- 1The Interuniversity Institute for Marine Sciences in Eilat, Eilat, Israel
- 2Fredy and Nadine Hermann Institute of Earth Sciences, The Hebrew University of Jerusalem, Jerusalem, Israel
- 3Department of Ecology, Evolution, and Behavior, Alexander Silberman Institute of Life Sciences, The Hebrew University of Jerusalem, Jerusalem, Israel
Garden eels are elongated zooplanktivorous fish that live in colonies on sandy bottoms, often adjacent to coral reefs. Each eel digs its own burrow, from which it partially emerges to forage on drifting zooplankton while being “anchored” with its tail inside the burrow. Feeding rates and foraging movements were examined in the garden eel Gorgasia sillneri and compared with corresponding measurements carried out as part of this study and by (Genin et al.)1 with 3 species of “free”, site-attached coral-reef fish. Feeding rates by the garden eels were substantially lower than those of the free fish. In the eels, those rates monotonically increased with increasing current speed up to ~20 cm/s, whereas in the free fish maximum rates were observed under moderate flows. A nearly linear increase in feeding rate as function of prey density was observed in both the garden eels and the free fish. However, the slope of that increase in the eels was over an order of magnitude more gradual than that reported for the free fish. The different functional responses of the two fish groups appear to be related to their morphology and maneuverability capabilities. Being elongated, anchored in a burrow and able to modulate body posture according to the flow speed allow the eels high feeding rates under strong currents. The tradeoff, compared with free fish, include limited maneuverability, slower swimming, and smaller foraging volume, rendering the eels’ functional response less efficient to increasing prey density. This cost appears to be compensated by the eels’ ability to occupy sandy, shelter-less bottoms, which in some locations are immensely more abundant than coral-covered rocks, where most planktivorous free fish live.
1 Introduction
A nearly ubiquitous mode of foraging for zooplankton among coral-reef fishes is being site-attached (Hobson, 1991), where the fish feed on drifting plankton while maintaining a position near a shelter (Popper and Fishelson, 1973). Once a prey is detected, typically from a distance of 15-20 cm (Genin et al.)1, a short (a few cm) strike is initiated, at the end of which the prey is captured. In coral reefs, zooplanktivorous fish form “a wall of mouths” (Hamner et al., 1988), thereby an important pathway of nutrient subsidy to the coral reef ecosystem (Erez, 1990; Morais and Bellwood, 2019).
Garden eels are unique members of the guild of site-attached zooplanktivorous fish as they feed while being “anchored” to the seafloor. Each eel lives in a burrow that it digs in the sand (Smith, 1989). To feed, the eel emerges from the burrow but keeps the distal part of its body inside the burrow, that serves the eels as a shelter, into which it retreats during the night and when threatened. Garden eels are visual zooplanktivores, feeding on copepods, gelatinous zooplankton, arrow worms, and other zooplankton (Fricke, 1970; Smith, 1989; Donham et al., 2017). Prey is captured individually.
Feeding and foraging behavior of “free” (non-anchored) reef-dwelling planktivorous fish have been extensively studied. Their feeding rate is strongly affected by prey density, exhibiting a nearly linear functional response to increasing prey density (Noda et al., 1992; Kiflawi and Genin, 1997; Genin et al.1). No such response is observed for an increase in prey flux due to faster flow (Kiflawi and Genin, 1997; Genin et al.1). The latter effect is due to a biomechanical limitation on the maximum angle that the fish can orient its body sideways of the oncoming current: the stronger the current the closer the fish is heading directly onto the flow direction (Kiflawi and Genin, 1997). Consequently, the effective volume across which the fish strikes its prey becomes smaller (narrower) as flow speed increases.
The effects of prey density and current speed on feeding rates by garden eels were studied by Khrizman et al. (2018) and Ishikawa et al. (2022). Similar to free fish, the eel’s feeding rate monotonically increases with increasing prey densities. However, for the Red Sea Garden eel Gorgasia sillneri, unlike free fish, its feeding rates also increase with increasing current speeds. This deviation from the trend observed for free fish was attributed by to the ability of the eels to modulate their body posture to minimize drag forces exerted on them by the flowing water (Khrizman et al., 2018). While foraging in weak currents, the eels stretch their bodies out of the burrows, keeping them relatively straight and reaching to all directions. When the currents are strong, the eels minimize drag forces by a partial retreat into the burrows, curving the body in a posture resembling a question mark, and orient the head onto the currents (Khrizman et al., 2018).
Swimming performance and maneuverability are essential for foraging on drifting prey (Webb, 1984) and depend on morphology and deformation of body and fins (Webb, 1984; Webb, 1994; Webb, 2011; Lauder, 2015). Most planktivorous coral-reef fishes use their pectoral and pelvic fins (Fulton, 2007). Eels, on the other hand, use mostly their body to generate undulatory waves that propagate down the body through the caudal fin (Lauder, 2015). However, the anchored garden eels are unable to use their caudal fin, as free eels do, because that fin is kept inside the burrow as an anchor. While the two aforementioned fish groups reside at similar depths, feed on the same taxa, both are visual predators, and strike individual prey, garden eels and free fish have remarkably distinct morphologies and swimming mechanisms. The objectives of this study were to: (1) measure the feeding rates and the functional response of garden eels with respect to changes in prey density and current speed; (2) compare those functional responses with those reported by (Genin et al.)1 for free, site-attached fish; and (3) assess the differences of foraging movements and maneuverability between the garden eels and the free fish.
2 Materials and methods
In this study, we compare several attributes of plankton foraging among anchored garden eels and free, site-attached coral-reef fishes. The analyses of those attributed in the garden eels are based on our own work, the methods of which are detailed below. The corresponding information on the free fish is based on an earlier study at our laboratory (Genin et al.)1, the methods of which are briefly described at the end of this section. Note that our study with the garden eels was carried out in situ, whereas the work with the free fish was carried out both in a flume and in situ. The ensuing limitations of such a comparison are addressed below.
2.1 In situ foraging by garden eels
2.1.1 Study animal
The garden eel Gorgasia sillneri (subfamily Heterocongrinae, Klausewitz, 1962) is highly abundant throughout the Red Sea (Clark, 1980). It forms large colonies, consisting of hundreds of individuals (Clark, 1980), usually on sandy bottoms near or inside sea-grass meadows at depth of 4-55 m (Fricke, 1970; Clark, 1980). The typical length of males and females is 75-95 cm and 55-75 cm, respectively (Clark, 1980). Their body is nearly circular, 10-16 mm in diameter (Fricke, 1969; Fricke, 1970; Clark, 1980). Gorgasia species have a small pectoral fin and a dorsal fin that extends along the entire body (Klausewitz, 1962). Pelvic fins are absent and the caudal fins have evolved as a tool for burrowing (De Schepper et al., 2007). During foraging, the eels remain “anchored” to the bottom by keeping the distal ~25-30% of their body inside the burrow (Fricke, 1970), depending on the ambient current speed (Khrizman et al., 2018).
2.1.2 Study site
The field work was carried out in the oligotrophic northern Gulf of Aqaba, Red Sea (29°36’ N, 34°56’ E) during November 2015 - March 2017. A detailed description of the local reef and its environmental conditions is found in Reiss and Hottinger (1984); Yahel et al. (2002), and Genin et al. (2009). Data were collected at 6 –9 m depth at two sites: the “Lighthouse”, 0.4 km south of the Interuniversity Institute for Marine Sciences (IUI) and “Taba/Princess”, adjacent to the Israel-Egypt border crossing, ~1.4 km to the south. The habitat is exposed to oscillating, long-shore tidal currents (Monismith and Genin, 2004) with a mean speed of ~10 cm/s and a maximum of 50 cm/s (Genin and Paldor, 1998).
2.1.3 Feeding rates
Following Khrizman et al. (2018), feeding rates were measured by visually counting strikes, both in situ by divers and by counts obtained from video records. Concurrent estimates of feeding rates, using video (y) and direct counts (x), obtained for different individuals during the same runs, indicated a similarity between the two methods (y=1.16x; R2 = 0.96; N=11 runs; the paired t-test indicated a non-significant difference between the two methods P>0.05; Supplementary Information Figure S1). The results of the two methods were pooled and the relative average of both methods where applicable was used. Overall, counts were obtained for a total of 10-52 eels per session, during a 1 min long interval per eel (N=702; Table 1).
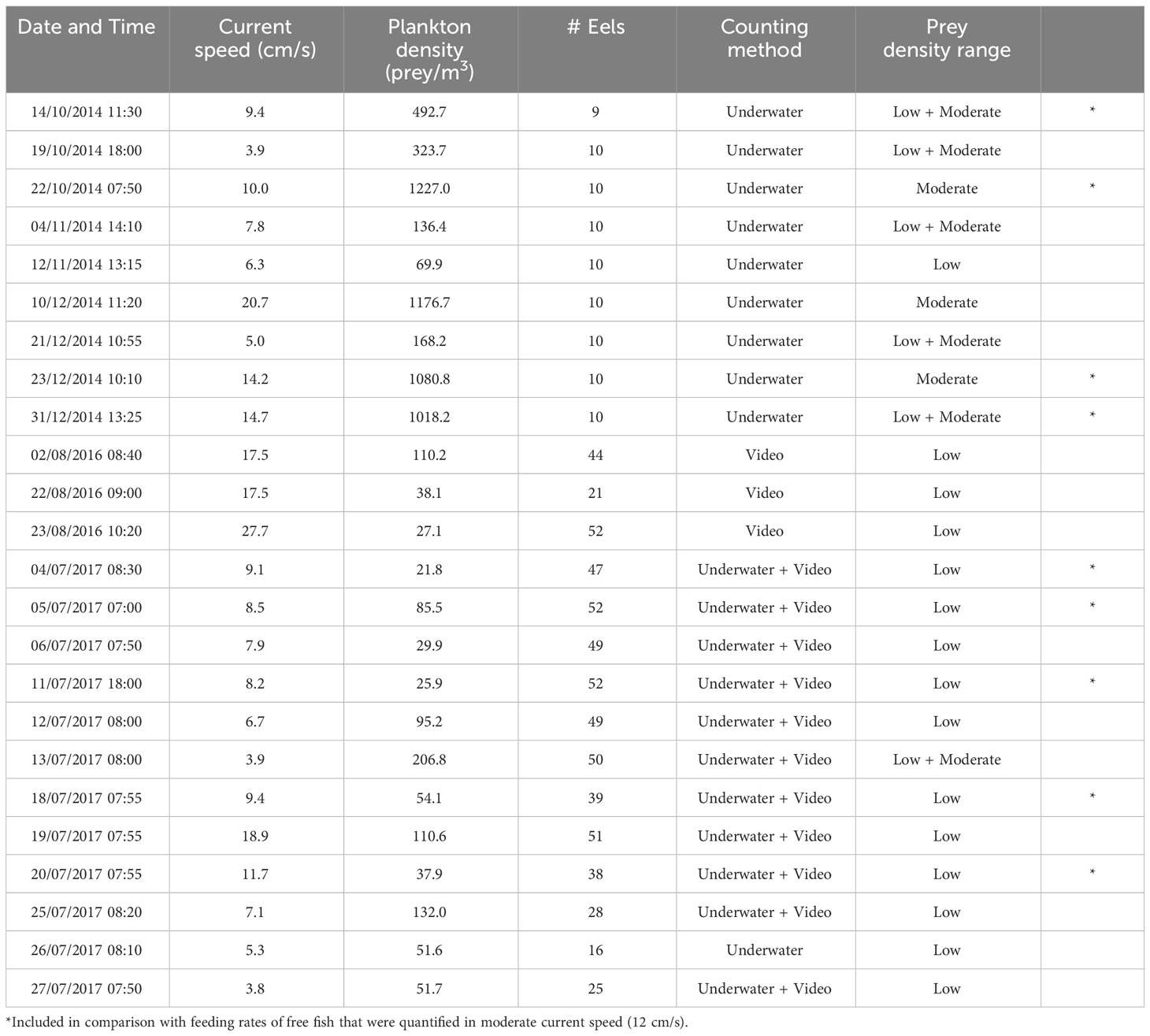
Table 1 Details on times, conditions and methods of the in-situ feeding experiments with the garden eel G.sillneri.
2.1.4 Prey density measurements
Zooplankton (prey) densities were measured in situ using a plankton net (200 μm mesh size; 50 cm mouth diameter) through two methods of operations: tows and stationary. For the first mode, the net was towed by 2 scuba divers for ca. 10 minutes up-current of the eel colony. Volume filtered by the net were measured using a TSK Flowmeter. For the stationary sampling, the net was moored 20 cm above the bottom upstream to the eel colony for a period of 30-60 minutes. Divers verified desired net extension during the sampling interval. Volume filtered were calculated based on the net’s mouth diameter, current speed measured during the deployment using a Aquadopp current meter (see below), and the filtering efficiency of the net under different current speeds. The net’s filtering efficiency was measured by comparing the ambient current speed with that at the mouth of the stationary net using two current meters (ADV, Sontek, Norway).
Despite the fact that both zooplankton and phytoplankton in the oligotrophic waters of the Gulf of Aqaba are small, both the eels and the free fish studied by (Genin et al.)1 and referred to in this study feed on zooplankters that are retained on 200 µm plankton net (A. Genin unpublished observations).
2.1.5 Current mesurements
Current velocities were measured concurrently with each video record, using a current profiler (2 MHz Aquadopp, Nortek, Norway). The profiler was placed on the bottom looking upward, using 0.4 m bin widths, starting at 0.2 m above bottom. Here we used the average of the two lowermost bins (0.2-1.0 m above seafloor), corresponding to the heights of the eels’ heads above bottom. Currents were recorded at 1 Hz and averaged over 2 min intervals.
2.1.6 Foraging movements
The foraging movements of garden eels were assessed from 3D reconstruction of the eels’ body shape and its change using in situ video records. Video records were acquired using two GoPro cameras positioned on a stand inside the eel colony. Videos were analyzed through DLTdv5 in Matlab (Hedrick, 2008) as described in Khrizman et al. (2018). Details regarding the method are provided in the Supplementary Information. The foraging movements calculated included (1) the volume of water across which the eel’s mouth moved (hereafter “foraging volume”), (2) the movement speed of the eel’s head (hereafter “swimming speed”), and (3) the orientation (angle) of the eel’s upper body with respect to the direction of the oncoming flow (hereafter “angle to the current”).
To calculate the foraging volume across which the eel foraged during a 20 min interval, we used the ~700 locations of the head recorded every 1.67 sec during that interval, yielding 3D “foraging polygons” of 6-15 neighboring eels in each interval (Figure 1, Supplementary Information Figure S2). The foraging volumes of a total of 79 eels were obtained during 8 different intervals collected from 6 video sessions (Supplementary Information Table S1). The speed of the head movements was calculated as distance divided by time between consecutive frames (intervals of 1.67 sec). A total of 36,158 speed calculations were made during 11 sessions (Supplementary Information Table S1), covering a wide range of current speeds (3-27 cm/s). To measure the angle of the eel’s upper body to the current direction, we digitized several points along the eel’s upper body and calculated the angle between the current direction and the direction of the eel’s upper section (Supplementary Information Figure S3). This analysis was performed for 3 eels every 10 sec during 3.5 min long sections, 3-9 sections per video session in each of 11 different sessions (Supplementary Information Table S1). The variance of the angles (in radians) was used as a proxy of the eels’ maneuverability.
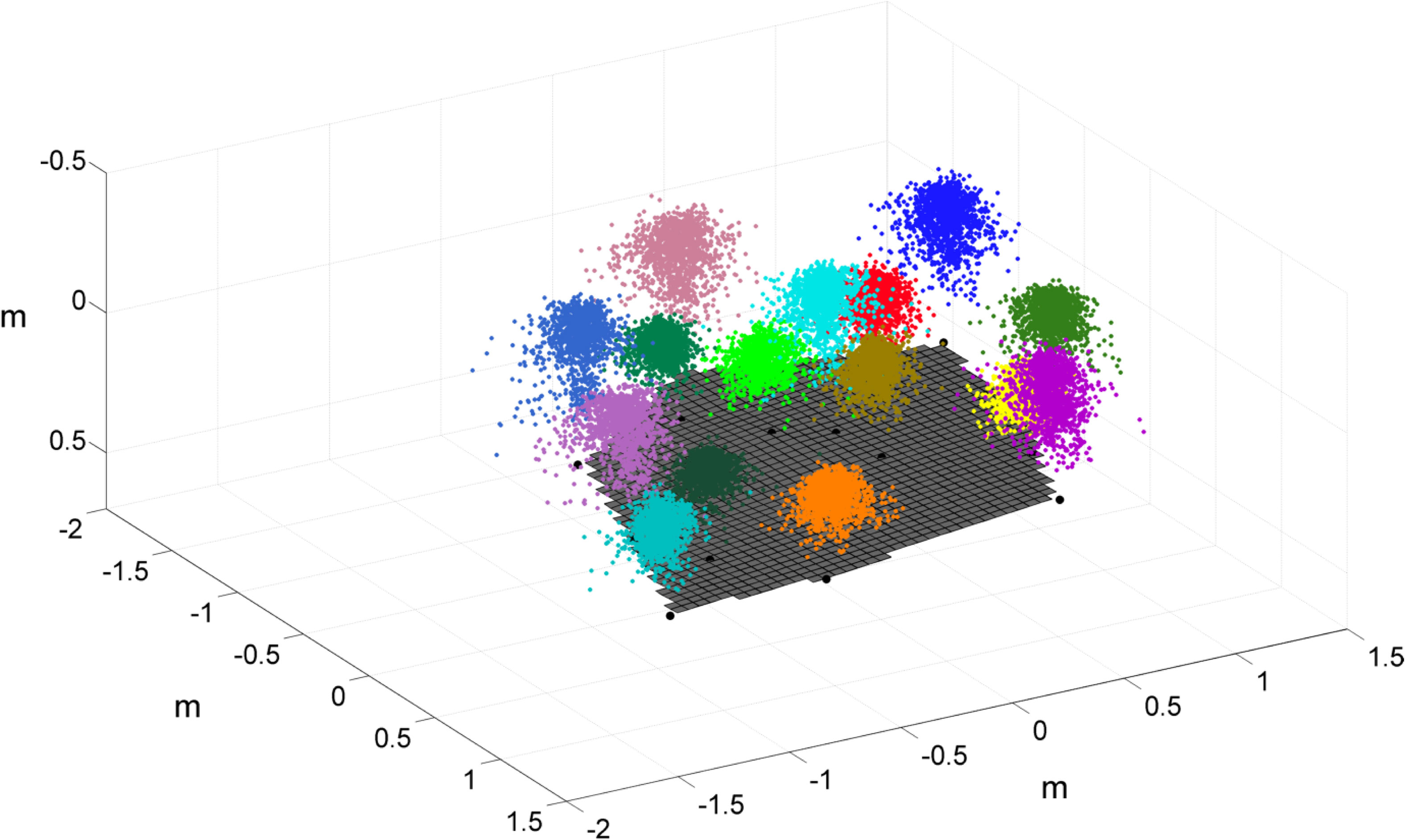
Figure 1 Reconstruction of foraging space of 15 garden eels during 40 min on 18/05/2016 at a depth of 7 m, under mean current speed of 7.5 cm/s. Each color indicates an individual eel, and each point indicates the location of its head in 1.67 sec. Black points - the eels’ burrows; grey surface - the seafloor.
2.2 Comparison with free fish
To compare the foraging behavior of the anchored eels with that of the free, site-attached planktivorous fish we used the data reported in by (Genin et al.)1 for the serranid Pseudanthias squamipinnis (Peters, 1855), and two pomacentrids - Dascyllus marginatus (Rüppell, 1829) and Neopomacentrus miryae Dor and Allen, 1977. P. squamipinnis is a common species in the Indo-Pacific Ocean, D. marginatus is endemic to the Red Sea, Gulf of Aden and Gulf of Oman, and N. miryae is endemic to the Red Sea. All three species are common in the Gulf of Aqaba (Brokovich, 2001) and reside in proximity to shelters provided by branching corals and perforated rocks.
2.2.1 Feeding rates
Feeding rates of the free fish were measured in a flume by (Genin et al.)1, whereas our measurements with the eels were carried out in situ. Since the effect of flow speed on feeding rates in the free fish was examined by (Genin et al.)1 under two prey densities (210 prey/m3 and 630 prey/m3), the data obtained for the eels were sorted into two groups covering similar conditions: one with a mean prey density of 113 prey/m3 (range: 22 – 493) and the other with a mean of 648 prey/m3 (range: 136 – 1227). Moreover, due to the absence of measurements under a combination of low current speeds and high prey densities, the effect of current speed on feeding rates by the garden eels was evaluated in the range of 8.2 – 17.5 cm/s (average of 11.8 cm/s), corresponding to the measurements of (Genin et al.)1 under a flow speed of 12 cm/s.
2.2.2 Body orientation
As part of our in-situ measurements, we recorded the angle to the flow, defined as the angle between the current direction and the longitudinal axis of the body (line connecting the fish’s snout and tail), for the free fish P. squamipinnis and D. marginatus. For these measurements, we deployed an up-looking video camera on the seafloor under a group of ~15 D. marginatus inhabiting the branching coral Stylophora pistilla and under a group of ~50 P. squamipinnis inhabiting a large knoll at 8-10 m depth in the coral reef off IUI. Current speed and direction were concurrently recorded using a S4 current meter (InterOcean, San Diego, USA) positioned at the height above bottom corresponding to the center of the fish group. The snout and the base of the tail of each of several, best seen fish were digitized in sequences of 30 frames each obtained during different days, covering flow speeds of 3-28 and 1.6-34 cm/s for P. squamipinnis (total N=3023 records) and D. marginatus (N =3025), respectively.
2.3 Concurrent tracking of garden eels and free fish
Our comparison of the foraging behavior between the eels and free fish benefitted from a single session (26 Dec. 2016) in which a branching coral (Pocillopora spp.) with 3 D. marginatus fish was recorded simultaneously with neighboring garden eels (Figure 2). This in situ record was used to compare the concurrent swimming speed, foraging volume, and nearest neighbor distances of the two taxa under identical conditions. Foraging volume, defined as the volume of the water across which the fish moved, and its swimming speed, both in 3D, were based on digitizing the precise location of the fish’s snout every 50 frames (1.67 sec).
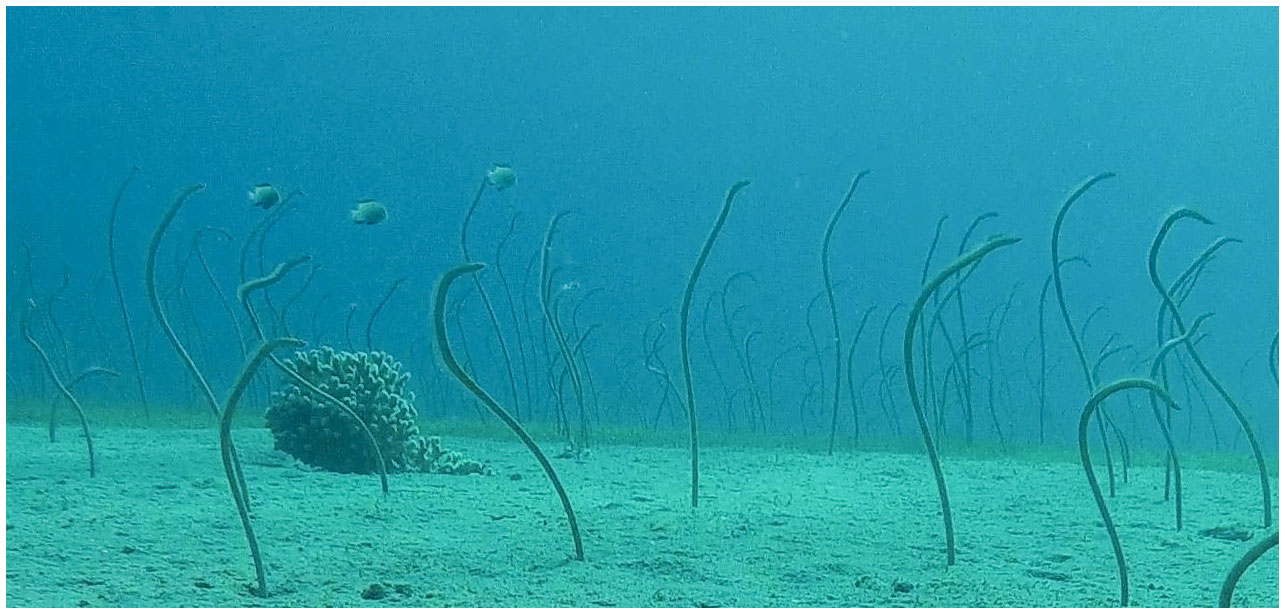
Figure 2 A photo taken from the simultaneous video recording of the eels together with 3 D. marginatus on 26 Dec. 2016 at a depth of 8 m.
2.4 Statistical analysis
Statistical tests were performed using SYSTAT (V. 13) and Matlab (R2017b). Regression analyses were used to test the effects of prey density and current speed on feeding rates and the effect of current speed on several attributes of the fish’s swimming angle in respect to the current direction. Since the latter relationships were nearly logarithmic, the regression analysis was performed on log-transformed data. Difference among the distributions of swimming speed under different conditions of current speeds in garden eels were examined using the Kolmogorov-Smirnov test. Differences in nearest-neighbor distances, foraging volumes and head movement speeds (swimming speeds) between G. sillneri and D. marginatus were tested using a two-sample t-test assuming unequal variances.
3 Results
3.1 Feeding rates
In G. sillneri, feeding rates monotonically increased with increasing prey densities. For moderate current speeds (8-14 cm/s), the slope of that functional response was 0.007 (R2 = 0.77; Supplementary Information Table S2), reflecting an increase from feeding rates of 15-20 prey/min at low prey densities to feeding rates of 25 prey/min (Figure 3A). Feeding rates of G. sillneri monotonically increased with increasing current speeds up to 20 cm/s, maintaining approximately the same feeding rates under stronger currents. The increase in feeding rates with flow speed was observed under both low and moderate densities of prey (Figure 3, Supplementary Information Table S3).
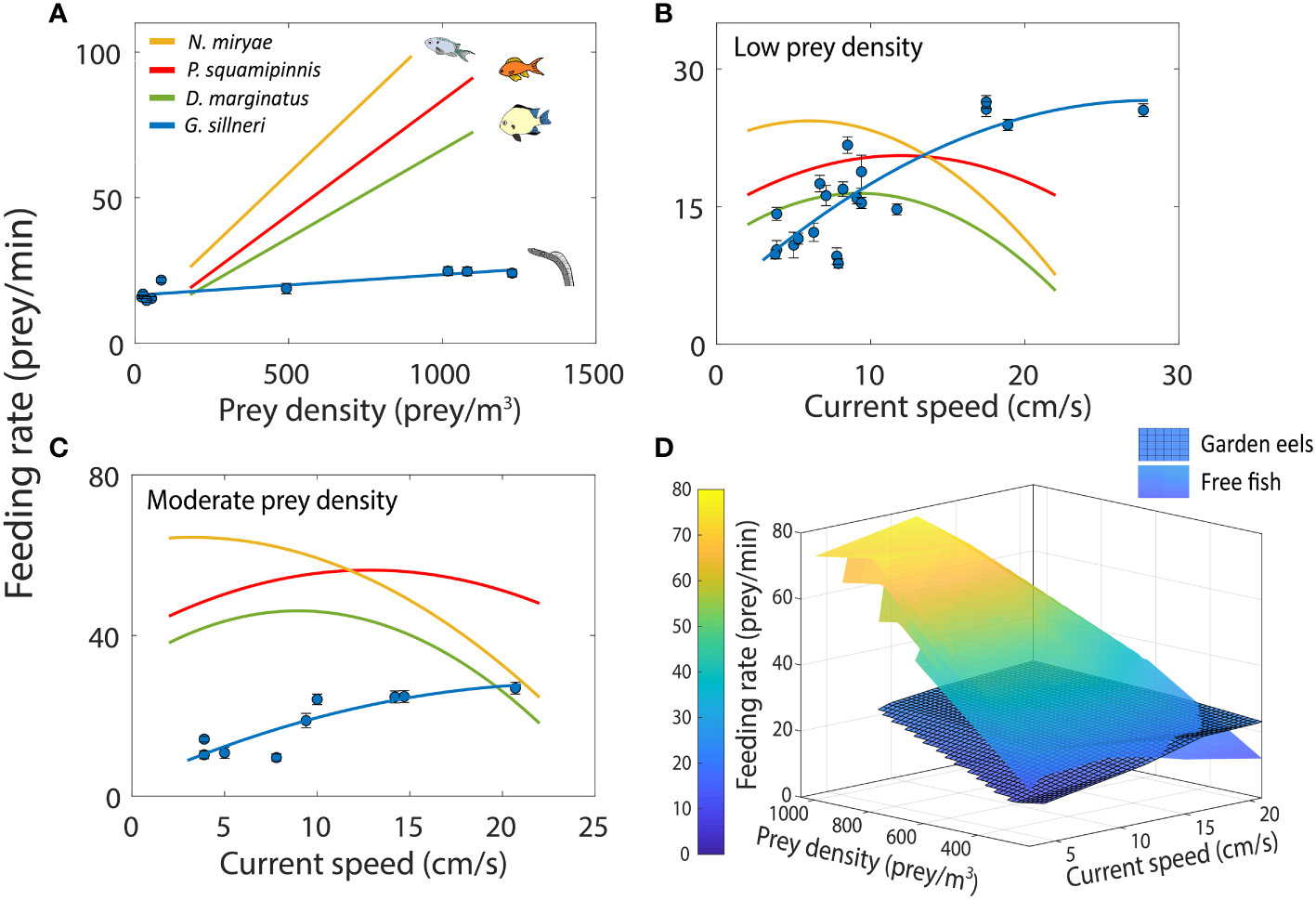
Figure 3 Feeding rates of garden eels (G. sillneri- blue) and free site-attached planktivorous fish (P. squamipinnis- red, D. marginatus- green, and N. miryae- yellow). (A) Feeding rates as a function of prey density for moderate current speed (12 cm/s for free fish and 8.2-14.7 cm/s for garden eels), (B) Feeding rates as a function of current speed at low prey density (210 prey/m3 for free fish and 27.8-492.7 prey/m3 for garden eels), (C) Feeding rates as a function of current speed at moderate prey density (630 prey/m3 for free fish and 136.4-1227.0 prey/m3 for garden eels), (D) Average feeding rates as a function of both current speed and prey density. For figures (A–C), error bars indicate standard error among individuals. Data for the free fish were obtained from (Genin et al.)1 (N=4 for P. squamipinnis, N=3 for D. marginatus and N. miryae, and 10<N<52 for G. sillneri).
3.2 Foraging movements
The mean orientation angle of the eels’ upper body with respect to the current direction (where 0° direction is facing directly onto the current) ranged 6.2° to 67.8°. Both the magnitude of that angle (Figure 4A) and its variance (Figure 4B) significantly decreased with increasing current speed (R2 = 0.53, P<0.00001 and R2 = 0.65, P<0.00001, respectively), with the decrease in the variance being ~2 times sharper than that of the magnitude. The decrease in the mean absolute angle and the variance with increasing current speeds were significantly more gradual in the eels than in each of the free fish (UNIANOVA – G. sillneri vs. D. marginatus P<0.02, P<0.001, and G. sillneri vs. P. squamipinnis P<0.003, P<0.001 for the mean angle and the variance, respectively). The differences in the slopes of D. marginatus and P. squamipinnis were significant for the variance (P<0.002) but not for the mean (Figure 4, Supplementary Information Table S4). The difference in the variance, reflecting the animal’s maneuverability, was most conspicuous under conditions of weak currents (3-10 cm/s; Figure 4B).
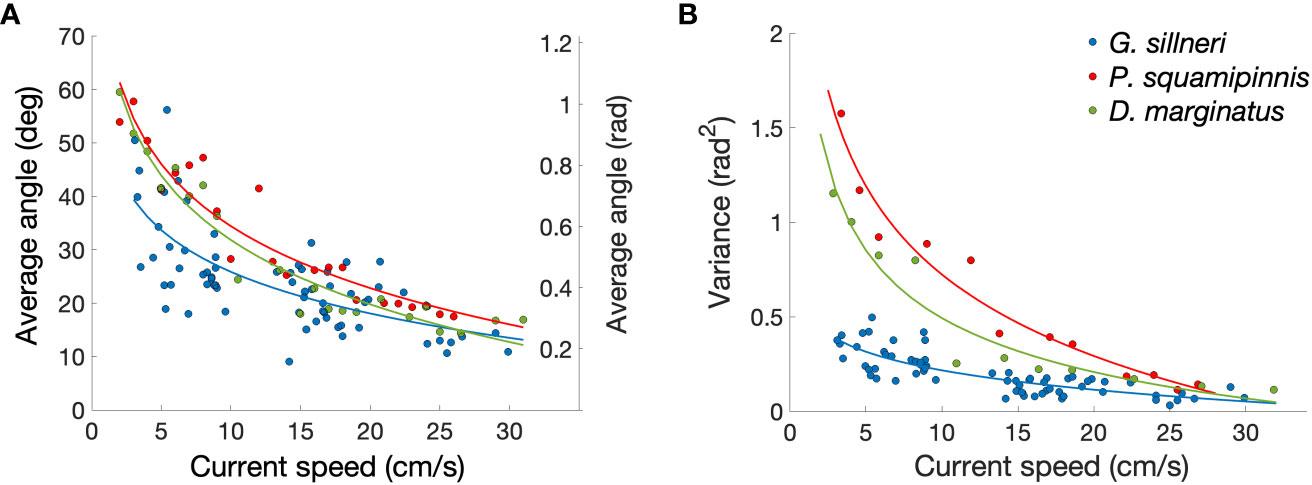
Figure 4 Angles between the fish’s body and the current direction in garden eels (G. sillneri-blue, N=4230, mean of 63 measurements per data point) and two free zooplanktivorous fish – P. squamipinnis (red, N=3023, mean of 144 measurements per data point) and D. marginatus (green, N=3025, mean of 97 measurements per data point). For the free fish, the axis was defined as the snout-tail line, while for the eels the axis was defined for the upper part of the body, as snout-maximum curvature line. (A) The average angle between the body main axis and the current direction. (B) The corresponding variance between the swimming angles.
The differences in the body orientation are shown in Supplementary Movie 1. During foraging under weak currents, both garden eels and free fish frequently changed their movement directions, although the frequency of those changes was markedly lower in G. sillneri. Under strong flows, P. squamipinnis were foraging with their head orientated directly onto flow most of the time. Under these conditions, a strike towards a plankter was often followed with a passive drift down current. For G. sillneri, such a down current drift was not observed since the strikes involved motions of the head only (not the entire body) in addition to the eels being “anchored” at the bottom.
The volume of the eels’ foraging space monotonically decreased with increasing current speed. Although the best fit was exponential (Figure 5; R2 = 0.35, N=75, P<0.0001), the large variance and wide range (3-90 L, CV = 0.51) rendered uncertain the mode of that change. The movement speed of the eels’ heads also decreased with increasing current speed (Figure 6A, R2 = 0.51; N=22; P<0.001), exhibiting a left-skewed distribution (slower head movements) under strong currents (Kolmogorov-Smirnov P<0.0001; Figure 6B).
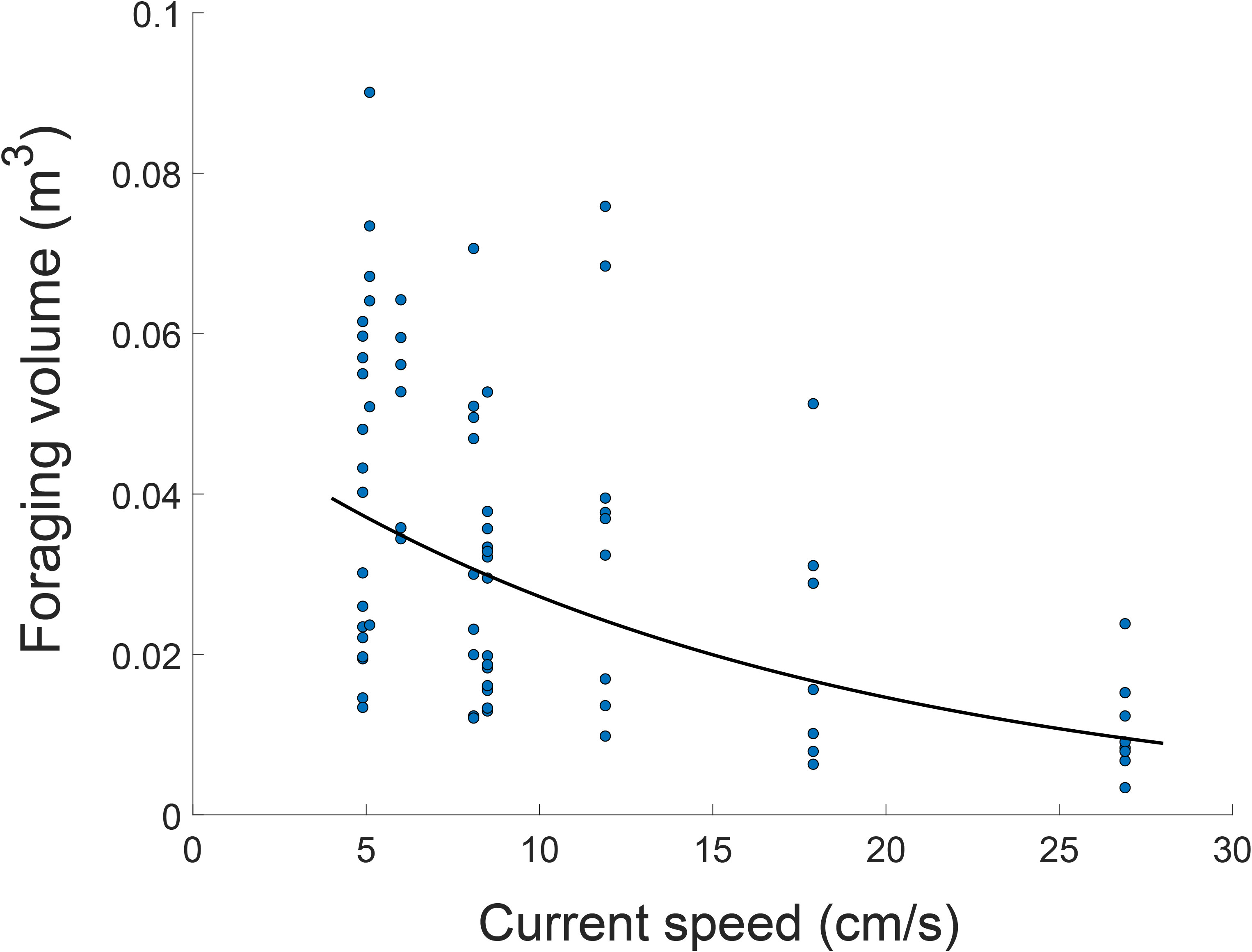
Figure 5 Foraging volume of garden eels as function of current speed. The best fit for the data (within confidence interval of 95%): y=0.0506*e-0.062*x, R2 = 0.345, P<0.000001.
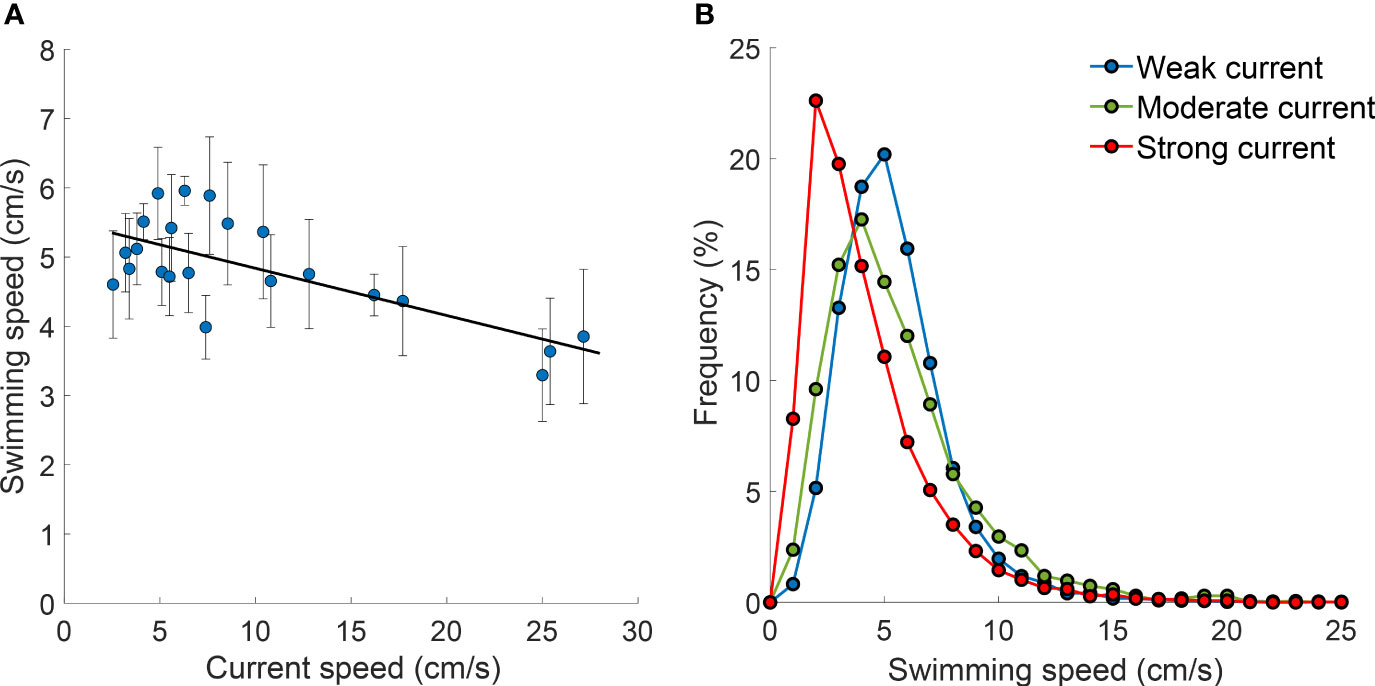
Figure 6 Swimming speed of garden eels as a function of current speed (A) Average swimming speed, defined as the speed of the eels’ head movements, examined in the ~10 min intervals. Error bars indicate sd. The linear fit to the data (within confidence interval of 95%): y=-0.0682*x+5.52, R2 = 0.51, N=22, P<0.001. (B) Frequency distribution of the eels’ swimming speed under three levels of average current speeds: weak (3.7 cm/s; blue), moderate (13.1 cm/s; green), and strong (26.1 cm/s; red).
When recorded together with the group of D. marginatus, the eels were significantly more separated one from another (Figure 7), with their nearest neighbor distance being on average x3 larger than the free fish (51.9 [ ± 13.0] and 17.1 [ ± 1.6] cm, respectively; two sample t-test P<0.0001; Figure 8A). The foraging volume of D. marginatus was 2.3 larger (0.032 [ ± 0.005] vs. 0.01 [ ± 0.004] m3; two sample t-test P<0.01; Figure 8B). D. marginatus swam 23% faster than the eels (4.9 vs 4.0 cm/s, respectively; two sample t-test P<0.0001; Figure 8C).
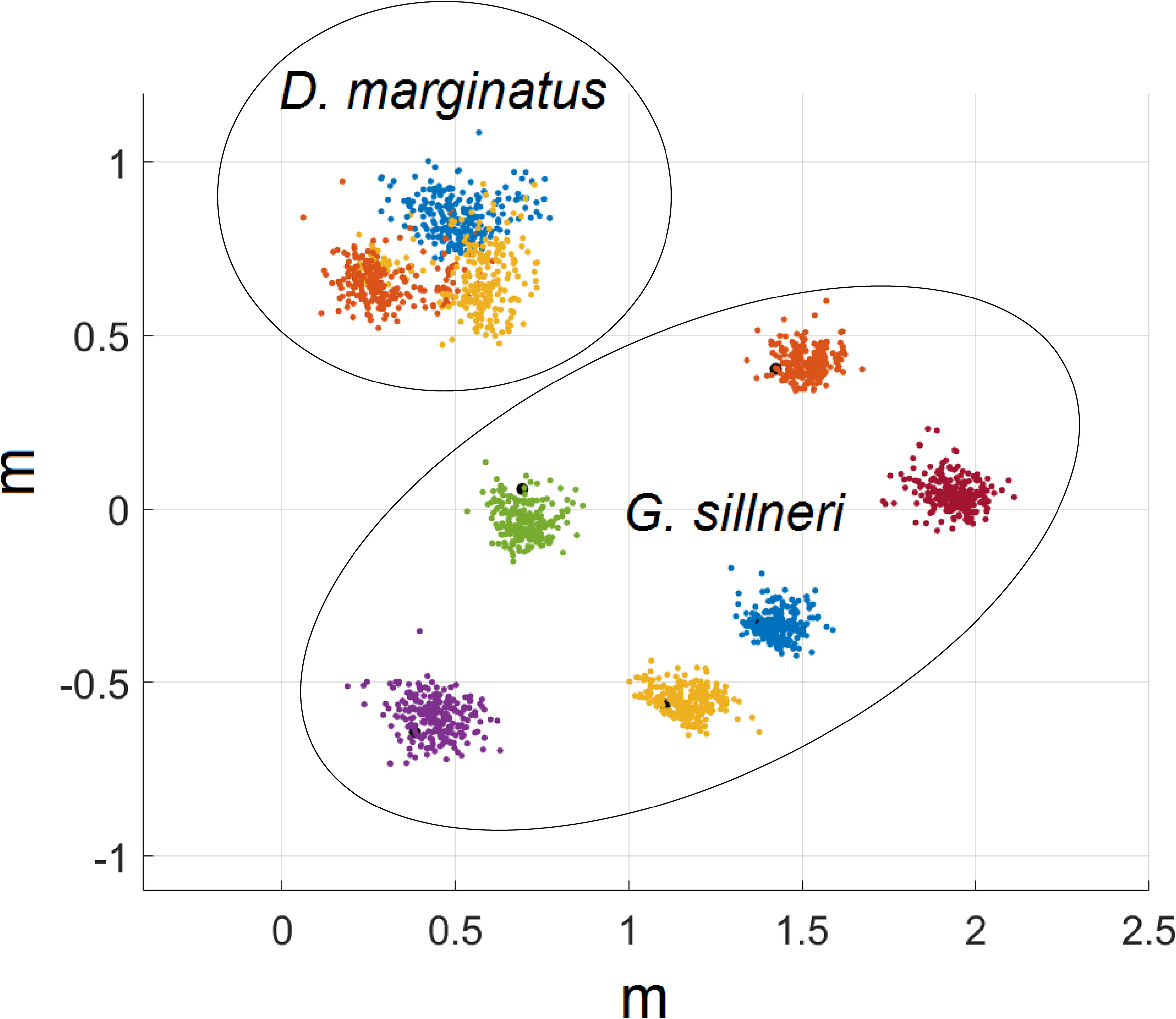
Figure 7 Foraging space of six garden eels (G. sillneri) and three free fish (D. marginatus) recorded simultaneously in situ under a current speed of 7.4 cm/s. Data points indicate the locations of the heads as viewed from above every 1.67 s during a time interval of 6.44 min. Each color indicates a different individual.
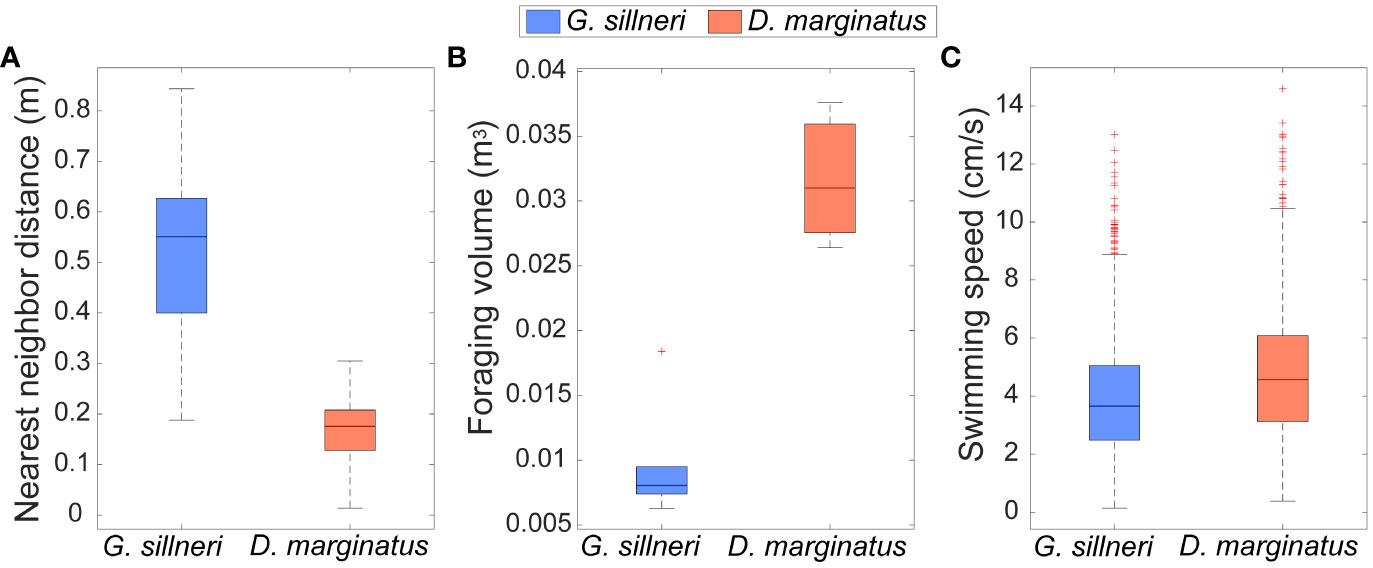
Figure 8 Box plot of the median (central mark), 25th and 75th percentiles (bottom and top edges), and range (whiskers) of the (A) nearest neighbor distance, (B) foraging volume, and (C) swimming speed of the six garden eels (blue bars) and three free fish (red) shown in Figure 7. Calculations were based on the locations of the heads recorded every 1.67 s during a time interval of 6.44 min. The difference between the garden eels and the free fish were significant for all the three parameters examined (p<0.001 for the nearest neighbor distance and swimming speed and p<0.01 for foraging volume; two sample t-test). Red dots indicate outliers.
4 Discussion
Both garden eels and the free fish are visual predators that capture drifting zooplankters while maintaining their position near a shelter: a burrow in the case of the garden eels and a branching coral or a complex rock in the case of the site-attached, free fish. The diets in both groups are similar, consisting mostly of copepods (Fricke, 1970; Smith, 1989; Donham et al., 2017).
While in the free fish, the effect of prey density on feeding rates was more pronounced than the effect of current speed, the opposite was found with the eels (Figure 3). Across the spectrum of prey densities and current speeds examined in our study and by (Genin et al.)1, feeding rates by the eels were substantially lower except for the combination of the strongest currents and lowest prey densities (Figure 3D). Note that for the free fish this comparison is based on measurements made in a flume using Artemia nauplii as prey (Genin et al.)1. Nevertheless, comparable feeding rates (22-40 bites/min) were found for Chromis dispilus, living in temperate rocky reefs (Kingsford and MacDiarmid, 1988). Also, a similarity between feeding rates measured in situ and in the flume was reported by (Genin et al.)1, who found that rates measured in the flume were similar to those based on in situ counts of bite rates in P. squamipinnis. Thus, the result of higher feeding rates of the free fish compared with the eels should hold.
The monotonic increase in feeding rates with increasing prey densities was found for both the free fish and the garden eels. However, the slope of this trend was 8-14 times steeper in the free fish (Figure 3A, Supplementary Information Table S2). For example, a doubling in prey density led to 1.84 – 1.89 times increase (92-94%) in the feeding rates of the free fish, compared with 1.21 times (60%) in the eels (Figure 3A, Supplementary Information Table S2). Similarly, a 3-fold increase in prey density led to nearly triplication of feeding rates in the free fish Chromis viridis (Kiflawi and Genin, 1997), compared with 1.4 times increase in the eels (Supplementary Information Table S2).
Why is the functional response to increasing prey density in the eels not as effective as that of the free fish? We suggest that the cause is the eels’ relatively poor maneuverability. In quasi-stationary fishes, fast movements and effective maneuverability are needed to rapidly capture zooplankters that drift with the flow, especially under conditions of high prey fluxes. The three species of free fish examined here, as well as C. viridis examined by Kiflawi and Genin (1997), are median paired fin swimmers, using mostly their pectoral fins for locomotion (Fulton and Bellwood, 2005; Fulton, 2007). This swimming mode is characterized by high maneuverability (Webb, 1994; Weihs, 2002). Garden eels are body-caudal fin swimmers, as most Anguilliformes are. Fish belonging to this group use body undulations (Blake, 2004; Lauder, 2015), generating a wave along their body to thrust themselves forward (Long et al., 1997). Moreover, unlike free eels, garden eels are incapable of using their posterior body part for movements because it is used as an anchor inside the burrow. Unfortunately, it was impossible to visualize the eels’ dorsal and pectoral fins, which may have a role in their foraging motion. Free fish accelerate and maneuver mostly by using fin strokes, overcoming external drag and viscosity forces. Anchored garden eels, on the other hand, move their body mostly by contracting and expanding the muscles along the body, the rapidity of which is limited internally, by the pace of muscle contraction and relaxation. The need to bend the body to reach a prey appears to impede maneuverability. This limitation is best reflected in the substantially lower variance of body angles with respect to the current direction in the eels compared with the free fish, especially under weak current speeds (Figure 4B).
As currents became stronger, garden eels decreased their foraging volume (Figure 5), became more narrowly oriented around the flow direction (Figure 4A), reduced the variance of that orientation (Figure 4B), and slowed down the speed of their head movements (Figure 6). Despite these effects, the eels’ functional response to increasing current speeds was surprisingly efficient, exhibiting a monotonous increase in feeding rates up to 20 cm/s (Figure 3). No such increase was observed in the free fish. On the contrary, above some threshold of relatively weak currents (6-12 cm/s), feeding rates by the free fish decreased with increasing current speed (Figure 3). In C. viridis (Kiflawi and Genin, 1997), a triplication of current speed (e.g., from 4 to 12 cm/s) did not increase feeding rates. In the eels such a triplication of current speed nearly doubled the feeding rate. The lack of increase in the feeding rates when prey flux increases due to faster currents in the free fish was explained in terms of biomechanical limitations on the fish’s orientation to the current (Hamner et al., 1988; Kiflawi and Genin, 1997). That is, as the currents become stronger, the orientation of the fish with respect to the flow gradually narrows down (Figure 4A) because the fish gradually minimizes the projection of its body side to the flow, presumably to avoid being swept down with the flow. In contrast, the garden eels are less likely to be swept down current because they are anchored to the bottom and are capable of modulating their body posture to minimize drag forces (Khrizman et al., 2018; Supplementary Movie S1). Also, as the cross section of an eel’s body is nearly circular, the drag force exerted on it by the flow does not vary much when the eel projects its side to the flow (Figure 4). Overall, the anchored and elongated garden eels appear to be better adapted to strong flows than the free fish. The effect of elongated morphology was also examined by Ishikawa et al. (2022) for another garden eel, Heteroconger hassi, which has a smaller body than G. sillneri (23 vs. 75 cm in length, respectively). H. hassi does not modulate its body posture to reduce the drag associated with stronger currents, which, in turn, led to non-increasing feeding rates under stronger flows (Ishikawa et al., 2022).
The opportunity to simultaneously record the eels and D. marginatus allowed us to directly compare their foraging movements under identical conditions. Figures 7, 8 showed that the free fish were significantly closer one to another, with their mean nearest-neighbor distance being 3 times smaller than that of G. sillneri and their mean foraging volume being 2.3 time larger. In addition, the head movements of the free fish were 23% faster. The relative crowdedness of the free fish was likely due to their association with a single coral that provided shelter for all members of the group. In the eels, on the other hand, each individual can select where to dig its burrow over a large sandy area, thereby balancing between living sufficiently separated one from another to reduce competition for food and yet sufficiently congregated to allow spawning, vigilance and other benefits of group living (Smith, 1989; Donham et al., 2017).
5 Conclusions
The anchored mode of feeding in garden eels, using burrows that they themselves dig, is unique among marine planktivorous fishes (e.g., Hobson and Chess, 1978; Sackley and Kaufman, 1996). This lifestyle enables the eels to occupy spacious, sandy bottoms that are, by and large, uninhabitable by side-attached planktivorous coral reef fishes. The ability of the eels to dig their burrows away from neighbors likely further lowers competition by reduction in intra-specific competition. The eels’ elongated morphology- a fundamental requirement for being planktivorous and living in a burrow - appears to limit their foraging maneuverability, with slower head movements and smaller foraging volumes, relative to free fish. On the other hand, the eels are capable of modulating their posture to effectively reduce drag forces imposed by strong currents (Khrizman et al., 2018), allowing their effective functional response to increasing flow speed. Free fish cannot achieve the latter because strong flows impose high drag forces that severely limit side-projection to the flow (Kiflawi and Genin, 1997).
We suggest that the aforementioned demarcation between garden eels and free fish presents a dichotomy between two different strategies related to planktivory among site-attached fishes. While free fish, having superb maneuverability, exhibit an effective functional response to increases in prey density but poor response to increasing flow speed, the anchored eels exhibit the opposite– effective response to increasing flow speed and relatively poor response to increases in prey density.
Data availability statement
The original contributions presented in the study are included in the article/Supplementary Material. Further inquiries can be directed to the corresponding author.
Ethics statement
The animal study was approved by Israel Nature and Parks Authority. The study was conducted in accordance with the local legislation and institutional requirements.
Author contributions
AK: Conceptualization, Data curation, Formal analysis, Investigation, Methodology, Software, Visualization, Writing – original draft, Writing – review & editing. IK: Data curation, Investigation, Writing – review & editing. DC: Data curation, Investigation, Writing – review & editing. AG: Conceptualization, Data curation, Formal analysis, Funding acquisition, Investigation, Methodology, Project administration, Resources, Supervision, Validation, Writing – review & editing.
Funding
The author(s) declare financial support was received for the research, authorship, and/or publication of this article. This research was funded by the Israel Science Foundation (grant 1211-14 to AG).
Acknowledgments
We thank Charlotte Wynn, Shir Bar, Yoav Lindeman and Anael Engel for their great help with the challenging work in the field and laboratory. We are grateful to the IUI staff for the assistance with logistics.
Conflict of interest
The authors declare that the research was conducted in the absence of any commercial or financial relationships that could be construed as a potential conflict of interest.
Publisher’s note
All claims expressed in this article are solely those of the authors and do not necessarily represent those of their affiliated organizations, or those of the publisher, the editors and the reviewers. Any product that may be evaluated in this article, or claim that may be made by its manufacturer, is not guaranteed or endorsed by the publisher.
Supplementary material
The Supplementary Material for this article can be found online at: https://www.frontiersin.org/articles/10.3389/fmars.2024.1330379/full#supplementary-material
Footnotes
- ^ Genin, A., Rickel, S., Zarubin, M., and Kiflawi, M. Effects of flow speed and prey density on the rate and efficiency of prey capture in 4 species of zooplanktivorous coral-reef fishes. Front. Mar. Sci. (Submitted to).
References
Blake R. W. (2004). Fish functional design and swimming performance. J. Fish Biol. 65 (5), 1193–1222. doi: 10.1111/j.0022-1112.2004.00568.x
Brokovich E. (2001). The community structure and biodiversity of reef fishes at the Northern Gulf of Aqaba (Red Sea) with relation to their habitat (Israel: Tel Aviv University). M.Sc. thesis (in Hebrew).
Clark E. (1980). Distribution, mobility, and behavior of the Red Sea garden eel. Natl. Geographic Res. Rep. 12, 91–102.
De Schepper N., De Kegel B., Adriaens D. (2007). Morphological specializations in Heterocongrinae (Anguilliformes: Congridae) related to burrowing and feeding. J. Morph. 268 (4), 343–356. doi: 10.1002/jmor.10525
Donham E., Foster M. S., Rice M. R., Cailliet G. M., Yoklavich M. M., Hamilton S. L. (2017). Natural history observations of Hawaiian Garden Eels, Gorgasia hawaiiensis (Congridae: heterocongrinae), from the island of Hawai’i. Pacific Sci. 71 (2), 135–147. doi: 10.2984/71.2.3
Erez J. (1990). On the importance of food sources in coral-reef ecosystems. Ecosyst. World 25, 411–418.
Fricke H. W. (1969). Biologie et compartementde Gorgasia sillneri (Klausewitz) et Taenioconger hassi (Klausewitz, Eibesfeldt). (Teleosteen). Comptes Rendus Hebdomadaires Des. Seances Del'Academie Des. Sci. 269 (17), 1678–1680.
Fricke H. W. (1970). Ökologische und verhaltensbiologische Beobachtungen an den Röhrenaalen Gorgasia sillneri und Taenioconger hassi (Pisces, Apodes, Heterocongridae). Z. für Tierpsychologie 27, 1076–1099. doi: 10.1111/j.1439-0310.1970.tb01918.x
Fulton C. J. (2007). Swimming speed performance in coral reef fishes: field validations reveal distinct functional groups. Coral Reefs 26 (2), 217–228. doi: 10.1007/s00338-007-0195-0
Fulton C. J., Bellwood D. R. (2005). Wave-induced water motion and the functional implications for coral reef fish assemblages. Limnol. Oceanogr. 50 (1), 255–264. doi: 10.4319/lo.2005.50.1.0255
Genin A., Monismith S. G., Reidenbach M. A., Yahel G., Koseff J. R. (2009). Intense benthic grazing of phytoplankton in a coral reef. Limnol. Oceanogr. 54 (3), 938–951. doi: 10.4319/lo.2009.54.3.0938
Genin A., Paldor N. (1998). Changes in the circulation and current spectrum near the tip of the narrow, seasonally mixed Gulf of Elat. Israel J. Earth Sci. 47, 87–92.
Hamner W. M., Jones M. S., Carleton J. H., Hauri I. R., Williams D. M. (1988). Zooplankton, planktivorous fish and water movement on windward reef-face: Great Barrier Reef, Australia. Bull. Mar. Sci. 42, 459–479.
Hedrick T. L. (2008). Software techniques for two-and three-dimensional kinematic measurements of biological and biomimetic systems. Bioinsp. Biomim. 3 (3), 034001. doi: 10.1088/1748-3182/3/3/034001
Hobson E. S. (1991). “Trophic relationships of fishes specialized to feed on zooplankters above coral reefs,” in The ecology of fishes on coral reefs (San Diego: Academic Press), 69–95.
Hobson E. S., Chess J. R. (1978). Trophic relationships among fishes and plankton in the lagoon at Enewetak Atoll, Marshall Islands. Fish. Bull. 76 (1), 133–153.
Ishikawa K., Wu H., Mitarai S., Genin A. (2022). Effects of prey density and flow speed on plankton feeding by garden eels: a flume study. J. Exp. Biol. 225 (8), jeb243655. doi: 10.1242/jeb.243655
Khrizman A., Ribak G., Churilov D., Kolesnikov I., Genin A. (2018). Life in the flow: unique adaptations for feeding on drifting zooplankton in garden eels. J. Exp. Biol. 221 (16). doi: 10.1242/jeb.179523
Kiflawi M., Genin A. (1997). Prey flux manipulation and the feeding rates of reef-dwelling planktivorous fish. Ecology 78 (4), 1062–1077. doi: 10.1890/0012-9658(1997)078[1062:PFMATF]2.0.CO;2
Kingsford M. J., MacDiarmid A. B. (1988). Interrelations between planktivorous reef fish and zooplankton in temperate waters. Mar. Ecol. Prog. Ser. Oldendorf 48 (2), 103–117. doi: 10.3354/meps048103
Klausewitz W. (1962). Gorgasi sillneri, ein neuer Rohrenaal aus dem Roten Meer. Senckenbergiana Biologica 43 (6), 433–435.
Lauder G. V. (2015). Fish locomotion: recent advances and new directions. Annu. Rev. Mar. Sci. 7, 521–545. doi: 10.1146/annurev-marine-010814-015614
Long J. H. Jr, Shepherd W., Root R. G. (1997). “Maneuverability and reversible propulsion: How eel-like fish swim forward and backward using travelling body waves,” in Proc. Special Session on Bio-Engineering Research Related to Autonomous Underwater Vehicles, 10th Int. Symp. Unmanned Untethered Submersible Technology. Arlington, VA: Office of Naval Research. 118–134.
Monismith S. G., Genin A. (2004). Tides and sea level in the Gulf of Aqaba (Eilat). J. Geophys. Res. 109, C04015. doi: 10.1029/2003JC002069
Morais R. A., Bellwood D. R. (2019). Pelagic subsidies underpin fish productivity on a degraded coral reef. Curr. Biol. 29 (9), 1521–1527. doi: 10.1016/j.cub.2019.03.044
Noda M., Kawabata K., Gushima K., Kakuda S. (1992). Importance of zooplankton patches in foraging ecology of the planktivorous reef fish Chromis chrysurus (Pomacentridae) at Kuchinoerabu Island, Japan. Mar. Ecol. Prog. Ser. 87, 251–263. doi: 10.3354/meps087251
Popper D., Fishelson L. (1973). Ecology and behavior of Anthias squamipinnis (Peters 1855) (Anthiidae, Teleostei) in the coral habitat of Eilat (Red Sea). J. Exp. Zool. 184 (3), 409–424. doi: 10.1002/jez.1401840314
Reiss Z., Hottinger L. (1984). “The Gulf of Aqaba,” in Ecological Micropaleontology (Berlin, Heidelberg: Springer-Verlag).
Sackley P. G., Kaufman L. S. (1996). Effect of predation on foraging height in a planktivorous coral reef fish, Chromis nitida. Copeia 1996 (3), 726–729. doi: 10.2307/1447539
Smith D. G. (1989). “Family congridae,” in Fishes of the western North Atlantic. Part 9, vol. 1 . Ed. Böhlke E. B. (New Haven, CT: Sears Foundation for Marine Research), 460–567.
Webb P. W. (1984). Body form, locomotion and foraging in aquatic vertebrates. Am. Zool. 24 (1), 107–120. doi: 10.1093/icb/24.1.107
Webb P. W. (1994). “The biology of fish swimming,” in Mechanics Physiology of Animal Swimming. Eds. Maddock L., Bone Q., Rayner J. V. (Cambridge: Cambridge University Press), 45–62.
Webb P. W. (2011). “Buoyancy, locomotion, and movement in fishes: maneuverability,” in Encyclopedia of fish physiology: from genome to environment. Ed. Farrell A. P. (London, UK: Academic Press), 575–580.
Weihs D. (2002). Stability versus maneuverability in aquatic locomotion. Integr. Comp. Biol. 42 (1), 127–134. doi: 10.1093/icb/42.1.127
Keywords: foraging, movement, flow, prey density, Red Sea, niche
Citation: Khrizman A, Kolesnikov I, Churilov D and Genin A (2024) Zooplanktivory in garden eels: benefits and shortcomings of being “anchored” compared with other coral-reef fish. Front. Mar. Sci. 11:1330379. doi: 10.3389/fmars.2024.1330379
Received: 30 October 2023; Accepted: 29 January 2024;
Published: 04 March 2024.
Edited by:
Stuart Humphries, University of Lincoln, United KingdomReviewed by:
Iain M. Suthers, University of New South Wales, AustraliaStein Kaartvedt, University of Oslo, Norway
Copyright © 2024 Khrizman, Kolesnikov, Churilov and Genin. This is an open-access article distributed under the terms of the Creative Commons Attribution License (CC BY). The use, distribution or reproduction in other forums is permitted, provided the original author(s) and the copyright owner(s) are credited and that the original publication in this journal is cited, in accordance with accepted academic practice. No use, distribution or reproduction is permitted which does not comply with these terms.
*Correspondence: Alexandra Khrizman, a2hyaXptYW5Ac3RhbmZvcmQuZWR1
†Present address: Alexandra Khrizman, Department of Earth System Science, Doerr School of Sustainability, Stanford University, Stanford, CA, United States