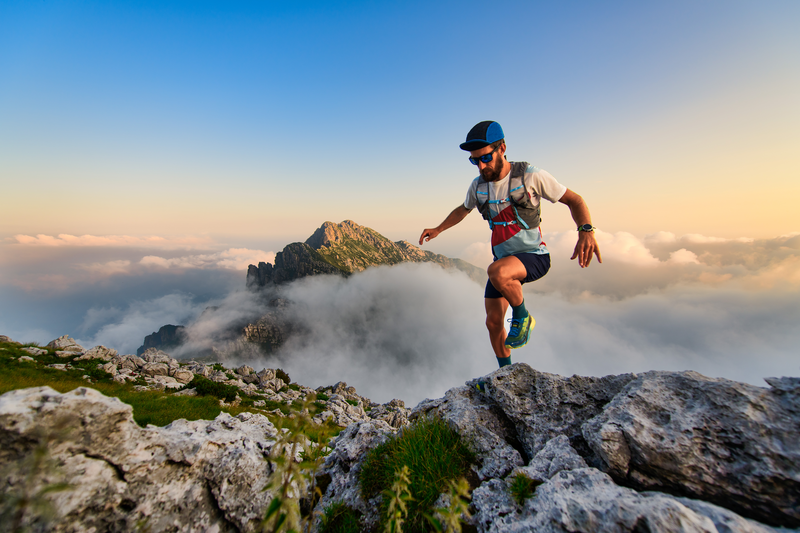
95% of researchers rate our articles as excellent or good
Learn more about the work of our research integrity team to safeguard the quality of each article we publish.
Find out more
BRIEF RESEARCH REPORT article
Front. Mar. Sci. , 19 April 2024
Sec. Discoveries
Volume 11 - 2024 | https://doi.org/10.3389/fmars.2024.1330118
This article is part of the Research Topic Novel Observations and Discoveries from the Red Sea View all 9 articles
Black corals (Hexacorallia: Antipatharia) are a major component of mesophotic and deep marine ecosystems. Due to their preference for light deprived environments, black corals have historically been considered azooxanthellate, yet recent works have found them in association with dinoflagellates of the family Symbiodiniaceae down to 396 m depth. While corals and Symbiodiniaceae generally establish a symbiotic relationship in shallow water environments, the implications of this association is less well understood at deeper depths, where low light penetration cannot sustain efficient photosynthetic activity for the algae. However, Symbiodinaceae are not obligate autotrophs, and their capacity for heterotrophic feeding categorizes them as mixotrophs. In this study, we investigated the presence and diversity of Symbiodiniaceae associated with the deep-sea black coral Bathypathes thermophila (Antipatharia: Schizopathidae), collected from 204 to 655 m depth in the Saudi Arabian Red Sea. Using high-throughput sequencing of the ITS2 region, we report (1) the deepest record to date of Symbiodiniaceae associated with an anthozoan from 655 m, and (2) the first Red Sea record of Antipatharia in association with Symbiodiniaceae. Our analyses revealed that 14 out of 27 colonies of B. thermophila were associated with Symbiodiniaceae of the genera Cladocopium and Durusdinium. We unveiled 16 novel ITS2 type profiles, possibly unique to black corals and/or to these depths, along with seven profiles that were already known from shallow-water hard corals. No significant pattern was detected in terms of community diversity in relation to depth or sampling locality. Our study supports the existence of black corals-Symbiodiniaceae association and warrants further research to better understand the evolutionary processes and physiological mechanisms driving this association, specifically in light deprived environments.
Antipatharia, commonly known as black corals, represent an order of anthozoan ubiquitous to all oceans. They are ahermatypic organisms typically found in low-light environments (Tazioli et al., 2007; Terrana et al., 2020). Their diversity and abundance increase along the bathymetric gradient (Tazioli et al., 2007), and they are considered crucial habitat formers in mesophotic and deep-ecosystems (Wagner et al., 2012; Bo et al., 2019). Among the seven Antipatharia families currently recognized, members of the families Antipathidae, Aphanipathidae, Leiopathidae, Myriopathidae, and Stylopathidae have adapted to live in both shallow and deep waters, while the families Cladopathidae and Schizopathidae include species predominantly found at bathyal depths (Brugler et al., 2013).
As opposed to their Scleractinia counterparts, which can form a symbiotic association with photosynthetic dinoflagellates of the family Symbiodiniaceae both in shallow and mesophotic waters, the preference of black corals to low-light and deep environments led to the assumption that they were azooxanthellate (Wagner et al., 2011). However, Symbiodiniaceae sequences of the genera Cladocopium and Durusdinium have been identified in association with ten Hawaiian black coral species ranging from 10 to 396 m depth (Wagner et al., 2011). In particular, Symbiodiniaceae sequences were encountered in the genera Bathypathes (Schizopathidae), Myriopathes (Myriopathidae), and Stichopathes (Antipathidae). Moreover, internal transcribed spacer 2 (ITS2) sequencing unveiled the presence of the genus Gerakladium in shallow water Cirrhipathes, within Indonesian waters (Bo et al., 2011). Histological analyses of various antipatharian species identified Symbiodiniaceae-like cells in the coral gastrodermis, indicating a potential endosymbiotic association (Bo et al., 2011; Wagner et al., 2011; Gress et al., 2021). However, the low algal density reported by Gress et al. (2021) (0-4 cells/mm3), and the light-deprived environment combined with the low density of algal symbionts found in the Antipatharia tissue by Wagner et al. (2011) (0-92 cells/mm3), led to hypothesize that a nutritional mutualism is unlikely to occur. This is in contrast with the high Symbiodiniaceae density detected by Bo et al. (2011) in shallow water Cirrhipathes (up to 10,000,000 cells/mm3), and the widely accepted notion that reef-building scleractinians typically engage in mutualistic relationships with algae.
Despite this apparently unconventional association at depths where photosynthesis cannot be sustained, several dinoflagellates exhibit heterotrophic or mixotrophic growth (Stoecker, 1999). Indeed, several studies have shown that Symbiodiniaceae metabolism is not strictly autotrophic (Jeong et al., 2012; Xiang et al., 2015; Jinkerson et al., 2022). Algae obtained from the environment and the tissue of the coral Alvepora japonica, exhibited heterotrophic feeding capabilities regardless of nutrient abundance (Jeong et al., 2012). Moreover, Xiang et al. (2015) revealed significant changes at the transcriptome level of the Symbiodiniaceae in response to different light conditions. In particular, the shift to dark light conditions induced profound alterations in the algae gene expression, affecting both light-responsive genes and those related to cell adhesion proteins, emphasizing the dynamic nature of the dinoflagellate metabolism in diverse light environments (Xiang et al., 2015). Algae of the family Symbiodiniaceae are not the only photosynthetic organisms that have been found below the compensation depth for photosynthesis. Grzymski et al. (2002) investigated the role of diatom chloroplasts in association with benthic foraminifera at 600 m depth, suggesting that the plastid role was to assimilate nitrate instead of inorganic carbon, as seen in the coral-Symbiodiniaceae symbiosis.
In the unique conditions of the Red Sea, where water masses are extremely oligotrophic, and light can penetrate up to 200 m depth (Fricke and Schuhmacher, 1983), no study has been conducted so far to examine the Antipatharia-Symbiodiniaceae association in the region. The Red Sea is a young ocean basin (Rasul et al., 2015) representing one of the hottest and most saline marine ecosystems in the world (Roder et al., 2013). It is characterized by extreme conditions, marked by temperature and salinity fluctuations due to latitudinal and bathymetric environmental gradients (Berumen et al., 2019; Manasrah et al., 2019). The Red Sea deep water temperature stabilizes with depth at 21°C (Roder et al., 2013; Qurban et al., 2014; Manasrah et al., 2019), a phenomenon attributed to the winter overturning occurring in the northern regions of the Gulf of Suez and Aqaba (Manasrah et al., 2004). Together with salinity around 40.5 (Roder et al., 2013), these conditions forge a unique and challenging habitat for organisms to adapt and thrive. Given the unique environmental conditions of the Red Sea, here, we investigated the presence of photoautotrophic dinoflagellates of the family Symbiodiniaceae in the deep-sea black coral Bathypathes thermophila. Moreover, we used high-throughput sequencing of the ITS2 region and provided the first characterization of the Symbiodiniaceae community associated with an Antipatharia from the Red Sea, from 204 to 665 m depth.
A total of 27 colonies of B. thermophila were collected during the Red Sea Deep Blue Expedition between October and November 2020 and during the Red Sea Decade Expedition between February and July 2022 on board the M/V OceanXplorer. Specimens were collected between 204 and 655 m depth (Table 1; Figure 1) using a combination of two Triton 3300/3 submersibles (Sub) with a Schilling T4 hydraulic manipulator, and Argus Mariner XL Remotely Operated Vehicle (ROV). After collection, the apical 10 cm was preserved in 99% ethanol for future molecular analyses. All collected specimens are preserved at King Abdullah University of Science and Technology - KAUST (Thuwal, Saudi Arabia).
Table 1 List of Bathypathes thermophila specimens analyzed in this study and environmental data at sampling.
Figure 1 (A) Map of the study area. (B) Sampling sites in the Red Sea. Black dots represent submersible and remotely operated vehicle (ROV) dives where colonies of Bathypathes thermophila have been sampled. Each number corresponds to a sample (listed in Table 1). (C) In situ picture of B. thermophila from Chimienti et al., 2022 (NTN028-6; corresponding to map’s reference number 2).
For the ROV and Sub dives, an RBR Maestro CTD was employed to record temperature, salinity, dissolved oxygen concentration, and depth at the sampling sites (Table 1).
Symbiodiniaceae genomic DNA was extracted using the QIAGEN DNeasy® Blood & Tissue Kit (Qiagen, Hilden, Germany), and the primers ITSintfor2 and ITS2-reverse (LaJeunesse, 2002) with Illumina adaptors were used to amplify the internal transcribed spacer-2 (ITS2) region of the dinoflagellate. Amplifications were conducted in a total volume of 25 µl, composed of 11 µl of 2X Qiagen Multiplex PCR Kit (Qiagen, Hilden, Germany), 2 µl of 10 µM primers, and 5 µl of DNA. The following PCR cycle profile was used: 15 min at 94°C, followed by 35 cycles of 94°C for 30 s, 52°C for 30 s, 72°C for 30 s, and a final extension step of 10 min at 72°C. PCR products were checked using a QIAxcel capillary electrophoresis machine, with the DNA Screening Kit (2400), a 3-kb marker, and the AM320 method (Qiagen, Hilden, Germany), revealing the successful amplification of ITS2 products in 20 specimens out of 27. Library preparation was performed for the 20 successfully amplified specimens, following the protocol described in Terraneo et al. (2023). The sequencing of the libraries was performed with the Illumina MiSeq platform (Illumina, San Diego, CA, USA) and kit reagents v3 (2×300 bp pair-ended reads) at KAUST Bioscience Core Lab (Thuwal, Saudi Arabia). Demultiplexed reads were uploaded to the SymPortal framework (https://SymPortal.org; Hume et al., 2019), following which we retrieved the post-MED ITS2 sequence relative abundances (Appendix 1) and the ITS2 profiles (proxies for Symbiodiniaceae genotypes) relative abundances (Appendix 2).
ITS2 amplification revealed the presence of Symbiodiniaceae in 20 out of 27 colonies of B. thermophila sampled (74%). Illumina Miseq successfully generated ITS2 sequences for 14 colonies (52%). Upon submission to SymPortal, the post-MED results yielded a total of 25 distinct ITS2 Symbiodiniaceae sequences found in association with B. thermophila, with 14 sequences belonging to the genus Cladocopium and 11 to the genus Durusdinium. The genus Cladocopium represented the 60% of the abundance of the Symbiodiniaceae community associated with the Red Sea endemic black coral, while Durusdinium the 40% (Figure 2, Appendix S1, S2). The two Symbiodiniaceae genera were recorded across the study area, from the Gulf of Aqaba to the South Red Sea, without exhibiting noticeable spatial distribution pattern.
Figure 2 Bar graphs (representing 100%) comparing the diversity of the Symbiodiniaceae community associated with Bathypathes thermophila at different levels: (A) genus, (B) ITS2 type profile. In bold are highlighted the new type profiles recovered. On the left, the Red Sea localities are indicated as follows: Gulf of Aqaba (GoA), North Red Sea (NRS), Central Red Sea (CRS), and South Red Sea (SRS). On the right side of the graph is presented the sampling depth for each sample.
Along the study depth range (204-655 m), Durusdinium was dominant below 600 m (except for a specimen collected at 230 m), while Cladocopium above 600 m (nine out of ten specimens). In seven of the 14 specimens, Cladocopium and Durusdinium co-occurred, whereas the remainder included sequences from a single genus (Figure 2). Four B. thermophila colonies from the North and central Red Sea were exclusively associated with Cladocopium while the remaining three from the central Red Sea with Durusdinium (Figure 2). From the SymPortal framework, a total of 23 distinct ITS2 type profiles were identified across all B. thermophila colonies (Figure 2C). Of these, 13 belonged to Cladocopium, and nine of them represented novel profiles (C1/C39/C1nf/C1b, C41/C1/C39, C65a, C1/C41-C1b, C3yg, C1od, C65, C1ii, C1l/C1-C42.2), whereas the remaining ten belonged to Durusdinium, with seven representing newly discovered profiles (D4c/D1, D1-D4f, D1lo, D1lh, D1ln, D4cc, D1/D2-D4-D4c-D1c). Overall, 34.5% and 31.5% of ITS2 profiles belonged to the C1 and D1 radiation, respectively. The remaining ones were associated with D4, C65, C41, C21, and C3 radiations. Among the retrieved type profiles, it was observed that the profiles C1/C39/C1nf/C1b and D4 were shared by two specimens (NTN0040-2 and NTN122BIO7 for D4, and NTN152BIO4 and NTN149BIO4 for C1/C39/C1nf/C1b). Conversely, all other profiles remained unique to individual specimens.
Our analyses also revealed that the five B. thermophila specimens collected in the restricted depth range spanning from 607 to 655 m were associated with novel ITS2 type profiles, all but one belonging to the genus Durusdinium (Figure 2).
In this study, we report for the first time the presence of Symbiodiniaceae in 20 colonies of B. thermophila from the Saudi Arabian Red Sea. Using high-throughput sequencing of the ITS2 region, we characterized the Symbiodiniaceae genotypes associated with the deep-specialist B. thermophila spanning depths from 204 to 655 m. Our findings unveiled sequences of Cladocopium and Durusdinium, with the first one representing the 60% of the Symbiodiniaceae community.
The distribution of Symbiodiniaceae interacting with shallow zooxanthellate corals along the Saudi Arabian coast can be influenced by the environmental gradients characterizing the Red Sea (Berumen et al., 2019). Salinity is higher in the North and lower in the South, while sea water temperature and chlorophyll values show an opposite trend (Berumen et al., 2019). A transition from the genus Cladocopium to the genus Durusdinium was reported by Terraneo et al. (2019) when moving from the North to the South of the basin in various Porites species. Likewise, a shift in the dominance of Cladocopium to Symbiodinium along the same latitudinal gradient was observed in the genera Pocillopora (Sawall et al., 2014) and Stylophora (Arrigoni et al., 2016). While these trends are reported from the shallow waters of the Red Sea (0-30 m), the same latitudinal shift in zooxanthellae was not encountered in the mesophotic zone (30-200m). Indeed, the results from Terraneo et al. (2023) and Vimercati et al. (2024) revealed a dominance of Cladocopium communities in mesophotic waters in five distinct scleractinian species, and the mesophotic-specialist Leptoseris cf. striatus, respectively. In the Gulf of Aqaba, Cladocopium has been also reported as the dominant Symbiodiniaceae genus associated with Stylophora pistillata (Ezzat et al., 2017) and various octocorals (Liberman et al., 2022) below 30 m depth. One possible explanation could be the relatively stable environmental conditions characteristic of mesophotic habitats compared to shallower water ones. Mesophotic zones often experience reduced environmental variability, including less fluctuation in temperature and light availability (Eyal et al., 2019) which may lead to a more consistent symbiotic association with Cladocopium across different latitudes.
The predominance of Cladocopium in low-light environments is attributed by Ezzat et al. (2017) to its higher efficiency in carbon fixation under reduced light conditions. In our study, we similarly found Cladocopium to be more abundant in light-deprived settings, representing the 60% of the Symbiodiniaceae abundance, however caution is warranted due to the small sample size. Among the ITS2 sequences linked with B. thermophila, the most prevalent belonged to the C1 radiation, constituting 34.5% of the Symbiodiniaceae community. The C1 radiation is widely distributed across different depths and has been previously found from shallow waters (< 30 m) down to 172 m depth (e.g., Hume et al., 2020; Osman et al., 2020; Rouzé et al., 2021; Terraneo et al., 2023; Vimercati et al., 2024). The genus is also highly abundant within the Gulf of Aqaba waters, the coldest region of the Red Sea, where sea surface temperatures fluctuate from 20°C in winter to 27°C in summer (Manasrah et al., 2019). Given that the Red Sea deep water temperature stabilizes at 21°C (Roder et al., 2013; Qurban et al., 2014; Manasrah et al., 2019), the high abundance of the C1 radiation at deeper depths found in our study might be explained by its preference for nutrient-limited and cooler waters (Sawall et al., 2014). Indeed, the environmental data obtained report comparable temperatures from the shallowest sampling point (204 m) to the deepest one (655 m) (22.1 ± 0.01 and 21.7 ± 0.001, respectively) (Table 1).
We identified eight distinct Durusdinium ITS2 type profiles, constituting 40% of the total retrieved sequences. This genus is commonly associated with shallow-water species in the Red Sea (Sawall et al., 2014; Ziegler et al., 2015; Terraneo et al., 2019; Hume et al., 2020). Other studies have explored the genetic diversity of Symbiodiniaceae communities associated with mesophotic scleractinian corals in the Red Sea, reporting the presence of Durusdinium in smaller percentage (Terraneo et al., 2023; Vimercati et al., 2024), consistently with what has been found outside of the basin (Wagner et al., 2011; Rouzé et al., 2021). Durusdinium is known for its presumed stress and thermal resilience (Baker, 2001; LaJeunesse et al., 2008; Wham et al., 2017) and is recognized for its opportunistic nature that sometimes yields detrimental impacts on coral health (Huang et al., 2020). However, colonies of Montastraea cavernosa hosting Durusdinium exhibited high survival rates when exposed to gradual warming to 35°C, and cooling to 15°C, revealing a broad temperature tolerance range (Silverstein et al., 2017). This adaptability likely contributes to the high abundance of various Durusdinium types at the considerable depths recorded in our study.
Remarkably, little research has focused on evaluating the genetic diversity of Symbiodiniaceae associated with zooxanthellate scleractinians, and this gap becomes even more pronounced for other taxa, including the order Antipatharia. Wagner et al. (2011) examined Hawaiian black corals collected at depths ranging from 10 to 396 m. From the analysis, they retrieved ITS2 sequences in 43% of the collected samples, revealing Symbiodiniaceae genotypes C15, C21, C26, C31, and limited occurrence of the genotype D1a. In another study, Bo et al. (2011) identified members of the Symbiodiniaceae genus Gerakladium within shallow-water Cirrhipathes sp. colonies in Indonesia. Our results indicate that the most common ITS2 sequence associated with B. thermophila belonged to the C1 and D1 radiations, occurring 34.5% and 31.5% times, respectively. We identified correspondences with previously encountered profiles spanning from shallow to mesophotic environments. The C21 genotype predominantly occurs within shallow water reefs in the Red Sea, where it associates with the Scleractinia S. pistillata (Hume et al., 2020), as well as in Belize with the hard coral M. cavernosa (Eckert et al., 2020). Again, the types C1/C1c and D1/D4 are associated with Porites and Pocillopora species, respectively, in the Malaysian reefs (Lee et al., 2022). The identification of the aforementioned types at depths greater than 300 m in this study indicates a potential opportunistic interaction with the coral holobiont. As already documented by Wagner et al. (2011), our findings provide further validation that black corals serve as a habitat for Symbiodiniaceae typically associated with shallow-water scleractinians.
Furthermore, the analyses of the Symbiodiniaceae ITS2 type profiles through SymPortal led to the discovery of 16 novel ITS2 type profiles, hinting at their potential restriction to the deep waters, and higlighting that the composition of Symbiodiniaceae communities may vary across different depths. Notably, among the five B. thermophila specimens collected at depths exceeding 600 m, each was found to be associated with distinct new type profiles. This suggests that they might have been selected to withstand peculiar environmental conditions present at such depths. However, a higher sampling effort is required to confirm the pattern observed in the present study.
The discovery of Symbiodiniaceae in association with a deep-sea species as B. thermophila challenges our understanding of the coral-algal association. It suggests that this partnership might be more versatile and adaptable than previously thought, and it needs to be better assessed in the broader context of the coral holobiont. This finding raises many further research questions. How do Symbiodiniaceae manage to thrive at such light-deprived depths? Do they provide any benefit to the coral, and what does the coral offer in return? It has largely been demonstrated that autotrophic nutrient uptake by Symbiodiniaceae experiences a notable decline in the lower mesophotic, as heterotrophy assumes a greater significance in fulfilling coral nutritional requirements (Kahng et al., 2019; Martinez et al., 2020; Ferrier-Pagès et al., 2022). In this study, members of the family Symbiodiniaceae were found below the depths where photosynthesis can be sustained, prompting a natural inquiry into how these dinoflagellates manage to thrive in a light-deprived environment (Wagner et al., 2011). Remarkably, Symbiodinaceae are not obligate autotrophs. They were reported to feed heterotrophically (Jeong et al., 2012), and therefore are better characterized as mixotrophs, with plastic transcriptomic responses to growth in low light or even darkness (Xiang et al., 2015). Specifically, Jeong et al. (2012) reported the absence of autotrophic grow in cultured free-living Symbiodiniaceae in nitrogen-depleted environments, yet observing growth when supplied with prey. This heterotrophic feeding ability may serve as a survival strategy in environments where photosynthesis cannot be sustained. Moreover, following an investigation of the physiological roles of diatom chloroplasts, Grzymski et al. (2002) found an association with benthic foraminifera at 600 m depth, and hypothesized that below the photic zone, algal endosymbionts could potentially utilize nitrate rather than inorganic carbon as an assimilatory source. Nitrate increases with depth in oligotrophic tropical and subtropical waters (Karl and Letelier, 2009; Kahng et al., 2014), and the same trend applies to the oligotrophic waters of the Red Sea (Triantafyllou et al., 2014). This physiological adaptation might occur when the coral holobiont faces limitations of reduced forms of accessible nitrogen. These findings together suggest a necessary revision of the generalized assumption that the association between zooxanthellae and their hosts is always symbiotic. Further research is essential to comprehensively delineate the nature of the Antipatharia-Symbiodiniaceae association at such depths and to uncover the physiological mechanisms enabling these symbionts to thrive in environments that do not sustain photosynthesis (Ferrier-Pagès et al., 2022). The hypothesis that antipatharians may act as a refuge for Symbiodiniaceae offers a new perspective, suggesting the potential for these associations to extend to other taxa at considerable depths.
In the present study, we provide the first-ever assessment of Symbiodiniaceae community diversity associated with the order Antipatharia in the Red Sea. Significantly, our research expanded the globally known bathymetric range for cnidaria-algae associations to 655 m. The findings indicate that the Symbiodiniaceae genera Cladocopium and Durusdinium observed across a wide depth range (204–655 m), can thrive within a broad range of environmental conditions. Moreover, the discovery of new Symbiodiniaceae types might be an indication of their potential exclusivity to deep environments, which highlights the importance of investigating zooxanthellate diversity to the whole order. There is a clear need for further studies encompassing histological and physiological analyses in order to understand the mechanisms and nature of the coral-Symbiodiniaceae relationship within this understudied order of coral.
The original contributions presented in the study are publicly available. This data can be found here: https://www.ncbi.nlm.nih.gov/sra/PRJNA1011006.
SV: Conceptualization, Data curation, Formal analysis, Investigation, Methodology, Software, Validation, Visualization, Writing – original draft, Writing – review & editing. TT: Conceptualization, Supervision, Validation, Writing – review & editing. CB: Methodology, Writing – review & editing. GC: Methodology, Writing – review & editing. NO: Supervision, Writing – review & editing. SV: Methodology, Writing – review & editing. BH: Writing – review & editing. FM: Methodology, Writing – review & editing. MN: Methodology, Writing – review & editing. AE: Data curation, Funding acquisition, Project administration, Resources, Writing – review & editing. SP: Writing – review & editing. MR: Data curation, Resources, Writing – review & editing. VP: Data curation, Funding acquisition, Project administration, Resources, Writing – review & editing. MQ: Funding acquisition, Project administration, Resources, Writing – review & editing. CD: Funding acquisition, Project administration, Resources, Writing – review & editing. FB: Conceptualization, Data curation, Funding acquisition, Resources, Supervision, Validation, Project administration, Writing – review & editing.
The author(s) declare financial support was received for the research, authorship, and/or publication of this article. This study was supported by KAUST (FCC/1/1973-49-01 and FCC/1/1973-50-01) and baseline research funds to FB. The Red Sea Deep Blue expedition was founded by NEOM. The Red Sea Decade Expedition (RSDE) was funded by the National Center for Wildlife (NCW) of Saudi Arabia.
We thank NEOM for facilitating and coordinating the Red Sea Deep Blue expedition and, specifically, in addition to AE, T. Habis, J. Myner, P. Marshall, G. Palavicini, P. Mackelworth, and A. Alghamdi. We thank National Center for Wildlife (NCW), M.Qurban, C. M. Duarte, J. E. Thompson, and N. C. Pluma Guerrero for facilitating and coordinating the Red Sea Decade Expedition. We want to thank OceanX and the crew of OceanXplorer for their operational and logistical support for the duration of this expedition. In particular, we would like to acknowledge the dive support, ROV, and submersible teams for sample collection, and OceanX for support of scientific operations on board OceanXplorer. We would also like to thank OceanX Media for documenting and communicating this work with the public. We also wish to thank Yulia Iakovleva for helping with the SymPortal sequences submission. Finally, we thank the KAUST Genomics Core Lab for helping with NGS.
The authors declare that the research was conducted in the absence of any commercial or financial relationships that could be construed as a potential conflict of interest.
All claims expressed in this article are solely those of the authors and do not necessarily represent those of their affiliated organizations, or those of the publisher, the editors and the reviewers. Any product that may be evaluated in this article, or claim that may be made by its manufacturer, is not guaranteed or endorsed by the publisher.
The Supplementary Material for this article can be found online at: https://www.frontiersin.org/articles/10.3389/fmars.2024.1330118/full#supplementary-material
Arrigoni R., Benzoni F., Terraneo T. I., Caragnano A., Berumen M. L. (2016). Recent origin and semi-permeable species boundaries in the scleractinian coral genus Stylophora from the Red Sea. Sci. Rep. 6, 34612. doi: 10.1038/srep34612
Baker A. C. (2001). Reef corals bleach to survive change. Nature 411, 765–766. doi: 10.1038/35081151
Berumen M. L., Voolstra C. R., Daffonchio D., Agusti S., Aranda M., Irigoien X., et al. (2019). “The Red Sea: Environmental gradients shape a natural laboratory in a nascent ocean,” in Coral reefs of the Red Sea (Springer, Cham: Springer International Publishing), 1–10. doi: 10.1007/978-3-030-05802-9_1
Bo M., Baker A. C., Gaino E., Wirshing H. H., Scoccia F., Bavestrello G. (2011). First description of algal mutualistic endosymbiosis in a black coral (Anthozoa: Antipatharia). Mar. Ecol. Prog. Ser. 435, 1–11. doi: 10.3354/meps09228
Bo M., Montgomery A. D., Opresko D. M., Wagner D., Bavestrello G. (2019). Antipatharians of the mesophotic zone: four case studies. Mesophotic Coral Ecosyst., 683–708. doi: 10.1007/978-3-319-92735-0_37
Brugler M. R., Opresko D. M., France S. C. (2013). The evolutionary history of the order Antipatharia (Cnidaria: Anthozoa: Hexacorallia) as inferred from mitochondrial and nuclear DNA: implications for black coral taxonomy and systematics. Zoological J. Linn. Soc. 169, 312–361. doi: 10.1111/zoj.2013.169.issue-2
Chimienti G., Terraneo T. I., Vicario S., Marchese F., Purkis S. J., Eweida A. A., et al. (2022). A new species of Bathypathes (Cnidaria, Anthozoa, Antipatharia, Schizopathidae) from the Red Sea and its phylogenetic position. ZooKeys 1116, 1. doi: 10.3897/zookeys.1116.79846
Eckert R. J., Reaume A. M., Sturm A. B., Studivan M. S., Voss J. D. (2020). Depth influences Symbiodiniaceae associations among Montastraea cavernosa corals on the Belize Barrier Reef. Front. Microbiol. 11. doi: 10.3389/fmicb.2020.00518
Eyal G., Tamir R., Kramer N., Eyal-Shaham L., Loya Y. (2019). The red sea: Israel. Mesophotic coral Ecosyst., 199–214.
Ezzat L., Fine M., Maguer J. F., Grover R., Ferrier-Pages C. (2017). Carbon and nitrogen acquisition in shallow and deep holobionts of the scleractinian coral S. pistillata. Front. Mar. Sci. 4. doi: 10.3389/fmars.2017.00102
Ferrier-Pagès C., Bednarz V., Grover R., Benayahu Y., Maguer J. F., Rottier C., et al. (2022). Symbiotic stony and soft corals: Is their host-algae relationship really mutualistic at lower mesophotic reefs? Limnology Oceanography 67, 261–271. doi: 10.1002/lno.11990
Fricke H. W., Schuhmacher H. (1983). The depth limits of Red Sea stony corals: an ecophysiological problem (a deep diving survey by submersible). Mar. Ecol. 4, 163–194. doi: 10.1111/j.1439-0485.1983.tb00294.x
Gress E., Eeckhaut I., Godefroid M., Dubois P., Richir J., Terrana L. (2021). “Investigation into the presence of symbiodiniaceae in antipatharians (Black corals),” in Oceans, vol. 2. (MDPI), 772–784. doi: 10.3390/oceans2040044
Grzymski J., Schofield O. M., Falkowski P. G., Bernhard J. M. (2002). The function of plastids in the deep-sea benthic foraminifer, Nonionella stella. Limnology oceanography 47, 1569–1580. doi: 10.4319/lo.2002.47.6.1569
Huang Y. Y., Carballo-Bolaños R., Kuo C. Y., Keshavmurthy S., Chen C. A. (2020). Leptoria phrygia in Southern Taiwan shuffles and switches symbionts to resist thermal-induced bleaching. Scientific Reports 10, 1–11. doi: 10.1038/s41598-020-64749-z
Hume B. C., Mejia-Restrepo A., Voolstra C. R., Berumen M. L. (2020). Fine-scale delineation of Symbiodiniaceae genotypes on a previously bleached central Red Sea reef system demonstrates a prevalence of coral host-specific associations. Coral Reefs 39, 583–601. doi: 10.1007/s00338-020-01917-7
Hume B. C., Smith E. G., Ziegler M., Warrington H. J., Burt J. A., LaJeunesse T. C., et al. (2019). SymPortal: A novel analytical framework and platform for coral algal symbiont next-generation sequencing ITS2 profiling. Mol. Ecol. Resour. 19, 1063–1080. doi: 10.1111/1755-0998.13004
Jeong H. J., Yoo Y. D., Kang N. S., Lim A. S., Seong K. A., Lee S. Y., et al. (2012). Heterotrophic feeding as a newly identified survival strategy of the dinoflagellate Symbiodinium. Proceedings of the National Academy of Sciences, 109 (31), 12604–12609. doi: 10.1073/pnas.1204302109
Jinkerson R. E., Russo J. A., Newkirk C. R., Kirk A. L., Chi R. J., Martindale M. Q., et al. (2022). Cnidarian-Symbiodiniaceae symbiosis establishment is independent of photosynthesis. Curr. Biol. 32, 2402–2415. doi: 10.1016/j.cub.2022.04.021
Kahng S. E., Akkaynak D., Shlesinger T., Hochberg E. J., Wiedenmann J., Tamir R., et al. (2019). Light, temperature, photosynthesis, heterotrophy, and the lower depth limits of mesophotic coral ecosystems. Mesophotic coral Ecosyst., 801–828. doi: 10.1007/978-3-319-92735-0_42
Kahng S. E., Copus J. M., Wagner D. (2014). Recent advances in the ecology of mesophotic coral ecosystems (MCEs). Curr. Opin. Environ. sustainability 7, 72–81. doi: 10.1016/j.cosust.2013.11.019
Karl D., Letelier R. (2009). “Marine habitats and conditions for microbial growth,” in Encyclopedia of Microbiology (Elsevier, Oxford), 258–277. doi: 10.1016/B978-012373944-5.00285-6
LaJeunesse T. J. M. B. (2002). Diversity and community structure of symbiotic dinoflagellates from Caribbean coral reefs. Mar. Biol. 141, 387–400. doi: 10.1007/s00227-002-0829-2
LaJeunesse T. C., Bonilla H. R., Warner M. E., Wills M., Schmidt G. W., Fitt W. K. (2008). Specificity and stability in high latitude eastern Pacific coral-algal symbioses. Limnology Oceanography 53, 719–727. doi: 10.4319/lo.2008.53.2.0719
Lee L. K., Leaw C. P., Lee L. C., Lim Z. F., Hii K. S., Chan A. A., et al. (2022). Molecular diversity and assemblages of coral symbionts (Symbiodiniaceae) in diverse scleractinian coral species. Mar. Environ. Res. 179, 105706. doi: 10.1016/j.marenvres.2022.105706
Liberman R., Benayahu Y., Huchon D. (2022). Octocorals in the Gulf of Aqaba exhibit high photosymbiont fidelity. Front. Microbiol. 13. doi: 10.3389/fmicb.2022.1005471
Manasrah R., Abu-Hilal A., Rasheed M. (2019). “Physical and chemical properties of seawater in the Gulf of Aqaba and Red Sea,” in Oceanographic and biological aspects of the Red Sea (Springer, Cham), 41–73. doi: 10.1007/978-3-319-99417-8_3
Manasrah R., Badran M., Lass H. U., Fennel W. (2004). Circulation and winter deep-water formation in the northern Red Sea. Oceanologia 46.
Martinez S., Kolodny Y., Shemesh E., Scucchia F., Nevo R., Levin-Zaidman S., et al. (2020). Energy sources of the depth-generalist mixotrophic coral Stylophora pistillata. Front. Mar. Sci. 7. doi: 10.3389/fmars.2020.566663
Osman E. O., Suggett D. J., Voolstra C. R., Pettay D. T., Clark D. R., Pogoreutz C., et al. (2020). Coral microbiome composition along the northern Red Sea suggests high plasticity of bacterial and specificity of endosymbiotic dinoflagellate communities. Microbiome 8, 1–16. doi: 10.1186/s40168-019-0776-5
Qurban M. A., Krishnakumar P. K., Joydas T. V., Manikandan K. P., Ashraf T. T. M., Quadri S. I., et al. (2014). In-situ observation of deep water corals in the northern Red Sea waters of Saudi Arabia. Deep Sea Res. Part I: Oceanographic Res. Papers 89, 35–43. doi: 10.1016/j.dsr.2014.04.002
Rasul N. M., Stewart I. C., Nawab Z. A. (2015). “Introduction to the Red Sea: its origin, structure, and environment,” in The Red Sea: The formation, morphology, oceanography and environment of a young ocean basin (Springer Berlin Heidelberg, Berlin, Heidelberg), 1–28. doi: 10.1007/978-3-662-45201-1
Roder C., Berumen M. L., Bouwmeester J., Papathanassiou E., Al-Suwailem A., Voolstra C. R. (2013). First biological measurements of deep-sea corals from the Red Sea. Sci. Rep. 3, 2802. doi: 10.1038/srep02802
Rouzé H., Galand P. E., Medina M., Bongaerts P., Pichon M., Pérez-Rosales G., et al. (2021). Symbiotic associations of the deepest recorded photosynthetic scleractinian coral (172 m depth). ISME J. 15, 1564–1568. doi: 10.1038/s41396-020-00857-y
Sawall Y., Al-Sofyani A., Banguera-Hinestroza E., Voolstra C. R. (2014). Spatio-temporal analyses of Symbiodinium physiology of the coral Pocillopora verrucosa along large-scale nutrient and temperature gradients in the Red Sea. PloS One 9, e103179. doi: 10.1371/journal.pone.0103179
Silverstein R. N., Cunning R., Baker A. C. (2017). Tenacious D: Symbiodinium in clade D remain in reef corals at both high and low temperature extremes despite impairment. J. Exp. Biol. 220, 1192–1196. doi: 10.1242/jeb.148239
Stoecker D. K. (1999). Mixotrophy among Dinoflagellates 1. J. Eukaryot. Microbiol. 46 (4), 397–401. doi: 10.1111/j.1550-7408.1999.tb04619.x
Tazioli S., Bo M., Boyer M., Rotinsulu H., Bavestrello G. (2007). Ecological observations of some common antipatharian corals in the marine park of Bunaken (North Sulawesi, Indonesia). Zoological Stud. 46, 227–241.
Terrana L., Bo M., Opresko D. M., Eeckhaut I. (2020). Shallow-water black corals (Cnidaria: Anthozoa: Hexacorallia: Antipatharia) from SW Madagascar. Zootaxa 4826, zootaxa–4826. doi: 10.11646/zootaxa.4826.1.1
Terraneo T. I., Fusi M., Hume B. C., Arrigoni R., Voolstra C. R., Benzoni F., et al. (2019). Environmental latitudinal gradients and host-specificity shape Symbiodiniaceae distribution in Red Sea Porites corals. J. Biogeography 46, 2323–2335. doi: 10.1111/jbi.13672
Terraneo T. I., Ouhssain M., Castano C. B., Aranda M., Hume B. C., Marchese F., et al. (2023). From the shallow to the mesophotic: a characterization of Symbiodiniaceae diversity in the Red Sea NEOM region. Front. Mar. Sci. 10. doi: 10.3389/fmars.2023.1077805
Triantafyllou G., Yao F., Petihakis G., Tsiaras K. P., Raitsos D. E., Hoteit I. (2014). Exploring the Red Sea seasonal ecosystem functioning using a three-dimensional biophysical model. J. Geophysical Research: Oceans 119, 1791–1811. doi: 10.1002/2013JC009641
Vimercati S., Terraneo T. I., Castano C. B., Barreca F., Hume B. C., Marchese F., et al. (2024). Consistent Symbiodiniaceae community assemblage in a mesophotic-specialist coral along the Saudi Arabian Red Sea. Front. Mar. Science. 11. doi: 10.3389/fmars.2024.1264175
Wagner D., Luck D. G., Toonen R. J. (2012). The biology and ecology of black corals (Cnidaria: Anthozoa: Hexacorallia: Antipatharia). Adv. Mar. Biol. 63, 67–132. doi: 10.1016/B978-0-12-394282-1.00002-8
Wagner D., Pochon X., Irwin L., Toonen R. J., Gates R. D. (2011). Azooxanthellate? Most Hawaiian black corals contain Symbiodinium. Proc. R. Soc. B: Biol. Sci. 278, 1323–1328. doi: 10.1098/rspb.2010.1681
Wham D. C., Ning G., LaJeunesse T. C. (2017). Symbiodinium glynnii sp. nov., a species of stress-tolerant symbiotic dinoflagellates from pocilloporid and montiporid corals in the Pacific Ocean. Phycologia 56, 396–409. doi: 10.2216/16-86.1
Xiang T., Nelson W., Rodriguez J., Tolleter D., Grossman A. R. (2015). Symbiodinium transcriptome and global responses of cells to immediate changes in light intensity when grown under autotrophic or mixotrophic conditions. The Plant Journal 82 (1), 67–80. doi: 10.1111/tpj.12789
Keywords: Symbiodiniaceae, Antipatharia, Red Sea, NGS, metabarcoding, ITS2, deep sea
Citation: Vicario S, Terraneo TI, Bocanegra Castano C, Chimienti G, Oury N, Vimercati S, Hume BCC, Marchese F, Nolan MKB, Eweida AA, Purkis SJ, Rodrigue M, Pieribone V, Qurban M, Duarte CM and Benzoni F (2024) Lost in the dark: Antipatharia-Symbiodiniaceae association in the deep waters of the Red Sea. Front. Mar. Sci. 11:1330118. doi: 10.3389/fmars.2024.1330118
Received: 30 October 2023; Accepted: 02 April 2024;
Published: 19 April 2024.
Edited by:
Nicolas James Pilcher, Marine Research Foundation, MalaysiaReviewed by:
Mark Mccauley, United States Department of the Interior, United StatesCopyright © 2024 Vicario, Terraneo, Bocanegra Castano, Chimienti, Oury, Vimercati, Hume, Marchese, Nolan, Eweida, Purkis, Rodrigue, Pieribone, Qurban, Duarte and Benzoni. This is an open-access article distributed under the terms of the Creative Commons Attribution License (CC BY). The use, distribution or reproduction in other forums is permitted, provided the original author(s) and the copyright owner(s) are credited and that the original publication in this journal is cited, in accordance with accepted academic practice. No use, distribution or reproduction is permitted which does not comply with these terms.
*Correspondence: Silvia Vicario, c2lsdmlhLnZpY2FyaW9Aa2F1c3QuZWR1LnNh
Disclaimer: All claims expressed in this article are solely those of the authors and do not necessarily represent those of their affiliated organizations, or those of the publisher, the editors and the reviewers. Any product that may be evaluated in this article or claim that may be made by its manufacturer is not guaranteed or endorsed by the publisher.
Research integrity at Frontiers
Learn more about the work of our research integrity team to safeguard the quality of each article we publish.