- 1Department of Functional Biology, University of Oviedo, Oviedo, Spain
- 2Centro de Experimentación Pesquera, Dirección General de Pesca Marítima, Consejería de Medio Rural y Cohesión Territorial del Principado de Asturias, Centro Integrado de Formación Profesional del Mar 2a planta, Gijón, Spain
- 3Centro Universitario para la Investigación y el Desarrollo del Agua (CUIDA), Edificio de Investigación del Campus de Mieres, University of Oviedo, Mieres, Spain
Introductio: Sea cucumbers are increasingly demanded as seafood in different cultures around the world. Holothurians could be potentially exploited in southern Bay of Biscay (Iberian Cantabrian coast) where they are not fished yet. Recent studies show relatively high pollution by emerging pollutants like microplastics (MPs) in the region; perhaps the exposure of holothurians to those MPs may put them at risk.
Material and methods: Here we analysed 60 samples of Holothuria forskali taken from the west and east of the Cape Peñas transition zone in different seasons. MPs were quantified from body wall tissue, and mitochondrial DNA sequences (16S and COI genes) were employed to determine population connectivity and infer replenishment capacity.
Results: Much higher MPs content in holothurian tissue than in water and sediments confirmed bioconcentration in this species. Significant differences between sampling locations were found, holothurian pollution being consistent with the occurrence of MPs sources like rivers, fishing ports and aquaculture. Genetic connectivity and no signals of diversity reduction in the most polluted samples suggest population replenishment capacity in H. forskali in the studied region. Results for a few samples of H. mammata from the same sampling points confirmed a higher MPs pollution at the west of Cape Peñas.
Discussion: According to these exploratory results, sea cucumbers seem to accumulate MPs especially in the western Cantabrian Sea. Further studies are recommended to understand the possible MPs threat for these holothurian species.
1 Introduction
Holothurians (Phylum Echinodermata, Class Holothuroidea) are epibenthic echinoderms, omnivorous non-selective sediment feeders (Hamel and Mercier, 1998; Hartati et al., 2017). They ingest the underlying substrate and recover edible materials (detritus, eggs, phytoplankton, etc.) by filtration, as well as floating plankton (Dar and Ahmad, 2006). Holothurians have been traditionally exploited in the central Indo-Pacific since 1700 (Conand, 1990; Purcell, 2014), and their fisheries are expanding all around the globe (Purcell et al., 2023). According to FAO (https://www.fao.org/3/cc5230en/cc5230en.pdf) 57,700 tonnes of wild sea cucumbers were harvested globally in the decade of 2011-2020. Tropical fisheries are the largest contributors to global catches (Conand, 2018; Conand et al., 2022), with 64 species commercialised so far. Sea cucumber prices are highly variable depending on the species, size, and origin. Mean price of final dried product is around 500 $/kg in Chinese retail markets (González-Wangüemert et al., 2018).
Due to the increasing demand from international markets, together with high price and easy harvesting, new holothurians markets target new species in temperate waters, such as the Mediterranean Sea (Antoniadou and Vafidis, 2011; González-Wangüemert et al., 2014; González-Wangüemert and Godino, 2016). Systematic holothurian fisheries started in Turkey in 1996, followed by Greece, Spain, France, and Italy, and focus on a few species (Hamel and Mercier, 2008; González-Wangüemert et al., 2014). FAO has just started to officially record sea cucumber landings per country in the Mediterranean fishing area (i.e., FAO 37), where they were previously undocumented (Vafidis and Chryssanthi, 2023). Currently, FAO recommends size and gear limitations (https://www.fao.org/3/i1384e/i1384e05a.pdf) that are followed by different countries. In Turkey, fishery regulation measures have been implemented since 2007, but due to an increase of illegal fishing new regulations were brought into force by the Ministry of Agriculture and Forestry in 2019 (Dereli and Aydin, 2021). Portuguese legislation about sea cucumber fisheries includes the species Holothuria forskali, Parastichopus regalis and Mesothuria intestinalis (Diário da República, 1.a série — N.o 235 — 6 de Dezembro de 2010, Annex I), catch being forbidden in protected areas such as Ria Formosa (South Portugal) (González-Wangüemert and Godino, 2016). In Spain, sea cucumber fisheries are established in the Mediterranean area, where more than 10 companies export to China sea cucumbers from different species (Holothuria tubulosa, H. forskali, H. mammata, and H. arguinensis) caught from Spanish waters (González-Wangüemert and Godino, 2016) according to the current export policies of fishery products of the Ministry of Agriculture, Fisheries and Food (https://www.mapa.gob.es/es/ministerio/servicios/analisis-y-prospectiva/informecomextanual2022_tcm30-657480_tcm30-507752.pdf). A recent legislation to control the H. forskali catches has been published in Galicia (NW Spain), but in the rest of regions sea cucumbers fisheries are not regulated, illegal catch being suspected in Andalusia (South Spain) (González-Wangüemert et al., 2018). In the southern Bay of Biscay, Exploitation of sea cucumbers in this region has not been explored in depth yet, despite the quantity of potential target species: 35 species of the seven orders of class Holothuroidea have been described from that region (Fernández-Rodríguez et al., 2019).
Sea cucumbers play a significant role in the reduction of organic load in the ecosystems, influencing actively nitrogen and phosphor cycles while modifying the substrate and the surrounding environment (Simões et al., 2019). Due to their feeding behaviour, they introduce environmental pollutants into their digestive system that can be transposed to other organs, being therefore bioindicators of environmental pollution (Grossmann, 2014; Zhu et al., 2018; Mohsen et al., 2020; Bulleri et al., 2021; Marrugo-Negrete et al., 2021). Emerging pollutants like microplastics (MPs thereafter), i.e., small plastic particles <5mm (Andrady, 2011), have been found in different holothurian species worldwide (for example, Plee and Pomory, 2020, in Florida; Iwalaye et al., 2020, in South Africa, or Tejedor-Junco et al., 2021, in the Canary Islands). Sea cucumbers have been proposed as suitable biomarkers of sediment contamination by MPs (Rios-Fuster et al., 2022; Fagiano et al., 2023), and not only for their non-selective sediment feeding strategy. Three pathways of MPs uptake are recognised in the genus Holothuria: by tentacles or direct feeding, by respiration (respiratory tree) and by the vascular system (access through madreporite) (Iwalaye et al., 2020).
Plastic particles may produce both chemical and physical damage to marine organisms when they are ingested, being considered a risk for natural life (Rochman, 2018). For this reason, the abundance and composition of MPs in species of fishing interest are increasingly studied (Daniel et al., 2021). MPs can affect holothurian species in different ways, such as increased oxidative stress and altered immune response (Mohsen et al., 2021). It is important to analyse the exposure of natural populations to these pollutants to identify potentially vulnerable populations before starting new exploitations. In the south Bay of Biscay, MPs containing polymers harmful to health and aquatic life, such as polyethyleneimine (PEI), polyethylene glycol (PEG), acrylic (PAN/PAA), polyester aniline derivatives or chloroquine, have been detected from organisms as diverse as algae (Bilbao-Kareaga et al., 2023), gastropod and bivalve molluscs (Janssens & Garcia-Vazquez, 2021; Masiá et al., 2022), cnidarians (Janssens & Garcia-Vazquez, 2021) or fish (Menéndez et al., 2022). Holothurians could be equally polluted in the region, perhaps putting natural populations at risk.
The distribution of MPs pollution in the region is quite patchy because beaches and organisms are differentially polluted by plastic and MPs depending on local sources like rivers, fishing ports, and even malfunctioning wastewater treatment plants (WWTP) (e.g., Masiá et al., 2021; Menéndez et al., 2022). Connectivity is essential for populations to respond to environmental stresses, since when a population is highly disturbed and disappears from a deme, it can be replenished with individuals from neighbouring demes (e.g., Klingbeil et al., 2022), as happens in top shells on the Cantabrian coast (e.g., Muñoz-Colmenero et al., 2015). Determining population connectivity patterns and replenishment levels is critical to understand the resilience of species (Steinberg et al., 2016), being essential for marine spatial planning and species management (Carr et al., 2017). Marine species that spend part of their life cycle as planktonic larvae have a high dispersal potential, exhibiting a weaker genetic structure and higher gene flow than species with low dispersal potential (Cowen and Paris, 2006; Galarza et al., 2009). This is the case of holothurians. In other regions, restrictions to gene flow between relatively distant populations have not been found in Holothuria poli (Valente et al., 2015), and the West Mediterranean H. mammata is considered a single panmictic metapopulation (Borrero-Pérez et al., 2011). Genetic differentiation has been found only on a large scale for H. poli (Gharbi and Said, 2011), H. mammata (Borrero-Pérez et al., 2011) and H. scabra (Uthicke and Benzie, 2001). However, the gene flow in marine species can be constrained by physical and ecological accidents (Hellberg et al., 2002). In the south Bay of Biscay, the Cape Peñas is a main hydrographic feature located in the middle of the coastline (Domínguez-Cuesta et al., 2019) that could represent a barrier for the connectivity of the species. The connectivity between sea cucumber populations in this region is still untested, and is necessary to understand the potential for population replenishment in cases of environmental disturbances.
The objective of this study was to analyse the potential risk of contamination by MPs in H. forskali inhabiting the central south Bay of Biscay, as well as the population connectivity between differentially polluted zones. A smaller sample of H. mammata from the same sampling points was also analysed to confirm the results obtained for H. forskali.
Departure hypotheses are:
A. For the patchy distribution of MPs in south Bay of Biscay coast, differences in MPs concentration between sea cucumbers from relatively close areas are expected (Masiá et al., 2019; Menéndez et al., 2022). Higher MPs concentration will occur in zones with more sources of MPs.
B. The presence of harmful chemicals such as PEI, Polyester, PEG and PAN is expected in holothurians, as in other organisms from the study region (Janssens and Garcia-Vazquez, 2021; Menéndez et al., 2022). For the regional patchiness, the MPs composition will be different in different zones.
C. Connectivity between populations of holothurian species is expected at a short scale (Borrero-Pérez et al., 2011; Valente et al., 2015); thus signals of genetic depletion in more polluted areas are not expected.
2 Materials and methods
2.1 Ethic statement
The specimens analysed were collected by professional divers and anaesthetised with magnesium chloride (MgCl2) at 5% to prevent animal suffering. The project was approved by the competent research ethics committee of the Government of Asturias Principality, General Directorate of Maritime Fisheries, project code IDI-2018-00201, approval date 5 of February of 2020.
2.2 Samples and sampling areas
H. forskali adults were sampled from two different points (Figure 1, Table 1): Palo Verde (Ribadesella) and Cape Blanco (El Franco), located at East and West, respectively, of the Cape Peñas. Cape Peñas is the main geomorphological feature situated in the middle of the coastline, where the main component in the coastal drift (North Atlantic Drift: NAD) goes eastward (Marquina et al., 2015; Domínguez-Cuesta et al., 2019), moving water from west to east. Rivers, fishing activities, and wastewater discharges of large urban concentrations, are principal contributors to marine MPs (e.g., Browne, 2015; Lebreton et al., 2018; Masiá et al., 2021; Meijer et al., 2021; Menéndez et al., 2022). Wastewater treatment plants (WWTPs) and main rivers in the study region are marked in Figure 1. Information on the hydrology of the Asturian coast is published and available on the official website of the Hydrological Confederation of the Cantabrian Sea (https://www.chcantabrico.es/organismo/las-cuencas-cantabricas/marco-fisico/hidrologia). The location of WWTPs in Asturias can be found on https://consorcioaa.com/saneamiento.
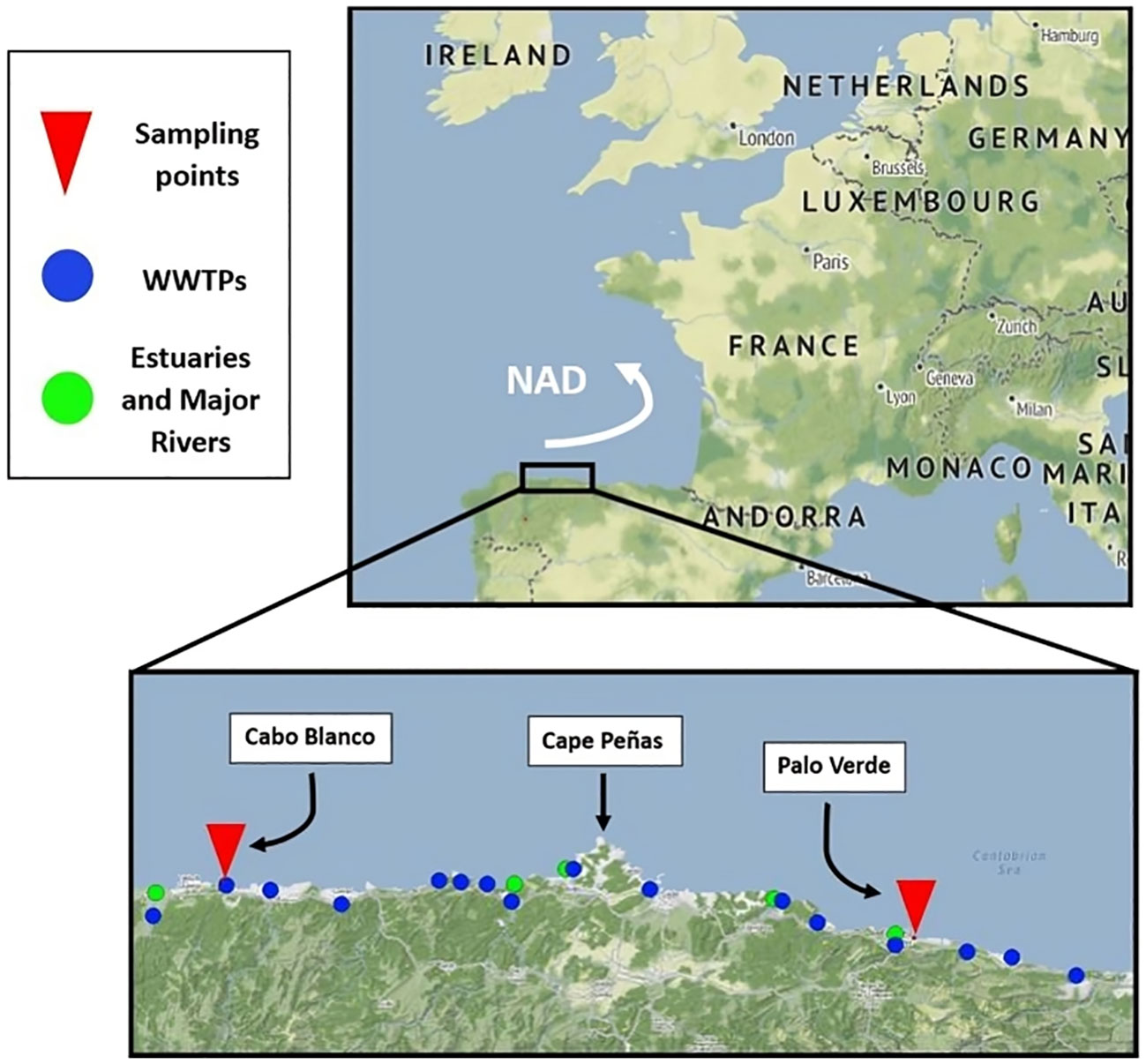
Figure 1 Study region in the Cantabrian Sea. Top, location of the sampling area in Europe showing the North Atlantic Drift (NAD) as a white arrow. Bottom, sampling points (red triangles): Cape Blanco, left (west) and Palo Verde, right (east). Wastewater treatment plants (WWTPs) are marked as blue dots. Main estuaries (green dots) are, from west to east: Eo, Nalon, Aviles, Villaviciosa and Sella.
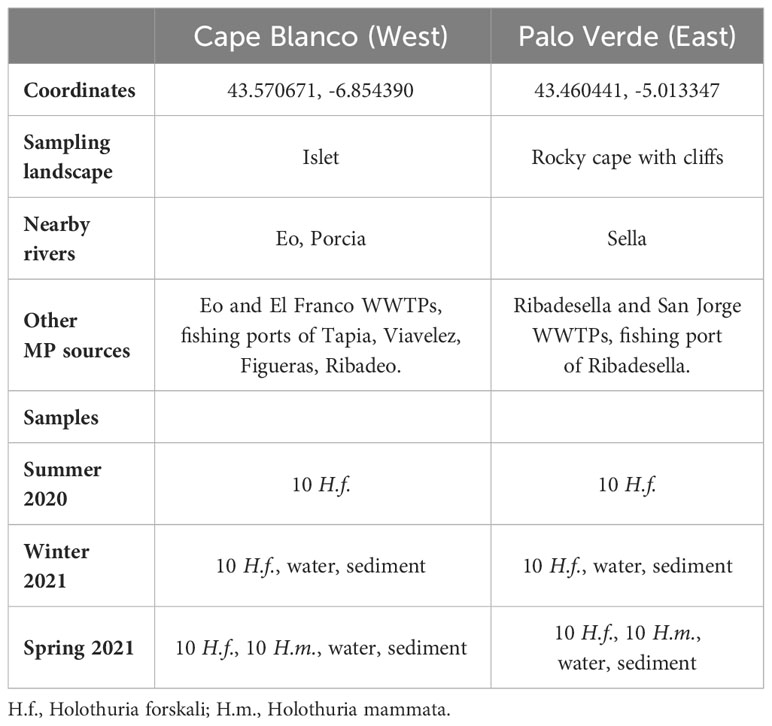
Table 1 Summary of sampling sites and samples taken by season (Summer 2020, Winter 2021 and Spring 2021).
The coordinates of the sampling points, main influencing rivers and potential MPs sources are presented in Table 1. Cape Blanco is located 14 km east of the Eo River which is 99 km long and has an annual average flow of 19.61 m³/s; in addition, the smaller Porcia River (31 km length, 3.1 m³/s annual mean flow) is 1.6 km west of Cape Blanco. The WWTP El Franco is 1.11 km west, and the fishing ports of Viavelez and Tapia are located respectively 1.4 km east and 7.5 km west of Cape Blanco, in addition to the fishing ports of Figueras and Ribadeo in the Eo estuary. The Eo estuary concentrates the oyster aquaculture in the region. On the other hand, Palo Verde is located 4 km east of the Sella River, of 66 km length with annual average flow of 18.07 m³/s. The fishing port and the WWTP of Ribadesella are 5 km west of this sampling point (Figure 1). Tourism visiting beaches and recreational areas occurs in the two zones. From the data summarised in Table 1, total river discharges and the number of fishing ports are higher at the west (Cape Blanco) than at the east (Palo Verde), while the other potential sources of plastic and MPs are apparently similar in the two locations.
Sampling was done through three consecutive seasons: summer 2020, winter 2021 and spring 2021. Ten adults of Holothuria forskali were sampled from each sampling point each season (Table 1), making a total of N = 60 H. forskali individuals. In spring 2021 10 adults of Holothuria mammata were taken from the same sampling points and processed as H. forskali, for comparison of the results with a second species living in the same conditions (N = 20 H. mammata). Holothurians were identified de visu by experts of the Asturias Fisheries Research Centre, where they were gutted, then they were transported to the laboratory of Natural Resources of the University of Oviedo in clean labelled bags for further processing.
Samples of water (10L) and sediment (50g) were taken together with sea cucumbers in winter and spring (Table 1). Water (two replicates of 5L each, being 10L in total per sampling point each season) was taken in bottles carefully cleaned with filtered distilled water. One replicate of 50g of sediment was taken manually with small glass jars just below or by each sample of holothurians and stored in the same jars. Unfortunately, environmental samples could not be taken in summer 2020 for logistic limitations due to bad weather conditions during the scheduled sampling time.
2.3 MPs extraction and processing
Water samples were filtered immediately after arrival in the laboratory through 0.45μm Polyethersulphone (PES) sterile filters (PALL Corporation®, 47 mm diameter), following the protocol described by Masiá et al. (2019). One litre was filtered through each filter to facilitate MPs counting. MPs extraction from sediment was carried out by flotation, following the protocol described by Besley et al. (2017). Briefly, 50g of previously dried sediment were added to 200mL of a hypersaline solution (358.9g of NaCl per litre of filtered distilled water), manually shaken for two minutes three times (Laglbauer et al., 2014), then left at room temperature for 24 hours. The final solution containing potential MPs was filtered through 0.45 µm pore PES filters. This step was carried out as carefully as possible to avoid sand falling over the filter (Menéndez et al., 2022). Two filters per sample were necessary due to saturation while filtering.
For holothurians, approximately 10g of edible tissue (body wall from gutted individuals) were taken longitudinally (mouth-anus axis, from the inner muscle to the skin) to ensure a reliable representation of the MPs accumulation along the organism, per individual. The material was cut with a clean scalpel in a laminar flow cabinet. Digestion was performed adding 20 mL of H2O2 (30% v/v) per gram of tissue. This solvent is not expected to degrade or destroy MPs (Liebezeit and Dubaish, 2012; Riani et al., 2023). The digestion mixture was stored in a 400 mL glass jar (covered with aluminium foil to avoid plastic contamination) at 65°C for 7 days to ensure that all organic material was digested (modified from Renzi et al., 2018). Daily shakes were performed. After digestion, the sample was diluted to 1L with filtered distilled water to facilitate the filtering process (Menéndez et al., 2022). During the filtration, the jar was rinsed with filtered distilled water to recover all potential material stuck in the walls. In total, five 0.45 µm pore PES filters were used per individual.
Filters were directly observed in a Leica 2000 Stereomicroscope (40x magnification) in closed and cleaned petri dishes. Only potential plastic particles smaller than 5 mm length were considered (Andrady, 2011; Andrady, 2017). MPs were classified by shape and colour. Shapes were fibres (cylindrical, length at least 3:1 width, continuous colouration), fragments (irregular shapes and colourations, sharp angles) and beads (spherical, commonly aggregated in pellets) (Hidalgo-Ruz et al., 2012; Cole, 2016; Lorenzo-Navarro et al., 2021; Monira et al., 2022). Colours were recorded as described by other authors; expectations are mostly black, blue, transparent, and other marginal colours (Abayomi et al., 2017; Gago et al., 2018; Wang et al., 2019; Ugwu et al., 2021).
For the chemical analysis, 15-20% of the main MPs found, principally blue and black fibres, were randomly selected (Uurasjärvi et al., 2021). They were picked from the petri dishes under laminar flow cabin. µFTIR analysis (Fourier-Transform Infrared Spectroscopy) was done (FTIR Varian 620-IR and Varian 670-IR, using a wavelength between 4000 and 500 cm-1 and a germanium glass, Varian 620-IR and Varian 670-IR cm-1), in the Autonomous University of Madrid. Results with a bibliographic search score over 60% were used. Polymers were checked in the ECHA (European Chemicals Agency) website (https://echa.europa.eu/es/home) for their potential toxicity for the aquatic life and/or for humans.
2.4 Contamination prevention
To prevent potential contamination from airborne MPs, all procedures were carried out into a vertical laminar flow cabinet. Researchers wore cotton lab coats all time. All liquids (reagents, water, and hypersaline solution) were filtered through 0.2 µm pore size PES filters. Materials in contact with samples/reagents (tweezers, vacuum pump recipients, petri dishes, iron plates, glass jars and aluminium foil) were carefully cleaned with filtered distilled water following instructions from Lusher et al. (2015). Blanks (n =) consisting in the digestion reagent were run simultaneously with the samples to control the contamination during manipulation, dilution, and filtration. All filters were stored after filtration in clean petri dishes into a closed paperboard box for, at least, 48 hours before starting the MPs counting.
2.5 DNA extraction, PCR, and sequencing
For all the individuals, a small piece of longitudinal inner muscle was taken (approx. 0.5g) for DNA extraction using Chelex-based protocol (Estoup et al., 1996). In order to increase the PCR success, DNA was previously diluted 1:10 in bidistilled water.
Two fragments of mitochondrial DNA, cytochrome oxidase I (COI) and 16S rDNA genes, were analysed. They have been employed in Valente et al. (2015) to determine the population genetic structure of Holothuria poli in the Mediterranean Sea. Within 16S rRNA gene a fragment of 486 base pairs (bp) was amplified following the modified protocol described by Valente et al. (2015), using the primers developed by Palumbi (1996): 16Sar-L 5’-CGCCTGTTTATCAAAAACAT-3’ and 16SB 5’-CTCCGGTTTGAACTCAGATCA-3’. The PCR protocol was an initial denaturation at 95°C for 3 min, then 40 cycles of denaturation at 94°C for 20 s, annealing at 45°C for 20s and extension at 72°C for 20 s. Finally, 72°C for 10 min for a final extension.
A fragment of 313 bp within cytochrome oxidase a subunit I (COI) gene was amplified using Leray et al. (2013) primers (mICOIintF 5’-GGWACWGGWTGAACWGTWTAYCCYC-3’ and jgHR 5’-TAIACYTCIGGRTGICCRAARAAYCA-3’). PCR protocol consisted of an initial denaturation at 95°C for 5 min, followed by 35 cycles of denaturation at 95°C for 15 s, annealing at 46°C for 15 s and extension at 72°C for 10 s, and a final extension at 72°C for 3 min.
For the amplification of both genes, PCR reactions contained 10 µmol of each primer, 2.5 mM MgCl2, 0.25 mM dNTPs, 1X Buffer GoTaq Promega, 0.15 μl of GoTaq Polymerase (5u/μL) and 2μL of diluted DNA, in a total final volume of 20 μL. PCR products were visualised using 2% agarose gel stained with 10 mg/mL Symple-Safe™ (2.5 µL, EURx, Gdansk, Poland). Amplicons were sent for Sanger sequencing at Macrogen Spain, Inc. (Madrid, Spain).
Sequences were edited and trimmed with BioEdit Sequence Alignment Editor software (Hall, 1999). Finally, the outcome sequences were checked for correct species and gene identity in the GenBank database (http://www.ncbi.nlm.nih.gov/genbank/, accessed in July 2023) using the BLAST algorithm and best match criterion.
2.6 Data analysis
2.6.1 Microplastics
MPs counts were transformed to MPs/g in all the elements (water, sediment, and holothurian tissue) to make the results comparable, using a density of 1.025 g/l for Cantabrian Sea water. First, considering the variables MPs/g for holothurians, season (1, 2 and 3 for the three consecutive seasons), location (0 for east and 1 for west), and species (0 for H. mammata and 1 for H. forskali), exploratory principal component analysis (PCA) was run to visualise the relationships between variables. The following settings were applied: correlation option; variables with r<0.8 pairwise correlation; significant components with >0.7 eigenvalue.
Due to small sample sizes, nonparametric tests were preferred for comparisons between samples. Chi-squared analyses were performed to compare samples for the proportion of MPs types (shape and colour) and composition (chemical), between locations or seasons for H. forskali and between locations for H. mammata.
For the quantitative variable MPs/g, normality was checked using Shapiro-Wilk test, and homogeneity of variance using Breusch–Pagan test. To test the effect of season (three levels: summer, winter, spring) and sampling location (two levels: west, Cape Blanco, and east, Palo Verde sampling points) on H. forskali means, two-way PERMANOVA analysis was performed, with 9,999 permutations and Euclidean distance. To test the effect of the location on the H. mammata mean of MP/g, one-way PERMANOVA was employed with the same settings.
Standard significance level of p<0.05 was adopted. Analyses were done on free software PAST v.2.17 (Hammer et al., 2001).
2.6.2 Genetic diversity and population differentiation
Haplotype diversity (Hd), number of haplotypes, exclusive haplotypes and polymorphic sites were calculated for each sample using DnaSP software v.6 (Rozas and Rozas, 1995). Relationships between haplotypes and their geographical distribution were analysed in haplotype networks constructed with the software Network version 10.2.0.0. (http://www.fluxus-engineering.com) with default settings.
Population genetic differentiation was estimated from FST value based on haplotype frequencies, and its significance was calculated by performing 2000 random permutations using ARLEQUIN software v.3.5.1.3 (Excoffier and Lischer, 2010). The level of significance adopted was standard p < 0.05.
3 Results
3.1 Microplastic content
Blanks exhibited a mean MPs concentration of 0.0076 ± 0.0079 MPs/g, which was two and even three orders of magnitude lower than those of sea cucumbers (see below), thus it can be assumed that no external contamination occurred during the digestion process. MPs were found from all types of samples, accounting for 1,063 particles in total: 683 from holothurians (Supplementary Table 1), 15 from sediment and 365 from water samples (Supplementary Table 2). The majority was fibres that represented the 95.7%, 100%, and 94.2% of all particles in sea cucumbers, sand and water, respectively.
In general, water had the lowest concentration of particles (maximum 0.012 ± 0.01 MPs/g in spring), followed by sediment (mean 0.05 ± 0.352 MPs/g). Holothurians exhibited much higher MPs concentrations than environmental samples, being H. forskali from the west in summer the highest (1.54 ± 1.135 MP/g) (Table 2). Sediment results were excluded from further analysis due to the small number of samples taken, with no replicates per location. According to a higher number of potential MPs sources, MPs concentration was apparently similar or higher at the west, especially in holothurians.
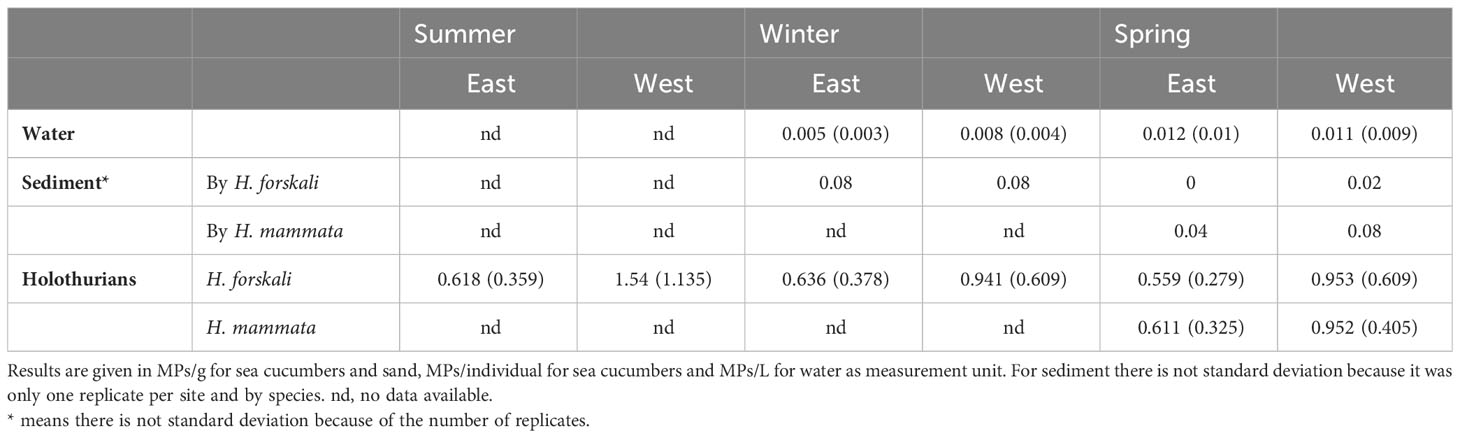
Table 2 Mean concentration (standard deviation in parentheses) of MPs in the different samples studied by season, species (or species associated) and location.
The principal component analysis (PCA) scatter plot provides a visual interpretation of the relationships between the variables considered (Figure 2). PC1 and PC2 explained the 40% and 37% of the variance respectively, being the season and location the factors with higher loads. The diagonal representing MPs concentration in holothurians was in the second quadrant together with the location (1 for west and 0 for east, corresponding to more potential sources of MPs pollution at the west) that was the closest diagonal. The diagonal representing the season (1-summer 2020, 2-winter 2021, 3-spring 2021) was in the quadrant II, opposite to the diagonal representing the species that was in the quadrant IV. H. forskali and H. mammata were segregated, with H. mammata concentrated in the quadrant II. For the two species, individuals sampled from the west (yellow signs) were clearly separated and above of those sampled from the east (blue signs), suggesting a strong effect of the location on the MPs concentration – as expected from a higher number of MPs sources near the west sampling point.
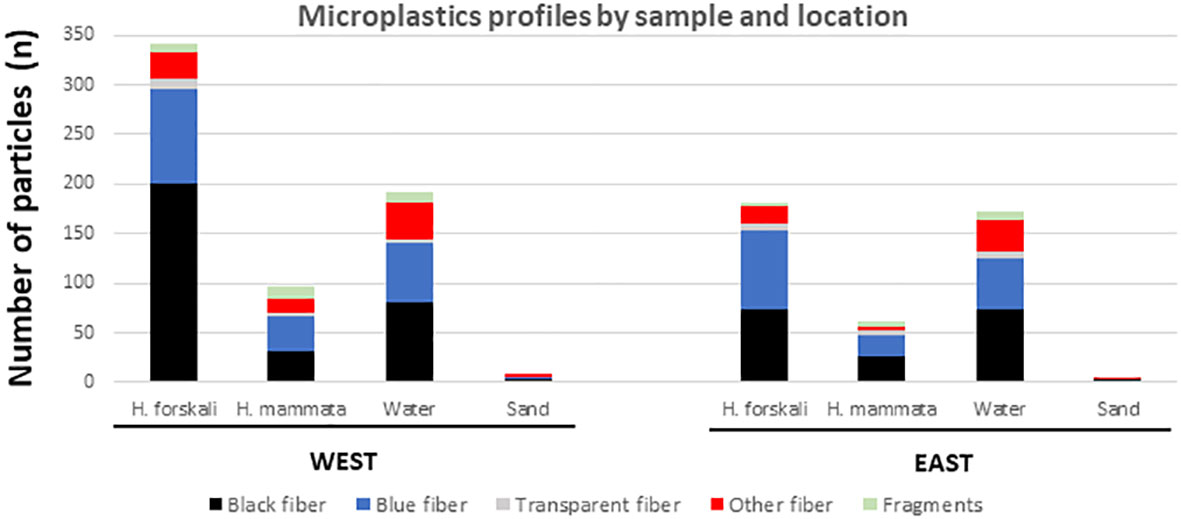
Figure 2 PCA scatter plot representing the relationships between the MPs concentration in holothurians (MPs/g) and the other variables considered: location, season, and species.
In H. forskali, that was the main focus of this study, location was highly significant in the two-way PERMANOVA, unlike the season that was not significant, nor the interaction (Table 3); this means that individuals sampled at the west exhibited significantly higher MPs concentration than those sampled at the east independently of the season, as seen in Table 2.
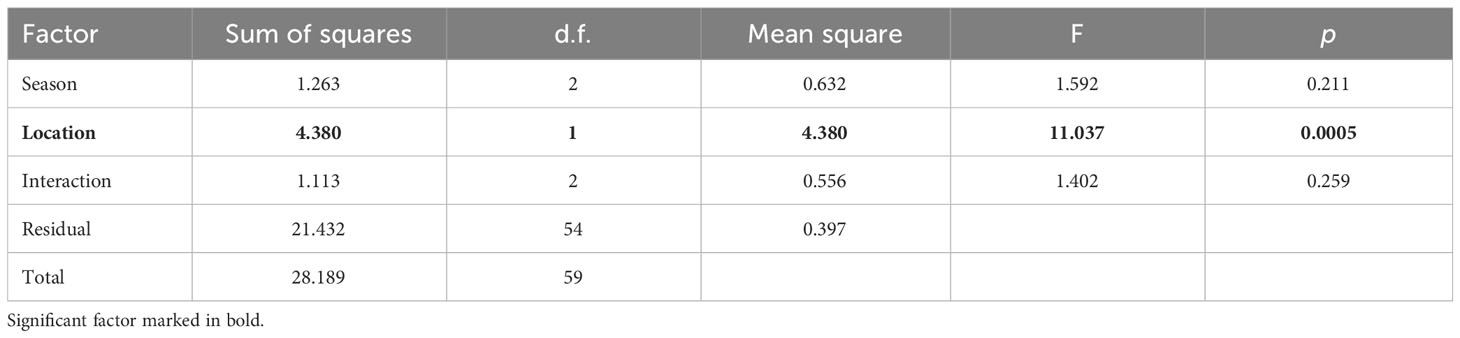
Table 3 Two-way PERMANOVA (9,999 permutations) testing the effect of the factors “Season” and “Location” on the variation of MPs concentration in Holothuria forskali (d.f. = degrees of freedom).
Regarding MPs types in H. forskali (n = 530 particles in total), most of them were mainly black and blue fibres, fragments being much less abundant (Supplementary Table 1). Chi-squared test comparing the seasonal profiles was not significant for the east (χ²=12.01; 8 d.f.; p=0.15 > 0.05, not significant) nor for the west location (χ²=12.47; 8 d.f.; p=0.13). Pooling together the seasonal samples of each location, the difference between east and west locations was highly significant (χ²=19.75; 4 d.f.; p=0.0006), with a higher proportion of fragments and black fibres at the west (Figure 3, first and third columns at left). The MPs types obtained from water samples (n = 365, sampled in winter and spring; fifth and seventh columns in Figure 3) were not significantly different between locations (χ²=1.38; 4 d.f.; p=0.84).
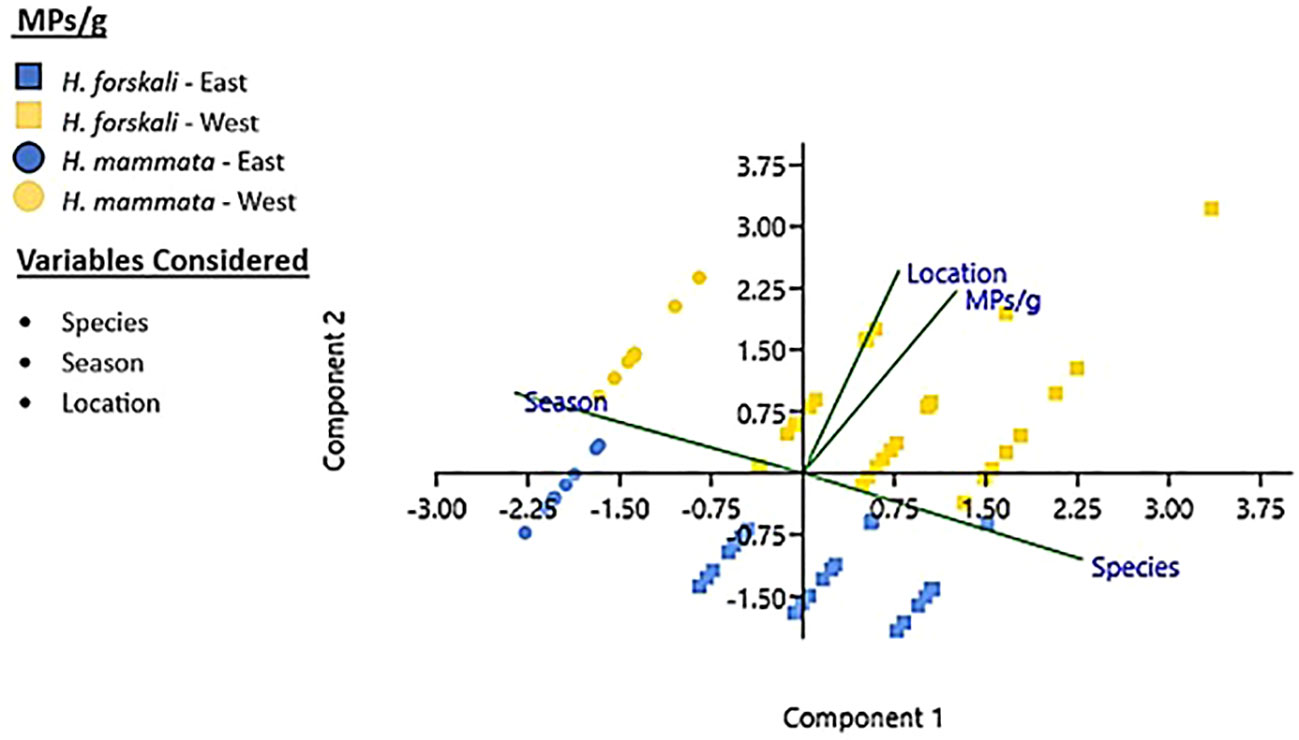
Figure 3 Profile of microplastics found from Holothuria forskali (Hf) and water samples in this study, for type (shape and colour) and chemical composition.
For the composition of the 151 MPs analysed from all the samples (the two holothurian species and water from the west and east locations), 18.8% were natural compounds, such as cotton and cellulose; the rest were plastics and other substances (Supplementary Table 3). The most abundant material was Rayon (45%) followed by Polyethyleneimine cellulose - PEI (15%), Polyester (9.7%), Polyethylene terephthalate - PET (5.8%), Polyacrylonitrile - PAN (2.6%), Nylon (1.3%), Polyethylene glycol - PEG (0.65%) and Urethane Alkid (0.65%) (Supplementary Table 3). The hazardousness of the compounds was checked in the ECHA website (http://echa.europe.eu). Four of them are recognised as harmful for aquatic life and/or the human health: PEI, Polyester, PAN, and PEG that summed a total of 42 particles (27.8% of the total). These compounds were expected in the second departure hypothesis.
Focusing on the artificial compounds found in H. forskali (Figure 3, second and fourth columns; Supplementary Table 3), the clear difference between east and west found for the quantity and types of MPs was not found for particles composition. The profile was relatively similar at east and west, with a relatively high proportion of rayon followed by PEI and polyester and no significant differences among the two locations (χ²=4.53; 3 d.f.; p=0.21). For the water the difference between west and east samples was also not significant (χ²=3.82; 3 d.f.; p=0.28). PEI particles were not found in water samples taken at the east (Figure 3, column at right), but this was not enough for statistical significance, being probably due to small sample size (= sampling effect, with only 17 artificial particles in east water sample; Supplementary Table 3).
In the second species considered, H. mammata, the results were similar to those found in H. forskali although the number of MPs detected was much smaller (n = 160 particles), because sampling for this species was done only in the spring. As shown in Table 2, the individuals sampled from the west location exhibited more MPs than those sampled at the east (means 0.952 with SD 0.405, versus 0.611 with SD 0.325, respectively). As in H. forskali, the difference of means was statistically significant (one-way PERMANOVA with 9,999 permutations gives a total sum of squares of 3.005 and within-group sum of squares of 2.423, F = 4.324, p = 0.047).
In H. mammata’s profile by type of MPs (Figure 4), the majority of particles were also black and blue fibres. As in H. forskali, fragments were more abundant at the west and transparent fibres at the east; however, the proportion of black and blue fibres was quite similar and the difference between west and east locations was not significant (χ² = 3.10; 4 d.f.; p=0.54). For the composition, in H. mammata from the west two particles were PAN, which is a harmful substance according to the ECHA. This substance was not found in H. forskali. The composition profile of west and east locations looked quite different (Figure 4, second and fourth columns), but the difference was not significant (χ² = 4.64; 4 d.f.; p=0.33); in this case samples sizes were very small, since only 10 and 12 particles from west and east location respectively were analysed with FT-IR.
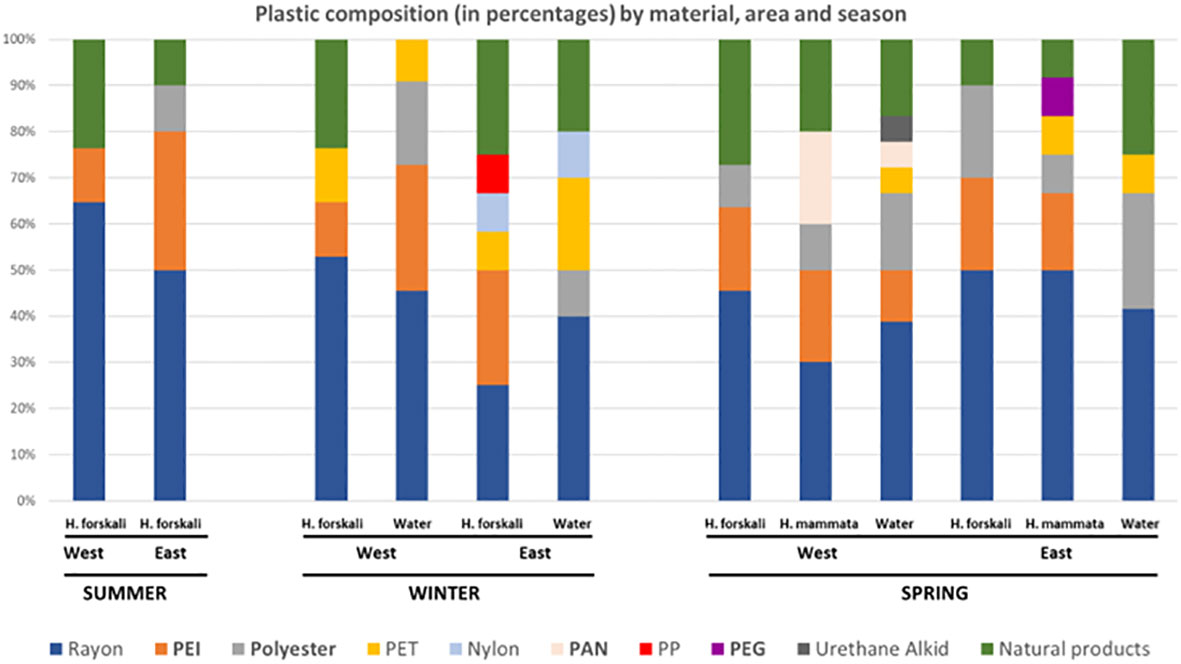
Figure 4 Composition of particles found from H. mammata (Hm) samples in west and east sampling points.
3.2 Genetic diversity and estimated population connectivity
Not all the individuals could be genetically analysed, possibly due to procedural issues. In total 72 sea cucumbers were successfully sequenced for the 16S rRNA gene, and 56 for COI gene. After editing the sequences, alignments of equal length were obtained for the two genes and species: 321 and 373 bp for 16S in H. forskali and H. mammata, respectively; and 237 and 313 bp for COI in H. forskali and H. mammata, respectively. BLAST analysis of the DNA sequences obtained confirmed the species visually assigned in all the cases (H. forskali or H. mammata), with more than 99% of identity for both genes. The sequences obtained were submitted to GenBank, where they are available with the accession numbers OR681936-OR681967 and OR682161-OR682170 for 16S gene, and OR681876-OR681886 and OR690923-OR690929 for COI gene.
3.2.1 Genetic diversity
For the gene 16S a total of 32 haplotypes among 54 individuals of H. forskali, and 10 haplotypes among 18 individuals of H. mammata, were detected (Table 4). For COI gene, the number of haplotypes found was 11 haplotypes among 36 H. forskali individuals, and 7 haplotypes among 20 H. mammata individuals (Table 4). High haplotype diversity was found for the two genes in the two species, ranking from 0.96 for 16S gene in H. forskali to 0.75 for COI gene in H. mammata. The haplotype diversity was higher for H. forskali for both markers. Many exclusive haplotypes (unique of a location) were found, especially in H. forskali. In H. forskali the number of haplotypes was similar for the west and the east locations, being respectively 20 and 19 for 16S gene, and 6 and 8 for COI gene; thus, signals of depleted diversity were not found with the present data.
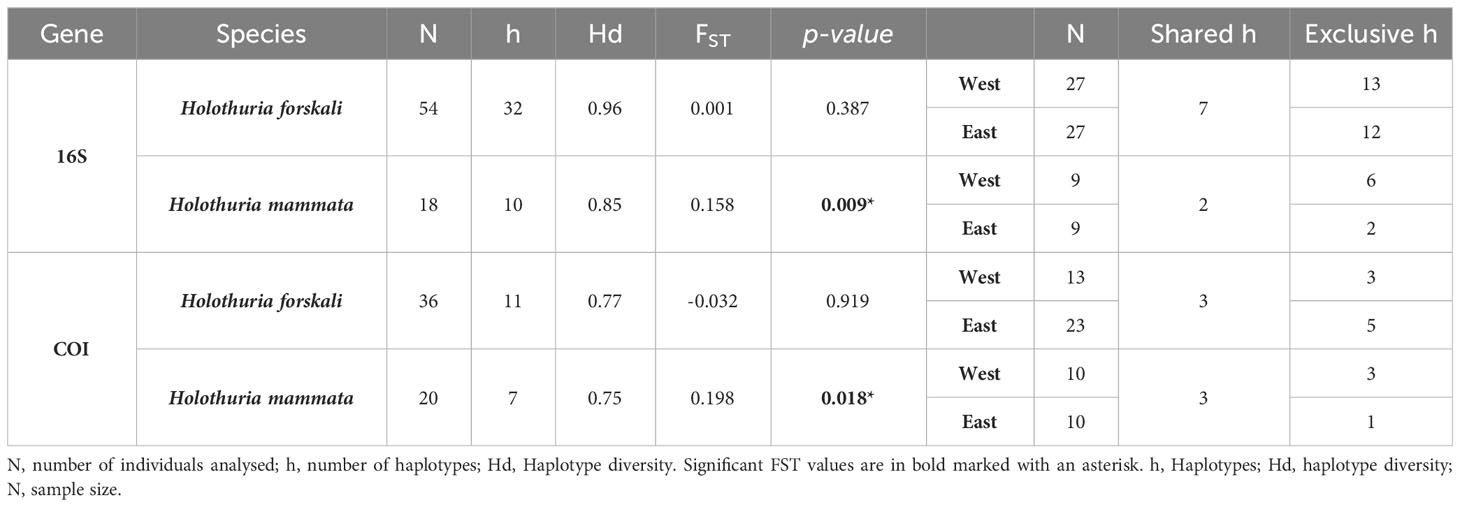
Table 4 Genetic diversity of 16S rRNA and COI genes for H. forskali and H. mammata in the two sampling locations considered in this study.
In the case of H. mammata diversity was somewhat lower, especially in the east location: the number of haplotypes was 8 in the west and 4 in the east location for 16S gene; 6 in the west and 4 in the east location for COI gene (Table 4). As for MPs, the results obtained for H. mammata must be taken with caution due to small sample sizes (18 and 20 individuals, for 16S and COI genes, respectively).
3.2.2 Population differentiation
Pairwise FST results did not show any significant difference between regions in H. forskali for any gene (Table 4), thus limitations to gene flow and connectivity were not found with these genes. In contrast, the two H. mammata samples exhibited significant FST suggesting gene flow restrictions for both genes (Table 4). Again, these results should be taken with caution due to small sample sizes for this species.
Parsimony haplotype networks for both genes are shown in Figure 5. They were consistent with lack of differentiation between the two locations, the haplotypes with the higher frequencies being all shared among locations (Figure 5). The networks exhibited irregular shapes, quite similar in the two species with many singletons and exclusive haplotypes and generally more complex for 16S than for COI gene, according to the relative diversity of these two genes in this study. Differences between west and east locations were not evident in these networks for H. mammata, suggesting that the observed FST significance could be simply due to small sample sizes.
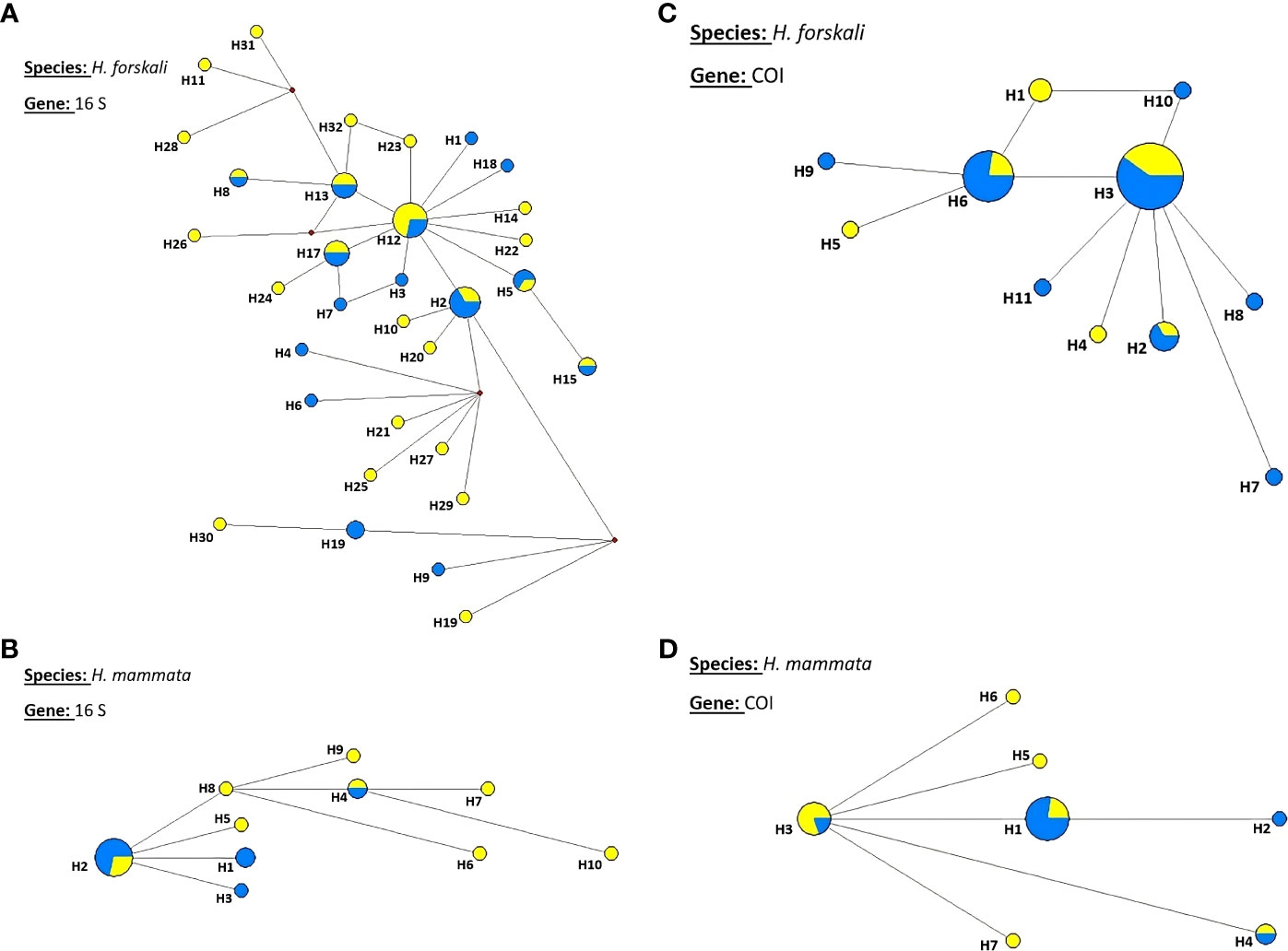
Figure 5 Parsimony haplotype networks for 16S and COI genes in H. forskali and H. mammata. (A) H. forskali- 16S; (B) H. mammata-16S; (C) H. forskali-COI; (D) H. mammata-COI. East location: blue, West location: yellow. The size of the circle is proportional to the number of individuals with each haplotype.
4 Discussion
To the best of our knowledge, this is the first study on MPs content and population connectivity of Holothuria forskali in the Bay of Biscay. The results obtained were consistent with previous studies from Greece and Italy reporting MPs in this species (Seary et al., 2013; Deudero and Alomar, 2015). Supporting Hypothesis 1, the level of MPs pollution was higher in the west, where more MPs sources occur, than in the east. Fishing and aquaculture, more concentrated near the west location, are important sources of MPs marine pollution (Xue et al., 2020; Chen et al., 2021) and would explain at least partially the observed difference. In this region, Masiá et al. (2021) reported a higher abundance of macro- and microplastics in beaches near ports and areas with intense fishing activity. Greater river discharge at the west would also explain a higher MPs pollution, since rivers are well known MPs sources (Campanale et al., 2020; Lestari et al., 2020). These MPs would accumulate in holothurians, which are sentinels of MPs pollution (Rios-Fuster et al., 2022; Fagiano et al., 2023).
From the perspective of the potential consumption of these holothurians - if they are harvested in a future – the MPs concentrations found in our study were considerably higher than those published in other studies. For example, Mohsen et al. (2023) found an average of 0.081 MPs/g in Apostichopus japonicus commercialised in Chinese markets, which is about ten times smaller than that found in our study on the Cantabrian Sea. Relatively high MPs concentrations have been found in other marine species from the same coast (in comparison with other regions), for example in mussels suggesting problematic accumulation of MPs in the area.
As expected in Hypothesis 2, the type and composition of those MPs was consistent with that reported for other organisms and water from the Cantabrian Sea (La Daana et al., 2017; Masiá et al., 2022; Menéndez et al., 2022), dominated by black and blue fibres while the particles of other colours are relatively scarce. The types of MPs were significantly different in the two locations, according to Hypothesis 2 and the patchiness found in the region in previous studies (Masiá et al., 2019; Masiá et al., 2021). However, Hypothesis 2 was not totally accomplished, since the expected difference in holothurians MPs composition between these locations was not found. Higher sample sizes analysed with FT-IR would increase the statistical power of this observational study, but perhaps the composition is not so different because the ultimate sources of pollution (single use plastics, fishing materials, industrial wastes, microbeads in cleaners and cosmetics etc.) are relatively similar across the region, as they are in other marine waters (Frias et al., 2016; Bråte et al., 2018; Zhang et al., 2019; Wu et al., 2020).
A (relative) novelty of this study was finding much higher MPs concentration in the holothurians than in the water samples. This is the first evidence of bioaccumulation of MPs in the edible tissue of sea cucumbers of the genus Holothuria. The presence of MPs in the body wall suggests they were translocated from the gut, or from the respiratory system as seen in other species (Brennecke et al., 2015; Jovanović et al., 2018; Elizalde-Velázquez et al., 2020). Bioaccumulation in sediment feeders is not strange. Fagiano et al. (2023) found that marine sediments hold two-fold microplastics than sea surface waters and are dominated by fibers. Bioconcentration of MPs is supported by previous studies in marine invertebrates (Sfriso et al., 2020; Álvarez-Ruiz et al., 2021; Kuehr et al., 2022). In the case of sea cucumbers, bioconcentration has been hypothesised by other authors (Iwalaye et al., 2020; Lombardo et al., 2022; Martines et al., 2023) and confirmed in the genus Apostichopus (Mohsen et al., 2022). H. forskali was also proposed as a bioindicator of MPs pollution (Rios-Fuster et al., 2022; Fagiano et al., 2023); however, these studies focused on MPs ingestion analysing the gastrointestinal tract, so direct comparisons with our results are not reliable. From our results, Holothuria joins the list of genera that bioaccumulate MPs in tissues other than the gut.
For the third hypothesis, it was apparently accomplished for H. forskali, or at least we found no evidences of lack of connectivity from our data. The connectivity inferred in this study for H. forskali is supported by its long planktonic larval duration (Ivy & Giraspy, 2006), which favours the panmixia and suggests the capacity to recover from population disturbance through recruitment from other areas (Valente et al., 2015). For H. mammata, although FST was significant, very small sample sizes prevent robust conclusions about connectivity. Historically, H. mammata has been described only in the Mediterranean Sea, the southern Portuguese coast and the Azores Islands (Mercier and Hamel, 2013). Recent eastwards dispersion along the Cantabrian basin could explain some differences in new colonised areas; however, H. mammata’s haplotype networks relatively similar to those of H. forskali would suggest a similar connectivity. Moreover, the occurrence of shared haplotypes might indicate that gene flow between populations is happening in H. mammata. This is consistent with Valente et al. (2015) and Gharbi and Said (2011) results for Mediterranean H. poli, where population genetic structure can be detected only in some cases at longer distances. Interestingly and pointing at a good capacity of population replenishment, the level of diversity was not lower in the more polluted deme at west. On the contrary, it was similar or higher that the diversity found at the cleaner east location in the case of H. mammata. This result would point at a good recovery capacity of Holothuria populations in case of environmental disturbances, as it happens for other marine organisms of high larval dispersal capacity (e.g., Muñoz-Colmenero et al., 2015; Klingbeil et al., 2022).
The preliminary genetic results obtained in this study point to greater haplotypic diversity of the 16S gene than that of the COI gene, for the two species and in the two locations examined (see Table 4 above). It is generally assumed that the 16S gene is highly conserved evolutionarily, more than COI (Jo et al., 2016). However, a few other studies show a higher Hd in 16S than in COI in holothurians and other marine invertebrates (Borrero-Pérez et al., 2011; Soliman et al., 2016 in H. mammata and H. edulis respectively). This issue has not been discussed in depth yet and here we cannot give a proper explanation for this observation. It would deserve further exploration with many more data and other species, to understand the evolutionary patterns of these genes in marine invertebrates.
5 Conclusions
The species Holothuria forskali, as well as H. mammata, are able to bioaccumulate MPs in edible tissues. In South Bay of Biscay samples, H. forskali exhibits a higher concentration of MPs in the west that in the east of Cape Peñas, according to the respective number of MPs sources in each area. Differences in the distribution of MPs types were found between sites, supporting previously observed patchiness of MPs pollution along the coast. Toxic compounds such as PEI, Polyester, PEG, and PAN, also found from other species and water on this coast, were confirmed in these holothurians. High diversity at mitochondrial genes and low FST values suggest population connectivity and replenishment capacity between polluted and unpolluted demes in H. forskali. Similar diversity results suggest a similar situation for H. mammata, although these results should be taken with caution due to limited sample size.
Data availability statement
The original contributions presented in the study are publicly available. The datasets generated for this study can be found in the GenBank, GenBank Accession numbers OR681936-OR681967, OR682161-OR682170, OR681876-OR681886, and OR690923-OR690929.
Ethics statement
The animal study was approved by General Directorate of Fisheries, Asturias, Spain. The study was conducted in accordance with the local legislation and institutional requirements.
Author contributions
DM: Investigation, Methodology, Visualization, Writing – original draft. AÁ: Formal analysis, Investigation, Methodology, Resources, Writing – review & editing. AA: Formal analysis, Methodology, Project administration, Supervision, Visualization, Writing – review & editing. EG: Conceptualization, Data curation, Formal analysis, Funding acquisition, Supervision, Validation, Writing – review & editing.
Funding
The author(s) declare financial support was received for the research, authorship, and/or publication of this article. This study was funded from the Government of Asturias Principality, Grant IDI/AYUD2021/50967, and the Spanish Ministry of Science and Innovation Grant PID2022-138523OB-I00.
Conflict of interest
The authors declare that the research was conducted in the absence of any commercial or financial relationships that could be construed as a potential conflict of interest.
Publisher’s note
All claims expressed in this article are solely those of the authors and do not necessarily represent those of their affiliated organizations, or those of the publisher, the editors and the reviewers. Any product that may be evaluated in this article, or claim that may be made by its manufacturer, is not guaranteed or endorsed by the publisher.
Supplementary material
The Supplementary Material for this article can be found online at: https://www.frontiersin.org/articles/10.3389/fmars.2024.1333141/full#supplementary-material
References
Abayomi O. A., Range P., Al-Ghouti M. A., Obbard J. P., Almeer S. H., Ben-Hamadou R. (2017). Microplastics in coastal environments of the Arabian Gulf. Mar. Pollut. Bull. 124 (1), 181–188. doi: 10.1016/j.marpolbul.2017.07.011
Álvarez-Ruiz R., Picó Y., Campo J. (2021). Bioaccumulation of emerging contaminants in mussel (Mytilus galloprovincialis): Influence of microplastics. Sci. Total Environ. 796, 149006. doi: 10.1016/j.scitotenv.2021.149006
Andrady A. L. (2011). Microplastics in the marine environment. Mar. Pollut. Bull. 62 (8), 1596–1605. doi: 10.1016/j.marpolbul.2011.05.030
Andrady A. L. (2017). The plastic in microplastics: A review. Mar. Pollut. Bull. 119 (1), 12–22. doi: 10.1016/j.marpolbul.2017.01.082
Antoniadou C., Vafidis D. (2011). Population structure of the traditionally exploited holothurian Holothuria tubulosa in the south Aegean Sea. Cah Biol. Mar. 52, 171–175.
Besley A., Vijver M. G., Behrens P., Bosker T. (2017). A standardized method for sampling and extraction methods for quantifying microplastics in beach sand. Mar. Pollut. Bull. 114 (1), 77–83. doi: 10.1016/j.marpolbul.2016.08.055
Bilbao-Kareaga A., Menéndez D., Peón P., Ardura A., Garcia-Vazquez E. (2023). Microplastics in jellifying algae in the Bay of Biscay. Implications for consumers’ health. Algal Res. 72, 103080. doi: 10.1016/j.algal.2023.103080
Borrero-Pérez G. H., González-Wangüemert M., Marcos C., Pérez-Ruzafa A. (2011). Phylogeography of the Atlanto-Mediterranean sea cucumber Holothuria (Holothuria) mammata: the combined effects of historical processes and current oceanographical pattern. Mol. Ecol. 20 (9), 1964–1975. doi: 10.1111/j.1365-294X.2011.05068.x
Bråte I. L. N., Hurley R., Iversen K., Beyer J., Thomas K. V., Steindal C. C., et al. (2018). Mytilus spp. as sentinels for monitoring microplastic pollution in Norwegian coastal waters: A qualitative and quantitative study. Environ. Pollut. 243, 383–393. doi: 10.1016/j.envpol.2018.08.077
Brennecke D., Ferreira E. C., Costa T. M. M., Appel D., da Gama B. A. P., Lenz M. (2015). Ingested microplastics (>100μm) are translocated to organs of the tropical fiddler crab Uca rapax. Mar. Pollut. Bull. 96 (1–2), 491–495. doi: 10.1016/j.marpolbul.2015.05.001
Browne M. A. (2015). “Sources and pathways of microplastics to habitats.” Bergmann M., Gutow L., Klages M. (Eds.). Mar. Anthropogenic Litter (Springer Open), 229–244. doi: 10.1007/978-3-319-16510-3_9
Bulleri F., Ravaglioli C., Anselmi S., Renzi M. (2021). The sea cucumber Holothuria tubulosa does not reduce the size of microplastics but enhances their resuspension in the water column. Sci. Total Environ. 781, 146650. doi: 10.1016/j.scitotenv.2021.146650
Campanale C., Stock F., Massarelli C., Kochleus C., Bagnuolo G., Reifferscheid G., et al. (2020). Microplastics and their possible sources: The example of Ofanto river in southeast Italy. Environ. pollut. 258, 113284. doi: 10.1016/j.envpol.2019.113284
Carr H. M., Robinson S. P., Wahle C., Davis G., Kroll S., Murray S., et al. (2017). The central importance of ecological spatial connectivity to effective coastal marine protected areas and to meeting the challenges of climate change in the marine environment. Aquat. Conservation: Mar. Freshw. Ecosystem 27 (S1), 6–29. doi: 10.1002/aqc.2800
Chen G., Li Y., Wang J. (2021). Occurrence and ecological impact of microplastics in aquaculture ecosystems. Chemosphere 274, 129989. doi: 10.1016/j.chemosphere.2021.129989
Cole M. (2016). A novel method for preparing microplastic fibers. Sci. Rep. 7, (1–7). doi: 10.1038/srep34519
Conand C. (1990). The fishery resources of Pacific island countries: Holothurians (No. 272). Food Agric. Org. (Rome: FAO).
Conand C. (2018). Recent information on worldwide illegal fisheries for sea cucumbers. SPC Beche-de-mer Inf. Bull. 38, 4.
Conand C., Claereboudt M., Dissayanake C., Ebrahim A., Fernando S., Godvinden R., et al. (2022). Review of fisheries and management of sea cucumbers in the Indian Ocean. Western Indian Ocean J. Mar. Sci. 21 (1), 125–148. doi: 10.4314/wiojms.v21i1.10
Cowen R. K., Paris C. B., Srinivasan A. (2006). Scaling of connectivity in marine populations. Science 311, 522–527. doi: 10.1126/science.1122039
Daniel D. B., Ashraf P. M., Thomas S. N., Thomson K. T. (2021). Microplastics in the edible tissues of shellfishes sold for human consumption. Chemosphere 264, 128554. doi: 10.1016/j.chemosphere.2020.128554
Dar M. A., Ahmad H. O. (2006). The feeding selectivity and ecological role of shallow water holothurians in the Red Sea. SPC Beche-de-mer Inf. Bull. 24, 11–21.
Dereli H., Aydin M. (2021). Sea cucumber fishery in Turkey: Management regulations and their efficiency. Regional Stud. Mar. Sci. 41, 101551. doi: 10.1016/j.rsma.2020.101551
Deudero S., Alomar C. (2015). Mediterranean marine biodiversity under threat: Reviewing influence of marine litter on species. Mar. pollut. Bull. 98 (1–2), 58–68. doi: 10.1016/j.marpolbul.2015.07.012
Domínguez-Cuesta M. J., Valenzuela P., Rodríguez-Rodríguez L., Ballesteros D., Jiménez-Sánchez M., Piñuela L., et al. (2019). “Cliff coast of asturias,” in The Spanish coastal systems (Cham: Springer), 49–77.
Elizalde-Velázquez A., Carcano A. M., Crago J., Green M. J., Shah S. A., Cañas-Carrell J. E. (2020). Translocation, trophic transfer, accumulation and depuration of polystyrene microplastics in Daphnia magna and Pimephales promelas. Environ. pollut. 259, 113937. doi: 10.1016/j.envpol.2020.113937
Estoup A., Lagiarder C. R., Perrot E., Chourrout D. (1996). Rapid one-tube DNA extraction for reliable PCR detection of fish polymorphic markers and transgenes. Mol. Mar. Biol. Biotechnol. 5, 295–298.
Excoffier L., Lischer H. E. (2010). Arlequin suite ver 3.5: a new series of programs to perform population genetics analyses under Linux and Windows. Mol. Ecol. Resour. 10 (3), 564–567.
Fagiano V., Compa M., Alomar C., Rios-Fuster B., Morató M., Capó X., et al. (2023). Breaking the paradigm: Marine sediments hold two-fold microplastics than sea surface waters and are dominated by fibers. Sci. Total Environ. 858, 159722. doi: 10.1016/j.scitotenv.2022.159722
Fernández-Rodríguez I., Arias A., Anadón N., Acuña J. L. (2019). Holothurian (Echinodermata) diversity and distribution in the central Cantabrian Sea and the Avilés Canyon System (Bay of Biscay). Zootaxa 4567 (2), 293. doi: 10.11646/zootaxa.4567.2.5
Frias J. P. G. L., Gago J., Otero V., Sobral P. (2016). Microplastics in coastal sediments from Southern Portuguese shelf waters. Mar. Environ. Res. 114, 24–30. doi: 10.1016/j.marenvres.2015.12.006
Gago J., Carretero O., Filgueiras A. V., Viñas L. (2018). Synthetic microfibers in the marine environment: A review on their occurrence in seawater and sediments. Mar. pollut. Bull. 127, 365–376. doi: 10.1016/j.marpolbul.2017.11.070
Galarza J. A., Carreras-Carbonell J., Macpherson E., Pascual M., Roques S., Turner G. F., et al. (2009). The influence of oceanographic fronts and early-life-history traits on connectivity among littoral fish species. Proc. Natl. Acad. Sci. 106 (5), 1473–1478. doi: 10.1073/pnas.0806804106
Gharbi A., Said K. (2011). Genetic variation and population structure of Holothuria poli from the eastern and western Mediterranean coasts in Tunisia. J. Mar. Biol. Assoc. United Kingdom 91 (8), 1599–1606. doi: 10.1017/S0025315411000245
González-Wangüemert M., Domínguez-Godino J. A., Cánovas F. (2018). The fast development of sea cucumber fisheries in the Mediterranean and NE Atlantic waters: from a new marine resource to its over-exploitation. Ocean Coast. Manage. 151, 165–177. doi: 10.1016/j.ocecoaman.2017.10.002
González-Wangüemert M., Godino J. D. (2016). Sea cucumbers as new marine resource in Europe. Front. Mar. Sci. Conference Abstract: IMMR | International Meeting on Marine Research 2016. doi: 10.3389/conf.FMARS.2016.04.00112
González-Wangüemert M., Maggi C., Valente S., Martínez-Garrido J., Vasco-Rodrigues N. (2014). Parastichopus regalis—The main host of Carapus acus in temperate waters of the Mediterranean Sea and northeastern Atlantic Ocean. SPC Beche-de-mer Inf. Bull. 34, 38–42.
Grossmann J. L. (2014). Evaluating the potential of microplastics ingestion to harm the sea cucumber Holothuria sanctori (University of Hamburg: Doctoral dissertation, PhD Thesis).
Hall T. A. (1999). BioEdit: A user-friendly biological sequence alignment editor and analysis program forWindows 95/98/NT. Nucleic Acids Symp. 41, 95–98.
Hamel J. F., Mercier A. (1998). Diet and feeding behaviour of the sea cucumber Cucumaria frondosa in the St. Lawrence estuary, eastern Canada. Can. J. Zool 76. doi: 10.1139/z98-040
Hamel J. F., Mercier A. (2008). “Population status, fisheries and trade of sea cucumbers in temperate areas of the Northern Hemisphere,” in Toral-Granda V., Lovatelli A., Vasconcellos M. (eds). Sea cucumbers. A global review of fisheries and trade. FAO Fisheries and Aquaculture Technical Paper No. 516 (Rome: FAO), 257–291.
Hammer Ø., Harper D. A., Ryan P. D. (2001). PAST: paleontological statistics software package for education and data analysis. Palaeontol Electron. 4 (1), 9.
Hartati R., Widianingsih W., Trianto A., Zainuri M., Ambariyanto A. (2017). The abundance of prospective natural food for sea cucumber Holothuria atra at Karimunjawa Island waters, Jepara, Indonesia. Biodiversitas J. Biol. Diversity 18 (3), 947–953. doi: 10.13057/biodiv/d180311
Hellberg M. E., Burton R. S., Neigel J. E., Palumbi S. R. (2002). Genetic assessment of connectivity among marine populations. Bull. Mar. Sci. 70, 273–290.
Hidalgo-Ruz V., Gutow L., Thompson R. C., Thiel -. (2012). Microplastics in the marine environment: A review of the methods used for identification and quantification. Environ. Sci. Technol. 46 (6), 3060–3075. doi: 10.1021/es2031505
Ivy G., Giraspy D. A. B. (2006). Development of large-scale hatchery production techniques for the commercially important sea cucumber Holothuria scabra var. versicolor (Conand 1986) in queensland, Australia. SPC Beche-De-Mer Inf. Bull. 24, 28–34.
Iwalaye O. A., Moodley G. K., Robertson-Andersson D. V. (2020). The possible routes of microplastics uptake in sea cucumber Holothuria cinerascens (Brandt 1835). Environ. pollut. 264, 114644. doi: 10.1016/j.envpol.2020.114644
Janssens L., Garcia-Vazquez E. (2021). Dangerous microplastics in topshells and anemones along the north coast of Spain. Mar. pollut. Bull. 173, 112945. doi: 10.1016/j.marpolbul.2021.112945
Jo J., Park C., Kim M., Park C. (2016). Phylogenetic analysis of the three color variations of the sea cucumber Apostichopus japonicus. J. Aquac. Res. Dev. 7 (418), 2. doi: 10.4172/2155-9546.1000418
Jovanović B., Gökdağ K., Güven O., Emre Y., Whitley E. M., Kideys A. E. (2018). Virgin microplastics are not causing imminent harm to fish after dietary exposure. Mar. pollut. Bull. 130, 123–131. doi: 10.1016/j.marpolbul.2018.03.016
Klingbeil W. H., Montecinos G. J., Alberto F. (2022). Giant kelp genetic monitoring before and after disturbance reveals stable genetic diversity in Southern California. Front. Mar. Sci. 9. doi: 10.3389/fmars.2022.947393
Kuehr S., Esser D., Schlechtriem C. (2022). Invertebrate species for the bioavailability and accumulation assessment of manufactured polymer-based nano- and microplastics. Environ. Toxicol. Chem. 41 (4), 961–974. doi: 10.1002/etc.5315
La Daana K. K., Officer R., Lyashevska O., Thompson R. C., O’Connor I. (2017). Microplastic abundance, distribution and composition along a latitudinal gradient in the Atlantic Ocean. Mar. pollut. Bull. 115 (1-2), 307–314. doi: 10.1016/j.marpolbul.2016.12.025
Laglbauer B. J. L., Franco-Santos R. M., Andreu-Cazenave M., Brunelli L., Papadatou M., Palatinus, et al. (2014). Macrodebris and microplastics from beaches in Slovenia. Mar. pollut. Bull. 89 (1–2), 356–366. doi: 10.1016/j.marpolbul.2014.09.036
Lombardo J., Solomando A., Cohen-Sánchez A., Pinya S., Tejada S., Ferriol P., et al. (2022). Effects of human activity on markers of oxidative stress in the intestine of Holothuria tubulosa, with special reference to the presence of microplastics. Int. J. Mol. Sci. 23 (16), 9018. doi: 10.3390/ijms23169018
Lebreton L., Slat B., Sainte-Rose B., Aitken J., Marthouse R, Hajbane S., et al. (2018). Evidence that the Great Pacific Garbage Patch is rapidly accumulating plastic. Sci. Rep. 8 (1), 4666. doi: 10.1038/s41598-018-22939-w
Leray M., Yang J. Y., Meyer C. P., Mills S. C., Agudelo N., Ranwez V., et al. (2013). A new versatile primer set targeting a short fragment of the mitochondrial COI region for metabarcoding metazoan diversity: Application for characterizing coral reef fish gut contents. Front. Zool 10 (1), 34. doi: 10.1186/1742-9994-10-34
Lestari P., Trihadiningrum Y., Wijaya B. A., Yunus K. A., Firdaus M. (2020). Distribution of microplastics in surabaya river, Indonesia. Sci. Total Environ. 726, 138560. doi: 10.1016/j.scitotenv.2020.138560
Liebezeit G., Dubaish F. (2012). Microplastics in beaches of the East Frisian islands Spiekeroog and Kachelotplate. Bull. Environ. Contamination Toxicol. 89, 213–217. doi: 10.1007/s00128-012-0642-7
Lusher A. L., Tirelli V., O’Connor I., Officer R. (2015). Microplastics in Arctic polar waters: The first reported values of particles in surface and sub-surface samples. Sci. Rep. 5 (1), 14947. doi: 10.1038/srep14947
Lorenzo-Navarro J., Castrillón-Santana M., Sánchez-Nielsen E., Zarco B., Herrera A., Martínez I., et al. (2021). Deep learning approach for automatic microplastics counting and classification. Sci. Total Environ. 765, 142728. doi: 10.1016/j.scitotenv.2020.142728
Marquina D., Fernández-Álvarez F.Á., Noreña C. (2015). Five new records and one new species of Polycladida (Platyhelminthes) for the Cantabrian coast (North Atlantic) of the Iberian Peninsula. J. Mar. Biol. Assoc. United Kingdom 95 (2), 311–322. doi: 10.1017/S0025315414001106
Marrugo-Negrete J., Pinedo-Hernández J., Marrugo-Madrid S., Navarro-Frómeta E., Díez S. (2021). Sea cucumber as bioindicator of trace metal pollution in coastal sediments. Biol. Trace Element Res. 199, 2022–2030. doi: 10.1007/s12011-020-02308-3
Martines A., Furfaro G., Solca M., Muzzi M., Di Giulio A., Rossi S. (2023). An Analysis of Microplastics Ingested by the Mediterranean Detritivore Holothuria tubulosa (Echinodermata: Holothuroidea) Sheds Light on Patterns of Contaminant Distribution in Different Marine Areas. Water 15 (8), 1597. doi: 10.3390/w15081597
Masiá P., Ardura A., Gaitán M., Gerber S., Rayon-Viña F., Garcia-Vazquez E. (2021). Maritime ports and beach management as sources of coastal macro-, meso-, and microplastic pollution. Environ. Sci. Pollut. Res. 28, 30722–30731. doi: 10.1007/s11356-021-12821-0
Masiá P., Ardura A., Garcia-Vazquez E. (2019). Microplastics in special protected areas for migratory birds in the Bay of Biscay. Mar. Pollut. Bull. 146, 993–1001. doi: 10.1016/j.marpolbul.2019.07.065
Masiá P., Ardura A., Garcia-Vazquez E. (2022). Microplastics in seafood: Relative input of Mytilus galloprovincialis and table salt in mussel dishes. Food Res. Int. 153, 110973. doi: 10.1016/j.foodres.2022.110973
Meijer L. J., Van Emmerik T., van der Ent R., Schmidt C., Lebreton L. (2021). More than 1000 rivers account for 80% of global riverine plastic emissions into the ocean. Sci. Adv. 7 (18), eaaz5803. doi: 10.1126/sciadv.aaz5803
Menéndez D., Álvarez A., Acle S., Peón P., Ardura A., Garcia-Vazquez E. (2022). Microplastics across biomes in diadromous species. Insights from the critically endangered Anguilla Anguilla. Environ. pollut. 305, 119277. doi: 10.1016/j.envpol.2022.119277
Mercier A., Hamel J. F. (2013). Holothuria mammata. The IUCN Red List of Threatened Species 2013. doi: 10.2305/IUCN.UK.2013-1.RLTS.T180514A1641636.en
Mohsen M., Lin C., Abdalla M., Liu S., Yang H. (2023). Microplastics in canned, salt-dried, and instant sea cucumbers sold for human consumption. Mar. pollut. Bull. 192, 115040. doi: 10.1016/j.marpolbul.2023.115040
Mohsen M., Lin C., Liu S., Yang H. (2022). Existence of microplastics in the edible part of the sea cucumber Apostichopus japonicus. Chemosphere 287, 132062. doi: 10.1016/j.chemosphere.2021.132062
Mohsen M., Zhang L., Sun L., Lin C., Wang Q., Liu S., et al. (2021). Effect of chronic exposure to microplastic fibre ingestion in the sea cucumber Apostichopus japonicus. Ecotoxicol Environ. Saf. 209, 111794. doi: 10.1016/j.ecoenv.2020.111794
Mohsen M., Zhang L., Sun L., Lin C., Wang Q., Yang H. (2020). Microplastic fibers transfer from the water to the internal fluid of the sea cucumber Apostichopus japonicus. Environ. pollut. 257, 113606. doi: 10.1016/j.envpol.2019.113606
Monira S., Roychand R., Bhuiyan M. A., Hai F. I., Pramanik B. K. (2022). Identification, classification and quantification of microplastics in road dust and stormwater. Chemosphere 299, 134389. doi: 10.1016/j.chemosphere.2022.134389
Muñoz-Colmenero M., Jeunen G. J., Borrell Y. J., Martinez J. L., Turrero. P., García-Vázquez E. (2015). Response of top shell assemblages to cyclogenesis disturbances. A case study in the Bay of Biscay. Mar. Environ. Res. 112 (Part B), 2–10. doi: 10.1016/j.marenvres.2015.06.012
Plee T. A., Pomory C. M. (2020). Microplastics in sandy environments in the Florida Keys and the panhandle of Florida, and the ingestion by sea cucumbers (Echinodermata: Holothuroidea) and sand dollars (Echinodermata: Echinoidea). Marine Pollution Bulletin. 158 111437. doi: 10.1016/j.marpolbul.2020.111437
Purcell S. W. (2014). Value, market preferences and trade of beche-de-mer from Pacific Island Sea cucumbers. PloS One 9 (4), e95075. doi: 10.1371/journal.pone.0095075
Purcell S. W., Lovatelli A., González-Wangüemert M., Solís-Marín F. A., Samyn Y., Conand C. (2023). Commercially important sea cucumbers of the world (Rome, Italy: FAO).
Renzi M., Blašković A., Bernardi G., Russo G. F. (2018). Plastic litter transfer from sediments towards marine trophic webs: a case study on holothurians. Mar. pollut. Bull. 135, 376–385. doi: 10.1016/j.marpolbul.2018.07.038
Riani M., Ducoli S., Depero L. E., Prica M., Tubić A., Ademovic Z., et al. (2023). A complete guide to extraction methods of microplastics from complex environmental matrices. Molecules 28 (15), 5710. doi: 10.3390/molecules28155710
Rios-Fuster B., Alomar C., Paniagua-González G., Garcinuño-Martínez R. M., Soliz-Rojas D. L., Fernández-Hernando P., et al. (2022). Assessing microplastic ingestion and occurrence of bisphenols and phthalates in bivalves, fish and holothurians from a Mediterranean marine protected area. Environ. Res. 214, 114034. doi: 10.1016/j.envres.2022.114034
Rochman C. M. (2018). Microplastics research—from sink to source. Science 360 (6384), 28–29. doi: 10.1126/science.aar7734
Seary R., Acasuso-Rivero C., Miliou A., Standerwick R., Demetriou M., Chuda D., et al. (2013). Multiple phase microplastic assessment of the Greek marine environment. Rapp Commun. Int. Mer. Medit. 40.
Rozas J., Rozas R. (1995). DnaSP, DNA sequence polymorphism: an interactive program for estimating population genetics parameters from DNA sequence data. Bioinformatics 11 (6), 621–625.
Sfriso A. A., Tomio Y., Rosso B., Gambaro A., Sfriso A., Corami F., et al. (2020). Microplastic accumulation in benthic invertebrates in Terra Nova Bay (Ross Sea, Antarctica). Environ. Int. 137, 105587. doi: 10.1016/j.envint.2020.105587
Simões T. D., Azevedo E., Silva F. H., Sousa J. N., Brito A. C., Pombo A., et al. (2019). “Ecological traits of sea cucumbers with commercial relevance from the north-eastern Atlantic coast,” in Front. Mar. Sci. Conference Abstract: IMMR’18 | International Meeting on Marine Research 2018. doi: 10.3389/conf.FMARS.2018.06.00147
Soliman T., Fernandez-Silva I., Reimer J. D. (2016). Genetic population structure and low genetic diversity in the over-exploited sea cucumber Holothuria edulis Lesson 1830 (Echinodermata: holothuroidea) in okinawa island. Conserv. Genet. 17 (4), 811–821. doi: 10.1007/s10592-016-0823-8
Steinberg R., van der Meer M., Walker E., Berumen M. L., Hobbs J. P. A., and van Herwerden L. (2016). Genetic connectivity and self-replenishment of inshore and offshore populations of the endemic anemonefish, Amphiprion latezonatus. Coral Reefs 35, 959–970. doi: 10.1007/s00338-016-1420-5
Tejedor-Junco M. T., Díaz V. C., González-Martín M., Tuya F. (2021). Presence of micr oplastics and antimicrobial-resistant bacteria in sea cucumbers under different anthropogenic influences in Gran Canaria (Canary Islands, Spain). Mar. Biol. Res. 17 (7-8), 537–544. doi: 10.1080/17451000.2021.1990960
Ugwu K., Herrera A., Gómez M. (2021). Microplastics in marine biota: A review. Mar. pollut. Bull. 169, 112540. doi: 10.1016/j.marpolbul.2021.112540
Uthicke S., Benzie J. A. H. (2001). Restricted gene flow between Holothuria scabra (Echinodermata: Holothuroidea) populations along the north-east coast of Australia and the Solomon Islands. Mar. Ecol. Prog. Ser. 216, 109–117. doi: 10.3354/meps216109
Uurasjärvi E., Sainio E., Setälä O., Lehtiniemi M., Koistinen A. (2021). Validation of an imaging FTIR spectroscopic method for analyzing microplastics ingestion by Finnish lake fish (Perca fluviatilis and Coregonus albula). Environ. pollut. 117780. doi: 10.1016/j.envpol.2021.117780
Vafidis D., Chryssanthi A. (2023). Holothurian fisheries in the hellenic seas: seeking for sustainability. Sustainability 15 (12), 9799. doi: 10.3390/su15129799
Valente S., Serrão E. A., González-Wangüemert M. (2015). West versus East Mediterranean Sea: Origin and genetic differentiation of the sea cucumber Holothuria poli. Mar. Ecol. 36 (3), 485–495. doi: 10.1111/maec.12156
Wang J., Lu L., Wang M., Jiang T., Liu X., Ru S. (2019). Typhoons increase the abundance of microplastics in the marine environment and cultured organisms: A case study in Sanggou Bay, China. Sci. Total Environ. 667, 1–8. doi: 10.1016/j.scitotenv.2019.02.367
Wu F., Pennings S. C., Tong C., Xu Y. (2020). Variation in microplastics composition at small spatial and temporal scales in a tidal flat of the Yangtze Estuary, China. Sci. Total Environ. 699, 134252. doi: 10.1016/j.scitotenv.2019.134252
Xue B., Zhang L., Li R., Wang Y., Guo J., Yu K., et al. (2020). Underestimated microplastic pollution derived from fishery activities and “hidden” in deep sediment. Environ. Sci. Technol. 54 (4), 2210–2217. doi: 10.1021/acs.est.9b04850
Zhang B., Wu D., Yang X., Teng J., Liu Y., Zhang C., et al. (2019). Microplastic pollution in the surface sediments collected from Sishili Bay, North Yellow Sea, China. Mar. pollut. Bull. 141, 9–15. doi: 10.1016/j.marpolbul.2019.02.021
Keywords: sea cucumbers, microplastics, marine pollution, mitochondrial DNA, population connectivity, Bay of Biscay
Citation: Menéndez D, Álvarez A, Garcia-Vazquez E and Ardura A (2024) Microplastics pollution in genetically connected populations of Holothuria forskali from south Bay of Biscay. Front. Mar. Sci. 11:1333141. doi: 10.3389/fmars.2024.1333141
Received: 04 November 2023; Accepted: 29 January 2024;
Published: 14 February 2024.
Edited by:
Ana Pombo, Center for Marine and Environmental Sciences (MARE- IPLeiria), PortugalReviewed by:
Chryssanthi Antoniadou, Aristotle University of Thessaloniki, GreeceGyda Christophersen, Møreforsking AS, Norway
Mohamed Mohsen, Jimei University, China
Copyright © 2024 Menéndez, Álvarez, Garcia-Vazquez and Ardura. This is an open-access article distributed under the terms of the Creative Commons Attribution License (CC BY). The use, distribution or reproduction in other forums is permitted, provided the original author(s) and the copyright owner(s) are credited and that the original publication in this journal is cited, in accordance with accepted academic practice. No use, distribution or reproduction is permitted which does not comply with these terms.
*Correspondence: Eva Garcia-Vazquez, egv@uniovi.es
†These authors share senior authorship