- 1Ruđer Bošković Institute, Division for Marine and Environmental Research, Zagreb, Croatia
- 2Institute for Adriatic Crops and Karst Reclamation, Split, Croatia
Due to orography-driven dynamics at a (sub-)kilometer scale (e.g., the bora wind) and a complex ocean bathymetry that includes numerous channels, depressions and ridges, the atmosphere-ocean dynamics within the semi-enclosed Adriatic region is not well reproduced by the available regional climate models. The Adriatic Sea and Coast (AdriSC) kilometer-scale atmosphere-ocean model was thus specifically developed to accurately assess the Adriatic climate hazards under both historical (1987-2017) and far-future (2070-2100) conditions. In this study, we analyze the impact of climate change on the projected Adriatic trends, variability and extreme events. In the atmosphere, our results mostly follow the already published literature: strong land-sea contrasts, increased droughts and extreme rainfall events, and decreased wind speeds in the coastal areas. In the ocean, strong and constant rise in surface and intermediate temperatures is associated with salinity decrease, except in surface during summer when salinity rises in the coastal areas. At the bottom and for the ocean circulation, our results exhibit strong contrasts. In the coastal areas, bottom temperature rises, and bottom salinity decreases at the same rate than in surface while changes in current speed are negligible. In the deepest part of the Adriatic, negative bottom temperature trends result in a rise 2.5°C slower than in surface while bottom salinity increases. Further, ocean currents accelerate in the surface and intermediate layers but decelerate at the bottom. These ocean results suggest a reduction of the dense water formation in the northern Adriatic, an intensification and shrinking of the southern Adriatic cyclonic gyre, and a strengthening of the vertical stratification in the deepest part of the Adriatic probably linked to changes in the Adriatic-Ionian water mass exchanges. Given the potential impact of these changes on the Adriatic coastal communities and marine life, this study highlights the need to increase the ongoing kilometer-scale modelling efforts in the Adriatic region with the aim to implement policies and adaptation plans better tailored to the local climate changes projected in this specific region.
1 Introduction
The Adriatic region (Figure 1), located in the northernmost part of the Mediterranean Sea, experiences a unique and complex climate characterized by strong atmosphere-ocean interactions and contrasts. The coastal regions of the Adriatic region present a Mediterranean climate, with hot, dry summers and mild, wet winters. Conversely, regions like the Alps, Apennines, and Dinarides extend their mountainous climate even to coastal areas due to long coastal ridges such as Velebit Mountain, which generates the downslope bora winds (Grisogono and Belušić, 2009). Moving further inland, the continental climate prevails with greater precipitation during warm seasons compared to the cold ones (Gajić-Čapka, 1993). The strongest Adriatic winds, bora and sirocco, significantly impact the local atmosphere-ocean climate, leading to pronounced differences in weather conditions across the region (Tojčić et al., 2023). Within the Adriatic Sea, exchange of water masses with the saltier and warmer Ionian Sea, persistence of substantial decadal oscillations driven by internal processes and large-scale atmospheric circulation patterns are the main drivers of the ocean circulation (Lionello et al., 2006; Civitarese et al., 2023). In particular, the Adriatic thermohaline circulation results from three main influences. The first is the wintertime generation of dense waters during bora events (Pullen et al., 2006; Janeković et al., 2014; Ličer et al., 2016) which can lower the sea surface temperatures by up to 4-6°C (Denamiel et al., 2020a). The second is the Adriatic-Ionian Bimodal Oscillating System (BiOS; Gačić et al., 2010; Mihanović et al., 2015; Denamiel et al., 2022) which greatly impacts the Adriatic salinity. The third is the presence of major rivers (Raicich, 1996) which produce a year-round decrease in salinity, particularly towards the northern Adriatic (Artegiani et al., 1997; Lipizer et al., 2014).
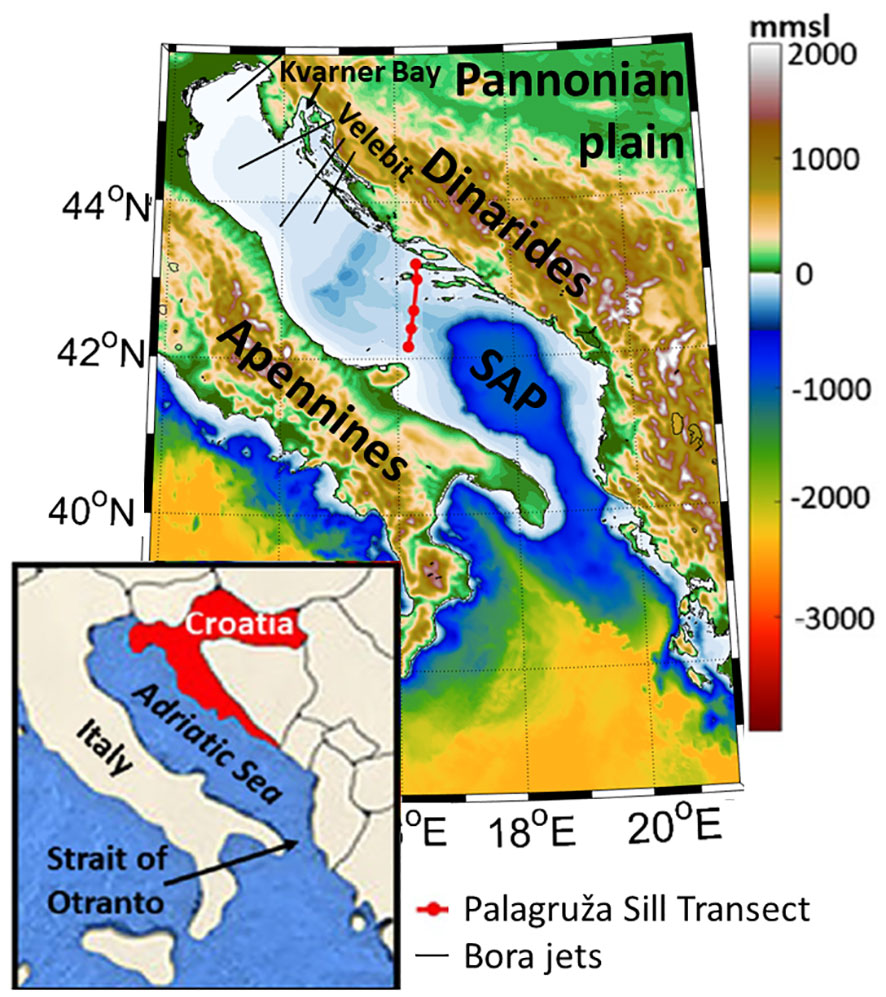
Figure 1 AdriSC WRF 3-km domain and topography, AdriSC ROMS 1-km domain and bathymetry, along with geographical features of the region – Apennines, Dinarides, Pannonian plain, Velebit Mountain, Kvarner Bay and Southern Adriatic Pit (SAP). Palagruža Sill Transect is presented in red, along with bora jets presented as black lines.
The Adriatic region, similarly, to the other Mediterranean regions, is a known hotspot in terms of multiple strong climate hazards and high vulnerability under the on-going global warming (IPCC, 2022). Air temperatures are already exhibiting increases in the present climate, with more pronounced warming in summer and less in winter (Ogrin, 2015; Radilović et al., 2020). Precipitation patterns are also shifting in various regions, with results by Gajić-Čapka et al. (2014) indicating generally weak trends in precipitation extremes but reveal more significant annual and seasonal changes in the mountainous and coastal hinterland regions. In the latter, drying tendencies occur, especially during the summer season, while the mainland experiences higher amount of precipitation. In the ocean, sea surface temperatures are following seasonal atmospheric trends but with somewhat higher rates, increasing at approximately 0.4-0.5°C over recent decades, as observed through in situ and satellite measurements (Pastor et al., 2018; Vilibić et al., 2019; Bonacci et al., 2021). Recent trends at the Adriatic’s longest surveyed transect, the Palagruža Sill (Figure 1), indicate weakened thermohaline circulation and increased salinity in the surface and intermediate layers (Vilibić et al., 2013). In terms of atmosphere-ocean climate projections, until now, the regional climate models (RCMs) developed over the entire Mediterranean Sea within the Med-CORDEX initiative (e.g., Ruti et al., 2016) formed the foundation for assessing the future Adriatic conditions under global warming. However, the RCM horizontal resolution, of the order of 10 km, has been proven to be insufficient to resolve the complex Adriatic dynamics in both the atmosphere (Denamiel et al., 2021a) and the ocean (Pranić et al., 2023). These RCMs thus struggle to reproduce the Adriatic thermohaline properties (Dunić et al., 2019) and many Adriatic coastal processes including the extreme bora events (Denamiel et al., 2021a) and the BiOS (Dunić et al., 2018; Pranić et al., 2021).
Consequently, the first kilometer-scale atmosphere–ocean climate model in the world, the Adriatic Sea and Coast (AdriSC) climate model (Denamiel et al., 2019; Denamiel et al., 2021b) with horizontal resolutions up to 3 km in the atmosphere and 1 km in the ocean, was implemented to better represent the Adriatic coastal dynamics. Till now, two 31-year long AdriSC simulations have been performed, a historical run during the 1987-2017 period forced by reanalysis products and an extreme warming run during the 2070-2100 period for a Representative Concentration Pathways 8.5 (hereafter rcp8.5) greenhouse scenario downscaling a single model of the Med-CORDEX ensemble. The AdriSC trends and variability have already been quantified for the historical simulation during the 1987-2017 period (Tojčić et al., 2023). The aim of this research is thus twofold. First, the AdriSC historical and extreme warming runs are used to assess the far-future changes of the Adriatic trends, variability and extreme events for the temperature, humidity, wind and rainfall in the atmosphere as well as for the temperature, salinity, and current in the ocean. Second, the potential implications, for the atmosphere-ocean dynamics, of the far-future changes projected by the AdriSC model, as well as, the limitations of the AdriSC results and their similarities and differences with the EURO- and Med- CORDEX RCMs, are thoroughly discussed.
The article is structured as follows. The AdriSC model set-up and the methods used in this study are described in Section 2, while the trends, variabilities and frequencies of extreme events are analyzed in Section 3. The impact of these results on the Adriatic region atmosphere-ocean dynamics and coastal communities is then further discussed in Section 4, while major conclusions are presented in Section 5.
2 Model and methods
2.1 AdriSC climate model
The Adriatic Sea and Coast (AdriSC) kilometer-scale atmosphere-ocean climate model (Denamiel et al., 2019; Denamiel, 2021) has been specifically implemented to accurately represent the Adriatic coastal dynamics under both historical (1987-2017) and extreme warming (2070-2100; for a Representative Concentration Pathways RCP 8.5 greenhouse scenario) conditions. It is based on the Coupled Ocean–Atmosphere–Wave–Sediment Transport (COAWST) modelling system developed by Warner et al. (2010) which dynamically couples the Weather Research and Forecasting (WRF; Skamarock et al., 2005) atmospheric model and the Regional Ocean Modelling System (ROMS; Shchepetkin and McWilliams, 2009) ocean model. The AdriSC setup (Table 1) consists in (1) two different nested grids at 15-km and 3-km resolution used in the WRF model and covering respectively the central Mediterranean area and the Adriatic-Ionian region and (2) two different nested grids at 3-km and 1-km resolution used in the ROMS model and covering respectively the Adriatic-Ionian region (similarly to the WRF 3-km grid) and the Adriatic Sea only. For the atmospheric grids, a 1:5 ratio is used to obtain slightly more accurate interpolation as the center of gravity of the outer grid cells is preserved within the inner grid (Chow et al., 2019). In addition, in climate modelling, due to multi-decadal runs, the 1:3 ratio is rarely used in order to preserve numerical resources; some experimental climate models even use 1:11 and 1:15 ratios (Zhou and Chow, 2014; Munoz-Esparza et al., 2017; Wiersema et al., 2018). In the vertical, all the AdriSC grids rely on terrain following coordinates: 58 levels refined in surface with a ratio of 3.62% of the height between the surface and 70 hPa (the top layer of the WRF model; Laprise, 1992) and 35 levels refined near both the sea surface and bottom floor for the ocean (Shchepetkin and McWilliams, 2009). More details on the set-up of the AdriSC modelling suite – which is installed and fully tested on the European Centre for Middle-range Weather Forecast (ECMWF) high-performance computing facilities – can be found in Denamiel et al. (2019); Denamiel et al. (2021b) and Pranić et al. (2021).
The AdriSC climate modelling strategy follows the Pseudo-Global Warming (PGW) method developed by Schär et al. (1996) in the atmosphere and recently extended to the ocean (Denamiel et al., 2020b). Consequently, climatological changes are derived based on the differences between future scenarios (here for the 2070-2100 period) with historical data (here for the 1987-2017 period). These changes are obtained using the LMDZ4-NEMOMED8 regional climate model, part of the Med-CORDEX ensemble (Hourdin et al., 2006; Beuvier et al., 2010), forced by the IPSL-CM5A-MR global climate model (simulations r1i1p1). These projections are imposed as forcing on top of the reanalysis products. Specifically, ERA-Interim (Balsamo et al., 2015) for the atmosphere, and the Mediterranean Forecasting System (MFS) MEDSEA v4.1 (Pinardi et al., 2003) for the ocean. The reanalysis products are the same ones used to produce the historical run (Denamiel et al., 2020a; Denamiel et al., 2020b).
The evaluation of the AdriSC climate model against an extensive dataset composed of in-situ observations and remote sensing products has shown that, for the 1987−2017 period, the skills of the newly developed coupled atmosphere-ocean kilometer-scale climate model outperform those of the RCMs implemented in the Mediterranean Sea (i.e., EURO- and Med-CORDEX) in both the atmosphere (Denamiel et al., 2021b) and the ocean (Pranić et al., 2021).
2.2 Methods
In this study, the far-future trends and variabilities over the entire Adriatic region as well as the number of extreme events are extracted from the AdriSC extreme warming run (2070−2100) and compare with the results of the historical run (1987-2017) already analyzed in Tojčić et al. (2023). They are derived for the surface WRF 3-km daily results – i.e., temperature at 2 m, rain, relative humidity at 2 m and wind speed at 10 m – in the atmosphere, and for the bottom, 100 m depth and surface ROMS 1-km daily results – temperature, salinity, and current speed – in the ocean.
Variances and trends are derived over the entire Adriatic region (WRF 3-km domain in the atmosphere and ROMS 1-km domain in the ocean). Trends are calculated over the 31-year period of both runs, with the Theil-Sen method (Mondal et al., 2012) and trend significances are calculated with the Mann-Kendall test (Mann, 1945; Kendall, 1975; Gilbert, 1987). Only trends with significance over 95% are taken into consideration and presented hereafter. Variances are calculated from the detrended daily data. The impact of climate change is calculated as percentages of trends/variances, computed as the ratio (multiplied by 100) of the difference between extreme and historical trends/variances and absolute value of historical trends/variances. The results are presented as spatial plots covering the whole studied areas. For convenience, the obtained original trends are converted to decadal trends, which are commonly used within the climate community.
The impact of climate change on the number of extreme events is derived for selected sub-domains. In the atmosphere, the variables are analyzed for the land and sea sub-domains in order to reflect the differences in heat capacity and heat transfer mechanisms. Indeed, land surfaces heat up and cool down more rapidly than sea masses, which results in distinct temperature gradients and air pressure variations. Consequently, local wind patterns, cloud formation, and precipitation distribution can significantly differ between land and sea areas. The ocean variables are analyzed for the deep and coastal sub-domains which cover the Southern Adriatic Pit (Figure 1) and the deep areas surrounding it, and the rest of the Adriatic with relatively shallow depths, respectively. These sub-domains reflect the differences between the shallow and nearshore areas which may experience more significant temperature and salinity fluctuations in response to extreme weather events, river discharges, tides, etc. and the more stable deep areas less impacted by these processes. For each of the sub-domains, monthly means are derived from the extreme warming and historical AdriSC daily results. The monthly analysis is conducted through two distinct approaches applied to each studied variable. First, the difference between extreme warming and historical monthly climatologies is computed, serving as a basic indicator of the monthly changes in average conditions. Second, the 10th and 90th percentiles of the historical monthly data are determined, serving as minimum and maximum historical thresholds needed to calculate the number of days within a month below or above these thresholds (i.e., the number of extreme low or high events). To quantify the change in occurrences of extreme values under extreme warming conditions, compared to the historical ones, the number of days in the historical run with values below (or above) the minimum (or maximum) historical thresholds are subtracted from the number of days in the far-future climate. This represents approximately 3 days per month. The monthly analysis of the extreme events is not performed both at 100 m depth for the coastal sub-domain, as only a few model grid points in this domain fall below 100 m, and for the extreme low rain events as the minimum threshold is 0 mm/day at almost all model domain grid points.
3 Results
3.1 Atmosphere
Decadal temperature trends in the Adriatic region indicate that significant warming continues during the 2070-2100 period (Figure 2, upper panels). Temperature trends at 2 m are higher over sea (up to 0.4–0.5°C per decade) than over land (up to 0.3°C per decade) similarly to the trends found in the historical run (Tojčić et al., 2023). The percentage of temperature trend shows that far-future warming is generally expected to be less intense compared to the historical conditions. This is particularly visible over most of the Adriatic Sea (up to a 10% decrease) and the southern Italy and Dinarides (15% decrease). However, trends are projected to increase in the southern Pannonian plains (around 7%). Variance of temperature at 2 m above 35°C2 is observed over land, with lower variability along the coast due to the influence of the sea and roughly two times less variability over the deep Adriatic Sea. Compared to the historical conditions, a general 5-10% increase in temperature variability is projected for this extreme warming scenario. Monthly analysis of the change in number of extreme air temperatures extreme events (Figure 2, lower panels) aligns with the spatial analysis, indicating more significant changes over sea, with over 20 more days per month over the historical maximum threshold and not a single day below the historical minimum threshold (i.e., about 3 less days per month than in the historical run), than over land. In particular, July-August exhibits nearly continuous extreme high temperatures over sea. Over land, around 10 days per month are projected to exceed the historical maximum threshold throughout the year, with over 20 days in July-August while no day below the minimum historical threshold is expected.
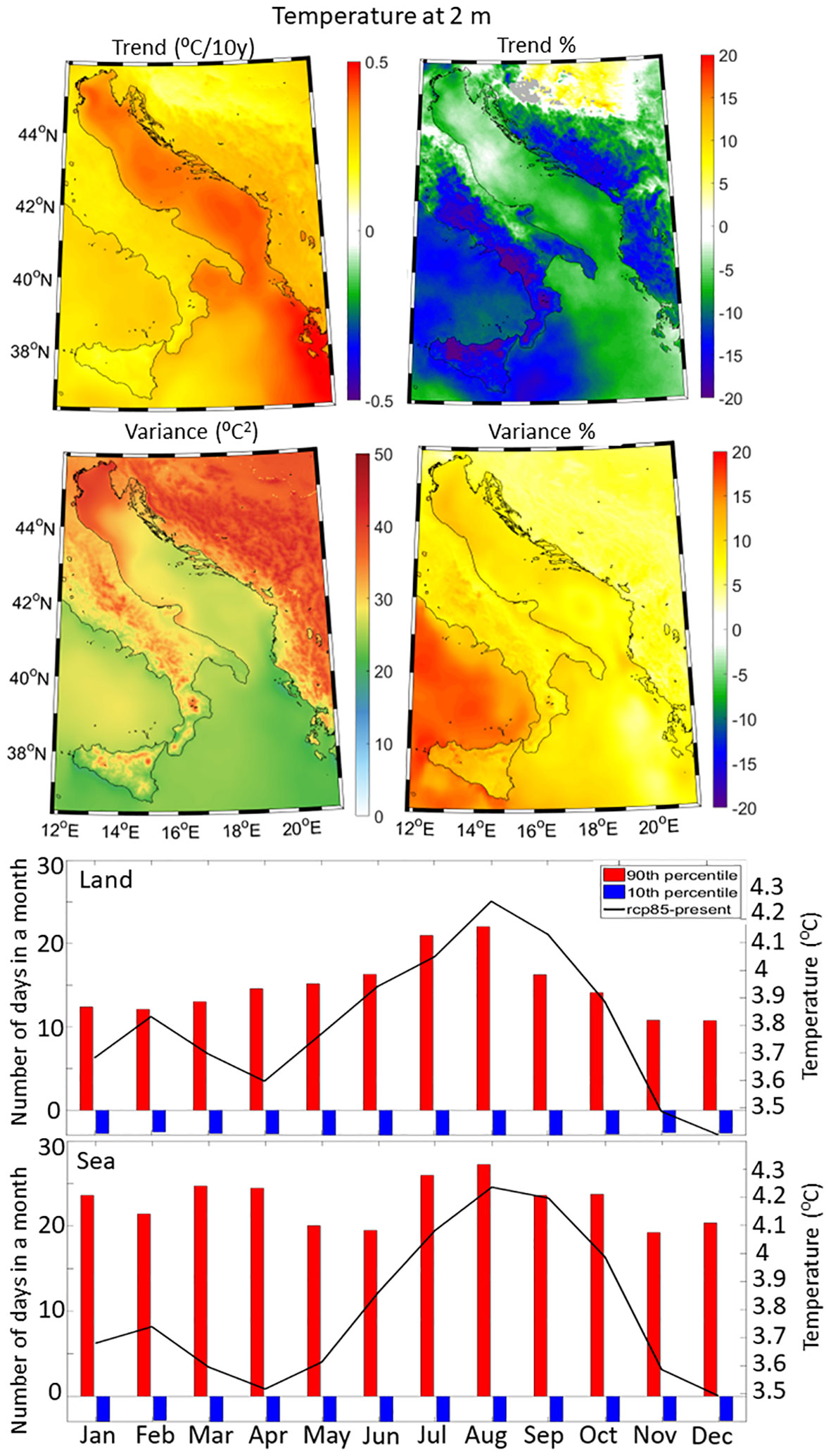
Figure 2 Trend, trend percentage, variance and variance percentage spatial plots (top panels) as well as changes in both monthly average conditions (black line) and number of daily extreme events per month calculated below/above the historical minimum (10th percentile)/maximum (90th percentile) thresholds (bar plots) over the land and sea sub-domains (bottom panels), for the air temperature at 2 m.
Relative humidity trends at 2 m (Figure 3, upper panels) are negative over sea (-0.6% to -0.4% per decade) and positive over land (0.3-0.5% per decade), mirroring present climate trends (Tojčić et al., 2023). These results probably originate from boundary condition forcing, as negative trends in relative humidity have been previously found in global analysis over most of the Mediterranean Sea (Vicente-Serrano et al., 2018). Negative trends are expected to intensify over the southern Adriatic Sea and most of the coastal areas but to decrease over the middle Adriatic Sea. Mountainous regions, like the Apennines and the Dinarides, exhibit patchy distributions, reflecting local effects of mountain ridges on humidity trends. Variability of relative humidity is expected to decrease in the far-future climate, ranging from 5% over sea to 15% in the northern Italy, Istria, and central Dalmatia coastal areas. Exceptions include mountainous regions like the Apennines, Sicily, and southeastern Dinarides. Monthly analysis for extreme relative humidity in the far-future climate (Figure 3, lower panels) reveals a decrease (less than a day per month) in dry days (below the minimum historical threshold) over land in September-December. This decrease is accompanied by an overall increase in mean relative humidity (up to 0.8%). Over sea, the decrease (less than one day per month) occurs in January-March. Moist days (above the maximum historical threshold) are expected to slightly decrease (less than a day per month) during the warm season over both land and sea sub-domains. The land-sea contrasts in terms of relative humidity are defined as positive climatology differences over land and negative ones over sea, except in May-August when both are negative.
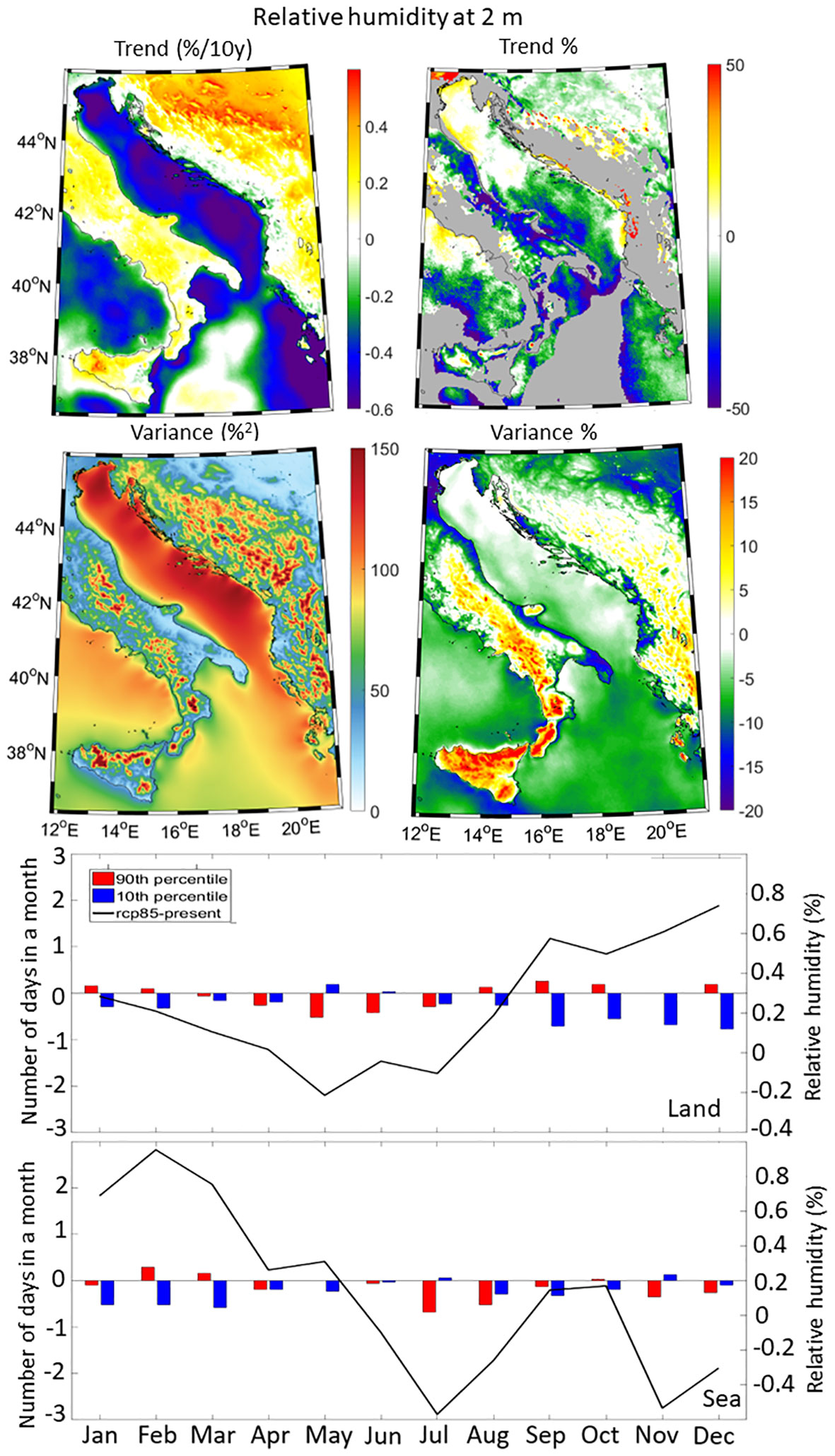
Figure 3 Same as Figure 2 but for the relative humidity at 2 m.
Rain decadal trends in the far-future climate (Figure 4, upper panels) are positive over sea and the coastal regions, except in the Rijeka area where large negative trends are projected (below -0.2 mm/day per decade). Coastal regions along the eastern coast and the northern Adriatic present larger decadal rain trends (up to 0.2 mm/day per decade) than in the rest of the domain (around 0.1 mm/day per decade). South of the Adriatic, trends are lower and mostly decreasing. Rain variance percentages indicate an overall increase of more than 50% in variability. Monthly analysis of rain (Figure 4, lower panels) reveals an increase in days with extreme rainfall in the far-future climate, particularly in October-January. The number of days with extreme rainfall decreases (up to a day per month) during the warm season (May-September) over both land and sea sub-domains, accompanied by an increase in mean rain intensity over the land and sea sub-domains varying between 0.2 and 0.8 mm/day during the cold season (September-January).
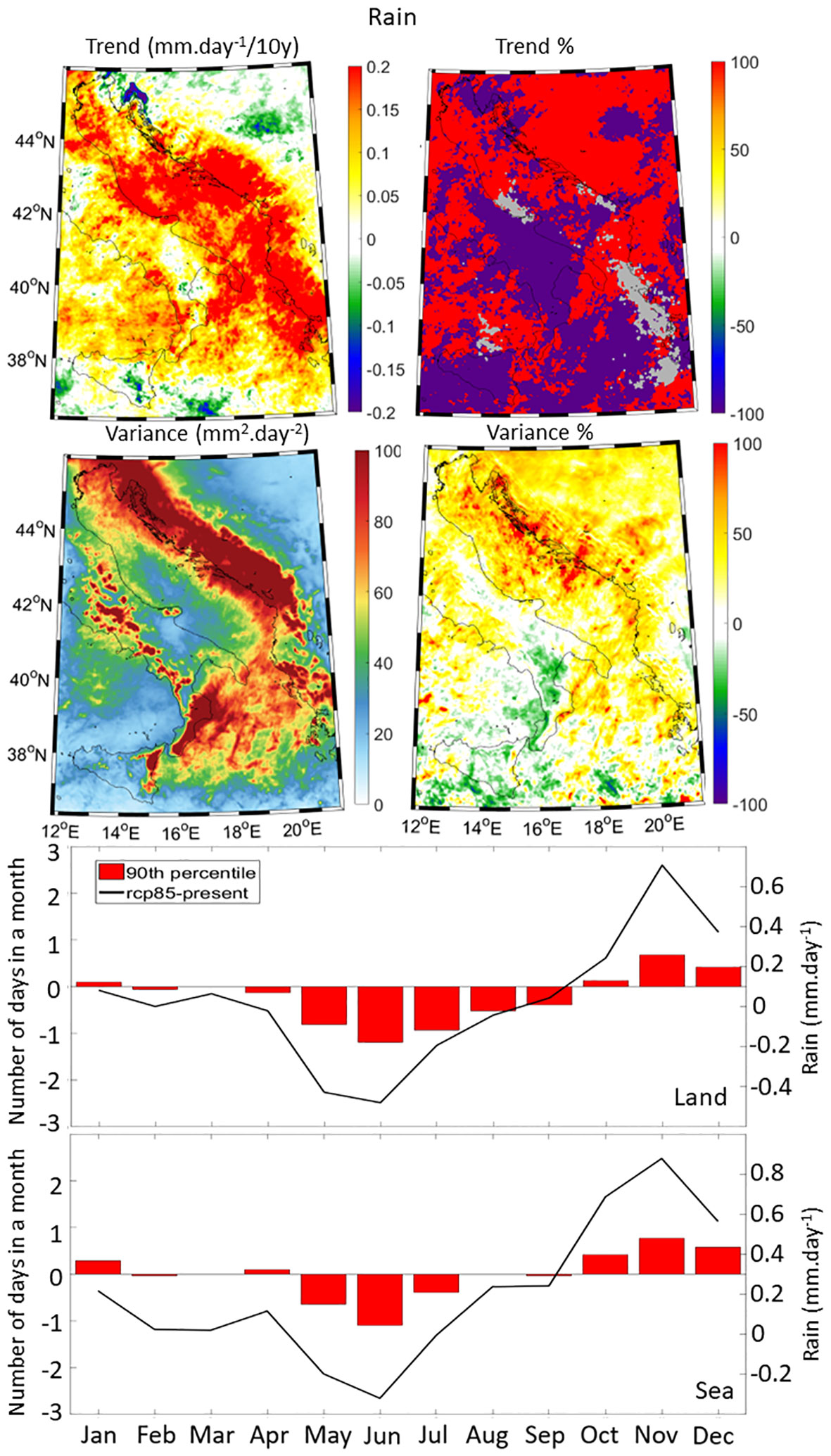
Figure 4 Same as Figure 2 but for the surface rain. The changes in the number of daily extreme events per month below the historical minimum threshold equal to 0 mm/day are not presented.
For the wind speed at 10 m (Figure 5, upper panels), positive decadal trends are mostly observed over the sea and coastal regions (mostly between 0.05 and 0.1 m/s per decade), except along the bora wind jets, where trends are slightly negative (between -0.025 and 0 m/s per decade). Mountainous regions and the Pannonian plains show mostly negative trends (around -0.05 m/s per decade). Variability in wind speed is projected to decrease over most of the domain, reflecting greater persistence of wind regimes. Exceptions are mountainous regions, where variability is expected to increase by approximately 10%. Highest total variances, reaching up to 25 m² s-², in wind speed at 10 m are found along the Velebit mountain, known for its strong bora wind events. Monthly wind speed analysis (Figure 5, lower panels) indicates a decrease in strong wind events throughout the year over the land domain, except in October-November. Over sea, a decrease is noted between January and March. Winter sees the most significant decrease in mean wind speed over both land and sea domains (up to -0.3 m/s), while July-August experiences a small increase over sea. The decrease of wind speed during winter, particularly in the northern part of the domain, might suggest a decrease in bora winds and turbulent heat flux, which might impact the northern Adriatic dense water formation (see Section 4 for detailed explanation).
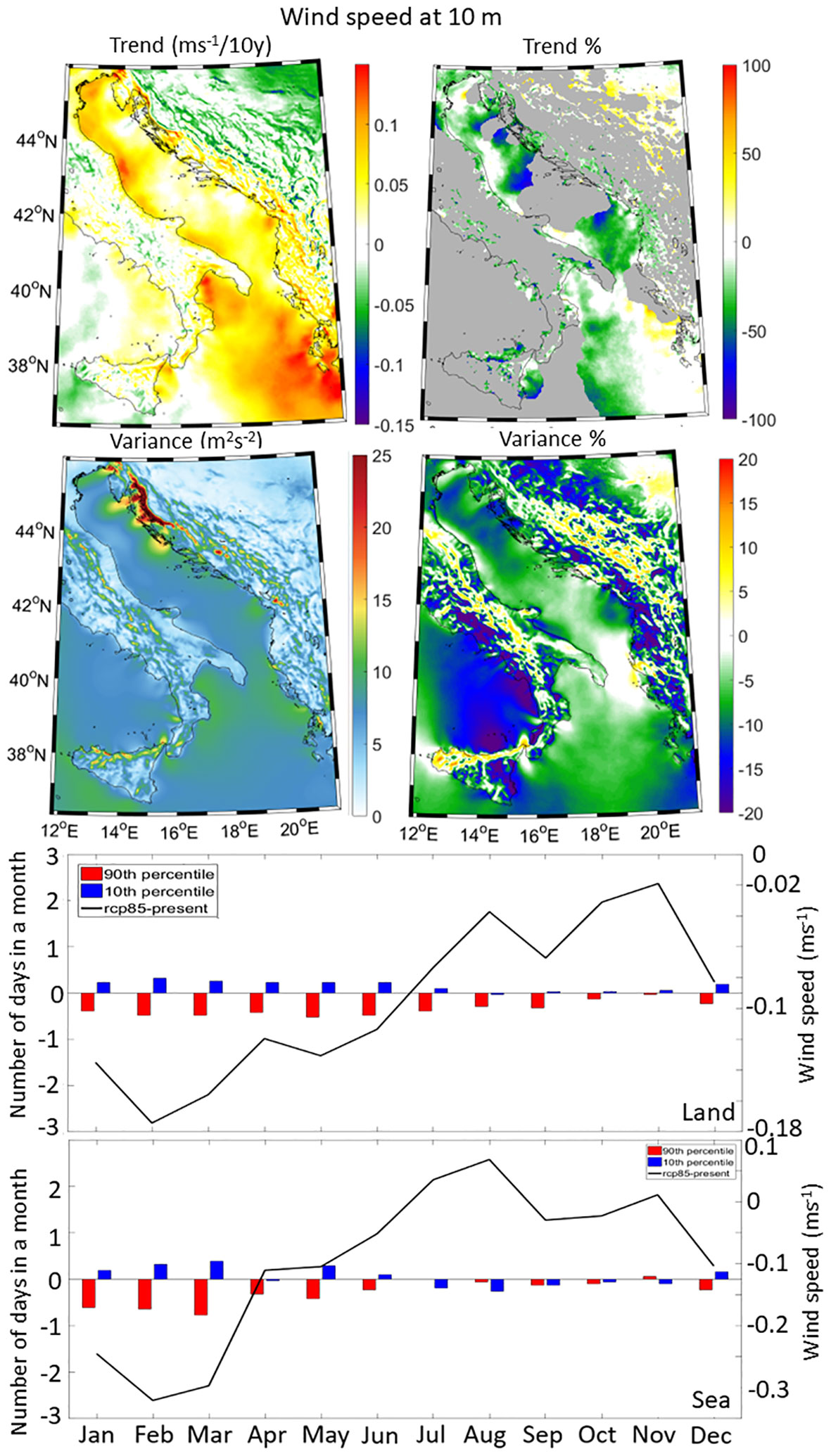
Figure 5 Same as Figure 2 but for the wind speed at 10 m.
3.2 Ocean
Sea surface temperature trends (Figure 6) predominantly range from 0.25 to 0.35°C per decade, with slightly higher values in the southern than in the northern Adriatic and maximum values at the perimeter of the Southern Adriatic Pit (SAP). Trend percentages (Figure 6) indicate a decrease in sea surface temperature trends in the far-future climate compare to the historical results, mostly around 30%, with higher values of approximately 50% in the central Adriatic and along the western coastal current but lower values below 10% in the SAP. Sea temperature trends at 100 m depth (Figure 7, upper panels) show an increase of approximately 0.4°C per decade. The Otranto Strait experiences the highest warming rates (around 0.5°C per decade), while the SAP shows the lower values (about 0.35°C per decade). Trend percentages indicate a decrease in temperature trends of 25-40% in the central Adriatic and an increase of 25-50% in the SAP and the Otranto Strait compare to the historical results. Decadal trends of sea bottom temperature (Figure 8, upper panels) demonstrate contrasting behavior between the SAP and the rest of the Adriatic Sea. The SAP exhibits negative trends (0.1-0.2°C per decade), while positive trends (up to 0.5°C per decade) are observed elsewhere, with the highest values along the eastern coast and the Dalmatian islands. Trend percentages indicate a general decrease in future sea bottom temperature trends, with the lowest values in the central Adriatic and along the Dalmatian coast and islands, dropping below 20%. Higher percentages are found in the SAP and the Otranto Strait, with trends up to 200% lower than for the historical results. Far-future temperature variability is expected to increase compare to the historical results, by 10-20% at the surface and up to 100% at 100 m in the SAP and the Otranto Strait. Monthly analysis reveals significant changes in sea temperature extremes in the far-future climate. Temperatures below the minimum historical threshold in the historical run no longer occur but temperatures above the maximum historical threshold persist in all months, except May-June when 5-8 days do not exceed this threshold. Differences in monthly climatologies show a sea surface temperature increase in the coastal Adriatic regions ranging from 3.4-3.5°C in winter to 4.1-4.4°C in summer. At 100 m, the increase is of 3.4-3.6°C, more pronounced in winter and less in spring and summer due to seasonal conditions. At the bottom, temperature differences also vary, with coastal areas experiencing similar values to those at 100 m, but significantly lower values in the deep sub-domain, ranging from 0.9 to 1°C.
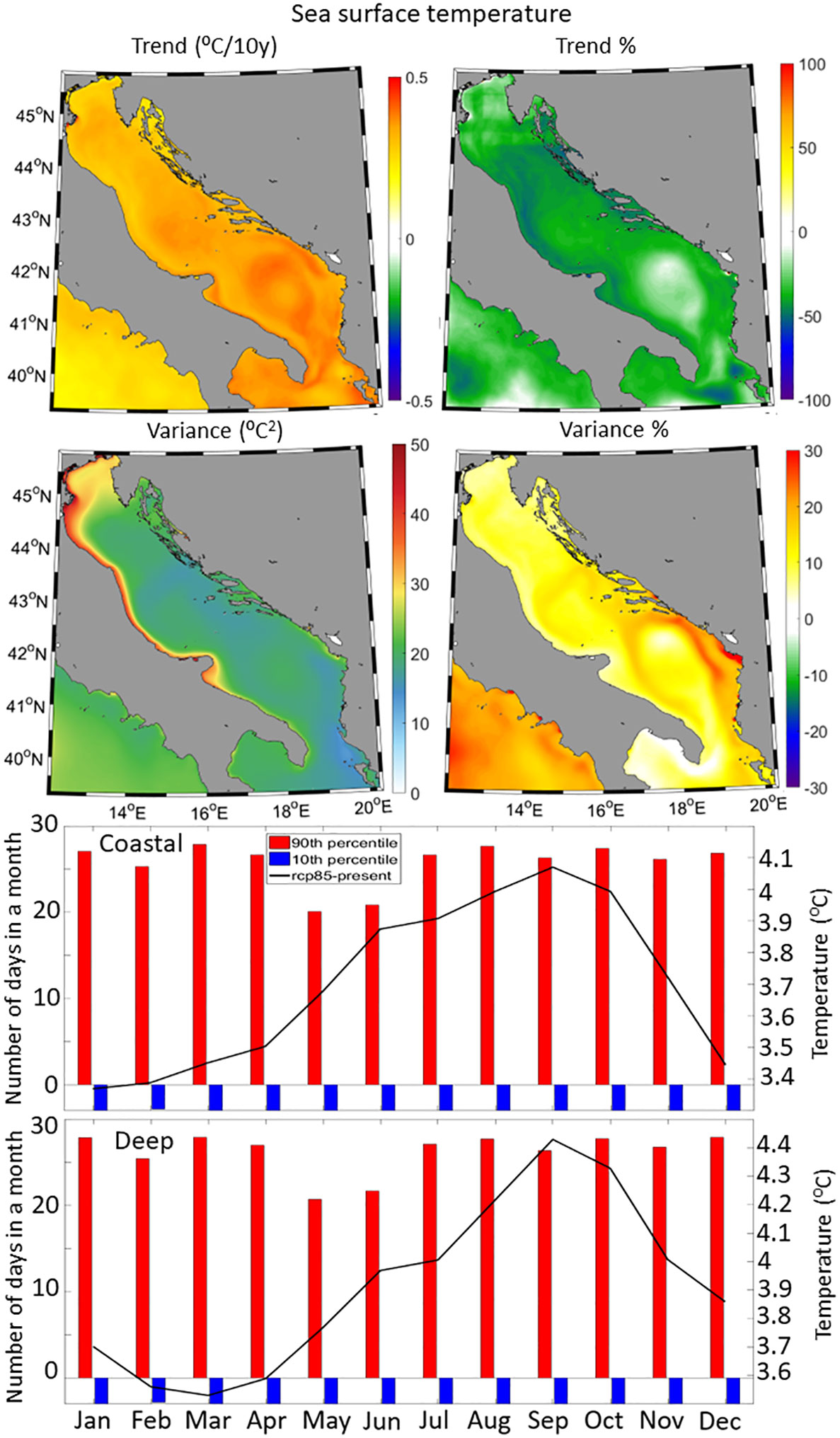
Figure 6 Trend, trend percentage, variance and variance percentage spatial plots (top panels) as well as changes in both monthly average conditions (black line) and number of daily extreme events per month calculated below/above the historical minimum (10th percentile)/maximum (90th percentile) thresholds (bar plots) over the coastal and deep sub-domains (bottom panels), for the sea surface temperature.
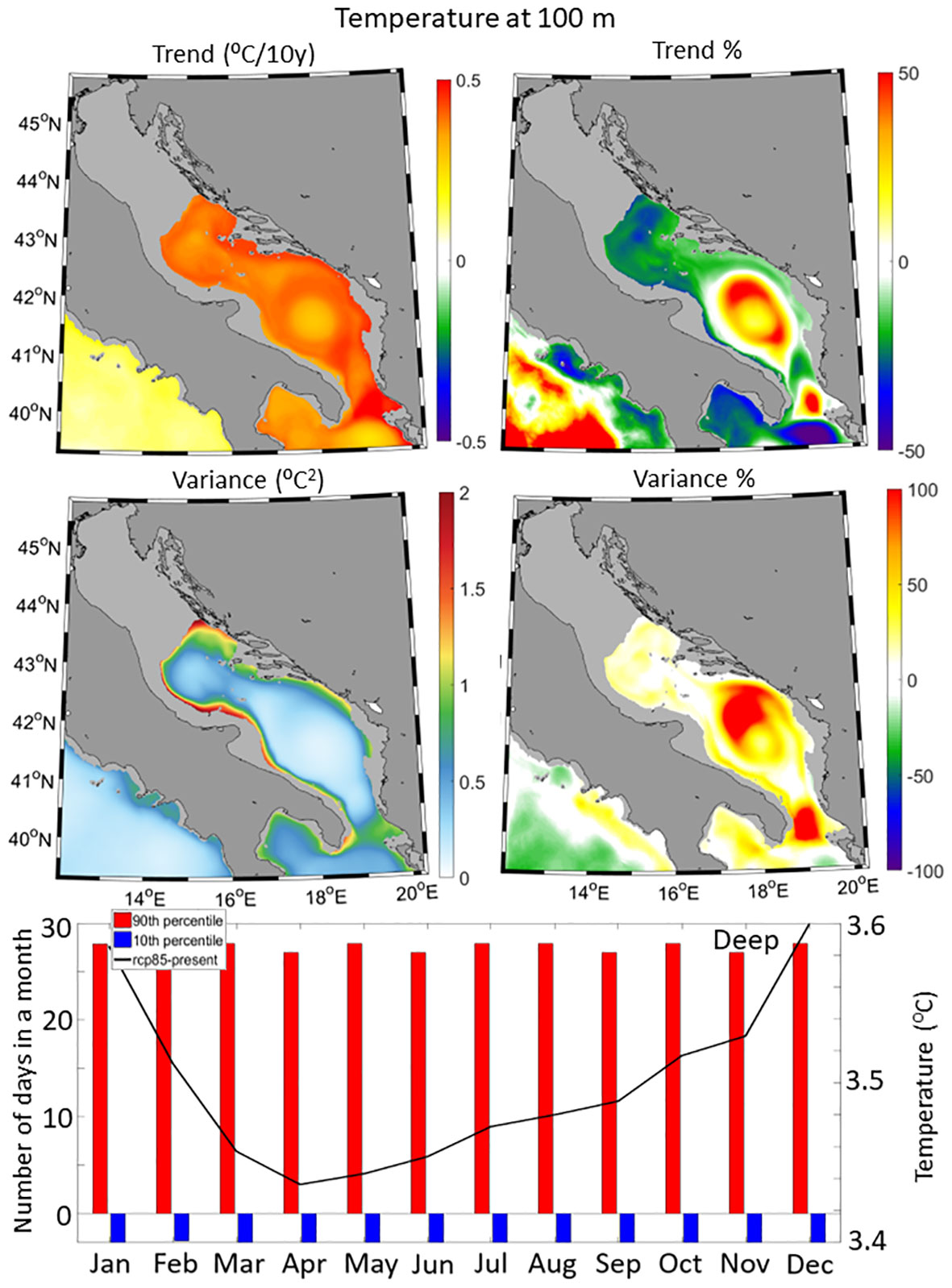
Figure 7 Same as Figure 6 but for the sea temperature at 100 m. The monthly results for the coastal sub-domain are not presented.
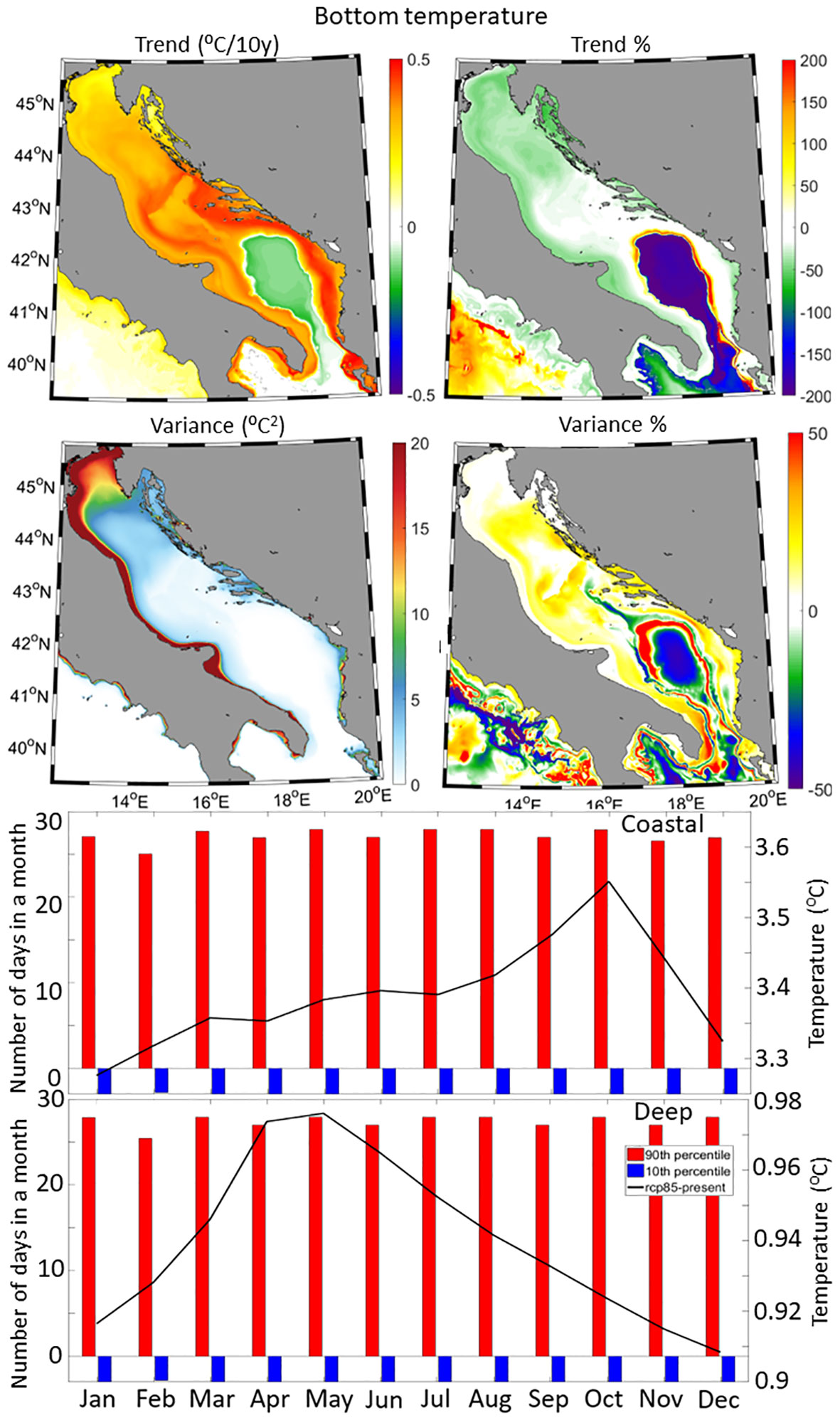
Figure 8 Same as Figure 6 but for the sea bottom temperature.
Surface salinity trends (Figure 9 upper panels) generally vary around 0.1 per decade, with slightly lower values in the Kvarner Bay and Dalmatian Island regions (0.05 per decade). Higher salinity trends are observed off the Po River delta (exceeding 0.15 per decade) and in the Otranto Strait. Compared to the historical trends, salinity is expected to increase by approximately 25% in the SAP and the eastern side of the Otranto Strait. Lower salinity trend differences are projected for the rest of the Adriatic, particularly along the Po River plume, the Kvarner Bay coastal area, and the Neretva River delta. At a depth of 100 m (Figure 10), salinity trends range between 0.05 and 0.1 per decade while, compare to the historical trends, far-future trends are expected to decrease by approximately 20% in the western Adriatic region but to increase in the eastern part, especially in the SAP. Variability at 100 m is generally low but is expected to be much larger than in the present climate. Bottom salinity trends (Figure 11 upper panels) are positive, with lower values in deeper regions and higher values in shallower areas, such as the northern Adriatic shelf. Salinity trends decrease along the Po River plume, in the northern Adriatic, and the Kvarner Bay, while they increase in the Southern Adriatic Pit and the Otranto Strait. Bottom salinity variability is projected to decrease in the Southern Adriatic Pit but increase in the rest of the Adriatic. At the surface (Figure 9), differences in the number of days of extreme high salinity events reveal positive changes during summer (up to 3 and 2 days more in the coastal and deep domains, respectively), but negative changes, associated with an average decrease in salinity of up to 0.2, otherwise. At 100 m depth, all differences are negative throughout the year with at least 14 days more of extreme low salinity events than under the historical conditions associated with a decrease in salinity up to 0.15. This clearly indicates lower salinity in the far-future climate, especially during the winter months. At the bottom, differences are negative in the coastal sub-domain leading to at least 4 days more of extreme low salinity events, while in the deep domain, they are positive leading to at least 18 days more of extreme high salinity events. A detailed discussion about the potential impact of climate warming on the salinity budget is presented in Section 4.
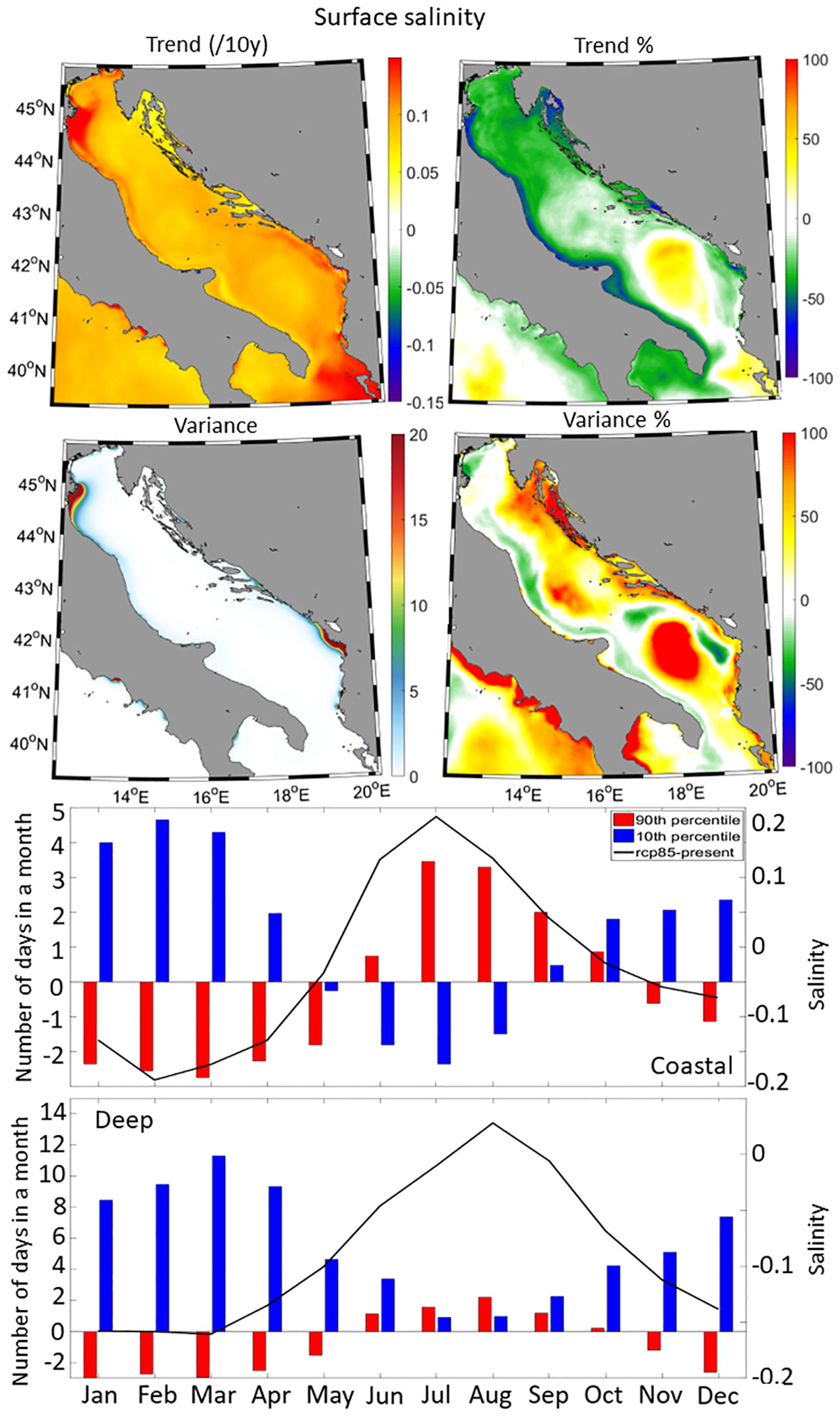
Figure 9 Same as Figure 6 but for the sea surface salinity.
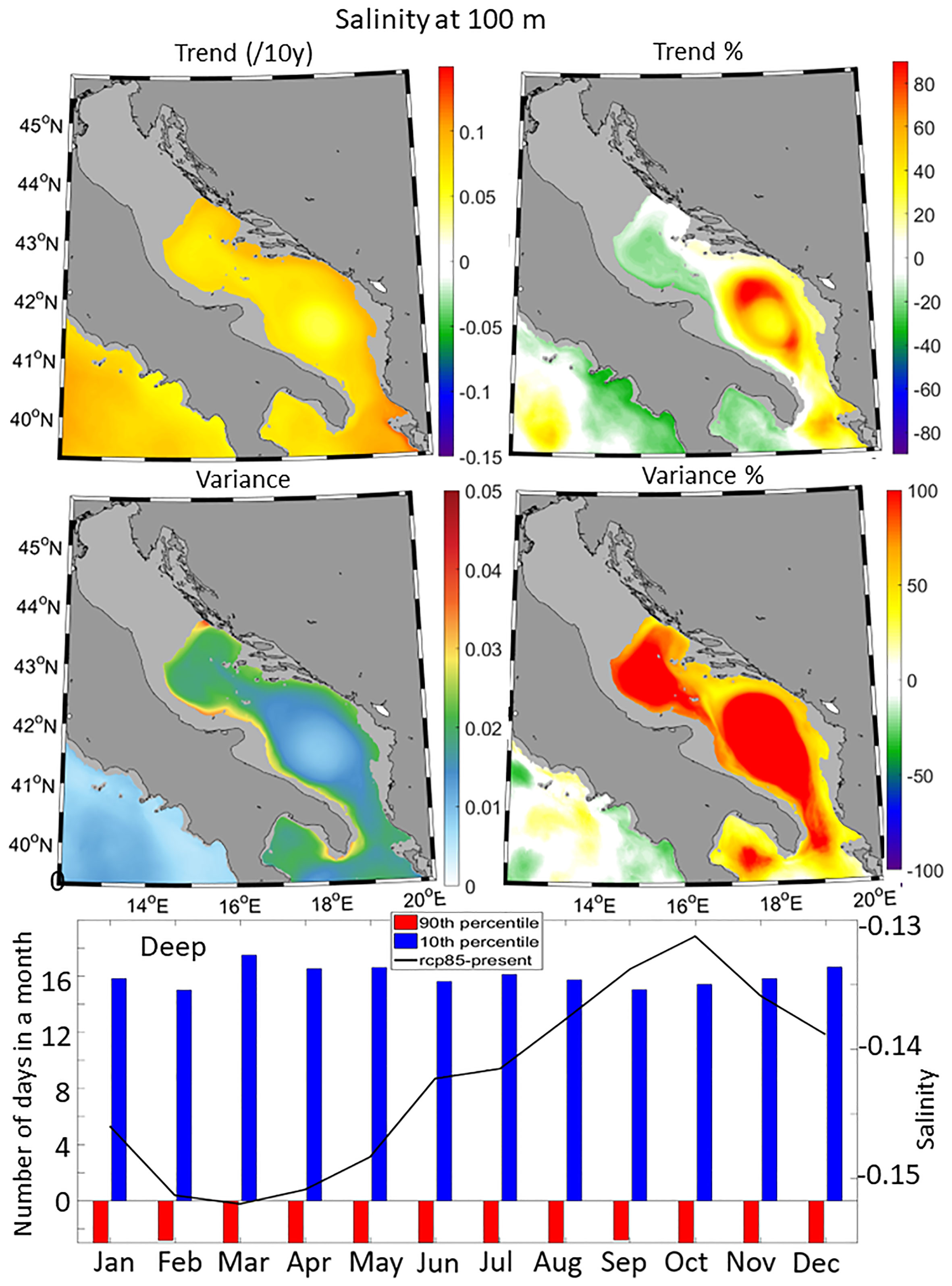
Figure 10 Same as Figure 6 but for the sea salinity at 100 m. The monthly results for the coastal sub-domain are not presented.
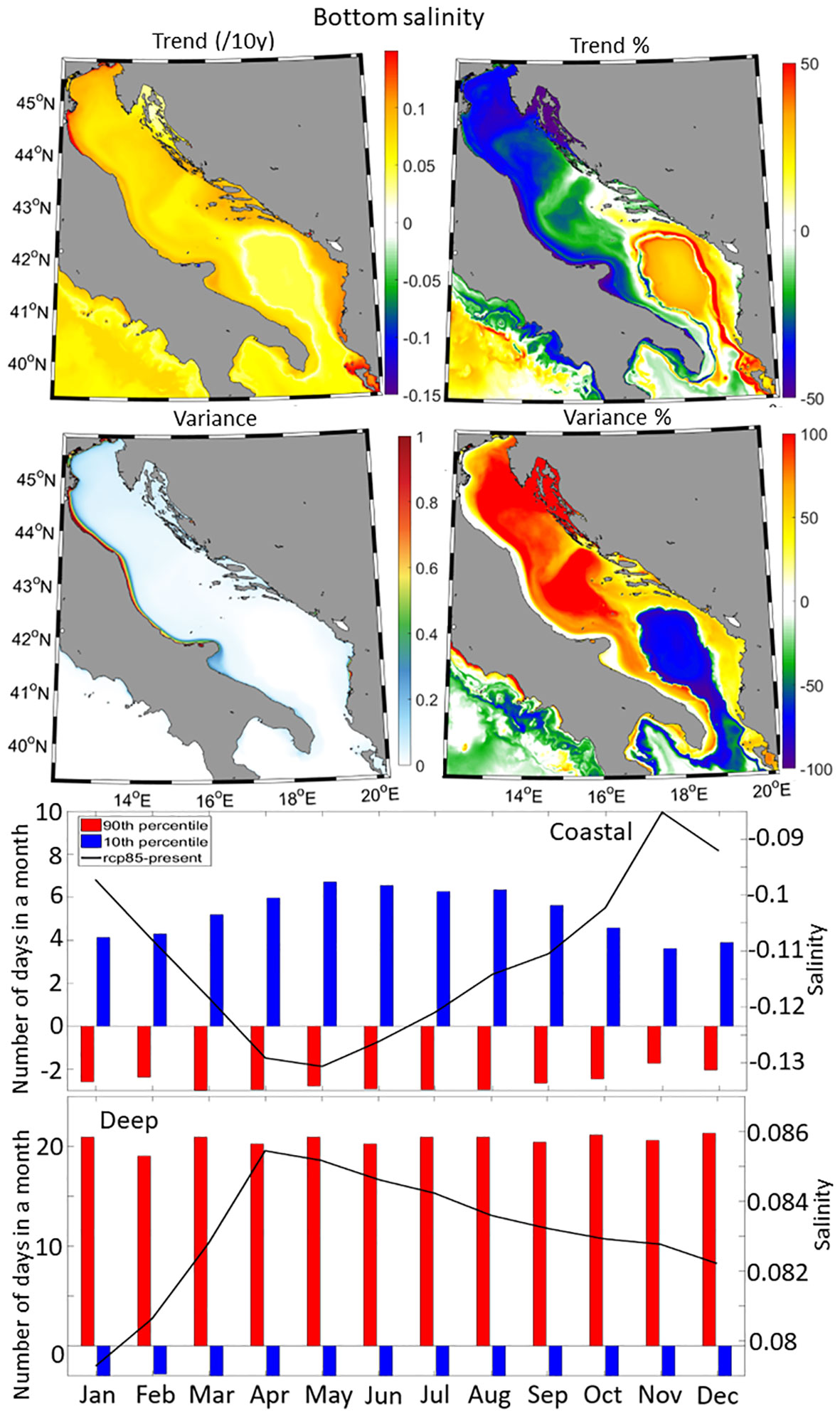
Figure 11 Same as Figure 6 but for the sea bottom salinity.
Far-future current speeds in the Adriatic Sea (Figures 12–14) show positive trends across all depths, like it has been documented for the present climate (Tojčić et al., 2023). At the surface (Figure 12), trends range from 0.005 to 0.01 m/s per decade in most areas, except for the SAP, where they reach up to 0.02 m/s per decade in the inner part of the cyclonic gyre and around -0.005 m/s per decade in its outer parts. Compared to historical trends, positive changes are observed in the central SAP, transversely over the northern Adriatic, and along the eastern and western coastal regions. At a depth of 100 m (Figure 13), current speed trends are similar to surface trends, with positive values up to 0.005 m/s per decade, primarily concentrated in the center of the SAP where both trends and variability increase by more than 100%. At the bottom (Figure 14), current speed trends remain generally low, below 0.002 m/s per decade, with the lowest values in the SAP. Compared to the historical trends, a strong increase in trends (more than 100%) associated with a strong decrease in variability (up to 100%) is expected in the SAP and the Otranto Strait. Monthly analysis of surface current speeds indicates a significant increase in the deep domain during wintertime, while summer months show lower increases. Consequently, the number of days with extreme high surface current speeds will double or triple in the deep domain in the far-future climate, while weak surface current events will be decrease by half. At 100 m depth, mean current speeds will increase, particularly in January-May, with almost half of the month characterized by extreme high current speeds. At the bottom, the deep domain will experience a weakening of the currents, particularly in summer when up to 11 days more of extreme weak events per month are expected to occur compared to the historical conditions. In the coastal domain, the changes in current speed are mostly negligible.
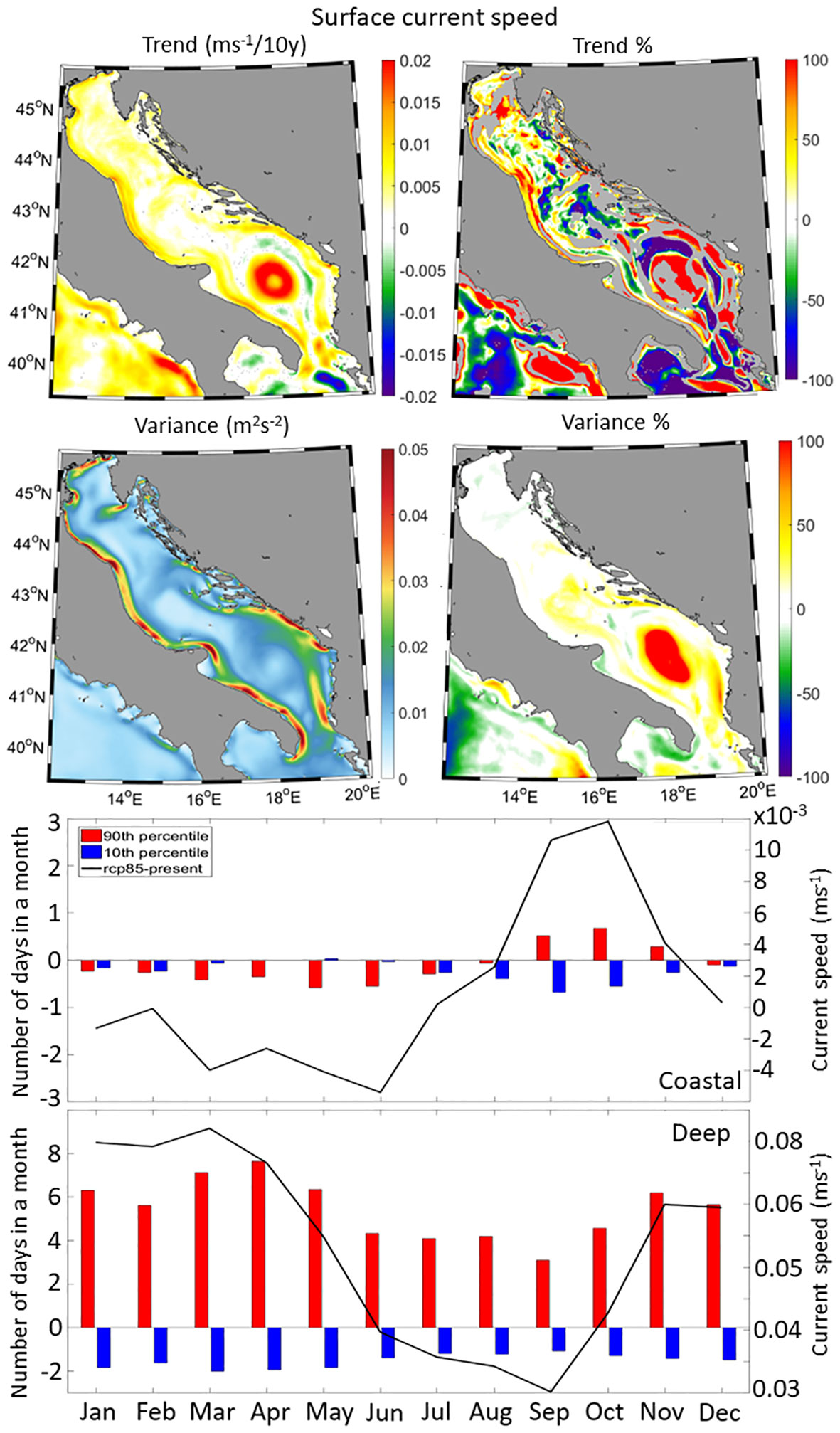
Figure 12 Same as Figure 6 but for the sea surface current speed.
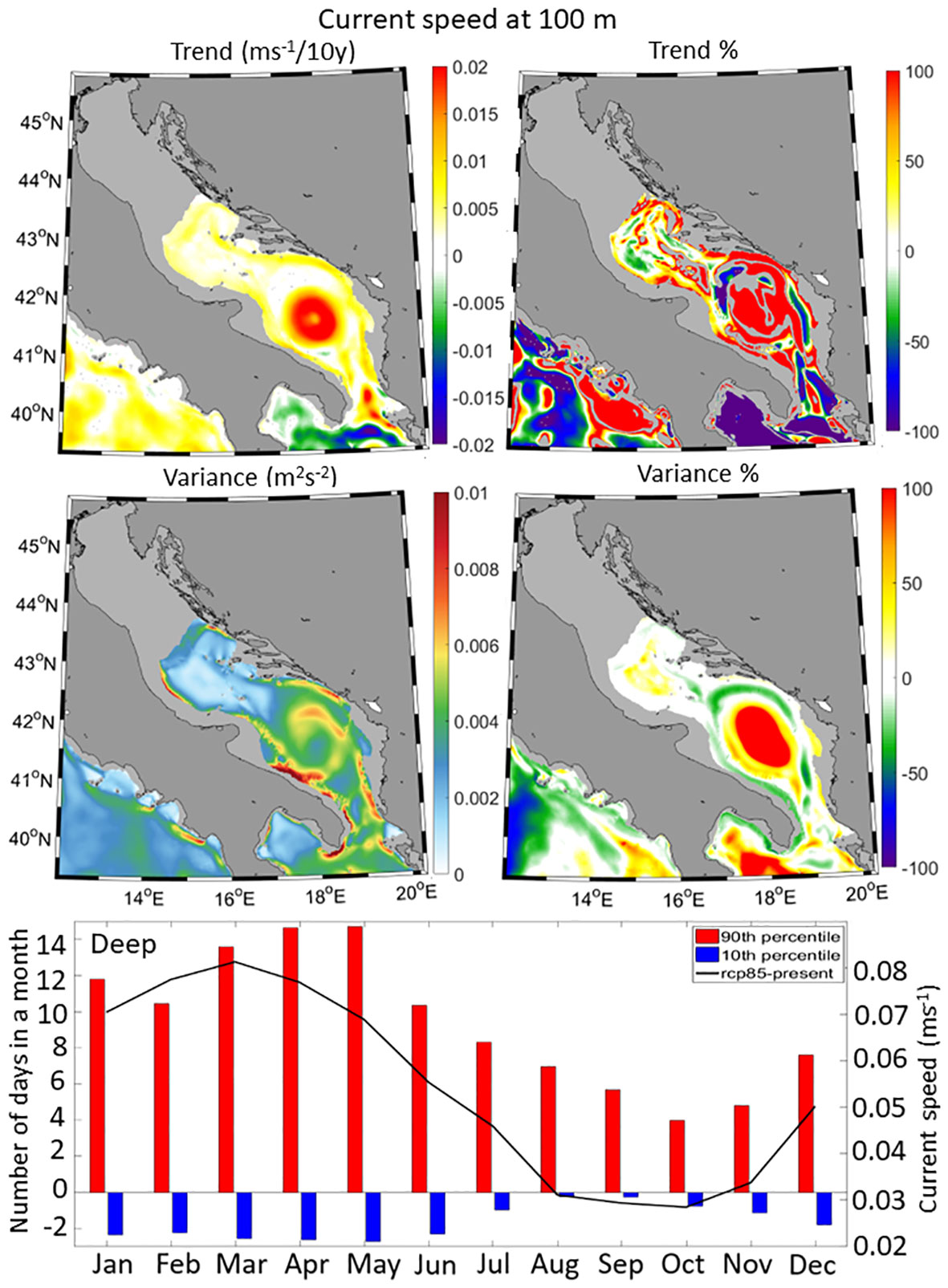
Figure 13 Same as Figure 6 but for the current speed at 100 m. The monthly results for the coastal sub-domain are not presented.
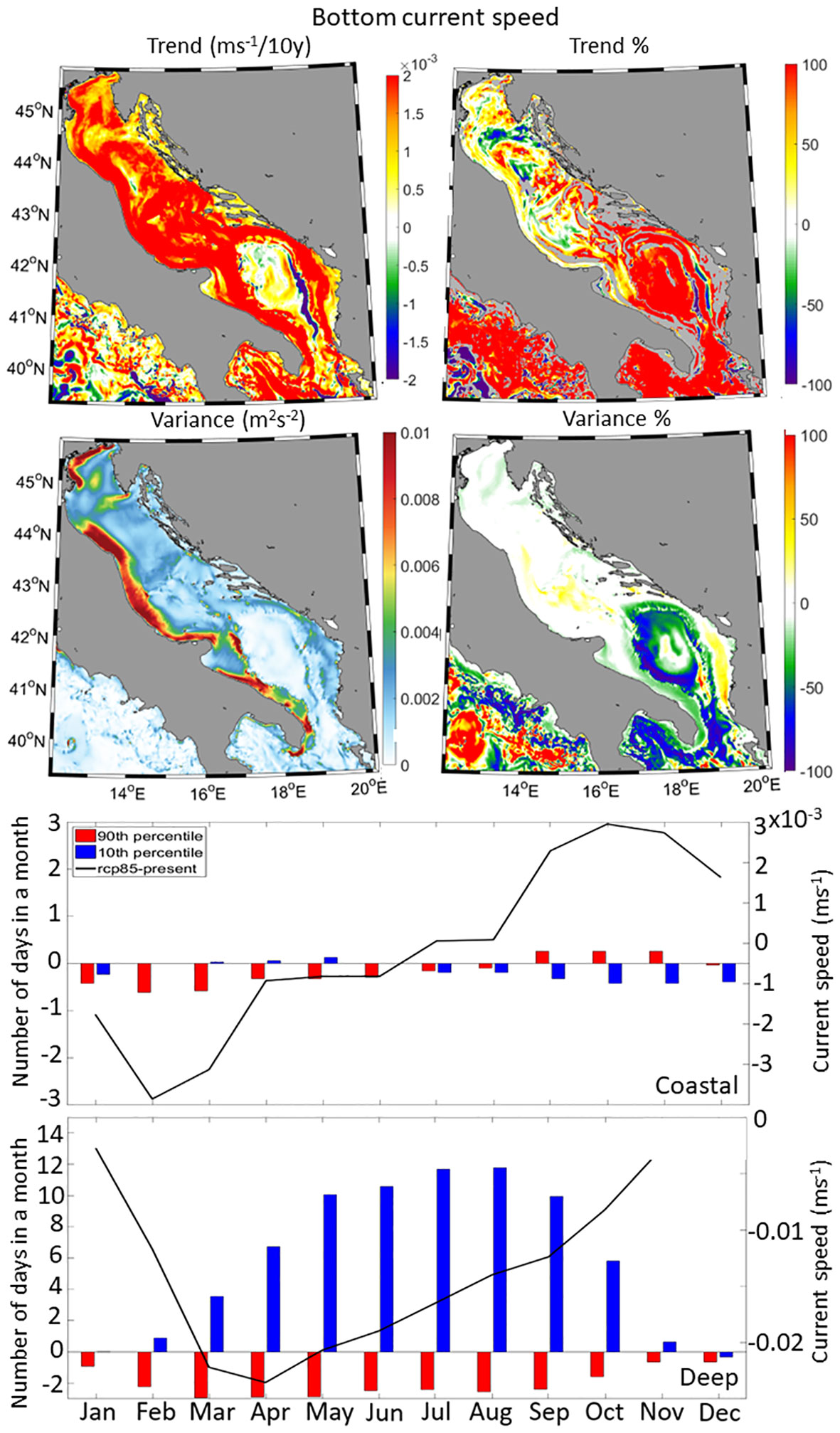
Figure 14 Same as Figure 6 but for the sea bottom current speed.
4 Discussion
In this study, the kilometer-scale historical and extreme warming climate runs of the AdriSC model were compared. The AdriSC model has already been proven to successfully capture the fine-scale dynamics in both the atmosphere (e.g., the bora wind, Denamiel et al., 2021a) and the ocean (e.g., surface cooling, dense water generation, BiOS regimes, Denamiel et al., 2020a; Denamiel et al., 2022; Pranić et al., 2023) under the historical conditions (1987-2017). However, our study is the first to analyze the AdriSC far-future projections which should capture the impact of climate change on extremes events (e.g., convective storms, dense water formation) not well represented in regional climate models with coarser resolutions. The following discussion is thus presenting the relevance of our results with the aim to, first, explain their potential implications for the Adriatic atmosphere-ocean dynamics, and second, compare them with the Mediterranean regional climate models.
4.1 Implications for the atmosphere-ocean dynamics
The presented AdriSC results reveal several changes that can impact the atmosphere-ocean dynamics across the Adriatic region. These changes, summarized in Table 2, are presented below for four different categories: (1) heatwaves, extreme rainfalls, and droughts, (2) dense water formation, (3) salinity budget and (4) Southern Adriatic dynamics.
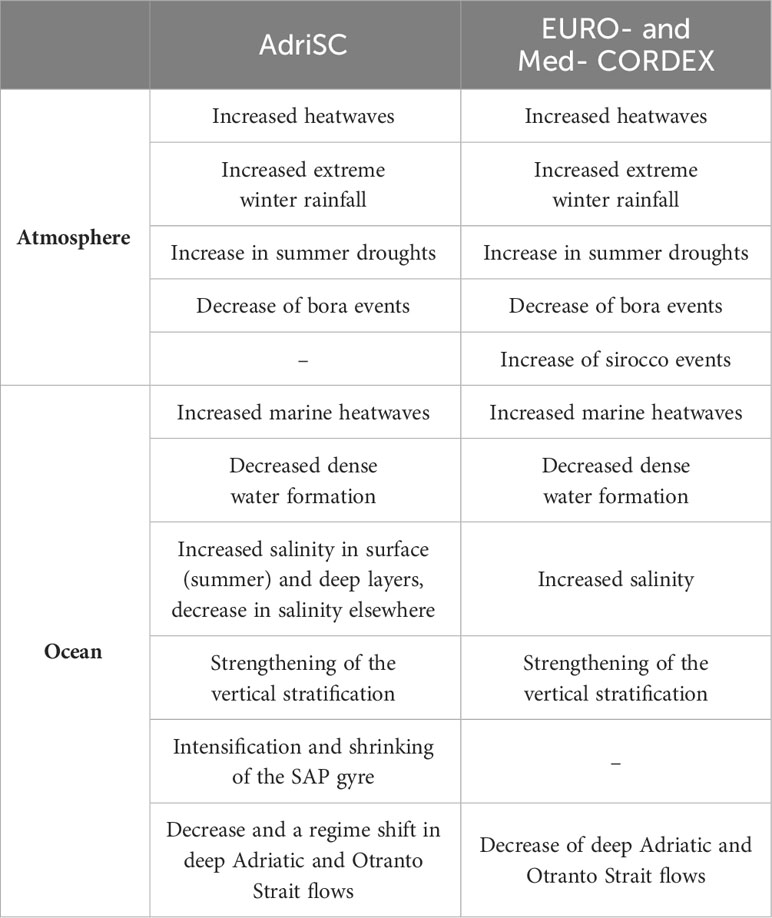
Table 2 Summary of the AdriSC model projections compared to the EURO- and Med-CORDEX RCP8.5 scenario ensembles.
4.1.1 Heatwaves, extreme rainfalls, and droughts
In the atmosphere, our findings suggest much warmer climate during the 2070-2100 period (with positive trends up to 0.5°C per decade) which leads to at least 10 more days per month of extreme heat over land than under the historical conditions. This suggests an increase in the number of heatwaves – as defined in the present climate by Robinson (2001) – which will be particularly intense and prolonged during July-August with 20 more days of extreme heat associated to an average increase in temperatures of 4.3°C. Over the sea, not only the air but also the ocean temperatures are warming at an even faster rate than inland. This leads to at least 20 more days per month of extreme heat than under the historical conditions from the surface to the bottom of the Adriatic Sea, with an average temperature increase ranging from 0.9-1°C at the bottom of the deepest part of the Adriatic to more than 4°C at the surface in the coastal areas. Under these conditions, marine heatwaves – as defined in the present climate by Hobday et al. (2016) – would be nearly continuous. In addition to the heatwaves, the warming conditions projected by the AdriSC model show an increase in rain variability of over 50% across the entire studied region, and up to 100% along the eastern Adriatic coast, compared to the historical conditions. Further, in average, the number of extreme precipitations projected with the AdriSC model will be reduced by up to 33% in May-July, associated with a decrease in relative humidity of 0.2-0.4%, and increased by up to 25% in October-December, associated with an increase in relative humidity of up to 0.8%. As lower relative humidity makes rainfall less likely to occur (Denson et al., 2021), these results go in the direction of expected increases in extreme rainfall in winter (Zittis et al., 2021) and droughts in summer, since in a warmer world, the hydrological cycle will intensify (Allen and Ingram, 2002; Giorgi et al., 2019).
4.1.2 Dense water formation
In the Adriatic, the primary driver of the dense water formation is the cooling of surface waters during the winter season by cold northerly bora winds. These winds enhance surface heat loss and evaporation, causing surface waters to cool rapidly and sink to the deeper layers (Pullen et al., 2006; Janeković et al., 2014; Ličer et al., 2016). The AdriSC far-future results project less intense and less frequent winds over the entire Adriatic region with both a decrease in speed (up to 0.18 m/s over land and 0.3 m/s over sea) and a decrease in the number of extreme high events over the sea (up to 25%) during January-April which is the period during which strong bora events occur. However, several other factors also influence the dense water formation. First, warmer waters during the winter season may slow down the cooling required for dense water formation, potentially reducing the intensity of the process. In the coastal areas of the Adriatic Sea, the AdriSC results show an increase in sea surface temperatures above 4°C during February-March when the dense water formation is known to occur under the historical conditions. Second, new precipitation patterns and river discharges due to climate change may affect the surface salinity values. During January-April, the AdriSC results show an increase in both precipitations over sea (ranging from 2.6 to 3.4 mm/day) and river discharge forcing (compared to the historical conditions, increase by 40-50% in January-February, no increase in March and decrease by less than 10% in April; see Figure 4 in Denamiel et al., 2020b). Providing that the extreme bora events occur during the same period for historical and far-future conditions, the sea surface salinity is thus likely to be largely decreased during the far-future bora events which is confirmed by the increase (decrease) of extreme low (high) sea surface salinity events in the coastal areas by up to 5 days (2 days) per month during this period. Consequently, the density of the dense waters generated by sea surface cooling will also decrease. Third, a decrease in relative humidity during bora events can produce an increase in latent heat losses despite the decrease in bora wind speeds (Denamiel et al., 2020a). The AdriSC results, however, show an increase of the relative humidity over sea by up to 0.8%. Consequently, following these preliminary results the dense water formation within the Adriatic Sea is likely to be largely reduced under far-future extreme warming conditions.
4.1.3 Salinity budget
Noticeable changes in salinity are seen in the AdriSC far-future projections. The increase in salinity in the surface and intermediate layers during summer (up to 0.2 associated with 3 more days per month of extreme high salinity events) signals the prevalence of evaporation over precipitation and river runoff. This phenomenon is already observed in the present climate as surface saline “lakes” (Mihanović et al., 2021). Further, strong surface salinity trends (more than 0.11 per decade) and variability (more than 20) are expected in the vicinity of the Po and Drini river plumes which have the highest discharges in the Adriatic Sea. Finally, within the coastal sub-domain, surface salinity is expected to decrease in October-April when the river discharge forcing has been increased by 10-50% under RCP8.5 conditions and when there is an increase in precipitations over land and sea. As the northern Adriatic is strongly influenced by local freshwater discharges (Raicich, 1996), the salinity budget in this region is mostly following the imposed changes in river discharges in the AdriSC model extreme warming run.
4.1.4 Southern Adriatic dynamics
Over the SAP, the AdriSC results show a decrease in salinity by at least 0.13-0.15 and an increase in temperatures by 3.4-3.6°C of the intermediate waters associated with higher current speeds (up to 0.08 m/s and at least 14 more days per month above the extreme high speeds compared to historical conditions) particularly during the January-May period. These results, which contrast with the coastal areas of the Adriatic Sea, suggest the intensification and shrinking of the southern Adriatic cyclonic gyre in the middle of which, under the historical conditions, upwelling of deep waters can occur (Gačić et al., 2002). Further, at the bottom, the increase in salinity by 0.08-0.09 and the increase in temperatures by 2.5°C less than in the intermediate layer associated with far less variability than under historical conditions (up to 200%) suggest a strengthening of the vertical stratification over the SAP. As the same patterns are observed over the Otranto Strait, this strengthening of the vertical stratification may result from the Adriatic-Ionian exchanges. This weakening and shallowing of the Adriatic-Ionian thermohaline circulation has also been simulated with coarse atmosphere-ocean models (Somot et al., 2006) and likely results from an increase stratification and a decrease in dense water production in the southern Adriatic (Parras-Berrocal et al., 2023). The peculiar behavior of the SAP compared to the coastal areas of the Adriatic could also be linked to boundary issues. However, the AdriSC open boundary is positioned well south of the strait of Otranto and the AdriSC historical simulation didn’t exhibit such behavior. Further, the regional climate model used in the PGW approach, the LMDZ4-NEMOMED8 model, doesn’t display systematic issues and is regularly used in the Med-CORDEX ensembles. The SAP behavior might thus be the consequence of an excessive warming of the Adriatic Sea compared to the central Mediterranean under the far-future climate conditions but the sensitivity to the density interplay at the strait of Otranto should be further studied to reach any firm conclusion.
All the processes discussed above need to be further investigated as trends, variability and extreme events averaged over large subdomains are not appropriate methodologies to understand the atmosphere-ocean dynamical properties.
4.2 Comparison to previous studies and limitations
Two different ensembles of regional climate models (RCMs) projecting climate change under RCP8.5 conditions are available in the Mediterranean region. The EURO-CORDEX ensemble (Coppola et al., 2021) at 0.11° resolution with 14 different RCMs driven by 8 CMIP5 global climate models (i.e., 68 members in total), is mostly composed of atmospheric models (few coupled with ocean models). The Med-CORDEX ensemble (Ruti et al., 2016) mostly composed of atmosphere-land-ocean models is formed of 17 Atmosphere RCMs at 10-50 km resolution, 8 Ocean RCMs at 7-8 km resolution, 4 Land-Surface RCMs and 14 Fully coupled RCMs at about 10 km resolution, driven by at least one of the 8 different CMIP5 global climate models use in EURO-CORDEX. As trends and variability are generally well reproduced by RCMs, these ensembles can thus be used to perform a comparative evaluation of the far-future AdriSC projections (see Table 2).
Ivušić et al. (2021) analyzed the EURO-CORDEX ensemble precipitations over the Adriatic region for the far-future period (2071-2100) with respect to the historical period (1971-2000). They found a considerable reduction of the total precipitations during the summer months associated with a decrease of the number of rainy days over the entire region and strong south-north gradients particularly during the winter months when the precipitation intensity could increase. Baronetti et al. (2022) analyzed both EURO- and Med- CORDEX simulations in northern Italy for the same periods and also found a north-south spatial gradient as well as an intensification of the droughts. As in EURO- and Med- CORDEX simulations, under the far-future AdriSC projections, the number of days with extreme precipitations is also expected to decrease during summer and to increase during winter over both the land and sea domains. In terms of south-north gradients, the most intense positive precipitation trends are mostly located over the Adriatic Sea and below 44°N of latitude, while the negative trends cover the Pannonian plain mostly above 44°N of latitude. However, following the trends and variability analyses, the land-sea contrasts also highlighted in Coppola et al. (2021) are much more pronounced than the south-north gradients in the AdriSC results.
Belušić Vozila et al. (2019) used two members of the EURO-CORDEX ensemble to analyze the far-future near-surface winds over the Adriatic region. They found a reduction in the number of bora events, an increase in the number of sirocco events in the northern Adriatic during the winter season, and a decrease of the intensity of both bora and sirocco events, except in the northern Adriatic for the bora events. The AdriSC far-future results also project a decrease in the intensity of the wind speeds over the entire Adriatic region particularly during winter time as well as a decrease in the number of extreme events above the maximum historical threshold over the sea sub-domain particularly during January-April which is the period during which strong bora events occur. The increase in sirocco events cannot be seen in the presented AdriSC results which average the wind speed conditions over the entire sea sub-domain.
Parras-Berrocal et al. (2023) analyzed the REMO-OASIS-MPIOM model results (Sein et al., 2015) of the Med-CORDEX ensemble and found that the projected dense water formation could be reduced by 75 % in the Adriatic Sea (84 % in the Aegean Sea, 83 % in the Levantine Sea) by the end of the century due to hydrographic changes in surface and intermediate water that strengthen the vertical stratification, hampering vertical mixing and thus convection. As documented by Soto-Navarro et al. (2020), the changes will manifest in an increase of temperature over the whole water column, more at the surface and less in deep layers, while most Med-CORDEX models are projecting an increase in salinity of the whole Adriatic for the RCP8.5 scenario. The AdriSC results are mostly aligned with these findings. First, the northern Adriatic dense water formation is expected to be reduced due to local changes (increased sea surface temperatures, decreased sea surface salinity, decreased wind speeds). Second, the changes in properties of the intermediate (decreased salinity and increased temperatures) and bottom (increased salinity and increased temperatures by 2.5°C less than in the intermediate layer) layers over the SAP seen by the AdriSC model also confirm a strengthening of the vertical stratification potentially linked to the Ionian-Adriatic exchanges.
Finally, the limitations of the AdriSC far-future projections are twofold. First, the PGW method uses the same synoptic forcing in both historical and extreme warming runs which implies that potential changes in intra- and interannual variability might be missed. Second, as the results of only one RCM are used in the AdriSC PGW approach, the climate uncertainty, derived by running ensembles of simulations forced by multiple global climate models under multiple warming scenarios (Semenov and Stratonovitch, 2010), is ignored.
5 Conclusions
The AdriSC projections of the far-future Adriatic climate indicate several extreme changes: (1) strong land-sea contrasts in the atmosphere, (2) intensification of the heatwaves and most likely of the extreme rainfalls and droughts, (3) dense water formation in the northern Adriatic likely to be strongly reduced, (4) potential intensification of the surface saline lake effect in during summer, (5) southern Adriatic cyclonic gyre likely to be shrinking and intensified, and (6) strengthening of the vertical stratification over the SAP.
Some of these changes have already begun to manifest in the recent years, like substantially higher temperatures, causing more frequent and prolonged heatwaves during the summer months (Climate Risk Profile: Croatia, 2021). The AdriSC climate model also suggests that the Adriatic region may experience some changes in precipitation patterns. Changes in timing and intensity of rainfall events could lead to increased risk of droughts or floods in different parts of the region. Precipitation extremes in the Adriatic region are a subject of interest and concern due to their impact on the environment, society, and economy (Krvavica et al., 2023). Droughts pose serious threats to agriculture, water resources, and ecosystems. They lead to reduced crop yields, and increased demand for irrigation, put pressure on water resources, affecting water availability for drinking, industrial purposes, and hydropower generation. In summer of 2022, in Istria, Croatia, water reduction measures were implemented due to severe drought (Turistička zajednica Istarske županije, 2022). Conversely, the Adriatic region is also experiencing extreme rainfall events, characterized by intense and heavy precipitation over short periods. These events can pose significant threats to people and infrastructure. In July 2023, Slovenia, Eastern Croatia and city of Zagreb area experienced extreme storms, associated with strong winds and heavy rain, that took three human lives and caused substantial infrastructural damages (Dešković, 2023). Further, in the Adriatic Sea, higher salinities, temperatures and marine heatwaves may also impact marine life, fisheries and aquaculture production (Pranovi et al., 2013), affecting the distribution and abundance of various species (Kinne, 1964; Einarson, 1993), and influencing migration patterns, in which species of cold seas like shrimps (Nephrops norvegicus, Canu et al., 2021) have bad perspective, although having nursery ground in the coldest deep part of the Adriatic Sea, the Jabuka Pit.
Given the negative impact of these climate events for humans, and for various economic sectors, including agriculture, tourism, fisheries, and infrastructure, understanding, and predicting these changes is crucial for policymakers and local communities to develop effective adaptation and mitigation strategies. Consequently, the AdriSC experiment should now be generalized by implementing and running more kilometer-scale atmosphere-ocean models forced by more regional models under more climate scenarios in the Adriatic region. This ensemble of simulations could then be used to derive robust climate hazard assessments at the local scale, with higher reliability of reproduction of extreme values and more reliable quantification and projections of the most extreme events in both atmosphere and ocean (e.g., coastal flooding, flashfloods, droughts, wildfires, etc.) than the available ensembles of coarser resolution Mediterranean models.
Data availability statement
The datasets presented in this study can be found in online repositories. The names of the repository/repositories and accession number(s) can be found below: The model results used to produce this article can be obtained under the Open Science framework (OSF) through the FAIR data repository at https://osf.io/h8sjd/(https://doi.org/10.17605/OSF.IO/H8SJD) and at ZENODO repository https://zenodo.org/records/10046622. The code of the AdriSC climate model used in this article can be obtained under the Open Science Framework (OSF) FAIR data repository https://osf.io/zb3cm/ (Denamiel, 2021).
Author contributions
IT: Data curation, Formal analysis, Investigation, Visualization, Writing – original draft. CD: Conceptualization, Formal analysis, Funding acquisition, Investigation, Methodology, Supervision, Writing – review & editing. IV: Formal analysis, Investigation, Methodology, Supervision, Writing – review & editing.
Funding
The author(s) declare financial support was received for the research, authorship, and/or publication of this article. Center of Excellence for Exascale in Solid Earth (ChEESE-2P), HORIZON-EUROHPC-JU-2021-COE-01, Grant Agreement No 101093038 and European Centre for Middle-range Weather Forecast (ECMWF) Special Projects: (1) Numerical modelling of the Adriatic-Ionian decadal and inter-annual oscillation: from realistic simulations to process oriented experiments. HRZZ (Croatian Science Foundation) Project: DOK-2021-02-7189.; (2) Using stochastic surrogate methods for advancing towards reliable meteotsunami early warning system.
Acknowledgments
The support of the European Centre for Medium-Range Weather Forecasts (ECMWF), which provided staff assistance, with a special thanks to Xavier Abellan and Carsten Maass, as well as the computing and archive facilities used in this research, is acknowledged.
Conflict of interest
The authors declare that the research was conducted in the absence of any commercial or financial relationships that could be construed as a potential conflict of interest.
Publisher’s note
All claims expressed in this article are solely those of the authors and do not necessarily represent those of their affiliated organizations, or those of the publisher, the editors and the reviewers. Any product that may be evaluated in this article, or claim that may be made by its manufacturer, is not guaranteed or endorsed by the publisher.
References
Allen M., Ingram W. (2002). Constraints on future changes in climate and the hydrologic cycle. Nature 419, 224–232. doi: 10.1038/nature01092
Artegiani A., Bregant D., Paschini E., Pinardi N., Raicich F., Russo A. (1997). The Adriatic Sea general circulation, Part I: Air–sea interactions and water mass structure. J. Phys. Oceanogr. 27, 1492–1514. doi: 10.1175/1520-0485(1997)027<1492:TASGCP>2.0.CO;2
Balsamo G., Albergel C., Beljaars A., Boussetta S., Brun E., Cloke H., et al. (2015). ERA-Interim/Land: a global land surface reanalysis data set. Hydrol. Earth Syst. Sci. 19, 389–407. doi: 10.5194/hess-19-389-2015
Baronetti A., Dubreuil V., Provenzale A., Fratianni S (2022). Future droughts in northern Italy: high-resolution projections using EURO-CORDEX and MED-CORDEX ensembles. Clim. Change 172, 22. doi: 10.1007/s10584-022-03370-7
Belušić Vozila A., Güttler I., Ahrens B., Obermann-Hellhund A., Telišman Prtenjak M. (2019). Wind over the Adriatic region in CORDEX climate change scenarios. J. Geophys. Res. Atmos. 124, 110–130. doi: 10.1029/2018JD028552
Beuvier J., Sevault F., Herrmann M., Kontoyiannis H., Ludwig W., Rixen M., et al. (2010). Modeling the Mediterranean Sea interannual variability during 1961–2000: Focus on the Eastern Mediterranean Transient. J. Geophys. Res. 115, C08017. doi: 10.1029/2009JC005950
Bonacci O., Bonacci D., Patekar M., Pola M. (2021). Increasing trends in air and sea surface temperature in the central Adriatic Sea (Croatia). J. Mar. Sci. Eng. 9, 358. doi: 10.3390/jmse9040358
Canu M., Laurent C. D., Morello E. B., Querin S., Scarcella G., Vrgoč N., et al. (2021). Nephrops norvegicus in the Adriatic Sea: Connectivity modelling, essential fish habitats, and management area network. Fish. Oceanogr. 30, 349–365. doi: 10.1111/fog.12522
Chow F. K., Schär C., Ban N., Lundquist K. A., Schlemmer L., Shi X. (2019). Crossing multiple gray zones in the transition from mesoscale to microscale simulation over complex terrain. Atmosphere 10, 274. doi: 10.3390/atmos10050274
Civitarese G., Gačić M., Batistić M., Bensi M., Cardin V., Dulčić J., et al. (2023). The BiOS mechanism: History, theory, implications. Prog. Oceanogr. 216, 103056. doi: 10.1016/j.pocean.2023.103056
Coppola E., Nogherotto R., Ciarlò J. M., Giorgi F., van Meijgaard E., Kadygrov N., et al. (2021). Assessment of the European climate projections as simulated by the large EURO-CORDEX regional and global climate model ensemble. J. Geophys. Res. Atmos. 126, e2019JD032356. doi: 10.1029/2019JD032356
Denamiel C., Šepić J., Ivanković D., Vilibić I. (2019). The Adriatic Sea and Coast modelling suite: evaluation of the meteotsunami fore-cast component. Ocean Model. 135, 71–93. doi: 10.1016/j.ocemod.2019.02.003
Denamiel C., Tojčić I., Vilibić I. (2020a). Far future climate, (2060–2100) of the northern Adriatic air–sea heat transfers associated with extreme bora events. Clim. Dyn. 55, 3043–3066. doi: 10.1007/s00382-020-05435-8
Denamiel C., Pranić P., Quentin F., Mihanović H., Vilibić I. (2020b). Pseudo-global warming projections of extreme wave storms in complex coastal regions: the case of the Adriatic Sea. Clim. Dyn. 55, 2483–2509. doi: 10.1007/s00382-020-05397-x
Denamiel C. (2021). AdriSC Climate Model: evaluation run. (Open Science Framework). doi: 10.17605/OSF.IO/ZB3CM
Denamiel C., Tojčić I., Vilibić I. (2021a). Balancing accuracy and efficiency of atmospheric models in the northern Adriatic during severe bora events. J. Geophys. Res. Atmos. 126, e2020JD033516. doi: 10.1029/2020JD033516
Denamiel C., Pranić P., Ivanković D., Tojčić I., Vilibić I. (2021b). Performance of the Adriatic Sea and coast (AdriSC) climate component—a COAWST V3.3-based coupled atmosphere–ocean modelling suite: atmospheric dataset. Geosci. Model. Dev. 14, 3995–4017. doi: 10.5194/gmd-14-3995-2021
Denamiel C., Tojčić I., Pranić P., Vilibić I. (2022). Modes of the BiOS-driven Adriatic Sea thermohaline variability. Clim. Dyn. 59, 1097–1113. doi: 10.1007/s00382-022-06178-4
Denson E., Wasko C., Peel M. (2021). Decreases in relative humidity across Australia. Environ. Res. Lett. 16. doi: 10.1088/1748-9326/ac0aca
Dešković M. (2023). Oluja razorila Zagreb. Available at: https://www.jutarnji.hr/vijesti/zagreb/uragan-debela-stabla-lomio-kao-sibice-susjedi-zrtve-nije-mogao-pobjeci-bio-je-nepokretan-15357204 (Accessed 10 October 2023).
Dunić N., Vilibić I., Šepić J., Mihanović H., Sevault F., Somot S., et al. (2019). Performance of multi-decadal ocean simulations in the Adriatic Sea. Ocean Model. 134, 84–109. doi: 10.1016/j.ocemod.2019.01.006
Dunić N., Vilibić I., Šepić J., Somot S., Sevault F. (2018). Dense water formation and BiOS-induced variability in the Adriatic Sea simulated using an ocean regional circulation model. Clim. Dyn. 51, 1211–1236. doi: 10.1007/s00382-016-3310-5
Einarson S. (1993). Effects of temperature, sea water osmolality and season on oxygen consumption and osmoregulation of the amphipod Gammarus oceanicus. Mar. Biol. 117, 599–606. doi: 10.1007/BF00349771
Gačić M., Borzelli G. L. E., Civitarese G., Cardin V., Yari S. (2010). Can internal processes sustain reversals of the ocean upper circulation? The Ionian Sea example. Geophys. Res. Lett. 37, L09608. doi: 10.1029/2010GL043216
Gačić M., Civitarese G., Miserocchi S., Cardin V., Crise A., Mauri E. (2002). The open-oceanconvection in the southern Adriatic: A controlling mechanism of the spring phytoplankton bloom. Cont. Shelf Res. 22, 1897–1908. doi: 10.1016/S0278-4343(02)00050-X
Gajić-Čapka M. (1993). Fluctuations and trends of annual precipitation in different climatic regions of Croatia Theor. Appl. Climatol. 47, 215–221. doi: 10.1007/BF00866242
Gajić-Čapka M., Cindrić K., Pasarić Z. (2014). Trends in precipitation indices in Croatia 1961–2010. Theor. Appl. Climatol. 121, 167–177. doi: 10.1007/s00704-014-1217-9
Giorgi F., Raffaele F., Coppola E. (2019). The response of precipitation characteristics to global warming from climate projections. Earth Syst. Dyn. 10, 73–89. doi: 10.5194/esd-10-73-2019
Grisogono B., Belušić D. (2009). A review of recent advances in understanding the meso- and microscale properties of the severe Bora wind. Tellus A 61, 1–16. doi: 10.1111/j.1600-0870.2008.00369.x
Hobday A. J., Alexander L. V., Perkins S. E., Smale D. A., Straub S. C., Oliver E. C. J., et al. (2016). A hierarchical approach to defining marine heatwaves. Prog. Oceanogr. 141, 227–238. doi: 10.1016/j.pocean.2015.12.014
Hourdin F., Musat I., Bony S., Braconnot P., Codron F, Dufresneet J. L, et al. (2006). The LMDZ4 general circulation model: climate performance and sensitivity to parametrized physics with emphasis on tropical convection. Clim. Dyn. 27, 787–813. doi: 10.1007/s00382-006-0158-0
IPCC (2022). Climate change 2022: impacts, adaptation, and vulnerability. Contribution of working group II to the sixth assessment report of the intergovernmental panel on climate change. Eds. Pörtner H.-O., Roberts D. C., Tignor M., Poloczanska E. S., Mintenbeck K., Alegría A., Craig M., Langsdorf S., Löschke S., Möller V., Okem A., Rama B. (Cambridge, UK and New York, NY, USA: Cambridge University Press), 3056. doi: 10.1017/9781009325844
Ivušić S., Güttler I., Horvath K. (2021). Future precipitation changes over the eastern Adriatic and Dinaric Alps areas in the latest EURO-CORDEX ensemble, EMS Annual Meeting 2021. online, 6–10 Sep 2021, EMS2021-12. doi: 10.5194/ems2021-12
Janeković I., Mihanović H., Vilibić I., Tudor M. (2014). Extreme cooling and dense water formation estimates in open and coastal regions of the Adriatic Sea during the winter of 2012. J. Geophys. Res. Oceans 119, 3200–3218. doi: 10.1002/2014JC009865
Kinne O. (1964). The effects of temperature and salinity on marine and brackish water animals: 2. Salinity temperature-salinity combinations. Oceanogr. Mar. Biol. Ann. Rev. 2, 281–339.
Krvavica N., Šiljeg A., Horvat B., Panđa L. (2023). Pluvial flash flood hazard and risk mapping in Croatia: Case study in the Gospić catchment. Sustainability 15, 1197. doi: 10.3390/su15021197
Laprise R. (1992). The Euler equations of motion with hydrostatic pressure as an independent variable. Mon. Weather Rev. 120, 197–207. doi: 10.1175/1520-0493(1992)120<0197:TEEOMW>2.0.CO;2
Ličer M., Smerkol P., Fettich A., Ravdas M., Papapostolou A., Mantziafou A., et al. (2016). Modelling the ocean and atmosphere during an extreme bora event in northern Adriatic using one-way and two-way atmosphere–ocean coupling. Ocean Sci. 12, 71–86. doi: 10.5194/os-12-71-2016
Lionello P., Malanotte-Rizzoli P., Boscolo R., Pinhas A., Artale V., Li L., et al. (2006). The Mediterranean climate: An overview of the main characteristics and issues. Developments Earth Environ. Sci. 4, 1–26. doi: 10.1016/S1571-9197(06)80003-0
Lipizer M., Partescano E., Rabitti A., Giorgetti A., Crise A. (2014). Qualified temperature, salinity and dissolved oxygen climatologies in a changing Adriatic Sea. Ocean Sci. 10, 771–797. doi: 10.5194/os-10-771-2014
Mann H. B. (1945). Non-parametric tests against trend. Econometrica 13, 163–171. doi: 10.2307/1907187
Mihanović H., Vilibić I., Dunić N., Šepić J. (2015). Mapping of decadal middle Adriatic oceanographic variability and its relation to the BiOS regime. J. Geophys. Res. Oceans 120, 5615–5630. doi: 10.1002/2015JC010725
Mihanović H., Vilibić I., Šepić J., Matić F., Ljubešić Z., Mauri E., et al. (2021). Observation, preconditioning and recurrence of exceptionally high salinities in the Adriatic Sea. Front. Mar. Sci. 8. doi: 10.3389/fmars.2021.672210
Mondal A., Kundu S., Mukhopadhyay A. (2012). Rainfall trend analysis by Mann-Kendall test: a case study of north-eastern part of Cuttack district, Orissa. Int. J. Geol. Earth Environ. Sci. 2, 70–78.
Munoz-Esparza D., Lundquist J. K., Sauer J. A., Kosović B., Linn R. R. (2017). Coupled mesoscale-LES modeling of a diurnal cycle during the CWEX-13 field campaign: From weather to boundary-layer eddies. J. Adv. Model. Earth Syst. 9, 1572–1594. doi: 10.1002/2017MS000960
Ogrin D. (2015). Long-term air temperature changes in Ljubljana (Slovenia) in comparison to Trieste (Italy) and Zagreb (Croatia). Morav. Geogr. Rep. 23, 17–26. doi: 10.1515/mgr-2015-0014
Parras-Berrocal I. M., Vazquez R., Cabos W., Sein D. V., Álvarez O., Bruno M., et al. (2023). Dense water formation in the Eastern Mediterranean under global warming scenario. EGUsphere. doi: 10.5194/egusphere-2023-159
Pastor F., Valiente J. A., Palau J. L. (2018). Sea surface temperature in the Mediterranean: Trends and spatial patterns, (1982–2016), Pure Appl. Geophys. 175, 4017–4029. doi: 10.1007/s00024-017-1739-z
Pinardi N., Allen I., Demirov E., De Mey P., Korres G., Lascaratos A., et al. (2003). The Mediterranean ocean Forecasting System: frst phase of implementation, (1998–2001). Ann. Geophys. 21, 3–20. doi: 10.5194/angeo-21-3-2003
Pranić P., Denamiel C., Janeković I., Vilibić I. (2023). Multi-model analysis of the Adriatic dense-water dynamics. Ocean Sci. 19, 649–670. doi: 10.5194/os-19-649-2023
Pranić P., Denamiel C., Vilibić I. (2021). Performance of the Adriatic Sea and coast (AdriSC) climate component—a COAWST V3.3-based one-way coupled atmosphere–ocean modelling suite: Ocean results. Geosci. Model. Dev. 14, 5927–5955. doi: 10.5194/gmd-14-5927-2021
Pranovi F., Caccin A., Franzoi P., Malavasi S., Zucchetta M., Torricelli P. (2013). Vulnerability of artisanal fisheries to climate change in the Venice Lagoona. J. Fish Biol. 83, 847–864. doi: 10.1111/jfb.12124
Pullen J., Doyle J. D., Signell R. P. (2006). Two-way air–sea coupling: a study of the Adriatic. Mon. Weather Rev. 134, 1465–1483. doi: 10.1175/MWR3137.1
Radilović S., Koračin D., Denamiel C., Belušić D., Güttler I., Vilibić I. (2020). Simulated and observed air temperature trends in the eastern Adriatic. Atmos. Sci. Lett. 21, e951. doi: 10.1002/asl.951
Raicich F. (1996). On the fresh balance of the Adriatic Sea. J. Mar. Syst. 9, 305–319. doi: 10.1016/S0924-7963(96)00042-5
Robinson P. J. (2001). On the definition of a heat wave. J. Appl. Meteor. Climatol. 40, 762–775. doi: 10.1175/1520-0450(2001)040<0762:OTDOAH>2.0.CO;2
Ruti P., Somot S., Giorgi F., Dubois C., Flaounas E., Obermann A., et al. (2016). Med-CORDEX initiative for Mediterranean climate studies. Bull. Am. Meteorol. Soc 97, 1187–1208. doi: 10.1175/BAMS-D-14-00176.1
Schär C., Frei C., Luthi D., Davies H. C. (1996). Surrogate climate-change scenarios for regional climate models. Geophys. Res. Lett. 23, 669–672. doi: 10.1029/96GL00265
Sein D. V., Mikolajewicz U., Gröger M., Fast I., Cabos W., Pinto J. G., et al. (2015). Regionally coupled atmosphere-ocean sea ice-marine biogeochemistry model ROM: 1. Description and validation. J. Adv. Model. Earth Sy. 7, 268–304. doi: 10.1002/2014MS000357
Semenov M., Stratonovitch P. (2010). Use of multi-model ensembles from global climate models for assessment of climate change impacts. Clim. Res. 41, 1–14. doi: 10.3354/cr00836
Shchepetkin A. F., McWilliams J. C. (2009). Computational kernel algo-rithms for fine-scale, multiprocess, longtime oceanic simulations. Handb. Numerical Anal. 14, 121–183. doi: 10.1016/S1570-8659(08)01202-0
Skamarock W. C., Klemp J. B., Dudhia J., Gill D. O., Barker D. M., Wang W., et al. (2005). A description of the advanced research WRF version 2 (No. NCAR/TN-468+STR). (University Corporation for Atmospheric Research). doi: 10.5065/D6DZ069T
Somot S., Sevault F., Déqué M. (2006). Transient climate change scenario simulation of the Mediterranean Sea for the twenty-first century using a high-resolution ocean circulation model. Clim. Dyn. 27, 851–879. doi: 10.1007/s00382-006-0167-z
Soto-Navarro J., Jordá G., Amores A., Cabos W., Somot S., Sevault F., et al. (2020). Evolution of Mediterranean Sea water properties under climate change scenarios in the Med-CORDEX ensemble. Clim. Dyn. 54, 2135–2165. doi: 10.1007/s00382-019-05105-4
Tojčić I., Denamiel C., Vilibić I. (2023). Kilometer-scale trends and variability of the Adriatic present climate, (1987–2017). Clim. Dyn 61, 2521–2545. doi: 10.1007/s00382-023-06700-2
Turistička zajednica Istarske županije (2022). Redukcija vode u Istri. Available at: https://www.istra.hr/hr/informacije/planiranje-putovanja/korisne-informacije/potrosnja-vode-u-istri (Accessed 10 October 2023).
Vicente-Serrano S. M., Nieto R., Gimeno L., Azorin-Molina C., Drumond A., El Kenawy A., et al. (2018). Recent changes of relative humidity: regional connections with land and ocean processes. Earth Syst. Sci. 9, 915–937. doi: 10.5194/esd-9-915-2018
Vilibić I., Šepić J., Proust N. (2013). Weakening thermohaline circulation in the Adriatic Sea. Clim. Res. 55, 217–225. doi: 10.3354/cr01128
Vilibić I., Zemunik P., Šepić J., Dunić N., Marzouk O., Mihanović H., et al. (2019). Present-climate trends and variability in thermohaline properties of the northern Adriatic shelf. Ocean Sci. 15, 1351–1362. doi: 10.5194/os-15-1351-2019
Warner J. C., Armstrong B., He R., Zambon J. B. (2010). Development of a coupled Ocean–Atmosphere–Wave–Sediment Transport (COAWST) modelling system. Ocean Model. 35, 230–244. doi: 10.1016/j.ocemod.2010.07.010
Wiersema D., Lundquist K., Chow F. (2018). “Development of a multi-scale modeling framework for urban simulations in the Weather Research and Forecasting model,” in Proceedings of the 23rd symposium on boundary layers and turbulence, Oklahoma City, OK, USA (Boston, MA, USA: American Meteorological Society).
Zhou B., Chow F. K. (2014). Nested large-eddy simulations of the intermittently turbulent stable atmospheric boundary layer over real terrain. J. Atmos. Sci. 71, 1021–1039. doi: 10.1175/JAS-D-13-0168.1
Keywords: Adriatic sea, far-future extreme warming, kilometer-scale atmosphere-ocean modelling, climate modeling, climate change
Citation: Tojčić I, Denamiel C and Vilibić I (2024) Kilometer-scale trends, variability, and extremes of the Adriatic far-future climate (RCP 8.5, 2070−2100). Front. Mar. Sci. 11:1329020. doi: 10.3389/fmars.2024.1329020
Received: 27 October 2023; Accepted: 15 January 2024;
Published: 30 January 2024.
Edited by:
Yang-Ki Cho, Seoul National University, Republic of KoreaReviewed by:
Francesco Maicu, Foundation Euro-Mediterranean Center on Climate Change (CMCC), ItalyDavide Pasquali, University of L’Aquila, Italy
Copyright © 2024 Tojčić, Denamiel and Vilibić. This is an open-access article distributed under the terms of the Creative Commons Attribution License (CC BY). The use, distribution or reproduction in other forums is permitted, provided the original author(s) and the copyright owner(s) are credited and that the original publication in this journal is cited, in accordance with accepted academic practice. No use, distribution or reproduction is permitted which does not comply with these terms.
*Correspondence: Iva Tojčić, iva.tojcic@irb.hr