphin: A review. Mar. Mammal Sci. 2, 34–63. doi: 10.1111/j.1748-7692.1986.tb00026.x
Shukla A. (2020). Multinomial logistic regression in R [Data set] (RPubs-RStudio). Available at: https://rpubs.com/Anupam/588952.
Stokes G. M. (1977). Life history studies of Southern flounder (Paralichthys lethostigma) and Gulf flounder (P. albigutta) in the Aransas Bay area of Texas. Texas Parks Wildlife Department 25, 1–36.
Todd V. L. G., Todd I. B., Gardiner J. C., Morrin E. C. N., MacPherson N. A., DiMarzio N. A., et al. (2015). A review of impacts of marine dredging activities on marine mammals. ICES J. Mar. Sci. 72, 328–340. doi: 10.1093/icesjms/fsu187
Torres L. G., Read A. J. (2009). Where to catch a fish? The influence of foraging tactics on the ecology of bottlenose dolphins (Tursiops truncatus) in Florida Bay, Florida. Mar. Mammal Sci. 25, 797–815. doi: 10.1111/j.1748-7692.2009.00297.x
Toth J., Hohn A. A., Able K. W., Gorgone A. M. (2011). Patterns of seasonal occurrence, distribution, and site fidelity of coastal bottlenose dolphins (Tursiops truncatus) in southern New Jersey, U.S.A. Mar. Mammal Sci. 27, 94–110. doi: 10.1111/j.1748-7692.2010.00396.x
U.S. Coast Guard. (2019). Ports and waterways safety assessment: Workshop report Corpus Christi, Texas. U.S (Corpus Christi, Texas: Coast Guard Navigation Center). Available at: https://www.navcen.uscg.gov/pdf/pawsa/WorkshopReports/Corpus_Christi_Sep_2019.pdf.
van Ginkel C., Becker D. M., Gowans S., Simard P. (2018). Whistling in a noisy ocean: Bottlenose dolphins adjust whistle frequencies in response to real-time ambient noise levels. Bioacoustics 27, 391–405. doi: 10.1080/09524622.2017.1359670
Visser F., Hartman K. L., Rood E. J. J., Hendriks A. J. E., Zult D. B., Wolff W. J., et al. (2011). Risso’s dolphins alter daily resting pattern to whale watching at the Azores. Mar. Mammal Sci. 27, 366–381. doi: 10.1111/j.1748-7692.2010.00398.x
Würsig B., Würsig M. (1979). Behavior and ecology of bottlenose dolphin, Tursiops truncatus, in the South Atlantic. Fisheries Bull. U.S. 77, 399–412.
Wells R. S. (2019). “Common bottlenose dolphin foraging: behavioral solutions that incorporate habitat features and social associates,” in Ethology and behavioral ecology of odontocetes. Ed. Würsig B. (Switzerland: Springer Nature), 331–344. doi: 10.1007/978-3-030-16663-215
Keywords: anthropogenic, behavior, bottlenose dolphin, habitat use, hotspot, ship channel, theodolite tracking, Tursiops truncatus
Citation: Mills EMM, Piwetz S and Orbach DN (2024) Behavioral hotspots of bottlenose dolphins in industrialized ship channels. Front. Mar. Sci. 11:1334252. doi: 10.3389/fmars.2024.1334252
Received: 06 November 2023; Accepted: 05 June 2024;
Published: 01 July 2024.
Edited by:
David M. P. Jacoby, Lancaster University, United KingdomReviewed by:
Roberto Carlucci, University of Bari Aldo Moro, ItalyRuth H. Carmichael, Dauphin Island Sea Lab, United States
Copyright © 2024 Mills, Piwetz and Orbach. This is an open-access article distributed under the terms of the Creative Commons Attribution License (CC BY). The use, distribution or reproduction in other forums is permitted, provided the original author(s) and the copyright owner(s) are credited and that the original publication in this journal is cited, in accordance with accepted academic practice. No use, distribution or reproduction is permitted which does not comply with these terms.
*Correspondence: Eliza M. M. Mills, eliza.m.mills@gmail.com