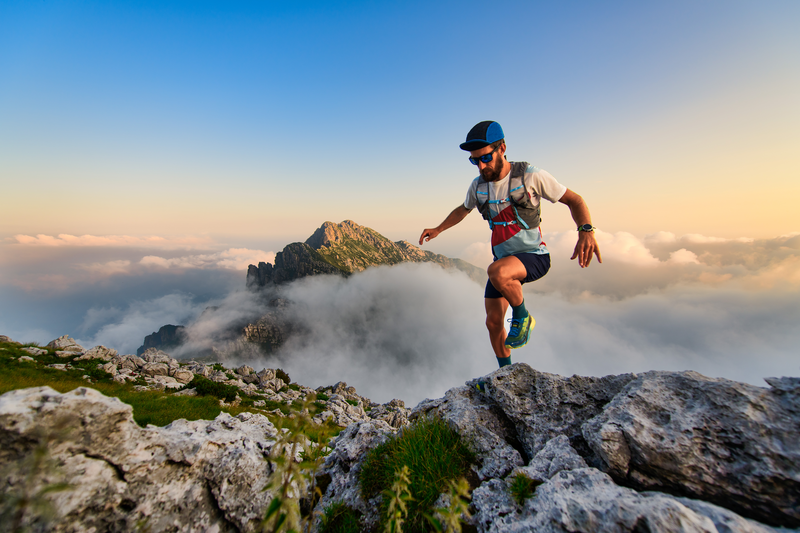
95% of researchers rate our articles as excellent or good
Learn more about the work of our research integrity team to safeguard the quality of each article we publish.
Find out more
ORIGINAL RESEARCH article
Front. Mar. Sci. , 23 January 2024
Sec. Marine Megafauna
Volume 11 - 2024 | https://doi.org/10.3389/fmars.2024.1327752
Baleen whales are one of the few vertebrate taxa for which there are no confirmed estimates of longevity or methods of age determination. Lamina counts in the waxy earplug are assumed to represent age, but ageing accuracy is completely unknown. In this study, bomb radiocarbon assays of the earplug growth sequence in three adult fin whales (Balaenoptera physalus) were used to prepare the most complete within-individual bomb radiocarbon chronologies yet reported for any vertebrate. The whale radiocarbon chronologies matched those of known-age carbonate reference chronologies very well, indicating that the earplug laminae were both metabolically stable and formed throughout the life of the whale. Earplug lamina counts accurately represented absolute ages of 65-85 yr to within 6% of the correct age. Detection of a significant declining trend in δ13C with year of lamina formation within individual whales was consistent with that of the Suess effect, again underlining the metabolic stability of the earplug laminae. Given our results, recent applications of earplug laminae for reconstructing diet and life history events appear to be firmly based, with the potential for further elemental and isotopic applications analogous to those of the otolith.
Despite their status as being among the largest and most charismatic organisms on the Earth (Albert et al., 2018), baleen whales (Mysticeti) are conspicuously devoid of an accepted method of reliable age determination. Age determination protocols of known accuracy exist for almost all other non-avian vertebrate taxa, taking advantage of annually deposited growth increments visible in otoliths (Campana, 2001), vertebrae (Ong et al., 2020) and other calcified tissues (Braaten et al., 2015) of teleosts and elasmobranchs, in bones and scutes of reptiles (Frýdlová et al., 2020), and in teeth and bones of terrestrial mammals (Klevezal, 2017). Among marine mammals, growth increments in the teeth have been confirmed to form annually throughout the lifetime of both seals (Frie et al., 2012) and toothed whales (Stewart et al., 2006). The absence of teeth and other growth-banded calcified tissues in baleen whales is thus not only unusual, it can be problematic for calculations of growth, longevity and mortality rate.
The absence of a direct and accurate age indicator for baleen whale species has prompted the development of several indirect proxies for age. Aspartic acid racemization in the eye lens (Olsen and Sunde, 2002), fatty acid composition of the blubber (Herman et al., 2009), stable isotope and hormone cycles in the baleen plate (Hunt et al., 2022), and ovarian corpora counts (George et al., 2021) have all been used to predict age, but all are correlative techniques with limited utility for individual ages. Bomb radiocarbon assays of the eye lens have been used to provide lower limits for age determinations, but eye lenses cannot be used to provide more precise ages (Boye et al., 2020). More recently, epigenetic clocks have been shown to be highly correlated with relative age across mammalian species (Lu et al., 2023, but again, the method is not as well suited for estimating individual age. The only direct ageing method, and the method most often applied to baleen whales, is that of lamina counts in the waxy earplug (Laws and Purves, 1956).
A whale’s ear plug is a laminated structure composed of keratin and lipids found in the auditory meatus of baleen whales (Rehorek et al., 2019). Although the exact cause of the formation of the laminations has yet to be determined, comparison with ovarian corpora counts has suggested annual formation through at least part of the life history (van Utrecht and van Utrecht-Cock, 1969), although biannual formation has also been reported (Chittleborough, 1960). Re-sighting observations made up to 27 yr apart are more consistent with annual formation than biannual formation (Gabriele et al., 2010), but to this point, there are no direct age assessments that confirm that earplug laminations provide an accurate measure of age for any species of baleen whale. Therefore, it is ironic that the earplug has become a standard against which many other ageing methods are judged (i.e. Christensen, 1981; Nielsen et al., 2013). Schweder (2000) noted that uncertainty about the interpretation of fin whale earplugs contributed to the subsequent over-harvesting and mismanagement of the Antarctic fin whale population. But to this day, all age interpretations of harvested baleen whales are based on the assumption that earplug growth increments provide an accurate measure of age.
Bomb radiocarbon assays are one of the best available techniques for determining the accuracy of an ageing method or the absolute age of a long-lived animal (Campana, 2001). The atmospheric testing of atomic bombs in the 1950s and 1960s resulted in a rapid and well-documented increase in atmospheric radiocarbon, which was subsequently deposited in the calcified tissues of all surface-dwelling organisms. In the pelagic marine environment, the bomb radiocarbon first became apparent around 1958, peaking around 1968. The period of radiocarbon increase was virtually synchronous around the world, thus making it an ideal dated marker for any organism forming growth bands during the 1960s (Campana, 2001; Andrews et al., 2016). In the current study, we assayed bomb radiocarbon along the entire growth sequence in three fin whale (Balaenoptera physalus) earplugs to determine if the ages based on earplug growth increment counts were indeed accurate measures of age. A second objective was to provide an initial assessment of the metabolic stability of the earplug laminae through comparison of the within-whale chronologies of Δ14C and δ13C values with known interannual trends, and thus comment on recent and potential applications of earplug laminae for reconstructing past location and diet.
Earplug samples were collected from three fin whales killed as part of a commercial hunt in Iceland in the years 2013 and 2015 (Table 1). The whales sampled were caught in the waters off western Iceland and flensed at the factory Hvalur H/F, located in Hvalfjörður. The whales were brought to shore within 24 hours of capture and then flensed, which took 3-6 hours. After flensing, the head was removed, after which technicians from the Marine and Freshwater Research Institute (MFRI) extracted the earplugs by cutting away the tissues surrounding the plug.
Age estimates were initially made by the MFRI before storage in formalin. In preparation for radiocarbon sample extraction, earplugs were rinsed in water and then photographed intact using an SLR camera under reflected light at a resolution of 4032 × 3024. The digital images were enhanced for contrast using Adobe Photoshop and the growth increments subsequently counted for age determination by SC and SVF; final ages were those of SC. Growth increment interpretation was similar to that used in other marine vertebrates (Campana, 2001), where adjacent opaque and translucent zones are together considered to have formed over one year. This interpretation is similar to that of Growth Layer Groups (GLG) in the whale literature (Aguilar and Lockyer, 1987).
Prior to sample extraction for radiocarbon analysis, earplugs were cut along the longitudinal midline with a scalpel. Using both the intact and sectioned images for guidance, a sample of each presumed annual growth increment along the growth sequence was isolated with a scalpel under a stereo microscope at 6.3 – 40X magnification, taking into account the cone-shaped, three-dimensional structure of the growth increments. Each growth increment sample weighed at least 15 mg and samples were weighed to the nearest 0.1 mg. All samples were reweighed after three days of drying at room temperature, which resulted in a mean weight loss of 56%. The year of formation for each sample was calculated as the year of whale collection minus the number of growth increments between the distal (most recently-formed) growth increment and that of the extracted growth increment. Samples were then sent to Beta Analytic for 14C assay where they were first acid leached to remove surface carbonates, then combusted to produce CO2 for 14C assay with accelerator mass spectrometry (AMS). AMS assays also provided δ13C (0/00) values, which were used to correct for isotopic fractionation effects and provide information on the source of the carbon. Radiocarbon values were subsequently reported as both percent modern carbon and Δ14C, which is the per mil (0/00) deviation of the sample from the radiocarbon concentration of 19th-century wood, corrected for sample decay prior to 1950. The mean standard deviation of the individual radiocarbon assays was about 20/00.
To assign dates of formation to the growth increments visible in the earplugs, we compared the earplug Δ14C data to Δ14C carbonate reference chronologies based on known-age material from the northwest Atlantic (NWA; Campana et al., 2008), shallow waters of the Barents Sea (Kalish et al., 2001), and that of a deepwater fish species (Reinhardtius hippoglossoides) from western Greenland (Campana et al., 2008). The Δ14C chronology of carbonates derives from dissolved inorganic carbon (DIC), so it is a good proxy for the dietary source of the Δ14C incorporated into the earplugs.
An earplug from a presumed 82 yr old fin whale is shown in Figure 1, which demonstrates the periodic laminae that we assumed to represent annual growth increments. Based on our experience with other marine vertebrates, these bands were relatively straightforward to identify and count throughout much of the growth sequence, particularly near the medial (first-formed) end. Increments became much narrower near the distal end, implying that an earplug that was broken or of poor visibility in that region could have substantial effects on the presumed age. Breakage at both ends of the right earplug of whale F15016 almost certainly explains its low age compared to its left counterpart, but the lower age in the right earplug of F13037 was probably due more to interpretation difficulties in the central part of the growth sequence (Table 1). The coefficient of variation (CV) between left and right earplug increment counts for whales F13037 and F13026 was 5%, which is the median ageing precision reported for many species (Campana, 2001). The mean CV for the three assayed earplugs between the two age readers was 4.4%.
Figure 1 Image of an earplug from a fin whale aged as 82 years old based on presumed annual growth bands (marked with yellow dots), photographed under reflected light, and slightly modified to enhance contrast. The medial (first-formed) end is to the left. Scale bar is 0.32 mm.
All three earplug radiocarbon chronologies showed classic bomb radiocarbon curves, increasing from Δ14C pre-bomb values of about -75 (pMC of 0.93) in the early 1950s, and peaking at Δ14C values of about 25 (pMC of 1.025) between 1980 and 1990 (Figure 2). The distribution of the earplug radiocarbon results was consistent with all of the known-age reference chronologies, indicating that the age determinations based on increment counts were reasonably accurate. Consistent age underestimation or overestimation would have been clearly evident as a shift of the entire fitted chronology to the left or the right of the reference chronology, and was most precisely assessed in a comparison of the initial year of increase between reference and whale radiocarbon chronologies. The initial year of radiocarbon increase above pre-bomb levels (calculated as per Campana et al., 2008) ranged between 1957 and 1959 for the three reference chronologies, while it ranged between 1954 and 1963 for the three whales: 1957 for F13026, 1963 for F13037, and 1954 for F15016. Assuming an initial period of increase beginning in 1958, 82-yr old whale F13026 was aged within one year of the correct age, 80-yr old whale F13037 was underaged by 5 yr, and 65-yr old whale F15016 was overaged by 4 yr. In the latter two cases, the “correct” ages would have been bracketed by the left and right earplug increment counts (Table 1). The relatively low asymptotic Δ14C values in the earplugs were most in keeping with the deepwater reference chronology, which began to increase in 1959. Although it was unclear which reference chronology was most applicable to Icelandic fin whales, the choice of reference chronology made little difference to the net result, since all reference chronologies shared similar years of increase. The similarity between whale and reference chronologies indicated that the earplug increment counts of all whales provided ages accurate to within 6% of the true age of the whale, with most of the error apparently associated with damage or loss at the distal end of the earplug. This level of accuracy and precision is very much in keeping with other bomb dating studies on beluga whale teeth (Stewart et al., 2006) and teleosts (Andrews et al., 2016). A key advantage of bomb dating over most age validation methods is that it validates the entire age of the animal and not just the periodicity of growth band formation over a short period of time. Although Boye et al. (2020) successfully differentiated pre-bomb from post-bomb ages using radiocarbon in the eye lens of humpback whales, the use of the eye lens core meant that only a single radiocarbon value was available for each whale. A unique aspect of our study is that each whale provided a complete radiocarbon chronology, in keeping with that normally associated with corals, thus allowing the absolute age of each individual whale to be determined with considerable accuracy.
Figure 2 (Top) Bomb radiocarbon chronology (percent modern carbon) in three fin whale earplugs (coloured symbols) show similar trajectories, but with offsets of up to 10 yr likely due to errors in the assignment of growth bands to year of formation. (Bottom) Earplug chronologies (Δ14C) relative to known-age carbonate reference chronologies of Atlantic cod otoliths in the northeast Atlantic (dotted line), otoliths and bivalves in the northwest Atlantic (dashed line), and deepwater turbot otoliths off western Greenland (solid line).
The mean value of δ13C in the earplugs was -22.0 (SD=0.9), which indicates a metabolic source for the earplug carbon that is similar to that reported for earplugs in other species of baleen whales (Mansouri et al., 2021), but one that is much more depleted in δ13C than the -14.3 value typical of beluga whale teeth in the Canadian Arctic (Stewart et al., 2006), and even more depleted than the δ13C value of -3.1 characteristic of both the DIC and Reindardtius otoliths (Campana et al., 2008). A significant declining trend in δ13C with year of increment formation (b = -0.017 ± 0.004. P < 0.001) is consistent with that of the Suess effect (Figure 3), whereby the burning of δ13C-depleted fossil fuels depleted atmospheric and marine δ13C concentrations over time (Gruber et al., 1999). Our demonstration that the earplug growth increments accurately reflect whale age provides even more rigor to studies that have used stable isotope profiles of the earplug to reconstruct changes in the behavioral ecology of individual whales over their lifetime (Mansouri et al., 2021).
Figure 3 Earplug chronologies (δ13C) in three fin whale earplugs (coloured symbols) all show a significant declining trend (fitted line) symptomatic of the Suess effect.
The longevities of 80-85 yr reported here are the oldest confirmed longevities for any species of baleen whale. Gabriele et al. (2010) reported a humpback whale re-sighting 27 yr after marking, while Hamilton et al. (1998) used photo-identification methods to identify a right whale 60 yr after initial sighting. George et al. (2021) inferred that bowhead whales could live over 100 yr based on recovery of ancient hunting tools embedded in the whale, but the actual year of harpoon use could not be confirmed. All other longevity reports were based on correlative methods or unvalidated earplug lamina counts [summarized in Hamilton et al. (1998) and George et al. (2021)]. However, our results suggest that reports of earplug-based fin whale longevities of 84 yr (Aguilar and Lockyer, 1987) and 114 yr (Ohsumi, 1979) may be reasonable.
Age validation methods such as bomb radiocarbon dating and recapture/resighting rely on animals of known age to test or confirm the accuracy of an alternative ageing method or protocol (Campana, 2001). Once validated, the alternative method is considered to be accurate, and can be used to provide routine ages in a comparable species and system with no further validation. However, the distinction between accuracy and precision is important; an ageing method can be accurate (unbiased) but imprecise, rendering the mean age of a large sample size absolutely correct, but individual age estimates unreliable. Previous studies on baleen whale ages have demonstrated that annual cycles in baleen stable carbon isotopes and ovarian corpora counts can give both accurate and precise age estimates, but only over certain parts of the life history (Lubetkin et al., 2012; George et al., 2021). Aspartic acid racemization of the eye lens can be used over the entire lifespan, but the standard error can exceed 20% for individuals (Rosa et al., 2013). The most promising of the alternative methods is the epigenetic DNA methylation clock, which is accurate at the across-species level (Lu et al., 2023) but biased at young and old ages within taxa, with mean ageing errors of over 20% for porpoises over 25 yr old (Peters et al., 2023). While improvements in the epigenetic approach appear likely, earplug laminae are currently the most precise and accurate of the ageing methods currently available for fin whales.
Ages from calcified otoliths underpin most fish ages around the world (Campana and Thorrold, 2001). Fin whale earplugs are not calcified, and thus do not have the same characteristics as the otolith; stable oxygen isotopes and trace elements in the calcified otolith allow reconstruction of temperature and location history. However, the ability to prepare complete earplug radiocarbon chronologies at the individual level was only possible in this study due to the large size of the earplug relative to otoliths or bivalves, thus allowing extraction of sufficient material from each annual growth increment. Given the presence of classic bomb curves and a Suess effect in each whale, the earplug laminae must have been metabolically stable and thus able to form and retain isotopic gradients over the lifetime of the animal. The metabolic stability and accurate age assignment of the earplug indicates that recent applications using isotopic analyses of the earplug growth increment sequence to reconstruct the feeding history of individual whales appears to be firmly based (Mansouri et al., 2021). Indeed, the non-calcified nature of the earplug wax allows time series reconstructions of hormonal and contaminant exposures that are simply not possible with the otolith (Trumble et al., 2013).
The original contributions presented in the study are included in the article/Supplementary Material. Further inquiries can be directed to the corresponding author.
The animal study was approved by Marine and Freshwater Research Institute, Iceland. The study was conducted in accordance with the local legislation and institutional requirements.
SC: Conceptualization, Formal analysis, Funding acquisition, Methodology, Project administration, Supervision, Writing – original draft, Writing – review & editing. SF: Data curation, Investigation, Methodology, Validation, Writing – review & editing. GS: Data curation, Investigation, Methodology, Project administration, Resources, Validation, Writing – review & editing.
The author(s) declare financial support was received for the research, authorship, and/or publication of this article. This work was supported by Icelandic Research Fund (RANNIS) Grant 173906-051.
We thank Valerie Chosson and Sverrir Daníel Halldórsson for sampling the whales in the field. Gísli Víkingsson was instrumental in organizing the sampling of these whales and getting this project off the ground. Gísli passed away in the summer of 2022. Beta Analytic carried out the radiocarbon assays; we thank Ron Hatfield for assisting with the assay protocol. Stephen Trumble and two reviewers provided helpful comments on the MS.
The authors declare that the research was conducted in the absence of any commercial or financial relationships that could be construed as a potential conflict of interest.
All claims expressed in this article are solely those of the authors and do not necessarily represent those of their affiliated organizations, or those of the publisher, the editors and the reviewers. Any product that may be evaluated in this article, or claim that may be made by its manufacturer, is not guaranteed or endorsed by the publisher.
The Supplementary Material for this article can be found online at: https://www.frontiersin.org/articles/10.3389/fmars.2024.1327752/full#supplementary-material
Aguilar A., Lockyer C. H. (1987). Growth, physical maturity, and mortality of fin whales (Balaenoptera physalus) inhabiting the temperate waters of the northeast Atlantic. Can. J. Zool. 65, 253–264. doi: 10.1139/z87-040
Albert C., Luque G. M., Courchamp F. (2018). The twenty most charismatic species. PloS One 13 (7), e0199149. doi: 10.1371/journal.pone.0199149
Andrews A. H., Siciliano D., Potts D. C., DeMartini E. E., Covarrubias S. (2016). Bomb radiocarbon and the Hawaiian archipelago: coral, otoliths and seawater. Radiocarbon 2016, 1–18. doi: 10.1017/RDC.2016.32
Boye T. K., Garde E., Nielsen J., Hedeholm R., Olsen J., Simon M. (2020). Estimating the age of West Greenland humpback whales through aspartic acid racemization and eye lens bomb radiocarbon methods. Front. Mar. Sci. 6, 811. doi: 10.3389/fmars.2019.00811
Braaten P. J., Campana S. E., Fuller D. B., Lott R. D., Bruch R. M., Jordan G. R. (2015). Age estimations of wild pallid sturgeon (Scaphirhynchus albus, Forbes & Richardson 1905) based on pectoral fin spines, otoliths and bomb radiocarbon: inferences on recruitment in the dam-fragmented Missouri River. J. Appl. Ichthyology 31 (5), 821–829. doi: 10.1111/jai.12873
Campana S. E. (2001). Accuracy, precision and quality control in age determination, including a review of the use and abuse of age validation methods. J. Fish Biol. 59, 197–242. doi: 10.1111/j.1095-8649.2001.tb00127.x
Campana S. E., Casselman J. M., Jones C. M. (2008). Bomb radiocarbon chronologies in the Arctic, with implications for the age validation of lake trout (Salvelinus namaycush) and other Arctic species. Can. J. Fish. Aquat. Sci. 65, 733–743. doi: 10.1139/f08-012
Campana S. E., Thorrold S. R. (2001). Otoliths, increments and elements: keys to a comprehensive understanding of fish populations? Can. J. Fish. Aquat. Sci. 58, 30–38. doi: 10.1139/f00-177
Chittleborough R. G. (1960). Marked humpback whale of known age. Nature 187, 164. doi: 10.1038/187164a0
Christensen J. (1981). Age determination of minke whales, Balaenoptera acutorostrata, from laminated structures in the tympanic bullae. Int. Whaling Commun. Rep. Commun. 31, 245–253.
Frie A. K., Hammill M. O., Hauksson E., Lind Y., Lockyer C., Stenman O., et al. (2012). Error patterns in age estimation and tooth readability assignment of grey seals (Halichoerus grypus): results from a transatlantic, image-based, blind-reading study using known-age animals. ICES J. Mar. Sci. 70, 418–430. doi: 10.1093/icesjms/fss169
Frýdlová P., Mrzílková J., Šeremeta M., Křemen J., Dudák J., Žemlička J., et al. (2020). Determinate growth is predominant and likely ancestral in squamate reptiles. Proc. R. Soc. B 287 (1941), 2020–2737. doi: 10.1098/rspb.2020.2737
Gabriele C. M., Lockyer C., Straley J. M., Jurasz C. M., Kato H. (2010). Sighting history of a naturally marked humpback whale (Megaptera novaeangliae) suggests ear plug growth layer groups are deposited annually. Mar. Mamm. Sci. 26, 443–450. doi: 10.1111/j.1748-7692.2009.00341.x
George J. C., Lubetkin S. C., Zeh J. E., Thewissen J. G. M., Wetzel D., Givens G. H. (2021). “Age estimation,” in The bowhead whale (NY: Academic Press), 309–322.
Gruber N., Keeling C. D., Bacastow R. B., Guenther P. R., Lueker T. J., Wahlen M., et al. (1999). Spatiotemporal patterns of carbon-13 in the global surface oceans and the oceanic Suess effect. Glob. Biogeochem. Cyc. 13, 307–335. doi: 10.1029/1999GB900019
Hamilton P. K., Knowlton A. R., Marx M. K., Kraus S. D. (1998). Age structure and longevity in North Atlantic right whales Eubalaena glacialis and their relation to reproduction. Mar. Ecol. Prog. Ser. 171, 285–292. doi: 10.3354/meps171285
Herman D. P., Ylitalo G. M., Robbins J., Straley J. M., Gabriele C. M., Clapham P. J., et al. (2009). Age determination of humpback whales Megaptera novaeangliae through blubber fatty acid compositions of biopsy samples. Mar. Ecol. Prog. Ser. 392, 277–293. doi: 10.3354/meps08249
Hunt K. E., Buck C. L., Ferguson S. H., Fernández Ajo A., Heide-Jørgensen M. P., Matthews C. J. (2022). Male Bowhead Whale reproductive histories inferred from baleen testosterone and stable isotopes. Integr. Organismal Biol. 4 (1), obac014. doi: 10.1093/iob/obac014
Kalish J. M., Nydal R., Nedreaas K. H., Burr G. S., Eine G. L. (2001). A time history of pre- and post-bomb radiocarbon in the Barents Sea derived from Arcto-Norwegian cod otoliths. Radiocarbon 43, 843–855. doi: 10.1017/S0033822200041515
Klevezal G. A. (2017). Recording structures of mammals. 1st ed (Routledge: London). doi: 10.1201/9780203741146
Laws R. M., Purves P. E. (1956). The ear plug of the Mysticeti as an indication of age with special reference to the North Atlantic fin whale (Balaenoptera physalus). Norsk Hvalfangst-Tidende 45, 413–425.
Lu A. T., Fei Z., Haghani A., Robeck T. R., Zoller J. A., Li C. Z., et al. (2023). Universal DNA methylation age across mammalian tissues. Nat. Aging 3 (9), 1144–1166. doi: 10.1038/s43587-023-00462-6
Lubetkin S. C., Zeh J. E., George J. C. (2012). Statistical modeling of baleen and body length at age in bowhead whales (Balaena mysticetus). Can. J. Zoology 90 (8), 915–931. doi: 10.1139/z2012-057
Mansouri F., Winfield Z. C., Crain D. D., Morris B., Charapata P., Sabin R., et al. (2021). Evidence of multi-decadal behavior and ecosystem-level changes revealed by reconstructed lifetime stable isotope profiles of baleen whale earplugs. Sci. Total Environ. 757, 143985. doi: 10.1016/j.scitotenv.2020.143985
Nielsen N. H., Garde E., Heide-Jorgensen M. P., Lockyer C. H., Ditlevsen S., Olafsdottir D., et al. (2013). Application of a novel method for age estimation of a baleen whale and a porpoise. Mar. Mammal Sci. 29, E1–E23. doi: 10.1111/j.1748-7692.2012.00588.x
Ohsumi S. (1979). Interspecies relationships among some biological parameters in cetaceans and estimation of the natural mortality coefficient of the Southern Hemisphere minke whales. Rep. Int. Whaling Comm. 20, 397–406.
Olsen E., Sunde J. (2002). Age determination of minke whales (Balaenoptera acutorostrata) using the aspartic acid racemization technique. Sarsia 87, 1–8. doi: 10.1080/003648202753631686
Ong J. J., Meekan M. G., Hsu H. H., Fanning L. P., Campana S. E. (2020). Annual bands in vertebrae validated by bomb radiocarbon assays provide estimates of age and growth of whale sharks. Front. Mar. Sci. 7, 188. doi: 10.3389/fmars.2020.00188
Peters K. J., Gerber L., Scheu L., Cicciarella R., Zoller J. A., Fei Z., et al. (2023). An epigenetic DNA methylation clock for age estimates in Indo-Pacific bottlenose dolphins (Tursiops aduncus). Evolutionary Appl. 16 (1), 126–133. doi: 10.1111/eva.13516
Rehorek S. J., Stimmelmayr R., George J. C., Suydam R., McBurney D. M., Thewissen J. G. M. (2019). Structure of the external auditory meatus of the bowhead whale (Balaena mysticetus) and its relation to their seasonal migration. J. Anat. 234 (2), 201–215. doi: 10.1111/joa.12908
Rosa C., Zeh J., Craig George J., Botta O., Zauscher M., Bada J., et al. (2013). Age estimates based on aspartic acid racemization for bowhead whales (Balaena mysticetus) harvested in 1998–2000 and the relationship between racemization rate and body temperature. Mar. Mammal Sci. 29 (3), 424–445. doi: 10.1111/j.1748-7692.2012.00593.x
Schweder T. (2000). Distortion of uncertainty in science: Antarctic fin whales in the 1950s. J. Int. Wildlife Law Policy 3 (1), 73–92. doi: 10.1080/13880290009353947
Stewart R. E. A., Campana S. E., Jones C. M., Stewart B. E. (2006). Bomb radiocarbon dating calibrates beluga (Delphinapterus leucas) age estimates. Can. J. Zool. 84, 1840–1852. doi: 10.1139/z06-182
Trumble S. J., Robinson E. M., Berman-Kowalewski M., Potter C. W., Usenko S. (2013). Blue whale earplug reveals lifetime contaminant exposure and hormone profiles. Proc. Natl. Acad. Sci. 110 (42), 16922–16926. doi: 10.1073/pnas.1311418110
Keywords: age validation, earplug, bomb radiocarbon, longevity, growth increments
Citation: Campana SE, Finnsdóttir SV and Sigurðsson GM (2024) Bomb radiocarbon determines absolute age of adult fin whales, and validates use of earplug growth bands for age determination. Front. Mar. Sci. 11:1327752. doi: 10.3389/fmars.2024.1327752
Received: 25 October 2023; Accepted: 04 January 2024;
Published: 23 January 2024.
Edited by:
Stephen J. Trumble, Baylor University, United StatesReviewed by:
Javier Almunia, Loro Parque Foundation, SpainCopyright © 2024 Campana, Finnsdóttir and Sigurðsson. This is an open-access article distributed under the terms of the Creative Commons Attribution License (CC BY). The use, distribution or reproduction in other forums is permitted, provided the original author(s) and the copyright owner(s) are credited and that the original publication in this journal is cited, in accordance with accepted academic practice. No use, distribution or reproduction is permitted which does not comply with these terms.
*Correspondence: Steven E. Campana, c3RldmVuLmUuY2FtcGFuYUBnbWFpbC5jb20=
Disclaimer: All claims expressed in this article are solely those of the authors and do not necessarily represent those of their affiliated organizations, or those of the publisher, the editors and the reviewers. Any product that may be evaluated in this article or claim that may be made by its manufacturer is not guaranteed or endorsed by the publisher.
Research integrity at Frontiers
Learn more about the work of our research integrity team to safeguard the quality of each article we publish.